- 1Department of Biology, Mount Allison University, Sackville, NB, Canada
- 2Prairie and Northern Wildlife Research Centre, Science and Technology Branch, Environment and Climate Change Canada, Saskatoon, SK, Canada
- 3Canadian Wildlife Service, Environment and Climate Change Canada, Sackville, NB, Canada
The Bay of Fundy, Canada is a critical staging area for Semipalmated Sandpipers (Calidris pusilla) during post-breeding migration. Recent range-wide population declines and changes in diet and migratory timing in the Bay of Fundy prompted a re-examination of staging ecology, including length of stay (last estimated in 1981), which is used in calculating migratory population estimates. We used radio-telemetry and the Motus Wildlife Tracking System to estimate individual length of stay and departure conditions for 159 Semipalmated Sandpipers in 2013 and 2014. Using tracking data we compared two estimation methods, minimum length of stay and mark-recapture modelling. Using minimum length of stay, the mean length of stay was approximately 21 days, an increase from the previous estimate of 15 days. Mark-recapture models suggested a much longer staging period that is inconsistent with other data. Sandpipers captured early in the staging period stayed longer on average than those captured later. Departures from the staging area were correlated with north-westerly winds, moderate to high wind speeds and low but rising atmospheric pressures. We suggest that Semipalmated Sandpipers in the Bay of Fundy are not operating on a time-selected migration schedule and instead wait for favourable weather conditions to depart, which occur more often later in the migratory period. Population trends in the Bay of Fundy should be re-evaluated in light of the increased length of stay.
Introduction
Shorebirds are highly migratory, with many species travelling from their Arctic breeding grounds to southern wintering areas and back each year (Myers et al., 1987). These areas are often separated by vast expanses of unsuitable habitat, resulting in long, non-stop flights between distinct staging sites (Myers, 1983; Morrison, 1984; Myers et al., 1987; Warnock, 2010). At these staging sites, migrants feed extensively and accumulate fat reserves needed to support flights to the next destination (Hicklin and Smith, 1979; Zwarts et al., 1990; Pfister et al., 1998). Large proportions of global populations often rely on a few key staging sites, and changes at these geographic bottlenecks can have significant impacts on the status of the population (Myers, 1983; Myers et al., 1987; Niles et al., 2009; Studds et al., 2017; Li et al., 2019). Thus, having an accurate understanding of staging ecology plays a key role in effective monitoring and conservation.
Knowledge of individual length of stay is a critical aspect of understanding how shorebirds use particular staging sites. Historically length of stay was expected to be dependent on rates of mass gain and migrants were thought to depart as soon as sufficient fat levels were acquired (Dunn et al., 1988; Alerstam and Lindström, 1990; Lyons et al., 2008; Turcotte et al., 2013). However, many studies have found a lack of a relationship between mass at capture and length of stay, suggesting other extrinsic factors play a role (Page and Middleton, 1972; Holmgren et al., 1993; Skagen and Knopf, 1994; Lyons and Haig, 1995; Warnock and Bishop, 1998). Some of these missing pieces, such as unrecognized variation in migratory strategy, may be resolvable in future using new tracking technologies. Linscott and Senner (2021) discussed non-fuelling behaviours, such as sleeping, recovery and social interactions, regularly observed at staging sites which could decouple length of stay from rate of weight gain. Further, the relationship between fuel load on capture and length of stay can vary with time in the season (Anderson et al., 2019) and staging site quality (Herbert et al., 2022). Finally, in addition to extensive within-season variation, length of stay may also vary among years or increase or decrease over a period of many years as a result of changes in habitat quality, prey availability, predator pressure or climate conditions (e.g., Ydenberg et al., 2004; Gordo, 2007).
Accurate knowledge of length of stay is particularly important in population estimates (Bishop et al., 2000), and unrecognized changes in length of stay can generate false population trends (Bishop et al., 2000; Ydenberg et al., 2004). There are different methods for estimating length of stay. Minimum length of stay is commonly used in tracking studies (Kennedy et al., 2017; Anderson et al., 2019; Herbert et al., 2022), though alternative methods like mark-recapture modeling, which can account for time at a site prior to capture, could provide more accurate estimates (Pradel et al., 2005; Pledger et al., 2009). To date there has been limited work directly comparing these methods in shorebirds, though what has been done suggests substantial discrepancies (Howell et al., 2019).
Staging periods are often longer than required to acquire the necessary fat stores, particularly on post-breeding migration (e.g., Pfister et al., 1998; Roques et al., 2021), possibly due to birds arriving at staging sites early and lingering throughout the season. Alerstam and Lindström (1990) suggested that early arrival might be beneficial, for example, when competing for food resources at staging sites (Schneider and Harrington, 1981). However, while extending time at a staging site by early arrival may be advantageous, late departure may pose added risks. There are numerous examples of later-arriving migrants staying for shorter periods than birds that arrived earlier in the season (Lank, 1983; Lyons and Haig, 1995; Warnock and Bishop, 1998; Warnock et al., 2004). Constraints such as predator presence and weather conditions may prevent extension of stays at staging areas. There is evidence that Western Sandpipers (Calidris mauri) have been shortening their length of stay to stay ahead of migrating raptors as Peregrine Falcon (Falco peregrinus) populations have recovered (Butler et al., 2003; Ydenberg et al., 2004; Hope et al., 2011). Favourable weather conditions assist migrating birds (Williams et al., 1977; Richardson, 1979; Stoddard et al., 1983; Anderson et al., 2019; Roques et al., 2021) and may be even more important than fat stores in migratory decisions by substantially reducing the cost of flight (Butler et al., 1997). However, during fall migrations the frequency and intensity of storms usually increases as the season progresses, which may prevent migrants from extending their staging period late into the season and contribute to observed seasonal patterns of length of stay (Morrison et al., 2012; Environment and Climate Change Canada, 2022).
Semipalmated Sandpipers (Calidris pusilla) are one of the most widely distributed and numerous shorebirds in North America (Andres et al., 2012), and a substantial portion of the world population stages in the Bay of Fundy, NS and NB, Canada during their fall migration (Hicklin, 1987). These small shorebirds arrive in the region from their Arctic breeding grounds, double their weight during their stay (Hicklin and Smith, 1979) and depart from the Bay of Fundy on a non-stop trans-oceanic flight of 3,000–4,000 km to South America (Hicklin and Gratto-Trevor, 2010). This species, like most shorebirds (Rosenberg et al., 2019), has experienced widespread population declines, possibly due to human harvest in northern South America (Morrison et al., 2012), reduction of prey and mass gain during spring stopover in Delaware Bay (Mizrahi et al., 2012), and climate change and related impacts on the breeding grounds (Andres et al., 2012; Peterson et al., 2013). In the Bay of Fundy, peak Semipalmated Sandpiper migration has shifted later since the 1980’s (Bliss et al., 2019), and diet has shifted from heavy reliance on the amphipod Corophium volutator (Hicklin and Smith, 1979, 1984) to a more generalist diet (Quinn and Hamilton, 2012; Gerwing et al., 2016; Neima, 2017). We have also seen that there are sub-populations within the Bay of Fundy that generally do not intermingle (Neima et al., 2020), and that diets vary among regions (Quinn and Hamilton, 2012; Neima, 2017). This indicates that conditions may have changed significantly since length of stay in the Bay of Fundy was last estimated in 1981 (Hicklin, 1987). In view of a potentially declining population, it is vital to have an accurate and current estimate of length of stay. We undertook this study to assess two main questions: (1) what is the current length of stay, and (2) has length of stay in the Bay of Fundy changed since it was last estimated in 1981, and if so by how much? We also compared methods for estimating length of stay, including minimum length of stay (e.g., Anderson et al., 2019) and mark-recapture modelling (Schaub et al., 2001; Pledger et al., 2009). For both model types, we assessed regional and temporal variation in length of stay and coupled that information with existing knowledge of diet in different arms of the Bay. Finally, we examined departure routes and conditions that may influence departure timing, as these could offer potential explanations for changing length of stay in this species.
Materials and methods
Study sites, capture methods, and radio-telemetry
We captured Semipalmated Sandpipers in August 2013 and 2014 using mist nets and Fundy pull-traps (Hicklin et al., 1989). We captured them in three different regions within the Bay of Fundy (Chignecto Bay, Cobequid Bay, and Minas Basin; Figure 1) and across time periods that reflect passage of both early and later migrants (see Supplementary Figure 1). Each bird was banded with an individually coded metal band for lifelong identification, one to two plastic colour bands to identify them as part of our project, and a field-readable coded plastic leg flag for visual identification in the field. To determine length of stay, we deployed a total of 179 individually coded 0.35-g Lotek radio-transmitters (NTQB-2, Lotek Wireless Inc., Newmarket, ON, Canada) on adult birds: 85 in 2013 and 94 in 2014. For details on capture and radio-transmitter specifications, see Neima et al. (2020). The total weight of the transmitter and leg bands was <2% of the body weight of a light sandpiper (20 g). We selectively tagged light birds, as low mass is indicative of recent arrival in the Bay of Fundy; 88% of radio-tagged birds were lighter than 30 g at capture (maximum = 33.6 g). We chose this approach because this is a staging site—used as a single stop by the majority of birds during their southbound migration (Warnock, 2010). Heavy birds are thus highly unlikely to have arrived recently, and tagging them would have limited our ability to track movements in the region, as well as seriously underestimated their staging duration using the minimum length of stay method.
