- Department of Ecology, Evolution, and Organismal Biology, Kennesaw State University, Kennesaw, GA, United States
The Trivers–Willard hypothesis states that mothers should adjust their offspring sex ratio according to their own condition and the environment they face during breeding. Past tests of this hypothesis have focused on how natural variation in weather, food availability, or predation pressure shapes sex allocation trade-offs. However, anthropogenic activities, such as urbanization, can alter all of the above characteristics presenting animals with novel challenges in optimizing their brood sex ratio. Previous research has examined how urban living influences individual body condition in several bird taxa, but few have explored subsequent impacts on secondary offspring sex ratio. One likely mediator of the link between environmental conditions, parental condition, and sex ratios is corticosterone (CORT), the primary glucocorticoid in birds. Research on CORT’s influence on sex ratios has focused solely on maternal CORT. However, for species with biparental care, paternal CORT or the similarity of maternal and paternal phenotypes may also help ensure that offspring demand matches parental care quality. To test these hypotheses, we explore offspring secondary sex ratios in European starlings (Sturnus vulgaris). We did not find an effect of site or parental body condition on the production of the more costly sex (males). Instead, we found preliminary evidence suggesting that the similarity of maternal and paternal CORT levels within a breeding pair may increase the likelihood of successfully fledging sons. Maternal and paternal CORT were not significant predictors of secondary sex ratio, suggesting that parental similarity, rather than parental CORT alone, could play a role in shaping secondary offspring sex ratios, but additional work is needed to support this pattern. Starlings are considered an urban-adapted species, making them a compelling model for future studies of the relationship between urbanization, parental body condition, and sex ratios.
Introduction
The study of sex allocation theory is a complex field that has evolved as our understanding of evolutionary ecology has improved. Fisher’s equal allocation theory (Fisher, 1930) suggests that parents should invest equally in the sexes to gain the largest fitness advantage; it assumes males and females contribute equally to the next generation because every offspring has one mother and one father. However, if one sex is more costly than the other to produce or raise, the observed population ratio can shift from equal production to equal investment in both sexes. Therefore, parents should invest more in the less costly sex to ensure that overall resource investment is equal between the sexes (Fisher, 1930). Trivers and Willard (1973) expanded this idea after noting that mothers can adjust the sex of their offspring in response to environmental conditions to improve their fitness through increased survival and future reproduction. The costs and benefits of producing each of the sexes can fluctuate under different environmental conditions, leading parents to favor one sex over the other depending on their current environment and intrinsic condition. When mothers are in poor condition (e.g., with poor body condition or facing low food availability), they should invest in the less costly sex, and when they are in better condition, they should invest in the more costly sex (Trivers and Willard, 1973). This change in investment can arise through changes in parental care behavior (Nager et al., 1999; Rubenstein, 2007). This adaptive sex allocation—or bet-hedging—could lead to biased offspring sex ratios within a brood based on the conditions at the time of breeding. However, these biases should cancel out at the population level and through time (Trivers and Willard, 1973).
Many different environmental conditions can affect individual condition—including weather, food availability and quality, disturbance frequency, or predator density—and shape offspring sex ratios. For example, common grackles (Quiscalus quiscula) bias the sex ratio of their broods toward the less expensive sex early in the season when they experience harsher weather and reduced food availability (Howe, 1977). Lesser spotted eagles (Aquila pomarina) favor the more expensive sex in years with increased prey availability and rainfall (Väli, 2012). In addition to the environmental variation that organisms have experienced throughout their evolutionary histories, recent anthropogenic change has drastically altered the availability of resources for organisms worldwide. Increased human population density on nearly all continents has led to altered environmental conditions in urban environments compared to rural ones that organisms face (Seress and Liker, 2015). Urban areas are higher in chemical, noise, and light pollution (Shanahan et al., 2013; Seress and Liker, 2015), which can negatively affect the health and behavior of organisms (Pickett et al., 2011; Gil and Brumm, 2013; Deviche and Davies, 2014; Visser and Spoelstra, 2014; Seress and Liker, 2015). Urban environments may also have altered meteorological conditions—including increased precipitation and higher temperatures through the urban heat island effect (Berry, 2008; Pickett et al., 2011). In some avian species, the urban heat island effect is linked with increased overwinter survival and improved post-winter body condition (Seress and Liker, 2015). Urban environments may also have altered food availability through a decline in insect prey (Boyes et al., 2021) and supplemental seed and suet at feeders.
The effects of urbanization on animal condition are complex; for example, birds consuming human foods and refuse may have nutritionally poorer diets (Ottoni et al., 2009). In contrast, feeders can improve the nutritional condition of wintering birds and increase adult body mass and survival (Amrhein, 2013), but they also increase the spread of infectious diseases and reduce nestling body mass and survival (Ottoni et al., 2009; Seress and Liker, 2015). The impact of urbanization on parental body condition has been inconsistent. While some studies find a positive relationship between urbanization and parental body mass (Auman et al., 2008), others find a negative impact of city living on body mass (Liker et al., 2008; Strubbe et al., 2020) or no impact of urban life on adult body condition at all (Bókony et al., 2012; Foltz et al., 2015; Meillère et al., 2015). Overall, the impact of urbanization on wildlife is context-dependent but often acts through altered behavior and physiological changes to affect an individual’s health and condition (Murray et al., 2019).
Urban-adapted avian species have emerged as common and excellent models to understand the impacts of urbanization on adult condition and possible impacts on offspring sex ratios. It is relatively easy to track their breeding activity and success, and they cover a wide range of habitats. Female birds are the heterogametic sex and can manipulate the primary or embryonic sex ratio (Navara, 2013). Furthermore, birds represent a wide variety of mating systems, levels of parental care, and—as they are oviparous—provide opportunities to study if and when sex ratio biases occur and how the timing of changes in parental body condition or environmental predictability will affect secondary or fledging sex ratios (Love and Williams, 2008).
A few studies have explored sex ratios in urbanized areas. Rejt et al. (2005) found that the egg or primary sex ratio of urban common kestrels (Falco tinnunculus) did not differ from the expected 50:50 ratio and that chick mortality did not significantly affect the secondary sex ratio. However, kestrels from more rural areas produced broods that were significantly male-biased compared to the broods of their more urban counterparts (Rejt et al., 2005). Other studies have found female-biased sex ratios in urban habitats but no bias in rural habitats and no effect of parental body condition (Bonderud et al., 2017). However, the relationship remains equivocal as a more recent study by Ágh et al. (2020) found that the brood sex ratio of great tits (Parus major) did not differ between urban and rural forest habitats.
Glucocorticoids are a possible link between environmental conditions, an animal’s body condition, and offspring sex ratios. In birds, corticosterone (CORT) is the primary glucocorticoid. One of this hormone’s primary functions is metabolic regulation. Baseline CORT maintains homeostasis, links an organism’s external and internal environments, manages energy allocation, and mediates trade-offs between investment in reproduction and survival (Sapolsky et al., 2000; Landys et al., 2006; Patterson et al., 2014). If urban environments are more energetically challenging to inhabit and lead to lower body condition, it is possible that baseline CORT concentrations may be modified to assist in coping with these demands. A meta-analysis by Dantzer et al. (2014) found that glucocorticoids increased with human disturbance across taxa. However, the relationships and interactions between urbanization, CORT, and body condition vary widely in avian species. A recent meta-analysis suggests that the relationship between CORT and urbanization in birds and reptiles is inconsistent and may depend on life-history stage and how tolerant species are to urban conditions (Injaian et al., 2020). As a result, the links between urbanization, CORT, parental body condition, and offspring sex ratios in birds remain unclear (Rejt et al., 2005; Bókony et al., 2012; Bonier, 2012; Foltz et al., 2015; Bonderud et al., 2017; Ágh et al., 2020; Iglesias-Carrasco et al., 2020; Strubbe et al., 2020).
In birds, maternal body condition and CORT can affect both the primary—the one a mother lays—and secondary sex ratio—the ratio of males to females in the offspring that survive to fledge. Mothers are thought to bias their primary sex ratio through CORT and other steroid hormones deposited into the yolk and albumen of the egg (Schwabl, 1993; Engelhardt and Groothuis, 2005; Downing and Bryden, 2008; Navara and Pinson, 2010). Maternal CORT can also significantly impact embryo and nestling development and survival after laying to alter the secondary sex ratio and match offspring needs with maternal care quality (Hayward and Wingfield, 2004; Rubolini et al., 2005; Saino et al., 2005; Schoech et al., 2011; Bowers et al., 2016; Strange et al., 2016; Tilgar et al., 2016; Parolini et al., 2019). Elevated maternal CORT can cause primary sex ratio biases within a season (Love et al., 2005; Bonier et al., 2007), but the relationship between secondary sex ratios and maternal CORT is more complicated (Riechert et al., 2013; Henderson et al., 2014). Elevated CORT has been related to female-biased secondary sex ratios and to smaller sons with reduced growth rates and immune response in European starlings (Sturnus vulgaris), where males are the slightly larger and more costly sex (Love et al., 2005; Love and Williams, 2008). It seems that if mothers have elevated CORT while laying, it can result in a brood with one sex that is predisposed to a higher and more rapid rate of mortality through physiological or behavioral changes. This sex-biased mortality leads to a sex ratio that better matches the mother’s ability to invest in her offspring and improves her fitness by allowing her to preserve resources and energy for her own survival (Love and Williams, 2008). Despite the role that biased secondary sex ratios may play in improving a mother’s future fitness, we understand less about how maternal body condition and CORT shape adaptive changes in the secondary sex ratios relative to primary sex ratios.
For species that show biparental care, maternal body condition or CORT is not the only factor likely to influence differential offspring mortality and the secondary sex ratio; paternal body condition too may play a role in determining sex ratios. However, few studies have considered paternal CORT’s effect on nestling care and chick quality, and none have evaluated the impact on secondary sex ratios. As a result, the relationship between elevated baseline CORT and male provisioning is unclear (Lynn, 2016). In house sparrows (Passer domesticus), there is a positive correlation between paternal baseline CORT during breeding and the number of offspring fledged. Also, a negative correlation exists between stress-induced CORT and nestling provisioning in fathers (Ouyang et al., 2011). A recent study on common terns supported a similar relationship: males with moderately increased baseline CORT were more active, demonstrated higher foraging effort, and increased nestling attendance (Riechert and Becker, 2017). They also found that more parental attendance during nestling provisioning led to increased fledging success (Riechert and Becker, 2017). Nevertheless, we know little about the role of paternal body condition or CORT in shaping secondary sex ratios.
