- School of Veterinary Science, Massey University, Palmerston North, New Zealand
The Sixth Assessment of the Intergovernmental Panel on Climate Change describes negative effects of climate change on animals occurring on a larger scale than previously appreciated. Animal species are increasingly experiencing more frequent and extreme weather in comparison with conditions in which the species evolved. Individual variation in behavioural and physiological responses of animals to stimuli from the environment is ubiquitous across all species. Populations with relatively high levels of individual variation are more likely to be able to survive in a range of environmental conditions and cope with climate change than populations with low levels of variation. Behavioural and physiological responses are linked in animals, and personality can be defined as consistent individual behavioural and physiological responses of animals to changes in their immediate environment. Glucocorticoids (cortisol and corticosterone) are hormones that, in addition to metabolic roles, are released when the neuroendocrine stress system is activated in response to stimuli from the environment perceived to be threatening. The size of a glucocorticoid response of an animal is an indication of the animal’s personality. Animals with reactive personalities have relatively high glucocorticoid responses, are relatively slow and thorough to explore new situations, and are more flexible and able to cope with changing or unpredictable conditions than animals with proactive personalities. Animals with reactive personalities are likely to be better able to cope with environmental changes due to climate change than animals with proactive personalities. A reaction norm shows the relationship between phenotype and environmental conditions, with the slope of a reaction norm for an individual animal a measure of phenotypic plasticity. If reaction norm slopes are not parallel, there is individual variation in plasticity. Populations with relatively high individual variation in plasticity of reaction norms will have more animals that can adjust to a new situation than populations with little variation in plasticity, so are more likely to persist as environments change due to climate change. Future studies of individual variation in plasticity of responses to changing environments will help understanding of how populations of animals may be able to cope with climate change.
Introduction
Environmental conditions are changing throughout the world due to climate change and other anthropogenic causes such as destruction of animal habitats. Changes in weather patterns associated with climate change include increased temperatures, both increases and decreases in rainfall, and increased frequency of storms. Individual animals may be able to cope with climate change by making behavioural changes and physiological adjustments that enable them to stay in their current range, or they may be able to move and survive in new areas. Animals with these characteristics may be favoured by natural selection. The ability of populations of animals to cope with climate change thus depends on individual variation in responses of animals to environmental stimuli, and on the extent to which individual animals are plastic and able to change their responses.
The central role of individual variation in the success of populations of animals in coping with climate change is explored in this review. An overview of the effects of climate change on animal populations is followed by a consideration of individual variation in responses of animals to changes in their environment, and of individual variation in plasticity in responses. The importance of individual characteristics known as personality in determining behavioural and physiological responses of animals and fitness is considered, including relationships between glucocorticoid responses, personality, and fitness. Animals with reactive personality styles are likely to be better at coping with climate change than proactive animals. A reaction norm approach enables identification of individual variation in plasticity of responses of animals to changing environments, and the last section of the review considers the use of reaction norms to help understand how populations of animals may cope with climate change.
Climate change
Climate change
It is important to define climate and to distinguish between climate and weather when considering climate change. Weather refers to atmospheric conditions such as wind, temperature, cloudiness, rainfall, humidity, and air pressure at a given time, while climate refers to average weather conditions over long periods of time (National Oceanic and Atmospheric Administration [NOAA], 2021). A standard period for averaging these variables is 30 years (World Meteorological Organization1).
Carbon dioxide, methane and nitrous oxide are together known as greenhouse gases. Greenhouse gas emissions due to human activities have increased since the pre-industrial period in the 1700s, leading to atmospheric concentrations of greenhouse gases that are unprecedented in at least the last 800 000 years. The increased concentrations of greenhouse gases, especially carbon dioxide, are causing changes in the Earth’s energy budget that in turn are leading to warming of the global climate (Intergovernmental Panel on Climate Change [IPCC], 2014). Changes in the global climate system include warming of the atmosphere with increased land and ocean temperatures, reductions in the Greenland and Arctic ice sheets, shrinkage of glaciers in the Northern and Southern Hemispheres, and sea level rise (Intergovernmental Panel on Climate Change [IPCC], 2014). These changes are commonly called climate change. Climate change, caused by anthropogenic increases in atmospheric greenhouse gases, is a combination of long-term changes in climate and short-term changes in the frequency and intensity of weather events that can have high impacts on local areas and on animals (see Figure 1). The Framework Convention on Climate Change (UNFCCC) defines climate change as: “a change of climate which is attributed directly or indirectly to human activity that alters the composition of the global atmosphere and which is in addition to natural climate variability observed over comparable time periods” (United Nations, 1992). Another term for climate change, human-induced rapid environmental change (HIREC), refers to environmental changes caused by human activities that are occurring more rapidly and at larger scales than environmental changes that organisms have likely experienced in their evolutionary past (Sih et al., 2010).
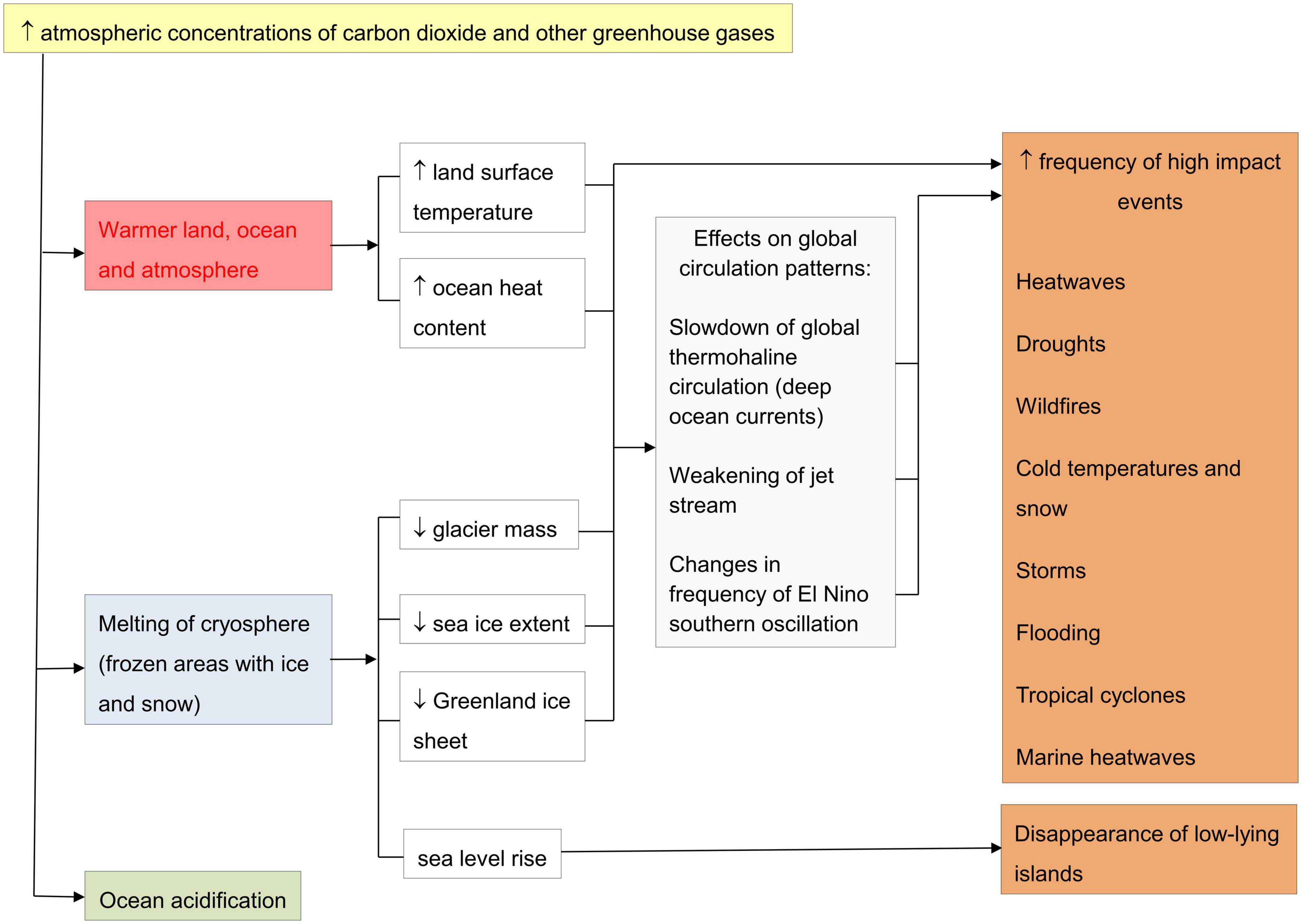
Figure 1. Effects of increasing atmospheric concentrations of carbon dioxide and other greenhouse gases on climate and weather [based on (World Meteorological Organization, 2021)].
The Intergovernmental Panel on Climate Change (IPCC) was established in 1988 and is the international body for the assessment of science related to climate change. While the IPCC includes changes due to natural processes in its definition of climate change, in practice IPCC considerations are focused on changes due to anthropogenic greenhouse gas emissions. The IPCC is now (2022) in its Sixth Assessment cycle (Intergovernmental Panel on Climate Change [IPCC], 2021b). The report from Working Group I (the Physical Science Basis) was published in August 2021 (Intergovernmental Panel on Climate Change [IPCC], 2021a) and the report from Working Group II (Impacts, Adaptation and Vulnerability) was published in February 2022 (Intergovernmental Panel on Climate Change [IPCC], 2021b). A third working group report (Mitigation of Climate Change), and the AR6 Synthesis Report, will be published later in 2022.
The Sixth Assessment explores the likely consequences for the world climate of five scenarios for emissions of greenhouse gases. Global surface temperature will continue to increase until at least mid-century under all scenarios. Global warming will reach 1.5°C by the early 2030s and will reach 2°C by mid-century unless there are deep reductions in carbon dioxide and other greenhouse gas emissions (Intergovernmental Panel on Climate Change [IPCC], 2021a). While it remains possible for emissions to be reduced to keep warming below 1.5°C, measures taken by governments to reduce national emissions have thus far not been sufficient to achieve this goal.
The Sixth Assessment states that climate change has altered marine, terrestrial and freshwater ecosystems all around the world, and that the effects of climate change have been experienced earlier, are more widespread, have been of greater magnitude, and are having more far-reaching consequences than previously anticipated (Intergovernmental Panel on Climate Change [IPCC], 2022b). It is predicted that magnitude of extreme conditions will become greater and previously rare weather conditions will become more common in coming decades (Intergovernmental Panel on Climate Change [IPCC], 2021a). Hot conditions on land have become more frequent and intense since the 1950s, while cold extremes have become less frequent. Terrestrial heatwaves that exceed the physiological thresholds of some species are regularly occurring, and marine heatwaves with generally negative consequences for animals (Cheung and Frölicher, 2020) are becoming more frequent (Intergovernmental Panel on Climate Change [IPCC], 2022a). Heavy precipitation (rainfall and snowfall) and storm events are more common and intense, and sea levels are rising (Intergovernmental Panel on Climate Change [IPCC], 2021a).
Effects of climate change on animals
Long term studies of responses of free-living animals to changes in climate variables generally identify the responses as being due to climate change. For example, Charmantier et al. (2008) reported an advance of 14 days over 47 years in the timing of egg laying in a great tit (Parus major) population in the United Kingdom. The advance in laying date was attributed to increased spring temperatures due to climate change leading to earlier emergence of caterpillars which are the primary food for nestlings. Similarly, advances of more than three days per decade in the timing of egg laying in two blue tit (Cyanistes caeruleus) populations in evergreen forest on Corsica in the Mediterranean corresponded with changes in temperature attributed to climate change (Bonamour et al., 2019).
The IPCC Sixth Assessment reported that, for terrestrial, freshwater, and marine animals and plants reported to be affected by climate change, half to two-thirds of the species have shifted their ranges to higher latitudes, and approximately two-thirds of species had advanced their timing of spring activities (Intergovernmental Panel on Climate Change [IPCC], 2022b). Responses of animals, including changes in physiology, geographic range and shifting seasonal timing are often not sufficient to cope with recent climate change. Foden et al. (2013) considered that 11 to 15% of amphibian species and 6 to 9% of bird species are both highly climate change vulnerable and already threatened with extinction (listed on the IUCN Red List). The Sixth Assessment considered that a median of 9% and a maximum of 14% of assessed terrestrial species were likely to face a very high risk of extinction (equivalent to the IUCN critically endangered status) at global warming of 1.5°C (Intergovernmental Panel on Climate Change [IPCC], 2022b). This level of warming will be reached in the 2030s if emissions of greenhouse gases continue at their current rate. Even if emissions are markedly reduced in coming years, there will be ongoing local population extinctions and extinctions of species due to climate change.
Local extinctions due to climate change were detected in 47% of plant and animal species examined, with local extinctions more prevalent in tropical than temperate habitats (Wiens, 2016; Intergovernmental Panel on Climate Change [IPCC], 2022a). A survey of plant distribution in Arizona mountains found local extinctions of 15 species of plants, including Quercus gambelii (Gambel oak), Muhlenbergia porteri (bush muhly) and Urochloa arizonica (AZ panic grass), in comparison with 50 years earlier (Brusca et al., 2013). In the alpine Himalayas of Sikkim 75 species of plants, including Rhododendron nivale (dwarf snow rhododendron), Potentilla fruticosa (shrubby cinquefoil) and Lepidium capitatum (Himalayan peppergrass), were locally extinct in comparison with 1850 (Telwala et al., 2013). Species extinctions have been attributed to climate change for the golden toad (Incilius periglenes) that lived in cloud forest in Costa Rica and for the Bramble Cays Melomys (Melomys rubicola), an Australian rodent. It seems likely that other species living in remote areas will also have become extinct due to climate change.
