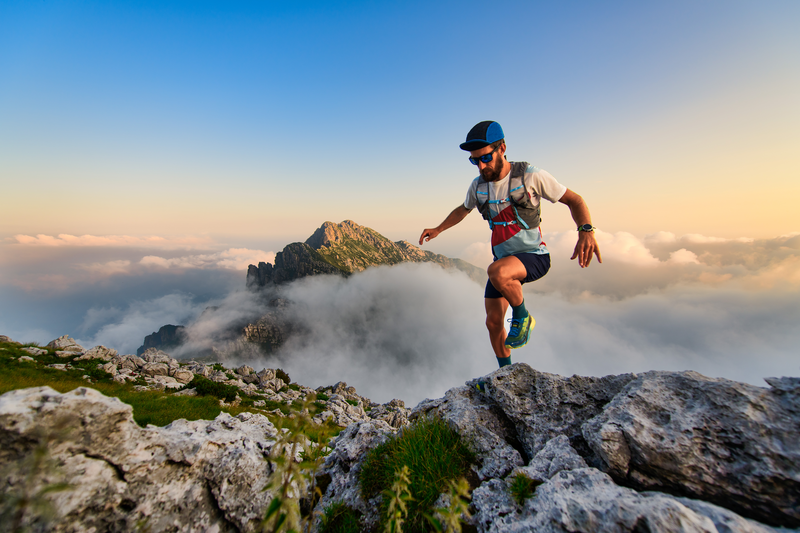
95% of researchers rate our articles as excellent or good
Learn more about the work of our research integrity team to safeguard the quality of each article we publish.
Find out more
REVIEW article
Front. Ecol. Evol. , 11 April 2022
Sec. Chemical Ecology
Volume 10 - 2022 | https://doi.org/10.3389/fevo.2022.876596
This article is part of the Research Topic Methods in Chemical Ecology: 2022/23 View all 8 articles
Despite their comparatively small brains, insects are able to survive and thrive in their environment. In the past, it was thought that insects are driven mainly by their instincts. However, today it is well established that they possess unique abilities to learn and use their experience in future decisions. Like many higher animals insects are able to acquire and retain information on when and where to forage, which mate to choose, where to lay their eggs and how to navigate in complex habitats. Learning can be surprisingly fast with only one single encounter with a suitable food source or oviposition site shaping an insect's preference for up to a lifetime. In this review, we discuss the scope and limits of insect learning, focusing in specific on olfactory learning, and we raise the question whether currently used learning paradigms in artificial lab set-ups are able to answer all ecologically relevant questions.
“This tiny insect has a brain?!” There is hardly any researcher working on insect behavior, neurobiology or cognition that hasn't heard this sentence. We patiently answer, that yes indeed, insects have a central nervous system and are able to learn, remember past experiences, and navigate in complex environments that require the integration of different sensory modalities (In less scientific jargon for the non-scientific community). Research of the past years has shown that insects not only possess short-, mid- and long-term memory, but also are capable of learning concepts, innovating, transmitting preferences from one generation to the next or learning by observing others (Leadbeater and Chittka, 2007; Avarguès-Weber and Giurfa, 2013; Giurfa, 2013; Chittka et al., 2019; Lihoreau et al., 2019). These abilities are important in all areas of life of the insect such as foraging, social interactions, reproductive behavior, and predator avoidance (Dukas, 2008; Dion et al., 2019). Since several reviews already cover the history and recent developments of insect cognition and learning, this will not be part of our review. For more information on these topics see: Dukas (2008), Giurfa (2013), Giurfa (2015), and Lihoreau et al. (2019). Here, we focus on olfactory-driven learning and how insects are able to use their unique cognitive abilities to learn essential information (for survival) in often as little as a single trial.
When insects interact with their environment, they constantly acquire and store new information. However, only if the information is retained over a longer period of time and results in a corresponding change of the insect's behavior, this is regarded as learning and memory (Papaj and Prokopy, 1989). Neither the size of the insect's brain nor the relatively short life span should, however, be considered constraints when it comes to learning. Studies in the past have shown that learning is possible even with a small neuronal network (Van Lenteren and Bakker, 1975; Laverty, 1980; Hammer and Menzel, 1995; Keene and Waddell, 2007) and the process of learning itself might not take more than a few seconds or minutes, which will still give the animal ample opportunity to utilize the newly acquired knowledge during its (short) lifetime (Dukas, 2008). When thinking of insect learning, fruit flies (Drosophila melanogaster) and honeybees (Apis mellifera) are small, but well-established insect model organisms for learning and memory (Davis, 2005; Giurfa and Sandoz, 2012; Menzel, 2012). Still, there are insects with even more minuscule brains that are able to learn: Parasitoid wasps are among the smallest insects on earth, with brains measuring only a few cubic micrometers but are, nevertheless, able to associate odors with a reward after as little as one trial (Van Der Woude et al., 2018). In the wild, these tiny insects use their ability to learn odors to recognize the olfactory bouquet of damaged plants that indicate their herbivorous hosts (Haverkamp and Smid, 2020).