An additional and little-researched relationship we should consider in species with biparental care is how the similarity of maternal and paternal body condition and CORT may impact reproductive success. We are only aware of one study on this topic (Ouyang et al., 2014) that showed that similarity in baseline CORT in pairs of great tits increased their breeding success and was related to pair bond longevity. Previous research in this system has demonstrated that baseline CORT levels are positively related to parental care and effort (Ouyang et al., 2013). As a result, pairs with similarly high or low CORT levels should have similar levels of investment. Ouyang et al. (2014) also found that the difference in baseline CORT was positively related to the difference in feeding rate. This finding supports the possibility that parents with similar levels of investment may be able to coordinate their care better and reduce sexual conflict between the parents (Baldan and Van Loon, 2022). This increased coordination should lead to increased synchronicity—regardless of whether CORT levels are similarly high or low—and higher provisioning rates (Bebbington and Hatchwell, 2016), thus increasing fledging success. Pairs also became more similar over time, and more similar pairs, whether with high or low CORT levels, were also more likely to stay together during and after the breeding season (Ouyang et al., 2014). Researchers have also related pair bond longevity to increased hatching and fledging success (Sánchez-Macouzet et al., 2014). However, the directionality and nature of the relationship between hormonal similarity, pair bond longevity, and reproductive success are unclear. The analysis of similarity is relatively novel and has previously been measured as a continuous index, but there may also be a threshold of similarity that parents must maintain to avoid brood reduction through nestling mortality. Nevertheless, it seems that parental similarity in CORT may affect nestling care and survival; thus, it could also affect the secondary sex ratio; but we are unsure of the effect similarity in parental body condition may have on offspring survival. If CORT is related to body condition, it may follow a similar pattern.
Here, we explore how maternal body condition and CORT, paternal body condition and CORT, and similarity among parents in body condition and CORT shape secondary sex ratios in an urban-adapted bird—the European starling. This species shows biparental care, where both mothers and fathers will brood and provision their young. In addition, this species shows size dimorphism where males are larger than females (Cabe, 2020), so sons may be the costlier sex to raise. Despite this cost, sons also have higher reproductive potential (i.e., greater variance in fitness) than females—as they can have multiple female mates or extra-pair paternity—making high-quality sons a preferred investment to increase parental fitness (Smith and Von Schantz, 1993). We would thus expect broods with a secondary sex ratio that is female-biased when parents are in poor body condition. Otherwise, the ratio should be unbiased or male-biased. We seek to address the following questions with our work: (1) Do starlings vary their secondary sex ratio between an urban site and a rural site? (2) Do maternal or paternal body condition and/or CORT levels shape differences in the secondary sex ratio of broods? (3) Is the similarity of paternal and maternal body condition and/or CORT reflected in the sex ratio of the brood? Due to the changes in parental condition associated with urban living, we predict that urban parents would fledge a higher proportion of offspring that are daughters than rural ones. We also predict that there would be a positive correlation between maternal or paternal CORT and the proportion of offspring that are daughters and a negative correlation with maternal or paternal body condition regardless of habitat. Finally, we expect that pairs that are more similar in their body condition and/or CORT would fledge a lower proportion of offspring that are daughters. By integrating physiological mechanisms with ultimate changes in brood sex ratios, we hope to add to our understanding of the impacts of urbanization on avian species and learn how this urban adapter flourishes under anthropogenic conditions.
Materials and methods
Study system
European starlings are an urban-adapted species commonly found across a gradient of urbanized and rural areas (Blair, 1996). They are secondary cavity nesters and readily breed in nest boxes, traffic lights, stadium lights, and tree cavities (Cabe, 2020), making them an excellent study species for this work. We monitored breeding starlings at two study sites, one in an urban habitat and one in a rural habitat. The urban site is a small farm located in the midst of a metro-Atlanta suburb with over 950 people per square kilometer (Acworth, GA, United States). The distance to the nearest restaurant is <1 km, and the distance to the nearest highway is < 0.25 km (i.e., within the home range of a starling). Paved and built structures surround it, with some vegetation throughout, and the site experiences relatively high human disturbance. The rural site is located at a farm surrounded by fields and vegetation, with a few houses and roads dispersed throughout (Taylorsville, GA, United States). The population density at the rural site is below 60 people per square kilometer, the distance to the nearest restaurant is > 16 km, and the distance to the nearest highway is > 3 km. The sites make a strong comparison because both are farm properties, but they differ primarily in the degree of urbanization within the breeding home range of a starling.
In addition to the qualitative differences in the study sites described above, the level of urbanization at each site was quantified using a semi-automated imaging processing software called ‘UrbanizationScore’ based on the methods of Liker et al. (2008). Briefly, the software downloads a satellite image of a 1 km2 area around a given geographical coordinate. It then scores the proportion of vegetation, buildings, and paved surfaces within each 100 m2 cell of the image. Next, these scores are used to calculate the landscape-cover variables. The variables are then combined using principal component analysis to generate an “urbanization score” for each study site. Finally, the sites are then ranked along a relative urban to rural gradient according to their score such that lower values are less urbanized (Czúni et al., 2012; Seress et al., 2014; Lipovits et al., 2015). The software indicated a difference in the degree of urbanization between the sites that supports our qualitative impressions (i.e., a lower score for our rural site). The satellite images used to calculate the index and the resulting PCA scores are available as Supplementary Figures 1, 2.
Field sampling
We monitored approximately 40 nest boxes mounted on posts at each site every few days until hatching during the 2020 and 2021 breeding seasons (March to June). When nestlings were between 4 and 16 days old (mean age at capture ± SE = 9.0 ± 0.6 days), we trapped both parents to collect a blood sample for hormone analyses (average time between the capture of mother and father ± SE = 2.7 ± 1.0 days). To catch the parents, we set up a Van Ert trap at the nest box entrance and waited for the parent to return and spring the trap by entering the nest box. We monitored nest boxes closely to rapidly remove the parent from the nest box upon capture. We collected a baseline blood sample in under 3 min of disturbance (mean ± SE = 157 ± 5.3 s), weighed birds with a Pesola scale, and measured their tarsus length. Body condition scores were calculated as the residuals of a linear regression between tarsus length and body mass, where higher values indicate better body condition (Ardia, 2005). Once sampling was complete, we fit each individual with a uniquely numbered USGS aluminum band. When the chicks reached 16–17 days old, they were also fitted with a permanent USGS aluminum band, and we collected a blood sample from the nestlings for molecular sexing. We continued to monitor the nests after sampling until all offspring had fledged; fledging can be differentiated from predation via disturbance to the nest caused by most predators.
All blood samples were collected by puncturing the brachial vein using a 25G needle. Whole blood was collected in heparinized capillary tubes and then transferred to a 1.5 ml Eppendorf microcentrifuge tube. A small amount of whole blood was transferred to a Whatman™ FTA™ card (Cytiva, Marlborough, MA, United States) and stored at room temperature for later genetic analyses. The remaining whole blood was centrifuged for 5 min, and the plasma was kept and transported on ice until it could be stored at –80°C.
Hormone assay
We measured baseline CORT concentration using a commercially available enzyme immunoassay (EIA) kit according to the manufacturer’s protocol (Arbor Assays DetectX Corticosterone Kit). This protocol was validated for our samples, where serial dilutions (1:25, 1:50, 1:100, and 1:200) of pooled plasma yielded similar hormone concentrations (mean 4.3% difference in CORT across dilutions). We selected a 1:25 dilution to ensure that baseline samples with low corticosterone concentrations still fell within the range of the standard curve. Briefly, plasma samples were thawed before combining 10 μl of plasma and 10 μl of dissociation reagent and incubating at room temperature for 5 min. Then we diluted the samples 1:25 in assay buffer. We included a standard curve (78.125–10,000 pg ml–1) and two blank controls on each plate. Next, 50 μl of each sample and standard were added to the wells of the plate. Then 25 μl of Conjugate and 25 μl of Antibody solution were added to every well. The plate was incubated at room temperature for 1 h while being shaken at 500 RPM. Next, each plate was washed, 100 μl of TMB substrate was added to all wells, then the plates were incubated for another 30 min at room temperature. Finally, 50 μl of stop solution was added to all wells, and the absorbance was analyzed at 450 nm with a plate reader (BioTek ELX808). Circulating CORT concentrations were calculated from the absorbance relative to the standard curve and expressed in ng ml–1. We ran samples in duplicate and within six months of collection. The intra-assay coefficient of variation (from duplicate samples) was 4.56%, and the inter-assay coefficient of variation (from a pooled sample with an aliquot run on every plate) was 10.3%.
Molecular sexing
To determine the sex of the fledged nestlings, we took a 1.2 mm punch from each FTA card. DNA was extracted from the punches using the DNEasy Blood & Tissue Kit (QIAGEN, Hilden, Germany) following a slightly modified protocol (Joint Experimental Molecular Unit, 2013). Briefly, the punch was placed in 180 μl of Buffer ATL and then incubated for 10 min at 94°C. Then 20 μl of proteinase K was added, and the solution was incubated for 1 h at 56°C. The remaining steps were completed according to the manufacturer’s protocol. Extracted DNA was stored at –20°C until ready for analysis using polymerase chain reaction (PCR). Primers used for PCR were P2/P8, which target the CHD gene (and its homolog) located on Z and W sex chromosomes and are widely used for genetic assays of sex in birds (Griffiths et al., 1998). PCR was carried out in a total volume of 25 μl using the KAPA HiFi HotStart PCR kit (KAPA Biosystems, Cape Town, South Africa). The thermal cycle profile for the PCR was initial denaturation at 95°C for 3 min, then 35 cycles of 98°C for 20 s, 61°C for 15 s, and 72°C for 15 s, followed by a final extension at 72°C for 5 min. PCR products were separated by electrophoresis for 60–75 min at 75 V in a 2% agarose gel stained with Midori Green Direct DNA stain. Because these birds have a Z/W chromosomal sex determination, two bands indicate a female, and one indicates a male.