It is also valuable to consider responses of animals to variables associated with climate change, with studies of these responses providing information that will help with prediction of effects of climate change on animals. Table 1 gives examples of responses of animals to changes in climate and weather variables. A review of studies of behavioural responses of invertebrates and vertebrates to climate variables found that changes in phenology were the most commonly reported responses (Beever et al., 2017). Changes in the timing of reproductive behaviour were the most common timing changes, followed by changes in timing of movements (dispersal or migration). Changes in the timing of feeding, foraging, and habitat use were also reported, along with changes in thermoregulation behaviour activities. For the studies that were considered, temperature was identified in 67% of the studies as the climate variable associated with changes in behaviour. Some changes in animal behaviour were attributed to indirect effects of climate variables such as changes in food resources or habitat structure.
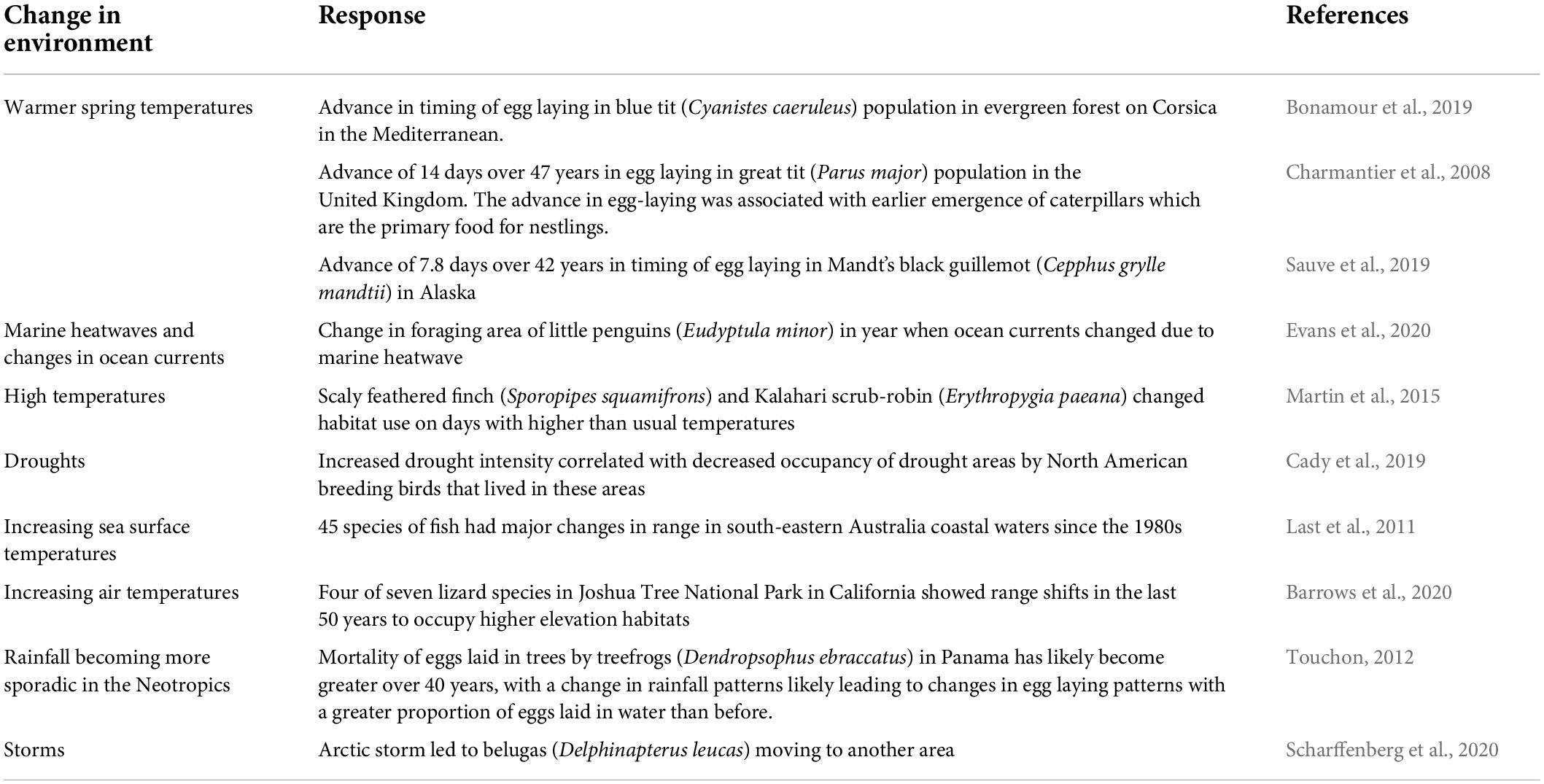
Table 1. Examples of responses of animals to changes in the environment associated with climate and weather variables.
Animal populations can persist in changing environments by individual animals changing their behaviour or their physiology, for example, by starting to feed at a different time of day or by increasing their evaporative heat loss in hot conditions, by moving to a different area, or by adapting through a change in the genetic composition of the population (Fuller et al., 2010). The ability of a population to cope with changing environments and persist in the face of climate change thus depends on the extent to which individual animals can adjust to the changes or move to a new area, and the extent to which the population can adapt to the changes through natural selection of characteristics that enable individual animals to cope with a changed environment.
Individual variation
Individual variation in behavioural and physiological traits
Animals have morphological, physiological, and behavioural characteristics known as traits, for example, plumage colour in a bird, level of activation of the hypothalamo-pituitary-adrenal axis and glucocorticoid secretion in response to a threat, or timing of departure in a migratory species. For every trait, there are differences between individual animals in the level or nature of the trait. Individual differences arise from a combination of experiences of the animal and additive genetic variation (Dochtermann et al., 2015). Examples of genetic variation contributing to individual differences in glucocorticoid responses are the development of lines of rainbow trout (Overli et al., 2002) and Japanese quail (Odeh et al., 2003) selected for low or high glucocorticoid responses. Individual differences resulting from experiences of an animal include maternal and paternal effects, epigenetic effects, and environmental effects that can have long-term consequences (Dochtermann et al., 2015), such as prolonged periods of food shortage.
Some examples of individual variation in behavioural and physiological traits are given in Table 2. Recognition of individual variation, and of its significance, led Charles Darwin to formulate the concept of natural selection in which some animals have characteristics or traits that enable them to be more likely to survive, successfully reproduce and pass on heritable traits to their offspring, leading over time to these traits becoming more frequent in the population (Darwin, 1859). Natural selection, the process in which heritable differences between organisms in survival and reproduction lead to increases in the proportion of beneficial traits within a population from one generation to the next, is a fundamental concept for biology (Gregory, 2009). In other words, natural selection is a process in which some organisms have higher fitness than others (produce more offspring than others) and hence the genotypes of these organisms increase in frequency in a population. Natural selection can only occur when there is individual variation in a population, hence individual variation is central to natural selection. Although individual variation is a key concept for biology, the importance of considering the range of individual characteristics in populations, rather than just mean values, has only become apparent relatively recently (Bolnick et al., 2003, 2011; Cockrem, 2013b; Mimura et al., 2017).
When a population has a large amount of individual variation in a trait, then there will be animals that are suited to a wider range of situations than in a population where there is a small amount of variation in the trait. Populations with relatively high levels of individual variation are thus more likely to be able to survive in a range of environmental conditions than populations with low levels of individual variation. For example, if animals in one population all forage in the same area each day whereas animals in another population forage in several areas, then there is greater individual variation in foraging location in the second population. The second population will thus be less vulnerable than the first population to a shortage of food in one area. Maldonado-Chaparro et al. (2017) found that benefits of plasticity in body mass growth rate outweighed costs of plasticity in yellow-bellied marmots (Marmota flaviventris) in Colorado. Their modelling approach showed that individual variation in compensatory growth following harsh conditions decreased the probability of population extinction in unfavourable climate situations.
There can be disadvantages associated with individual variation, and Sih et al. (2004) noted that, in a simple view, natural selection should reduce variation and all individuals should be optimal in all environments. However, there can be multiple optimal behaviours for an environmental situation. An optimal behaviour for an animal in a situation involves a trade-off of costs and benefits. Individuals can differ in their trade-offs and hence exhibit different behaviours within the same environment, and there can be a range of behavioural types with similar fitness in a population (Sih et al., 2004). For example, specialists and generalists can coexist in a population (Moran, 1992). An extensive search of the literature for vertebrate and invertebrate species by Forsman and Wennersten (2016) found that greater genotypic and phenotypic individual variation in populations was indeed associated with lower vulnerability to environmental changes, smaller changes in population size, greater success and establishment in new areas, larger geographic ranges, and smaller risks of extinction. Experimental studies considered by Forsman and Wennersten (2016) generally showed that individual variation was more important under challenging conditions than benign conditions.
In addition to differences between animals in the level of a trait, there can also be differences between animals in the extent to which a trait can change when environmental conditions change. Differences between animals in the flexibility (plasticity) of traits can have important implications for the likelihood of success in coping with changing environments (Cockrem, 2013a), so in addition to individual variation in traits it is important to consider individual variation in plasticity of traits.
Individual variation in phenotypic plasticity
When an animal can change its behaviour or physiology in response to changes in its environment, the animal is showing phenotypic plasticity. Phenotypic plasticity is the capacity of a genotype to have different phenotypes in different environmental conditions (see Figure 2A), so phenotypic plasticity in a trait is the capacity of the trait to alter as environmental conditions change (see Valladares et al., 2006; Nussey et al., 2007). There is individual variation in the extent to which animals are flexible and plastic and can adjust to changing or unpredictable conditions (see Table 3). Results from a long-term study of a Dutch population of great tits by Nussey et al. (2005) are a good example of individual variation in plasticity in a trait. Birds with high plasticity in the timing of reproduction were able to advance their laying dates in years with warm spring temperatures and to delay their laying dates in years with cool spring temperatures. These birds were better able to match their reproductive timing with the peak in the availability of caterpillars to feed their offspring than birds with low plasticity in the timing of reproduction. Plasticity of laying dates was heritable, and there was a positive relationship between plasticity and fitness. Natural selection could therefore lead to changes in the composition of the population, in terms of laying date, with the proportion of birds with higher plasticity of laying date increasing and the population laying date being able to advance. The long-term success of the population will depend on the extent to which changes in the mean laying date are able to keep up with changes in caterpillar food availability that are occurring in response to warming spring temperatures due to climate change.
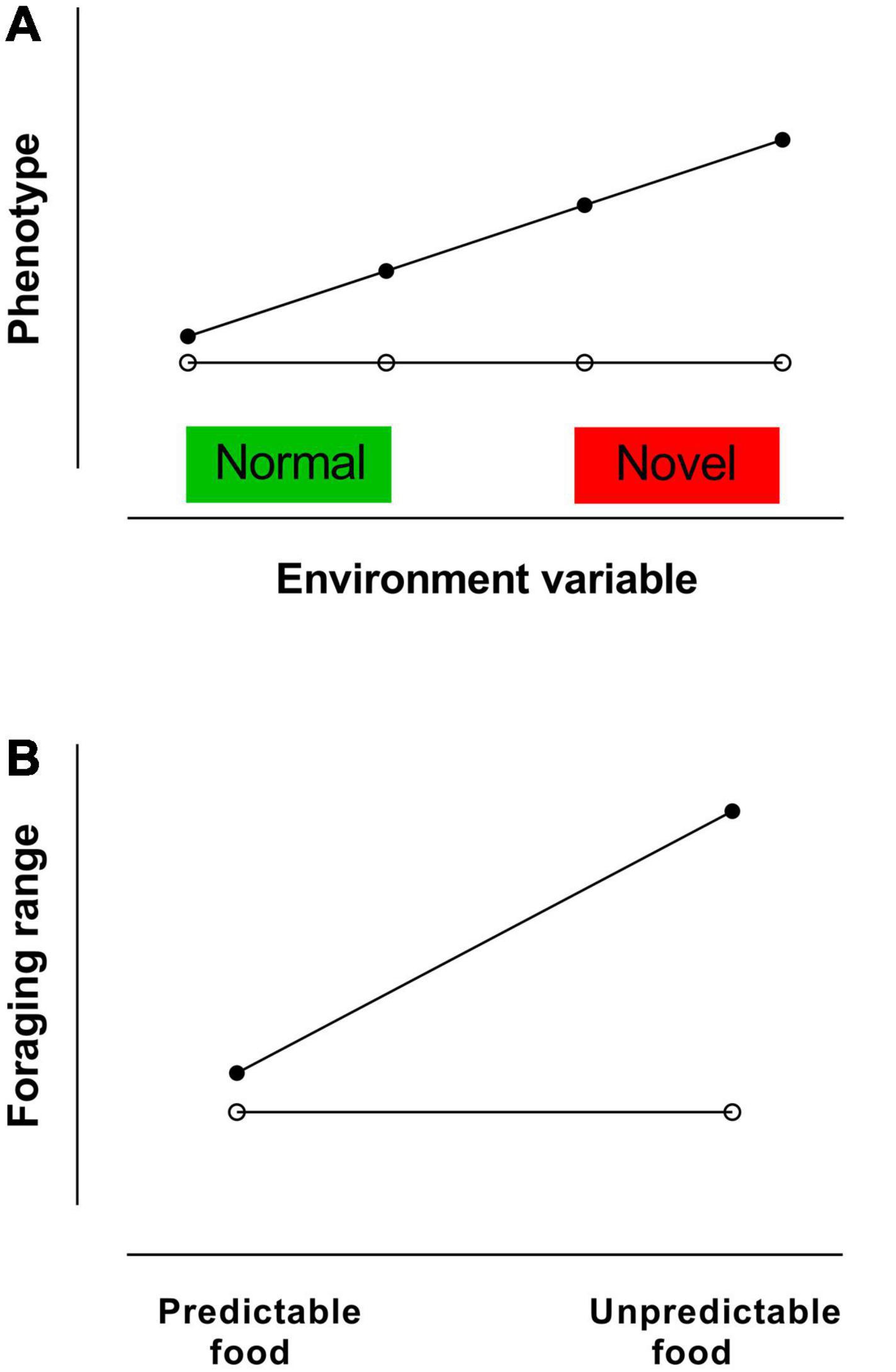
Figure 2. (A) Relationship between phenotype and level of an environmental variable for an animal with plasticity in responses to the variable in comparison with an animal that does not have phenotypic plasticity. (B) Example of relationships between foraging range and predictability of food supply for an animal with a reactive personality and an animal with a proactive personality. Reactive animals are more plastic than proactive animals and are more likely to respond to a change from a predictable to an unpredictable food situation by increasing their foraging range or changing their foraging locations.