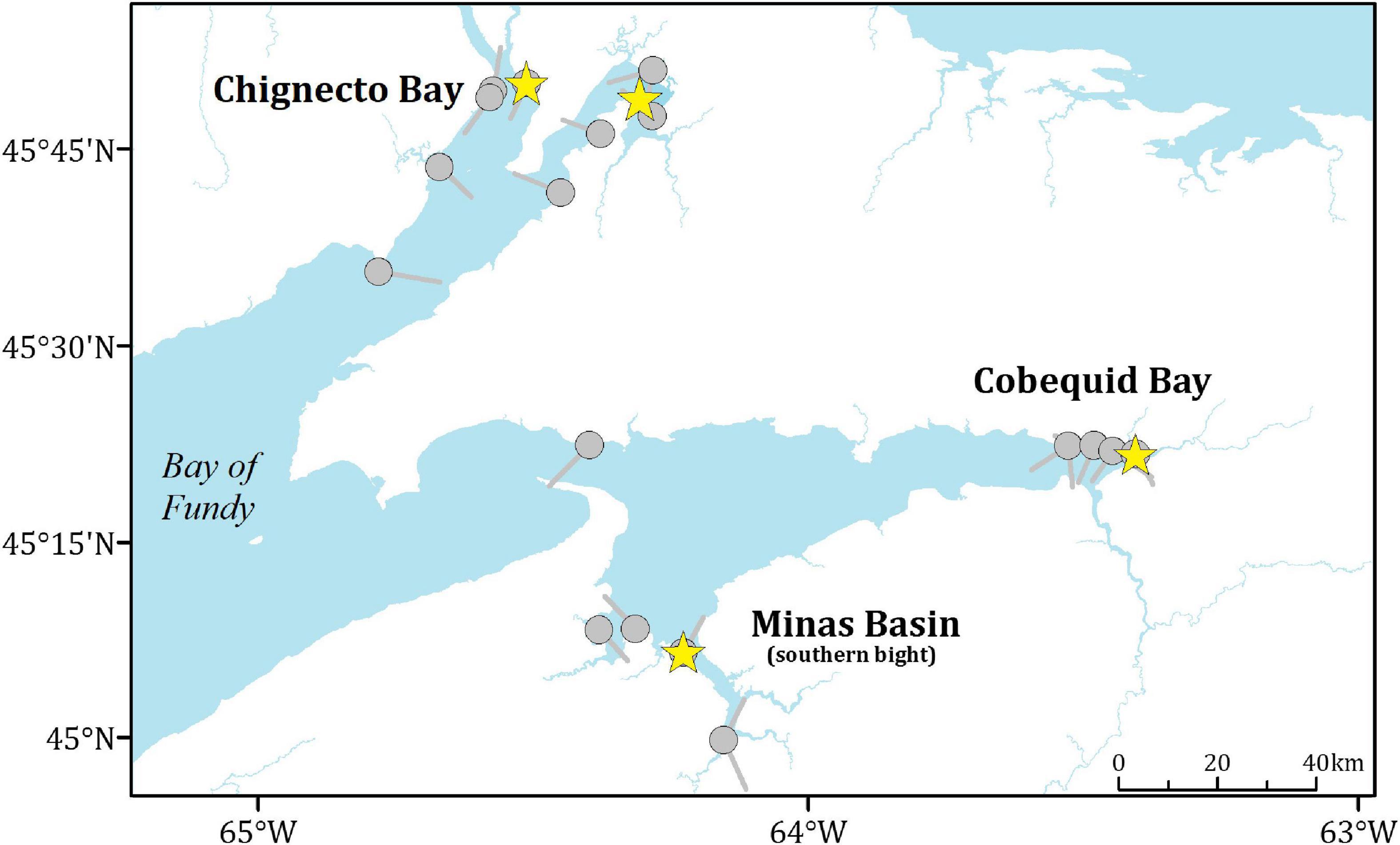
Figure 1. Map of the upper Bay of Fundy showing the three regions of the bay (Chignecto Bay, Cobequid Bay, and Minas Basin), catch locations (yellow stars), receiver locations and antenna orientations (grey circles and lines).
We tracked the birds throughout the staging period using a combination of aerial surveys, mobile ground tracking and an array of automated stationary receivers placed at key foraging and roost sites (Figure 1). Stationary receivers were either a Lotek DL model (Lotek Wireless Inc., Newmarket, ON, Canada) or a Sensorgnome (Taylor et al., 2017). For details on receiver configuration and function, see Taylor et al. (2017) and Neima et al. (2020). In addition to our own array within the Bay of Fundy, we also had access to data collected by receivers stationed along the coast of Nova Scotia and the north eastern United States,1 allowing us to track birds following their departure from the region. We augmented the stationary receiver array with mobile ground and aerial tracking as described in Neima et al. (2020).
Statistical analysis
All analyses were performed using R, version 4.0.4 (R Core Team, 2021), with an R Studio interface (RStudio Team, 2020). Parametric assumptions were tested using Cochrans test of homogeneity of variance and Shapiro-Wilk normality tests combined with visual inspection of Q-Q plots. As data were unbalanced, Type III sums of squares were used to test for an interaction, and when no interaction was present, Type II was used (Langsrud, 2003; Hector et al., 2010). Tukey’s HSD was used for post hoc comparisons when appropriate.
We performed a linear regression of length of stay against relative fuel loads for each region and year combination to determine whether mass at tagging affected length of stay. Relative fuel loads at capture were calculated using the following equation from Anderson et al. (2019):
Estimated lean mass was calculated using separate regression equations for adults and juveniles, generated by Anderson et al. (2019) using wing cord to account for variability in size among individuals.
We calculated the individual minimum length of stay as the time from the release of the tagged bird until its last detection in the region. When calculating the mean length of stay for each year, we excluded birds that stayed less than 5 days and were not detected on receivers outside the Bay of Fundy, as these birds were well outside the population distribution (Figure 2, N2013 = 4, N2014 = 12). The majority of those (9/16) were detected for less than 1 day, likely the result of lost or defective transmitters. Of the remaining seven birds excluded, all but two were tracked for only 2 or 3 days. Excluded birds weighed between 23.3 and 30.8 g at capture. At that weight, 5 days was not sufficient time to accumulate the remaining fat necessary to migrate successfully, making it unlikely that these data represent true length of stay. We also excluded one bird in 2014 with extremely unusual behaviour–it left the Bay of Fundy and was detected on the eastern US coast and then returned to Fundy (Neima et al., 2020). This left 81 birds in 2013 (95%) and 78 in 2014 (83%) for the minimum length of stay analysis.
To compare minimum length of stay between regions, we included only birds that had stayed in one region for their entire staging period [95% of birds, Neima et al. (2020)]. Due to significant interactions between region and year, we split by year. For both years we completed ANCOVAs with length of stay as the dependent variable and region as a categorical predictor. Date of tagging (day of year) and relative fuel loads were included as covariates in both years. In 2014 length of stay was log transformed to meet assumptions. We extracted beta coefficients for continuous predictors using the lm.beta R package (Behrendt, 2022). We used Tukey’s post hoc comparisons to examine differences in length of stay between regions.
We also estimated length of stay using mark-recapture models. We fit Pradel Survival and Seniority models using the RMark interface (Laake and Rexstad, 2022) for program Mark2, closely following methods described in Howell et al. (2019). We estimate survival (φ, interpreted as site, or regional fidelity), recapture (p), and seniority (γ, interpreted as probability of being at the site prior to capture) parameters. φ is the combination of true survival (S) and site fidelity (F), but for these models we only used data from birds with confirmed departures from the region. Therefore we assume S = 1, allowing us to interpret φ as site fidelity. The telemetry towers were constantly scanning for tags, so we expected recapture probability (p) to be high. Given the variability in the landscape within the Bay we assume it was not perfect, and therefore set p at 0.90. Both φ and γ were held constant to estimate length of stay. We analyzed data from 2013 and 2014 separately to account for differences in capture history lengths between years. Capture histories were generated using detection data. Days where a tagged bird were detected in the region received a “1,” and days they weren’t detected were “0,” beginning on the date the first tag was deployed and continuing until the last tagged bird departed the region. We included location of tagging (area of the Bay) as a categorical variable, and date of tagging and fuel loads as covariates. We generated an estimated length of stay for each area of the Bay, and an average for each year.
To directly compare estimates of length of stay using the two methods, we ran a second set of minimum length of stay models using only birds which had a confirmed departure from the region (N2013 = 37, N2014 = 53), the same dataset used for mark-recapture models. We completed separate ANCOVAs for each year due to an interaction. Length of stay was the dependent variable, region was the categorical predictor, and date of tagging and relative fuel loads were covariates.
Confirmed departures from the Bay of Fundy were obtained when birds were detected by the Motus receiver array on the coast of Nova Scotia between New Harbour (45.16746°, –61.45481°) and Outer Island (43.45638°, –65.7436°), and in one case, on the eastern coast of the United States (Figure 4). We examined effects of weather conditions on departure timing using weather data from the Halifax Stanfield International Airport (44.88483°, –63.51165°, weatherspark.com) that corresponded with timing of detections from these receiver arrays. Weather data were partitioned by hour, so we modelled the number of bird departures per hour against wind speeds (m/s), wind direction (N, E, S, W), atmospheric pressure (mbar), change in atmospheric pressure (mbar/h), and year (2013, 2014, dummy coded as 0 and 1, respectively) using a Poisson distribution with a log-link function to deal with count data. The final detection for each bird was considered its departure. We then used the dredge function and AIC model selection to identify top models predicting bird departures [R package “MuMIn”; Barton (2020)]. Models with AICc values < 2 were averaged using the model.avg function in MuMin, and we present the conditional averaged coefficients.
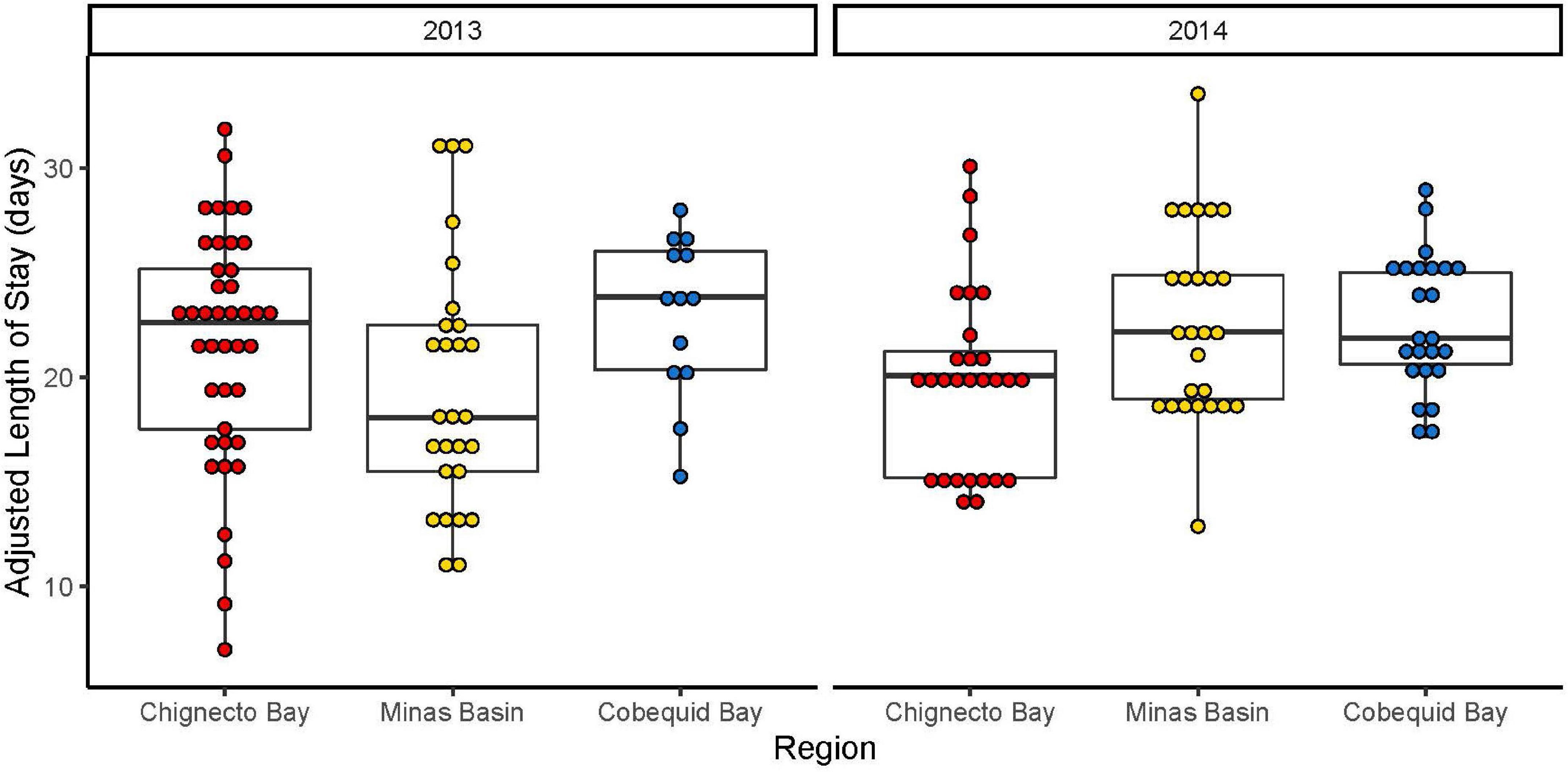
Figure 3. Adjusted length of stay (controlling for date of capture and relative fuel load) of Semipalmated Sandpipers using separate regions (Chignecto Bay, Cobequid Bay, and Minas Basin) of the Bay of Fundy in 2013 and 2014. Boxes represent the median (midline) and first and third quartile (ends). Details of capture timing are provided in Neima et al. (2020) and Supplementary Figure 1.
