- Institute of Ecology and Environmental Sciences—Paris, UMR 7618 (CNRS-SU-IRD-UPEC- UPC-INRAe), Bondy, France
Urban agriculture has been of growing interest for a decade because it can address many economic and societal issues in the development of modern cities. However, urban agriculture is often limited by the availability of fertile and non-contaminated soils in the cities. Recycling excavated mineral wastes from building activities to construct fertile soils may be a more sustainable alternative than the importation of topsoils from rural zones. The present study aims to evaluate the possibility to grow green vegetables on soils made with excavated deep horizon of soils and green waste compost. During three consecutive seasons, we tested in situ the effects of different amounts of compost (10, 20, and 30%) and the addition of an earthworm species (Lumbricus terrestris) on the production of lettuce (Lactuca sativa L.), arugula (Eruca sativa Mill.), and spinach (Spinacia oleracea L.) in mono- and co-culture. Our results demonstrate that it is possible to reuse mineral and organic urban wastes to engineer soils adapted to agriculture. Here, we observed that higher doses of compost significantly increased plant biomass, especially when earthworms were introduced. For example, in the autumn, going from 10 to 30% of compost in the soil mixture allows to multiply by 2 the arugula biomass, and even by 4 in the presence of earthworms. These results were partly due to the positive effects of these two factors on soil physical properties (micro- and macro-porosity). This preliminary study also showed that some plants (arugula) are more adapted than others (lettuce) to the soil properties and that it only takes few months to get the highest yields. These promising results for the development of urban agricultures encourage to test many other combination of plant and earthworm species but also to conduct experiments over long-term periods.
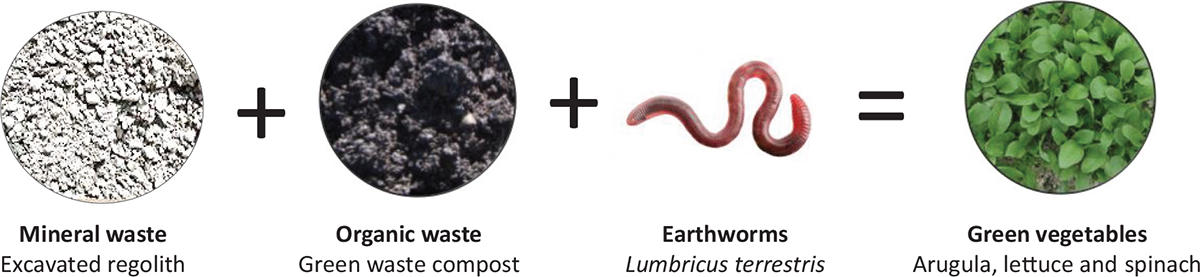
Graphical Abstract. Excavated mineral wastes from building activities can be recycled with compost to construct fertile soils under the action of earthworms.
Introduction
Since 2007, half of the world population is leaving in urban environments, and this ratio is expected to reach 60% before the end of the decade (United Nations [UN], 2019). The huge development of urban populations consumes so much resource that natural provision cannot be sustained any more (Swilling, 2011). That is particularly the case of topsoil, which is imported to the cities from rural areas for the creation of green spaces. Concomitantly, urban agriculture has flourished in developed countries, bearing more and more weight in the local urbanism plans and in public policies. The services provided by urban agriculture strengthened its potential to contribute to quality of life in cities (Zasada, 2011; Lin et al., 2015). However, the soil quality in cities is often severely degraded (Morel et al., 2015) due to harsh environmental conditions, which may limit the development of such agronomic activities. Urban soils often bear strong legacies of human land-use, road traffic and industrialization, which can be quantified by the degree of various organic and/or mineral pollutants found at different depths. For instance, a study along a gradient of urban pressure in public green spaces of the Paris region (France) showed that most of the soils were highly contaminated with trace metals such cadmium (Cd), lead (Pb), copper (Cu), and Zinc (Zn), especially in woods (Foti et al., 2017). These contaminations make soils unsuitable for gardening or food production. Urban gardeners are particularly exposed to sanitary risks, either through the consumption of their own food that may accumulate harmful substances or by contact and inhalation of the contaminated soil.
To face the problem of soil pollution and lack of available space, soil-less agriculture as hydroponics, aquaponics or other artificial substrates are sometimes considered as an alternative. However, these technical solutions are limited by their high cost and the suboptimal nutrient supply to plants (Yep and Zheng, 2019). Moreover, they cannot entirely replace essential ecosystem services that are associated to soil biodiversity, such as nutrients cycle and water purification (Millenium Ecosystem Assessment, 2005). Thus, cities stakeholders often import huge quantity of topsoils from rural area to recover or replace urban soils. In France, the quantity of soil imported for the creation of green spaces has been estimated to 3 million m3 each year (Damas and Coulon, 2016). These practices, which are dependent of the availability of natural soils, are poorly sustainable since they rely on the extension of artificial land. The ecological and economic costs of importing soils from rural areas are continuously increasing. Therefore, alternatives that are more virtuous have to be found. Paradoxically, a solution may result from the increasing activities of construction and demolition in the cities. Indeed, urban renewal sites generate huge quantities of mineral wastes. In particular, the construction of foundations for buildings, garages or subways requires the excavation of large volumes of deep soil horizons or bedrocks that have no immediate use. Treated as wastes, such materials are commonly exported and managed outside the cities, because the urban space is scarce. In Paris, more than 15 Mt of excavated deep horizons (EDH) are treated in landfill sites every year (Ile-de-France, 2019). Should a small fraction of the latter be reuse as a substitute of natural soil, it could avoid the importation from rural areas. In New York City, it has been estimated that 1.7 Mt of surplus native soil is generated each year (Walsh et al., 2019).
Creating fertile soils by combining organic and mineral anthropogenic materials may have appeared a challenge as it takes several hundred years to obtain a few centimeters of fertile soil in natural conditions. However, during the last 15 years (Lehmann, 2011), a number of studies published in this field of research showed promising results. Most of these constructed soils may be qualified as constructed Technosols. According to the World Reference Base, Technosols are defined by soils subjected to a strong human influence and containing at least 20% of artifacts (IUSS Working Group WRB, 2014). Not all engineered soils can be included in this definition. For example, human-transported natural soil material does not qualify as Technosol (Schad, 2018). Regardless of the soil classification used, engineered soils have been shown to provide a wide variety of functions and ecosystem services (Morel et al., 2015). Urban mineral wastes such as EDH have been successfully used in several urban forestry projects (Cannavo et al., 2018; Yilmaz et al., 2018; Pruvost et al., 2020), however, very few demonstrations have been made regarding their use for urban agriculture. Yet, it has been demonstrated that excavated sediments in New York city can be used as constructed soils to reduce contamination risks for urban residents (Egendorf et al., 2018).
The objective of the present study was to evaluate the effect of compost addition and earthworms inoculation to improve the agronomic properties of EDH. Laboratory experiments performed on the same materials with ryegrass showed that the combination of compost and earthworms increased both soil water retention and plant biomass productivity (Deeb et al., 2016a,b). Here we tested in situ (1) the dose effect of green waste compost, (2) the influence of earthworm introduction and (3) the interactions between these two factors on the production of green vegetables. For this study, three types of salads were tested in mono- and co-culture. The experience was repeated tree times in spring, summer and autumn. We hypothesis that the highest biomass productivity will be reached on the highest concentration of compost in presence of earthworms.