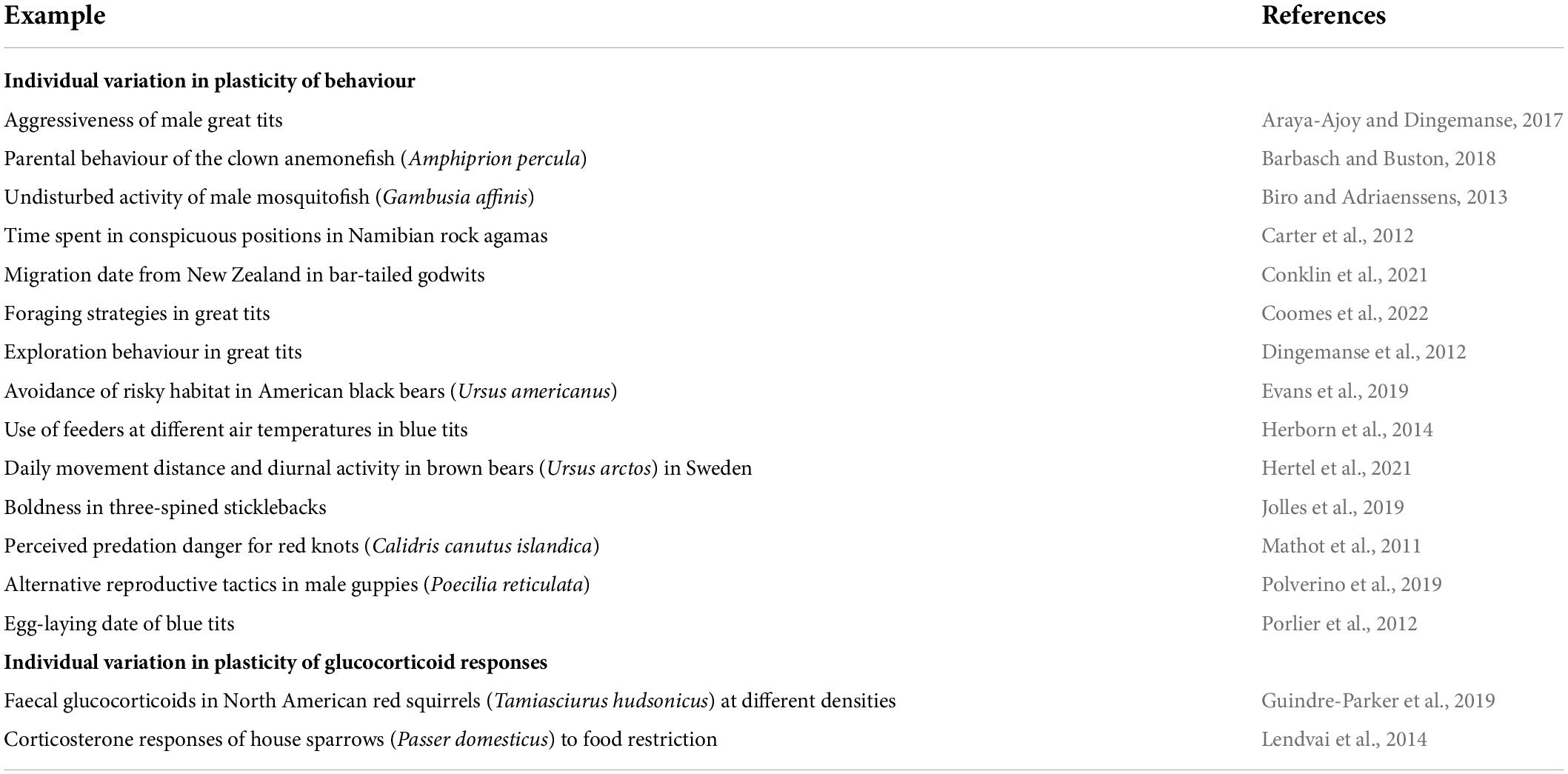
Table 3. Examples of individual variation in plasticity of behavioural and physiological responses of animals to changes in their environment.
Populations that have relatively high individual variation in phenotypic plasticity are more likely to have animals that can adjust to changing environments than populations with low individual variation in plasticity. Variation within a population in levels of phenotypic plasticity is thus related to the ability of populations to cope with changing environments associated with climate change, leading to the question of whether plasticity in relevant traits will be sufficient for a species to keep up with environmental changes (Wong and Candolin, 2014). Studies of individual variation in plasticity of behavioural and physiological responses of animals to changes in their environment (see Table 3 for examples) are thus important for understanding responses of populations of animals to climate change.
Personality
Personality and responses to changes in the immediate environment
Patterns of daily behaviour and of responses to environmental changes are characteristics of individual animals that are remarkably consistent. Levels of flexibility in responses to stimuli from the environment, in other words, the amount of phenotypic plasticity in traits, are also characteristics of each animal. Terms for the individual differences in behaviour that are consistently expressed in different situations include behavioural syndrome (Dingemanse and Wright, 2020), temperament (Reale et al., 2007), and personality (Carere and Eens, 2005; Dingemanse et al., 2010; Stamps and Groothuis, 2010). Coping style, a related term defined as a coherent set of behavioural and physiological stress responses which is consistent over time (Koolhaas et al., 1999), refers to the capacity of individual animals to cope with environmental challenges.
Relationships between behavioural and physiological traits in animals have been explored in numerous studies. Reviews of such studies, for example McMahon et al. (2022), found reports of negative associations, positive associations, or no association between behavioural and physiological traits. This is not surprising, as the studies have been conducted in a wide range of captive and experimental situations, with varying methodologies, sample sizes, and numbers of behavioural variables measured. The studies have generally considered only a single physiological variable, and there is a need for studies incorporating several physiological processes at once to better characterise physiological profiles in relation to behavioural profiles (McMahon and Cavigelli, 2021; McMahon et al., 2022). Nonetheless, when results from many studies are considered together, regular patterns emerge for physiological profiles in relation to behavioural characteristics and it becomes apparent that behavioural and physiological responses are linked in animals. Personality can be defined as consistent individual behavioural and physiological responses of animals to changes in their immediate environment (Cockrem, 2007, 2013a,b). Coevolution of linked behavioural and physiological traits leads to consistency of these traits (Wolf and McNamara, 2012), and to the individual differences in suites of correlated behaviours expressed in different situations which are features of personalities (Carere and Eens, 2005). Personality is heritable, with approximately 52% of animal personality variation identified in a review of published studies attributable to additive genetic variation (Dochtermann et al., 2015).
While personality characteristics vary across a spectrum, animals can be classified into two broad groups. Animals that are highly responsive are said to have a reactive personality, and animals that have relatively smaller responses are said to have proactive personalities (Koolhaas et al., 1999; Sih et al., 2004; Cockrem, 2007). Proactive and reactive personality styles are equivalent to proactive and reactive coping styles. Personality styles can be apparent in animals in a wide range of situations. For example, proactive common brush tail possums (Trichosurus vulpecula) in Australia had a more diverse and higher quality diet than reactive possums (Herath et al., 2021), and reactive but not proactive Atlantic cod (Gadus morhua) reduced their home ranges as sea temperatures increased in a Norwegian fjord (Villegas-Ríos et al., 2018). Proposed characteristics of animals with proactive and reactive personalities are shown in Table 4. Animals with proactive personalities can be considered to have active responses to immediate threats (“fight or flight” responses), to be relatively bold and aggressive, to be fast and superficial in their exploration of new situations, to be relatively rigid and routine-like in behaviour, and to have relatively low glucocorticoid responses to threatening stimuli [see Cockrem (2007) and Koolhaas et al. (2010)]. These animals have relatively low sensitivity to their immediate environment. Animals with reactive personalities can be considered to have passive responses to immediate threats (freeze-hide behaviour), have higher glucocorticoid responses to threats than proactive animals, and to be relatively slow and thorough to explore new situations [see Cockrem (2007) and Koolhaas et al. (2010)]. Reactive animals have relatively high sensitivity to their immediate environment and are more plastic (flexible) and more able to cope with changing circumstances than animals with proactive personalities (Geffroy et al., 2020). Differences between animals in cognition (mechanisms for the acquisition and processing of information from the environment) may contribute to the differences between personality styles in the sensitivity of animals to their immediate environment (Sih and Del Giudice, 2012).
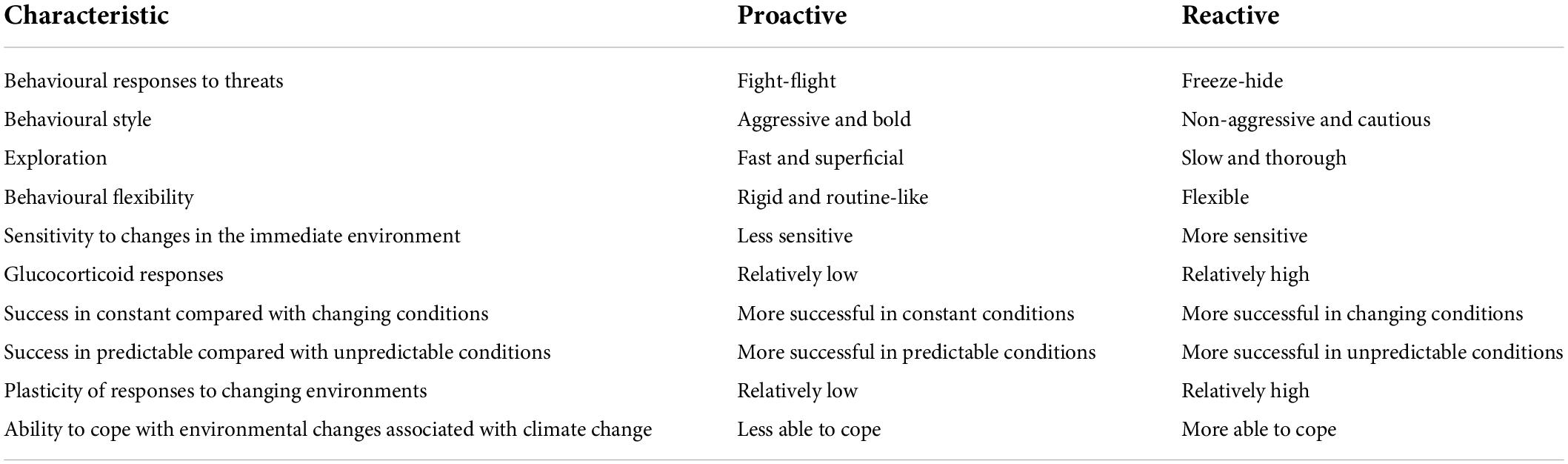
Table 4. Proposed characteristics of animals with proactive and reactive personalities [adapted from Cockrem (2007)].
Personality and coping with climate change
The environments in which animals have evolved are, at varying rates, changing due to climate change. Some populations of animals will be better able to cope with these environmental changes than other populations. There may be individuals in a population that can survive and breed in environmental conditions that differ from those experienced by the population in the past or can move to live in a new area. Alternatively, adaptation to novel environmental conditions can occur when individual animals have phenotypic plasticity in traits and can express phenotypes that enable the animals to survive. Natural selection for phenotypes that confer fitness in the changing situation (Fox et al., 2019) will lead to changes in the composition of the population. The survival of populations of animals will depend in part on the amount of individual variation in behavioural and physiological traits that contribute to fitness, together with the amount of individual variation in plasticity of these traits.
Plasticity can be adaptive (beneficial) or non-adaptive (Wilson, 1998). Adaptive plasticity can be acted upon by natural selection (Ghalambor et al., 2007), is favoured over fixed strategies when an environment is changing (Berrigan and Scheiner, 2004), and allows a genotype to have a broad tolerance to environmental conditions across multiple environments (Ghalambor et al., 2007). Plasticity that is non-adaptive and not subject to natural selection nonetheless enables animals to change in response to changing environmental conditions, and may “buy time” for a population to evolve and survive in environments that are changing due to climate change (Diamond and Martin, 2021). Plasticity may also have costs (Murren et al., 2015). Suggestions for potential costs of plasticity have included costs associated with cognition (Geffroy et al., 2020), energetic costs of the sensory and regulatory mechanisms of plasticity, and costs associated with the process of acquiring information about the environment which may be risky, involve energy for sampling, or reduce foraging or mating efficiency (DeWitt et al., 1998). Sih et al. (2004) noted that natural selection can favour the evolution of limited plasticity in situations where individuals have poor information about their environment, or if individuals avoid situations where limited plasticity would be a disadvantage. Conversely, Berrigan and Scheiner (2004) described how natural selection will favour plasticity over fixed strategies when the mean fitness of individuals with the plastic strategy exceeds that of individuals with the fixed strategy. Heterogeneity of the environment, in terms of changes in the environment with time or differences between areas in environmental conditions, was considered to be a necessary, but not sufficient, condition for plasticity to be favoured (Berrigan and Scheiner, 2004).
