- 1Department of Ecology, Brandenburg University of Technology Cottbus–Senftenberg, Cottbus, Germany
- 2Institute of Animal Ecology, Justus Liebig University Giessen, Giessen, Germany
- 3Department of Landscape Ecology, Institute for Natural Resource Conservation, Kiel University, Kiel, Germany
Nitrogen fertilization of permanent grasslands affects soil fauna communities by modifying their taxonomic composition, population dynamics and feeding activity. However, it is not well understood if the edaphic fauna adapts to these external inputs so that the immediate response to fertilizer application depends on the long-term nutrient management strategy. We performed a field experiment in permanent grasslands under agricultural management in three regions across Germany. We used experimental fertilization with an organic plant-sourced fertilizer along a long-term nutrient management gradient to study the immediate and long-term effects of fertilization and their interdependence on the taxonomic composition and feeding activity of the soil mesofauna (Nematoda, Oribatida, and Collembola). Sampling season, soil properties, vegetation structure, and geographic location were considered as additional predictor variables to reflect heterogeneity in environmental conditions. The taxonomic composition, richness and total abundance of soil mesofauna communities were significantly affected by long-term nutrient management, but not by experimental fertilization. However, N pulses rapidly (within days) reduced the feeding activity estimated with bait-lamina strips independent of long-term nutrient management strategies. Experimental addition of organic plant-sourced fertilizer may have led to a rapid build-up of microbial biomass, providing alternative food sources for the soil mesofauna and causing a shift away from the bait-lamina substrate. Our study indicates that community changes associated with the long-term nutrient management regime in permanent grasslands do not alter the strong functional response of the soil mesofauna to N pulses. There is an urgent need to develop nutrient management strategies for permanent grasslands that take into account both the conservation of the edaphic faunal community and changes of ecosystem functions caused by rapid responses of the soil mesofauna to fertilizer inputs.
Introduction
Permanent agricultural grasslands provide important habitats to many plant and animal species (Veen et al., 2009). Thus, they are a key conservation measure at the national and EU scale (Hristov et al., 2020). Yet, EU regulation allows for a broad range of nutrient management intensities, including the quantity of fertilizer and livestock densities. Decades of research have shown that the structure and richness of soil invertebrate communities can be altered by long-term land use (Birkhofer et al., 2008) and short-term anthropogenic stressors (Zaitsev et al., 2006), with effects being modulated by factors such as landscape characteristics (Zaitsev et al., 2013). It is thus very likely that grassland management severely affects soil biodiversity (e.g., Brussaard, 1997; Bardgett and Van Der Putten, 2014). However, a comprehensive understanding of the functional consequences associated with these changes is still lacking (Eisenhauer et al., 2017; Delgado-Baquerizo et al., 2020).
The edaphic mesofauna is unable to overcome the physical barriers of the soil, as soil animals such as Collembola or Acari cannot actively construct their own passages and pore spaces in soil (Potapov et al., 2022). Most functional effects of this group are thus related to its feeding activity, with changes in these activities being primarily caused by changes in both available food sources and community composition (Wolters and Joergensen, 1991). Fertilizer application has previously been shown to reduce the soil fauna feeding activity in tropical oil palm plantations (for chemical fertilizers: Tao et al., 2016) or to increase feeding activity in annual crop fields (for organic fertilizers: Pfotzer and Schüler, 1997). Understanding the effect of fertilizer application on the feeding activity of belowground communities is essential for evaluating the functional consequences of management strategies. The long-term application of synthetic fertilizers may lead to soil acidification and affect soil fauna negatively (Birkhofer et al., 2008). Alternatively, organic fertilizers, either animal (e.g., manure or slurry) or plant-sourced (e.g., green manure or biofertilizers) materials, may promote soil fauna abundances and activity due to provision of dead organic matter as food resource for detritivores or due to enhanced microbial growth and provision of food resources to microbivorous and fungivorous soil fauna (Birkhofer et al., 2012). In the study presented here, we focused on whether the immediate trophic response of soil communities of permanent agricultural grasslands to fertilizer application occurs independently of taxonomic composition. Alternatively, it could be modulated by changes in taxonomic composition associated with long-term nutrient management strategies. In contrast to forests and annual crop fields (Geissen and Brümmer, 1999; Birkhofer et al., 2008; Ding et al., 2019; Shaw et al., 2019), effects of long-term fertilization (e.g., fertilizer application, livestock density) on soil mesofauna communities of permanent grasslands are not well studied (Forge et al., 2005). Huhta et al. (1986) suggested that soil invertebrate communities are less sensitive to moderate fertilizer pulses compared to soil microbes and that fertilizer application affects soil fauna primarily indirectly through changes in vegetation and soil properties (see also Birkhofer et al., 2011; Zhu et al., 2016). However, the short-term alteration of soil microbial and fungal communities by fertilization (Lazcano et al., 2013) likely has an indirect effect of the activity of soil mesofauna. Nutrient availability changes the feeding behavior of soil mesofauna (Tao et al., 2016) which can influence soil functioning through altered nutrient cycles. Livestock grazing has similar effects, as nutrients are deposited in pastures (Haynes and Williams, 1993; Chaneton et al., 1996).