Materials and Methods
Parent Materials
The excavated materials found in Paris region are generally made of unconsolidated carbonated bedrock (regolith) originated from alluvial sediments deposited during the Eocene. Here, the EDH came from an underground parking construction in the north of Paris (48° 54′ 13.208″ N 2° 19′ 58.98″ E). We used around 8 tons of materials for the experiment. After sieving at 10 mm, four samples were collected randomly to perform chemical analyses. EDH was composed of 714 ± 35 g.kg–1 CaCO3. The texture was 373 ± 23 g.kg–1 sand, 427 ± 23 g.kg–1 silt and 199 ± 6 g.kg–1 clay (method NFX 31-107). The X-ray diffraction performed with a Siemens D500 diffractometer (CuKa, 40 kV, 30 mA) identified quartz, calcite and dolomite as major minerals, and also, sepiolite, a fibrous clay mineral of sedimentary origin. The pH (ISO 10390) was high, 8.3 ± 0.1, the organic C content was 3.8 ± 0.1 g.kg–1, the total N was 0.3 ± 0.1 g.kg–1 and the bulk density was 1.3 ± 0.1 g.cm–3. The green waste compost (GWC) was produced from turf grass mowing and trimmings from trees and shrubs. The pH (ISO 10390) of the GWC was 7.9 ± 0.1, the organic C content was 322.4 ± 15.8 g.kg-1, the total N was 21.8 ± 1.9 g.kg-1 (C/N ratio of 14.8), and the bulk density was 0.6 ± 0.1 g.cm-3. The water holding capacity (pF 2.5) was estimated to 28% for EDH and 56% for GWC, respectively. Both parent materials were provided by ECT Company, which is specialized in the management of excavated materials from construction and tunneling sites.
Experimental Design
The experimental site was located at the IRD Campus, Bondy, France (48°54′52″N 2°29′14″E). The experiment started in April 2017 and consisted in 96 wooden garden pots, divided into four blocks. Each block contains all 24 treatments randomly distributed (Supplementary Figure 1): 4 Cultures (3 monocultures, 1 co-culture) on 3 different mixtures of soils (with gradual amount of GWC) with or without (earthworms). Before mixing, both materials (EDH and GWC) were passed through an automatic screener with a limited grain size of 10 mm. Then, three different soils (named T10, T20, and T30) were prepared by adding 10, 20, or 30% of GWC (V/V), respectively. Each mixture of materials (39 L) was homogenized (27 rpm for about 5 min long) using 100 L electric concrete mixer and then put in a garden wooden pot (44 × 44 cm; 28 cm depth) internally coated with a permeable geotextile and previously filled with 8 cm of gravel to allow drainage. So, the depth of the Technosol was initially 20 cm. The initial bulk density of the soils was 1.26, 1.19, and 1.11 g.cm–3, respectively, for T10, T20 and T30 modalities. One week after filling the pots, half of them (48 in total) were inoculated with 8 adult earthworms of the species Lumbricus terrestris. The number of individuals per m2 is higher than the natural density found in arable lands in northwestern France (Decaëns et al., 2008). It was an intended choice to counterbalance the possible escape of earthworms from pots. A previous study assured the suitability of GWC as food source (Pey et al., 2014). This species is native and widespread species in Europe, although it is commonly known as the “Canadian Nightcrawler” bait worm (Steckley, 2020). The specimens were purchased from a company (Lombri’Carraz, Mery, France).
Plant Growth Conditions
The plants used in this study are three species that are commonly included in a mixed crop of salads sold as mesclun, in France. They are lettuce (Lactuca sativa L.), arugula (Eruca sativa Mill.) and spinach (Spinacia oleracea L.), who belong to the families Asteraceae, Brassicaceae, and Amaranthaceae, respectively. Those three species are commonly integrated in mixed salad produced and consumed in France, under the name of “mesclun.” They are all suitable for spring, summer and fall cultivation. All seeds were purchased from an organic seed company (Agrosemens, Rousset, France). One week after the addition of earthworms, around 220 seeds per pot were sown for monocultures and 73 seeds of each plant for the co-cultures. Three harvest cycles were done, each one following 5 weeks of growth in a different season. The first harvest took place in the spring (May 19th), the second in the summer (June 27th), and the last one in autumn (October 25th). Between each harvest, the eventual late germinated seeds and weeds were removed and the soil labored for a new sowing. The rainfall and temperature were recorded at meteorological station situated near the experimental site (Le Bourget; 48°96′15″N; 2°43′75″E) (see Supplementary Figure 2). The rainfall accumulation in the first period was 60.9 mm and the mean temperature varied between 7 and 17 degrees. In the second period, the rainfall was 32.6 mm and the mean temperature varied between 15 and 27 degrees. In the third period, the rainfall was 43.8 mm and the mean temperature varied between 11 and 20 degrees. All pots were irrigated every 2 days in the case of no rainfall (1.2 L per pot).
Plant Analyses
Germination rate was calculated by the ratio of harvested plants and the number of seeds sown. The evolution of vegetal covering was monitored by image analysis of surface pictures taken every 5 days. Images were analyzed in the software “imageJ” (version 1.53c), which quantifies the proportion of green area. When it was necessary, light and contrast were adjusted using the software “Gimp” (version 2.10). Results obtained in function of time allowed a broad visualization of the growth rates. Plants production are expressed as dry shoot biomass per meter square, which was calculated by multiplying the production within a pot (0.193 m2) by its corresponding meter square surface. Individual biomass was calculated as the ratio between biomass and plants number in each pot. The fresh biomass was obtained by weighting leaves right after the harvest. The conversion to dry biomass (obtained after a week of drying at 60°C) is given in Supplementary Figure 3. The co-culture biomass evenness (E) was calculated using the Simpson-Yule index: E = 1/Σpi2, where pi is the proportion of biomass of each plant (Magurran, 1988).
Soil Analyses
The soil parameters were measured after the last harvest. The soil water content was measured with a dielectric sensor WET-AT (Delta-T Devices, Cambridge, United Kingdom), a probe consisting of three metal rods, which have been inserted vertically into the soil. The pH analysis of all experimental units (pH-meter Hanna HI 221) was measured as described above. Under stirring, the values were registered when the pH measurement stabilized. The bulk density was determined by two different methods. One using a graduated cylinder and another using the petrol method. The first one measures the soil weight extracted with a 100 cm3 stainless cylinder. Samples are weighted fresh and after drying at 150°C for 48 h. The second method is the measure of the Archimedes’ buoyancy from aggregates within petrol, which allow the estimation of macro and micro pores (Monnier et al., 1973).
Statistics
All statistical analyses were carried out using R software version 3.3.1 (R Development Core Team).1 After normality and homoscedasticity verifications (Shapiro and Bartlett test), plants parameters (germination rate, area covered, and shoot biomass) were analyzed using a two-way ANOVA with three levels of compost and two levels of worms for each plant treatment and each season of culture. When significant (P < 0.05) effect were found, comparisons among means were performed using Least Significant Difference (LSD) test (“agricolae” package). Graphics and linear regressions realized on soil parameters (pH, soil density, moisture, macro- and micro-porosity) were done using SigmaPlot 14.0 (Systat Software Inc.).
