- 1Key Laboratory of Southwest China Wildlife Resources Conservation (Ministry of Education), China West Normal University, Nanchong, China
- 2Key Laboratory of Artificial Propagation and Utilization in Anurans of Nanchong City, China West Normal University, Nanchong, China
Sexual dimorphism is regarded as the consequence of differential responses by males and females to selection pressures. Limb muscle plays a very important role during amplexus, which is likely to be under both natural and sexual selection in anurans. Here, we studied the effects of natural and sexual selection on limb muscle mass in males and females across 64 species of anurans. The results showed that there were non-significant differences in relative limb muscle mass between the sexes among species, exhibiting no sexual dimorphism in limb muscle. Absolute and relative limb muscle mass positively displayed correlations with snout-vent length (SVL)for both sexes. However, neither male-biased operational sex ratio (OSR) nor environmental harshness [e.g., coefficient of variation (CV) in temperature and CV in rainfall] can explain relative limb muscle mass (e.g., forelimb, hindlimb, and total limb muscle) within each sex. The findings suggest that environmental harshness and sexual selection cannot play important roles in promoting variations in limb muscle among anuran species.
Introduction
Sexual dimorphism is widespread in nature, and it results from differential responses by males and females to selection pressures (Darwin, 1871; Andersson, 1994; Blanckenhorn, 2005). Most studies have shown that sexual dimorphism evolution arises as a consequence of sexual selection and natural selection (Shine, 1989; Andersson, 1994; Liao et al., 2013a,b; Olarte et al., 2020). On the one hand, sexual selection promotes evolution of the morphology and behavior that enhance opportunities of male mating success (Andersson, 1994; Liao and Lu, 2011). On the other hand, natural selection favors sex-specific adaptations through reducing competition between the sexes for habitats or preys (Shine, 1989; Liao and Chen, 2012; Liao, 2013; Clifton et al., 2020).Under the two selections, females and males diverge in a variety of morphological traits (e.g., body size, head dimensions, body shape, and hind muscles mass) over evolutionary time (Andersson, 1994; Lee and Corrales, 2002; Liao et al., 2015; Zhang et al., 2020; Munoz-munoz et al., 2021).
In anurans, males and females often differ in limb muscle mass as well as body size (Lee and Corrales, 2002; Liao et al., 2012a,b). Limb muscles are important organs associated with amplexus during the male-male competition (Lee, 2001; Liao et al., 2012a,b; Mi, 2012). Stronger forelimb muscle in males can be assumed to produce a relatively larger force where males have an advantageous to clasping the female or repel other rivals (Peters and Aulner, 2000; Liao and Lu, 2011; Mi, 2012; Gastón and Vaira, 2020) while stronger hindlimb muscle are responded to prevent being replaced by another male through kicking other rivals (Lee and Corrales, 2002; Mi, 2013). As a result, a conspicuous sexual dimorphism in fore- and hind-limb muscle mass is expected in frogs where males have much stronger limb muscle than females (Lee, 2001; Liao et al., 2012a; Mi, 2013). However, some studies also reveal that females have stronger hindlimb muscle than males (Liao et al., 2012b; Zhang et al., 2020) while a previous study found a non-significant differences in hindlimb musclemass (Zhang et al., 2013).
Environmental pressures (i.e., resource availability, competition, or predator risk) are considered as a main evolutionary force in shaping the differentiation of organs size, such as brain size, livers, testes mass, digestive tract length and muscles mass between the sexes and among populations (Lüpold et al., 2011; Jiang et al., 2015; Mai et al., 2017, 2019; Zhong et al., 2017; Tang et al., 2018; Zhao et al., 2019; Obregón et al., 2021; Sowersby et al., 2021; Zamora-Camacho, 2021). For instance, variations in food resources availability among different habitats affect energy intake and energy allocation, and thereby leading to differences in digestive tract length, testes mass and limb muscle mass between the sexes among populations (Jin et al., 2016; Yang et al., 2017; Adams et al., 2020; Cai et al., 2020). Meanwhile, because the intensity of sexual selection is positively associated with male-biased operational sex ratio (OSR, calculated as the number of males to the number of fertilizable females in a breeding aggregation at a given time; Emlen and Oring, 1977) among different environmental conditions, the stronger male-male competition is predicted to be related to select for larger limb muscle mass in a toad (Yang et al., 2017). Hence, both natural and sexual selection can promote variations in limb muscle mass in males and females, thus possibly predicting sexual dimorphism in limb muscle in anurans (Yekta and Blackburn, 1992).
In the present study, we investigate the effects of natural and sexual selection on sexual dimorphism in limb muscle mass across 64 species of anurans. We first investigate whether sexual dimorphism in limb muscle mass occurs in anurans. We then investigate whether the limb muscle mass is correlated with body size within each sex. Finally, we investigate whether male-biased OSR and environmental harshness (e.g., variations in mean annual temperature and rainfall) affect the variations in limb muscle mass for both sexes among species.
Materials and Methods
Data Collection
We collected a total of 64 anuran species during the breeding seasons between 2010 and 2020 in China. We sampled each species at a single site using a sampling-lined method. All individuals were captured within each sampling line at night using a 12-V flashlight. We confirmed their sexes through secondary sexual traits (e.g., nuptial pads in males and eggs in females). Sample size per species ranged from 1 to 6 individuals within each sex (Supplementary Table 1). Body size (snout-vent length: SVL) of all individuals was measured to the nearest 0.01 mm with a caliper. All frogs were stored in 4% neutral buffered formalin for dissections. After 2 months, we extracted muscles involving in the clasping of the female by the male during the axillary amplexus (Lee, 2001) and preventing being replaced by another male through kicking other rivals (Lee and Corrales, 2002), including four forelimb muscles (pectoralis series: pectoralis epicoracoides, pectoralis sternalis, pectoralis abdominalis; deltoideus triangularis, triceps branchii, and flexor carpi radialis, Liao et al., 2012a; Diogo and Molnar, 2014) and four hindlimb muscles (sartorius, plantaris longus, triceps femoris, and gastrocnemius, Liao et al., 2012b; Diogo and Ziermann, 2014) from all individuals (Supplementary Table 2). We then dried these muscles using a thermostat drier of 60°C for 48 h before quantifying their dry weight to the nearest 0.1 mg using an electronic balance (Liao et al., 2012a,b).We calculated relative limb muscle mass as a model: RLM = log10(limb muscle mass)/log10(SVL) (Liao et al., 2012a).