Statistical analyses
During the study, we monitored 36 nests that fledged their young and for which we could calculate the secondary sex ratio of surviving nestlings (rural n = 32, urban n = 4; 2020 n = 13, 2021 n = 23). For each nest, we calculated the proportion of the fledged offspring that were daughters (proportion of daughters), such that a nest that fledged only daughters had a value of 1 and a nest of only sons had a value of 0. Nests that fledged no offspring due to predation, abandonment, or other factors were excluded from the dataset. Reproductive success (mean ± SE) at our rural site was 0.78 ± 0.04, and at our urban site was 1.0 ± 0.0, calculated as the likelihood of fledging at least one of the hatchlings in the nest (Two sample Z-test, z = 1.36, p = 0.17). The success of a nesting attempt (any nest with at least one egg laid would be an attempt) is lower, however, as nesting failure can also happen during the egg phase. If we include all nesting attempts observed, we found that the rural nest survival rate (mean ± SE) from egg to fledging was 0.54 ± 0.06, which was significantly higher than the survival rate for the urban site, which was 0.33 ± 0.17 (Two sample Z-test, z = 5.04, p = 0.03). Our study only considered fledged offspring, those that left the nest alive after day 17, as they are the most ecologically relevant and represent the parents’ fitness through possible contributions to future generations. We are not able to present any data on sex specific-mortality or the primary sex ratio as starling parents are very efficient at removing unhatched eggs and deceased nestlings from their nests. As a result, even by checking nests daily, we cannot determine the sex of every embryo or chick and cannot accurately determine the primary or laying sex ratio.
First, we used a generalized linear mixed model (GLMM) with a binomial error structure and logit link function to determine if the site impacts the proportion of daughters. The response variable was the proportion of fledglings that were daughters, and the fixed effects were site, the number of fledged offspring, and the nesting attempt number (as starlings can raise two broods in 1 year, and parental quality is related to nesting attempt; Verboven and Verhulst, 1996; Hoffmann et al., 2015). We also included a random intercept for nest ID composed of the year and nest box number to account for the non-independence of multiple breeding attempts by the same pair as starlings typically renest in the same box within a season. However, it is important to note that parental identity did not significantly affect our other analyses, so any uncertainty about parentage most likely did not affect model outcomes. To test for population-level deviation from the expected 1:1 ratio, we used a binomial test as well as estimated marginal means using the R package emmeans version 1.7.4-1 (Lenth, 2022). All models used the total number of fledged offspring for the weight parameter.
Second, we used additional GLMMs with a binomial error structure, logit link function, and random intercept to determine if maternal or paternal CORT or body condition influenced the proportion of daughters. These analyses were completed with data from 2021 only (maternal n = 22; paternal n = 13) as we were not able to capture adults in 2020 due to COVID-19 field restrictions. We built two separate models—one for mothers and one for fathers—with the same fixed and random effects: fixed effects included the parental baseline CORT, parental body condition, site, and the number of fledged offspring. Individual ID was included as a random effect to account for multiple attempts by the same adult. Neither baseline CORT concentration nor body condition score were correlated with capture date or time (Spearman’s rank correlations, p > 0.05 in all tests); thus, these were not included in our models.
Finally, to determine the relationship between the similarity of parental CORT and body condition on secondary sex ratios, we used a generalized linear model (GLM) with a binomial error structure and logit link. Given that similarity is a relatively novel measure and we are unsure how variation in this index may influence reproductive success, we calculated similarity for breeding pairs in two ways. First, we calculated a continuous similarity index for CORT concentration and body condition as the absolute value of the difference between the maternal and paternal values based on the analysis used by Ouyang et al. (2014). Lower similarity index values indicate more similar parents regardless of whether the mother or father was in better body condition. In addition to this continuous similarity index, we also calculated a simplified similarity index by classifying each pair as having high similarity or low similarity in relation to mean similarity in our study (mean ± SE similarity in CORT = 5.96 ± 2.05, high CORT similarity n = 9, low CORT similarity n = 4; mean ± SE similarity in body condition = 7.57 ± 1.60, high body condition similarity n = 8, low body condition similarity n = 5)—for this simplified index, the values below the mean were classified as high similarity and vice versa. This analysis is founded on the idea that the relationship between similarity and offspring sex ratios may not be continuous. There may be some threshold level of similarity or matching required for parents to maintain a sufficient level of parental care. Parents may not experience offspring mortality—and thus biased sex ratios—until they pass this threshold and become too dissimilar. Using the mean to create our categories allows us to identify the most dissimilar parents relative to the rest of the sample. Finally, this comparative analysis provides additional insight since we do not have a reference or scale on which to measure what high or low similarity truly is from the organism’s perspective.
We used two GLMs—one for each version of the similarity indexes described—to explore whether parental similarity shaped secondary sex ratios. The fixed effects in this model were the similarity of baseline CORT, the similarity of body condition, site, and the number of offspring fledged. We note that in the similarity analyses, there were two instances where one parent fledged two broods and thus was repeatedly included in the dataset. Because our sample size was too small to fit this model with a random effect of parent ID with only two instances of repeated samples, we did not include a random effect in the GLM. However, we note that the parent renested with a different individual both times. Our analyses’ qualitative results and conclusions remained unchanged when we systematically excluded one repeated observation from the model. Thus, we do not believe that pseudo-replication is an issue biasing our results. All data analysis was completed in the R environment version 4.1.1 (R Core Team, 2021), and the mixed-effects models were created using the package lme4 version 1.1.27.1 (Bates et al., 2015). Model fit was checked using the package DHARMa version 0.4.5 (Hartig, 2022), and no issues were detected based on the diagnostic plots.
Results
We compared mean nestling body mass within each site and found that, as expected, males do tend to be larger than females at our urban site (mean ± SE for urban males = 75.27 ± 1.62 g, urban females = 62.29 ± 3.10 g. T-test comparing urban male and female mass; t10.27 = –3.71, p < 0.01). Interestingly, while males also tended to weigh more than females at the rural site, we did not find a significant difference in body mass between the sexes (rural males = 73.13 ± 1.17 g, rural females = 70.53 ± 0.99 g. T-test comparing rural male and female mass; t86.48 = –1.70, p = 0.09).
When comparing the proportion of fledged offspring that are daughters between the urban and rural sites, we found no significant effect of site on the secondary sex ratio of starling broods (Figure 1 and Table 1). Additionally, the population level sex ratio did not differ from 1:1 at either site (Exact binomial test; rural proportion of daughters = 0.56, p = 0.27; urban proportion of daughters = 0.57, p = 0.79) and the confidence intervals of the estimated marginal means overlapped with 0.5 (rural EMM ± SE = 0.53 ± 0.06, p = 0.61; urban EMM ± SE = 0.52 ± 0.15, p = 0.89). Nestling sex ratios did not differ between first and second broods or with the number of fledged offspring (Table 1).
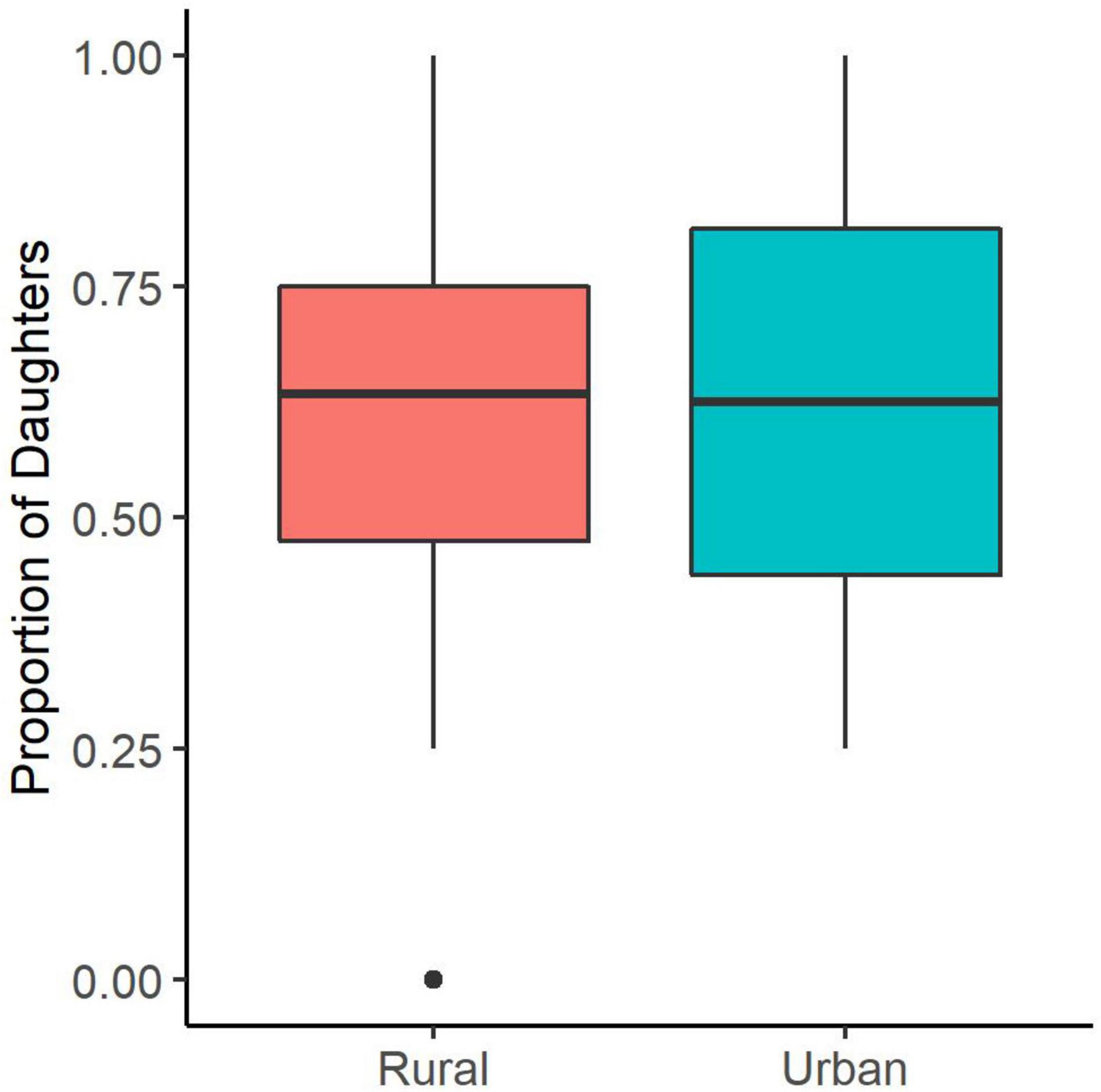
Figure 1. No difference in the proportion of daughters at the urban and rural sites. Mid-line represents the median, boxes are the first and third quartiles, whiskers show minimum and maximum values, and closed circles are outliers.
In our second and third models, neither maternal nor paternal CORT concentration or body condition significantly influenced the secondary sex ratio (Figure 2 and Table 2). We also found that the site and the number of young fledged did not significantly affect secondary sex ratios for either parent (Table 2). Finally, individual parental CORT concentrations were not correlated with body condition scores.