Results
Plants Germination and Development
The germination rate varied greatly with the plant species and the season (Supplementary Figure 4). In the spring, the overall germination rate was the highest with more than 80% for all cultures, and the lowest in the summer with only 51%. Globally, the influence of compost was only significant for the monocultures of arugula and spinach in spring and autumn (Table 1 and Supplementary Table 1). For arugula in spring, increasing compost dose from 10 to 30% increased by 12 or 30% the germination rate, without or with earthworms, respectively. In contrast, for arugula in the autumn, an increase in germination rate with compost content was only observed in the absence of earthworms. It improved by 88% between soils with 10 and 30% of the compost dose (Supplementary Figure 4). Besides, earthworms have very little impact on germination. They reduced by 7% the spinach germination in spring and offset the effect of compost for the arugula in autumn (Supplementary Figure 4). The highest dose of compost improved spinach germination by approximately 5% compared to the lowest dose.
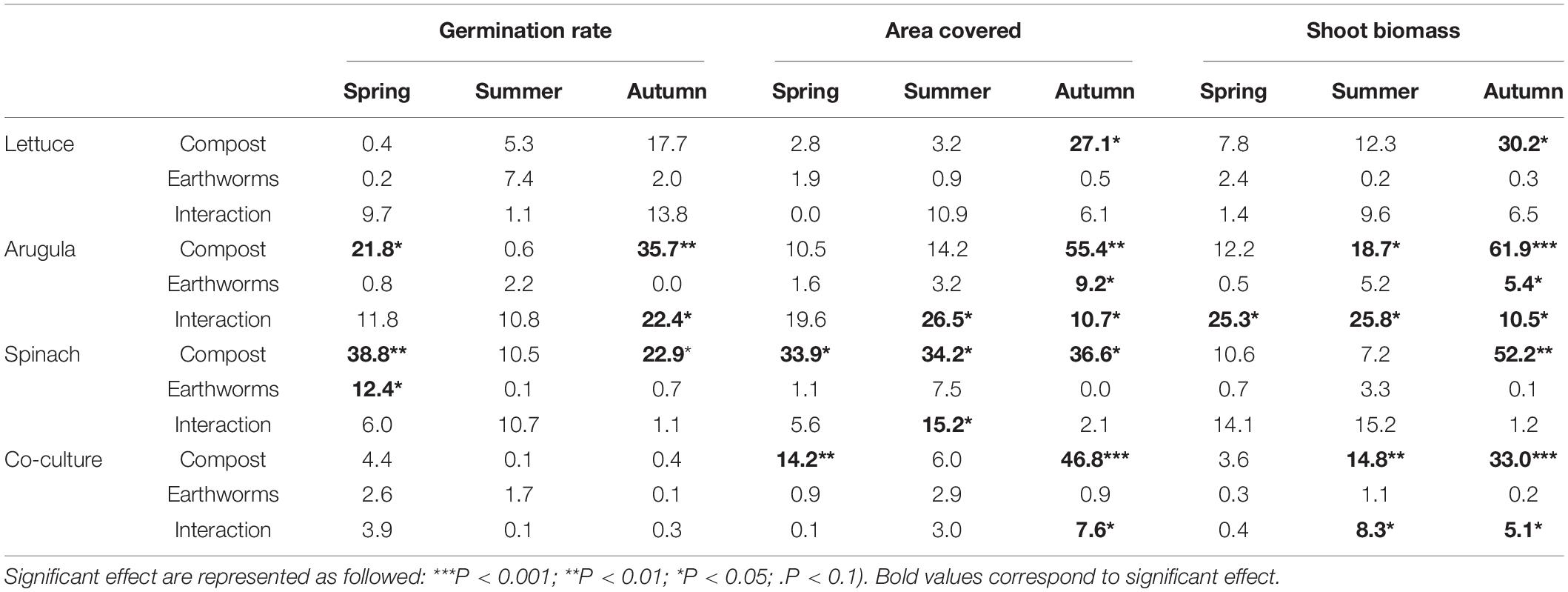
Table 1. Proportion (%) of the variance explained by the relative amount of compost, the presence of earthworms and the interactions between the two latter factors for each monoculture and the co-culture of all plants (n = 4).
As for the germination rate, the leaves coverage also varied with the plant species and the season. The overall area covered by leaves was higher in the spring (55%) than in the summer (24%) and autumn (43%), which followed the seasonal variation in the germination rate (Supplementary Figure 5). The lettuce development contrasts with others during spring and summer, with only 33 and 13% of area covered, while other cultivars and the co-culture had similar means, with 57–63% in the spring and 25–29% in the summer. Compost dose and earthworms significantly affected plant coverage, but to different degrees depending on season and cultivar. In autumn, the addition of compost increased significantly the area covered for all cultures (+29, +65, +53, +49%, respectively, for lettuce, arugula, spinach and co-culture) (Supplementary Figure 5). The addition of earthworms positively influenced co-culture and arugula monoculture, but exclusively in the soil containing 30% compost. The area covered by the co-culture and arugula were, respectively, 17 and 60% higher with earthworms. In spring, the effect of compost only influenced spinach and the co-culture. While the effects of compost on spinach cover were negatives (51% less coverage with 30% compost compared to 10%), it was positive on the leaf coverage in the co-culture (40% higher with 30% compost compared to 10%). In the summer, only spinach and arugula leaves were influenced by the studied factors (Table 1). Spinach was again negatively impacted by higher doses of compost (41% less in 30% compost compared to 10%), but on the other hand, they were positively affected by the addition of earthworms in soils with 10% (covered area was 50% higher). The arugula coverage positively responded to the increasing amounts of compost, but only with the addition of earthworms. In this case, the area covered by arugula with 30% compost was about 3 times higher than with 10% of compost.
Final Biomass Production
Globally, the final biomass production followed the same trend as the area covered for each culture. High correlations (0.63 < r2 < 0.98) were found between the final area covered and the dry biomass (Supplementary Figure 6). For all cultivars, the slope of the linear regressions were much higher in the summer than in autumn and spring, indicating higher biomass per area covered at this season. The final biomass measurement also showed the effects of compost and earthworms on plants production according to the season (Figure 1). The biomass production tended to be different between soils with only 10% compost and the two others, when earthworms were not considered. With earthworms, the soil containing 30% compost tended to be considerably more productive (Figure 1). The effect of compost as a single factor was mainly observed in the autumn, when increasing doses of compost positively influenced all cultures (+54, +123, +86, 109%, respectively, for lettuce, arugula and spinach monocultures, and for co-culture). Compost also influenced the co-culture biomass in the summer (+66% production). On the other hand, the earthworms in the soils containing 30% compost boosted arugula production in all seasons (+215, +416, 130%, respectively, for spring, summer, and autumn). Earthworms also accentuated the effects of compost when added at a 30% level on the biomass of co-culture, both in summer (+ 153%) and autumn (+ 31%). In the co-cultures, the arugula and the spinach dominated the shoot biomass, especially in the first two seasons (Figure 2). Changes in the relative proportion of each plant was revealed by the evenness index (Figure 2), which globally increased throughout the 3 seasons, from 71% in the spring to 81% in the autumn. In the summer, it could be mentioned that the evenness of the shoot biomass was higher in Technosol made with 10% compost in presence of earthworms, compared to those made with 20 and 30% compost.