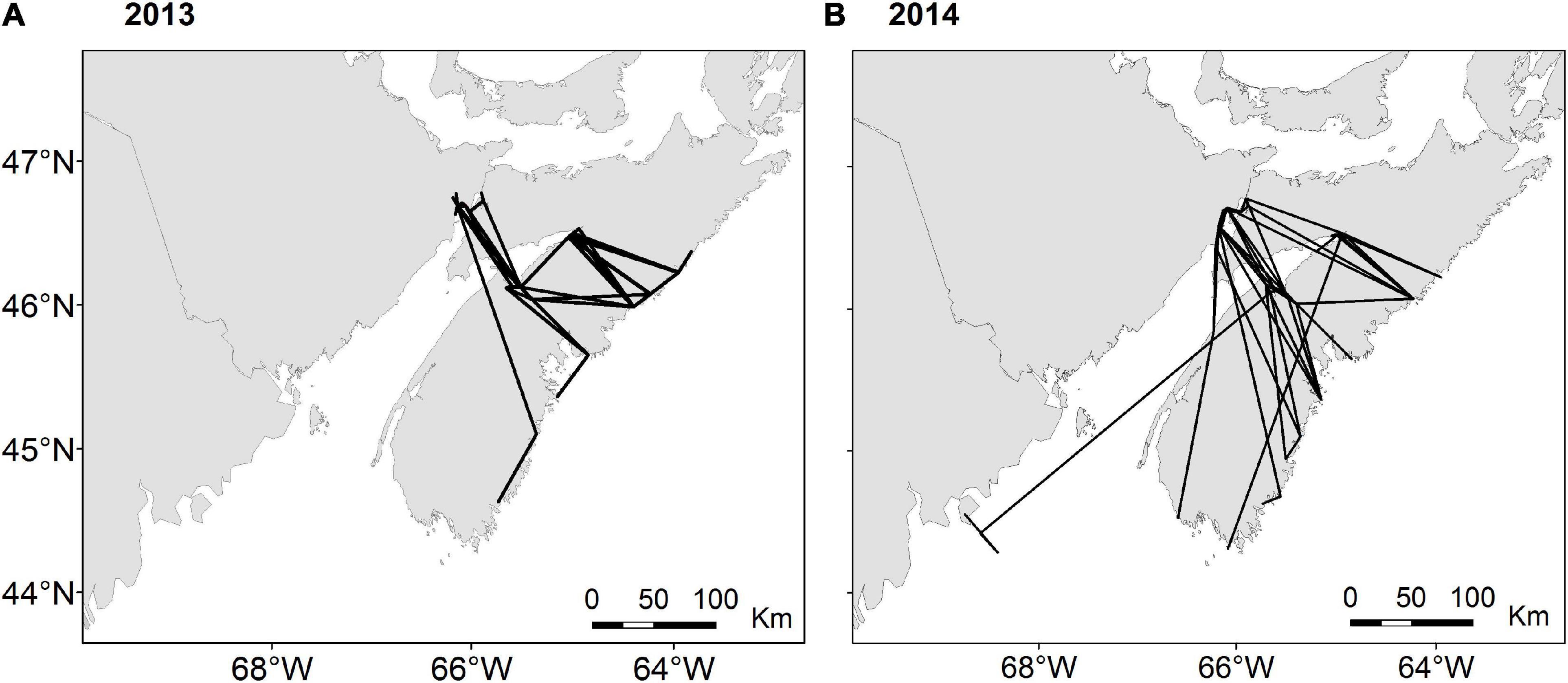
Figure 4. Routes taken by all Semipalmated Sandpipers detected following departure from the Bay of Fundy in (A) 2013 (N = 37) and (B) 2014 (N = 55). All detections along the outer coast were “fly-by” events where individuals were detected for <1 h.
Results
Using the minimum length of stay method, the average length of stay was estimated to be 21.1 ± 6.7 in 2013 and 21.4 ± 4.9 in 2014. We found very little evidence of a relationship between relative fuel load at tagging and length of stay in Cobequid or Chignecto Bay, but there was a negative relationship with fuel loads in the Minas Basin in both years [2013: F(1,25) = 8.97, p = 0.006, R2 = 0.26; 2014: F(1,24) = 5.87, p = 0.02, R2 = 0.20]. While the relationship was not significant in all regions, the trends were similar, so we included relative fuel load as a covariate in our models.
There was a significant interaction between region and year in the analysis of minimum length of stay [F(2,151) = 6.77, p = 0.001], so we split by year to further assess differences. In 2013, there were significant differences in length of stay among regions [F(2,76) = 4.03, p = 0.02]. Sandpipers tagged in the Minas Basin stayed significantly less time than birds from Chignecto or Cobequid Bay (Minas vs. Cobequid p = 0.02, Minas vs. Chignecto p = 0.04), spending ∼4 days less in the region (Table 1 and Figure 3). There was no effect of tagging date or relative fuel loads on length of stay in 2013 [date of tagging: F(1,76) = 1.00, p = 0.32; Relative Fuel Loads: F(1,76) = 2.83, p = 0.10]. In 2014, differences in length of stay varied among regions as well [F(2,73) = 4.36, p = 0.02], and there was a significant effect of tagging date [F(1,73) = 21.05, p < 0.001, Std. β Coeff = –0.545], with birds tagged earlier in the season staying longer than those tagged later in the season. There was no effect of fuel loads on length of stay [F(1,73) = 0.17, p = 0.68]. Sandpipers tagged in Chignecto differed from those tagged in Cobequid or the Minas Basin (Chignecto vs. Minas Basin p = 0.04; Chignecto vs. Cobequid p = 0.04) and stayed approximately 3 days less in the region (Table 1).
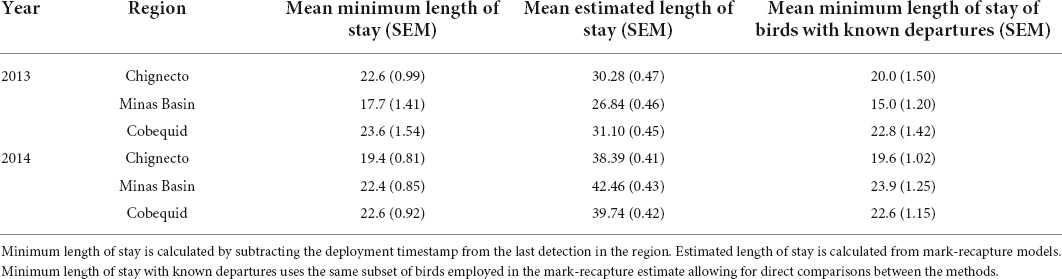
Table 1. Mean length of stay (days) controlling for date of capture and relative fuel loads at capture of tagged sandpipers for each region of the Bay of Fundy in 2013 and 2014.
Using mark-recapture models, the estimated length of stay was much higher than the minimum method, with means between 30 and 40 days (Table 1). This difference remained when we repeated our analyses of minimum length of stay using the same dataset as mark-recapture models (only birds with known departures); removal of birds for which we did not detect departure had little effect on mean lengths of stay (Table 1). Large differences between minimum length of stay and mark-recapture models are likely due to the estimation of the seniority parameter in the mark-recapture models. This is the estimate of how long the birds were at the site prior to capture. The gamma parameter estimates birds were present 14.6 days prior to capture in 2013, and 22.4 days in 2014 (see “Discussion”).
We detected 37 birds outside the Bay of Fundy as they departed the region in 2013 and 53 birds in 2014. All birds except one departed the Bay of Fundy by flying over mainland Nova Scotia to the southeast coast. The bird that took a different path was detected along the coast of Maine, after presumably flying out the mouth of the Bay of Fundy (Figure 4). No other birds were detected along the US coast following departure from the Bay of Fundy, suggesting that they departed over the ocean toward South America. All detections along the coast lasted under 1 h in both years (median 2013 = 8 min, median 2014 = 4 min), indicating that these were “fly-by” detections as birds flew over or past receivers without stopping. Departures were more frequent on certain dates than others (Figure 5). AICc model selection revealed that the best model for bird departures included atmospheric pressure, wind direction and speed, and year, with the second-best model adding change in atmospheric pressure (Table 2). In the top model, north-westerly winds (standardized beta-coefficients relative to E; N = 2.21, W = 1.68, S = –1.48), moderate to high wind speeds (1.07), and low atmospheric pressures (–1.64) were correlated with more departures (Figures 6, 7). The significant effect of year (0.75) simply reflects the fact that more birds were detected departing in 2014 than 2013.