We calculated the species-specific male-biased OSR [expressed as the proportion of males among all adult individuals (Liao et al., 2018)] for every sampling line as the number of males divided by the number of females for three nights using marked recapture methods (Mai et al., 2020). Environmental harshness (e.g., variations in average annual temperatureand rainfall) has been hypothesized to affect energy intake and energy allocation, and thus resulting in difference in limb muscle mass in anurans (Yang et al., 2017). Hence, we used coefficient of variation (CV = SD/mean) of temperature and rainfall to measure environmental harshness (Van Woerden et al., 2012; Luo et al., 2017). We calculated CV of temperature and rainfall based on average temperature and rainfall collected at each site in everyday from Chinese Meteorological Stations1 between 2013 and 2017 (Supplementary Table 1).
Phylogeny Reconstruction
To reconstruct the phylogeny for 64 anuran species, weused the sequences of six mitochondrial ribosome genes (CYTB, 12S, 16S, COI, ND2, and ND4) and three nuclear genes (RAG1, TYR, and RHOD) from GenBank [for accession numbers and sequence coverage (see Supplementary Table 3)]. All sequences for each taxon were obtained from the same specimen. For each locus, we aligned the sequences using the multi-sequence alignment (MUSCLE) in MEGA v.10.2.2, and saved the aligned sequences in .fas format (Tamura et al., 2021). We then used the modelTest() function in the phangorn package (Schliep, 2011)in the R 4.1.0 software (R Development Core Team, 2021) based on the corrected Akaike Information Criterion (AICc) to determine the best nucleotide substitution model for each gene. The best nucleotide substitution model was GTR + Γ + I for all genes except RHOD, for which HKY + Γ + I had stronger support, and GTR + Γ + I was thus used as the best substitution model for all genes.
Using BEAUTi and BEAST v.1.8.3 (Drummond et al., 2012; also see details in Jiang et al., 2022), we constructed the phylogeny with unlinked substitution models, a relaxed uncorrelated log-normal clock, a Yule speciation process, and the best-supported nucleotide substitution models. We omitted time calibration due to a lack of fossil dates. We ran the Markov Chain Monte Carlo (MCMC) simulation for 100 million generations where every 10,000th tree was sampled using the BEAST implementation in the CIPRES Science Gateway.2 For all tree statistics, the effective sample size values exceeding 200 showed satisfying convergence of the Bayesian chain and adequate model mixing in the program Tracer v.1.6.0 (Rambaut and Drummond, 2014; Chen et al., 2021a,b). We used TreeAnnotator v.1.8.3 (Drummond et al., 2012) to generate maximum clade credibility trees with mean node heights and a10% burn-in for all species (Supplementary Figure 1).
Statistical Analysis
All analyses were conducted using phylogeny-controlled general least squares (PGLS) models in R statistical version 4.1.0 (R Development Core Team, 2021). In each model, we used the maximum-likelihood estimate of phylogenetic dependence (Pagel’s λ). The scale of λ-values ranges from 0 (i.e., phylogenetic independence) to 1 (i.e., complete phylogenetic non-independence) (Freckleton et al., 2002). First, we applied PGLS models to analyze relationships between limb muscle mass and SVL within each sex using 64 species. Then we ran phylogenetic paired t-test to test differences in mean mass of limb muscle between males and females when controlling for effects of body size of the respective species. Finally, we ran multi-predictor models to test the associations between relative limb muscle mass and either male-biased OSR or environmental harshness controlling for SVL effect. All numeric variables were log10-transformed before analyze to make parameter estimates comparable, and model assumptions were also met.
Results
Body size of females was significantly larger than males (phylogenetic paired t-test: t61 = −3.450, P = 0.001; phylogenetic scaling parameter, λ = 0.074). Total limb mass and forelimb muscle mass of females was significantly heavier than those of males (total limb: t61 = 2.022, P = 0.048, λ = 0.064; forelimb: t61 = 2.357, P = 0.022, λ < 0.001). Hindlimb muscle mass of females tended to be larger than those of males (t61 = 1.978, P = 0.052, λ = 0.071). Forelimb, hindlimb and total limb muscle mass significantly regressed on SVL within each sex (Table 1 and Figure 1). When the influence of SVL was controlled, relative limb muscle mass did not differ between the sexes (total limb: t61 = −1.030, P = 0.307; forelimb: t61 = −0.503, P = 0.617; hindlimb: t61 = −1.257, P = 0.214). However, relative forelimb, hindlimb and total limb muscle mass increased with SVL for both sexes (Table 2 and Figure 2).

Table 1. Relationship between limb muscle mass and body size (SVL) in both males and females among species of anurans.