Here we address the questions (a) if experimental fertilization with an organic plant-sourced fertilizer rapidly (over a few days) affects the taxonomic composition or feeding activity of the soil mesofauna compared to the long-term strategy of nutrient management over 3 years and (b) if effects of N-pulses through experimental fertilization are independent of the long-term strategy of nutrient management. To answer these questions, we studied effects of experimental fertilization on the taxonomic composition and feeding activity of the soil mesofauna (Collembola, Oribatida, and Nematoda) immediately after application in permanent grasslands along a gradient of long-term nutrient management strategies and in three regions across Germany [German Research Foundation (DFG) Biodiversity Exploratories, Fischer et al., 2010]. We hypothesize that (H1) feeding activity of soil invertebrates increases rapidly in subplots after the experimental application of an organic fertilizer and (H2) taxonomic composition of the soil mesofauna primarily responds to long-term nutrient management strategies, but not rapidly to experimental fertilization. Finally, we hypothesize that feeding activity in grasslands with very low long-term fertilization levels or livestock densities respond more strongly to experimental fertilization than those with higher nutrient inputs (H3).
Materials and Methods
Sampling Sites and Experimental Design
Sampling was conducted in spring and autumn 2009 on 12 permanent agricultural grasslands in each of the three regions of the DFG Biodiversity Exploratories in Germany (Fischer et al., 2010): Schwäbische Alb (AEG), Hainich-Dün (HEG), and Schorfheide-Chorin (SEG) (Table 1). The sampling sites were selected along a gradient of land-use intensities based on a standardized annual questionnaire for farmers including information about the mowing frequency, livestock density and the amount of N fertilization (Vogt et al., 2019). In our study, we used the available 3-year averages (2006–2008) of the livestock density (livestock unit days of grazing ha–1 year–1) and N fertilizer amount (kg nitrogen ha–1 year–1) (Table 1) standardized across the three study regions as predictor variables for the long-term nutrient management strategy of each grassland (Blüthgen et al., 2012). Sampling season, soil properties, vegetation structure and geographic location were recorded as additional predictors to reflect environmental heterogeneity (Table 2 and section “Soil Fauna, Vegetation, and Soil Properties” for details). At each grassland site, two subplots with a size of 5 m × 5 m were established with a minimum distance from the grassland edge of 20 and 2 m distance from each other. We randomly selected one subplot as control and the other as experimental fertilizer treatment subplot. The latter was fertilized with 2,000 kg ha–1 of an organic plant-sourced commercial fertilizer [N = 6.67% (=133.4 kg N ha–1), P2O2 = 0.85%, K2O = 0.86%; AGROBIOSOL®, SW-Düngesysteme, Wolfenbüttel, Germany] in spring and autumn 2009 (for dates see Table 1). This plant-sourced fertilizer is certified for organic agriculture in the EU and is produced by fermenting agricultural by-products (e.g., soy flour or sugars), fungal biomass growth and subsequent drying of the material. The control subplots were not experimentally fertilized.