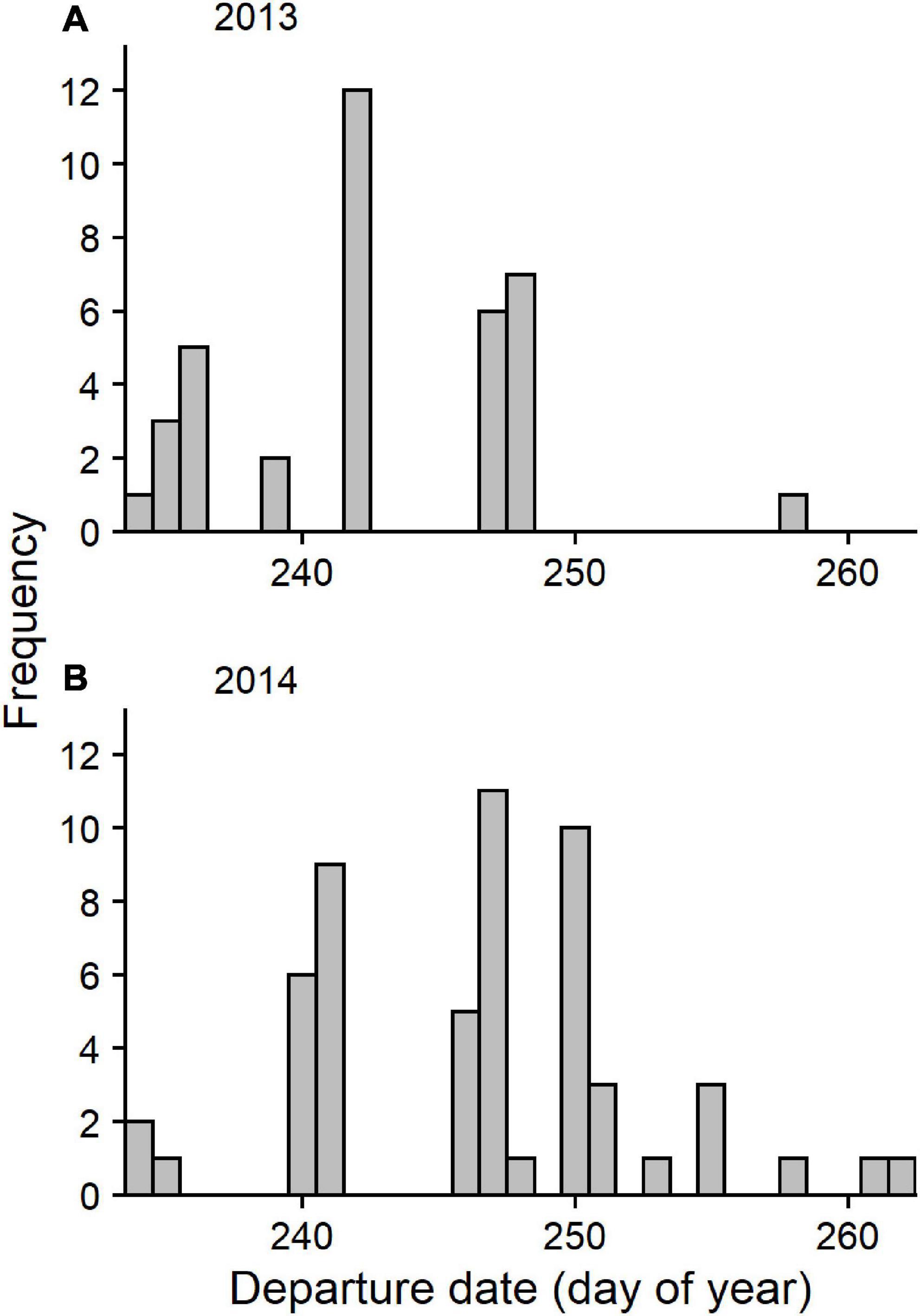
Figure 5. Frequency of departures by tagged Semipalmated Sandpipers from the Bay of Fundy on each day of the fall staging period in (A) 2013 and (B) 2014.
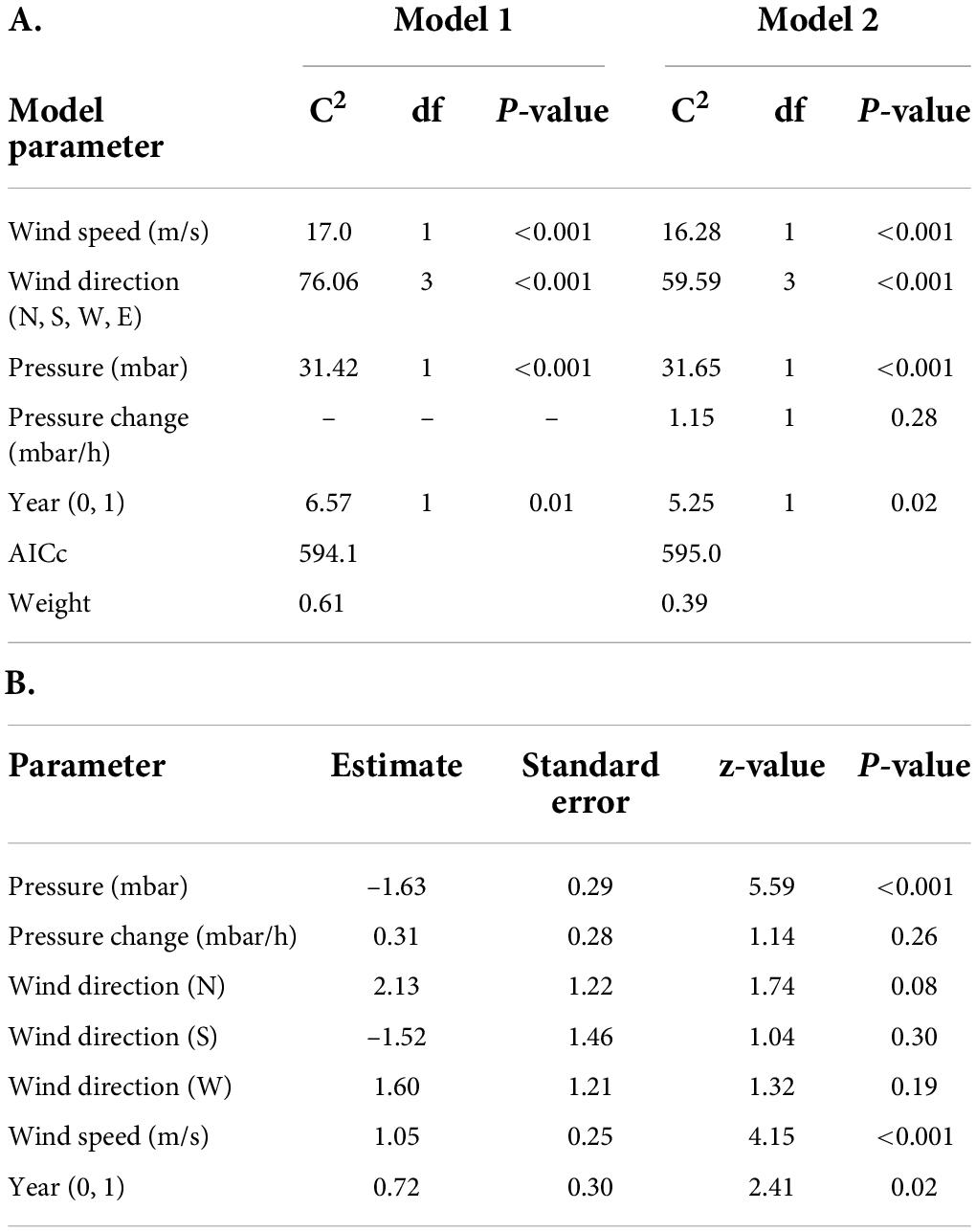
Table 2. (A) Top models and their corresponding parameters as selected by AIC model selection, predicting Semipalmated Sandpiper departures from the Bay of Fundy based on weather conditions. Only models with delta AICc values < 2 are presented. Weight represents the relative likelihood of a model. (B) Standardized model-averaged coefficients showing conditional averages for parameters from the top models. In the model year was coded as 0 for 2013 and 1 for 2014.
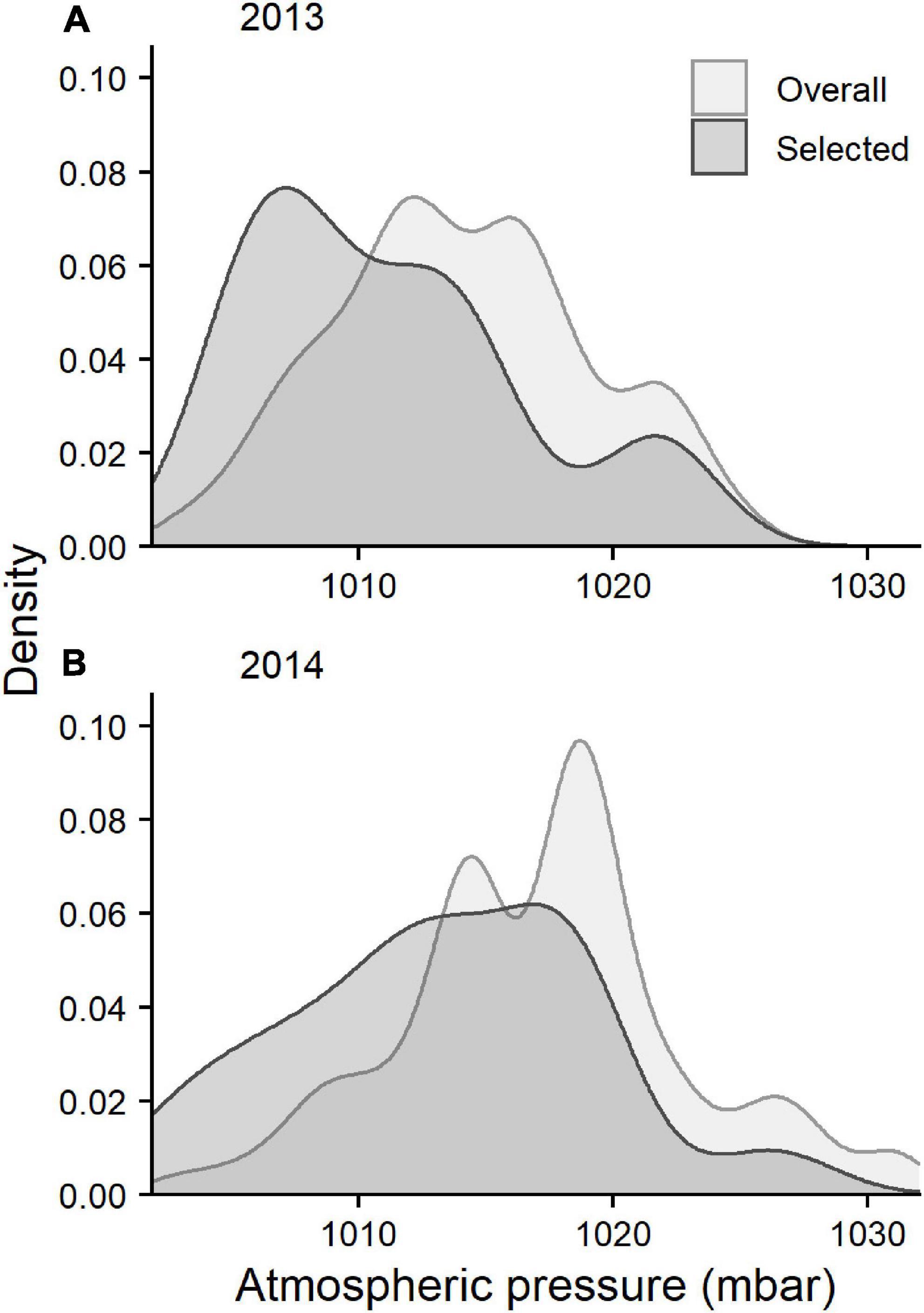
Figure 6. Kernel density plots of atmospheric pressure throughout the Semipalmated Sandpiper departure period (August 22 to September 19) from the Bay of Fundy (“overall”) and during hours when birds departed (“selected”) in (A) 2013 and (B) 2014.