In contrast to humans, many insects heavily rely on olfactory rather than visual information to learn new things about their environment. The main advantage is that odors can be perceived over greater distances than visual cues and that they can be traced back to the source even when the view of the source is obstructed. Therefore, insects often utilize them to navigate toward foraging, oviposition or nest sites. Desert ants (Cataglyphis fortis), for example, navigate successfully in the arid environment of the Tunisian saltpans. Although they are known to use path integration and visual cues to find their way in the vast landscape, they also rely on olfactory landmarks to locate their nest or promising food sources (Knaden and Graham, 2016). Odors do also play a role in social interactions of insects: Honeybees communicate with conspecifics through pheromones, use olfaction to locate flowers and communicate olfactory information about possible food sources with other bees in the hive (Paoli and Galizia, 2021). Blowing learned odors into the bee hive is enough to trigger the bees to fly out and search for food at foraging sites that have previously been associated with this specific odor (Reinhard et al., 2004). Apart from using olfaction to navigate the environment and localize food sources, odors can also be learned and remembered in a mating context. Male fruit flies (D. melanogaster) are able to learn which mates to choose and to connect odor information to the receptivity of the other sex. Males transfer their pheromone cis-vaccenyl acetate (cVA) to females during mating. Other male flies innately show reduced courtship toward these freshly mated females as those now emit the male pheromone cVA. However, if the male additionally experiences rejection by a mated female emitting cVA, male courtship behavior is subsequently further reduced, suggesting that the innate courtship inhibition of cVA can be further enhanced by learning (Keleman et al., 2012).
Olfactory memory can be categorized in two main categories: Appetitive memory and aversive memory (Figure 1). Appetitive memory is formed if a conditioned stimulus (CS), i.e., the odor, is associated with a positive unconditioned stimulus (US) like a sugar reward. Aversive memory is formed if the CS is associated with a negative US such as an electric shock or a bitter compound (Kahsai and Zars, 2011). Both types of memory are key for the survival of a species as it is important to approach possible food sources (appetitive) and avoid dangerous substances such as toxins (aversive). To better understand where such associations are formed, let us briefly introduce the detection and early processing of olfactory information in the insect brain. When smelling odors, insects detect the volatile compounds with fine structures, so-called “sensilla”, on their antennae and maxillary palps. These sensilla house sensory neurons expressing odorant receptors that determine to which odorant(s) a given neuron will respond to. When an odorant binds to a fitting receptor, the neuron becomes activated and the signal is sent from the antennae or maxillary palps to the central brain of the insect, where it is being processed. The first processing center of the brain is the antennal lobe (AL). From there the odor information is passed on by projection neurons (PNs) to higher brain regions like the mushroom body (MB) or the lateral horn (LH). While the latter is known to mainly drive innate olfactory behavior, the MB acts as a coincidence detector that helps the insect to learn to associate a given odor with a reward or punishment (Carey and Carlson, 2011). The mushroom body output neurons (MBONs) which are connected to the MB control the approach or avoidance of an olfactory cue, with dopaminergic neurons (DANs) encoding the positive or negative valence of a stimulus (Cognigni et al., 2018). Recent work from Eschbach et al. (2021) in D. melanogaster has further shown that there are connections between the neurons of the LH and MBONs and that downstream of the MBONs a distinct subset of convergence neurons (CNs) is integrating innate and learned valences. Depending on which valence is higher, the insect will show different behaviors. That means that even though a naïve insect might perceive an odor as innately attractive, there is the possibility to alter this innate preference by increasing the negative valence of the odor through learning (e.g., through punishment while smelling the odor). This in turn will alter the insect's behavior from approach to avoidance.
Figure 1. Learning of appetitive and aversive (olfactory) cues in the lab and in the field. Appetitive-Lab setting: Honeybees (A. mellifera) learn to extend their proboscis when an odor (CS; gray cloud) is supplied in the presence of sugar water (US). Aversive-Lab setting: Fruit flies (D. melanogaster) learn to avoid an odor (CS) when perceiving the odor together with an electric shock (US). Appetitive-Ecology: Hawkmoths (Manduca sexta) quickly remember the smell of flowers (CS) that provide a nectar reward (US). Aversive—Ecology: The ectatomine ant Ectatomma tuberculatum learns to quickly escape the net (CS) of the golden silk spider Nephila clavipes (US) which would try to prey on the ant. Since the ant (CS) has a strong bite (US), the spider in turn learns to avoid getting near the ant if it is caught in its net.