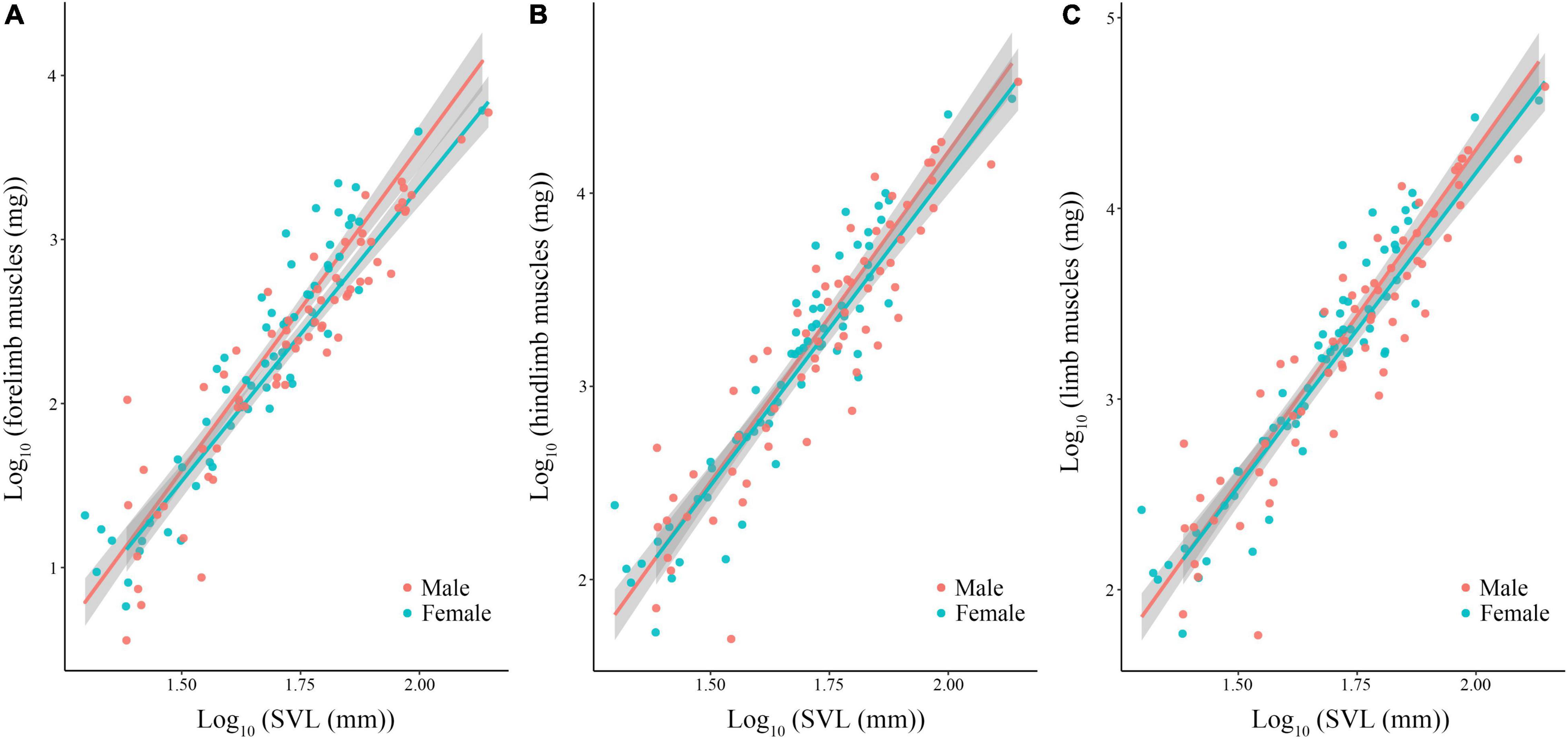
Figure 1. Limb muscle mass [forelimb (A), hindlimb (B), and total limb (C)] regressed on body size (SVL) among species of anurans. Each dot is a species.
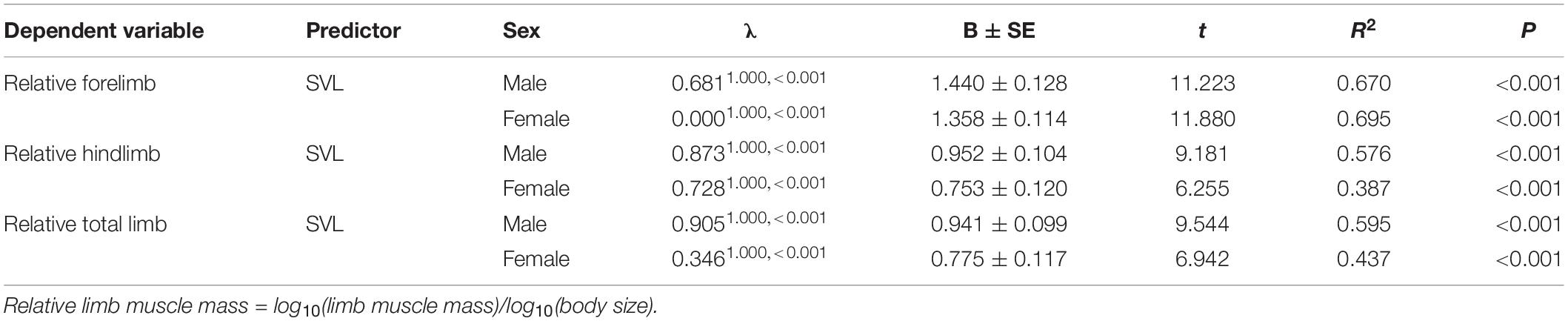
Table 2. Relationship between relative limb muscle mass and body size (SVL) in males and females among species of anurans.