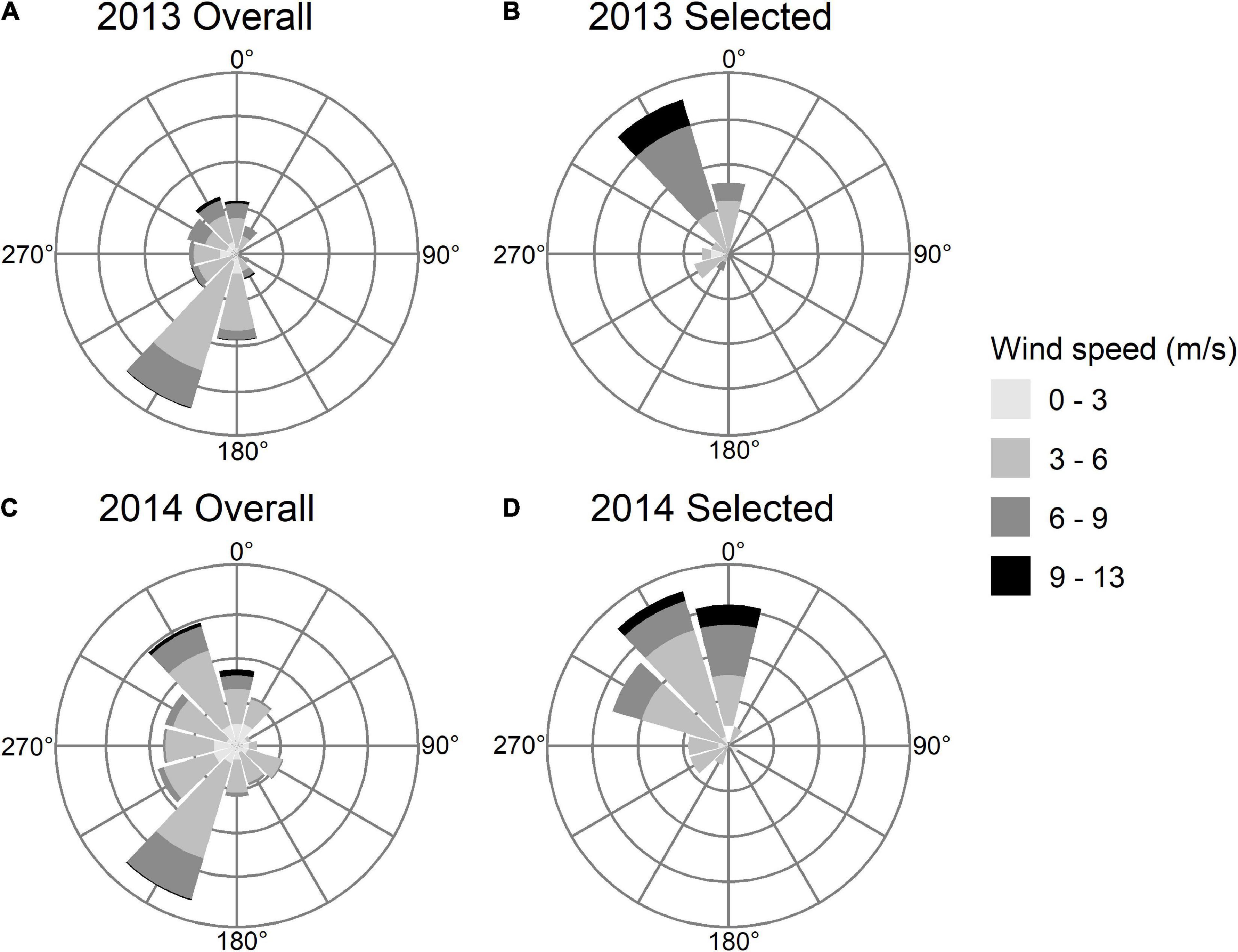
Figure 7. Distribution of wind speed and direction throughout the Semipalmated Sandpiper departure period (August 22 to September 19) from the Bay of Fundy [(A) 2013 Overall and (C) 2014 Overall] and during hours when birds departed [(B) 2013 Selected and (D) 2014 Selected]. Size of the wedge indicates overall frequency of winds and number of birds departing during particular wind conditions in the “Overall” and “Selected” graphs, respectively.
Discussion
Estimating length of stay
Accurate estimates of length of stay are critical in calculating staging population size; a decrease or increase in length of stay can make it appear as though the population is increasing or declining when it is in fact stable (Bishop et al., 2000; Ydenberg et al., 2004). Based on radio-tracking data and using a minimum length of stay approach, we estimated that on average Semipalmated Sandpipers stage in the Bay of Fundy for approximately 21 days during post-breeding migration, though we noted a large range in staging periods in both years (range 8–31 days). There are two sources of error in our radio-telemetry estimate. First, birds were present in the Bay of Fundy for an unknown time before they were captured, generating an underestimate of staging period. We attempted to minimize this by selecting birds that weighed less than 30 g, indicating they had arrived in the region recently. Because this site is used primarily as a staging area where birds make a single long stop on route to South America (i.e., they arrive with little fat), we are confident that this approach is not introducing bias. Heavy birds would have almost certainly been in the region for a substantial time before capture, so tagging them would have artificially reduced the minimum length of stay. Second, there is evidence that stress of capture, handling and marking results in increased length of stay (Warnock and Bishop, 1998; Warnock et al., 2004). The handling effect reported for Western Sandpipers and Dunlin (Calidris alpina) averaged 5–9 days (Warnock and Bishop, 1998; Warnock et al., 2004). However, birds in that study use a short multi-hop strategy. As Semipalmated Sandpipers stage in the Bay of Fundy for much longer periods, they may be better able to absorb the handling lag within their normal staging period and the extension of length of stay may be somewhat less than in the previous studies. We have limited data from Semipalmated Sandpipers captured twice in short succession (within 1–3 days) at Petit Cap, a coastal site on the Northumberland Strait geographically close to the Bay of Fundy (n = 18, unpublished data). Assuming a predicted weight gain rate of 1 g/day [as in Mann et al. (2017) and based on Davidson (1984) and Zwarts et al. (1990)], we calculated the difference between what birds would be predicted to have weighed and what they did weigh on recapture. Birds were on average 1.8 g lighter than predicted (observed weight change –2.8 g to +4.5 g). This minor deficit that birds incur gives us confidence that the handling delay imposed by their capture is not approaching the effects noted by Warnock and Bishop (1998) and Warnock et al. (2004).
Mark-recapture methods to estimate staging duration (Schaub et al., 2001; Pledger et al., 2009) are an alternative to the minimum length of stay approach discussed above that avoid the problem of not knowing when a bird arrived. To assess whether such an approach may be appropriate in our study system, we compared results from the two methods and looked at existing data from other studies in which birds that had known arrival dates were tracked. Our minimum length of stay estimate was substantially lower than the estimated length of stay generated from mark-recapture models. This remained true when we duplicated our analyses using the same birds as the mark-recapture models, indicating the trend is consistent with birds of known departures and is not an artefact of tag loss. This result is consistent with findings from a similar methodological comparison in Howell et al. (2019) and raises questions about the utility of using mark-recapture models for long distance migrants that are using a single staging site at which they are known to arrive light and leave heavy. These models have been shown to increase accuracy for songbirds (Schaub et al., 2001), which are known to use a different migratory strategy (hop vs. jump, per language distinctions in Warnock, 2010). Songbirds often have shorter “stopovers,” unlike the Semipalmated Sandpipers in our study which “stage” at just one or two sites for longer periods of time.
In both our study and in Howell et al. (2019), mark-recapture models appear to overestimate length of stay. This conclusion is supported by other data in the respective regions representing the “true length of stay,” (i.e., no effect of handling stress, and a known arrival and departure date). Limited data exist on SESA using the Bay of Fundy after being tagged outside the region or tracked using geolocators. Those results are far more consistent with our minimum length of stay estimates than the mark-recapture estimates. Brown et al. (2017) recorded staging periods in the Bay of Fundy of 18–31 days for Semipalmated Sandpipers fitted with geolocators in the Arctic (n = 3). Further, birds radiotagged on Coats Island, NU, Canada and in James Bay, ON, Canada between 2014 and 2018 were detected for 7–27 days in the Bay of Fundy (n = 10, Friis, Smith, Neima, Paquet and Hamilton, unpublished data). Finally, our minimum length of stay estimates are closer to estimates from the Gulf of Maine, a nearby staging area for SESA where the estimated staging period was found to be 9–15 days (Holberton et al., 2019).
We suggest that the difference in estimates between the two methods in our study is derived from an overestimation of the seniority parameter in the mark-recapture models, which estimated tagged sandpipers had been in the region between 14 and 22 days prior to capture. Given our prior knowledge that these birds typically arrive lean (20–22 g), it is highly unlikely birds were present 2–3 weeks prior to capture and weighed only a few grams more than their lean mass (tagging weights were between 21 and 33 g). In our mark-recapture models the estimates of φ and γ were very similar, which is unrealistic in this system and likely contributes to the overestimate of length of stay. We suggest that the models may have performed better if we had tagged birds of random weights. Our study was designed to collect data on both length of stay and sandpiper movement within the region (Neima et al., 2020), so we tagged light birds to be able to track them for the majority of their staging period. This did not bias our data toward birds in poor condition because they routinely arrive light and leave heavy, but it did bias the sample to recent arrivals, and the seniority parameter failed to capture that. Given our findings and those of Howell et al. (2019), we suggest that additional work should be done with birds tagged across a wide range of weights to determine whether these models perform well estimating length of stay for long-distance migrants at staging locations. If they do, they would be a valuable option for researchers studying these species, especially when sample size or prior knowledge may be limited, and it may not be possible to target recent arrivals.
Has length of stay changed?
Our radio-tracking estimate of a 21-day length of stay for Semipalmated Sandpipers in the Bay of Fundy is substantially longer than Hicklin’s (1987) 15-day estimate. Birds were handled in both our and Hicklin’s studies, so handling effects did not generate this change. However, the increase could be partially due to the change in method, from colour-marking cohorts to radio-tracking individuals. To assess the potential effects of this change in methodology, we attempted to approximate Hicklin’s method using resightings of flagged individuals. In 2015 and 2016 we conducted a resighting effort that was consistent with Hicklin’s (1987) work [see Neima et al. (2020) for details of methodology], with the exception that all resighted birds had been banded in previous years or elsewhere in the flyway prior to arrival in the Bay of Fundy. Because of this, we anticipated that the estimated length of stay of our flagged birds would not be influenced by a handling effect, unlike Hicklin’s (1987) birds, and consequently would be shorter than Hicklin’s estimated length of stay, assuming true length of stay remained unchanged. Instead, we found that flagged birds had an estimated length of stay of 17–18 days, which is 2–3 days longer than the 15 days reported by Hicklin in the 1970s. If we take into consideration that Hicklin’s marked birds were likely influenced by a handling effect, whereas our resighted birds were not, the difference is probably even greater. Thus, based on an estimated handling delay of ∼2 days (see above), we suggest that length of stay in the Bay of Fundy has increased by at least 4–5 days (27–33%) since the 1970’s.
Regional and temporal variation in length of stay
We found no consistent differences in length of stay between areas within the Bay of Fundy. This suggests that a variety of habitats, in which diets vary (Quinn and Hamilton, 2012; Neima, 2017), can adequately prepare birds for migration (Neima et al., 2020). This is unsurprising given how Semipalmated Sandpipers are highly opportunistic foragers (Skagen and Oman, 1996; Davis and Smith, 2001; Skagen, 2006) and can access high quality alternate prey items (Kuwae et al., 2008; Quinn et al., 2017; Mogle, 2021). In 2014, early arriving birds stayed longer than those caught later in the season. The exception was in the Minas Basin, where time of tagging did not affect length of stay. The overall trend of earlier arrivals staying longer at staging areas is common among Calidrid shorebirds (Lank, 1983; Lyons and Haig, 1995; Warnock and Bishop, 1998; Warnock et al., 2004). In 2014, this may have been amplified by extreme tidal events early in the season that flooded roost sites and caused sustained periods of flight and thus increased energy expenditure for early migrant birds (Mann et al., 2017). This phenomenon may explain why the magnitude of the difference in length of stay between early and late migrants varied among sites in 2014. We observed the greatest differences in locations where high tides were most extreme [i.e., Cobequid Bay, Mann et al. (2017)].