When it comes to storing information, four different types of memory have been described: Short-term memory (STM), mid-term memory (MTM), long-term memory (LTM), and anesthesia-resistant memory (ARM) (Dezazzo and Tully, 1995). The difference between LTM and ARM is that LTM formation is associated with new protein synthesis after training and can be disrupted by amnesia-inducing agents while ARM is/cannot (Tully et al., 1994). Recent studies on D. melanogaster have shown that the transcription factor Apterous (Ap) is needed for memory consolidation, that is the formation of a stable memory (Inami et al., 2021), and that memory consolidation requires neuronal activity beyond the learning experience (Cognigni et al., 2018). Inami et al. (2021) have further shown that both Ap and its cofactor Chip (Chi) are required for the maintenance of LTMs in the MB. When looking at the neuronal circuits involved, acquisition and expression of very early memories take place in the so-called γ neurons of the MB. For ARM and LTM formation the vertical lobes of the MB, α' and β' or α and β, respectively, play the most important role (Guven-Ozkan and Davis, 2014). The exact coding of the memories seems, however, to be more complex than first suggested and might include several feedback loops, some of which still need to be elucidated (Cognigni et al., 2018).
In the past it was believed that in order for LTMs to form, the insect has to experience CS and US together multiple times and that these experiences also have to be spaced apart over time (Dezazzo and Tully, 1995). This view is, however, being challenged by a mounting body of evidence that insects are able to form LTMs after just a single trial.
One of the most important model organisms to shed light on different types of memory and the corresponding expression of related genes is the fruit fly (D. melanogaster). It offers the necessary tool kit to connect genetic information with the activity of neuronal networks and behavior (Davis, 2005). Classical (Pavlovian) aversive olfactory conditioning is usually conducted with groups of flies. These flies are exposed to two different odors, one odor (CS+) is paired with an electric shock (US), and the other odor (CS–) is not. After exposure, the flies are tested in a T-maze where they have to choose between the two different odors (Tully and Quinn, 1985). In D. melanogaster one trial learning was thought to lead to the retention of memory for up to a few hours only (Keene and Waddell, 2007). Also, olfactory LTM was thought to require 10 spaced trials, in 15 min intervals, with the CS (odor) being presented at the same time as the US (electric shock) (Figure 2) (Tully et al., 1994). Several recent studies on fruit fly (D. melanogaster) memory and behavior have drawn a quite different picture when it comes to the formation of LTM.
Figure 2. Classical conditioning vs. one trial learning. Classical conditioning: In classical olfactory conditioning with D. melanogaster one odor (CS+) is presented together with an electric stimulus (US) and another odor (CS–) is presented without US. Generally, several (e.g., 10) interspaced trials are conducted in order for the insect to from an LTM and learn to associate the CS+ with the US. One trial learning: In contrast to classical conditioning, one trial learning only requires one experience of the CS+ together with the US in order for the insect to show LTM formation. The example shows the desert ant Cataglyphis fortis, that after only once experiencing an innately neutral odor together with a food crumb, will target this odor immediately on consecutive foraging runs (Huber and Knaden, 2018).
In their study, Krashes and Waddell (2008) could show that using a new appetitive conditioning protocol, LTM could be formed after a single trial. The authors exposed starved flies to an odor without sugar reward followed by another odor with sugar reinforcement. Flies were then further starved until testing (but had access to water). Olfactory memory for the reinforced odor persisted until 36 h. As most of the flies perished after that (presumably of starvation), longer memory retention could not be assessed, but seems very likely. Especially so, since protein synthesis inhibitor lowered memory retention, which shows that LTM was indeed formed. What is important to know about the authors' findings is that if flies were fed between training and testing, memory performance went down drastically. This indicates that motivation is a key aspect in memory retention since continuously starved flies performed very well during the memory test.