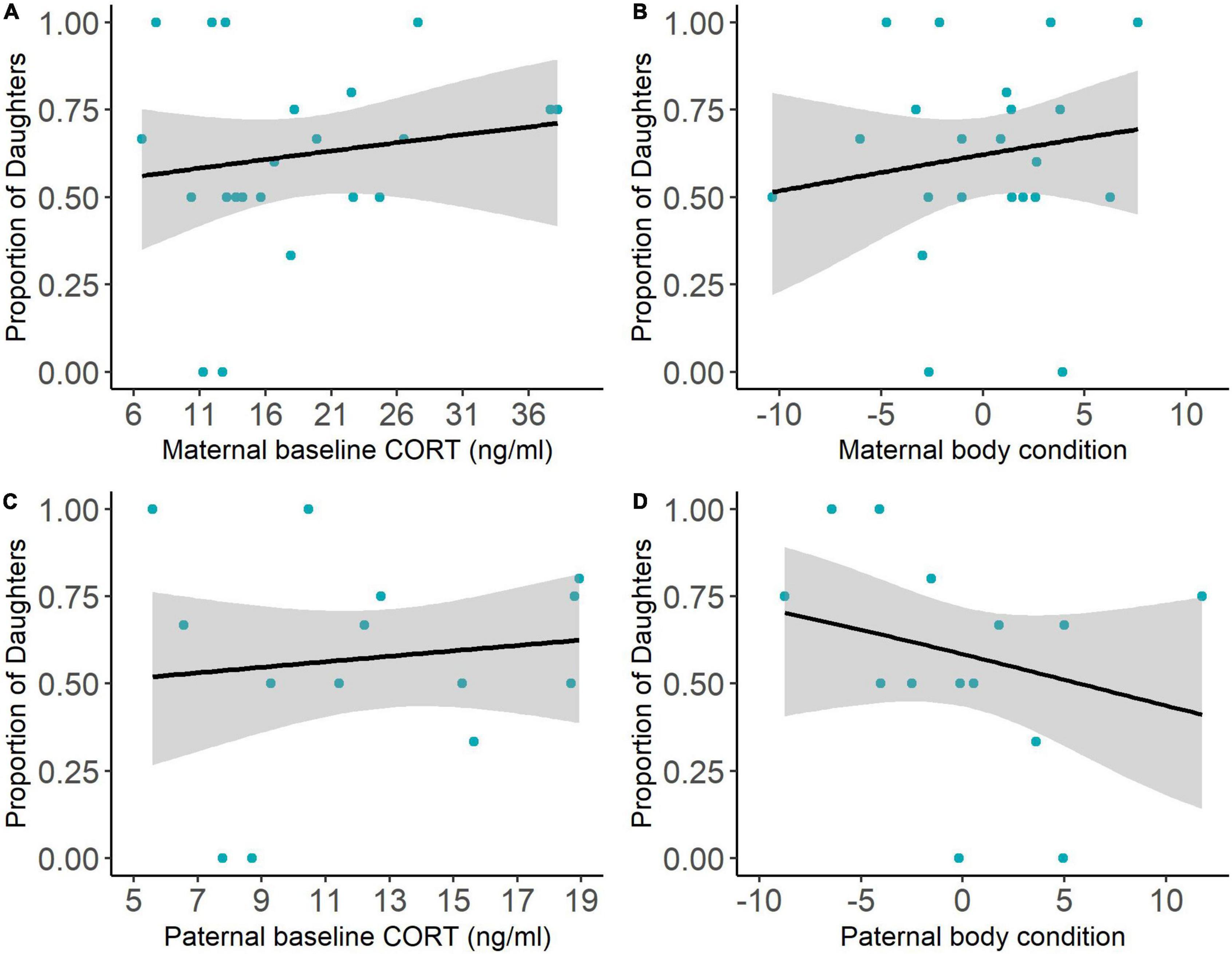
Figure 2. We found no relationship between parental condition and the proportion of daughters. Maternal baseline CORT concentration (A), maternal body condition (B), paternal baseline CORT (C), and paternal body condition (D) were all unrelated to the proportion of daughters. Gray areas around the line represent the 95% confidence interval.
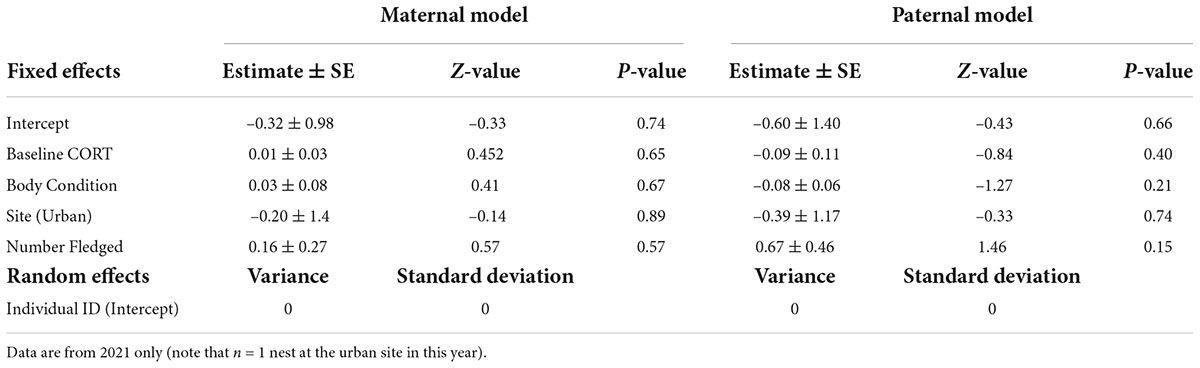
Table 2. Model estimates for the relationship between the proportion of daughters, maternal baseline CORT concentrations and body condition, and paternal baseline CORT concentrations and body condition.
Our final models investigated the influence of parental similarity on secondary sex ratios. The continuous index of parental CORT concentration similarity was not a predictor of the secondary sex ratios (Figure 3A and Table 3). However, when looking at our analysis with the simplified similarity index—whether each breeding pair had below or above average similarity—we found that parents with lower than average CORT similarity scores fledged a higher proportion of daughters (Figure 4A and Table 4). The similarity of parental body condition did not significantly influence brood sex ratio in either model (Figures 3B, 4B and Tables 3, 4). Finally, the site and the number of fledged offspring from the nest did not influence the proportion of daughters in these models (Tables 3, 4).
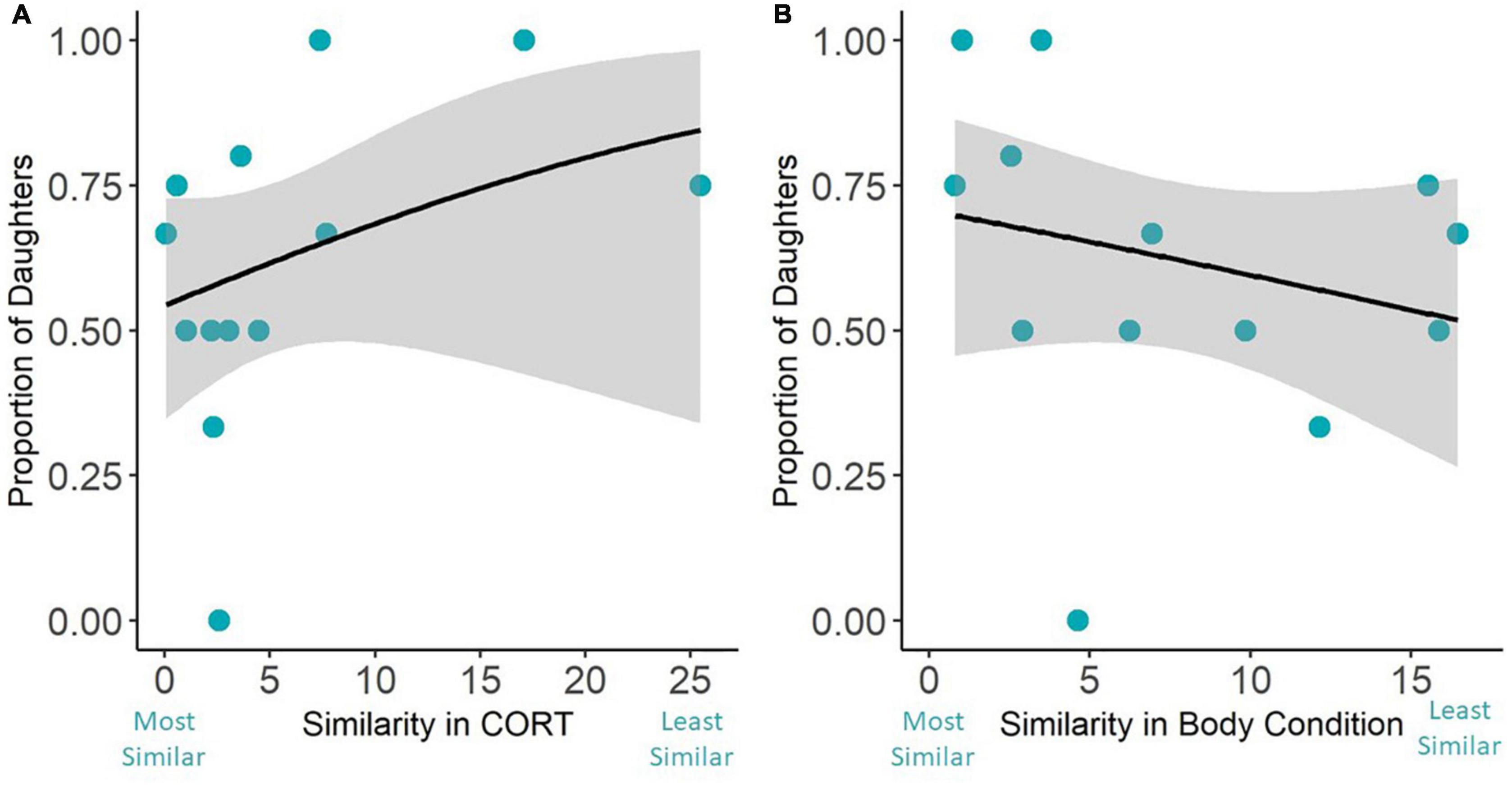
Figure 3. The similarity in parental baseline CORT concentrations (A) and the similarity of parental body condition (B) was not related to the sex ratio of the brood. Gray areas around the line represent the 95% confidence interval.