Our results suggest that Semipalmated Sandpipers in the Bay of Fundy, especially early migrants, are not operating on a time-selected migration schedule (Alerstam and Lindström, 1990). This result is consistent with studies that have found that Semipalmated Sandpipers stay at staging sites longer than necessary to acquire adequate fat stores for migration in both autumn and spring (Dunn et al., 1988; Skagen and Knopf, 1994; Lyons and Haig, 1995; Pfister et al., 1998; Roques et al., 2021). Using an estimated rate of mass gain of 1 g/day (Davidson, 1984; Zwarts et al., 1990), we calculated that all birds in our study with known departure dates could have reached weights ≥ 38.7 g, which would have been sufficient to reach South America in a single flight (Hicklin, 1987). Many of these birds stayed long enough to theoretically reach weights much higher than possible for this species. This suggests that many Semipalmated Sandpipers are staying in the Bay of Fundy longer than necessary to gain the required fat stores, contributing to the observed variability in length of stay.
Conditions affecting departure timing
Our departure data clearly indicate that the Bay of Fundy is a launching point for migration across the Atlantic to South America. Almost all birds detected leaving the region crossed mainland Nova Scotia and departed over the ocean, not appearing on receivers on the coast of the United States. This confirms the migration path proposed by previous radar, observational studies and computer simulation (Williams et al., 1977; Richardson, 1979; Lank, 1983) and is consistent with the observations of Holberton et al. (2019) for Semipalmated Sandpipers departing the northern Gulf of Maine. Thus it appears they have a consistent departure strategy.
We found strong evidence that departure timing is correlated with suitable weather conditions, consistent with results from studies of this species in different parts of their range (Anderson et al., 2019; Herbert et al., 2022). Given the high cost of a non-stop flight of up to 60 h, favourable weather conditions may be crucial for successful migration (Lank, 1983; Stoddard et al., 1983; Butler et al., 1997). Our birds left during periods when pressures were low but rising and winds were from the north-west, consistent with the passage of a cold front. Others have noted similar patterns and concluded that these winds would carry migrants south-east out over the Atlantic and down into the eastern trade winds, which would push them back west to South America (Williams et al., 1977; Richardson, 1979; Stoddard et al., 1983). This route could allow shorebirds to maintain a constant heading, relying on tail winds and wind drift with minimal compensation to reach their destination [see Linscott et al. (2022) for definitions of responses to wind flow direction]. Work on passerines suggests that migrants that use a time-minimizing strategy may be less selective of wind conditions than those that are energy-minimizers (McCabe et al., 2018). Given their length of stay, Semipalmated Sandpipers in the Bay of Fundy are probably energy rather than time-minimizers [see also Roques et al. (2021)]. This result is consistent with existing theory and evidence suggesting that southbound migrants favour an energy-minimizing strategy (e.g., Hedenström and Alerstam, 1997; Duijns et al., 2019) and adds more evidence that McCabe et al.’s (2018) conclusion applies to a broad range of bird taxa.
Given the importance of tailwinds during departure, individuals should maintain their energy reserves at high levels so that they may depart as soon as conditions become favourable (Butler et al., 1997). This is consistent with birds spending more time at staging sites than necessary, as we saw in this study, and as observed by Roques et al. (2021). Favourable wind patterns are more common in early autumn than in August. This could explain why earlier migrants tend to have increased lengths of stay. Early migrants may be waiting for favourable wind conditions, while these conditions are available for late migrants as soon as they have sufficient fat stores. While the frequency of tail winds increases throughout the fall, so does the frequency of intense storms, which may represent a significant mortality risk to migrating shorebirds (Williams et al., 1977; Morrison et al., 2012; Watts et al., 2021). This may prevent migrants from lingering in the Bay of Fundy, thus curtailing length of stay for later migrants.
Prey availability and predation pressure may also explain differences in length of stay between early and late migrating sandpipers. Semipalmated Sandpipers have been found to stay longer at high quality sites (Herbert et al., 2022). Density of C. volutator, a historically important prey item for sandpipers in the Bay of Fundy, declines later in the season due to natural mortality and predation by shorebirds (Schneider and Harrington, 1981; Hamilton et al., 2006; Barbeau et al., 2009), although this seasonal pattern seems less strong in other prey items (Gerwing et al., 2015). Studies on Western Sandpipers suggest that sandpipers have reduced their migratory mass and shortened their stopovers as a result of increased Peregrine Falcon presence (Butler et al., 2003; Ydenberg et al., 2004). In Atlantic Canada, Semipalmated Sandpipers have shifted toward using safer habitats as Peregrine Falcon populations have recovered (Hope et al., 2020). However, Peregrine Falcon migration on the Atlantic coast occurs later in September and October (Schmutz et al., 1991; Worcester and Ydenberg, 2008; Buskirk, 2012), well after most Semipalmated Sandpipers have departed for South America. Therefore, it is unlikely that predator migration strongly influences departure decisions, though it may prevent birds from lingering late into fall.
Conclusion
Lengths of stay of Semipalmated Sandpipers staging in the Bay of Fundy were highly variable, although it appears that the average has increased over the last three decades and is now 18–21 days based on a minimum length of stay estimation approach. This has implications for population estimates, which until now have used the historic 15-day staging period (Hicklin, 1987). We found no consistent variation in staging period among regions, suggesting that a variety of diets are all provisioning birds equally for migration, which is encouraging in a conservation context. Earlier arrivals stayed longer than late arrivals, and many birds stayed longer than was necessary to accumulate the necessary fat reserves, likely waiting for favourable wind conditions to assist migration. Favourable conditions were more frequent later in the season, which may have delayed departures of early migrants, whereas late migrants were able to depart as soon as they had adequate energy stores. Constraints toward the end of the migratory period such as increasing frequency and intensity of storms may also limit length of stay in late migrants. Given that overall migration timing has shifted later in the past 30–40 years (Bliss et al., 2019), in future these constraints may render later migrants at a disadvantage.
While we cannot be certain what has caused this increased staging duration, there are multiple possible hypotheses that should be considered in a conservation context. First, a longer stay in this region may signal that birds are arriving in poorer condition from the Arctic. There are numerous examples of prey mismatch or other issues related to climate change that can affect condition of birds prior to migration (Meltofte et al., 2007; McKinnon et al., 2012; Kwon et al., 2019). A comparison of historic and recent banding data could help in assessing whether carryover effects may occur in our system. The prey base in the Bay of Fundy has also changed to some extent, and sandpiper diets appear to have followed [see discussion in Quinn and Hamilton (2012) and Gerwing et al. (2016)]. However, given that birds linger longer than they need to for sufficient weight gain, diet alone is not likely to be responsible for this change. Disturbance at high tide is another possibility. Both extreme high tides (Mann et al., 2017) and recovery of Peregrine Falcons in the region (Dekker et al., 2011) may lead to birds flying more at high tide when they should be roosting. This is an energetic cost that could extend staging in the region. Finally, there may be other pressures selecting for a longer stay. For example, vulnerability to hunting in South America (Watts and Turrin, 2016) may favour birds that delay their arrival in the region. Additional work to assess these possibilities is needed. Another important next step in understanding changes in sandpiper migration through the Bay of Fundy is to investigate survivorship between early and late migrants and among regions, and to re-evaluate population trends in light of the increased length of stay.
Data availability statement
The raw data supporting the conclusions of this article will be made available by the authors, without undue reservation.
Ethics statement
This animal study was reviewed and approved by Mount Allison University Animal Care Committee.
Author contributions
DH, CG-T, and JP conceived the original idea and designed the study. DH, CG-T, JP, and SN contributed to data collection. SN and RL completed the analyses with contributions from DH. SN led the writing with significant contributions from RL, DH, CG-T, and JP. RL and DH led revisions. All authors contributed to the article and approved the submitted version.
Funding
Funding support was provided by Environment and Climate Change Canada, Natural Sciences and Engineering Research Council of Canada Discovery Grant 327321 (DH) and CGSM award (SN), Canada Summer Jobs, New Brunswick Wildlife Trust Fund, New Brunswick Innovation Foundation, and a Career Launcher Internship (Colleges and Institutes Canada).
Acknowledgments
We thank the team behind the Motus Wildlife Tracking System, especially Phil Taylor, John Brzustowski, and Stu MacKenzie for support throughout the project. Many thanks also to the landowners who allowed access to beaches and mudflats and hosted towers, including the Cape Enrage Interpretive Centre, Beaubassin Research Station, Joggins Fossil Institute, Fundy Ocean Research Center for Energy, Burntcoat Head Park, Elmsdale Landscaping, Shirley and Merv Ferguson, Peggy and Blair Hamilton, Roy Bishop, Chester and Donna Sharp, Ginny Lee, Mary Majka, and David Christie. We especially thank the staff at the Nature Conservancy of Canada Johnson’s Mills Shorebird Reserve and Interpretive Centre and The Hopewell Rocks for their invaluable assistance. We appreciate field assistance from Beth MacDonald, Abby White, Claire Mussels, Brennan Obermeyer, Jenna Black, Hilary Mann, Hannah Kienzle, Amy MacDonald, Sydney Bliss, Nicole Tweddle, Sylvain Lemieux, Lauren Jonah, Jeremy Dussault, Shaun Allain, Brittany Dixon, Kirsten Snoek, Karine Duffy, Conor McKibbon-Green, Savannah Leblanc, David Drolet, Erica Geldart, and various visiting shorebird researchers and birders who joined in our banding efforts or provided resighting data. We also thank to Christy Morrissey, Jessica Howell, and Devin De Zwaan who provided modelling support. Finally, we appreciate helpful comments from reviewers and the associate editor that guided our revisions of this manuscript.
Conflict of interest
The authors declare that the research was conducted in the absence of any commercial or financial relationships that could be construed as a potential conflict of interest.
Publisher’s note
All claims expressed in this article are solely those of the authors and do not necessarily represent those of their affiliated organizations, or those of the publisher, the editors and the reviewers. Any product that may be evaluated in this article, or claim that may be made by its manufacturer, is not guaranteed or endorsed by the publisher.
Supplementary material
The Supplementary Material for this article can be found online at: https://www.frontiersin.org/articles/10.3389/fevo.2022.897197/full#supplementary-material
Footnotes
- ^ www.motus-wts.org for complete receiver array.
- ^ www.phidot.org
References
Alerstam, T., and Lindström, Å (1990). “Optimal bird migration: The relative importance of time, energy, and safety,” in Bird migration, ed. P. D. E. Gwinner (Berlin: Springer), 331–351. doi: 10.1007/978-3-642-74542-3_22
Anderson, A. M., Duijns, S., Smith, P. A., Friis, C., and Nol, E. (2019). Migration distance and body condition influence shorebird migration strategies and stopover decisions during southbound migration. Front. Ecol. Evol. 7:251.