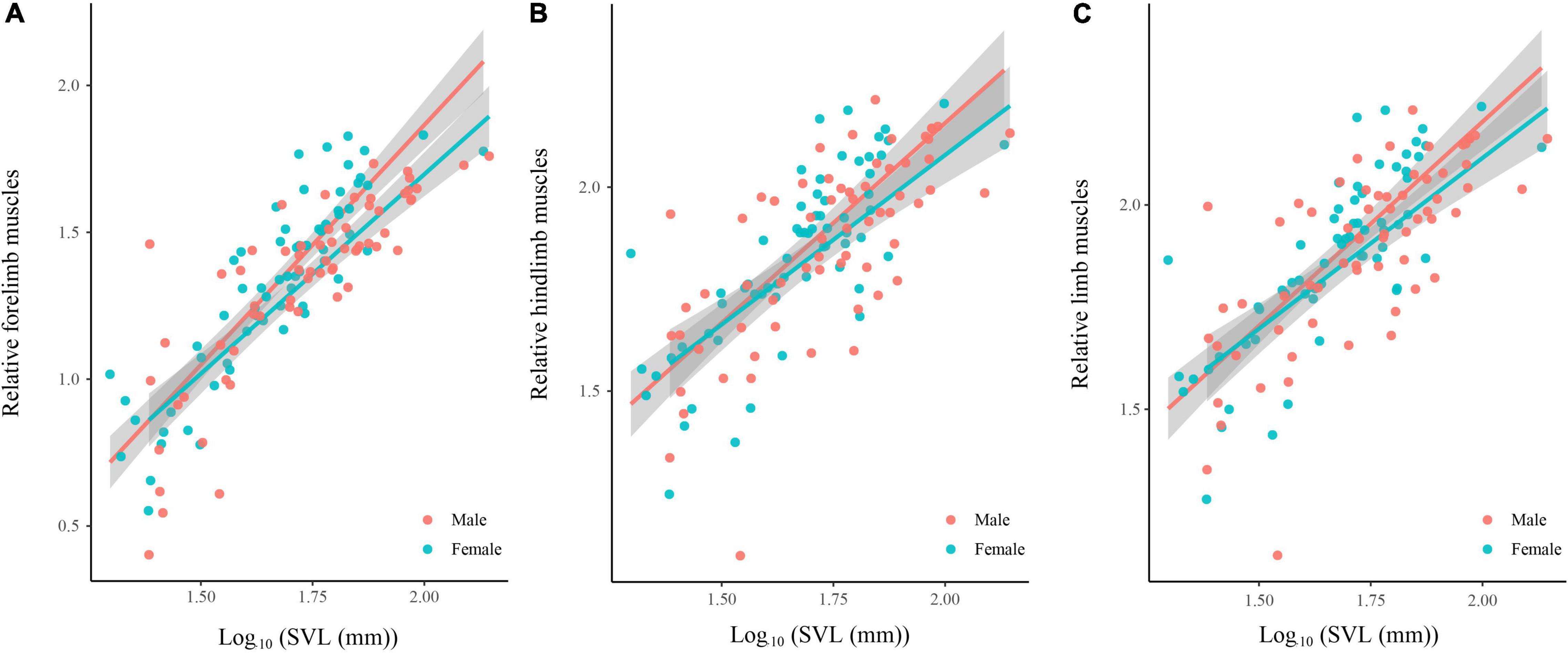
Figure 2. Relative limb muscle mass [forelimb (A), hindlimb (B), and total limb (C)] regressed on body size (SVL) among species of anurans. Relative limb muscle mass = log10(limb muscle mass)/log10(SVL). Each dot is a species.
Phylogeny-controlled general least squares models revealed that relative forelimb, hindlimb and total limb muscle masswere not positively correlated with male-biased OSRin males and females, respectively, when controlling for the effects of SVL and phylogeny (Table 3). Meanwhile, relative limb muscle mass (e.g., forelimb, hindlimb, and total limb) cannot be explained by environmental harshness (e.g., CV in temperature and rainfall) for both sexes when the effects of SVL and phylogeny were controlled (Table 3).
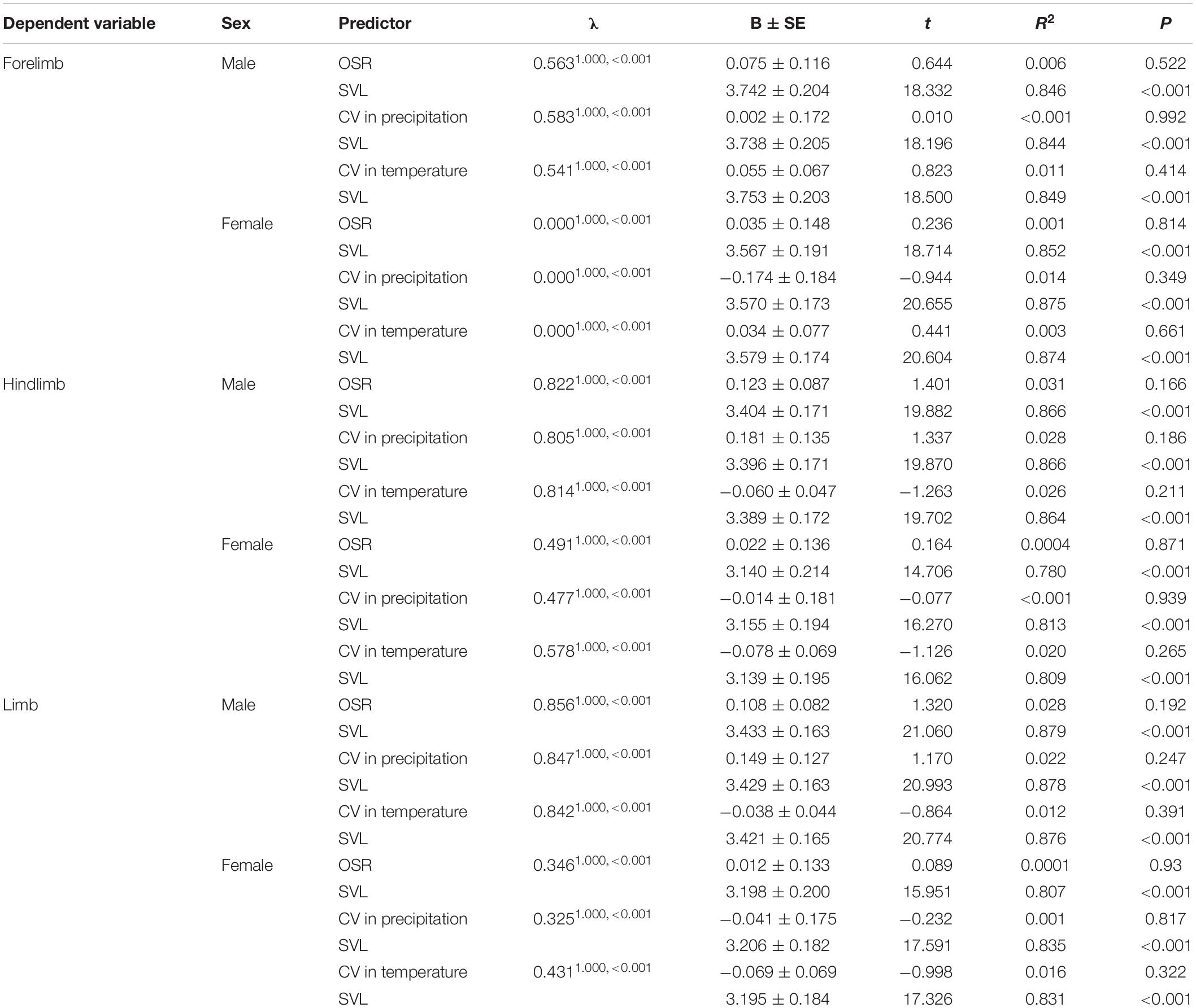
Table 3. Effects of male-biased operational sex ratio (OSR) and environmental harshness[coefficient of variation (CV) in precipitation and coefficient of variation in temperature] on limb muscle mass among species of anurans.
Discussion
Our results demonstrate that absolute and relative forelimb, hindlimb and total limb muscle mass increase with increasing SVL within each sex among 64 species of anurans. There is a lack of sexual dimorphism in relative limb muscle mass although females have larger SVL than males. Moreover, neither male-biased OSR nor environmental harshness can explain variations in relative forelimb, hindlimb and total limb muscle mass for both sexes.
Consistent with the previous studies on limb muscle-SVL relationship in anurans (Lee, 2001; Lee and Corrales, 2002; Liao et al., 2012a,b; Mi, 2012, 2013; Zhang et al., 2013; Mao et al., 2014; Petrović et al., 2017), we found that absolute and relative mass of forelimb, hindlimb and total limb muscle was positively correlated with SVL for both sexes when considering the effects of SVL and phylogeny. This pattern suggested that large-bodied species had heavier limb muscle than small-bodied species because larger-bodied species need more heavier limb muscle to sustain weigher bodies.
For anurans, adult females significantly exceeded adult males in body size in most species (Shine, 1979; Monnet and Cherry, 2002). In this study, we found that females were larger body sizes than males in 53 species, exhibiting a female-biased sexual size dimorphism, which can be result of fecundity selection on female body size (Liao et al., 2013b,2015). Previous studies have shown that sexual dimorphism in limb muscle is probably correlated with their use by the males during amplexus and male-male competition because limb muscle involved in amplexus in larger individuals can produce greater forces (Lee, 2001; Clark and Peters, 2006; Mi, 2012, 2013; Zamora-Camacho, 2018).Our exploratory study revealed that relative limb muscles mass did not differ between males