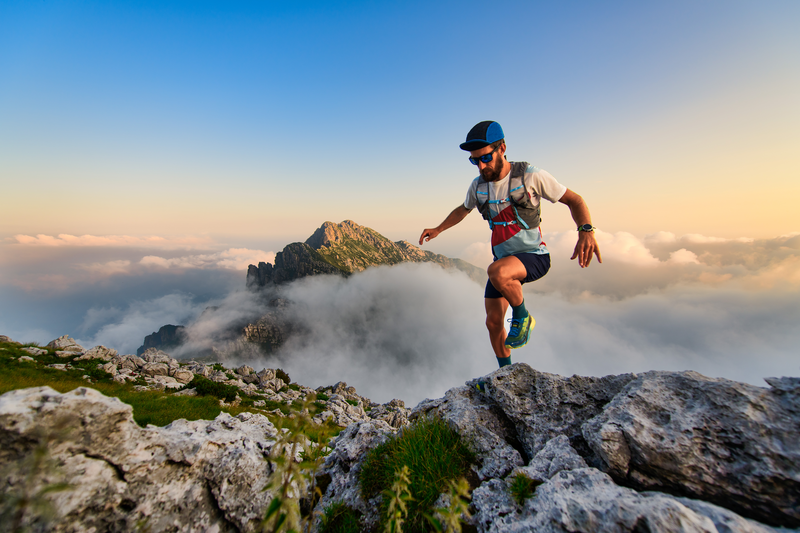
95% of researchers rate our articles as excellent or good
Learn more about the work of our research integrity team to safeguard the quality of each article we publish.
Find out more
ORIGINAL RESEARCH article
Front. Ecol. Evol. , 26 April 2022
Sec. Ecophysiology
Volume 10 - 2022 | https://doi.org/10.3389/fevo.2022.874276
This article is part of the Research Topic Ecological and Evolutionary Relevance of Phenotypic Plasticity in a Changing World View all 7 articles
Predation is a key driver of phenotypic diversification with prey having evolved sets of correlated anti-predator traits. Changes in anti-predator traits can be studied on an evolutionary as well as on a developmental timescale. Using a common garden setup, we studied inter- and intraspecific correlations of behavioural and morphological defences in four damselfly species that either occur in habitats dominated by predatory fish (fish habitats) or fishless habitats by raising larvae either with predatory fish or in a control treatment. We found inter- as well as intraspecific trait compensation (negative correlations) between behavioural and morphological defences. Compared to fishless habitat species, fish habitat species invested more in behavioural defences and less in morphological defences. This was mirrored by fish habitat species investing more in behavioural defences and less in morphological defences when reared with predatory fish whereas fishless habitat species invested less in morphological defences only. Our results emphasise the role of context-specific combinations of defensive traits to avoid predation. We suggest, considering changes in multiple correlated traits on different timescales when studying the evolution of anti-predator traits.
Predators are well-known to structure phenotypic abundance and occurrence as well as to generate species divergence (Wellborn et al., 1996; Vamosi, 2005; Schluter, 2009; Piovia-Scott et al., 2017). In response to the strong selective impact of predation, prey evolved a multitude of defensive traits to avoid and evade predation (Edmunds, 1974; Tollrian and Harvell, 1999). It is apparent that prey animals typically recruit sets of correlated behavioural and morphological anti-predator traits to avoid predation [reviewed in DeWitt and Langerhans (2003) and Bourdeau and Johansson (2012)]. Trait correlations among defensive traits have been studied in aquatic organisms like snails [e.g., Cotton et al. (2004), Bourdeau (2009), Brönmark et al. (2012), and Ahlgren et al. (2015)], odonates [e.g., Mikolajewski and Johansson (2004) and Mikolajewski et al. (2010)], amphibians [e.g., Hossie et al. (2017) and Pacheco et al. (2019)], and fish [e.g., Hulthén et al. (2014), Kern et al. (2016), and Marshall and Wund (2017)], as well as terrestrial organisms such as moths (Vogelweith et al., 2014), snakes (Mayer et al., 2016), and rodents (Agnani et al., 2020). Functionally and mechanically independent behavioural and morphological defences can either be negatively (trait compensation) or positively (trait cospecialization) correlated (DeWitt et al., 1999; DeWitt and Langerhans, 2003). In the case of trait cospecialization, prey applies both traits simultaneously to enhance the overall defence. In the case of trait compensation, prey applies only one trait, most likely to trade-off costs of defensive traits (DeWitt et al., 1999; DeWitt and Langerhans, 2003).
Habitats differ in occurrence of predators, resulting in well-distinguished predator gradients (Gunzburger and Travis, 2004; Sorace and Gustin, 2009; Renner et al., 2012; Mori and Saitoh, 2014). The expression of behavioural and morphological defences strongly depends on the current predation regime (Laurila et al., 2004; Relyea, 2004a; Bourdeau, 2009; Mikolajewski et al., 2010; Marshall and Wund, 2017). Such different phenotypes could for instance arise through developmental plasticity [i.e., intraspecific differences; e.g., Relyea (2001), Hoverman and Relyea (2009), and Herzog et al. (2016)] or can be the result of evolutionary changes [i.e., interspecific differences; e.g., Mikolajewski et al. (2006), Stoks and McPeek (2006), Langerhans (2009), and Jiang and Mikolajewski (2018)]. In cases where closely related species occupy different levels of the predator gradient, we are able to study inter- and intraspecific phenotypic changes using different predator treatments in a common garden experiment and by this to compare developmental with evolutionary timescales (Mikolajewski et al., 2015a).
Because different predator species exhibit distinct hunting modes and foraging styles, selection on prey phenotypes varies along predator gradients (Werner and McPeek, 1994; McPeek et al., 1996; Mikolajewski et al., 2006; Stoks and McPeek, 2006; Marchinko, 2009). The freshwater predator gradient is an excellent model system to study predator-related changes in correlated defensive traits. Permanent freshwater habitats exhibit a well-described predator gradient from habitats being dominated by predatory fish (hereafter referred to as “fish habitat”) and habitats that lack predatory fish [hereafter referred to as “fishless habitat”; Wellborn et al. (1996)]. Because predatory fish are highly efficient visual predators, selection on morphological and behavioural traits in fishless habitats [that are usually dominated by predatory invertebrates like large dragonfly larvae, notonectids, and beetle larvae; Wellborn et al. (1996)], is often considerably reduced in comparison to fish habitats (Stoks and De Block, 2000; Mikolajewski et al., 2015a).