Traits that contribute to fitness are some of the traits that comprise the individual characteristics of animals known as personalities. It has been suggested that slow exploring reactive animals are less successful in stable environments than fast exploring proactive animals but are more successful in changeable environments (Dingemanse and Reale, 2005; Cockrem, 2007). For example, reactive animals more likely than proactive animals to respond to a change from a predictable to an unpredictable food situation by increasing their foraging range (see Figure 2B) or by changing their foraging locations. Animals with reactive personalities are likely to be better able to cope with environmental changes due to climate change than animals with proactive personalities, as suggested for birds by Cockrem (2013a). This suggestion is consistent with Sih (2013) who proposed that reactive, highly exploratory animals may be better able to cope with novel situations than proactive animals, and hence better able to cope with human-induced rapid environmental change (HIREC). In wild boars in Europe, slow explorers raised more offspring than fast explorers (Vetter et al., 2016), and fast exploring juvenile rabbits had lower survival than slow exploring rabbits (Rodel et al., 2015). Shy animals are more plastic than bold animals (Adriaenssens and Johnsson, 2011; Dammhahn and Almeling, 2012; Kareklas et al., 2016; Jolles et al., 2019). A study of free-living blue tits in Scotland showed that neophobic (reactive personality) birds changed their foraging behaviour at a feeder in response to changes in air temperature, whereas neophilic (proactive personality) birds did not change their foraging behaviour (Herborn et al., 2014). The reactive birds were more behaviourally flexible, had greater plasticity, and were better able to cope with a change in the environment than the proactive birds (Herborn et al., 2014).
If reactive animals that show plasticity in response to environmental change have higher fitness than other animals, then there is the potential for natural selection to lead to a change in the composition of the population so there is a greater proportion of animals with reactive personalities. Also, proactive animals may be more likely to move away from a habitat that changes than are reactive animals, so the remaining population would have a higher proportion of reactive animals than before the environmental change. The proportions of animals with different personalities may thus change over time in populations of animals that are experiencing environmental changes due to climate change, as suggested by Geffroy et al. (2020).
Glucocorticoids, personality, and fitness
Glucocorticoid hormones are secreted in reptiles, birds and mammals by the adrenal gland which is part of the hypothalamo-pituitary-adrenal (HPA) axis. In fish and amphibians, glucocorticoids are secreted by the interrenal gland in the hypothalamo-pituitary-interrenal (HPI) axis. Cortisol is the predominant glucocorticoid in fish and most mammals, except rodents, and corticosterone is the predominant glucocorticoid in amphibians, reptiles, and birds.
Glucocorticoids are metabolic hormones with a primary function to increase blood glucose concentrations. These hormones have a wide range of other actions, including actions on behaviour, the immune system, the cardiovascular system, and the reproductive system (Sapolsky et al., 2000). Glucocorticoids are involved in responses of animals to stimuli from the environment that are perceived to be threatening (Cockrem, 2013b). They are often called stress hormones, almost always in the absence of a definition of stress, even though their primary roles are not in stress responses (MacDougall-Shackleton et al., 2019). Glucocorticoids are secreted into the blood, and glucocorticoid concentrations are measured in plasma and serum. Concentrations of glucocorticoids and of glucocorticoid metabolites are also measured in an increasingly wide variety of other sample types including saliva, urine, faeces, feathers, hair, and blubber. It should be noted that, despite widespread assumptions in the literature, concentrations measured in samples other than plasma, serum or saliva are not directly related to blood concentrations (Romero and Beattie, 2022). Glucocorticoid concentrations in blood samples collected from animals shortly after they are captured or restrained are often called baseline glucocorticoids, while changes in glucocorticoid concentrations after capture, restraint, or exposure to a stimulus thought to be threatening for an animal, are called glucocorticoid responses. However, glucocorticoid concentrations in blood increase rapidly when an animal is captured, restrained, or confined, measured concentrations may not accurately reflect concentrations in undisturbed animals, and the term baseline can be misleading.
Glucocorticoids are often measured in animals in relation to questions about responses of animals to changes in their environment. Glucocorticoid responses to stimuli from the environment are generally called stress responses. Stimuli that activate glucocorticoid responses act via receptors which send signals to what can be called the neuroendocrine stress system (see Figure 3). It is proposed that responses of animals to threatening or potentially threatening changes in the external and internal environments arise from neural pathways and processes which can be called the neuroendocrine stress system, with stimuli that activate the system known as stressors. This proposed system consists of neural pathways in the brain that convey information from the external and internal environments to the hypothalamus, neural structures that process this information, the HPA or HPI axis, and components of the sympathetic nervous system. Visual, auditory, olfactory and touch stimuli from the external environment that activate receptors in the eyes, ears, nose and skin and lead to activation of the neuroendocrine stress system can be called emotional stressors (Cockrem, 2007). Activation of these receptors generates signals that are processed by the limbic forebrain. It is suggested that individual characteristics known as personality influence whether a stimulus is perceived to be a threat and hence whether changes in behaviour, activity of the HPA or HPI axis, the sympathetic nervous system and other physiological pathways are activated (Cockrem, 2007). Stimuli that activate the neuroendocrine stress system without processing of the signals by the limbic forebrain can be called physical stressors (Cockrem, 2007). These stimuli can come from the internal environment via activation of glucoreceptors, baroreceptors and osmoreceptors, from the external or internal environments via the activation of thermoreceptors or pain receptors, and from humoral signals of inflammation such as cytokines.
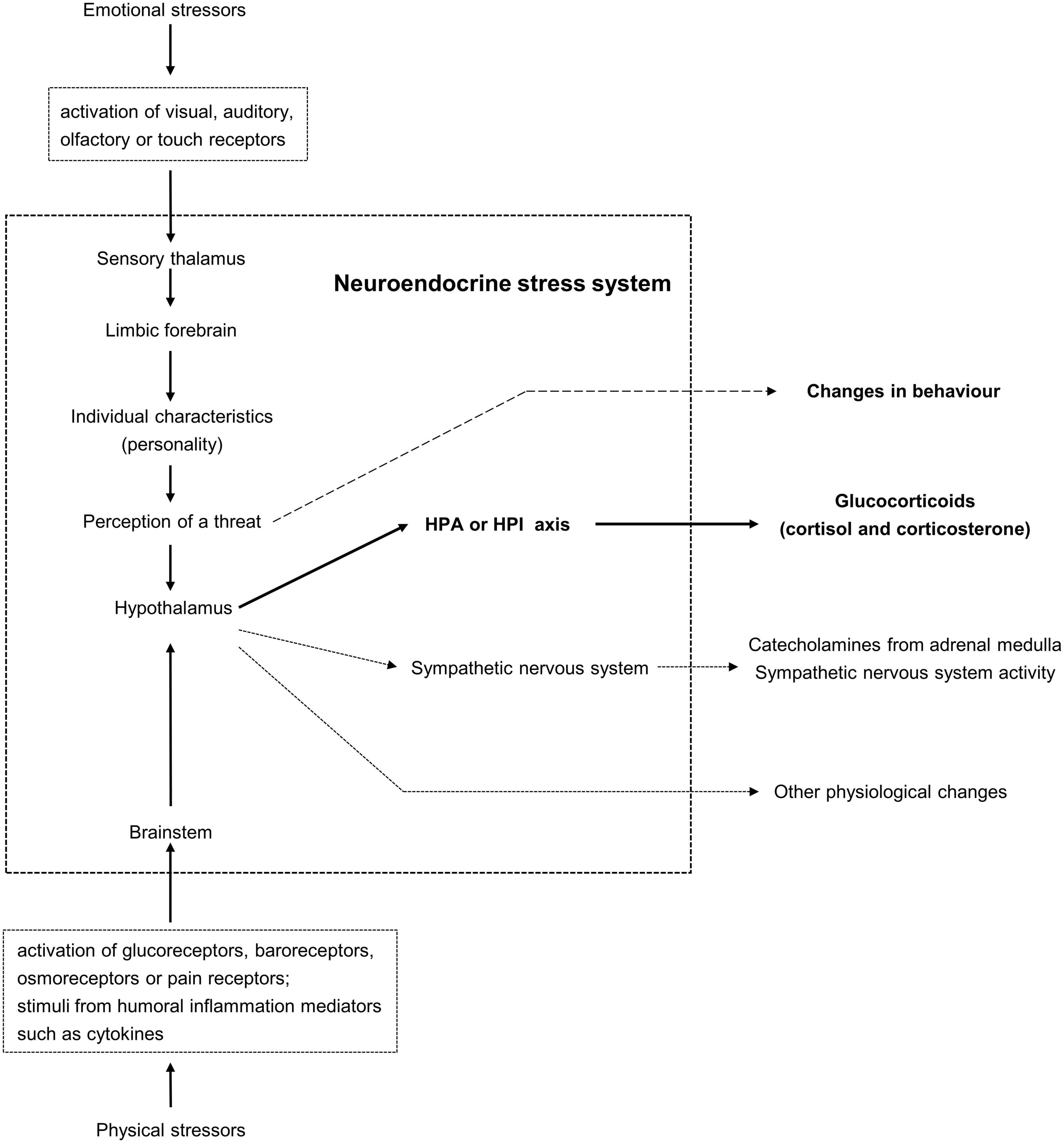
Figure 3. Schematic view of what is proposed to be called the neuroendocrine stress system. Responses of animals to threatening or potentially threatening changes in the external and internal environments arise from neural pathways and processes which can be called the neuroendocrine stress system, with stimuli that activate the system known as stressors.
A glucocorticoid response is a response to a stimulus from the immediate environment that is perceived as a threat, usually a visual stimulus. Glucocorticoid responses can be detected as increased concentrations of glucocorticoids in the blood within several minutes of a stimulus beginning (Romero and Reed, 2005). The duration of a response depends on the extent to which the stimulus is perceived to be a threat and on the duration of the stimulus. There is marked variation between individual animals in their glucocorticoid responses (Cockrem, 2013b). Some animals consistently have little or no response to a stimulus that initiates a large response in other animals, so a stimulus that is perceived as very threatening by one animal can be perceived quite differently by another animal. Higher glucocorticoid responses in some animals compared with others reflect an increased biological sensitivity to context in the animals with higher glucocorticoid responses (Boyce and Ellis, 2005). In other words, some animals are more aware of, and more sensitive to stimuli from their immediate environment than other animals. It has been suggested that the size of a glucocorticoid response of an animal is determined by individual characteristics of the animal which can be called personality (see Figure 4; Cockrem, 2007, 2013a).
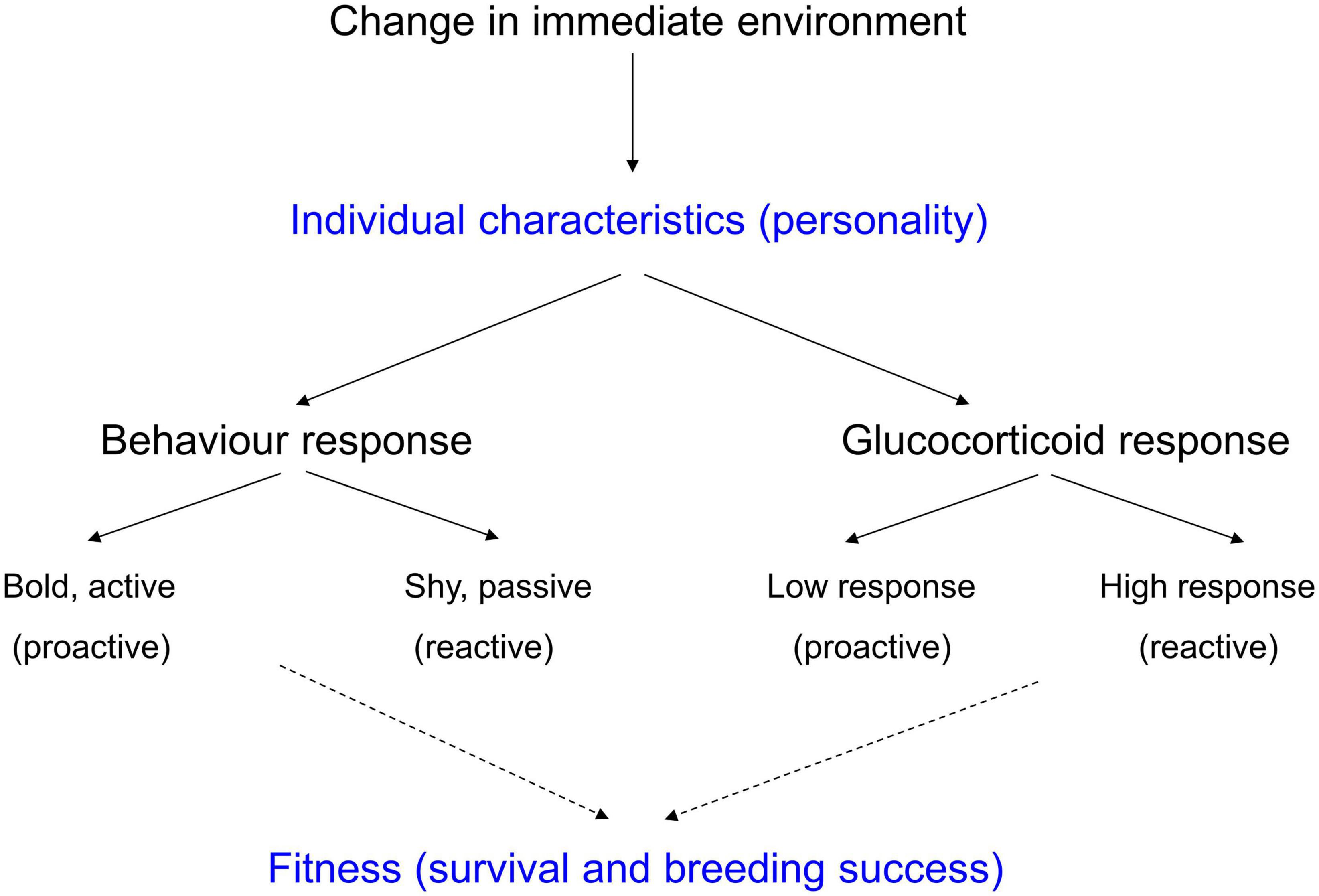
Figure 4. The influence of individual characteristics (personality) on behaviour responses, and glucocorticoid responses to changes in the immediate environment. These responses both influence fitness [based on Cockrem (2007, 2013a)].