and females, when removing SVL effect. This finding is different from previous studies that sexual dimorphism in limb muscle mass in frogs may be attributable to the importance which those limb muscle help males to act on clasping females and develop to resist attempted take-overs by competing males (Lee, 2001; Liao et al., 2012a; Mi, 2012). Furthermore, a lacking sexual dimorphism in limb muscle mass across 64 species may be attributed to the fact that the main weakness in this study is that all these speciesare considered equally in their mating system and type of amplexus. Indeed, sexual selection favors more robust forearms in a scramble-type mating system and other characteristics are more important in inter and intersexual selection in leks or territorial systems (Andersson, 1994). Hence, it is possible that we cannot detect the importance of the muscle mass of the forearms in those species where it does matter at the time of acquiring a mate. Meanwhile, these species displaying the type of amplexus also affect variations in limb muscle mass because the robust forearms are related to axillary amplexus where the male surrounds the female and holds her firmly (Lee, 2001). In this study, the only forearm muscle used was the flexor carpi radialis. However, other muscles, especially from the forearm, have already been associated to the clasping behavior of males by Gaupp (1896), with clear differences in flexor carpi radialis, abductor indicis longus, and extensor carpi radialis caput superius between sexesfor Pelophylax cf. esculenta. Oka et al. (1984) found that sexual differences in forearm muscles (e.g., flexor antibrachii medialis caput superius, abductor indicis longus, extensor carpi radialis caput superius, and flexor antibrachii lateralis superficialis caput superius) that might also participate in the amplexus in Bufo japonicus. Therefore, forearm muscles in more frogs species should also be tested for sexual dimorphism in similar future studies.
Robust limb muscle benefit males to grasp females firmly and resist take-over attempts from competitors during the male-male competition process, thus promoting male mating success in anurans (Wells, 1977; Howard and Kluge, 1985). Male-biased OSR is positively related to the intensity of male-male competition (Wells, 2007). Consequently, relative limb muscle mass is predicted to be positively correlated with male-biased OSR in frogs and toads. Inconsistent with the prediction that the relative mass of limb muscle should increase with increasing male-biased OSR among different environments (Yang et al., 2017), we found male-biased OSR variation did not display a correlation with relative mass of limb muscle in males among species, suggesting that stronger male-male competition cannot promote relative larger limb muscle mass to avoid competitors from replacing.