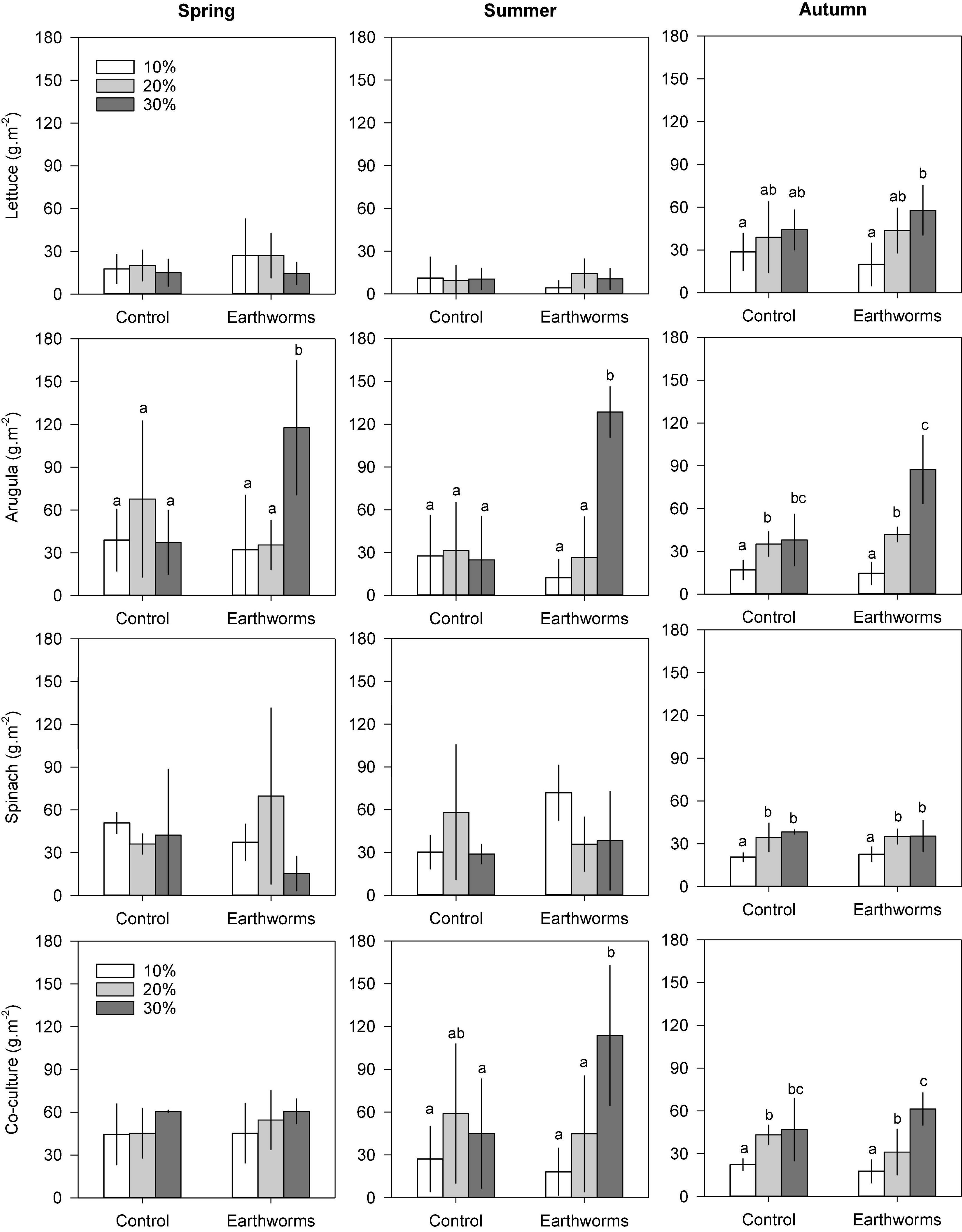
Figure 1. Shoot biomass (dry weight per m2) of lettuce, arugula, spinach in monoculture and co-culture obtained at each season (spring, summer, and autumn) as function of earthworms presence for the three Technosols composition. The error bars are the standard errors of the mean (n = 4). Different letters above the histogram indicate significant differences (P < 0.05) between treatments.
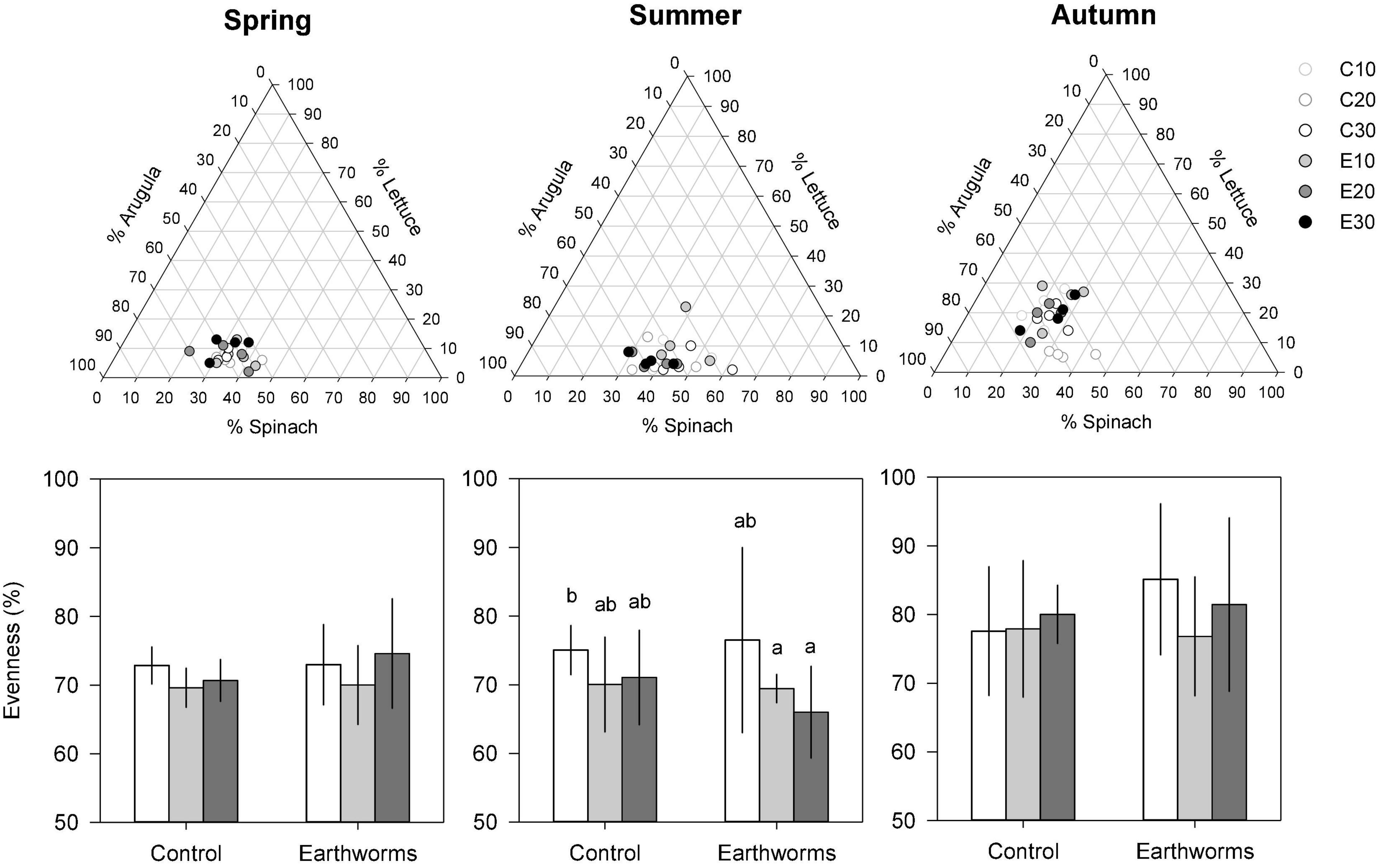
Figure 2. Proportion of lettuce, rocket and spinach biomass obtained in co-culture at each season (spring, summer, and autumn) as function of the compost proportion in Technosols with (E10, E20, E30) or without (C10, C20, C30) earthworms (upper triangles). The biomass evenness is indicated below. The error bars are the standard errors of the mean (n = 4). Different letters above the histogram indicate significant differences (P < 0.05) between treatments.