It has been assumed that fitness in animals [the ability of animals to survive and breed (Orr, 2009)] is determined by baseline glucocorticoids or by glucocorticoid responses [see the Cort-Fitness Hypothesis; (Bonier et al., 2009)]. However, fitness in animals is not determined by glucocorticoid hormones alone, and differences between animals in fitness are not a direct consequence of differences between animals in baseline glucocorticoids or the size of the glucocorticoid responses. Relationships between glucocorticoids, personality and fitness are usually overlooked in the literature on the significance of glucocorticoid responses in relation to fitness in animals. Survival and breeding success depend on the behaviour and physiology of animals, not just on glucocorticoids, and is not surprising that there is no clear support for any of the three main hypotheses about glucocorticoids and fitness (Breuner and Berk, 2019; Romero and Gormally, 2019).
Reaction norms and responses of animals to changing environments
Reaction norms
The persistence of populations of animals in changing environments in coming decades will depend, in part, on the abilities of individual animals to show plasticity in behavioural and physiological characteristics that affect the likelihood of successful breeding and survival. Understanding of relationships between individual characteristics and environmental variables requires the description and quantification of the relationships. The reaction norm approach is widely used in studies of plants and animals for situations where a phenotype varies in relation to an environment variable. The term reaction norm was first used in its current sense by Schmalhausen et al. (1949), and began to be used regularly in discussions of phenotypic plasticity from the 1980s [e.g., Stearns (1989)]. A reaction norm can be defined as “the set of phenotypes that can be produced by an individual genotype that is exposed to different environmental conditions” (Schlichting and Pigliucci, 1998). Other definitions have included “the way that an individual’s phenotype varies across environments” (Ghalambor et al., 2007) and “a function describing the change in a genotype’s phenotype across an environmental gradient” (Nussey et al., 2007).
Reaction norms are commonly depicted on graphs with a trait on the y-axis and an environment variable on the x-axis. The environment variable may be continuous, for example ambient temperature, or discrete, for example two situations in which a trait was measured. The slope of a reaction norm for an individual animal is a measure of phenotypic plasticity. Figure 2A shows reaction norms for an animal with the same phenotype at different levels of an environment variable (slope equals zero, no plasticity in the phenotypic trait), and for an animal whose phenotype changed at different levels of the environment variable (positive slope, plasticity in the trait). Figure 2B shows reaction norms for foraging range in relation to levels of food availability.
Individual animals can have phenotypes that do not change in different environment conditions (parallel reaction norms with slopes that equal zero, no plasticity), phenotypes that change at the same rate (parallel reaction norms with same positive or negative slopes, same plasticity), or phenotypes that change at different rates (non-parallel reaction norms with different slopes, individual variation in plasticity). These three situations are shown in Figure 5.
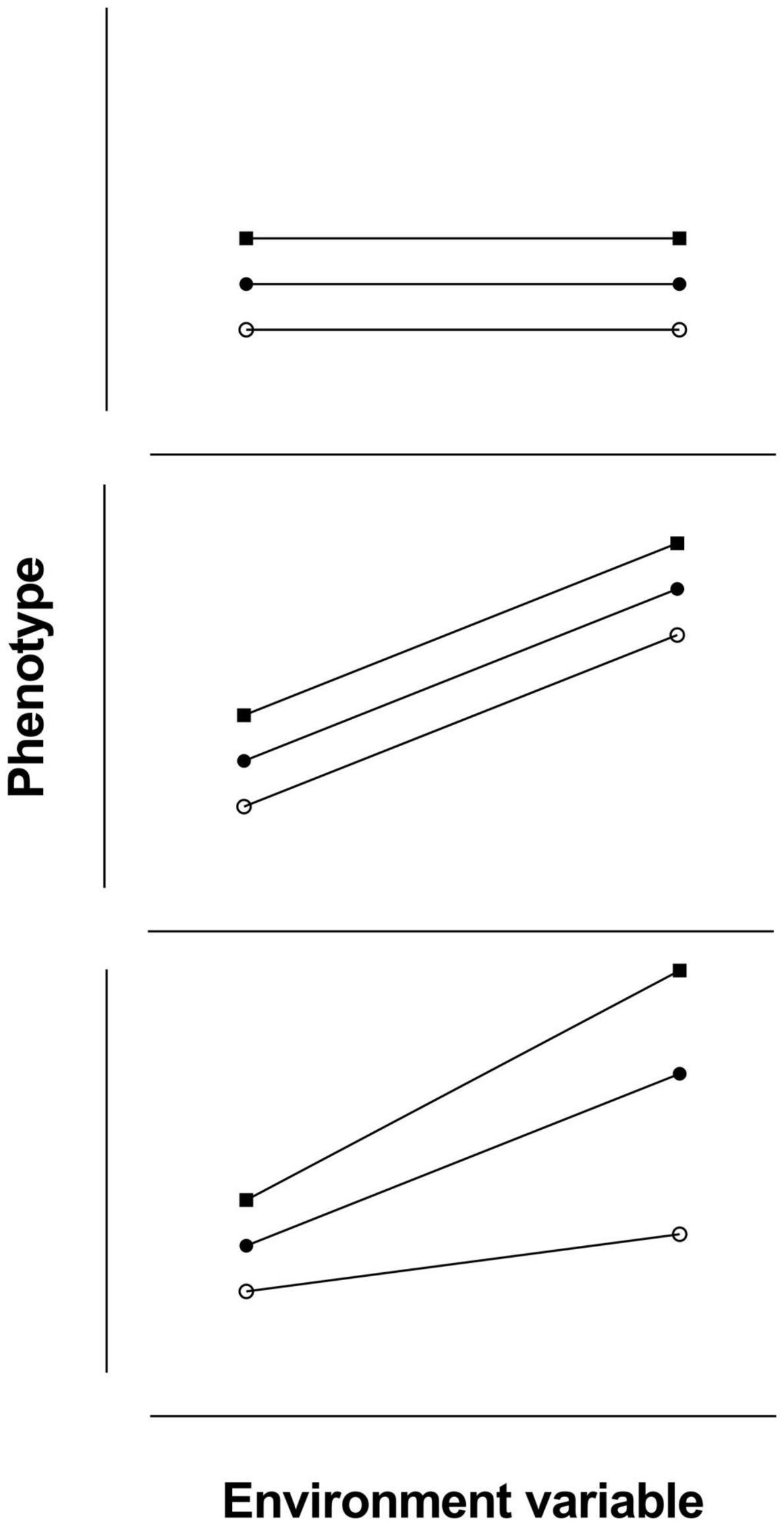
Figure 5. Reaction norms for individual animals with no phenotypic plasticity (reaction norms parallel with slopes equal to zero; upper panel), the same degree of phenotypic plasticity (reaction norms parallel with slopes not equal to zero; middle panel), and individual variation in plasticity (reaction norms not parallel with slopes not equal to zero; lower panel).
The slope of a reaction norm, the rate of change in a phenotype in relation to changes in an environmental variable, can be used to predict the phenotype of an animal outside the measured range of the environmental variable. However, in some animals the slope at levels of the environment variable outside the historical range may differ from the slope measured within the historical range (Figure 6). Reaction norm measurements are thus needed both in free-living animals exposed to natural environment conditions, and in experimental situations where levels of an environment variable can be lower or higher than the historical range of levels to which the species has been exposed naturally.
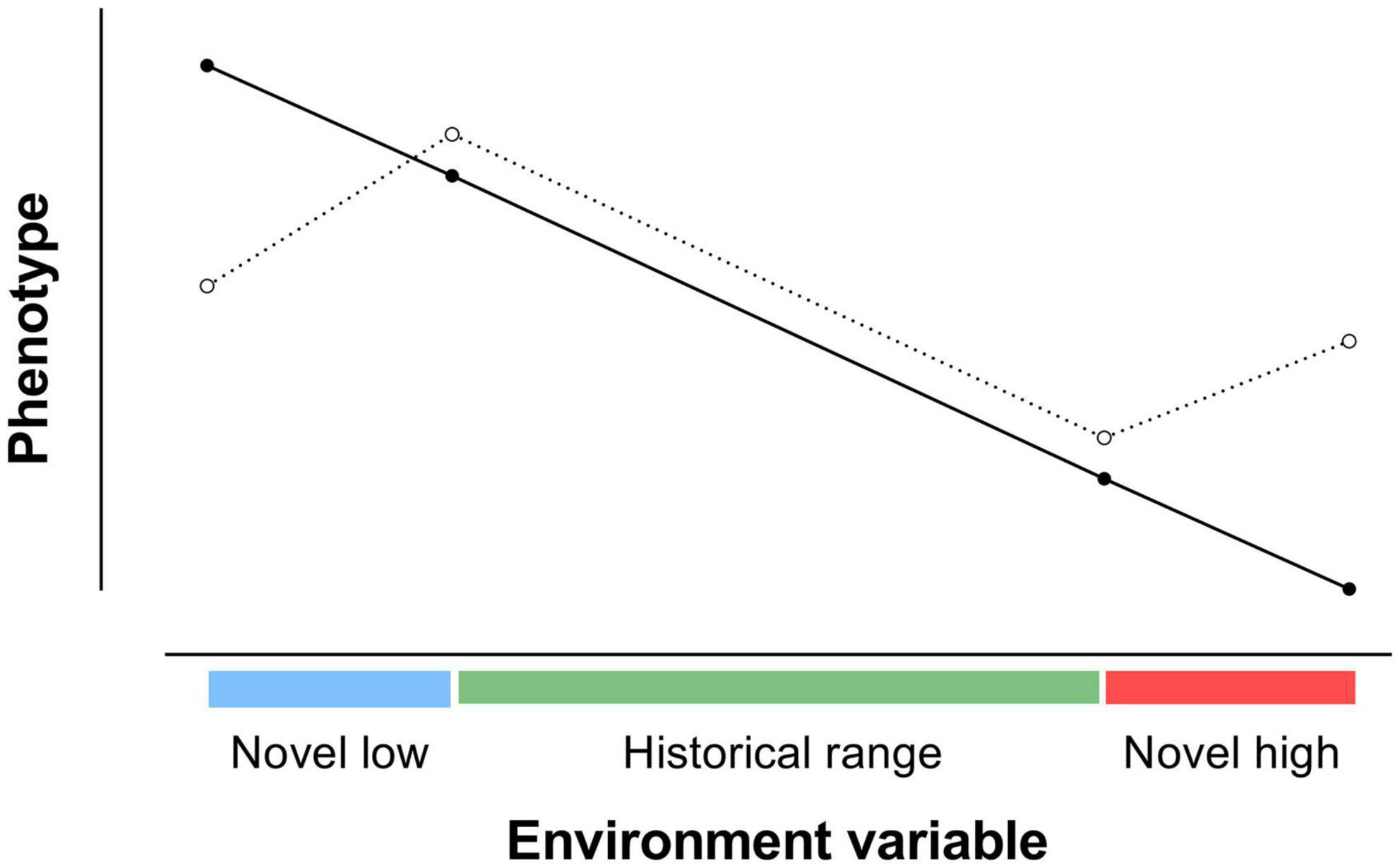
Figure 6. Reaction norms for an animal with the same slope of reaction norm in historical and novel environmental conditions and for an animal with different slopes in novel conditions in comparison with an historical range of conditions
. The reaction norm for the second animal in historical conditions did not predict the reaction norm in novel conditions [adapted from Ghalambor et al. (2007)].
The use of reaction norms for understanding how individual animals and populations may cope with climate change
Recognition of the importance of individual variation in plasticity of responses of animals to changing environments, and the use of reaction norms to identify this variation, is relatively recent. Dingemanse et al. (2010) described behavioural reaction norms for identification of individual plasticity in relation to animal personality. Cockrem (2013a) showed how reaction norms can be used to quantify plasticity and individual variation in plasticity of glucocorticoid responses and suggested that reaction norms for corticosterone responses in birds could be used to predict how birds could cope with environmental changes due to climate change. Brommer (2013) reviewed studies that showed individual variation in plasticity of traits in animals, Valladares et al. (2014) modelled reaction norms to show how individual variation in plasticity of response to temperature could be used to predict changes in species distribution, and Taff and Vitousek (2016) described how reaction norms could be used to study individual variation in endocrine flexibility. Some reaction norm studies that reported individual variation in plasticity for free living animals and for animals in experimental situations are listed in Table 5.