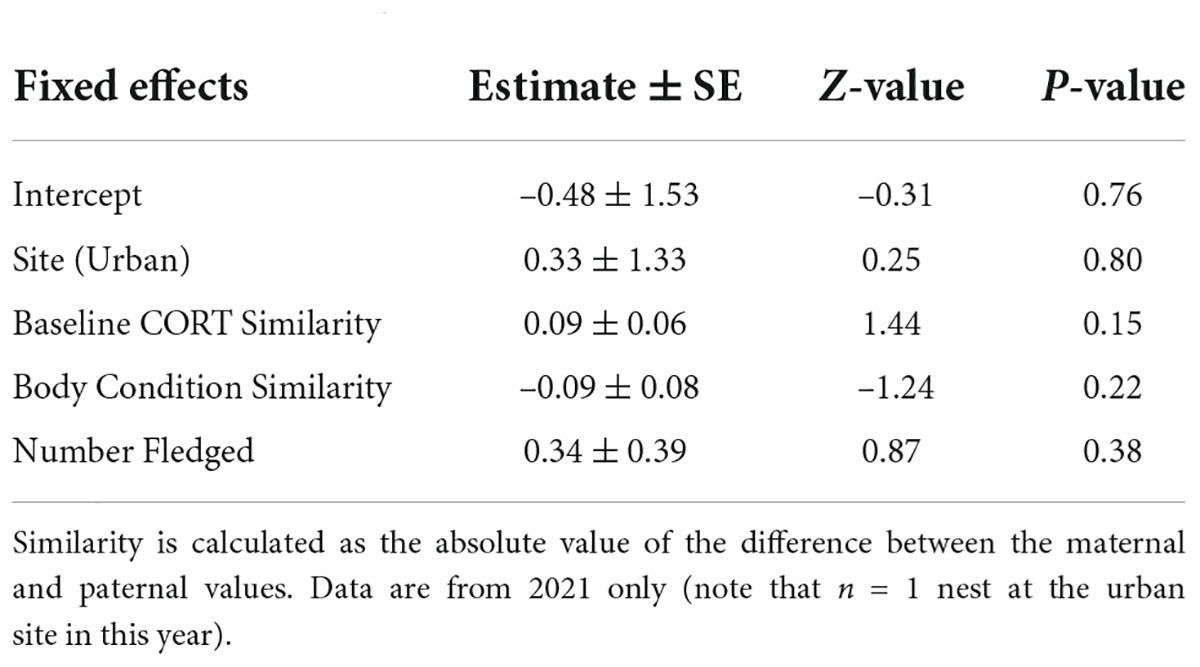
Table 3. Model estimates of the influence of similarity of maternal and paternal baseline CORT concentrations and body condition on the proportion of daughters for the continuous index.
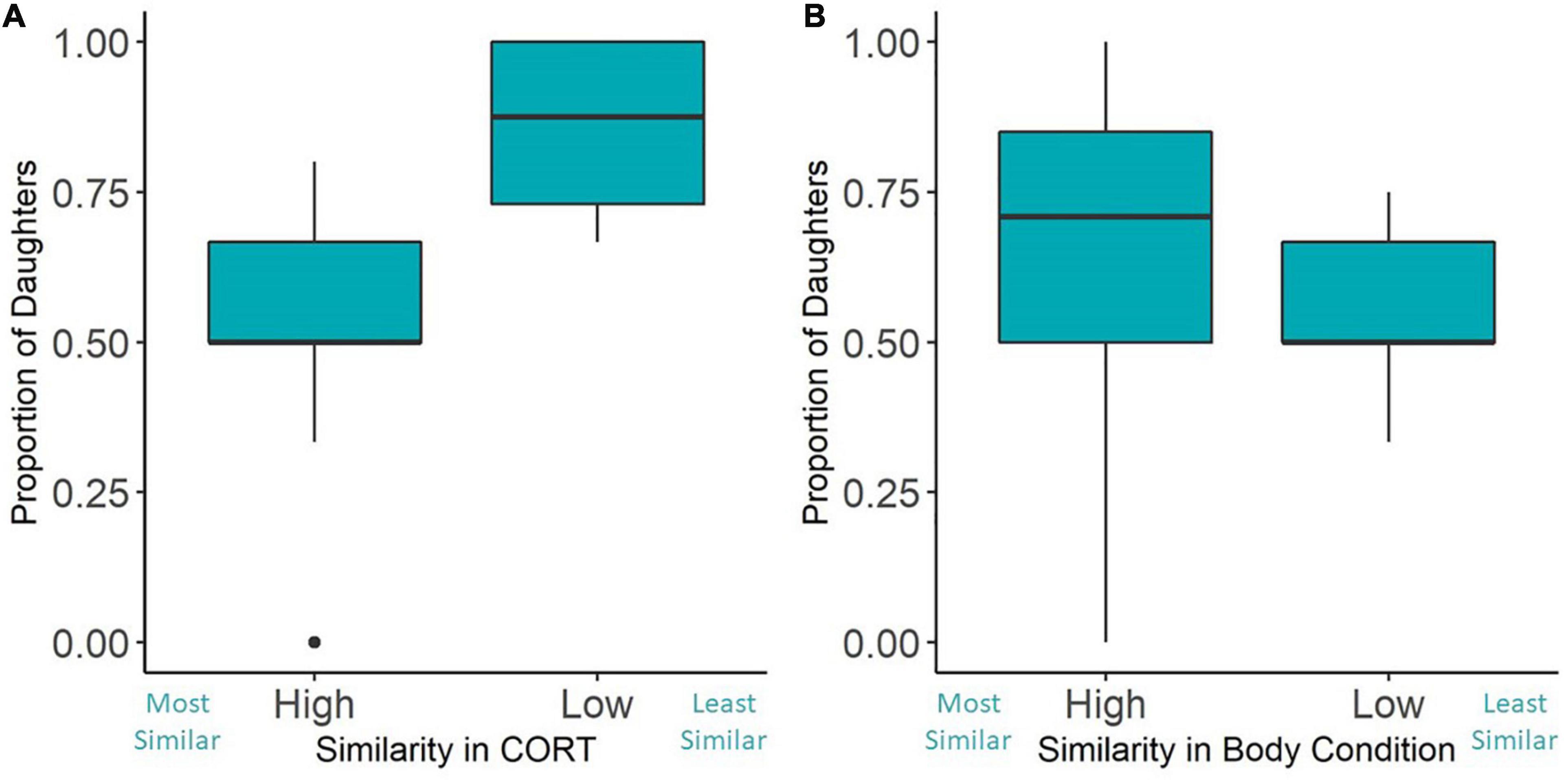
Figure 4. Pairs with below-average baseline CORT similarity fledged a significantly higher proportion of daughters than pairs with above-average similarity (A), while high or low similarity in body condition did not affect secondary sex ratios (B). Mid-line represents the median, boxes are the first and third quartiles, whiskers show minimum and maximum values, and closed circles are outliers.
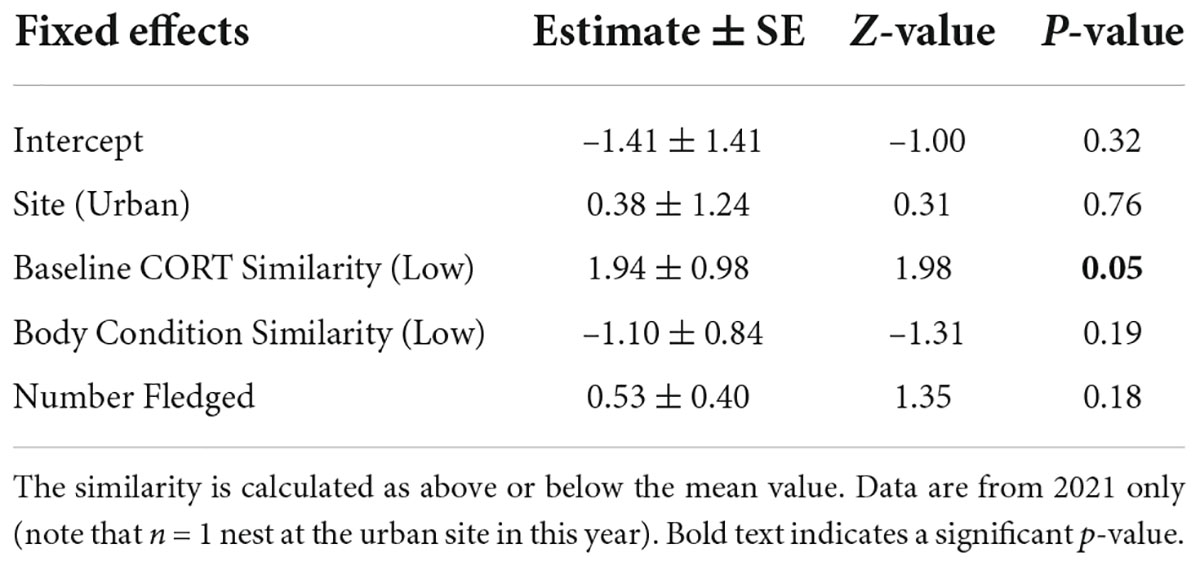
Table 4. Model estimates of the influence of similarity of maternal and paternal baseline CORT concentrations and body condition on the proportion of daughters for the categorical index.
Discussion
Our study did not find an effect of site or parental body condition or CORT on secondary sex ratios in starlings. Instead, we found partial support that the similarity of parental CORT levels (but not body condition) may increase the likelihood of successfully fledging sons, which may be the more costly sex and provide greater possible future fitness. These findings suggest that while parental condition alone was not related to secondary sex ratios, at least one measure of parental similarity may be related to secondary offspring sex ratios for this urban-adapted species. This finding is consistent with prior research examining the effect of parental similarity on reproductive success (Ouyang et al., 2014). This previous study on great tits showed that pairs with more similar baseline CORT stayed together for more seasons and had elevated reproductive success. One possible explanation for their result is that similarity in CORT represents similarity in investment or parental effort (Ouyang et al., 2014). Our study also supports this idea, as more similar pairs could fledge more of the more costly sex. If both parents invest equally in their offspring, their feeding efforts may be better coordinated. Previous research has shown that synchronicity in provisioning increases the total provisioning rate (Bebbington and Hatchwell, 2016), which could reduce the chance of insufficient provisioning, resulting in less sex-specific mortality or additional overall resources for the more costly sex. In addition, higher levels of coordination and more optimally timed feeding trips were related to increased reproductive success (Bebbington and Hatchwell, 2016). An alternative possibility is that parents respond to (and invest in their young according to) each other’s condition.