Here, we investigate correlations of behavioural and morphological anti-predator traits in four species of the damselfly genus Coenagrion. Two species mainly occur in fish habitats whereas the other two species occur mainly in fishless habitats (Kuhn and Burbach, 1998; Dijkstra and Lewington, 2006). Furthermore, previous research showed that Coenagrion damselfly larvae can detect and respond to chemical cues of predatory fish (Chivers et al., 1996). Thus, by rearing larvae of all four species either with or without predatory fish, we were able to study inter- as well as intraspecific trait correlations. As a behavioural trait we quantified larval activity. Reducing activity is a common strategy in damselfly larvae to avoid detection and encounters with predatory fish (McPeek, 1990; Stoks and Johansson, 2000; Strobbe et al., 2011), but is costly because of reduced encounters with food and decelerated growth rate (Stoks et al., 2005a,b; Biro et al., 2006). As a morphological trait, we measured larval caudal lamellae size. Larval damselflies use their caudal lamellae to evade invertebrate predators by accelerating burst escape swimming (McPeek et al., 1996; McPeek, 1997; Stoks et al., 1999; Stoks and De Block, 2000; Strobbe et al., 2010). However, burst escape swimming does not operate against predatory fish as larval burst escape swimming is not fast enough to evade predatory fish (Convey, 1988; Stoks and De Block, 2000; Gyssels and Stoks, 2005). As a result, damselfly species occurring in fish habitats express smaller caudal lamellae (McPeek, 1995; McPeek et al., 1996).
Comparing fish habitat species with fishless habitat species, we expected that (a) fishless habitat species invest less into behavioural defences than fish habitat species and by this show higher activity (see above). Furthermore, we expected that (b) fish habitat species invest less into morphological defences than fishless habitat species, and by this, express smaller caudal lamellae (see above). This would result in interspecific trait compensation of behavioural and morphological defences comparing fish habitat species with fishless habitat species. Comparing larvae of one species raised under the fish treatment with larvae raised under the control treatment, we expected that (c) only fish habitat species would plastically reduce activity in the fish treatment. Further, we expected that (d) only fish habitat species would develop smaller lamellae in the fish treatment. Thus, we also predicted intraspecific trait compensation of behavioural and morphological defences within fish habitat species but not within fishless habitat species. Prediction (c) and (d) are based on previous findings that showed that freshwater prey might show reduced or even lack responses to allopatric predator species (Richardson, 2001; Stoks et al., 2003; Åbjörnsson et al., 2004; Jiang and Mikolajewski, 2018).
In this study we focus on four European Coenagrion species [Coenagrion lunulatum (Charpentier, 1840), Coenagrion mercuriale (C., 1840), Coenagrion ornatum (Selys, 1850), and Coenagrion pulchellum (Vander Linden, 1825)] that occupy permanent waters (Dijkstra and Lewington, 2006). All four species emerge in early May (Sternberg and Buchwald, 1999) with females laying eggs during late spring and summer. Larvae hatch approximately 2–3 weeks after oviposition. Larvae overwinter and finish development in the following year. Species show distinct differences with regards to predator occurrence. Whereas C. lunulatum and C. pulchellum can dominantly be found in small permanent ponds lacking predatory fish, C. mercuriale and C. ornatum dominantly occupy low order streams that contain small predatory fish species such as three-spined sticklebacks, Gasterosteus aculeatus and European perch, Perca fluviatilis (Sternberg and Buchwald, 1999).
To test for differences between fish habitat species and fishless habitat species as well as the effect of presence/absence of predatory fish on trait correlations, we reared larvae of all four species in a full factorial experiment. Therefore, egg clutches of all four species were collected in June 2017 following the method described in Mikolajewski et al. (2015b) [n = 4; 4; 7 and 8 for C. mercuriale, C. ornatum, C. pulchellum, and C. lunulatum, respectively; sampling location for C. mercuriale and C. ornatum: Artern (51°43′ N, 11°23′ E); sampling location for C. pulchellum and C. lunulatum: Wilmersdorfer Soll (58°86′ N, 42°95′ E)]. Egg clutches were stored separately in opaque plastic containers (7 × 4 × 10 cm) filled to 8 cm with aged tap-water. All containers were kept in a climate room at 21°C and a day-night light cycle corresponding to beginning of June. Larvae of all four species hatched after ∼2 weeks. Larvae received Artemia nauplii twice daily ad libitum until they were separated. The rearing experiment took place in six black 90 L plastic containers filled with 85 L of aged-tap-water (Mikolajewski et al., 2008). Half of the containers contained one perch, P. fluviatilis (body length approx. 10 cm). Perch is a well-known predator of odonate larvae (Rask, 1986). The fish were fed dead blood worms (Chironmidae) twice a week ad libitum. In early July 2017, we randomly distributed larvae of one species among opaque plastic vials (6 × 6 × 10 cm) floating in the tubs. Each tub had one vial per species that held 20 larvae of mixed heritage at the start of the experiment. Larval density within vials corresponded to densities being observed in nature (Banks and Thompson, 1987). We placed a 5 × 5 cm piece of plastic gauze in each vial for larvae to perch on. To ensure vials were floating, they were stabilised by a ring of Styrofoam board. To ensure water exchange between containers and vials, each vial had two circular holes (Ø 6 cm) covered with plastic mesh (mesh width 500 μm). Thus, larvae in the fish treatment could sense the fish by chemical cues but were safe from predation (Ferrari et al., 2010). Containers were stored on campus of Freie Universität Berlin. To prevent complete freezing during winter, we stored containers from December 2017 to March 2018 in a climate room at 5°C. Damselfly larvae were fed ad libitum with Daphnia spec. twice a week, ensuring that vials always held some daphnids as food. Larvae stayed in their respective vials until the start of the behavioural assays (i.e., approx. 9 months). For sample sizes used in this experiment see Supplementary Table 1.
Behavioural assays were carried out during April and May 2018 using only larvae that had reached one of the last three developmental instars before metamorphosis. Larvae of all species were of similar size based on head width (F = 1.833, DF = 3/119, P = 0.145). All behavioural trials were conducted by the same person (MM) in a climate room at a constant temperature of 20°C. For the observations, we used circular plastic bowls (Ø 15 cm). The sides of the bowls were covered with black paper and bottoms of the bowls were roughened with sandpaper to enable larval foraging. Bowls were placed 70 cm underneath a 20 W light bulb and filled to a height of 5 cm with water mixed from the tubs of the corresponding treatment. Before usage in the trials, the water was sieved through a 500 μm Nitex screen to exclude larger items. We used separate bowls and Nitex screens for either water with or without chemical cues from predatory fish. This way, we ensured that water contained or lacked chemical cues by perch. 48 h before each observation, larvae were collected from the vials and held separately in opaque plastic cups (ø 14.5 cm, filled to a height of 5.5 cm) with water mixed from the three containers of the larvae’s respective treatment. We fed the larvae with ten D. pulex and only larvae that had consumed all D. pulex after the 48 h period were used in trials.
All replicates were carried out between 10:00 and 17:00. Ten minutes before behavioural trials, we added 25 D. pulex as prey. After each replicate we exchanged the water and replaced all remaining daphnids with new individuals. Each larva was observed for 10 min. We gently introduced one larva to the bottom of the bowl and recorded behaviour after a brief adaptation time (30 s). The following six variables were recorded during observations (Jiang and Mikolajewski, 2018): (i) orientation (= total number of orientations toward prey without advancing toward the prey); (ii) advance (=total number of directed advances toward prey); (iii) strike (= total number of strikes at prey with the extendable labium); (iv) capture (=total number of successfully caught prey items); (v) walk (=total number of walks defined by movements with contact to the bottom but not in the direction of prey); and (vi) swim (=total number of swims defined by movements with no contact with the bottom but not in the direction of prey). Walk was easily distinguishable from orient and advance because larvae would adapt a lurking position whenever targetting a daphnid as prey. After the trial, each larva was stored individually in 90% alcohol for subsequent measurements (see below).