Soil Properties
After 6 months of construction, the earthworms revealed or at least reinforced the effect of compost on soil properties (Figure 3). The pH of all Technosols was in average 8.3 ± 0.1, which is approximately the same pH as the initial EDH used as parent material. The influence of the compost dose was not observed, excepted when earthworms were presents. In this case, the increase of compost proportion decrease slightly (0.1 unit) but significantly the pH. As expected, the higher the proportion of compost, the lower the density of the soil. This result can be related to the increase of the microporosity with compost (Figure 3). It can also be due to the increase of the macroporosity, but only in the presence of earthworms (+10% between 10 and 30% of compost in the mix). The increase of soil water content, measured at the end of the last harvest (late October), was also observed with the increasing amount of compost in the soil, but only in the pots containing earthworms (Figure 3), with about 12% more humidity between 10 and 30% of compost in the mix. It should also be noted that all soils were confronted with crusts formation after rainfall or irrigation, especially under high radiation. However, we did not quantified this phenomenon in the present study.
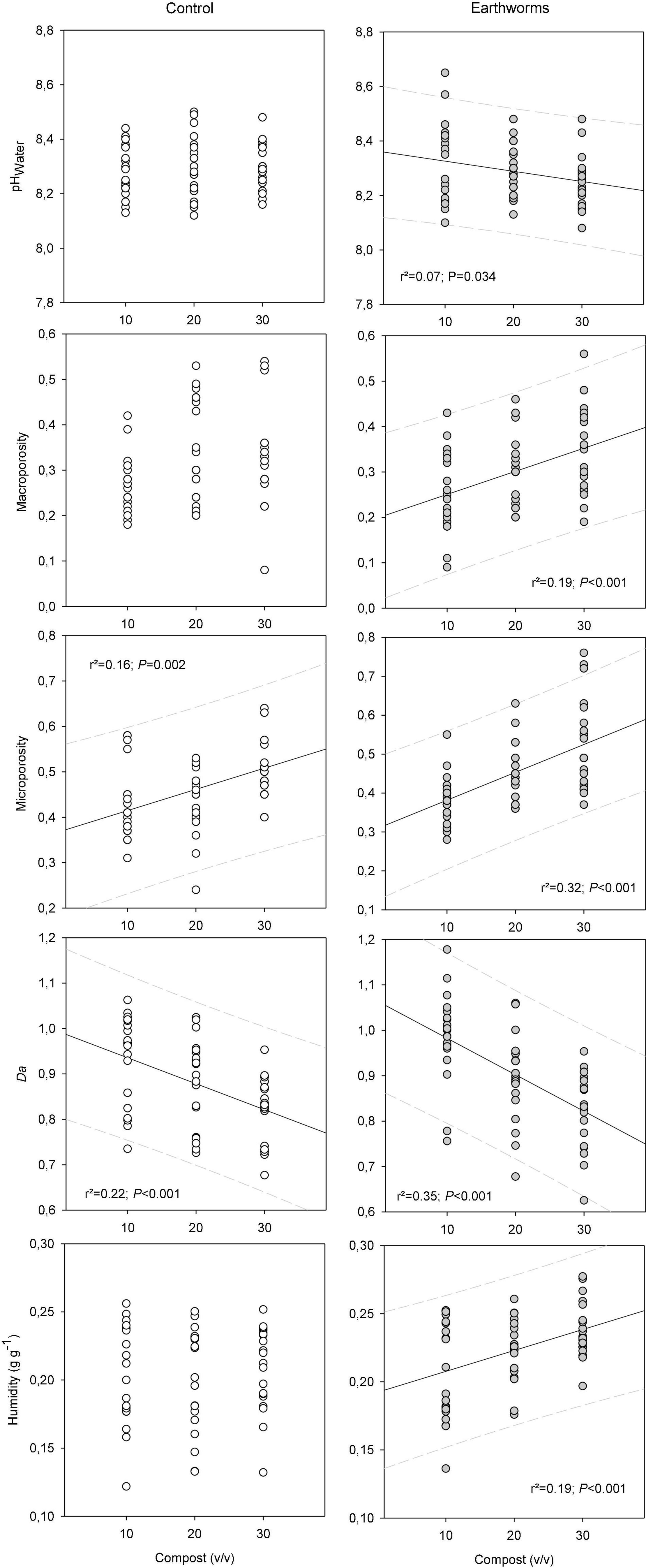
Figure 3. Relationships between soil pH, macro-, micro-porosity, density and humidity and the compost proportion in Technosols with (gray, right) or without (white, left) earthworms. Solid lines represents the linear regression when the model is significant (P < 0.05; n = 60). Dotted-lines represent the 95% confidence intervals.
Discussion
This study clearly demonstrated that compost and earthworms have strong interactive effects on soil fertility and plant growth. As mentioned before, this hypothesis was already tested in laboratory and under controlled conditions with the same materials but a different earthworm’s species (Deeb et al., 2016a,b). However, in situ demonstration of the positive interaction between organic matter addition and earthworms inoculation on soil quality and primary production are not so frequent. In a totally different context, Senapati et al. (1999) showed that a combination of organic matter inputs and earthworms (P. corethrurus), enhance the quality of a tropical soil in India and enhanced the production of tea by 80–276%. The present study confirm that earthworms inoculation can significantly improve the fertility of engineered soils. Here, arugula production was improved two to five times with earthworms in soil containing 30% compost, depending on the season. For comparison, it is estimated that the addition of earthworms in croplands improves yield by about 25% (van Groenigen et al., 2014). Inoculations of earthworms has been shown to accelerate the restoration of degraded soils (Butt, 2008; Edwards et al., 2019). Their role on ecosystem and their beneficial effects on crop systems are widely recognized (Blouin et al., 2013). Earthworms can improve soil structural stability that optimize nutrient cycling and improve plant growth and health (Bertrand et al., 2015). This positive effect on plant production could be due to the hydrostructural structural improvement of the soil as we observed an increased in the microporosity and moisture in the presence of the earthworm, which is in line with previous results obtained in laboratory with the endogenic earthworm species A. caliginosa and L. perenne plant (Deeb et al., 2016b).