Environmental harshness such as CV in temperature and rainfall affects directly active seasons length and food availability in anurans (Wells, 2007; Mai et al., 2019). As a result, species living longer active season and accessing more food resources can grow stronger limb muscle than species living shorter active season and accessing fewer food resources in frogs (Yang et al., 2017). In this study, we found that the mass of forelimb muscle, hindlimb muscle and total combinedlimb muscle was not affected by CV in temperature and rainfall, suggesting that when available food resources is reduced in increased environmental harshness, the rate of survival success mainly depends on decreased active season and increased hibernation to decline energetic reserves of limb muscle in frogs (our unpublished data).
In conclusion, we illustrate the positive association between absolute and relative limb muscle mass and SVL across anuran species. Anuran limb muscle mass does not differ between males and females, thus displaying a lack of sexual dimorphism in limb muscles. Also, variations in limb muscle mass cannot be shaped by neither male-biased OSR nor CV in temperature and rainfall, suggesting that environmental harshness and sexual selection cannot shape variations in limb muscle mass in anurans.
Data Availability Statement
The datasets presented in this study can be found in online repositories. The names of the repository/repositories and accession number(s) can be found in the article/Supplementary Material.
Ethics Statement
The Animal Ethics Committee at China West Normal University approved the sacrifice of animals.
Author Contributions
YJY, YJ, and WBL conceived, wrote, and edited the manuscript. WBL and ZPM secured funding. All authors contributed to editing the article and approved the submitted version.
Funding
This work was supported by the National Natural Sciences Foundation of China (32170429 and 31970393), the Key Project of Science and Technology of Sichuan Province (22NSFSC2743). The reported experiments comply with the current laws of China concerning animal experimentation, and permit to collect amphibians from the Ethical Committee for Animal Experiments in China Council on Animal Care (CCAC) was in hand at the time of sampling.
Conflict of Interest
The authors declare that the research was conducted in the absence of any commercial or financial relationships that could be construed as a potential conflict of interest.
Publisher’s Note
All claims expressed in this article are solely those of the authors and do not necessarily represent those of their affiliated organizations, or those of the publisher, the editors and the reviewers. Any product that may be evaluated in this article, or claim that may be made by its manufacturer, is not guaranteed or endorsed by the publisher.
Acknowledgments
We thank Chunlan Mai, Wei Liu, Shengnan Chen, and Chuan Chen to help collecting samplings in fieldwork.
Supplementary Material
The Supplementary Material for this article can be found online at: https://www.frontiersin.org/articles/10.3389/fevo.2022.879885/full#supplementary-material
Footnotes
References
Adams, D. C., Glynne, E., and Kaliontzopoulou, A. (2020). Interspecific allometry for sexual shape dimorphism: macroevolution of multivariate sexual phenotypes with application to Rensch’s rule. Evolution 74, 1908–1922. doi: 10.1111/evo.14049
Blanckenhorn, W. U. (2005). Behavioral causes and consequences of sexual size dimorphism. Ethology 111, 977–1016.
Cai, L., Yu, J. P., Mai, C. L., and Liao, W. B. (2020). The effects of prey items diversity and digestible materials in stomach on digestive tract length in Hylarana guentheri. Asian Herpetol. Res. 11, 155–160.
Chen, C., Jin, L., Jiang, Y., and Liao, W. B. (2021a). Effects of life histories on genome size variation in squamata. Asian Herpetol. Res. 12, 289–294.
Chen, C., Jiang, Y., Jin, L., and Liao, W. B. (2021b). No evidence for effects of ecological and behavioral factors on eye size evolution in anurans. Front. Ecol. Evol. 9:755818. doi: 10.3389/fevo.2021.755818
Clark, D. L., and Peters, S. E. (2006). Isometric contractile properties of sexually dimorphic forelimb muscle in the marine toad Bufo marinus Linnaeus 1758: functional analysis and implications for amplexus. J. Exp. Biol. 209, 3448–3456. doi: 10.1242/jeb.02396
Clifton, I. T., Chamberlain, J. D., and Gifford, M. E. (2020). Role of phenotypic plasticity in morphological differentiation between water snake populations. Integr. Zool. 15, 329–337. doi: 10.1111/1749-4877.12431
Diogo, R., and Molnar, J. (2014). Comparative anatomy, evolution, and homologies of tetrapod hindlimb muscles, comparison with forelimb muscles, and deconstruction of the forelimbehindlimb serial homology hypothesis. Anat. Rec. 297, 1047–1075. doi: 10.1002/ar.22919
Diogo, R., and Ziermann, J. M. (2014). Development of fore- and hindlimb muscles in frogs: morphogenesis, homeotic transformations, digit reduction, and the forelimbehindlimb enigma. J. Exp. Zool. B Mol. Dev. Evol. 322, 86–105. doi: 10.1002/jez.b.22549
Drummond, A. J., Suchard, M. A., Xie, D., and Rambaut, A. (2012). Bayesian phylogenetics with BEAUti and the BEAST 1.7. Mol. Biol. Evol. 29, 1969–1973. doi: 10.1093/molbev/mss075
Emlen, S. T., and Oring, L. W. (1977). Ecology, sexual selection, and the evolution of mating systems. Science 197, 215–223.
Freckleton, R. P., Harvey, P. H., and Pagel, M. (2002). Phylogenetic analysis and comparative data: a test and review of evidence. Am. Nat. 160, 712–726. doi: 10.1086/343873
Gastón, M. S., and Vaira, M. (2020). Male mating success is related to body condition and stress-induced leukocyte response in an anuran with scramble competition. Can. J. Zool. 98, 391–398.
Gaupp, E. (1896). A. Ecker’s und R. Wiedersheim’s Anatomie des Frosches. I. Braunschweig: Friedrich Vieweg und Sohn.