To assess differences in lamellae size, we carried out morphological measurements of larval caudal lamellae using a stereomicroscope (Olympus Microscope SZX16) connected to a CCD-colour-camera (ColorView II). Damselfly larvae possess three caudal lamellae of which the two lateral lamellae are identical to each other. Therefore, photos of the medial and left lateral lamellae were taken with millimetre paper as a size reference. Subsequently, photos were analyzed using the image processing software “ImageJ” (Abràmoff et al., 2004). We quantified lamellae morphometrics that are likely to be related to lamellae size (McPeek, 1995; Strobbe et al., 2009). In short, for the two lamellae, we recorded the (i) area, (ii) perimeter, (iii) length of major axis, (iv) the largest width perpendicular to the major axis, (v) the distance between the upper end of the lamella base to the lamella tip, and (vi) the distance between the lower end of the lamella base to the lamella tip, respectively (Figure 1). Using the same protocol, we also measured head width of each larva to standardise the response variables for body size in subsequent analyses (see below). All morphological variables were measured in mm or mm2.
Figure 1. Caudal lamella of a Coenagrion larva. Marked are distances measured for each lamella. Distances measured were (i): lamella area, (ii): perimeter of caudal lamella, (iii): length of major axis, (iv): the largest width perpendicular to the major axis, (v): the distance between the upper end of the lamella base and the lamella tip, and (vi): the distance between the lower end of the lamella base and the lamella tip. Measurements were taken using “ImageJ” [NIH; Abràmoff et al. (2004)]. All measurements were made in mm or mm2.
All statistical analyses were performed using R 4.0.1 (R Core Team, 2020). Prior to data analysis, we explored the data set and did not find any unexplainable outliers (Zuur et al., 2010). Because of multicollinearity among our variables, we applied a principal component analysis (PCA) on the correlation matrices for the behavioural and morphological variables, using package “psych” (Revelle, 2017). Extracted principal components (PCs) are orthogonal to each other and therefore uncorrelated (Jolliffe, 2002). PC axes were rotated using varimax method (McPeek, 1990). We extracted the first three PCs (Table 1) as they had eigenvalues >1 (Jolliffe, 2002; Supplementary Figure 1). In all subsequent analysis, we used PC scores from the extracted PCs.
Table 1. The principal component (PC) loadings for the variables describing (A) lamellae morphometrics and (B,C) behavioural variables examined in this study.
To analyse differences between habitats and treatments in behavioural and morphological anti-predator traits, we fitted separate linear mixed-effect models with the three PCs as response variables, using packages “lmer4” (Bates et al., 2015) and “car” (Fox and Weisberg, 2011). PCs were log10(x + 3) transformed to meet normal distribution of residuals errors. As predictor variables, we used species (C. lunulatum, C. mercuriale, C. ornatum, or C. pulchellum) nested within habitat (fish habitat or fishless habitat), treatment (fish or control), and the interaction term. We added log-transformed head width as an additive independent variable to control for body size differences (Mikolajewski et al., 2015a,2016) and used tub number as a random effect to control for differences between larvae from different tubs but of the same treatment.
The extracted PCs captured 86.1% variation of the original data set (Table 1). PC1 (hereafter referred to as “Lamella Size”) explained 61.3% of variation and was highly positively correlated with the variables describing medial and lateral lamellae size metrics (Table 1A). PC2 (hereafter referred to as “Foraging Activity”) explained 17.7% of variation and was highly positively correlated with the variables orient, advance, strike, and capture (Table 1B). PC3 (hereafter referred to as “Movement Activity”) explained 7.1% of variation and was highly positively correlated with the variables walk and swim (Table 1C).
Overall, lamellae of fish habitat species were smaller compared to lamellae of fishless habitat species (Wald-χ2 = 126.789, DF = 1, P < 0.001; Figure 2A). Foraging activity (Wald-χ2 = 5,293, DF = 1, P = 0.021; Figure 2B) but not movement activity (Wald-χ 2 = 2.360, DF = 1, P = 0.124; Figure 2C) was lower in fish habitat species than in fishless habitat species. This resulted in a pattern of trait compensation of foraging activity with lamella size comparing fish- and fishless habitat species. Here, fish habitat species invest in reduced foraging but express smaller lamellae whereas fishless habitat species show lower investment in behaviour by expressing higher foraging activity but also exhibit larger lamellae. Within fishless habitat species, C. pulchellum had larger lamellae than C. lunulatum and within fish habitat species C. mercuriale had larger lamellae than C. ornatum (Wald-χ2 = 6.916, DF = 2, P = 0.031; Figure 2A). No other significant two- or three-way interaction has been detected (see Supplementary Table 2).
Figure 2. Differences in (A) PC1: Lamella Size, (B) PC2: Foraging Activity, and (C) PC3: Movement Activity between larval damselflies of fishless habitat species (Coenagrion lunulatum and Coenagrion pulchellum) and fish habitat species (Coenagrion mercuriale and Coenagrion ornatum) that were either raised with predatory fish (black circles) or in a control treatment (white circles). Error bars correspond to standard errors.
Overall, in the fish treatment individuals expressed smaller lamellae than in the control (Wald-χ2 = 9.289, DF = 1, P = 0.002; Figure 2A) which did not differ between individuals from fishless and fish habitats (Wald-χ2 < 0.001, DF = 1, P = 0.991; Figure 2A). Foraging activity did not differ between treatments (Wald-χ2 = 0.402, DF = 1, P = 0.526; Figure 2B) which was the case for individuals from fishless and fish habitats (Wald-χ2 = 0.219, DF = 1, P = 0.640, Figure 2B). However, individuals reduced movement activity in the fish compared to the control treatment (Wald-χ2 = 6.164, DF = 1, P = 0.013; Figure 2C), but this was only true for individuals stemming from fish habitats (Wald-χ2 = 4.994, DF = 1, P = 0.025; Figure 2C). This resulted in a pattern of trait compensation of movement activity with lamella size within fish habitat species. Here, individuals of fish habitat species in the fish treatment, show higher investment in behaviour by reducing movement, but also express smaller lamellae in the presence of predatory fish. This pattern was not present in fishless habitat species as individuals of these species did not show reduced movement activity. No other significant two- or three-way interaction has been detected (see Supplementary Table 2).
Selection by predators on prey defensive traits plays a key role in promoting the diversity of prey phenotypes [reviewed in Langerhans (2007) and Schluter (2009)]. To avoid and/or repel predators, prey relies on sets of correlated defensive traits (DeWitt and Langerhans, 2003; Pigliucci, 2003; Relyea, 2004b). Here, we show that larvae of damselfly species, occurring in fish habitats, express lower foraging activity and smaller caudal lamellae than species from fishless habitats, resulting in interspecific trait compensation. This pattern of trait correlation was mirrored by intraspecific differences in individuals of fish habitat species, expressing lower movement activity and smaller caudal lamellae in the presence of predatory fish than in the control whereas individuals of fishless habitat species expressed smaller lamellae only. The convergence of developmental and evolutionary trajectories is not surprising given the identical selective environmental conditions, with predation being a major driver of phenotypic appearance.