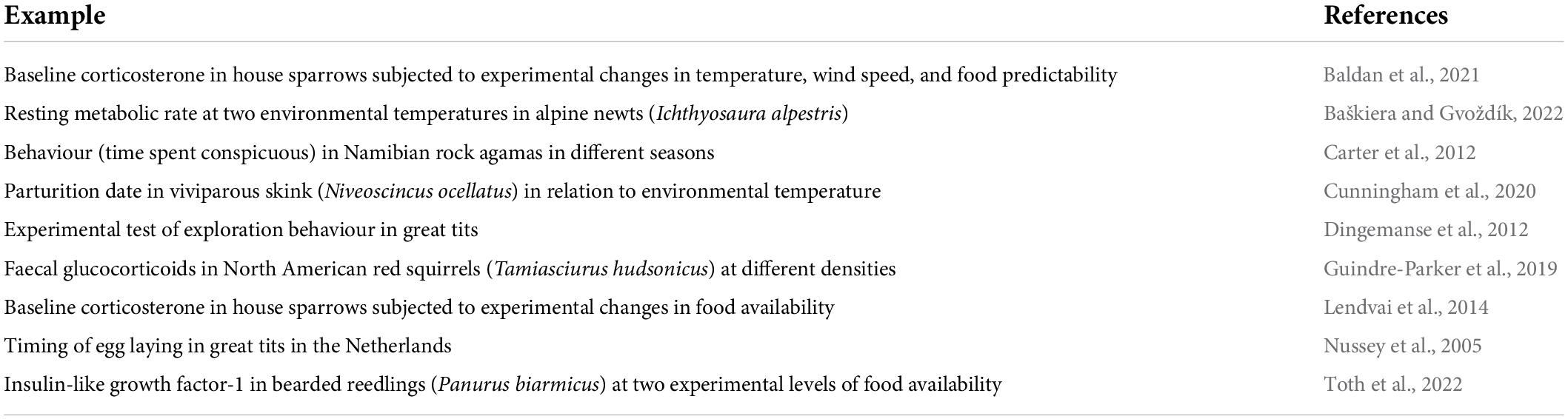
Table 5. Examples of reaction norm studies that showed individual variation in slopes (individual variation in plasticity).
The reaction norm approach enables identification of individual variation in plasticity of responses of animals to changing environments. Reaction norm graphs for individual animals, such as those in Figure 5, show the extent to which animals in a population have phenotypic plasticity for a trait in different environmental conditions. If the slopes of reaction norms are low, then the animals have low plasticity and the capabilities of animals in the population to change a trait in response to changing environmental conditions are low. Conversely, if reaction norm slopes are high, then animals have relatively high capabilities to change as environmental conditions change. Importantly, when the slopes of reaction norms for individual animals are not parallel, then the reaction norm graph shows individual variation in plasticity and hence the extent to which individual animals differ in their responses to changing conditions.
Phenotypic plasticity, the capacity of a trait to have different phenotypes in different environmental conditions, is important for individual animals and hence for populations to be able to cope with changes in their environments associated with climate change (Sih et al., 2011; Wong and Candolin, 2014; Fox et al., 2019). Individual variation in plasticity is also important (Kelly, 2019). Populations with relatively high individual variation in plasticity of reaction norms, will have more animals that can adjust to a new situation than populations with little variation in plasticity. The populations with high variation in plasticity are more likely to persist as environments change due to climate change than populations with low individual variation in plasticity. Studies that use reaction norms to identify phenotypic plasticity and individual variation in plasticity in responses of animals to changes in environment variables can inform conservation policy (Cooke et al., 2021), and will be valuable for understanding how populations of animals may cope with climate change.
Conclusion
The Sixth Assessment of the Intergovernmental Panel on Climate Change, published in 2021 and 2022, describes negative effects of climate change on animals occurring more rapidly and on a larger scale than previously appreciated. Animal species are increasingly experiencing adverse weather conditions that differ in frequency and intensity from the conditions in which the species evolved. Individual variation in behavioural and physiological responses of animals to stimuli from the environment is ubiquitous across all species. There is also individual variation in the extent to which animals are flexible and plastic and can adjust to changing or unpredictable conditions. Populations that have relatively high individual variation in plasticity are more likely to have some individuals that can cope with climate change than populations with low individual variation in plasticity. Reaction norms, which show phenotypic plasticity in traits, are useful for identifying this plasticity. Future studies of individual variation in plasticity of responses to changing environments will help understanding of how populations of animals may be able to cope with climate change.
Author contributions
The author confirms being the sole contributor of this work and has approved it for publication.
Funding
This research was supported by Massey University.
Conflict of interest
The author declares that the research was conducted in the absence of any commercial or financial relationships that could be construed as a potential conflict of interest.
Publisher’s note
All claims expressed in this article are solely those of the authors and do not necessarily represent those of their affiliated organizations, or those of the publisher, the editors and the reviewers. Any product that may be evaluated in this article, or claim that may be made by its manufacturer, is not guaranteed or endorsed by the publisher.
Footnotes
References
Adriaenssens, B., and Johnsson, J. I. (2011). Shy trout grow faster: Exploring links between personality and fitness-related traits in the wild. Behav. Ecol. 22, 135–143. doi: 10.1093/beheco/arq185
Aplin, L. M., Farine, D. R., Mann, R. P., and Sheldon, B. C. (2014). Individual-level personality influences social foraging and collective behaviour in wild birds. Proc. R. Soc. Lond. B Biol. Sci. 281:9. doi: 10.1098/rspb.2014.1016
Araya-Ajoy, Y. G., and Dingemanse, N. J. (2017). Repeatability, heritability, and age-dependence of seasonal plasticity in aggressiveness in a wild passerine bird. J. Anim. Ecol. 86, 227–238. doi: 10.1111/1365-2656.12621
Bajer, K., Horvath, G., Molnar, O., Torok, J., Garamszegi, L. Z., and Herczeg, G. (2015). European green lizard (Lacerta viridis) personalities: Linking behavioural types to ecologically relevant traits at different ontogenetic stages. Behav. Process. 111, 67–74. doi: 10.1016/j.beproc.2014.11.020
Baldan, D., Negash, M., and Ouyang, J. Q. (2021). Are individuals consistent? Endocrine reaction norms under different ecological challenges. J. Exp. Biol. 224:jeb240499. doi: 10.1242/jeb.240499
Barbasch, T. A., and Buston, P. M. (2018). Plasticity and personality of parental care in the clown anemonefish. Anim. Behav. 136, 65–73. doi: 10.1016/j.anbehav.2017.12.002
Barrows, C. W., Sweet, L. C., Rangitsch, J., Lalumiere, K., Green, T., Heacox, S., et al. (2020). Responding to increased aridity: Evidence for range shifts in lizards across a 50-year time span in Joshua Tree National Park. Biol. Conserv. 248:108667. doi: 10.1016/j.biocon.2020.108667
Baškiera, S., and Gvoždík, L. (2022). Individual variation in thermal reaction norms reveal metabolic-behavioral relationships in an ectotherm. Front. Ecol. Evol. 10:850941. doi: 10.3389/fevo.2022.850941
Beever, E. A., Hall, L. E., Varner, J., Loosen, A. E., Dunham, J. B., Gahl, M. K., et al. (2017). Behavioral flexibility as a mechanism for coping with climate change. Front. Ecol. Environ. 15:299–308. doi: 10.1002/fee.1502
Berrigan, D., and Scheiner, S. M. (2004). “Modeling the evolution of phenotypic plasticity,” in Phenotypic Plasticity : Functional and Conceptual Approaches, eds T. J. DeWitt and S. M. Scheiner (Oxford: Oxford University Press), 82–97.
Birnie-Gauvin, K., Koed, A., and Aarestrup, K. (2021). Repeatability of migratory behaviour suggests trade-off between size and survival in a wild iteroparous salmonid. Funct. Ecol. 35, 2717–2727. doi: 10.1111/1365-2435.13917
Biro, P. A., and Adriaenssens, B. (2013). Predictability as a personality trait: Consistent differences in intraindividual behavioral variation. Am. Nat. 182, 621–629. doi: 10.1086/673213
Bolnick, D. I., Amarasekare, P., Araujo, M. S., Burger, R., Levine, J. M., Novak, M., et al. (2011). Why intraspecific trait variation matters in community ecology. Trends Ecol. Evol. 26, 183–192. doi: 10.1016/j.tree.2011.01.009
Bolnick, D. I., Svanback, R., Fordyce, J. A., Yang, L. H., Davis, J. M., Hulsey, C. D., et al. (2003). The ecology of individuals: Incidence and implications of individual specialization. Am. Nat. 161, 1–28. doi: 10.1086/343878
Bonamour, S., Chevin, L. M., Charmantier, A., and Teplitsky, C. (2019). Phenotypic plasticity in response to climate change: The importance of cue variation. Philos. Trans. R. Soc. B Biol. Sci. 374:20180178. doi: 10.1098/rstb.2018.0178
Bonier, F., Moore, I. T., Martin, P. R., and Robertson, R. J. (2009). The relationship between fitness and baseline glucocorticoids in a passerine bird. Gen. Comp. Endocrinol. 163, 208–213. doi: 10.1016/j.ygcen.2008.12.013
Boyce, W. T., and Ellis, B. J. (2005). Biological sensitivity to context: I. An evolutionary–developmental theory of the origins and functions of stress reactivity. Develop. Psychopathol. 17, 271–301. doi: 10.1017/S0954579405050145
Breuner, C. W., and Berk, S. A. (2019). Using the van Noordwijk and de Jong resource framework to evaluate glucocorticoid-fitness hypotheses. Integr. Comp. Biol. 59, 243–250. doi: 10.1093/icb/icz088
Brommer, J. E. (2013). Phenotypic plasticity of labile traits in the wild. Curr. Zool. 59, 485–505. doi: 10.1093/czoolo/59.4.485
Brusca, R. C., Wiens, J. F., Meyer, W. M., Eble, J., Franklin, K., Overpeck, J. T., et al. (2013). Dramatic response to climate change in the Southwest: Robert Whittaker’s 1963 Arizona Mountain plant transect revisited. Ecol. Evol. 3, 3307–3319. doi: 10.1002/ece3.720
Cady, S. M., O’Connell, T. J., Loss, S. R., Jaffe, N. E., and Davis, C. A. (2019). Species-specific and temporal scale-dependent responses of birds to drought. Glob. Change Biol. 25, 2691–2702. doi: 10.1111/gcb.14668
Careau, V., Mariette, M. M., Crino, O., Buttemer, W. A., and Buchanan, K. L. (2020). Repeatability of behavior and physiology: No impact of reproductive investment. Gen. Comp. Endocrinol. 290:113403. doi: 10.1016/j.ygcen.2020.113403
Carere, C., and Eens, M. (2005). Unravelling animal personalities: How and why individuals consistently differ. Behaviour 142, 1149–1157. doi: 10.1163/156853905774539436
Carlson, B. S., Rotics, S., Nathan, R., Wikelski, M., and Jetz, W. (2021). Individual environmental niches in mobile organisms. Nat. Commun. 12:4572. doi: 10.1038/s41467-021-24826-x
Carter, A., Goldizen, A., and Heinsohn, R. (2012). Personality and plasticity: Temporal behavioural reaction norms in a lizard, the Namibian rock agama. Anim. Behav. 84, 471–477. doi: 10.1016/j.anbehav.2012.06.001
Charmantier, A., McCleery, R. H., Cole, L. R., Perrins, C., Kruuk, L. E. B., and Sheldon, B. C. (2008). Adaptive phenotypic plasticity in response to climate change in a wild bird population. Science 320, 800–803. doi: 10.1126/science.1157174
Cheung, W. W. L., and Frölicher, T. L. (2020). Marine heatwaves exacerbate climate change impacts for fisheries in the northeast Pacific. Sci. Rep. 10:6678. doi: 10.1038/s41598-020-63650-z
Choi, S., Grocutt, E., Erlandsson, R., and Angerbjorn, A. (2019). Parent personality is linked to juvenile mortality and stress behavior in the arctic fox (Vulpes lagopus). Behav. Ecol. Sociobiol. 73:162. doi: 10.1007/s00265-019-2772-y
Cockrem, J. F. (2007). Stress, corticosterone responses and avian personalities. J. Ornithol. 148, S169–S178. doi: 10.1007/s10336-007-0175-8
Cockrem, J. F. (2013a). Corticosterone responses and personality in birds: Individual variation and the ability to cope with environmental changes due to climate change. Gen. Comp. Endocrinol. 190, 153–163. doi: 10.1016/j.ygcen.2013.02.021
Cockrem, J. F. (2013b). Individual variation in glucocorticoid stress responses in animals. Gen. Comp. Endocrinol. 181, 45–58. doi: 10.1016/j.ygcen.2012.11.025
Cockrem, J. F., Agnew, P., Barrett, D. P., Candy, E. J., and Potter, M. A. (2017). Individual variation of corticosterone responses of little penguins (Eudyptula minor) sampled in two successive years at Oamaru, New Zealand. Gen. Comp. Endocrinol. 244, 86–92. doi: 10.1016/j.ygcen.2016.01.010
Cockrem, J. F., Bahry, M. A., and Chowdhury, V. S. (2019). Cortisol responses of goldfish (Carassius auratus) to increased water temperature, chasing and air exposure. Gen. Comp. Endocrinol. 270, 18–25. doi: 10.1016/j.ygcen.2018.09.017
Conklin, J. R., Battley, P. F., and Potter, M. A. (2013). Absolute consistency: Individual versus population variation in annual-cycle schedules of a long-distance migrant bird. PLoS One 8:9. doi: 10.1371/journal.pone.0054535
Conklin, J. R., Lisovski, S., and Battley, P. F. (2021). Advancement in long-distance bird migration through individual plasticity in departure. Nat. Commun. 12:9. doi: 10.1038/s41467-021-25022-7
Cooke, S. J., Bergman, J. N., Madliger, C. L., Cramp, R. L., Beardall, J., Burness, G., et al. (2021). One hundred research questions in conservation physiology for generating actionable evidence to inform conservation policy and practice. Conserv. Physiol. 9:coab009. doi: 10.1093/conphys/coab009
Coomes, J. R., Davidson, G. L., Reichert, M. S., Kulahci, I. G., Troisi, C. A., and Quinn, J. L. (2022). Inhibitory control, exploration behaviour and manipulated ecological context are associated with foraging flexibility in the great tit. J. Anim. Ecol. 91, 320–333. doi: 10.1111/1365-2656.13600
Cunningham, G. D., While, G. M., Olsson, M., Ljungstrom, G., and Wapstra, E. (2020). Degrees of change: Between and within population variation in thermal reaction norms of phenology in a viviparous lizard. Ecology 101:e03136. doi: 10.1002/ecy.3136
Dammhahn, M., and Almeling, L. (2012). Is risk taking during foraging a personality trait? A field test for cross-context consistency in boldness. Anim. Behav. 84, 1131–1139. doi: 10.1016/j.anbehav.2012.08.014
Darwin, C. (1859). On the Origin of Species by Means of Natural Selection, or the Preservation of Favoured Races in the Struggle for Life. London: John Murray.