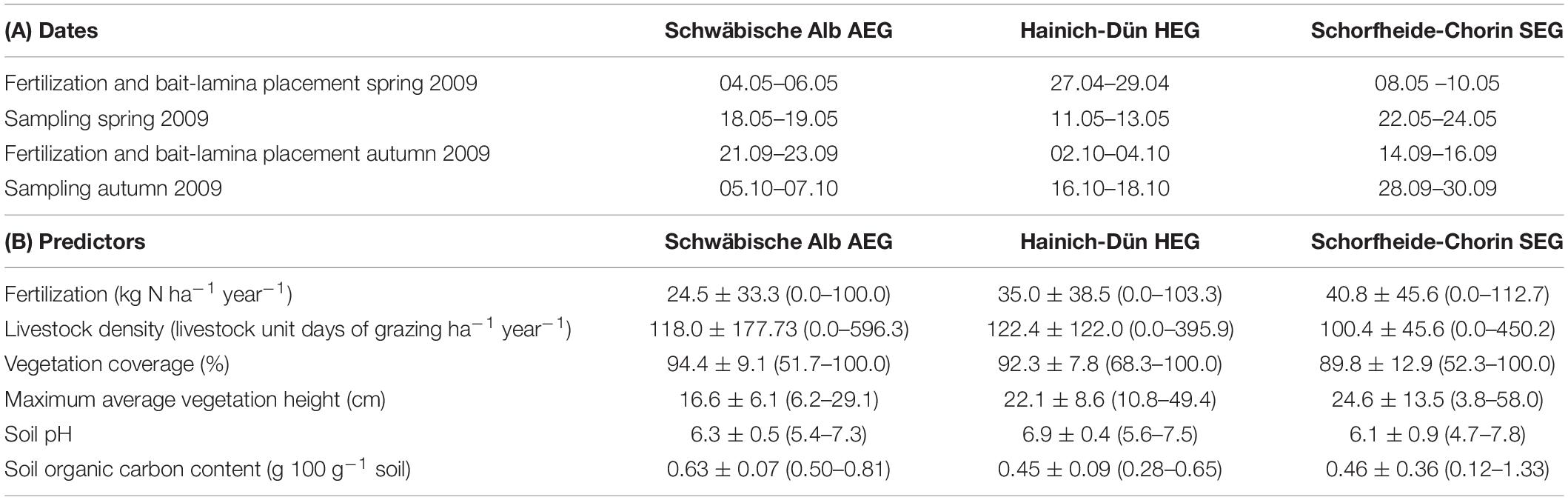
Table 1. (A) Dates of experimental fertilization, placement and collection of bait-lamina strips and soil fauna sampling in spring and autumn 2009, (B) 3-year averages (2006–2008) of N fertilization and livestock density, maximum average vegetation height, vegetation surface coverage, soil pH and soil organic carbon content (means ± SD and ranges) for the 12 grasslands sites in each of the three study regions.
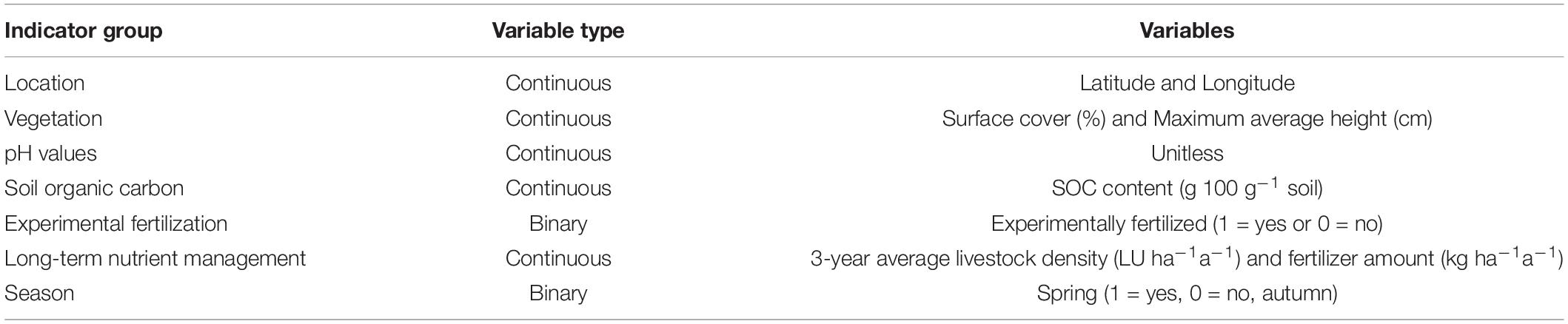
Table 2. Indicator groups and the individual variables in each indicator group as used in all distance-based linear models (DistLM, McArdle and Anderson, 2001).
Feeding Activity
The bait-lamina test provides a standardized method to estimate the feeding activity of soil mesofauna (Törne, 1990; Kratz, 1998). In spring and autumn 2009 feeding activity of soil fauna was investigated by using the bait-lamina test following the schedule in Table 1. Bait-lamina strips with a size of 6 mm × 160 mm had 16 holes of 1.5 mm diameter arranged in a single vertical row with 5 mm intervals. The bait substrate consisted of cellulose, agar-agar, bentonite, and bran flakes following Eisenbeis et al. (1996). Consumption of this bait substrate over time provides an estimate of the feeding activity of soil macro- and mesofauna under different environmental conditions (Birkhofer et al., 2011) or agricultural management practices (Birkhofer et al., 2021). To avoid displacement of the bait, the bait-lamina strips were inserted into the soil in a preformed vertical slit using a stainless-steel metal knife. The top hole was inserted to surface level and the bottom hole reached 7 cm depth. At each date, each subplot received 8 bait-lamina strips in a 2 × 4 arrangement with 10 cm spacing between strips. Bait-lamina strips were collected after 14 days and the feeding activity was assessed by counting the number of completely empty holes (Vorobeichik and Bergman, 2021).