Motivation also seems to be the key aspect in water-reward memory in D. melanogaster. Water-reward LTM (wLTM) can be formed after a single trial and lasts more than 24 h. It is associated with de novo protein synthesis and CREB-mediated gene transcription in the α and β surface and γ dorsal neurons of the MB (Lee et al., 2020). Serotonergic neurons called dorsal paired medial (DPM) neurons modulate MBON neural activity in order to consolidate wLTM in the fly brain (Lee et al., 2021). However, only thirsty flies remember an odor (CS) presented together with a water reward (US). Since food-starved flies do not remember odors that are presented together with water, the motivation needed for memory formation also seems to be specific for the circumstance (Lin et al., 2014). D. melanogaster larvae are also able to form associations between an odor (CS) and a sugar reward (US) after a single trial. In contrast to the adult flies, they do, however, not form LTMs, but only STMs. It has no influence whether the US is a sugar reward that is sweet and nutritious (fructose), solely sweet (arabinose) or solely nutritious (sorbitol) (Weiglein et al., 2019). This raises the question how the ecology of the larvae is playing into their ability to store and retain information. Given that the larvae spend their whole life until pupation most likely in the same fruit, it might not be of importance for the larvae to associate a food odor with the fruit's sugar content. When looking at aversive cues, Weiglein et al. (2019) found that aversive STM is only formed for high-concentration sodium chloride but not for a bitter tastant (quinine). Again, here the ecology of the larvae could play a role. Adult D. melanogaster females are known to detect bitter compounds with their labellum and legs and lay their eggs in overripe fruit low in bitter compounds (Dweck et al., 2021). Hence, if the larvae never leave the fruit they hatched in, they do not need to assess the palatability of the fruit in terms of bitterness. Desiccation is, in contrast, a serious threat to a fly larva. Therefore, learning possible desiccation cues like an odor (CS) associated with high-concentration sodium chloride (US) might be essential for survival.
Also, in the second important insect model used for learning and memory, the honeybee, different types of memory have been thoroughly investigated. As in D. melanogaster, LTM was thought to require a long consolidation phase and multiple spaced trials. Ecologically speaking, it was argued, this might assure that only very important memories get stored. For example when a bee encounters the same rewarding flower species multiple times during a foraging bout (Menzel, 1999). Still, one-trial learning has already been mentioned as a side note in a very early publication: Takeda (1961) observed that when using the classical appetitive olfactory conditioning approach, some honeybees showed the proboscis extension reflex (PER) after a single trial. A more recent study has shed light on this discrepancy in information.
In their study, Villar et al. (2020) could show that honeybees were underestimated when it comes to their ability to form LTMs. The authors found that a single appetitive training trail can induce LTM that persists several days and that protein-synthesis-dependent memories are formed as early as 4 h after the trial. So why do the authors see such a drastic difference to previous appetitive conditioning protocols? Honeybees are usually captured at the hive entrance, or worse, taken directly out of the beehive for testing. That means there is no control for whether the bee is a forager, builder, nurse or cleaner in the hive. Yet, only foragers are highly motivated to find and learn food sources. To control for selecting only true foragers, Villar et al. (2020) caught foragers at the feeder to ensure foraging motivation. The authors only performed experiments when the field conditions allowed for high motivation in foragers. That means they did not run experiments during bad weather, winter or periods of Asian-hornet predation. Further, a novel olfactometer was used that allowed a more precise temporal control of the stimulus presentation and thereby significantly reduced odor generalization.
The knowledge about memory formation in these model organisms is of incomparable value. Still, there seems to be more to the acquisition and retention of olfactory memories than can be shown with strict classical olfactory conditioning. Especially if one looks at the diversity of insect species and their different ecologies, the question arises if “one shoe fits all.”
Different insect species, even when phylogenetically closely related, can exhibit different learning rates and abilities (Smid et al., 2007). The closely related wasp species pair Cotesia glomerata and Cotesia rubecula show natural differences in foraging behavior. While C. glomerata is a gregarious generalist that lays up to 20 eggs in its host Pieris brassicae, the solitary C. rubecula is considered a specialist and only deposits one single egg into its host Pieris rapae. Cotesia glomerata usually encounters many of its hosts in a single plant, making the encounter highly predictable, while C. rubecula only will find one host per plant and has to search a variable environment for its host species. Of course, this means that the predictability of a host encounter is very different for both species. Obviously, this has led to very different learning abilities: While C. glomerata is able to form LTM after a single trial, C. rubecula needs three trials that are temporally spaced apart to form LTM. The speed of memory consolidation also varies drastically between the two species. While C. glomerata only takes 4 h to consolidate LTM, C. rubecula takes up to 3 days (Smid et al., 2007). That does not mean that one species is a “bad” and the other one a “good learner.” It just means that different learning types have to be seen in an ecological context. If the environment is highly unpredictable, long-lasting memories might not be an advantage. In this case, short lasting memories that are updated continuously, or even an active form of forgetting, can be adaptive. The same goes in the other direction. If the environment is very stable and predictable, long lasting memories are more advantageous (Smid and Vet, 2016).