Andres, B. A., Gratto-Trevor, C., Hicklin, P., Mizrahi, D., Guy Morrison, R. I., and Smith, P. A. (2012). Status of the semipalmated sandpiper. Waterbirds 35, 146–148. doi: 10.1186/2193-1801-2-567
Barbeau, M. A., Grecian, L. A., Arnold, E. E., Sheahan, D. C., and Hamilton, D. J. (2009). Spatial and temporal variation in the population dynamics of the intertidal amphipod Corophium volutator in the upper bay of fundy. Canada. J. Crustac. Biol. 29, 491–506. doi: 10.1651/08-3067.1
Barton, K. (2020). MuMIn: Multi-model inference. r package version 1.43.17. Available online at: https://CRAN.R-project.org/package=MuMInAD (accessed June 1, 2022).
Behrendt, S. (2022). lm.beta: Add standardized regression coefficients to lm-objects. R package version 1.6-2. Available online at: https://CRAN.R-project.org/package=lm.betaAD (accessed June 1, 2022).
Bishop, M. A., Meyers, P. M., and McNeley, P. F. (2000). A method to estimate migrant shorebird numbers on the Copper River Delta, Alaska. J. Field Ornithol. 71, 627–637. doi: 10.1648/0273-8570-71.4.627
Bliss, S. E., Hamilton, D. J., Gratto-Trevor, C., and Paquet, J. (2019). Are disproportionate losses of eastern breeding Semipalmated Sandpipers (Calidris pusilla) driving declines in the upper Bay of Fundy, Canada? Wilson J. Ornithol. 131, 260–271. doi: 10.1676/17-63
Brown, S., Gratto-Trevor, C., Porter, R., Weiser, E. L., Mizrahi, D., Bentzen, R., et al. (2017). Migratory connectivity of semipalmated sandpipers and implications for conservation. Condor Ornithol. Appl. 119, 207–224. doi: 10.1650/CONDOR-16-55.1
Buskirk, J. V. (2012). Changes in the annual cycle of North American raptors associated with recent shifts in migration timing. Auk 129, 691–698. doi: 10.1525/auk.2012.12061
Butler, R. W., Williams, T. D., Warnock, N., and Bishop, M. A. (1997). Wind assistance: A requirement for migration of shorebirds? Auk 114, 456–466. doi: 10.2307/4089246
Butler, R. W., Ydenberg, R. C., and Lank, D. B. (2003). Wader migration on the changing predator landscape. Wader Study Group Bull. 100, 130–133.
Davidson, N. C. (1984). How valid are flight range estimates for waders? Ringing Migr. 5, 49–64. doi: 10.1080/03078698.1984.9673831
Davis, C. A., and Smith, L. M. (2001). Foraging strategies and niche dynamics of coexisting shorebirds at stopover sites in the southern great plains. Auk 118, 484–495. doi: 10.1093/auk/118.2.484
Dekker, D., Dekker, I., Christie, D., and Ydenberg, R. (2011). Do staging Semipalmated Sandpipers spend the high-tide period in flight over the ocean to avoid falcon attacks along shore? Waterbirds 34, 195–201. doi: 10.1675/063.034.0208
Duijns, S., Anderson, A. M., Aubry, Y., Dey, A., Flemming, S. A., Francis, C. M., et al. (2019). Long-distance migratory shorebirds travel faster towards their breeding grounds, but fly faster post-breeding. Sci. Rep. 9, 1–13. doi: 10.1038/s41598-019-45862-0
Dunn, P. O., May, T. A., McCollough, M. A., and Howe, M. A. (1988). Length of stay and fat content of migrant Semipalmated Sandpipers in Eastern Maine. Condor 90, 824–835. doi: 10.2307/1368839
Environment and Climate Change Canada (2022). Canadian climate normals. Available online at: https://climate.weather.gc.ca/climate_normals/index_e.html, (accessed 9 March, 2022).
Gerwing, T. G., Gerwing, A. M. A., Drolet, D., Barbeau, M. A., and Hamilton, D. J. (2015). Spatiotemporal variation in biotic and abiotic features of eight intertidal mudflats in the upper Bay of Fundy, Canada. Northeast. Nat. 22, 1–44. doi: 10.1656/045.022.m1201
Gerwing, T. G., Kim, J.-H., Hamilton, D. J., Barbeau, M. A., and Addison, J. A. (2016). Diet reconstruction using next-generation sequencing increases the known ecosystem usage by a shorebird. Auk 133, 168–177. doi: 10.1642/AUK-15-176.1
Gordo, O. (2007). Why are bird migration dates shifting? A review of weather and climate effects on avian migratory phenology. Climate Res.S 35, 37–58. doi: 10.3354/cr00713
Hamilton, D. J., Diamond, A. W., and Wells, P. G. (2006). Shorebirds, snails, and the amphipod (Corophium volutator) in the upper Bay of Fundy: Top–down vs. bottom–up factors, and the influence of compensatory interactions on mudflat ecology. Hydrobiologia 1, 285–306. doi: 10.1007/s10750-006-0062-y
Hector, A., Von Felten, S., and Schmid, B. (2010). Analysis of variance with unbalanced data: An update for ecology & evolution. J. Animal Ecol. 79, 308–316. doi: 10.1111/j.1365-2656.2009.01634.x
Hedenström, A., and Alerstam, T. (1997). Optimum fuel loads in migratory birds: Distinguishing between time and energy minimization. J. Theor. Biol. 189, 227–234. doi: 10.1006/jtbi.1997.0505
Herbert, J. A., Mizrahi, D., and Taylor, C. M. (2022). Migration tactics and connectivity of a Nearctic-Neotropical migratory shorebird. J. Animal Ecol. 91, 819–830. doi: 10.1111/1365-2656.13670
Hicklin, P. W., and Smith, P. C. (1979). The diets of five species of migrant shorebirds in the Bay of Fundy. Proc. Nova S. Inst. Sci. 29, 483–488.
Hicklin, P. W., and Smith, P. C. (1984). Selection of foraging sites and invertebrate prey by migrant Semipalmated Sandpipers, Calidris pusilla (Pallas), in Minas Basin, Bay of Fundy. Can. J. Zool.S. 62, 2201–2210. doi: 10.1139/z84-321
Hicklin, P. W., Hounsell, R. G., and Finney, G. H. (1989). Fundy pull trap: A new method of capturing shorebirds (Nuevo Método para la Captura de Playeros (Charadriiformes)). J. Field Ornithol. 60, 94–101.
Hicklin, P., and Gratto-Trevor, C. L. (2010). “Semipalmated Sandpiper (Calidris pusilla),” in The birds of North America, ed. P. G. Rodewald (Ithaca, NY: Cornell Lab of Ornithology). doi: 10.2173/bna.semsan.02
Holberton, R. L., Taylor, P. D., Tudor, L. M., O’Brien, K. M., Mittelhauser, G. H., and Breit, A. (2019). Automated VHF radiotelemetry revealed site-specific differences in fall migration strategies of Semipalmated Sandpipers on stopover in the Gulf of Maine. Front. Ecol. Evol. 7:327. doi: 10.3389/fevo.2019.00327
Holmgren, N., Ellegren, H., and Pettersson, J. (1993). Stopover length, body mass and fuel deposition rate in autumn migrating adult Dunlins Calidris alpina: Evaluating the effects of moulting status and age. Ardea Wageningen 81, 9–20.
Hope, D. D., Lank, D. B., Smith, B. D., and Ydenberg, R. C. (2011). Migration of two calidrid sandpiper species on the predator landscape: How stopover time and hence migration speed vary with geographical proximity to danger. J. Avian Biol. 42, 522–529. doi: 10.1111/j.1600-048X.2011.05347.x
Hope, D. D., Lank, D. B., Smith, B. D., Paquet, J., and Ydenberg, R. C. (2020). Migrant Semipalmated Sandpipers (Calidris pusilla) have over four decades steadily shifted towards safer stopover locations. Front. Ecol. Evol. 8:3. doi: 10.3389/fevo.2020.00003
Howell, J. E., McKellar, A. E., Espie, R. H. M., and Morrissey, C. A. (2019). Spring shorebird migration chronology and stopover duration at an important staging site in the North American Central Flyway. Waterbirds 42, 8–21. doi: 10.1675/063.042.0102
Kennedy, L. V., Morbey, Y. E., Mackenzie, S. A., Taylor, P. D., and Guglielmo, C. G. (2017). A field test of the effects of body composition analysis by quantitative magnetic resonance on songbird stopover behaviour. J. Ornithol. 158, 593–601. doi: 10.1007/s10336-016-1399-2
Kuwae, T., Beninger, P. G., Decottignies, P., Mathot, K. J., Lund, D. R., and Elner, R. W. (2008). Biofilm grazing in a higher vertebrate: The Western Sandpiper. Calidris mauri. Ecology 89, 599–606. doi: 10.1890/07-1442.1
Kwon, E., Weiser, E. L., Lanctot, R. B., Brown, S. C., Gates, H. R., Gilchrist, G., et al. (2019). Geographic variation in the intensity of warming and phenological mismatch between Arctic shorebirds and invertebrates. Ecol. Monogr. 89, 1–20. doi: 10.1002/ecm.1383
Laake, J., and Rexstad, E. (2022). RMark–an alternative approach to building linear models in MARK, 109. http://www.phidot.org/software/mark/docs/book/pdf/app_3.pdf (accessed June 1, 2022).
Langsrud, Ø (2003). ANOVA for unbalanced data: Use Type II instead of Type III sums of squares. Stat. Comput. 13, 163–167. doi: 10.1023/A:1023260610025
Lank, D. B. (1983). Migratory behavior of semipalmated Sandpipers at inland and coastal staging areas (Doctoral of Philosophy). Ithaca, NY: Cornell University.
Li, X., Hou, X., Song, Y., Shan, K., Zhu, S., Yu, X., et al. (2019). Assessing changes of habitat quality for shorebirds in stopover sites: A case study in the Yellow River Delta. China. Wetl. 39, 67–77. doi: 10.1007/s13157-018-1075-9
Linscott, J. A., and Senner, N. R. (2021). Beyond refueling: Investigating the diversity of functions of migratory stopover events. Ornithol. Appl. 123, 1–14.
Linscott, J. A., Navedo, J. G., Clements, S. J., Loghry, J. P., Ruiz, J., Ballard, B. M., et al. (2022). Compensation for wind drift prevails for a shorebird on a long-distance, transoceanic flight. Move. Ecol. 10, 1–16. doi: 10.1186/s40462-022-00310-z
Lyons, J. E., and Haig, S. M. (1995). Fat content and stopover ecology of spring migrant Semipalmated Sandpipers in South Carolina Condor 97, 427–437. doi: 10.2307/1369028
Lyons, J. E., Collazo, J. A., and Guglielmo, C. G. (2008). Plasma metabolites and migration physiology of Semipalmated Sandpipers: Refueling performance at five latitudes. Oecologia 155, 417–427. doi: 10.1007/s00442-007-0921-x
Mann, H., Hamilton, D. J., Paquet, J. M., Gratto-Trevor, C. L., and Neima, S. G. (2017). Effects of extreme tidal events on Semipalmated Sandpiper (Calidris pusilla) migratory stopover in the Bay of Fundy. Canada. Waterbirds 40, 41–49. doi: 10.1675/063.040.0106
McCabe, J. D., Olsen, B. J., Osti, B., and Koons, P. O. (2018). The influence of wind selectivity on migratory behavioral strategies. Behav. Ecol. 29, 160–168. doi: 10.1093/beheco/arx141
McKinnon, L., Picotin, M., Bolduc, E., Juillet, C., and Bêty, J. (2012). Timing of breeding, peak food availability, and effects of mismatch on chick growth in birds nesting in the High Arctic. Can. J. Zool. 90, 961–971. doi: 10.1139/z2012-064
Meltofte, H., Piersma, T., Boyd, H., McCaffery, B., Ganter, B., and Golovnyuk, et al. (2007). Effects of climate variation on the breeding ecology of Arctic shorebirds. – Meddelelser om Grønland Bioscience 59. Copenhagen: Danish Polar Center.