Soil Fauna, Vegetation, and Soil Properties
In each subplot, four intact soil cores with a diameter of 8 cm and a depth of 5 cm were sampled, resulting in 288 soil cores per sampling season (36 grassland sites × 2 subplots × 4 soil cores). Soil fauna was extracted using a modified Kempson extraction method (Kempson et al., 1963). Heat extraction was performed over 8 days with stepwise (5°C) increasing temperatures every day from 20 to 60°C. All adult Oribatida and Collembola from these samples were identified to species level. To sample Nematoda communities in each subplot, nine samples were collected as intact soil cores with a diameter of 3 cm and a depth of 10 cm. We extracted nematodes from 100 g bulk soil per subplot following a modified Oostenbrink elutriator-cottonwool filter method. An aliquot containing about 100 nematode individuals was randomly taken from each extracted sample for identification. The specimens were then preserved in 4% formalin and identified to genus level. Nematoda abundance was not analyzed, as only a predefined number of individuals was identified to genus level and counted.
Vegetation cover and maximum average height were measured in three 1 m × 1 m quadrats per subplot at each sampling date. Surface cover was visually estimated on a percentage scale and maximum average height was measured for the highest plant in each corner of the 1 m × 1 m quadrat. Soil pH was measured for each subplot in a solution of CaCl2 (10 g bulk soil in 25 ml CaCl2) using an electrical pH sensor (with a resolution of two decimals). Soil organic carbon content was quantified for each subplot with the loss-on-ignition (LOI) method for bulk soil quantities between 2.89 and 3.57 g. Bulk soil samples for pH value and soil organic carbon content measurements consisted of three samples of the upper 5 cm in each subplot.
Statistical Analyses
To account for the large number of potentially relevant predictors in this study (Table 2) and the multivariate nature of taxonomic community composition data, we used distance-based linear models (DistLM) as an appropriate method for multivariate, multiple regression analyses of community data (McArdle and Anderson, 2001). These regression models allow for the analyses of uni- and multivariate dependent data based on the selection of an appropriate resemblance measure to create resemblance matrices for all pairs of samples. For a general flowchart of the DistLM analyses refer to Supplementary Figure 1. Community composition data were analyzed separately for the three taxonomic groups (Collembola, Oribatida, and Nematoda) based on log (x + 1) transformed abundance data of all species (Collembola, Oribatida) or genera (Nematoda) using the Bray-Curtis similarity measure. Transformation of abundances was performed to weight down the importance of very abundant compared to less abundant taxa (Anderson et al., 2008). The univariate variables feeding activity, Collembola, Oribatida and Nematoda taxonomic richness and total abundance were individually analyzed based on untransformed data and Euclidean distances. Model selection in all DistLMs was based on the AIC selection criterion and a stepwise selection procedure (combining forward and backward selection) using the indicator groups listed in Table 2. Location, as individual plot coordinates, was fitted prior to all model selection procedures to account for geographic distribution. In case of significant relationships between indicator groups and univariate dependent variables, Pearson correlations were calculated between the dependent variable and each individual independent variable in the respective indicator group (Table 2). In case of a significant relationship between a categorical indicator group (coded binary) and a univariate dependent variable, means and 95% confidence intervals were compared between levels of the respective indicator variable(s). Effects of indicators selected in DistLMs and the respective individual predictor variables on multivariate community data are shown in distance-based RDA ordinations. Distance-based linear models test for sequential, additive effects under hypotheses H1 and H2, but not for interdependencies between predictors (H3). We therefore used additional permutational analysis of variance (PERMANOVA, Anderson, 2014) models to explicitly test if effects of experimental fertilization statistically depend on the long-term nutrient management strategy of each grassland to address H3. In PERMANOVA models, we used experimental fertilization as categorical fixed factor and the 3-year average of fertilizer amount as covariable including the interaction between both model terms. All PERMANOVA models were based on the same resemblance matrices used for DistLMs and were calculated with type III sums of squares and 9999 permutations of residuals under a reduced model (Anderson, 2014). All statistical analyses were performed in PRIMER 7.0.21 with the PERMANOVA + 1 add-on (PRIMER-e).