By investigating trait correlations between closely related species, we were able to study trait correlation on an evolutionary timescale (Mikolajewski et al., 2016; Reilly et al., 2016; Messier et al., 2017; Coley et al., 2018). Our results indicate interspecific trait compensation in morphological and behavioural defences in Coenagrion species. Both trait compensation (Abrahams, 1995; Relyea, 2000; Rundle and Brönmark, 2001; Mikolajewski and Johansson, 2004; Vogelweith et al., 2014) and trait cospecialization (Smith and Van Buskirk, 1995; Relyea, 2001; Mikolajewski and Johansson, 2004; Dayton et al., 2005; Mikolajewski et al., 2010) have frequently been reported on an interspecific level. Cospecialization is expected when strengthening both defences is more effective in reducing mortality caused by predation than strengthening a single defence would be (Garcia and Sih, 2003). Conversely, compensation is expected when enhancing one defensive trait produces a strong defence while trading off costs for other defences (Mikolajewski and Johansson, 2004).
It is unsurprising that in fish habitat species, lamella size is traded-off for foraging activity. Increasing lamella size represents an ineffective defence as larval burst escape swimming is not fast enough to outswim predatory fish (Convey, 1988; Stoks and De Block, 2000; Gyssels and Stoks, 2005; Strobbe et al., 2010). Reducing foraging activity on the other hand is an effective defence as it reduces the encounter rate with predatory fish and predation by fish therefore imposes strong selection on foraging activity (Werner and Anholt, 1993; Stoks and McPeek, 2006; Strobbe et al., 2011; Jiang and Mikolajewski, 2018). Conversely, fishless habitat species trade-off foraging activity for lamella size. In fishless habitats, predatory invertebrates such as large dragonfly larvae are the main predators of damselfly larvae (Wellborn et al., 1996; Stoks and McPeek, 2006). Most invertebrate predators can be evaded by larval burst escape swimming (Stoks, 1998; Bose and Robinson, 2013). Therefore, the underlying morphological trait of burst escape swimming, lamella size, represents an effective defence in this habitat type and selection by invertebrate predators acts strongly on lamella size in fishless habitat species [e.g., McPeek (1995, 1997) and Strobbe et al. (2009)]. Investment into behavioural defences (i.e., reducing foraging activity), however, is lower in fishless habitat species. Reducing activity decreases encounter rates with predators (Werner and Anholt, 1993; Stoks and McPeek, 2006; Strobbe et al., 2011; Jiang and Mikolajewski, 2018), but carries the cost of reduced encounter rates with food and reduced growth rate (Stoks et al., 2005a,b; Biro et al., 2006). Increased activity is common in freshwater prey facing invertebrate predators compared to prey facing predatory fish [e.g., Richardson (2001), Stoks and McPeek (2006), and Jiang and Mikolajewski (2018)]. This prey usually relies on other defensive traits, such as larger appendages like fins or lamellae to accelerate burst escape swimming (Wellborn et al., 1996). While these morphological defences carry costs as well [e.g., Tollrian (1995), Van Buskirk and Schmidt (2000), Benard and Fordyce (2003), Flenner et al. (2009), Van Donk et al. (2011), and Vinterstare et al. (2019)], reducing foraging activity is likely costlier [e.g., Steiner and Pfeiffer (2009)] and fishless habitat species instead rely on lamella size, underlying burst escape swimming, instead of reducing foraging. Thus, differences in predator-driven selection and costs associated with defensive traits led to this pattern of interspecific trait compensation in Coenagrion species with fishless habitat species trading off behavioural avoidance for larger lamellae and vice versa in fish habitat species.
By rearing larvae of all four species either with or without a predatory fish, we were able to study intraspecific trait correlation mediated by developmental plasticity [e.g., Relyea (2001); Hoverman and Relyea (2009), and Herzog et al. (2016)]. Interestingly, only individuals of fish habitat species showed plastic reduction in movement activity whereas all species plastically expressed smaller caudal lamellae in the presence of predatory fish. This resulted in a pattern of intraspecific trait compensation for fish habitat species which mirrored the correlational pattern we found comparing species from different habitat types. Conversely, no pattern of intraspecific correlation was observed in fishless habitat species (see below).
Our results correspond to previous work reporting that prey species respond behaviourally to co-existing predator species while being oblivious of allopatric predator species [e.g., Stoks et al. (2003), Åbjörnsson et al. (2004), Jiang and Mikolajewski (2018), and Kadye et al. (2020)], supporting the prey naïvety-hypothesis. However, all Coenagrion species regardless of habitat plastically reduced lamellae size in the fish treatment, resulting in intraspecific trait compensation in fish habitat species and no observable trait correlation in fishless habitat species. This hints at functional and mechanistic differences in morphological and behavioural responses to predators. For example, Dijk et al. (2016) found that tadpoles with reduced morphological defences (i.e., smaller tail fins) but with no differences in behaviour were less likely to be attacked by a predator, possibly due to the “lure effect” [see also Crowder and Cooper (1982), Morin (1984a,b), and Robinson et al. (1991)]. Offering an alternative explanation, Relyea et al. (2021) showed that plasticity might evolve more quickly in morphological defences than in behavioural defences. Likewise, Hossie et al. (2017) found that the strength of morphological and/or behavioural anti-predator responses in tadpoles strongly differed with exposure to different predators and whether predator and prey shared a co-evolutionary history or not. In the case of Coenagrion damselfly larvae, fish habitat species increase behavioural avoidance while saving on a morphological defence. Conversely, fishless habitat species only save on a presumably maladaptive morphological defence in response to a predator that is only rarely encountered in the specific natural habitats (Kuhn and Burbach, 1998; Dijkstra and Lewington, 2006). Thus, we show that intraspecific correlations between anti-predator traits may vary among species, again driven by the predator gradient (Wellborn et al., 1996).
Our results indicate intraspecific trait compensation in fish habitat species, contrasting previous results of a meta-analysis by Hossie et al. (2017) on intraspecific anti-predator trait correlation in tadpoles. Hossie et al. (2017) showed that tadpoles most frequently cospecialise behavioural (i.e., activity reduction) with morphological (i.e., inducing deeper or larger tail fins) defences as a response to perceived predation risk by predatory fish. Both prey types show similar responses on the behavioural axis by reducing activity, most likely because visually hunting predators such as fish are attracted by movements (McPeek, 1990; Skelly, 1994; Wellborn et al., 1996; Stoks and De Block, 2000; Strobbe et al., 2011). However, they show diverging responses on the morphological axis. Tadpoles can escape predatory fish by swimming and thus, the underlying morphological trait: tail size, operates against predatory fish (Teplitsky et al., 2005). Damselfly larvae, however, cannot reach swimming speeds high enough to escape predatory fish by relying on burst escape swimming, and therefore, caudal lamellae size does not operate against predatory fish in damselfly larvae (Convey, 1988; Stoks and De Block, 2000; Gyssels and Stoks, 2005). Thus, functional differences in morphological defences might have resulted in diverging correlational patterns in both prey taxa with tadpoles showing trait cospecialization and damselfly larvae showing trait compensation.