The ecology of the insect should also be considered when it comes to which type of cues are learned or in which context the cue is learned. The desert ant Cataglyphis fortis is known for its survival in the arid salt pans of North Africa where food is scarce and ants have to run far to find it. That means if the ant encounters a food item it is of high importance to learn how it smells quickly in order to be able to recognize possible similar food sources from afar (Figure 2). The ant also needs to be able to remember different types of food odors since it cannot always predict which type of food it is going to find on each consecutive foraging trips. There are times where e.g., cicadas or flying ant sexuals are quite abundant, but there are also times where hardly any dead insect found will be the same. Huber and Knaden (2018) could show that C. fortis is able to remember a food odor after only one experience, can remember at least 14 consecutively learned food odors, and can remember odors for up to a lifetime. Interestingly, the nest odor is learned completely differently by the species. It takes repeated learning trials to remember and is quickly forgotten if the odor is not present at the nest anymore (Huber and Knaden, 2018). This means that the same ant species learns the connection between an odor (CS) and a specific US in one trial during food search, but is incapable of this in another context, i.e., during nest search. What also needs to be thought of is that insects have different predispositions in terms of which cues they are able to learn easily and which cues a more difficult for them to learn. This type of learning has been described as “prepared learning” (Smid and Vet, 2016). In their study on the evolution of prepared learning Dunlap and Stephens (2014) could show that fruit flies (D. melanogaster) show increased sensitivity to (odor) cues that had been reliable over 40 generations than to cues that were not. This indicates that learning reliable cues can be adaptive for an insect and that these cues can become imperative to a species over generations.
In the past there have been disagreements on how fast information can be learned and whether LTM can be formed after a single experience. Like in the examples mentioned above, one trial learning has been shown in several other invertebrate species: In the snail Lymnaea stagnalis, LTM is formed after a single trial in a foraging situation. The snail is able to associate the organic compound amyl acetate with a food reward. Interestingly, in this invertebrate LTM formation can only be blocked in a short time window 10 min to 1 h after training, which points to a very rapid memory consolidation process (Fulton et al., 2005). This is an important finding, since it means that LTMs can be formed very fast and consolidated quickly in order to ensure survival. This should be especially important when it comes to predator avoidance. We argue that learning the danger of a predator quickly and within a single trial could be highly adaptive for a species. That one trial learning of predator avoidance is actually possible has been shown in an ecologically relevant study by Henaut et al. (2014). The golden silk spider Nephila clavipes and the ectatomine ant Ectatomma tuberculatum are found in the same Neotropical habitat and are known to regularly encounter each other on the same plants (Figure 1). If an ant falls off a leave, the possibility is high that it gets trapped in the net of the arachnid. Since the ant possesses strong mandibles and a sting, this puts the spider in a dangerous situation. Experiments showed that if the spider is bitten by the ant only once, it will suppress its catching response and avoid this ant species in the future. If tested, it is also able to discriminate successfully between the ant and a non-threatening prey (fruit fly) item after a single trial. Similarly, the ant learns to escape the web of the spider more quickly if once entangled in it. It learns to cut the less sturdy threats of the web and gets out of the trap more efficiently (Henaut et al., 2014).
Also learning environmental cues that indicate valuable foraging or oviposition sites quickly can be key to survival and successful reproduction. When looking at insects in specific, we could show that the hawkmoth Manduca sexta is not only able to associate a neutral odor with a sugar reward after only one trial when visiting a flower (Adam et al., 2021), but it is further able to remember a suitable host plant for oviposition after only one visit to the leaf of this plant (Nataraj et al., 2021). The parasitic wasp Lariophagus distinguendus also easily learns to connect an artificial odor with the presence of its host (i.e., its oviposition site). As in the hawkmoth Manduca sexta and the above mentioned Cotesia glomerata only one trial is needed to learn where to oviposit (Collatz et al., 2006). Similarly, a single conditioning trial is enough for the parasitic wasps Trichogramma evanescence and Nasonia vitripennis to learn an odor that indicates the presence of their hosts. While memory retention does not vary in small and large conspecifics of T. evanescence, smaller wasps of N. vitripennis have lower levels of memory retention than larger individuals, indicating cognitive costs in brain scaling (Van Der Woude et al., 2018). Despite fast retention of memory, learned information should, however, also be susceptible to fast alterations in case environmental factors change. Balderrama (1980) could show that the American cockroach (Periplaneta americana) is able to learn to reverse its odor preference within one trial. When tested, the cockroach shows no significant decay of this LTM over an interval of 1–7 days. This indicates that important memories are kept for long time periods if not a lifetime, but can be over-written with more salient information in case it proves adaptive.
In the past insect learning and memory has been underestimated. This was in part due to how learning and memory experiments were conducted under artificial lab conditions. Refining the experimental set-ups in the lab as well as designing research studies in an ecologically relevant framework has shown that insects are indeed able to learn salient olfactory information in as little as one trial. We argue that it should be highly adaptive for a species to learn olfactory cues indicating predators, food sources or oviposition sites quickly. Therefore, one trial learning and quick LTM formation should be found in more insect species. We hypothesize that even the model organism D. melanogaster may be able to form long-term memories within one trial when tested in an ecologically relevant context. We think that considering the insects' ecology and motivation when designing experiments to test for one trial learning is key (Table 1). Also keeping in mind that some cues are easier to learn for one species than for another is important. These cues might even vary between life stages of the given species since insects can change their ecology quite drastically over their life span. In summary, one trial olfactory learning could be a general trait in insects and we look forward to more studies in this field.