Mizrahi, D. S., Peters, K. A., and Hodgetts, P. A. (2012). Energetic condition of Semipalmated and Least Sandpipers during northbound migration staging periods in Delaware Bay. Waterbirds 35, 135–145. doi: 10.1675/063.035.0113
Mogle, M. J. (2021). Dynamics of intertidal biofilm in relation to Semipalmated Sandpiper (Calidris pusilla) migratory stopover and assessments of benthic invertebrate and biofilm nutritional content in the upper Bay of Fundy, Canada. [M.Sc. thesis]. Sackville, NB: Mount Allison University.
Morrison, R. I. G. (1984). “Migration systems of some New World shorebirds,” in Shorebirds: Migration and foraging behavior, Vol. 6, eds J. Burger and B. L. Olla (New York, NY: Plenum Press), 125–202.
Morrison, R. I. G., Mizrahi, D. S., Ross, R. K., Ottema, O. H., de Pracontal, N., and Narine, A. (2012). Dramatic declines of Semipalmated Sandpipers on their major wintering areas in the Guianas, Northern South America. Waterbirds 35, 120–134. doi: 10.1675/063.035.0112
Myers, J. P. (1983). Conservation of migrating shorebirds: Staging areas, geographic bottlenecks, and regional movements. Am. Birds 37, 23–25.
Myers, J. P., Morrison, R. I. G., Antas, P. Z., Harrington, B. A., Lovejoy, T. E., Sallaberry, M., et al. (1987). Conservation strategy for migrating species. Am. Sci. 75, 19–26. doi: 10.1038/scientificamerican0287-26
Neima, S. G. (2017). Stopover ecology of Semipalmated Sandpipers (Calidris pusilla) during fall migration through the upper Bay of Fundy, Canada. master’s thesis. Sackville, NB: Mount Allison University.
Neima, S., Hamilton, D., Gratto-Trevor, C., and Paquet, J. (2020). Intra-and interannual regional fidelity of Semipalmated Sandpipers (Calidris pusilla) during migratory stopover in the upper Bay of Fundy, Canada. Avian Conserv. Ecol. 5:14. doi: 10.5751/ACE-01561-150114
Niles, L. J., Bart, J., Sitters, H. P., Dey, A. D., Clark, K. E., Atkinson, P., et al. (2009). effects of horseshoe crab harvest in delaware bay on red knots: Are harvest restrictions working? BioScience 59, 153–164. doi: 10.1525/bio.2009.59.2.8
Page, G., and Middleton, A. L. A. (1972). Fat deposition during autumn migration in the Semipalmated Sandpiper. Bird-Banding 43, 85–96. doi: 10.2307/4511852
Peterson, S. L., Rockwell, R. F., Witte, C. R., and Koons, D. N. (2013). The legacy of destructive snow goose foraging on supratidal marsh habitat in the Hudson Bay Lowlands. Arct. Antarctic. Alp. Res. 45, 575–583. doi: 10.1657/1938-4246.45.4.575
Pfister, C., Kasprzyk, M. J., and Harrington, B. A. (1998). Body-fat levels and annual return in migrating Semipalmated Sandpipers. Auk 115, 904–915. doi: 10.2307/4089509
Pledger, S., Efford, M., Pollock, K., Collazo, J., and Lyons, J. (2009). “Stopover duration analysis with departure probability dependent on unknown time since arrival,” in Modeling demographic processes in marked populations, eds D. L. Thomson, E. G. Cooch, and M. J. Conroy (Boston, MA: Springer US), 349–363. doi: 10.1007/978-0-387-78151-8_15
Pradel, R., Schaub, M., Jenni, L., and Lebreton, J.-D. (2005). Migrating birds stop over longer than usually thought: Reply. Ecology 86, 3418–3419. doi: 10.1890/05-1881
Quinn, J. T., and Hamilton, D. J. (2012). Variation in diet of Semipalmated Sandpipers (Calidris pusilla) during stopover in the upper Bay of Fundy, Canada. Cana. J. Zool. 90, 1181–1190. doi: 10.1139/z2012-086
Quinn, J. T., Hamilton, D. J., and Hebert, C. E. (2017). Fatty acid composition and concentration of alternative food of Semipalmated Sandpipers (Calidris pusilla) in the upper Bay of Fundy, Canada. Can. J. Zool. 95, 565–573. doi: 10.1139/cjz-2016-0246
R Core Team (2021). R: A language and environment for statistical computing. R foundation for statistical computing. Vienna: URL.
Richardson, W. J. (1979). Southeastward shorebird migration over Nova Scotia and New Brunswick in autumn: A radar study. Can. J. Zool. 57, 107–124. doi: 10.1139/z79-009
Roques, S., Lank, D. B., Cam, E., and Pradel, R. (2021). More than just refueling: Lengthy stopover and selection of departure weather by sandpipers prior to transoceanic and transcontinental flights. Ibis 163, 519–535. doi: 10.1111/ibi.12891
Rosenberg, K. V., Dokter, A. M., Blancher, P. J., Sauer, J. R., Smith, A. C., Smith, P. A., et al. (2019). Decline of the North American avifauna. Science 366, 120–124. doi: 10.1126/science.aaw1313
Schaub, M., Pradel, R., Jenni, L., and Lebreton, J.-D. (2001). Migrating birds stop over longer than usually thought: An improved capture-recapture analysis. Ecology 82, 852–859. doi: 10.1890/0012-9658(2001)082[0852:MBSOLT]2.0.CO;2
Schmutz, J. K., Fyfe, R. W., Banasch, U., and Armbruster, H. (1991). Routes and timing of migration of falcons banded in Canada. Wilson Bull. 103, 44–58.
Schneider, D. C., and Harrington, B. A. (1981). Timing of shorebird migration in relation to prey depletion. Auk 98, 801–811. doi: 10.1093/auk/98.4.801
Skagen, S. K. (2006). Migration stopovers and the conservation of arctic-breeding calidridine sandpipers. Auk 123, 313–322. doi: 10.1093/auk/123.2.313
Skagen, S. K., and Knopf, F. L. (1994). Residency patterns of migrating sandpipers at a midcontinental stopover. Condor 96, 949–958. doi: 10.2307/1369104
Skagen, S. K., and Oman, H. D. (1996). Dietary flexibility of shorebirds in the Western Hemisphere. Can. Field Nat. 110, 419–444.
Stoddard, P. K., Marsden, J. E., and Williams, T. C. (1983). Computer simulation of autumnal bird migration over the western North Atlantic. Anim. Behav. 31, 173–180. doi: 10.1016/S0003-3472(83)80186-9
Studds, C. E., Kendall, B. E., Murray, N. J., Wilson, H. B., Rogers, D. I., Clemens, R. S., et al. (2017). Rapid population decline in migratory shorebirds relying on the Yellow Sea tidal mudflats as stopover sites. Nat. Commun. 8:14895. doi: 10.1038/ncomms14895
Taylor, P., Crewe, T., Mackenzie, S., Lepage, D., Aubry, Y., Crysler, Z., et al. (2017). The motus wildlife tracking system: A collaborative research network to enhance the understanding of wildlife movement. Avian Conserv. Ecol. 12, 1–11. doi: 10.5751/ACE-00953-120108
Turcotte, Y., Lamarre, J.-F., and Bêty, J. (2013). Staging ecology of Semipalmated Plover (Charadrius semipalmatus) and Semipalmated Sandpiper (Calidris pusilla) juveniles in the St. Lawrence River Estuary during fall migration. Can. J. Zool. 91, 802–809. doi: 10.1139/cjz-2013-0101
Warnock, N. (2010). Stopping vs. staging: The difference between a hop and a jump. J. Avian Biol. 41, 621–626. doi: 10.1111/j.1600-048X.2010.05155.x
Warnock, N., and Bishop, M. A. (1998). Spring stopover ecology of migrant Western Sandpipers. Condor 100, 456–467. doi: 10.2307/1369711
Warnock, N., Takekawa, J. Y., and Bishop, M. A. (2004). Migration and stopover strategies of individual Dunlin along the Pacific coast of North America. Can. J. Zool. 82, 1687–1697. doi: 10.1139/z04-154
Watts, B. D., and Turrin, C. (2016). Assessing hunting policies for migratory shorebirds throughout the Western Hemisphere. Wader Study 123, 6–15. doi: 10.18194/ws.00028
Watts, B. D., Smith, F. M., Hines, C., Duval, L., Hamilton, D. J., Keyes, T., et al. (2021). Whimbrel populations differ in trans-atlantic pathways and cyclone encounters. Sci. Rep. 11:12919. doi: 10.1038/s41598-021-92429-z
Williams, T. C., Williams, J. M., Ireland, L. C., and Teal, J. M. (1977). Autumnal bird migration over the western North Atlantic Ocean. Am. Birds 31, 251–267. doi: 10.1590/1414-431X20155005
Worcester, R., and Ydenberg, R. (2008). Cross-continental patterns in the timing of southward peregrine falcon migration in North America. J. Rap. Res. 42, 13–19. doi: 10.3356/JRR-07-16.1
Ydenberg, R. C., Butler, R. W., Lank, D. B., Smith, B. D., and Ireland, J. (2004). Western Sandpipers have altered migration tactics as peregrine falcon populations have recovered. Proc. R. Soc. Lond. Biol. Sci. 271, 1263–1269. doi: 10.1098/rspb.2004.2713
Keywords: Semipalmated Sandpiper, Calidris pusilla, staging site use, migration, length of stay, radio-telemetry, Motus Wildlife Tracking System
Citation: Neima SG, Linhart RC, Hamilton DJ, Gratto-Trevor CL and Paquet J (2022) Length of stay and departure strategies of Semipalmated Sandpipers (Calidris pusilla) during post-breeding migration in the upper Bay of Fundy, Canada. Front. Ecol. Evol. 10:897197. doi: 10.3389/fevo.2022.897197
Received: 15 March 2022; Accepted: 05 August 2022;
Published: 02 September 2022.
Edited by:
Jaime A. Collazo, North Carolina State University, United StatesReviewed by:
Jose A. Masero, University of Extremadura, SpainNathan R. Senner, University of South Carolina, United States
Copyright © 2022 Neima, Linhart, Hamilton, Gratto-Trevor and Paquet. This is an open-access article distributed under the terms of the Creative Commons Attribution License (CC BY). The use, distribution or reproduction in other forums is permitted, provided the original author(s) and the copyright owner(s) are credited and that the original publication in this journal is cited, in accordance with accepted academic practice. No use, distribution or reproduction is permitted which does not comply with these terms.
*Correspondence: Diana J. Hamilton, ZGhhbWlsdG9AbXRhLmNh