It is important to note that, while this relationship exists, we only see a significant effect of similarity in CORT when grouping pairs by above and below the mean similarity in our study. This categorical analysis uses the mean similarity as a threshold to rank pairs’ similarity (high CORT similarity n = 9, low CORT similarity n = 4). The continuous index provides a more complete picture of how sex ratio changes with similarity. Though the continuous and categorical similarity index showed similar qualitative patterns (parents with more similar CORT produced more sons), the continuous CORT similarity index was not significantly related to secondary sex ratios. This discrepancy may result from our small overall sample size, which may have limited our power to detect a relationship. Alternatively, the relationship we detected using the discretized index may be an artifact of our small sample size. Thus, the result from the categorical model can be considered preliminary. However, we still suggest that a key dissimilarity threshold may be required before offspring mortality occurs and secondary sex ratios are affected. Parents with intermediate similarity may show offspring with reduced condition, but without selective brood reduction, this may not affect secondary sex ratios. To date, few other studies have explored how parental similarity in CORT shapes their reproductive success—more work is needed across other study systems and with larger sample sizes to understand whether this pattern is common across taxa.
We were surprised to find no difference in brood sex ratios between sites, as we expected urban starlings to face more frequent disturbances than their rural counterparts, which could thus impact parental body condition or CORT. One possibility is that our small sample of urban nests hinders our ability to detect a difference in secondary sex ratios between an urban and rural site. As a result, more work with large sample sizes would be needed to draw conclusions on the impact of urbanization in shaping sex ratios. As urban adapters, starlings may be coping well with the challenges presented in urban areas so that a shift in the secondary sex ratio is not required to preserve maternal condition. This is supported by a post hoc analysis that found that adult body condition (rural: 0.44 ± 0.80; urban: –6.29 ± 4.82; t2.11 = 1.38, p = 0.3), CORT concentrations (rural: 14.91 ± 1.18; urban: 15.24 ± 2.11; t3.42 = –0.14, p = 0.9), and the total number of fledged offspring (rural: 3.06 ± 0.21; urban 3.5 ± 0.5; Wilcoxon signed-rank p = 0.42) did not differ between the sites (all values are reported as mean ± SE). Interestingly, we also found that the population-level variation does not differ from the equal allocation ratio of 1:1 predicted by Fisher (1930) at either site. Individual starlings in our study may show female-biased broods, but at the site level, there was no bias. If parental condition is similar between sites, this lack of population-level bias agrees with the Trivers–Willard hypothesis prediction that individual variations in sex ratio will cancel out (Trivers and Willard, 1973). However, it is difficult to draw definitive conclusions about sex ratio biases at our sites without additional data.
Our findings are consistent with earlier work that showed no influence of environmental variables on sex ratios in this species (Bradbury et al., 1997). While comparing sex ratios across individuals did not reveal the pattern we expected, examining within-individual variation in brood sex ratio at each site may reveal a different relationship. Oddie and Reim (2002) did not find a relationship between brood sex ratios and several environmental characteristics when comparing between mothers, but they did find biases when examining sex ratios of the same female across years. It is possible that the Trivers–Willard Hypothesis could still apply in this context but that the conditions in our urban site were not taxing enough so that the effect on parental body condition was small during our study and or that the biases varied across years in such a way that it was not detected. While there was a quantitative difference in the urbanization level of our two study sites, our urban site was only moderately developed when compared with the conditions experienced in larger cities that starlings are known to inhabit. Expanding this work across a broader gradient of environmental conditions could yield more insight into the role of anthropogenic environments in shaping secondary sex ratios and allow us to draw more robust conclusions.
Another finding of our study that contradicted our original prediction was that there was no significant effect of individual parental condition—either CORT or body condition—on the sex ratio of the brood. Some observational studies have also found no impact of maternal CORT on offspring sex ratio (Riechert et al., 2013; Henderson et al., 2014), but we know little about whether paternal CORT may act similarly to maternal CORT in adaptive sex allocation. One possibility is that there may be confounding factors obscuring the relationship between CORT and secondary sex ratios. For example, age and breeding experience, which we could not account for in our study, correlate with CORT concentrations and how individuals respond to stressors (Heidinger et al., 2006; Angelier et al., 2007). There may also be another hormonal mechanism that would be a better predictor of investment when examined in concert with CORT concentrations. For example, prolactin is a candidate hormone that could shape sex ratios due to its role in stimulating parental care behaviors and how it interacts with CORT (Angelier and Chastel, 2009). Finally, the CORT concentrations we measured may not fully capture the glucocorticoid phenotype of an individual. Accounting for within-individual plasticity in hormones could provide a more complete and accurate understanding of when parents are truly stressed and must change their behavior to conserve resources (Guindre-Parker, 2020).
Like our findings with CORT, parental body condition also showed no relationship with the sex ratio of fledged offspring. The lack of relationship between maternal body condition and sex ratio is surprising based on previous studies. It is possible that we were unable to detect a relationship between body condition and secondary sex ratios due to our sample size or because we did not sample animals under harsh enough environmental conditions. Our findings align with previous work that found only moderate bias with change in condition in Gouldian finches (Erythrura gouldiae) (Pryke et al., 2011). It is also possible that variation in body condition needs to be more drastic to induce a change in the fledging sex ratio. For example, Henderson et al. (2014) only found a significant change in the sex ratio of blue tits (Cyanistes caeruleus) in the year when mothers were in superior body condition. Perhaps in our study, mothers were in similar body condition to other years and did not bias their sex ratio. Longer-term research may be necessary to uncover the environmental or individual context when parental body condition does shape altered secondary sex ratios. One limitation of our study is that the body condition index uses only morphological metrics, even though individual condition is more complex than size and weight alone. Future work should include alternative measures of body condition, such as immunity or a scaled mass index.
While maternal and paternal body condition did not correlate with their investment in one sex over the other, we did find partial support for the possibility that the similarity of parental CORT may increase the parents’ ability to fledge the more costly sex. It is interesting that individual measures do not influence the brood sex ratio, but pair-based measures—such as similarity—might. The contrast in these findings raises questions about how parental investment changes with body condition for the sexes and how these sex-based differences in reproductive investment may shape pair similarity in starlings. While socially monogamous, European starlings do have extra-pair paternity at low rates (Smith and Von Schantz, 1993), and males, as in many species, show higher variance in reproductive success. Therefore, mothers may experience more pressure to maintain their investment and fledge a balanced ratio, regardless of their condition, whereas fathers may vary their investment in offspring care while still gaining fitness. This conclusion aligns with the findings that mothers behave as if they are committed to a certain amount or quality of parental care at the outset of breeding regardless of several environmental and individual variables (Fowler and Williams, 2015). In contrast, it is quicker and easier for males to produce a new brood than females, and this difference in initial investment in reproduction means that when they are in worse condition, they can provide less support to their mate without risking their entire investment for the season. Future work could explore how parental similarity in CORT shapes investment in parental care as a behavioral mechanism linking CORT similarity and sex ratios. For example, Oddie and Reim (2002) found that if the same female mated with a male in better condition in the next breeding season, their brood would be male-biased, and the inverse was also true. Perhaps a similar effect is occurring within our populations.
In conclusion, our research investigated the influence of parental CORT and parental body condition on secondary sex ratios in an urban-adapted species. The secondary sex ratio did not differ between the urban and rural site in our study, but our sample sizes were small. Future work with larger sample sizes should explore secondary brood sex ratios across an expanded gradient of urban and rural sites as many species—including starlings—breed in more urban areas than our urban study site. We also found that maternal and paternal body condition and CORT were not predictors of the sex ratio of fledged offspring but that pairs may be more successful at raising more costly sons when they show more similar baseline CORT. Our results suggest that for biparental species, the similarity of parental condition may be more important than the individual condition of either parent in shaping their reproductive investment and fitness. However, future work on parental similarity will be needed as very few studies have explored the role of similarity in shaping reproductive success and our findings can be considered preliminary. One exciting avenue for future research would be to explore whether parents are more likely to be similar—and thus more successful at raising more costly young—under some environmental conditions but not others. Finally, future secondary sex ratio research should expand upon our work to explore how urban-adapted and urban-avoiding species may respond to anthropogenic change differently. One possibility raised by our study is that urban-adapted species like the European starling can cope with urbanization relatively well and thus may not adjust sex ratios as urbanization increases. However, it remains less clear whether urban-avoiding species would show negative responses to our increasingly urbanized world.
Data Availability Statement
The original contributions presented in this study are included in the article/Supplementary Material, further inquiries can be directed to the corresponding author/s.
Ethics statement
The animal study was reviewed and approved by Institutional Animal Care and Use Committee, Kennesaw State University.
Author contributions
DK, TP, and SG-P contributed to the conception and design of the study. DK, CL, and SG-P collected the data and organized/maintained the database. DK performed the statistical analysis and wrote the first draft of the manuscript. All authors contributed to manuscript revision and read and approved the submitted version.
Funding
This research was funded by Kennesaw State University.
Conflict of Interest
The authors declare that the research was conducted in the absence of any commercial or financial relationships that could be construed as a potential conflict of interest.
Publisher’s Note
All claims expressed in this article are solely those of the authors and do not necessarily represent those of their affiliated organizations, or those of the publisher, the editors and the reviewers. Any product that may be evaluated in this article, or claim that may be made by its manufacturer, is not guaranteed or endorsed by the publisher.
Acknowledgments
We are grateful to the Gaines family, M. Blackwell, and the Kennesaw State University (KSU) field station for supporting our work on their properties. We thank K. DeCarlo, L. Belga, H. Lowin, K. Brown, S. Edge, B. Elliott, E. Maltos, and D. Kozlovsky for their assistance with field work. We also thank the reviewers whose feedback improved our manuscript.