EA: writing—original draft. BH and MK: writing—review and editing and supervision. BH: funding acquisition. All authors contributed to the article and approved the submitted version.
This study was supported by Max Planck Society.
The authors declare that the research was conducted in the absence of any commercial or financial relationships that could be construed as a potential conflict of interest.
All claims expressed in this article are solely those of the authors and do not necessarily represent those of their affiliated organizations, or those of the publisher, the editors and the reviewers. Any product that may be evaluated in this article, or claim that may be made by its manufacturer, is not guaranteed or endorsed by the publisher.
Adam, E., Hansson, B. S., and Knaden, M. (2021). Moths sense but do not learn flower odors with their proboscis during flower investigation. J. Exp. Biol. 224:jeb242780. doi: 10.1242/jeb.242780
Avarguès-Weber, A., and Giurfa, M. (2013). Conceptual learning by miniature brains. Proc. R. Soc. B Biol. Sci. 280:20131907. doi: 10.1098/rspb.2013.1907
Balderrama, N. (1980). One trial learning in the American cockroach, Periplaneta americana. J. Insect Physiol. 26, 499–504. doi: 10.1016/0022-1910(80)90123-7
Carey, A. F., and Carlson, J. R. (2011). Insect olfaction from model systems to disease control. Proc. Natl. Acad. Sci. U.S.A. 108, 12987–12995. doi: 10.1073/pnas.1103472108
Chittka, L., Giurfa, M., and Riffell, J. A. (2019). Editorial: the mechanisms of insect cognition. Front. Psychol. 10:2751. doi: 10.3389/fpsyg.2019.02751
Cognigni, P., Felsenberg, J., and Waddell, S. (2018). Do the right thing: neural network mechanisms of memory formation, expression and update in Drosophila. Curr. Opin. Neurobiol. 49, 51–58. doi: 10.1016/j.conb.2017.12.002
Collatz, J., Müller, C., and Steidle, J. L. (2006). Protein synthesis-dependent long-term memory induced by one single associative training trial in the parasitic wasp Lariophagus distinguendus. Learn. Mem. 13, 263–266. doi: 10.1101/lm.192506
Davis, R. L. (2005). Olfactory memory formation in Drosophila: from molecular to systems neuroscience. Annu. Rev. Neurosci. 28, 275–302. doi: 10.1146/annurev.neuro.28.061604.135651
Dezazzo, J., and Tully, T. (1995). Dissection of memory formation: from behavioral pharmacology to molecular genetics. Trends Neurosci. 18, 212–218. doi: 10.1016/0166-2236(95)93905-d
Dion, E., Monteiro, A., and Nieberding, C. M. (2019). The role of learning on insect and spider sexual behaviors, sexual trait evolution, and speciation. Front. Ecol. Evol. 6:225. doi: 10.3389/fevo.2018.00225
Dukas, R. (2008). Evolutionary biology of insect learning. Annu. Rev. Entomol. 53, 145–160. doi: 10.1146/annurev.ento.53.103106.093343
Dunlap, A. S., and Stephens, D. W. (2014). Experimental evolution of prepared learning. Proc. Nat. Acad. Sci. 111, 11750–11755. doi: 10.1073/pnas.1404176111
Dweck, H., Talross, G., Wang, W., and Carlson, J. (2021). Evolutionary shifts in taste coding in the fruit pest Drosophila suzukii. Elife 10:e64317. doi: 10.7554/eLife.64317
Eschbach, C., Fushiki, A., Winding, M., Afonso, B., Andrade, I. V., Cocanougher, B. T., et al. (2021). Circuits for integrating learned and innate valences in the insect brain. Elife 10:e62567. doi: 10.7554/eLife.62567
Fulton, D., Kemenes, I., Andrew, R. J., and Benjamin, P. R. (2005). A single time-window for protein synthesis-dependent long-term memory formation after one-trial appetitive conditioning. Eur. J. Neurosci. 21, 1347–1358. doi: 10.1111/j.1460-9568.2005.03970.x
Giurfa, M. (2013). Cognition with few neurons: Higher-order learning in insects. Trends Neurosci. 36, 285–294. doi: 10.1016/j.tins.2012.12.011
Giurfa, M. (2015). Learning and cognition in insects. Wiley Interdiscip. Rev. Cogn. Sci. 6, 383–395. doi: 10.1002/wcs.1348