Intriguingly, McPeek (1997) and Stoks et al. (1999) [see also Strobbe et al. (2009) and Strobbe et al. (2010)] reported the lack of plasticity in lamellae size in damselfly larvae of the genera Enallagma and Lestes, contrasting to our results. However, Black et al. (2019) reported functional plasticity in breaking joint sizes in two Enallagma-species, by investigating intraspecific differences driven by predator cues. Strikingly, the studies of McPeek (1997), Stoks et al. (1999), and this study is the experimental duration [1 week, 1 month, 9 months, for McPeek (1997), Stoks et al. (1999), and this study, respectively; the experimental duration in Black et al. (2019) was roughly 2 months]. Thus, experimental duration in the studies by McPeek (1997) and Stoks et al. (1999) might have been too short, to induce an effect of predator presence on lamellae size in damselfly larvae [see also DeWitt et al. (1998) and Diggle (2002)].
Phenotypic changes are frequently studied either on evolutionary timescales (i.e., comparing populations or species) or on developmental timescales (i.e., comparing individuals raised under different conditions). Thereby, research might ignore the interactive effect of natural selection and phenotypic plasticity on shaping phenotypic diversity [e.g., Schlichting (2021)]. Moreover, research on phenotypic changes is often limited only to single traits while most traits with a similar function likely change in concert and a single-trait focus could limit our understanding of phenotypic change [e.g., DeWitt and Langerhans (2003)]. Our research is important in linking different timescales with trait correlations in a freshwater predator gradient. Although our sample size was rather limited, our findings indicate that when studying the evolution of traits, it is important to consider a multiple timescale and trait approach to better understand how selection drives phenotypic divergence.
The datasets presented in this study can be found in online repositories. The names of the repository/repositories and accession number(s) can be found below: Open Science Framework (OSF): https://osf.io/qxzuk/.
BJ, DM, and MM conceived and designed the study with assistance from OB. BJ and DM collected the egg clutches for this study with assistance from OB. MM and BJ performed the study and collected the data. MM, BJ, and DM analyzed the data and MM and DM wrote the draft of the manuscript with assistance from BJ. All authors contributed to the article and approved the submitted version.
The China Scholarship Council (CSC) provided a Ph.D.-stipend for BJ.
The authors declare that the research was conducted in the absence of any commercial or financial relationships that could be construed as a potential conflict of interest.
All claims expressed in this article are solely those of the authors and do not necessarily represent those of their affiliated organizations, or those of the publisher, the editors and the reviewers. Any product that may be evaluated in this article, or claim that may be made by its manufacturer, is not guaranteed or endorsed by the publisher.
We would like to thank M. Friedrichs for collecting the perch. We acknowledge support by the OpenAccess Publication Fund of Freie Universität Berlin for paying the APC. Two reviewers helped improve the quality of this manuscript.
The Supplementary Material for this article can be found online at: https://www.frontiersin.org/articles/10.3389/fevo.2022.874276/full#supplementary-material
Åbjörnsson, K., Hansson, L. A., and Brönmark, C. (2004). Responses of prey from habitats with different predator regimes: local adaptation and heritability. Ecology 85, 1859–1866. doi: 10.1890/03-0074
Abrahams, M. V. (1995). The interaction between antipredator behaviour and antipredator morphology: experiments with fathead minnows and brook sticklebacks. Can. J. Zool. 73, 2209–2215. doi: 10.1139/z95-261
Abràmoff, M. D., Magalhães, P. J., and Ram, S. J. (2004). Image processing with ImageJ. J. Biophoton. 11, 36–42.
Agnani, P., Thomson, J., Schradin, T., and Careau, V. (2020). The fast and the curious II: performance, personality, and metabolism in Karoo bush rats. Behav. Ecol. Sociobiol. 74:123. doi: 10.1007/s00265-020-02908-y
Ahlgren, J., Chapman, B. B., Nilsson, P. A., and Brönmark, C. (2015). Individual boldness is linked to protective shell shape in aquatic snails. Biol. Lett. 11:20150029. doi: 10.1098/rsbl.2015.0029
Banks, M. J., and Thompson, D. J. (1987). Regulation of damselfly populations: the effects of larval density on larval survival, development rate and size in the field. Freshw. Biol. 17, 357–365. doi: 10.1111/J.1365-2427.1987.TB01055.X
Bates, D., Mächler, M., Bolker, B., and Walker, S. (2015). Fitting linear mixed-effects models using lme4. J. Stat. Softw. 67, 1–48. doi: 10.18637/jss.v067.i01
Benard, M. F., and Fordyce, J. A. (2003). Are induced defenses costly? Consequences of predator-induced defenses in western toads, Bufo boreas. Ecology 84, 68–78. doi: 10.2307/3107998
Biro, P. A., Abrahams, M. V., Post, J. R., and Parkinson, E. A. (2006). Behavioural trade-offs between growth and mortality explain evolution of submaximal growth rates. J. Anim. Ecol. 75, 1165–1171.
Black, K. L., Fudge, D., Jarvis, W. M., and Robinson, B. W. (2019). Functional plasticity in lamellar autotomy by larval damselflies in response to predatory larval dragonfly cues. Evol. Ecol. 33, 257–272. doi: 10.1007/s10682-019-09979-y
Bose, A. P. H., and Robinson, B. W. (2013). Invertebrate predation predicts variation in an autotomy-related trait in larval damselfly. Evol. Ecol. 27, 27–38. doi: 10.1007/s10682-012-9581-3
Bourdeau, P. E. (2009). Prioritized phenotypic responses to combined predators in a marine snail. Ecology 90, 1659–1669. doi: 10.1890/08-1653.1
Bourdeau, P. E., and Johansson, F. (2012). Predator-induced morphological defences as by-products of prey behaviour: a review and prospectus. Oikos 121, 1175–1190. doi: 10.2307/23261003
Brönmark, C., Lakowitz, T., Nilsson, P. A., Ahlgren, J., Lennartsdotter, C., and Hollander, J. (2012). Costs of inducible defence along a resource gradient. PLoS One 7:e30467. doi: 10.1371/journal.pone.0030467
Chivers, D. P., Wisenden, B. D., and Smith, R. J. F. (1996). Damselfly larvae learn to recognize predators from chemical cues in the predator’s diet. Anim. Behav. 52, 315–320. doi: 10.1006/anbe.1996.0177
Coley, P. D., Endara, M. J., and Kursar, T. A. (2018). Consequences of interspecific variation in defenses and herbivore host choice for the ecology and evolution of Inga, a speciose rainforest tree. Oecologia 187, 361–337. doi: 10.1007/s00442-018-4080-z
Convey, P. (1988). Competition for perches between larval damselflies: the influence of perch use on feeding efficiency, growth rate and predator avoidance. Freshw. Biol. 19, 15–28. doi: 10.1111/j.1365-2427.1988.tb00323.x
Cotton, P. A., Rundle, S. D., and Smyth, K. E. (2004). Trait compensation in marine gastropods: shell shape, avoidance behavior, and susceptibility to predation. Ecology 85, 1581–1584. doi: 10.1890/03-3104
Crowder, L. B., and Cooper, W. E. (1982). Habitat structural complexity and the interaction between bluegills and their prey. Ecology 63, 1802–1813. doi: 10.1007/s00442-004-1644-x
Dayton, G. H., Saenz, D., Baum, K. A., Langerhans, R. B., and DeWitt, T. J. (2005). Body shape, burst speed and escape behavior of larval anurans. Oikos 111, 582–591. doi: 10.1111/j.1600-0706.2005.14340.x
DeWitt, T. J., and Langerhans, R. B. (2003). Multiple prey traits, multiple predators: keys to understanding complex community dynamics. J. Sea Res. 49, 143–155. doi: 10.1016/S1385-1101(02)00220-4
DeWitt, T. J., Sih, A., and Hucko, J. A. (1999). Trait compensation and cospecialization in a freshwater snail: size, shape and antipredator behaviour. Anim. Behav. 58, 397–407. doi: 10.1006/anbe.1999.1158
DeWitt, T. J., Sih, A., and Wilson, D. S. (1998). Costs and limits of phenotypic plasticity. Trends Ecol. Evol. 13, 77–81. doi: 10.1016/S0169-5347(97)01274-3
Diggle, P. K. (2002). A developmental morphologist’s perspective on plasticity. Evol. Ecol. 16, 267–283. doi: 10.1023/A:1019680527788
Dijk, B., Laurila, A., Orizaola, G., and Johansson, F. (2016). Is one defence enough? Disentangling the relative importance of morphological and behavioural predator-induced defences. Behav. Ecol. Sociobiol. 70, 237–246. doi: 10.1007/s00265-015-2040-8
Dijkstra, K., and Lewington, R. (2006). Field Guide to the Dragonflies of Britain and Europe. London: British Wildlife Publishing.