In this study, we used L. terrestris, who feeds mainly with organic matter (Griffith et al., 2013) and creates a few large vertical burrows (Bastardie et al., 2003), which are well known for improving water infiltration (Edwards et al., 1990). When the organic matter is not sufficiently available, L. terrestris, tends to go into diapause (Bouché, 1977). This could explain why the effect of earthworm was lower or not significant in soils containing less than 30% of compost. Furthermore, L. terrestris burrowing volume and depth are strongly affected by soil bulk density (Ducasse et al., 2021). Since the bulk density of EDH is about twice that of compost, soils containing 30% compost are significantly less compact than the others, so burrows are probably larger and deeper, which may stimulate both roots and microbial activities, as suggested by the small but significant effect on the pH. If one of these assumptions is true, higher doses of compost may enhance the impact of this earthworm species, which is the most commercially available and best studied species for soil restauration (Steckley, 2020). The benefits of introducing earthworms and adding organic matter show the enormous potential of this approach for the restoration of degraded soils and soil engineering in general. Many more studies should be conducted to evaluate the survival and the potential effects of other earthworm species in combination with other sources of organic matter and taking into account possible toxic effects related to the presence of certain chemical compounds in the soil beneath. To date, very few studies have been carried out to test the influence of different earthworms on the functioning of Technosols (Pey et al., 2013).
A lot of research have been done to develop a quality standard for composted green wastes as amendments, especially in Europe (Carlile, 2008; Barrett et al., 2016). Contrastingly, few studies have investigated the agronomic capacity of mineral waste such as EDH with compost addition. Used alone as growing media, green waste compost provides ideal conditions for soilless agriculture (Hartz et al., 1996; Grard et al., 2018). Lettuce, arugula and spinach usually grow better in soils amended with compost and are even less vulnerable to biological diseases (Maftoun et al., 2005; Giménez et al., 2019). Cultivated during a short period, as in our study, the plants nutrient requirement is relatively low (Grahn et al., 2015) and we expected a rapid response to the compost addition as previously observed in laboratory conditions (Deeb et al., 2016a,b, 2017). That was also the case in this outdoor experiment, but only about 6 months after soils construction. For instance, going from 10 to 30% of compost in the soil mixture allows to multiply by 2 the arugula biomass and even by 4 in the presence of earthworms. Again, the effects of compost are often either enhanced or enhanced by the presence of earthworms, depending on the type of plant or the season. Here, the high amount of carbonates in the mineral materials used may also have limited the mineralization of the organic matter and delayed the nutrients feedback to the plant (Virto et al., 2018). Indeed, organic amendments are less effective in carbonate-rich soils than in non-calcareous soils (Romanyà and Rovira, 2009). In the present study, the soils experienced three different seasons and thus, several wet and dry cycles that conditioned the biological activity carried out by microorganisms and the roots. Both physical and biological phenomena are well-known factors on soil structuring (Oades, 1984; Degens and Sparling, 1995; Chenu and Cosentino, 2011). The stability of structures has probably been reinforced over time, though it is considered short in the pedogenesis scale. Nonetheless, other studies on constructed soils have already highlighted their rapid evolution, particularly those where macrofauna have colonized (Séré et al., 2010; Jangorzo et al., 2014).
Pure mineral materials have a low capacity to support plants development. Here, by simply mixing EDH with organic matter such as compost, we were able to produce an average of nearly half a kilogram of vegetables per m2 across all crops and seasons, including all types of soil with and without earthworms. The constructed soils assured a high rate of germination of all leafy vegetables when climate was wet. However, in the summer when temperature and radiation were elevated, the germination rate of the lettuce was considerably more impacted than the other two plants. This is may be due to the fragility of lettuce seedlings, which may have been more affected by surface crusting quickly in the summer. Crusts formation is a common problem in these soils and the reason is probably the high amount of carbonates in the parent material (Valentin and Bresson, 1998). Moreover, the high pH of the soils may have slowed the root development of all plants (Tang and Turner, 1999), but arugula to a lesser extent, as this plant is less sensitive to soil alkalinity (Garg and Sharma, 2014). These first results encourage the testing of many other crops adapted to this type of soil, including also aromatic plants or flower crops.
Conclusion
In this outdoor study, we demonstrated for the first time that mixtures of excavated deep horizons of soils and green waste compost are able to support the development of green vegetables. After 6 months of soil construction and three growing seasons, we could observe a significant effect of both compost dose and earthworms inoculation on plant production, especially for arugula. These promising results encourage further researches to improve engineered soils for urban agriculture by testing different organic amendments and/or mineral wastes as well as more demanding cultures (e.g., tomatoes or eggplants). Moreover, the impact of different earthworms species on soils restoration is still poorly explored now. It would be interesting to investigate the effect of combining species belonging to different functional groups. Finally, we show in this preliminary study that it was necessary to wait few months to observe a significant effect of compost on soil fertility. Therefore, monitoring these engineered soils over long-term period is a mandatory step to assess their evolution and thus, better inform decision makers about the choice of substrate for urban agriculture.
Data Availability Statement
The raw data supporting the conclusions of this article will be made available by the authors, without undue reservation.
Author Contributions
TZL conceived the study, directed research, performed some statistical analyses. JHRA performed the experiment under the supervision of TZL and CH. AP-B, HA-B, and TD contributed to the analyses. TL and JHRA wrote the article. All authors contributed substantially to revisions.
Funding
The authors thank the French National Research Institute for Sustainable Development (IRD) for its financial support.
Conflict of Interest
The authors declare that the research was conducted in the absence of any commercial or financial relationships that could be construed as a potential conflict of interest.
Publisher’s Note
All claims expressed in this article are solely those of the authors and do not necessarily represent those of their affiliated organizations, or those of the publisher, the editors and the reviewers. Any product that may be evaluated in this article, or claim that may be made by its manufacturer, is not guaranteed or endorsed by the publisher.
Acknowledgments
We thank the IRD for hosting the experiment and for the financial support. Also, we thank all colleagues who participated in the construction of the soils and in the harvest of the salads. The following list is not exhaustive: Hugues Boucher, Emilie Brunet, Sandrine Caquineau, Camille Clovez, Annabelle de Oliveira, Georges Denoni, Marie-Therese D’hier, Caroline Doucouré, Ninon Duval, Véronique Fournier, Laurence Goury, Marie-Christine Layrolle, Françoise Leuiller, Magloire Mandeng-Yogo, Mercedes Mendez, Hana Nabil, Margot Neyret, Thierry Pilorge, Frank Renaud, Camille Ribler, Norbert Silvera, Lotfi Smaili, Brigitte Surugue, and Christine Tournadre.