Howard, R. D., and Kluge, A. G. (1985). Proximate mechanisms of sexual selection in wood frogs. Evolution 39, 260–277. doi: 10.1111/j.1558-5646.1985.tb05665.x
Jiang, A., Zhong, M. J., Xie, M., Lou, S. L., Jin, L., Robert, J., et al. (2015). Seasonality and age is positively related to brain size in Andrew’s toad (Bufo andrewsi). Evol. Biol. 42, 339–348.
Jiang, Y., Chen, C., and Liao, W. B. (2022). Anuran interorbital distance variation: the role of ecological and behavioral factors. Integr. Zool. [Epub ahead of print]. doi: 10.1111/1749-4877.12653
Jin, L., Yang, S. N., Liao, W. B., and Lüpold, S. (2016). Altitude underlies variation in the mating system, somatic condition, and investment in reproductive traits in male Asian grass frogs (Fejervaryalimnocharis). Behav. Ecol. Sociobiol. 70, 1197–1208.
Lee, J. C. (2001). Evolution of a secondary sexual dimorphism in the toad, Bufo marinus. Copeia 2001, 928–935.
Lee, J. C., and Corrales, A. D. (2002). Sexual dimorphism in hind-limb muscle mass is associated with male reproductive success in Bufo marinus. J. Herpetol. 36, 502–505.
Liao, W. B. (2013). Evolution of sexual size dimorphism in a frog obeys the inverse of Rensch’s rule. Evol. Biol. 40, 493–499.
Liao, W. B., and Chen, W. (2012). Inverse Rensch-rule in a frog with female biased sexual size dimorphism. Naturwissenschaften 99, 427–431. doi: 10.1007/s00114-012-0913-5
Liao, W. B., Huang, Y., Zeng, Y., Zhong, M. J., Luo, Y., and Lüpold, S. (2018). Ejaculate evolution in external fertilizers: influenced by sperm competition or sperm limitation? Evolution 72, 4–17. doi: 10.1111/evo.13372
Liao, W. B., Liu, W. C., and Merilä, J. (2015). Andrew meets Rensch: sexual size dimorphism and the inverse of Rensch’s rule in Andrew’s toad (Bufo andrewsi). Oecologia 177, 389–399. doi: 10.1007/s00442-014-3147-8
Liao, W. B., and Lu, X. (2011). Proximate mechanisms leading to large male-mating advantage in the Andrew’s toad, Bufo andrewsi. Behaviour 148, 1087–1102.
Liao, W. B., Wu, Q. G., and Barrett, K. (2012a). Evolution of sexual dimorphism in the fore of Andrew’s toad (Bufo andrewsi) in response to putative sexual selection. Anim. Biol. 62, 83–93.
Liao, W. B., Liao, Y. M., Xiao, W. M., Chen, W., Mi, Z. P., and Li, C. (2012b). Sexual dimorphism in hind limb muscle mass of the Andrew’s Toad (Bufo andrewsi) in relation to sexual selection. N. Western J. Zool. 8, 252–256.
Liao, W. B., Zeng, Y., and Yang, J. D. (2013a). Sexual size dimorphism in anurans: roles of mating system and habitat types. Front. Zool. 10:65. doi: 10.1186/1742-9994-10-65
Liao, W. B., Zeng, Y., Zhou, C. Q., and Jehle, R. (2013b). Sexual size dimorphism in anurans fails to obey Rensch’s rule. Front. Zool. 10:10. doi: 10.1186/1742-9994-10-10
Luo, Y., Zhong, M. J., Huang, Y., Li, F., Liao, W. B., and Kotrschal, A. (2017). Seasonality and brain size are negatively associated in frogs: evidence for the expensive brain framework. Sci. Rep. 7:16629. doi: 10.1038/s41598-017-16921-1
Lüpold, S., Westneat, D. F., and Birkhead, T. R. (2011). Geographical variation in sperm morphology in the red-winged blackbird (Agelaius phoeniceus). Evol. Ecol. 25, 373–390.
Mai, C. L., Liao, W. B., Kotrschal, A., and Lüpold, S. (2020). Relative brain size is predicted by the intensity of intrasexual competition in frogs. Am. Nat. 196, 169–179. doi: 10.1086/709465
Mai, C. L., Liu, Y. H., Jin, L., Mi, Z. P., and Liao, W. B. (2017). Altitudinal variation in somatic condition and reproductive investment of male Yunnan pond frogs (Dianrana pleuraden). Zool. Anz. 266, 189–195.
Mai, C. L., Yu, J. P., and Liao, W. B. (2019). Ecological and geographical reasons for the variation of digestive tract length in anurans. Asian Herpetol. Res. 10, 246–252.
Mao, M., Mi, Z. P., Yang, Z. S., and Zhou, C. Q. (2014). Sexual dimorphism in the of the dark-spotted frog, Pelophylax nigromaculata. Herpetol. J. 24, 147–153.
Mi, Z. P. (2012). Sexual dimorphism in the fore of the Asiatic toad Bufo gargarizans. Herpetol. J. 22, 219–224.
Mi, Z. P. (2013). Sexual dimorphism in the hindlimb muscles of the Asiatic toad (Bufo gargarizans) in relation to male reproductive success. Asian Herpetol. Res. 4, 56–61.
Monnet, J.-M., and Cherry, M. I. (2002). Sexual size dimorphism in anurans. Proc. R. Soc. B 269, 2301–2307.
Munoz-munoz, F., Pages, N., Durao, A. F., England, M., Werner, D., and Talavera, S. (2021). Narrow versus broad: sexual dimorphism in the wing form of western European species of the subgenus Avaritia (Culicoides, Ceratopogonidae). Integr. Zool. 16, 769–784. doi: 10.1111/1749-4877.12516
Obregón, R. L., Scolaro, J. A., Ibargüengoytía, N. R., and Medina, M. (2021). Thermal biology and locomotor performance in Phymaturus calcogaster: are Patagonian lizards vulnerable to climate change?Integr. Zool. 16, 53–66. doi: 10.1111/1749-4877.12481
Oka, Y., Ohtani, R., Satou, M., and Ueda, K. (1984). Sexually dimorphic muscles in the forelimb of the Japanese toad, Bufo japonicus. J. Morphol. 180, 297–308.