Edmunds, M. (1974). Defence in Animals: A Survey of Anti-Predator Defences. Harlow: Longman Publishing Group.
Ferrari, M. C., Wisenden, B. D., and Chivers, D. P. (2010). Chemical ecology of predator–prey interactions in aquatic ecosystems: a review and prospectus. Can. J. Zool. 88, 698–724. doi: 10.1139/Z10-029
Flenner, I., Olne, K., Suhling, F., and Sahlén, G. (2009). Predator-induced spine length and exocuticle thickness in Leucorrhinia dubia (Insecta: Odonata): a simple physiological trade-off? Ecol. Entomol. 34, 735–740. doi: 10.1111/j.1365-2311.2009.01129.x
Garcia, T. S., and Sih, A. (2003). Color change and color-dependent behavior in response to predation risk in the salamander sister species Ambystoma barbouri and Ambystoma texanum. Oecologia 137, 131–139. doi: 10.1007/s00442-003-1314-4
Gunzburger, M. S., and Travis, J. (2004). Evaluating predation pressure on green treefrog larvae across a habitat gradient. Oecologia 140, 422–429. doi: 10.1007/s00442-004-1610-7
Gyssels, F. G. M., and Stoks, R. (2005). Threat-sensitive responses to predator attacks in a damselfly. Ethology 111, 411–423. doi: 10.1111/j.1439-0310.2005.01076.x
Herzog, Q., Rabus, M., Ribeiro, B. W., and Laforsch, C. (2016). Inducible Defenses with a” Twist”: Daphnia barbata abandons bilateral symmetry in response to an ancient predator. PLoS One 11:e0148556. doi: 10.1371/journal.pone.0148556
Hossie, T., Landolt, K., and Murray, D. L. (2017). Determinants and co-expression of anti-predator responses in amphibian tadpoles: a meta-analysis. Oikos 126, 173–184. doi: 10.1111/oik.03305
Hoverman, J. T., and Relyea, R. A. (2009). Survival trade-offs associated with inducible defences in snails: the roles of multiple predators and developmental plasticity. Funct. Ecol. 23, 1179–1188. doi: 10.1111/j.1365-2435.2009.01586.x
Hulthén, K., Chapman, B. B., Nilsson, P. A., Hollander, J., and Brönmark, C. (2014). Express yourself: bold individuals induce enhanced morphological defences. Proc. R. Soc. B Biol. Sci. 281:20132703. doi: 10.1098/rspb.2013.2703
Jiang, B., and Mikolajewski, D. J. (2018). Shift in predation regime mediates diversification of foraging behaviour in a dragonfly genus. Ecol. Entomol. 43, 525–533. doi: 10.1111/een.12530
Kadye, W. T., Leigh, S., and Booth, A. J. (2020). Predator naïve minnows respond to their conspecific alarm substance but not the odour from a non-native predator. Afr. J. Ecol. 58, 757–765. doi: 10.1111/aje.12768
Kern, E. M. A., Robinson, D., Gass, E., Godwin, J., and Langerhans, R. B. (2016). Correlated evolution of personality, morphology and performance. Anim. Behav. 117, 79–86. doi: 10.1016/j.anbehav.2016.04.007
Kuhn, K., and Burbach, K. (1998). Libellen in Bayern. Stuttgart: Bayerisches Landesamt für Umweltschutz & Bund Naturschutz in Bayern.
Langerhans, R. B. (2007). Evolutionary Consequences of Predation: Avoidance, Escape, Reproduction, and Diversification. Predation in Organisms. Berlin: Springer.
Langerhans, R. B. (2009). Trade-off between steady and unsteady swimming underlies predator-driven divergence in Gambusia affinis. J. Evol. Biol. 22, 1057–1075. doi: 10.1111/j.1420-9101.2009.01716.x
Laurila, A., Järvi-Laturi, M., Pakkasmaa, S., and Merilä, J. (2004). Temporal variation in predation risk: stage-dependency, graded responses and fitness costs in tadpole antipredator defences. Oikos 107, 90–99. doi: 10.1111/j.0030-1299.2004.13126.x
Marchinko, K. B. (2009). Predation’s role in repeated phenotypic and genetic divergence of armor in threespine stickleback. Evolution 63, 127–138. doi: 10.1111/j.1558-5646.2008.00529.x
Marshall, C. A., and Wund, M. A. (2017). The evolution of correlations between behavioural and morphological defence in Alaskan threespine stickleback fish (Gasterosteus aculeatus): evidence for trait compensation and co-specialization. Evol. Ecol. Res. 18, 305–322.