Giurfa, M., and Sandoz, J. C. (2012). Invertebrate learning and memory: fifty years of olfactory conditioning of the proboscis extension response in honeybees. Learn. Mem. 19, 54–66. doi: 10.1101/lm.024711.111
Guven-Ozkan, T., and Davis, R. L. (2014). Functional neuroanatomy of Drosophila olfactory memory formation. Learn. Memory 21, 519–526. doi: 10.1101/lm.034363.114
Hammer, M., and Menzel, R. (1995). Learning and memory in the honeybee. J. Neurosci. 15, 1617–1630. doi: 10.1523/JNEUROSCI.15-03-01617.1995
Haverkamp, A., and Smid, H. M. (2020). A neuronal arms race: the role of learning in parasitoid–host interactions. Curr. Opin. Insect Sci. 42, 47–54. doi: 10.1016/j.cois.2020.09.003
Henaut, Y., Machkour-M'rabet, S., and Lachaud, J. P. (2014). The role of learning in risk-avoidance strategies during spider-ant interactions. Anim. Cogn. 17, 185–195. doi: 10.1007/s10071-013-0651-x
Huber, R., and Knaden, M. (2018). Desert ants possess distinct memories for food and nest odors. Proc. Natl. Acad. Sci. U.S.A. 115, 10470–10474. doi: 10.1073/pnas.1809433115
Inami, S., Sato, T., Kurata, Y., Suzuki, Y., Kitamoto, T., and Sakai, T. (2021). Consolidation and maintenance of long-term memory involve dual functions of the developmental regulator Apterous in clock neurons and mushroom bodies in the Drosophila brain. PLoS Biol. 19:e3001459. doi: 10.1371/journal.pbio.3001459
Kahsai, L., and Zars, T. (2011). “Learning and memory in drosophila: behavior, genetics, and neural systems,” in International Review of Neurobiology, ed N. Atkinson (London, UK: Academic Press), 139–167. doi: 10.1016/B978-0-12-387003-2.00006-9
Keene, A. C., and Waddell, S. (2007). Drosophila olfactory memory: single genes to complex neural circuits. Nat. Rev. Neurosci. 8, 341–354. doi: 10.1038/nrn2098
Keleman, K., Vrontou, E., Krüttner, S., Yu, J. Y., Kurtovic-Kozaric, A., and Dickson, B. J. (2012). Dopamine neurons modulate pheromone responses in Drosophila courtship learning. Nature 489, 145–149. doi: 10.1038/nature11345
Knaden, M., and Graham, P. (2016). The sensory ecology of ant navigation: From natural environments to neural mechanisms. Annu. Rev. Entomol. 61, 63–76. doi: 10.1146/annurev-ento-010715-023703
Krashes, M. J., and Waddell, S. (2008). Rapid consolidation to a radish and protein synthesis-dependent long-term memory after single-session appetitive olfactory conditioning in Drosophila. J. Neurosci. 28, 3103–3113. doi: 10.1523/JNEUROSCI.5333-07.2008
Laverty, T. M. (1980). The flower-visiting behaviour of bumble bees: floral complexity and learning. Can. J. Zool. 58, 1324–1335. doi: 10.1139/z80-184
Leadbeater, E., and Chittka, L. (2007). Social learning in insects: From miniature brains to consensus building. Curr. Biol. 17, R703–R713. doi: 10.1016/j.cub.2007.06.012
Lee, W.-P., Chiang, M.-H., Chang, L.-Y., Lee, J.-Y., Tsai, Y.-L., Chiu, T.-H., et al. (2020). Mushroom body subsets encode CREB2-dependent water-reward long-term memory in Drosophila. PLoS Genet. 16:e1008963. doi: 10.1371/journal.pgen.1008963
Lee, W. P., Chiang, M. H., Chang, L. Y., Shyu, W. H., Chiu, T. H., Fu, T. F., et al. (2021). Serotonin signals modulate mushroom body output neurons for sustaining water-reward long-term memory in Drosophila. Front. Cell Dev Biol. 9:755574. doi: 10.3389/fcell.2021.755574
Lihoreau, M., Dubois, T., Gomez-Moracho, T., Kraus, S., Monchanin, C., and Pasquaretta, C. (2019). “Chapter One - Putting the ecology back into insect cognition research,” in Advances in Insect Physiology, ed R. Jurenka (London, UK: Academic Press), 1–25. doi: 10.1016/bs.aiip.2019.08.002
Lin, S., Owald, D., Chandra, V., Talbot, C., Huetteroth, W., and Waddell, S. (2014). Neural correlates of water reward in thirsty Drosophila. Nat. Neurosci. 17, 1536–1542. doi: 10.1038/nn.3827
Menzel, R. (1999). Memory dynamics in the honeybee. J. Comparat. Physiol. A 185, 323–340. doi: 10.1007/s003590050392
Menzel, R. (2012). The honeybee as a model for understanding the basis of cognition. Nat. Rev. Neurosci. 13, 758–768. doi: 10.1038/nrn3357