Supplementary Material
The Supplementary Material for this article can be found online at: https://www.frontiersin.org/articles/10.3389/fevo.2022.884134/full#supplementary-material
Footnotes
References
Barrett, G. E., Alexander, P. D., Robinson, J. S., and Bragg, N. C. (2016). Achieving environmentally sustainable growing media for soilless plant cultivation systems – A review. Sci. Hortic. 212, 220–234. doi: 10.1016/j.scienta.2016.09.030
Bastardie, F., Capowiez, Y., de Dreuzy, J.-R., and Cluzeau, D. (2003). X-ray tomographic and hydraulic characterization of burrowing by three earthworm species in repacked soil cores. Appl. Soil Ecol. 24, 3–16. doi: 10.1016/S0929-1393(03)00071-4
Bertrand, M., Barot, S., Blouin, M., Whalen, J., de Oliveira, T., and Roger-Estrade, J. (2015). Earthworm services for cropping systems. A review. Agron. Sustain. Dev. 35, 553–567. doi: 10.1007/s13593-014-0269-7
Blouin, M., Hodson, M. E., Delgado, E. A., Baker, G., Brussaard, L., Butt, K. R., et al. (2013). A review of earthworm impact on soil function and ecosystem services: earthworm impact on ecosystem services. Eur. J. Soil Sci. 64, 161–182. doi: 10.1111/ejss.12025
Butt, K. R. (2008). Earthworms in Soil Restoration: lessons Learned from United Kingdom Case Studies of Land Reclamation. Restor. Ecol. 16, 637–641. doi: 10.1111/j.1526-100X.2008.00483.x
Cannavo, P., Guénon, R., Galopin, G., and Vidal-Beaudet, L. (2018). Technosols made with various urban wastes showed contrasted performance for tree development during a 3-year experiment. Environ. Earth Sci. 77:650. doi: 10.1007/s12665-018-7848-x
Carlile, W. R. (2008). The use of composted materials in growing media. Acta Hortic. 779, 321–328. doi: 10.17660/ActaHortic.2008.779.39
Chenu, C., and Cosentino, D. (2011). “Microbial regulation of soil structural dynamics,” in The Architecture and Biology of Soils: Life in Inner Space, eds K. Ritz and I. Young (Wallingford: CABI), 37–70. doi: 10.1079/9781845935320.0037
Damas, O., and Coulon, A. (2016). Créer des sols fertiles : du déchet à la végétalisation urbaine. Paris: Editions Le Moniteur, 335.
Decaëns, T., Margerie, P., Aubert, M., Hedde, M., and Bureau, F. (2008). Assembly rules within earthworm communities in North-Western France - a regional analysis. Appl. Soil Ecol. 39, 321–335. doi: 10.1016/j.apsoil.2008.01.007
Deeb, M., Desjardins, T., Podwojewski, P., Pando, A., Blouin, M., and Lerch, T. Z. (2017). Interactive effects of compost, plants and earthworms on the aggregations of constructed Technosols. Geoderma 305, 305–313. doi: 10.1016/j.geoderma.2017.06.014
Deeb, M., Grimaldi, M., Lerch, T. Z., Pando, A., Gigon, A., and Blouin, M. (2016a). Interactions between organisms and parent materials of a constructed Technosol shape its hydrostructural properties. Soil 2, 163–174. doi: 10.5194/soil-2-163-2016
Deeb, M., Grimaldi, M., Lerch, T. Z., Pando, A., Podwojewski, P., and Blouin, M. (2016b). Influence of Organic Matter Content on Hydro-Structural Properties of Constructed Technosols. Pedosphere 26, 486–498. doi: 10.1016/S1002-0160(15)60059-5
Degens, B. P., and Sparling, G. P. (1995). Repeated wet-dry cycles do not accelerate the mineralization of organic C involved in the macro-aggregation of a sandy loam soil. Plant Soil 175, 197–203. doi: 10.1007/BF00011355
Ducasse, V., Darboux, F., Auclerc, A., Legout, A., Ranger, J., and Capowiez, Y. (2021). Can Lumbricus terrestris be released in forest soils degraded by compaction? Preliminary results from laboratory and field experiments. Appl. Soil Ecol. 168:104131. doi: 10.1016/j.apsoil.2021.104131
Edwards, C. A., Arancon, N. Q., and Sherman, R. L. (eds) (2019). Vermiculture technology: earthworms, organic wastes, and environmental management. Boca Raton: CRC press.
Edwards, W. M., Shipitalo, M. J., Owens, L. B., and Norton, L. D. (1990). Effect of Lumbricus terrestris L. burrows on hydrology of continuous no-till corn fields. Geoderma 46, 73–84. doi: 10.1016/0016-7061(90)90008-W
Egendorf, S. P., Cheng, Z., Deeb, M., Flores, V., Paltseva, A., Walsh, D., et al. (2018). Constructed soils for mitigating lead (Pb) exposure and promoting urban community gardening: the New York City Clean Soil Bank pilot study. Landsc. Urban Plan. 175, 184–194. doi: 10.1016/j.landurbplan.2018.03.012
Foti, L., Dubs, F., Gignoux, J., Lata, J.-C., Lerch, T. Z., Mathieu, J., et al. (2017). Trace element concentrations along a gradient of urban pressure in forest and lawn soils of the Paris region (France). Sci. Total Environ. 598, 938–948. doi: 10.1016/j.scitotenv.2017.04.111
Garg, G., and Sharma, V. (2014). Eruca sativa (L.): botanical Description, Crop Improvement, and Medicinal Properties. J. Herbs Spices Med. Plants 20, 171–182. doi: 10.1080/10496475.2013.848254
Giménez, A., Fernández, J. A., Pascual, J. A., Ros, M., López-Serrano, M., and Egea-Gilabert, C. (2019). An agroindustrial compost as alternative to peat for production of baby leaf red lettuce in a floating system. Sci. Hortic. 246, 907–915. doi: 10.1016/j.scienta.2018.11.080
Grahn, C. M., Benedict, C., Thornton, T., and Miles, C. A. (2015). Baby-leaf salad green production guide for western Washington. Pullman, Washington: Washington State University.
Grard, B. J.-P., Chenu, C., Manouchehri, N., Houot, S., Frascaria-Lacoste, N., and Aubry, C. (2018). Rooftop farming on urban waste provides many ecosystem services. Agron. Sustain. Dev. 38:2. doi: 10.1007/s13593-017-0474-2
Griffith, B., Türke, M., Weisser, W. W., and Eisenhauer, N. (2013). Herbivore behavior in the anecic earthworm species Lumbricus terrestris L.? Eur. J. Soil Biol. 55, 62–65. doi: 10.1016/j.ejsobi.2012.12.002
Hartz, T. K., Costa, F. J., and Schrader, W. L. (1996). Suitability of Composted Green Waste for Horticultural Uses. HortScience 31, 961–964. doi: 10.21273/HORTSCI.31.6.961
IUSS Working Group WRB (2014). World Reference Base for Soil Resources. International soil classification system for naming soils and creating legends for soil maps. (World Soil Resources no. 106). Rome: Food and Agricultural Organization of the United Nations.