DeWitt, T. J., Sih, A., and Wilson, D. S. (1998). Costs and limits of phenotypic plasticity. Trends Ecol. Evol. 13, 77–81.
Diamond, S. E., and Martin, R. A. (2021). “Buying time: plasticity and population persistence,” in Phenotypic Plasticity & Evolution: Causes, Consequences, Controversies, 1st Edn, ed. D. W. Pfennig (Boca Raton, FL: CRC Press), 185–209. doi: 10.1201/9780429343001-8
Dingemanse, N. J., and Reale, D. (2005). Natural selection and animal personality. Behaviour 142, 1159–1184. doi: 10.1163/156853905774539445
Dingemanse, N. J., and Wright, J. (2020). Criteria for acceptable studies of animal personality and behavioural syndromes. Ethology 126, 865–869. doi: 10.1111/eth.13082
Dingemanse, N. J., Bouwman, K. M., van de Pol, M., van Overveld, T., Patrick, S. C., Matthysen, E., et al. (2012). Variation in personality and behavioural plasticity across four populations of the great tit Parus major. J. Anim. Ecol. 81, 116–126. doi: 10.1111/j.1365-2656.2011.01877.x
Dingemanse, N. J., Kazem, A. J. N., Reale, D., and Wright, J. (2010). Behavioural reaction norms: Animal personality meets individual plasticity. Trends Ecol. Evol. 25, 81–89. doi: 10.1016/j.tree.2009.07.013
Dochtermann, N. A., Schwab, T., and Sih, A. (2015). The contribution of additive genetic variation to personality variation: Heritability of personality. Proc. R. Soc. Lond. B Biol. Sci. 282:20142201. doi: 10.1098/rspb.2014.2201
Dosmann, A. J., Brooks, K. C., and Mateo, J. M. (2015). Within-individual correlations reveal link between a behavioral syndrome, condition, and cortisol in free-ranging Belding’s ground squirrels. Ethology 121, 125–134. doi: 10.1111/eth.12320
Eccles, G. R., Bethell, E. J., Greggor, A. L., and Mettke-Hofmann, C. (2021). Individual variation in dietary wariness is predicted by head color in a specialist feeder, the Gouldian finch. Front. Ecol. Evol. 9:772812. doi: 10.3389/fevo.2021.772812
Evans, M. J., Hawley, J. E., Rego, P. W., and Rittenhouse, T. A. G. (2019). Hourly movement decisions indicate how a large carnivore inhabits developed landscapes. Oecologia 190, 11–23. doi: 10.1007/s00442-018-4307-z
Evans, R., Hindell, M., Kato, A., Phillips, L. R., Ropert-Coudert, Y., Wotherspoon, S., et al. (2020). Habitat utilization of a mesopredator linked to lower sea-surface temperatures & prey abundance in a region of rapid warming. Deep Sea Res. II Top. Stud. Oceanogr. 175:104634. doi: 10.1016/j.dsr2.2019.104634
Farwell, M., Fuzzen, M. L. M., Bernier, N. J., and McLaughlin, R. L. (2014). Individual differences in foraging behavior and cortisol levels in recently emerged brook charr (Salvelinus fontinalis). Behav. Ecol. Sociobiol. 68, 781–790. doi: 10.1007/s00265-014-1691-1
Foden, W. B., Butchart, S. H. M., Stuart, S. N., Vie, J. C., Akcakaya, H. R., Angulo, A., et al. (2013). Identifying the world’s most climate change vulnerable species: A systematic trait-based assessment of all birds, amphibians and corals. PLoS One 8:13. doi: 10.1371/journal.pone.0065427
Forsman, A., and Wennersten, L. (2016). Inter-individual variation promotes ecological success of populations and species: Evidence from experimental and comparative studies. Ecography 39, 630–648. doi: 10.1111/ecog.01357
Fox, R. J., Donelson, J. M., Schunter, C., Ravasi, T., and Gaitan-Espitia, J. D. (2019). Beyond buying time: The role of plasticity in phenotypic adaptation to rapid environmental change. Philos. Trans. R. Soc. B 374:20180174. doi: 10.1098/rstb.2018.0174
Fuller, A., Dawson, T., Helmuth, B., Hetem, R. S., Mitchell, D., and Maloney, S. K. (2010). Physiological mechanisms in coping with climate change. Physiol. Biochem. Zool. 83, 713–720. doi: 10.1086/652242
Geffroy, B., Alfonso, S., Sadoul, B., and Blumstein, D. T. (2020). A world for reactive phenotypes. Front. Conserv. Sci. 1:611919. doi: 10.3389/fcosc.2020.611919
Ghalambor, C. K., McKay, J. K., Carroll, S. P., and Reznick, D. N. (2007). Adaptive versus non-adaptive phenotypic plasticity and the potential for contemporary adaptation in new environments. Funct. Ecol. 21, 394–407. doi: 10.1111/j.1365-2435.2007.01283.x
Gregory, T. R. (2009). Understanding natural selection: Essential concepts and common misconceptions. Evolution 2, 156–175. doi: 10.1007/s12052-009-0128-1
Guindre-Parker, S., McAdam, A. G., van Kesteren, F., Palme, R., Boonstra, R., Boutin, S., et al. (2019). Individual variation in phenotypic plasticity of the stress axis. Biol. Lett. 15:20190260. doi: 10.1098/rsbl.2019.0260
Herath, A. P. H. M., Wat, K. K. Y., Banks, P. B., and McArthur, C. (2021). Animal personality drives individual dietary specialisation across multiple dimensions in a mammalian herbivore. Funct. Ecol. 35, 2253–2265. doi: 10.1111/1365-2435.13893
Herborn, K. A., Heidinger, B. J., Alexander, L., and Arnold, K. E. (2014). Personality predicts behavioral flexibility in a fluctuating, natural environment. Behav. Ecol. 25, 1374–1379. doi: 10.1093/beheco/aru131
Hertel, A. G., Royauté, R., Zedrosser, A., and Mueller, T. (2021). Biologging reveals individual variation in behavioural predictability in the wild. J. Anim. Ecol. 90, 723–737. doi: 10.1111/1365-2656.13406
Intergovernmental Panel on Climate Change [IPCC] (2014). in Climate Change 2014: Synthesis Report. Contribution of Working Groups I, II and III to the Fifth Assessment Report of the Intergovernmental Panel on Climate Change, eds Core Writing Team R. K. Pachauri and L. Meyer (Geneva: IPCC).
Intergovernmental Panel on Climate Change [IPCC] (2021a). in Climate Change 2021. The Physical Science Basis. Summary for Policymakers. Working Group I contribution to the Sixth Assessment Report of the Intergovernmental Panel on Climate Change, eds V. Masson-Delmotte, P. Zhai, A. Pirani, S. L. Connors, C. Péan, Y. Chen, et al. (Cambridge: Cambridge University Press).
Intergovernmental Panel on Climate Change [IPCC] (2021b). Intergovernmental panel on climate change (IPCC). Sixth assessment report (ARG6). Geneva: IPCC.
Intergovernmental Panel on Climate Change [IPCC] (2022a). Climate Change 2022. Impacts, Adaptation and Vulnerability. Full Report. Working Group II contribution to the Sixth Assessment Report of the Intergovernmental Panel on Climate Change. Cambridge: Cambridge University Press.
Intergovernmental Panel on Climate Change [IPCC] (2022b). Climate Change 2022. Impacts, Adaptation and Vulnerability. Technical Summary. Working Group II contribution to the Sixth Assessment Report of the Intergovernmental Panel on Climate Change. Cambridge: Cambridge University Press.
Jawor, J. M., Mcglothlin, J. W., Casto, J. M., Greives, T. J., Snajdr, E. A., Bentley, G. E., et al. (2006). Seasonal and individual variation in response to GnRH challenge in male dark-eyed juncos (Junco hyemalis). Gen. Comp. Endocrinol. 149, 182–189. doi: 10.1016/j.ygcen.2006.05.013
Jolles, J. W., Briggs, H. D., Araya-Ajoy, Y. G., and Boogert, N. J. (2019). Personality, plasticity and predictability in sticklebacks: Bold fish are less plastic and more predictable than shy fish. Anim. Behav. 154, 193–202. doi: 10.1016/j.anbehav.2019.06.022
Kareklas, K., Arnott, G., Elwood, R. W., and Holland, R. A. (2016). Plasticity varies with boldness in a weakly-electric fish. Front. Zool. 13:7. doi: 10.1186/s12983-016-0154-0
Kelly, M. (2019). Adaptation to climate change through genetic accommodation and assimilation of plastic phenotypes. Philos Trans. R. Soc. B 374:20180176. doi: 10.1098/rstb.2018.0176
Kempenaers, B., Peters, A., and Foerster, K. (2008). Sources of individual variation in plasma testosterone levels. Philos. Trans. R. Soc. B Biol. Sci. 363, 1711–1723.
Koolhaas, J. M., de Boer, S. F., Coppens, C. M., and Buwalda, B. (2010). Neuroendocrinology of coping styles: Towards understanding the biology of individual variation. Front. Neuroendocrinol. 31:307–321. doi: 10.1016/j.yfrne.2010.04.001
Koolhaas, J. M., Korte, S. M., De Boer, S. F., Van Der Vegt, B. J., Van Reenen, C. G., Hopster, H., et al. (1999). Coping styles in animals: Current status in behavior and stress-physiology. Neurosci. Biobehav. Rev. 23, 925–935. doi: 10.1016/S0149-7634(99)00026-3
Kortet, R., Vainikka, A., Janhunen, M., Piironen, J., and Hyvarinen, P. (2014). Behavioral variation shows heritability in juvenile brown trout Salmo trutta. Behav. Ecol. Sociobiol. 68, 927–934. doi: 10.1007/s00265-014-1705-z
Kurten, N., Schmaljohann, H., Bichet, C., Haest, B., Vedder, O., Gonzalez-Solis, J., et al. (2022). High individual repeatability of the migratory behaviour of a long-distance migratory seabird. Mov. Ecol. 10:16. doi: 10.1186/s40462-022-00303-y
Lapiedra, O., Chejanovski, Z., and Kolbe, J. J. (2017). Urbanization and biological invasion shape animal personalities. Glob. Change Biol. 23, 592–603. doi: 10.1111/gcb.13395
Last, P. R., White, W. T., Gledhill, D. C., Hobday, A. J., Brown, R., Edgar, G. J., et al. (2011). Long-term shifts in abundance and distribution of a temperate fish fauna: A response to climate change and fishing practices. Glob. Ecol. Biogeogr. 20, 58–72. doi: 10.1111/j.1466-8238.2010.00575.x
Lendvai, A. Z., Ouyang, J. Q., Schoenle, L. A., Fasanello, V., Haussmann, M. F., Bonier, F., et al. (2014). Experimental food restriction reveals individual differences in corticosterone reaction norms with no oxidative costs. PLoS One 9:e110564. doi: 10.1371/journal.pone.0110564
Low, M., Arlt, D., Knape, J., Part, T., and Oberg, M. (2019). Factors influencing plasticity in the arrival-breeding interval in a migratory species reacting to climate change. Ecol. Evol. 9, 12291–12301. doi: 10.1002/ece3.5716
MacDougall-Shackleton, S. A., Bonier, F., Romero, L. M., and Moore, I. T. (2019). Glucocorticoids and “stress” are not synonymous. Integr. Org. Biol. 1:obz017. doi: 10.1093/iob/obz017
Maldonado-Chaparro, A. A., Read, D. W., and Blumstein, D. T. (2017). Can individual variation in phenotypic plasticity enhance population viability? Ecol. Model. 352, 19–30. doi: 10.1016/j.ecolmodel.2017.02.023
Martin, R. O., Cunningham, S. J., and Hockey, P. A. R. (2015). Elevated temperatures drive fine-scale patterns of habitat use in a savanna bird community. Ostrich 86, 127–135. doi: 10.2989/00306525.2015.1029031
Mathies, T., Felix, T. A., and Lance, V. A. (2001). Effects of trapping and subsequent short-term confinement stress on plasma corticosterone in the brown treesnake (Boiga irregularis) on Guam. Gen. Comp. Endocrinol. 124, 106–114. doi: 10.1006/gcen.2001.7694
Mathot, K. J., van den Hout, P. J., Piersma, T., Kempenaers, B., Reale, D., and Dingemanse, N. J. (2011). Disentangling the roles of frequency-vs. state-dependence in generating individual differences in behavioural plasticity. Ecol. Lett. 14, 1254–1262. doi: 10.1111/j.1461-0248.2011.01698.x
McMahon, E. K., and Cavigelli, S. A. (2021). Gaps to address in ecological studies of temperament and physiology. Integr. Comp. Biol. 61, 1917–1932. doi: 10.1093/icb/icab118
McMahon, E. K., Youatt, E., and Cavigelli, S. A. (2022). A physiological profile approach to animal temperament: How to understand the functional significance of individual differences in behaviour. Proc. R. Soc. B Biol. Sci. 289:20212379. doi: 10.1098/rspb.2021.2379
Mella, V. S. A., Ward, A. J. W., Banks, P. B., and McArthur, C. (2015). Personality affects the foraging response of a mammalian herbivore to the dual costs of food and fear. Oecologia 177, 293–303. doi: 10.1007/s00442-014-3110-8
Metcalfe, N. B., Van Leeuwen, T. E., and Killen, S. S. (2016). Does individual variation in metabolic phenotype predict fish behaviour and performance? J. Fish Biol. 88, 298–321. doi: 10.1111/jfb.12699
Mimura, M., Yahara, T., Faith, D. P., Vázquez-Domínguez, E., Colautti, R. I., Araki, H., et al. (2017). Understanding and monitoring the consequences of human impacts on intraspecific variation. Evol. Appl. 10, 121–139. doi: 10.1111/eva.12436
Moran, N. A. (1992). The evolutionary maintenance of alternative phenotypes. Am. Nat. 139, 971–989. doi: 10.1086/285369
Murren, C. J., Auld, J. R., Callahan, H., Ghalambor, C. K., Handelsman, C. A., Heskel, M. A., et al. (2015). Constraints on the evolution of phenotypic plasticity: Limits and costs of phenotype and plasticity. Heredity 115, 293–301. doi: 10.1038/hdy.2015.8
Narayan, E. J., Cockrem, J. F., and Hero, J. M. (2011). Urinary corticosterone metabolite responses to capture and captivity in the cane toad (Rhinella marina). Gen. Comp. Endocrinol. 173, 371–377. doi: 10.1016/j.ygcen.2011.06.015
National Oceanic and Atmospheric Administration [NOAA] (2021). National Oceanic and Atmospheric Administration’s National Weather Service. Washington, DC: NOAA.