Supplementary Material
The Supplementary Material for this article can be found online at: https://www.frontiersin.org/articles/10.3389/fevo.2022.894583/full#supplementary-material
References
Ágh, N., Pipoly, I., Szabó, K., Vincze, E., Bókony, V., Seress, G., et al. (2020). Does offspring sex ratio differ between urban and forest populations of great tits (Parus major)? Biol. Futura 71, 99–108. doi: 10.1007/s42977-020-00024-6
Amrhein, V. (2013). “Wild bird feeding (probably) affects avian urban ecology,” in Avian Urban Ecology, eds D. Gil and H. Brumm (Oxford: Oxford University Press), 29–37. doi: 10.1093/acprof:osobl/9780199661572.003.0003
Angelier, F., and Chastel, O. (2009). Stress, prolactin and parental investment in birds: A review. Gen. Comp. Endocrinol. 163, 142–148. doi: 10.1016/j.ygcen.2009.03.028
Angelier, F., Weimerskirch, H., Dano, S., and Chastel, O. (2007). Age, experience and reproductive performance in a long-lived bird: a hormonal perspective. Behav. Ecol. Sociobiol. 61, 611–621. doi: 10.1007/s00265-006-0290-1
Ardia, D. R. (2005). Super Size Me: An Experimental Test of the Factors Affecting Lipid Content and the Ability of Residual Body Mass to Predict Lipid Stores in Nestling European Starlings. Funct. Ecol. 19, 414–420. doi: 10.1111/j.1365-2435.2005.00997.x
Auman, H. J., Meathrel, C. E., and Richardson, A. (2008). Supersize Me: Does Anthropogenic Food Change the Body Condition of Silver Gulls? A Comparison Between Urbanized and Remote, Non-urbanized Areas. Waterbirds 31, 122–126. doi: 10.1675/1524-4695200831[122:SMDAFC]2.0.CO;2
Baldan, D., and Van Loon, E. E. (2022). Songbird parents coordinate offspring provisioning at fine spatio-temporal scales. J. Anim. Ecol. 91, 1316–1326. doi: 10.1111/1365-2656.13702
Bates, D., Mächler, M., Bolker, B., and Walker, S. (2015). Fitting Linear Mixed-Effects Models Using lme4. J. Stat. Softw. 67, 1–48. doi: 10.3929/ethz-b-000105397
Bebbington, K., and Hatchwell, B. J. (2016). Coordinated parental provisioning is related to feeding rate and reproductive success in a songbird. Behav. Ecol. 27, 652–659. doi: 10.1093/beheco/arv198
Berry, B. J. L. (2008). “Urbanization,” in Urban Ecology: An International Perspective on the Interaction between Humans and Nature, eds J. Marzluff, E. Shulenberger, W. Endlicher, and C. ZumBrunnen (New York, NY: Springer), 25–48.
Blair, R. B. (1996). Land use and avian species diversity along an urban gradient. Ecol. Appl. 6, 506–519. doi: 10.2307/2269387
Bókony, V., Seress, G., Nagy, S., Lendvai, ÁZ., and Liker, A. (2012). Multiple indices of body condition reveal no negative effect of urbanization in adult house sparrows. Land. Urban Plann. 104, 75–84. doi: 10.1016/j.landurbplan.2011.10.006
Bonderud, E. S., Otter, K. A., Murray, B. W., Marini, K. D., Burg, T. M., and Reudink, M. W. (2017). Effects of parental condition and nesting habitat on sex allocation in the mountain chickadee. Behaviour 154, 1101–1121. doi: 10.1163/1568539X-00003460
Bonier, F. (2012). Hormones in the city: Endocrine ecology of urban birds. Hormones Behav. 61, 763–772. doi: 10.1016/j.yhbeh.2012.03.016
Bonier, F., Martin, P. R., and Wingfield, J. C. (2007). Maternal corticosteroids influence primary offspring sex ratio in a free-ranging passerine bird. Behav. Ecol. 18, 1045–1050. doi: 10.1093/beheco/arm075
Bowers, E. K., Bowden, R. M., Thompson, C. F., and Sakaluk, S. K. (2016). Elevated corticosterone during egg production elicits increased maternal investment and promotes nestling growth in a wild songbird. Hormones Behav. 83, 6–13. doi: 10.1016/j.yhbeh.2016.05.010
Boyes, D. H., Evans, D. M., Fox, R., Parsons, M. S., and Pocock, M. J. O. (2021). Street lighting has detrimental impacts on local insect populations. Sci. Adv. 7:eabi8322. doi: 10.1126/sciadv.abi8322
Bradbury, R. B., Cotton, P. A., Wright, J., and Griffiths, R. (1997). Nestling Sex Ratio in the European Starling Sturnus vulgaris. J. Avian Biol. 28, 255–258. doi: 10.2307/3676977
Cabe, P. R. (2020). “European Starling (Sturnus vulgaris), version 1.0,” in Birds of the World, ed. S. M. Billerman (Ithaca, NY: Cornell Lab of Ornithology). doi: 10.2173/bow.eursta.01
Czúni, L., Lipovits, Á, and Seress, G. (2012). “Estimation of Urbanization Using Visual Features of Satellite Images,” in Proceedings of the AGILE’2012 International Conference on Geographic Information Science, Avignon 233–238.
Dantzer, B., Fletcher, Q. E., Boonstra, R., and Sheriff, M. J. (2014). Measures of physiological stress: a transparent or opaque window into the status, management and conservation of species? Conserv. Physiol. 2:cou023. doi: 10.1093/conphys/cou023
Deviche, P., and Davies, S. (2014). “Reproductive phenology of urban birds: environmental cues and mechanisms,” in Avian Urban Ecology, eds D. Gil and H. Brumm (Oxford: Oxford University Press), 98–115. doi: 10.1093/acprof:osobl/9780199661572.003.0008
Downing, J. A., and Bryden, W. L. (2008). Determination of corticosterone concentrations in egg albumen: A non-invasive indicator of stress in laying hens. Physiol. Behav. 95, 381–387. doi: 10.1016/j.physbeh.2008.07.001
Engelhardt, N. V., and Groothuis, T. G. G. (2005). Measuring Steroid Hormones in Avian Eggs. Ann. N.Y. Acad. Sci. 1046, 181–192. doi: 10.1196/annals.1343.015
Foltz, S. L., Davis, J. E., Battle, K. E., Greene, V. W., Laing, B. T., Rock, R. P., et al. (2015). Across time and space: Effects of urbanization on corticosterone and body condition vary over multiple years in song sparrows (Melospiza melodia). J. Exper. Zool. Part A 323, 109–120. doi: 10.1002/jez.1906
Fowler, M. A., and Williams, T. D. (2015). Individual variation in parental workload and breeding productivity in female European starlings: is the effort worth it? Ecol. Evol. 5, 3585–3599. doi: 10.1002/ece3.1625
Gil, D., and Brumm, H. (2013). “Acoustic communication in the urban environment: patterns, mechanisms, and potential consequences of avian song adjustments”. Avian Urban Ecology (ed) D. Gil and H. Brumm (Oxford: OUP Oxford) 69–83. doi: 10.1093/acprof:osobl/9780199661572.003.0006
Griffiths, R., Double, M. C., Orr, K., and Dawson, R. J. G. (1998). A DNA test to sex most birds. Mol. Ecol. 7, 1071–1075. doi: 10.1046/j.1365-294x.1998.00389.x
Guindre-Parker, S. (2020). Individual Variation in Glucocorticoid Plasticity: Considerations and Future Directions. Integr. Comp. Biol. 60, 79–88. doi: 10.1093/icb/icaa003
Hartig, F. (2022). DHARMa: Residual Diagnostics for Hierarchical (Multi-Level/Mixed) Regression Models. R Package Version 0.4.5. Available online at: https://CRAN.R-project.org/package=DHARMa
Hayward, L. S., and Wingfield, J. C. (2004). Maternal corticosterone is transferred to avian yolk and may alter offspring growth and adult phenotype. Gen. Comp. Endocrinol. 135, 365–371. doi: 10.1016/j.ygcen.2003.11.002
Heidinger, B. J., Nisbet, I. C. T., and Ketterson, E. D. (2006). Older parents are less responsive to a stressor in a long-lived seabird: a mechanism for increased reproductive performance with age? Proc. R. Soc. B 273, 2227–2231. doi: 10.1098/rspb.2006.3557
Henderson, L. J., Evans, N. P., Heidinger, B. J., Adams, A., and Arnold, K. E. (2014). Maternal Condition but Not Corticosterone Is Linked to Offspring Sex Ratio in a Passerine Bird. PLoS One 9:e110858. doi: 10.1371/journal.pone.0110858
Hoffmann, J., Postma, E., and Schaub, M. (2015). Factors influencing double brooding in Eurasian Hoopoes Upupa epops. IBIS 157, 17–30. doi: 10.1111/ibi.12188
Howe, H. F. (1977). Sex-Ratio Adjustment in the Common Grackle. Science 198, 744–746. doi: 10.1126/science.198.4318.744
Iglesias-Carrasco, M., Aich, U., Jennions, M. D., and Head, M. L. (2020). Stress in the city: meta-analysis indicates no overall evidence for stress in urban vertebrates. Proc. R. Soc. B 287:20201754. doi: 10.1098/rspb.2020.1754
Injaian, A. S., Francis, C. D., Ouyang, J. Q., Dominoni, D. M., Donald, J. W., Fuxjager, M. J., et al. (2020). Baseline and stress-induced corticosterone levels across birds and reptiles do not reflect urbanization levels. Conserv. Physiol. 8:coz110. doi: 10.1093/conphys/coz110
Joint Experimental Molecular Unit (2013). FTA Cards DNA Extraction Procedure. Available online at: https://jemu.myspecies.info/sites/jemu.myspecies.info/files/DNA%20Extraction%20-%20FTA%20Cards.pdf (accessed Jan 26, 2021).
Landys, M. M., Ramenofsky, M., and Wingfield, J. C. (2006). Actions of glucocorticoids at a seasonal baseline as compared to stress-related levels in the regulation of periodic life processes. Gen. Comp. Endocrinol. 148, 132–149. doi: 10.1016/j.ygcen.2006.02.013
Lenth, R. V. (2022). emmeans: Estimated Marginal Means, aka Least-Squares Means. R Package Version 1.7.5. Available online at: https://CRAN.R-project.org/package=emmeans
Liker, A., Papp, Z., Bókony, V., and Lendvai, Á. Z. (2008). Lean Birds in the City: Body Size and Condition of House Sparrows along the Urbanization Gradient. J. Anim. Ecol. 77, 789–795. doi: 10.1111/j.1365-2656.2008.01402.x
Lipovits, Á, Czúni, L., and Seress, G. (2015). “A Tool for Quantifying the Urban Gradient,” in Athens: ATINER’S Conference Paper Series, No: PLA2015-1709, Chalkokondili 1–10.