Mayer, M., Shine, R., and Brown, G. P. (2016). Bigger babies are bolder: effects of body size on personality of hatchling snakes. Behaviour 153, 313–323. doi: 10.1163/1568539x-00003343
McPeek, M. A. (1990). Behavioral differences between Enallagma Species (Odonata) Influencing differential vulnerability to predators. Ecology 71, 1714–1726. doi: 10.2307/1937580
McPeek, M. A. (1995). Morphological evolution mediated by behavior in the damselflies of two communities. Evolution 49, 749–769. doi: 10.1111/j.1558-5646.1995.tb02311.x
McPeek, M. A. (1997). Measuring phenotypic selection on an adaptation: lamallae of damselflies experiencing dragonfly predation. Evolution 51, 459–466. doi: 10.1111/j.1558-5646.1997.tb02433.x
McPeek, M. A., Schrot, A. K., and Brown, J. M. (1996). Adaptation to predators in a new community: swimming performance and predator avoidance in damselflies. Ecology 77, 617–629. doi: 10.2307/2265635
Messier, J., Lechowicz, M. J., McGill, B. J., Violle, C., and Enquist, B. J. (2017). Interspecific integration of trait dimensions at local scales: the plant phenotype as an integrated network. J. Ecol. 105, 1775–1790. doi: 10.1111/1365-2745.12755
Mikolajewski, D. J., Block, M. D., Rolff, J., Johansson, F., Beckerman, A. P., and Stoks, R. (2010). Predator-driven trait diversification in a dragonfly genus: covariaton in behavioral and morphological antipredator defense. Evolution 64, 3327–3335. doi: 10.1111/j.1558-5646.2010.01078.x
Mikolajewski, D. J., and Johansson, F. (2004). Morphological and behavioral defenses in dragonfly larvae: trait compensation and cospecialization. Behav. Ecol. 15, 614–620. doi: 10.1093/beheco/arh061
Mikolajewski, D. J., Johansson, F., Wohlfahrt, B., and Stoks, R. (2006). Invertebrate predation selects for the loss of a morphological antipredator trait. Evolution 60, 1306–1310. doi: 10.1111/j.0014-3820.2006.tb01208.x
Mikolajewski, D. J., Rüsen, L., Mauersberger, R., Johansson, F., and Rolff, J. (2015a). Relaxed predation results in reduced phenotypic integration in a suite of dragonflies. J. Evol. Biol. 28, 1354–1363. doi: 10.1111/jeb.12658
Mikolajewski, D. J., De Block, M., and Stoks, R. (2015b). The interplay of adult and larval time constraints shapes species differences in larval life history. Ecology 96, 1128–1138. doi: 10.1890/14-0262.1
Mikolajewski, D. J., Scharnweber, K., Jiang, B., Leicht, S., Mauersberger, R., and Johansson, F. (2016). Changing the habitat: the evolution of intercorrelated traits to escape from predators. J. Evol. Biol. 29, 1394–1405. doi: 10.1111/jeb.12879
Mikolajewski, D. J., Stoks, R., Rolff, J., and Joop, G. (2008). Predators and cannibals modulate sex-specific plasticity in life-history and immune traits. Funct. Ecol. 22, 114–120. doi: 10.1111/j.1365-2435.2007.01357.x
Mori, T., and Saitoh, T. (2014). Flood disturbance and predator–prey effects on regional gradients in species diversity. Ecology 95, 132–141. doi: 10.1890/13-0914.1
Morin, P. J. (1984a). The impact of fish exclusion on the abundance and species composition of larval odonates: results of short-term experiments in a North Carolina farm pond. Ecology 65, 53–60. doi: 10.2307/1939457
Morin, P. J. (1984b). Odonate guild composition: experiments with colonization history and fish predation. Ecology 65, 1866–1873. doi: 10.2307/1937784
Pacheco, E. O., Almeida-Gomes, M., Santana, D. J., and Guariento, R. D. (2019). Space use and phenotypic plasticity in tadpoles under predation risk. Hydrobiology 837, 77–86. doi: 10.1007/s10750-019-3962-3
Pigliucci, M. (2003). Phenotypic integration: studying the ecology and evolution of complex phenotypes. Ecol. Lett. 6, 265–272. doi: 10.1046/j.1461-0248.2003.00428.x
Piovia-Scott, J., Yang, L. H., and Wright, A. N. (2017). Temporal variation in trophic cascades. Annu. Rev. Ecol. Eol. S. 48, 281–300. doi: 10.1146/annurev-ecolsys-121415-032246
R Core Team (2020). R: A Language and Environment for Statistical Computing. Vienna: R Foundation for Statistical Computing.
Rask, M. (1986). The diet and diel feeding activity of perch, Perca fluviatilis L., in a small lake in southern Finland. Ann. Zool. Fenn. 23, 49–56.
Reilly, S. M., Montuelle, S. J., Schmidt, A., Krause, C., Naylor, E., and Essner, R. L. Jr. (2016). Functional evolution of jumping in frogs: interspecific differences in take-off and landing. J. Morph. 277, 379–393. doi: 10.1002/jmor.20504
Relyea, R. A. (2000). Trait-mediated indirect effects in larval anurans: reversing competition with the threat of predation. Ecology 81, 2278–2289. doi: 10.2307/177114
Relyea, R. A. (2001). Morphological and behavioral plasticity of larval anurans in response to different predators. Ecology 82, 523–540. doi: 10.1890/0012-9658(2001)082[0523:mabpol]2.0.co;2
Relyea, R. A. (2004a). Fine-tuned phenotypes: tadpole plasticity under 16 combinations of predators and competitors. Ecology 85, 172–179. doi: 10.1890/03-0169
Relyea, R. A. (2004b). “Integrating phenotypic plasticity when death is on the line,” in Phenotypic Integration: Studying the Ecology and Evolution of Complex Phenotypes, eds M. Pigliucci and K. A. Preston (Oxford: Oxford University Press).
Relyea, R. A., Stephens, P. R., and Hammond, J. I. (2021). Phylogenetic patterns of trait and trait plasticity evolution: insights from tadpoles. Evolution 75, 2568–2588. doi: 10.1111/evo.14338
Renner, M., Arimitsu, M. L., and Piatt, J. F. (2012). Structure of marine predator and prey communities along environmental gradients in a glaciated fjord. Can. J. Fish. Aquat. Sci. 69, 2029–2045. doi: 10.1139/f2012-117
Revelle, W. R. (2017). psych: Procedures for Personality and Psychological Research. R package version 2.2.3. Available online at: https://cran.r-project.org/web/packages/psych/index.html (accessed November 5, 2018).
Richardson, J. M. L. (2001). A comparative study of activity levels in larval anurans and response to the presence of different predators. Behav. Ecol. 12, 51–58. doi: 10.1093/oxfordjournals.beheco.a000378
Robinson, J. V., Shaffer, L. R., Hagemier, D. D., and Smatresk, N. J. (1991). The ecological role of caudal lamellae loss in the larval damselfly, Ischnura posita (Hagen)(Odonata: Zygoptera). Oecologia 87, 1–7. doi: 10.1007/BF00323773
Rundle, S. D., and Brönmark, C. (2001). Inter- and intraspecific trait compensation of defence mechanisms in freshwater snails. Proc. Biol. Sci. 268, 1463–1468. doi: 10.1098/rspb.2001.1682
Schlichting, C. D. (2021). Plasticity and Evolutionary Theory: Where We Are and Where We Should Be Going. Phenotypic Plasticity & Evolution. Boca Raton, FL: CRC Press.