Olarte, O., Sanchez-Montes, G., and Martinez-Solano, I. (2020). Integrative demographic study of the Iberian painted frog (Discoglossus galganoi): inter-annual variation in the effective to census population size ratio, with insights on mating system and breeding success. Integr. Zool. 15, 498–510. doi: 10.1111/1749-4877.12452
Peters, S. E., and Aulner, D. A. (2000). Sexual dimorphism in forelimb muscles of the bullfrog, Rana catesbeiana: a functional analysis of isometric contractile properties. J. Exp. Biol. 203, 3639–3654. doi: 10.1242/jeb.203.23.3639
Petroviæ, T. G., Vukov, T. D., and Kolarov, N. T. (2017). Sexual dimorphism in size and shape of traits related to locomotion in nine anuran species from Serbia and Montenegro. Folia Zool. 66, 11–21.
R Development Core Team (2021). R: A Language and Environment for Statistical Computing. Vienna: R Foundation for Statistical Computing.
Rambaut, A., and Drummond, A. (2014). Tracer v1.6. Available online at: http://tree.bio.ed.ac.uk/software/tracer/ (accessed December 16, 2021).
Schliep, K. P. (2011). Phangorn: phylogenetic analysis in R. Bioinformatics27, 592–593. doi: 10.1093/bioinformatics/btq706
Shine, R. (1989). Ecological causes for the evolution of sexual dimorphism: a review of the evidence. Q. Rev. Biol. 64, 419–461. doi: 10.1086/416458
Sowersby, W., Eckerström-Liedholm, S., Kotrschal, A., Näslund, J., Rowiǹski, P., Gonzalez-Voyer, A., et al. (2021). Fast life-histories are associated with larger brain size in killifishes. Evolution 75, 2286–2298. doi: 10.1111/evo.14310
Tamura, K., Stecher, G., and Kumar, S. (2021). MEGA11: molecular evolutionary genetics analysis version 11. Mol. Biol. Evol. 38, 3022–3027. doi: 10.1093/molbev/msab120
Tang, T., Luo, Y., Huang, C. H., Liao, W. B., and Huang, W. C. (2018). Variation in somatic condition and testis mass in Feirana quadranus along an altitudinal gradient. Anim. Biol. 68, 277–288.
Van Woerden, J. T., Willems, E. P., van Schaik, C. P., and Isler, K. (2012). Large brains buffer energetic effects of seasonal habitats in catarrhine primates. Evolution 66, 191–199. doi: 10.1111/j.1558-5646.2011.01434.x
Wells, K. D. (2007). The Ecology and Behavior of Amphibians. Chicago, IL: University of Chicago Press.
Yang, S. N., Liao, W. B., Zhong, M. J., and Huang, X. F. (2017). Geographical variation in limb muscle mass of the Andrew’s toad (Bufo andrewsi). Anim. Biol. 67, 17–28.
Yekta, N., and Blackburn, D. (1992). Sexual dimorphism in mass and protein content of the forelimb muscles of the northern leopard frog Rana pipiens. Can. J. Zool. 70, 670–674.
Zamora-Camacho, F. J. (2018). Locomotor performance in a running toad: roles of morphology, sex and agrosystem versus natural habitat. Biol. J. Linn. Soc. 123, 411–421.
Zamora-Camacho, F. J. (2021). Sex and habitat differences in size and coloration of an amphibian’s poison glands match differential predator pressures. Integr. Zool. [Epub ahead of print]. doi: 10.1111/1749-4877.12597
Zhang, L., Yang, J., Chen, X., Zhao, Y., and Lu, X. (2013). No sexual dimorphism in limb muscles of a frog not engaging in amplexus. Anim. Biol. 63, 397–405.
Zhang, L. X., Yuan, X. Y., Sheng, Y. S., Yu, F., Zhong, X. T., Chen, X. H., et al. (2020). Strong limb tactics of the Boulenger’s Lazy toad, Scutiger boulengeri: inferred from limb muscleslimb muscle. Asian Herpetol. Res. 11, 360–364.
Zhao, L., Mai, C. L., Liu, G. H., and Liao, W. B. (2019). Altitudinal implications in organ size in the Andrew’s toad (Bufo andrewsi). Anim. Biol. 69, 365–376.
Keywords: anurans, limb muscles, operational sex ratio, selection pressure, sexual dimorphism
Citation: Yang YJ, Jiang Y, Mi ZP and Liao WB (2022) Testing the Role of Environmental Harshness and Sexual Selection in Limb Muscle Mass in Anurans. Front. Ecol. Evol. 10:879885. doi: 10.3389/fevo.2022.879885
Received: 20 February 2022; Accepted: 10 May 2022;
Published: 26 May 2022.
Edited by:
Diego Baldo, CONICET Institute of Subtropical Biology (IBS), ArgentinaReviewed by:
Virginia Abdala, IBNCONICET-UNT, ArgentinaPaulo Pinheiro, Universidade de São Paulo, Brazil
Copyright © 2022 Yang, Jiang, Mi and Liao. This is an open-access article distributed under the terms of the Creative Commons Attribution License (CC BY). The use, distribution or reproduction in other forums is permitted, provided the original author(s) and the copyright owner(s) are credited and that the original publication in this journal is cited, in accordance with accepted academic practice. No use, distribution or reproduction is permitted which does not comply with these terms.
*Correspondence: Wen Bo Liao, TGlhb2JvXzBfMEAxMjYuY29t
†These authors have contributed equally to this work