Nussey, D. H., Postma, E., Gienapp, P., and Visser, M. E. (2005). Selection on heritable phenotypic plasticity in a wild bird population. Science 310, 304–306. doi: 10.1126/science.1117004
Nussey, D. H., Wilson, A. J., and Brommer, J. E. (2007). The evolutionary ecology of individual phenotypic plasticity in wild populations. J. Evol. Biol. 20, 831–844. doi: 10.1111/j.1420-9101.2007.01300.x
Odeh, F. M., Cadd, G. G., and Satterlee, D. G. (2003). Genetic characterization of stress responsiveness in Japanese quail. 1. analyses of line effects and combining abilities by diallel crosses. Poult. Sci. 82, 25–30. doi: 10.1093/ps/82.1.25
Orr, H. A. (2009). Fitness and its role in evolutionary genetics. Nat. Rev. Genet. 10, 531–539. doi: 10.1038/nrg2603
Overli, O., Pottinger, T. G., Carrick, T. R., Overli, E., and Winberg, S. (2002). Differences in behaviour between rainbow trout selected for high- and low-stress responsiveness. J. Exp. Biol. 205, 391–395.
Polverino, G., Palmas, B. M., Evans, J. P., and Gasparini, C. (2019). Individual plasticity in alternative reproductive tactics declines with social experience in male guppies. Anim. Behav. 148, 113–121. doi: 10.1016/j.anbehav.2018.12.014
Porlier, M., Charmantier, A., Bourgault, P., Perret, P., Blondel, J., and Garant, D. (2012). Variation in phenotypic plasticity and selection patterns in blue tit breeding time: Between- and within-population comparisons. J. Anim. Ecol. 81, 1041–1051. doi: 10.1111/j.1365-2656.2012.01996.x
Reale, D., Reader, S. M., Sol, D., McDougall, P. T., and Dingemanse, N. J. (2007). Integrating animal temperament within ecology and evolution. Biol. Rev. 82, 291–318. doi: 10.1111/j.1469-185X.2007.00010.x
Rodel, H. G., Zapka, M., Talke, S., Kornatz, T., Bruchner, B., and Hedler, C. (2015). Survival costs of fast exploration during juvenile life in a small mammal. Behav. Ecol. Sociobiol. 69, 205–217. doi: 10.1007/s00265-014-1833-5
Romero, L. M., and Beattie, U. K. (2022). Common myths of glucocorticoid function in ecology and conservation. J. Exp. Zool. A Ecol. Integr. Physiol. 337, 7–14. doi: 10.1002/jez.2459
Romero, L. M., and Gormally, B. M. G. (2019). How truly conserved is the “well-conserved” vertebrate stress response? Integr. Comp. Biol. 59, 273–281. doi: 10.1093/icb/icz011
Romero, L. M., and Reed, J. M. (2005). Collecting baseline corticosterone samples in the field: Is under 3 min good enough? Comp. Biochem. Physiol. A Mol. Integr. Physiol. 140, 73–79. doi: 10.1016/j.cbpb.2004.11.004
Romero, L. M., Meister, C. J., Cyr, N. E., Kenagy, G. J., and Wingfield, J. C. (2008). Seasonal glucocorticoid responses to capture in wild free-living mammals. Am. J. Physiol. Regul. Integr. Comp. Physiol. 294, R614–R622. doi: 10.1152/ajpregu.00752.2007
Sapolsky, R. M., Romero, L. M., and Munck, A. U. (2000). How do glucocorticoids influence stress responses? Integrating permissive, suppressive, stimulatory, and preparative actions. Endocr. Rev. 21, 55–89. doi: 10.1210/er.21.1.55
Sauve, D., Divoky, G., and Friesen, V. L. (2019). Phenotypic plasticity or evolutionary change? An examination of the phenological response of an arctic seabird to climate change. Funct. Ecol. 33, 2180–2190. doi: 10.1111/1365-2435.13406
Scharffenberg, K. C., Whalen, D., MacPhee, S. A., Marcoux, M., Iacozza, J., Davoren, G., et al. (2020). Oceanographic, ecological, and socio-economic impacts of an unusual summer storm in the Mackenzie Estuary. Arctic Sci. 6, 62–76. doi: 10.1139/as-2018-0029
Schlichting, C. D., and Pigliucci, M. (1998). Phenotypic Evolution: A Reaction Norm Perspective. Sunderland, MA: Sinauer Associates.
Schmalhausen, I., Dordick, I., and Dobzhansky, T. (1949). Factors of Evolution : The Theory of Stabilizing Selection. Philadelphia: Blakiston.
Schwarz, J. F. L., Mews, S., DeRango, E. J., Langrock, R., Piedrahita, P., Paez-Rosas, D., et al. (2021). Individuality counts: New comprehensive approach to foraging strategies of a tropical marine predator. Oecologia 195, 313–325. doi: 10.1007/s00442-021-04850-w
Sih, A. (2013). Understanding variation in behavioural responses to human-induced rapid environmental change: A conceptual overview. Anim. Behav. 85, 1077–1088. doi: 10.1016/j.anbehav.2013.02.017
Sih, A., and Del Giudice, M. (2012). Linking behavioural syndromes and cognition: A behavioural ecology perspective. Philos. Trans. R. Soc. B 367, 2762–2772. doi: 10.1098/rstb.2012.0216
Sih, A., Bell, A. M., Johnson, J. C., and Ziemba, R. E. (2004). Behavioral syndromes: An integrative overview. Q. Rev. Biol. 79, 241–277.
Sih, A., Ferrari, M. C. O., and Harris, D. J. (2011). Evolution and behavioural responses to human-induced rapid environmental change. Evol. Appl. 4, 367–387. doi: 10.1111/j.1752-4571.2010.00166.x
Sih, A., Stamps, J., Yang, L. H., McElreath, R., and Ramenofsky, M. (2010). Grand challenges. behavior as a key component of integrative biology in a human-altered world. Integr. Comp. Biol. 50, 934–944. doi: 10.1093/icb/icq148
Stamps, J., and Groothuis, T. G. G. (2010). The development of animal personality: Relevance, concepts and perspectives. Biol. Rev. 85, 301–325. doi: 10.1111/j.1469-185X.2009.00103.x
Stearns, S. C. (1989). The evolutionary significance of phenotypic plasticity. phenotypic sources of variation among organisms can be described by developmental switches and reaction norms. Bioscience 39, 436–445. doi: 10.2307/1311135
Taff, C. C., and Vitousek, M. N. (2016). Endocrine flexibility: Optimizing phenotypes in a dynamic world? Trends Ecol. Evol. 31, 476–488. doi: 10.1016/j.tree.2016.03.005
Telwala, Y., Brook, B. W., Manish, K., and Pandit, M. K. (2013). Climate-induced elevational range shifts and increase in plant species richness in a Himalayan biodiversity epicentre. PLoS One 8:e57103. doi: 10.1371/journal.pone.0057103
Toth, Z., Mahr, K., Olevecki, G., Ori, L., and Lendvai, A. Z. (2022). Food restriction reveals individual differences in insulin-like growth factor-1 reaction norms. Front. Ecol. Evol. 10:826968. doi: 10.3389/fevo.2022.826968
Touchon, J. C. (2012). A treefrog with reproductive mode plasticity reveals a changing balance of selection for nonaquatic egg laying. Am. Nat. 180, 733–743. doi: 10.1086/668079
United Nations (1992). United Nations Framework Convention on Climate Change. New York, NY: United Nations.
Valladares, F., Matesanz, S., Guilhaumon, F., Araujo, M. B., Balaguer, L., Benito-Garzon, M., et al. (2014). The effects of phenotypic plasticity and local adaptation on forecasts of species range shifts under climate change. Ecol. Lett. 17, 1351–1364. doi: 10.1111/ele.12348
Valladares, F., Sanchez-Gomez, D., and Zavala, M. A. (2006). Quantitative estimation of phenotypic plasticity: bridging the gap between the evolutionary concept and its ecological applications. J. Ecol. 94, 1103–1116. doi: 10.1111/j.1365-2745.2006.01176.x
Vetter, S. G., Brandstatter, C., Macheiner, M., Suchentrunk, F., Gerritsmann, H., and Bieber, C. (2016). Shy is sometimes better: Personality and juvenile body mass affect adult reproductive success in wild boars, Sus scrofa. Anim. Behav. 115, 193–205. doi: 10.1016/j.anbehav.2016.03.026
Videlier, M., Bonneaud, C., Cornette, R., and Herrel, A. (2014). Exploration syndromes in the frog Xenopus (Silurana) tropicalis: Correlations with morphology and performance? J. Zool. 294, 206–213. doi: 10.1111/jzo.12170
Villegas-Ríos, D., Réale, D., Freitas, C., Moland, E., and Olsen, E. M. (2018). Personalities influence spatial responses to environmental fluctuations in wild fish. J. Anim. Ecol. 87, 1309–1319. doi: 10.1111/1365-2656.12872
Wiens, J. J. (2016). Climate-related local extinctions are already widespread among plant and animal species. PLoS Biol. 14:e2001104. doi: 10.1371/journal.pbio.2001104
Williams, T. D., Kitaysky, A. S., and Vezina, F. V. (2004). Individual variation in plasma estradiol-17β and androgen levels during egg formation in the European starling Sturnus vulgaris: Implications for regulation of yolk steroids. Gen. Comp. Endocrinol. 136, 346–352. doi: 10.1016/j.ygcen.2004.01.010
Wilson, D. S. (1998). Adaptive individual differences within single populations. Philos. Trans. R. Soc. Lond B 353, 199–205. doi: 10.1098/rstb.1998.0202
Wolf, M., and McNamara, J. M. (2012). On the evolution of personalities via frequency-dependent selection. Am. Nat. 179, 679–692. doi: 10.1086/665656
Wong, B. B. M., and Candolin, U. (2014). Behavioral responses to changing environments. Behav. Ecol. 26, 665–673. doi: 10.1093/beheco/aru183
Keywords: individual variation, personality, climate change, reaction norms, glucocorticoids, HIREC, phenotypic plasticity
Citation: Cockrem JF (2022) Individual variation, personality, and the ability of animals to cope with climate change. Front. Ecol. Evol. 10:897314. doi: 10.3389/fevo.2022.897314
Received: 16 March 2022; Accepted: 15 August 2022;
Published: 02 September 2022.
Edited by:
Kwasi Connor, University of California, Irvine, United StatesReviewed by:
Frédéric Angelier, UMR7372 Centre d’Études Biologiques de Chizé (CEBC), FranceTal Avgar, Utah State University, United States
Copyright © 2022 Cockrem. This is an open-access article distributed under the terms of the Creative Commons Attribution License (CC BY). The use, distribution or reproduction in other forums is permitted, provided the original author(s) and the copyright owner(s) are credited and that the original publication in this journal is cited, in accordance with accepted academic practice. No use, distribution or reproduction is permitted which does not comply with these terms.
*Correspondence: John F. Cockrem, J.F.Cockrem@massey.ac.nz