Schluter, D. (2009). Evidence for ecological speciation and its alternative. Science 323, 737–741. doi: 10.1126/science.1160006
Skelly, D. K. (1994). Activity level and the susceptibility of anuran larvae to predation. Anim. Behav. 47, 465–468. doi: 10.1006/anbe.1994.1063
Smith, D. C., and Van Buskirk, J. (1995). Phenotypic design, plasticity, and ecological performance in two tadpole species. Am. Nat. 145, 211–233. doi: 10.1086/285737
Sorace, A., and Gustin, M. (2009). Distribution of generalist and specialist predators along urban gradients. Landsc. Urban. Plan. 90, 111–118. doi: 10.1016/j.landurbplan.2008.10.019
Steiner, U. K., and Pfeiffer, T. (2009). Optimizing time and resource allocation trade-offs for investment into morphological and behavioral defense. Am. Nat. 169, 118–129. doi: 10.1086/509939
Stoks, R. (1998). Effect of lamellae autotomy on survival and foraging success of the damselfly Lestes sponsa (Odonata: Lestidae). Oecologia 117, 443–448. doi: 10.1007/s004420050679
Stoks, R., and De Block, M. (2000). The influence of predator species and prey age on the immediate survival value of antipredator behaviours in a damselfly. Arch. Hydrobiol. 147, 417–430. doi: 10.1127/ARCHIV-HYDROBIOL/147/2000/417
Stoks, R., De Block, M., and McPeek, M. A. (2005a). Alternative growth and energy storage responses to mortality threats in damselflies. Ecol. Lett. 8, 1307–1316. doi: 10.1111/j.1461-0248.2005.00840.x
Stoks, R., De Block, M., Van De Meutter, F., and Johansson, F. (2005b). Predation cost of rapid growth: behavioural coupling and physiological decoupling. J. Anim. Ecol. 74, 708–715. doi: 10.1111/j.1365-2656.2005.00969.x
Stoks, R., De Block, M., Van Gossum, H., and De Bruyn, L. (1999). Phenotypic shifts caused by predation: selection or life-history shifts? Evol. Ecol. 13, 115–129. doi: 10.1023/a:1006656616539
Stoks, R., and Johansson, F. (2000). Trading off mortality risk against foraging effort in damselflies that differ in life cycle length. Oikos 91, 559–567. doi: 10.1034/j.1600-0706.2000.910318.x
Stoks, R., and McPeek, M. A. (2006). A tale of two diversifications: reciprocal habitat shifts to fill ecological space along the pond permanence gradient. Am. Nat. 168, S50–S72. doi: 10.1086/509045
Stoks, R., McPeek, M. A., and Mitchell, J. L. (2003). Evolution of prey behavior in response to changes in predation regime: damselflies in fish and dragonfly lakes. Evolution 57, 574–585. doi: 10.1554/0014-3820(2003)057[0574:EOPBIR]2.0.CO;2
Strobbe, F., McPeek, M., De Block, M., De Meester, L., and Stoks, R. (2009). Survival selection on escape performance and its underlying phenotypic traits: a case of many-to-one mapping. J. Evol. Biol. 22, 1172–1182. doi: 10.1111/j.1420-9101.2009.01733.x
Strobbe, F., McPeek, M. A., De Block, M., and Stoks, R. (2010). Survival selection imposed by predation on a physiological trait underlying escape speed. Funct. Ecol. 24, 1306–1312. doi: 10.1111/j.1365-2435.2010.01752.x
Strobbe, F., McPeek, M. A., De Block, M., and Stoks, R. (2011). Fish predation selects for reduced foraging activity. Behav. Ecol. Sociobiol. 65, 241–247. doi: 10.1007/s00265-010-1032-y
Teplitsky, C., Plenet, S., Léna, J.-P., Mermet, N., Malet, E., and Joly, P. (2005). Escape behavior and ultimate causes of specific induced defences in an anuran tadpole. J. Evol. Biol. 18, 180–190. doi: 10.1111/j.1420-9101.2004.00790.x
Tollrian, R. (1995). Predator-induced morphological defenses: costs, life history shifts, and maternal effects in Daphnia pulex. Ecology 76, 1691–1705. doi: 10.2307/1940703
Tollrian, R., and Harvell, C. D. (1999). The Ecology and Evolution of Inducible Defenses. Princeton: Princeton University Press.
Vamosi, S. M. (2005). On the role of enemies in divergence and diversification of prey: a review and synthesis. Can. J. Zool. 83, 894–910. doi: 10.1139/z05-063
Van Buskirk, J., and Schmidt, B. R. (2000). Predator-induced phenotypic plasticity in larval newts: trade-offs, selection, and variation in nature. Ecology 81, 3009–3028. doi: 10.2307/177397
Van Donk, E., Ianora, A., and Vos, M. (2011). Induced defences in marine and freshwater phytoplankton: a review. Hydrobiologia 668, 3–19. doi: 10.1007/s10750-010-0395-4
Vinterstare, J., Hegemann, A., Nilsson, P. A., Hulthén, K., and Brönmark, C. (2019). Defence versus defence: are crucian carp trading off immune function against predator-induced morphology? J. Anim. Ecol. 88, 1510–1521.
Vogelweith, F., Thiéry, D., Moret, Y., Colin, E., Motreuil, S., and Moreau, J. (2014). Defense strategies used by two sympatric vineyard moth pests. J. Insect. Physiol. 64, 54–61. doi: 10.1016/j.jinsphys.2014.03.009
Wellborn, G. A., Skelly, D. K., and Werner, E. E. (1996). Mechanisms creating community structure across a freshwater habitat gradient. Annu. Rev. Ecol. Syst. 27, 337–363. doi: 10.1146/annurev.ecolsys.27.1.337
Werner, E. E., and Anholt, B. R. (1993). Ecological consequences of the trade-off between growth and mortality rates mediated by foraging activity. Am. Nat. 142, 242–272. doi: 10.1086/285537
Werner, E. E., and McPeek, M. A. (1994). Direct and indirect effects of predators on two anuran species along an environmental gradient. Ecology 75, 1368–1382. doi: 10.2307/1937461
Keywords: Coenagrion, defensive traits, phenotypic plasticity, predation, trait correlations
Citation: Mühlenhaupt M, Jiang B, Brauner O and Mikolajewski DJ (2022) Inter- and Intraspecific Trait Compensation of Behavioural and Morphological Defences in a Damselfly Genus. Front. Ecol. Evol. 10:874276. doi: 10.3389/fevo.2022.874276
Received: 11 February 2022; Accepted: 04 April 2022;
Published: 26 April 2022.
Edited by:
Mauricio J. Carter, Universidad Andres Bello, ChileReviewed by:
Andreas Walzer, University of Natural Resources and Life Sciences Vienna, AustriaCopyright © 2022 Mühlenhaupt, Jiang, Brauner and Mikolajewski. This is an open-access article distributed under the terms of the Creative Commons Attribution License (CC BY). The use, distribution or reproduction in other forums is permitted, provided the original author(s) and the copyright owner(s) are credited and that the original publication in this journal is cited, in accordance with accepted academic practice. No use, distribution or reproduction is permitted which does not comply with these terms.
*Correspondence: Max Mühlenhaupt, bWF4Lm11ZWhsZW5oYXVwdEB1bmktYmllbGVmZWxkLmRl
Disclaimer: All claims expressed in this article are solely those of the authors and do not necessarily represent those of their affiliated organizations, or those of the publisher, the editors and the reviewers. Any product that may be evaluated in this article or claim that may be made by its manufacturer is not guaranteed or endorsed by the publisher.
Research integrity at Frontiers
Learn more about the work of our research integrity team to safeguard the quality of each article we publish.