Nataraj, N., Adam, E., Hansson, B. S., and Knaden, M. (2021). Host plant constancy in ovipositing Manduca sexta. J. Chem. Ecol. 47, 1042–1048. doi: 10.1007/s10886-021-01309-3
Paoli, M., and Galizia, G. C. (2021). Olfactory coding in honeybees. Cell Tissue Res. 383, 35–58. doi: 10.1007/s00441-020-03385-5
Papaj, D. R., and Prokopy, R. J. (1989). Ecological and evolutionary aspects of learning in phytophagous insects. Annu. Rev. Entomol. 34, 315–350. doi: 10.1146/annurev.en.34.010189.001531
Reinhard, J., Srinivasan, M. V., and Zhang, S. (2004). Scent-triggered navigation in honeybees. Nature 427, 411–411. doi: 10.1038/427411a
Smid, H. M., and Vet, L. E. M. (2016). The complexity of learning, memory and neural processes in an evolutionary ecological context. Curr. Opin. Insect Sci. 15, 61–69. doi: 10.1016/j.cois.2016.03.008
Smid, H. M., Wang, G., Bukovinszky, T., Steidle, J. L. M., Bleeker, M. A. K., Van Loon, J. J. A., et al. (2007). Species-specific acquisition and consolidation of long-term memory in parasitic wasps. Proc. R. Soc. B Biol. Sci. 274, 1539–1546. doi: 10.1098/rspb.2007.0305
Takeda, K. (1961). Classical conditioned response in the honey bee. J. Insect Physiol. 6, 168–179. doi: 10.1016/0022-1910(61)90060-9
Tully, T., Preat, T., Boynton, S. C., and Del Vecchio, M. (1994). Genetic dissection of consolidated memory in Drosophila. Cell 79, 35–47. doi: 10.1016/0092-8674(94)90398-0
Tully, T., and Quinn, W. G. (1985). Classical conditioning and retention in normal and mutant Drosophila melanogaster. J. Comparat. Physiol. A 157, 263–277. doi: 10.1007/BF01350033
Van Der Woude, E., Huigens, M. E., and Smid, H. M. (2018). Differential effects of brain size on memory performance in parasitic wasps. Anim. Behav. 141, 57–66. doi: 10.1016/j.anbehav.2018.05.011
Van Lenteren, J. C., and Bakker, K. (1975). Discrimination between parasitised and unparasitised hosts in the parasitic wasp Pseudeucoila bochei: a matter of learning. Nature 254, 417–419. doi: 10.1038/254417a0
Villar, M. E., Marchal, P., Viola, H., and Giurfa, M. (2020). Redefining single-trial memories in the honeybee. Cell Rep. 30, 2603–2613.e3. doi: 10.1016/j.celrep.2020.01.086
Keywords: insect learning, one trial learning, ecology, insect cognition, insect memory, olfaction, olfactory learning
Citation: Adam E, Hansson BS and Knaden M (2022) Fast Learners: One Trial Olfactory Learning in Insects. Front. Ecol. Evol. 10:876596. doi: 10.3389/fevo.2022.876596
Received: 15 February 2022; Accepted: 21 March 2022;
Published: 11 April 2022.
Edited by:
Stefan Dötterl, University of Salzburg, AustriaReviewed by:
Sufang Zhang, Research Institute of Forest Ecology, Environment and Protection, Chinese Academy of Forestry, ChinaCopyright © 2022 Adam, Hansson and Knaden. This is an open-access article distributed under the terms of the Creative Commons Attribution License (CC BY). The use, distribution or reproduction in other forums is permitted, provided the original author(s) and the copyright owner(s) are credited and that the original publication in this journal is cited, in accordance with accepted academic practice. No use, distribution or reproduction is permitted which does not comply with these terms.
*Correspondence: Markus Knaden, bWtuYWRlbkBpY2UubXBnLmRl
†ORCID: Elisabeth Adam orcid.org/0000-0003-4382-8994
Bill S. Hansson orcid.org/0000-0002-4811-1223
Markus Knaden orcid.org/0000-0002-6710-1071
Disclaimer: All claims expressed in this article are solely those of the authors and do not necessarily represent those of their affiliated organizations, or those of the publisher, the editors and the reviewers. Any product that may be evaluated in this article or claim that may be made by its manufacturer is not guaranteed or endorsed by the publisher.
Research integrity at Frontiers
Learn more about the work of our research integrity team to safeguard the quality of each article we publish.