Jangorzo, N. S., Watteau, F., Hajos, D., and Schwartz, C. (2014). Nondestructive monitoring of the effect of biological activity on the pedogenesis of a Technosol. J. Soils Sediments 15, 1705–1715. doi: 10.1007/s11368-014-1008-z
Lehmann, S. (2011). Optimizing Urban Material Flows and Waste Streams in Urban Development through Principles of Zero Waste and Sustainable Consumption. Sustainability 3, 155–183. doi: 10.3390/su3010155
Lin, B. B., Philpott, S. M., and Jha, S. (2015). The future of urban agriculture and biodiversity-ecosystem services: challenges and next steps. Basic Appl. Ecol. 16, 189–201. doi: 10.1016/j.baae.2015.01.005
Maftoun, M., Moshiri, F., Karimian, N., and Ronaghi, A. M. (2005). Effects of Two Organic Wastes in Combination with Phosphorus on Growth and Chemical Composition of Spinach and Soil Properties. J. Plant Nutr. 27, 1635–1651. doi: 10.1081/PLN-200026005
Magurran A. E. (ed.). (1988). “Why diversity?,” in Ecological diversity and its measurement (Dordrecht: Springer), 1–5. doi: 10.1007/978-94-015-7358-0
Millenium Ecosystem Assessment (2005). Ecosystems and human well-being: synthesis. Washington, DC: World Resources Institute.
Monnier, G., Stengel, P., and Fiès, J. C. (1973). Une methode de mesure de la densite apparente de petits agglomerats terreux. Application a l’analyse des systemes de porosite du sol. Ann. Agron. 24, 533–545.
Morel, J. L., Chenu, C., and Lorenz, K. (2015). Ecosystem services provided by soils of urban, industrial, traffic, mining, and military areas (SUITMAs). J. Soils Sediments 15, 1659–1666. doi: 10.1007/s11368-014-0926-0
Oades, J. M. (1984). Soil organic matter and structural stability: mechanisms and implications for management. Plant Soil 76, 319–337.
Pey, B., Cortet, J., Capowiez, Y., Nahmani, J., Watteau, F., and Schwartz, C. (2014). Technosol composition affects Lumbricus terrestris surface cast composition and production. Ecol. Eng. 67, 238–247. doi: 10.1016/j.ecoleng.2014.03.039
Pey, B., Cortet, J., Watteau, F., Cheynier, K., and Schwartz, C. (2013). Structure of earthworm burrows related to organic matter of a constructed Technosol. Geoderma 202-203, 103–111. doi: 10.1016/j.geoderma.2013.03.010
Pruvost, C., Mathieu, J., Nunan, N., Gigon, A., Pando, A., Lerch, T. Z., et al. (2020). Tree growth and macrofauna colonization in Technosols constructed from recycled urban wastes. Ecol. Eng. 153:105886. doi: 10.1016/j.ecoleng.2020.105886
Romanyà, J., and Rovira, P. (2009). Organic and inorganic P reserves in rain-fed and irrigated calcareous soils under long-term organic and conventional agriculture. Geoderma 151, 378–386. doi: 10.1016/j.geoderma.2009.05.009
Schad, P. (2018). Technosols in the World Reference Base for Soil Resources – history and definitions. Soil Sci. Plant Nutr. 64, 138–144. doi: 10.1080/00380768.2018.1432973
Senapati, B. K., Lavelle, P., Giri, S., Pashanasi, B., Alegre, J., Decaëns, T., et al. (1999). “In-soil earthworm technologies for tropical agroecosystems,” in Earthworm management in tropical agroecosystems, eds P. Lavelle, L. Brussaard, and P. Hendrix (Wallingford: CAB International), 199–237.
Séré, G., Schwartz, C., Ouvrard, S., Renat, J.-C., Watteau, F., Villemin, G., et al. (2010). Early pedogenic evolution of constructed Technosols. J. Soils Sediments 10, 1246–1254. doi: 10.1007/s11368-010-0206-6
Steckley, J. (2020). Cash cropping worms: how the Lumbricus terrestris bait worm market operates in Ontario, Canada. Geoderma 363:114128. doi: 10.1016/j.geoderma.2019.114128
Swilling, M. (2011). Reconceptualising urbanism, ecology and networked infrastructures. Soc. Dyn. 37, 78–95. doi: 10.1080/02533952.2011.569997
Tang, C., and Turner, N. C. (1999). The influence of alkalinity and water stress on the stomatal conductance, photosynthetic rate and growth of Lupinus angustifolius L. and Lupinus pilosus Murr. Aust. J. Exp. Agric. 39, 457–464.
United Nations [UN] (2019). World Urbanization Prospects 2018: Highlights (Population Division No. ST/ESA/SER.A/421). United Nations, Department of Economic and Social Affairs: New York, NY.
Valentin, C., and Bresson, L. M. (1998). “Soil Crusting,” in Methods for Assessment of Soil Degradation, Advances in Soil Science, eds R. Lal, W. H. Blum, C. Valentine, and B. A. Stewart (Boca Raton, Fla: CRC Press), 89–107.
van Groenigen, J. W., Lubbers, I. M., Vos, H. M. J., Brown, G. G., De Deyn, G. B., and van Groenigen, K. J. (2014). Earthworms increase plant production: a meta-analysis. Sci. Rep. 4:6365. doi: 10.1038/srep06365
Virto, I., Antón, R., Apesteguía, M., and Plante, A. (2018). “Role of Carbonates in the Physical Stabilization of Soil Organic Matter in Agricultural Mediterranean Soils,” in Soil Management and Climate Change, eds M. Munoz and R. Zornoza (Amsterdam: Elsevier), 121–136. doi: 10.1016/B978-0-12-812128-3.00009-4
Walsh, D., McRae, I., Zirngibl, R., Chawla, S., Zhang, H., Alfieri, A., et al. (2019). Generation rate and fate of surplus soil extracted in New York City. Sci. Total Environ. 650, 3093–3100. doi: 10.1016/j.scitotenv.2018.09.284
Yep, B., and Zheng, Y. (2019). Aquaponic trends and challenges – A review. J. Clean. Prod. 228, 1586–1599. doi: 10.1016/j.jclepro.2019.04.290
Yilmaz, D., Cannavo, P., Séré, G., Vidal-Beaudet, L., Legret, M., Damas, O., et al. (2018). Physical properties of structural soils containing waste materials to achieve urban greening. J. Soils Sediments 18, 442–455. doi: 10.1007/s11368-016-1524-0
Keywords: constructed technosols, excavated deep soil horizon, green waste compost, earthworm, green vegetables
Citation: Araujo JHR, Pando-Bahuon A, Hartmann C, Aroui-Boukbida H, Desjardins T and Lerch TZ (2022) Making Green(s) With Black and White: Constructing Soils for Urban Agriculture Using Earthworms, Organic and Mineral Wastes. Front. Ecol. Evol. 10:884134. doi: 10.3389/fevo.2022.884134
Received: 25 February 2022; Accepted: 28 April 2022;
Published: 18 May 2022.
Edited by:
Bryant Scharenbroch, University of Wisconsin–Stevens Point, United StatesReviewed by:
Michael McTavish, University of Toronto, CanadaJérôme Cortet, Université Paul Valéry, Montpellier III, France
Copyright © 2022 Araujo, Pando-Bahuon, Hartmann, Aroui-Boukbida, Desjardins and Lerch. This is an open-access article distributed under the terms of the Creative Commons Attribution License (CC BY). The use, distribution or reproduction in other forums is permitted, provided the original author(s) and the copyright owner(s) are credited and that the original publication in this journal is cited, in accordance with accepted academic practice. No use, distribution or reproduction is permitted which does not comply with these terms.
*Correspondence: Thomas Z. Lerch, thomas.lerch@u-pec.fr