Love, O. P., Chin, E. H., Wynne-Edwards, K. E., and Williams, T. D. (2005). Stress Hormones: A Link between Maternal Condition and Sex-Biased Reproductive Investment. Am. Nat. 166, 751–766. doi: 10.1086/497440
Love, O. P., and Williams, T. D. (2008). The Adaptive Value of Stress-Induced Phenotypes: Effects of Maternally Derived Corticosterone on Sex-Biased Investment, Cost of Reproduction, and Maternal Fitness. Am. Nat. 172, E135–E149. doi: 10.1086/590959
Lynn, S. E. (2016). Endocrine and neuroendocrine regulation of fathering behavior in birds. Hormones Behav. 77, 237–248. doi: 10.1016/j.yhbeh.2015.04.005
Meillère, A., Brischoux, F., Parenteau, C., and Angelier, F. (2015). Influence of urbanization on body size, condition, and physiology in an urban exploiter: a multi-component approach. PLoS One 10:e0135685. doi: 10.1371/journal.pone.0135685
Murray, M. H., Sánchez, C. A., Becker, D. J., Byers, K. A., Worsley-tonks, K. E., and Craft, M. E. (2019). City sicker? A meta-analysis of wildlife health and urbanization. Front. Ecol. Environ. 17:575. doi: 10.1002/fee.2126
Nager, R. G., Monaghan, P., Griffiths, R., Houston, D. C., and Dawson, R. (1999). Experimental demonstration that offspring sex ratio varies with maternal condition. Proc. Natl. Acad. Sci. PNAS 96, 570–573. doi: 10.1073/pnas.96.2.570
Navara, K. J. (2013). The Role of Steroid Hormones in the Adjustment of Primary Sex Ratio in Birds. Integ. Comp. Biol. 53, 923–937. doi: 10.1093/icb/ict083
Navara, K. J., and Pinson, S. E. (2010). Yolk and albumen corticosterone concentrations in eggs laid by white versus brown caged laying hens. Poultry Sci. 89, 1509–1513. doi: 10.3382/ps.2009-00416
Oddie, K. R., and Reim, C. (2002). Egg sex ratio and paternal traits: using within-individual comparisons. Behav. Ecol. 13, 503–510. doi: 10.1093/beheco/13.4.503
Ottoni, I., de Oliveira, F. F. R., and Young, R. J. (2009). Estimating the diet of urban birds: The problems of anthropogenic food and food digestibility. Appl. Anim. Behav. Sci. 117, 42–46. doi: 10.1016/j.applanim.2008.11.002
Ouyang, J., Muturi, M., Quetting, M., and Hau, M. (2013). Small increases in corticosterone before the breeding season increase parental investment but not fitness in a wild passerine bird. Hormones Behav. 63, 776–781. doi: 10.1016/j.yhbeh.2013.03.002
Ouyang, J., Oers, K., Quetting, M., and Hau, M. (2014). Becoming more like your mate: hormonal similarity reduces divorce rates in a wild songbird. Anim. Behav. 98, 87–93. doi: 10.1016/j.anbehav.2014.09.032
Ouyang, J., Sharp, P. J., Dawson, A., Quetting, M., and Hau, M. (2011). Hormone levels predict individual differences in reproductive success in a passerine bird. Proc. Biol. Sci. 278, 2537–45. doi: 10.1098/rspb.2010.2490
Parolini, M., Possenti, C. D., Secomandi, S., Carboni, S., Caprioli, M., Rubolini, D., et al. (2019). Prenatal independent and combined effects of yolk vitamin E and corticosterone on embryo growth and oxidative status in the yellow-legged gull. J. Exper. Biol. 222:jeb199265. doi: 10.1242/jeb.199265
Patterson, S. H., Hahn, T. P., Cornelius, J. M., and Breuner, C. W. (2014). Natural selection and glucocorticoid physiology. J. Evol. Biol. 27, 259–274. doi: 10.1111/jeb.12286
Pickett, S. T. A., Cadenasso, M. L., Grove, J. M., Boone, C. G., Groffman, P. M., Irwin, E., et al. (2011). Urban ecological systems: Scientific foundations and a decade of progress. J. Environ. Manag. 92, 331–362. doi: 10.1016/j.jenvman.2010.08.022
Pryke, S. R., Rollins, L. A., and Griffith, S. C. (2011). Context-dependent sex allocation: Constraints on the expression and evolution of maternal effects. Evolution 65, 2792–2799. doi: 10.1111/j.1558-5646.2011.01391.x
R Core Team (2021). R: a Language and Environment for Statistical Computing. 4.1.1. Vienna, Aus: R Foundation for Statistical Computing
Rejt, L., Gryczynska-Siemiatkowska, A., Rutkowski, R., and Malewska, A. (2005). Does Egg sex ratio in Urban kestrels (Falco tinnunculus) differ from parity? Polish J. Ecol. 53, 545–552.
Riechert, J., and Becker, P. H. (2017). What makes a good parent? Sex-specific relationships between nest attendance, hormone levels, and breeding success in a long-lived seabird. Auk 134, 644–658. doi: 10.1642/AUK-17-13.1
Riechert, J., Chastel, O., and Becker, P. (2013). Mothers under stress? Hatching sex ratio in relation to maternal baseline corticosterone in the common tern (Sterna hirundo). J. Comp. Physiol. A. 199, 799–805. doi: 10.1007/s00359-013-0840-8
Rubenstein, D. (2007). Temporal but Not Spatial Environmental Variation Drives Adaptive Offspring Sex Allocation in a Plural Cooperative Breeder. Am. Nat. 170, 155–165. doi: 10.1086/518671
Rubolini, D., Romano, M., Boncoraglio, G., Ferrari, R. P., Martinelli, R., Galeotti, P., et al. (2005). Effects of elevated egg corticosterone levels on behavior, growth, and immunity of yellow-legged gull (Larus michahellis) chicks. Hormones Behav. 47, 592–605. doi: 10.1016/j.yhbeh.2005.01.006
Saino, N., Romano, M., Ferrari, R. P., Martinelli, R., and Møller, A. P. (2005). Stressed mothers lay eggs with high corticosterone levels which produce low-quality offspring. J. Exp. Zool. 303A, 998–1006. doi: 10.1002/jez.a.224
Sánchez-Macouzet, O., Rodríguez, C., and Drummond, H. (2014). Better stay together: pair bond duration increases individual fitness independent of age-related variation. Proc. R. Soc. B 281:20132843. doi: 10.1098/rspb.2013.2843
Sapolsky, R. M., Romero, M., and Munck, A. U. (2000). How Do Glucocorticoids Influence Stress Responses? Integrating Permissive, Suppressive, Stimulatory, and Preparative Actions. Endocrine Rev. 21, 55–89. doi: 10.1210/er.21.1.55
Schoech, S. J., Rensel, M. A., and Heiss, R. S. (2011). Short- and long-term effects of developmental corticosterone exposure on avian physiology, behavioral phenotype, cognition, and fitness: A review. Curr. Zool. 57, 514–530. doi: 10.1093/czoolo/57.4.514
Schwabl, H. (1993). Yolk is a source of maternal testosterone for developing birds. Proc. Natl. Acad. Sci. 90, 11446–11450. doi: 10.1073/pnas.90.24.11446
Seress, G., and Liker, A. (2015). Habitat urbanization and its effects on birds. Acta Zool. Acad. Sci. Hungaricae 61, 373–408. doi: 10.17109/AZH.61.4.373.2015
Seress, G., Lipovits, Á, Bókony, V., and Czúni, L. (2014). Quantifying the urban gradient: A practical method for broad measurements. Land. Urban Plann. 131, 42–50. doi: 10.1016/j.landurbplan.2014.07.010
Shanahan, D. F., Fuller, R. A., Warren, P. S., and Strohbach, M. W. (2013). “The challenges of urban living,” in Avian Urban Ecology, eds D. Gil and H. Brumm (Oxford: Oxford University Press), 3–20. doi: 10.1093/acprof:osobl/9780199661572.003.0001
Smith, H. G., and Von Schantz, T. (1993). Extra-Pair Paternity in the European Starling: The Effect of Polygyny. Condor 95, 1006–1015. doi: 10.2307/1369436
Strange, M. S., Bowden, R. M., Thompson, C. F., and Sakaluk, S. K. (2016). Pre- and Postnatal Effects of Corticosterone on Fitness-Related Traits and the Timing of Endogenous Corticosterone Production in a Songbird. J. Exper. Zool. Part A 325, 347–359. doi: 10.1002/jez.2022
Strubbe, D., Salleh Hudin, N., Teyssier, A., Vantieghem, P., Aerts, J., and Lens, L. (2020). Phenotypic signatures of urbanization are scale-dependent: a multi-trait study on a classic urban exploiter. Landsc. Urban Plan. 197:103767. doi: 10.1016/j.landurbplan.2020.103767
Tilgar, V., Mägi, M., Lind, M., Lodjak, J., Moks, K., and Mänd, R. (2016). Acute embryonic exposure to corticosterone alters physiology, behaviour and growth in nestlings of a wild passerine. Hormones Behav. 84, 111–120. doi: 10.1016/j.yhbeh.2016.06.008
Trivers, R. L., and Willard, D. E. (1973). Natural Selection of Parental Ability to Vary the Sex Ratio of Offspring. Science 179, 90–92. doi: 10.1126/science.179.4068.90
Väli, Ü (2012). Factors Limiting Reproductive Performance and Nestling Sex Ratio in the Lesser Spotted Eagle Aquila pomarinaat the Northern Limit of Its Range: the Impact of Weather and Prey Abundance. Acta Ornithol 47, 157–168. doi: 10.3161/000164512X662269
Verboven, N., and Verhulst, S. (1996). Seasonal Variation in the Incidence of Double Broods: The Date Hypothesis Fits Better than the Quality Hypothesis. J. Anim. Ecol. 65, 264–273. doi: 10.2307/5873
Keywords: urban, rural, glucocorticoid, Trivers-Willard, Sturnus vulgaris, sex ratio adjustment
Citation: Kilgour DAV, Linkous CR, Pierson TW and Guindre-Parker S (2022) Sex ratios and the city: Secondary offspring sex ratios, parental corticosterone, and parental body condition in an urban-adapted bird. Front. Ecol. Evol. 10:894583. doi: 10.3389/fevo.2022.894583
Received: 11 March 2022; Accepted: 28 June 2022;
Published: 19 July 2022.
Edited by:
Amanda Wilson Carter, The University of Tennessee, Knoxville, United StatesReviewed by:
Veronika Bókony, Centre for Agricultural Research, HungaryAshley Love, University of Connecticut, United States
Juan Gallego Rubalcaba, McGill University, Canada
Copyright © 2022 Kilgour, Linkous, Pierson and Guindre-Parker. This is an open-access article distributed under the terms of the Creative Commons Attribution License (CC BY). The use, distribution or reproduction in other forums is permitted, provided the original author(s) and the copyright owner(s) are credited and that the original publication in this journal is cited, in accordance with accepted academic practice. No use, distribution or reproduction is permitted which does not comply with these terms.
*Correspondence: Denyelle A. V. Kilgour, RGVueWVsbGVAbWV0cm9jYXN0Lm5ldA==