Results
Soil Fauna Feeding Activity
Experimental fertilization significantly affected soil fauna feeding activity estimated with bait lamina strips (Table 3A), with feeding activity being 1.6 times lower in experimentally fertilized subplots (Figure 1). Long-term nutrient management and soil pH were selected as additional predictors in the final model, but they had no significant effect on soil fauna feeding activity and only explained very low proportion in the variation of feeding activity (Table 3A). The effect of experimental fertilization did not depend significantly on the long-term fertilization strategy (PERMANOVA: Pseudo-F1,68 = 0.30, P = 0.583).
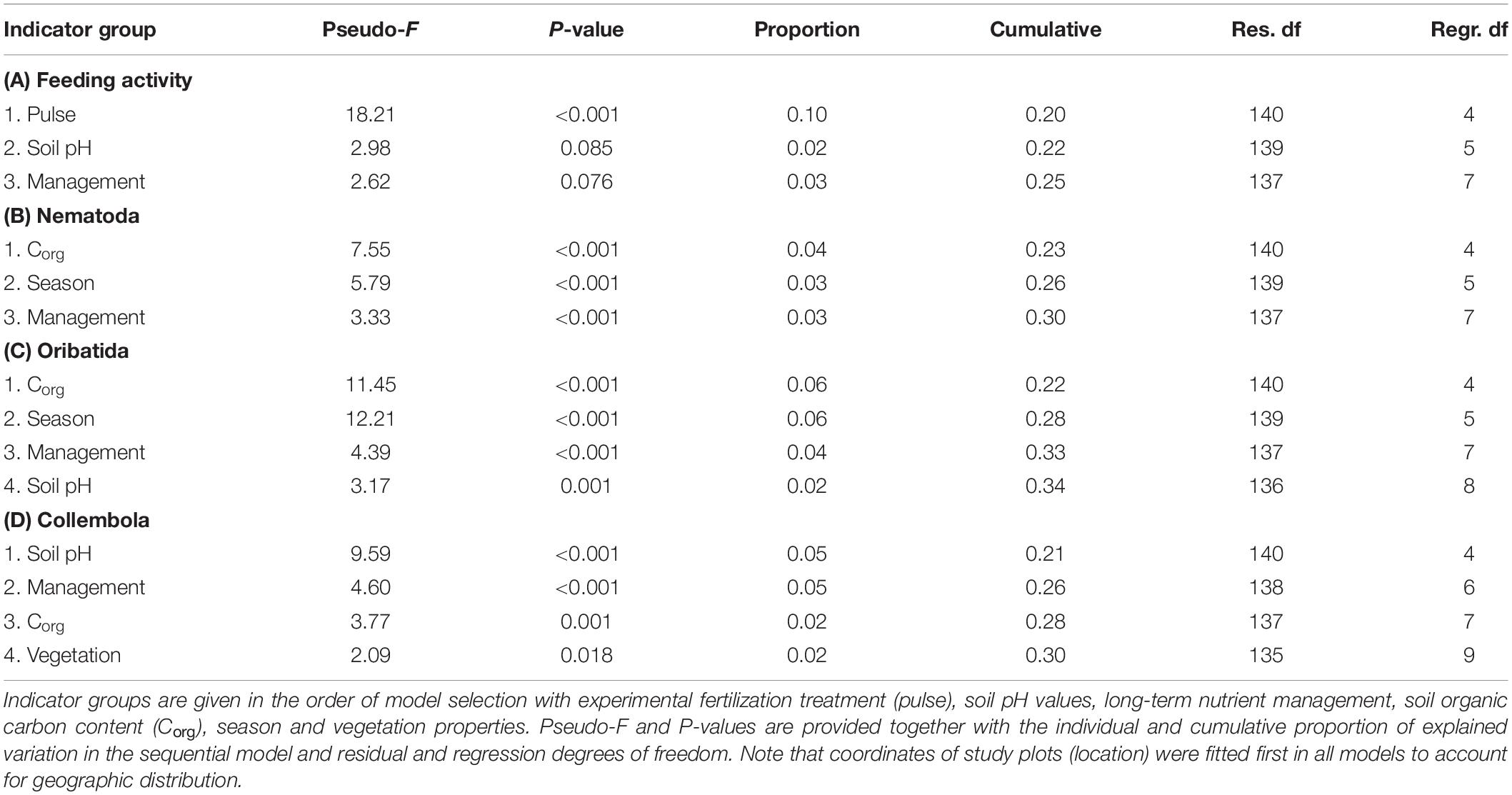
Table 3. Results of distance-based linear models (DistLMs) testing for effects of indicator groups (Table 2) as predictors for (A) soil fauna feeding activity, (B) Nematoda, (C) Oribatida, and (D) Collembola community composition.
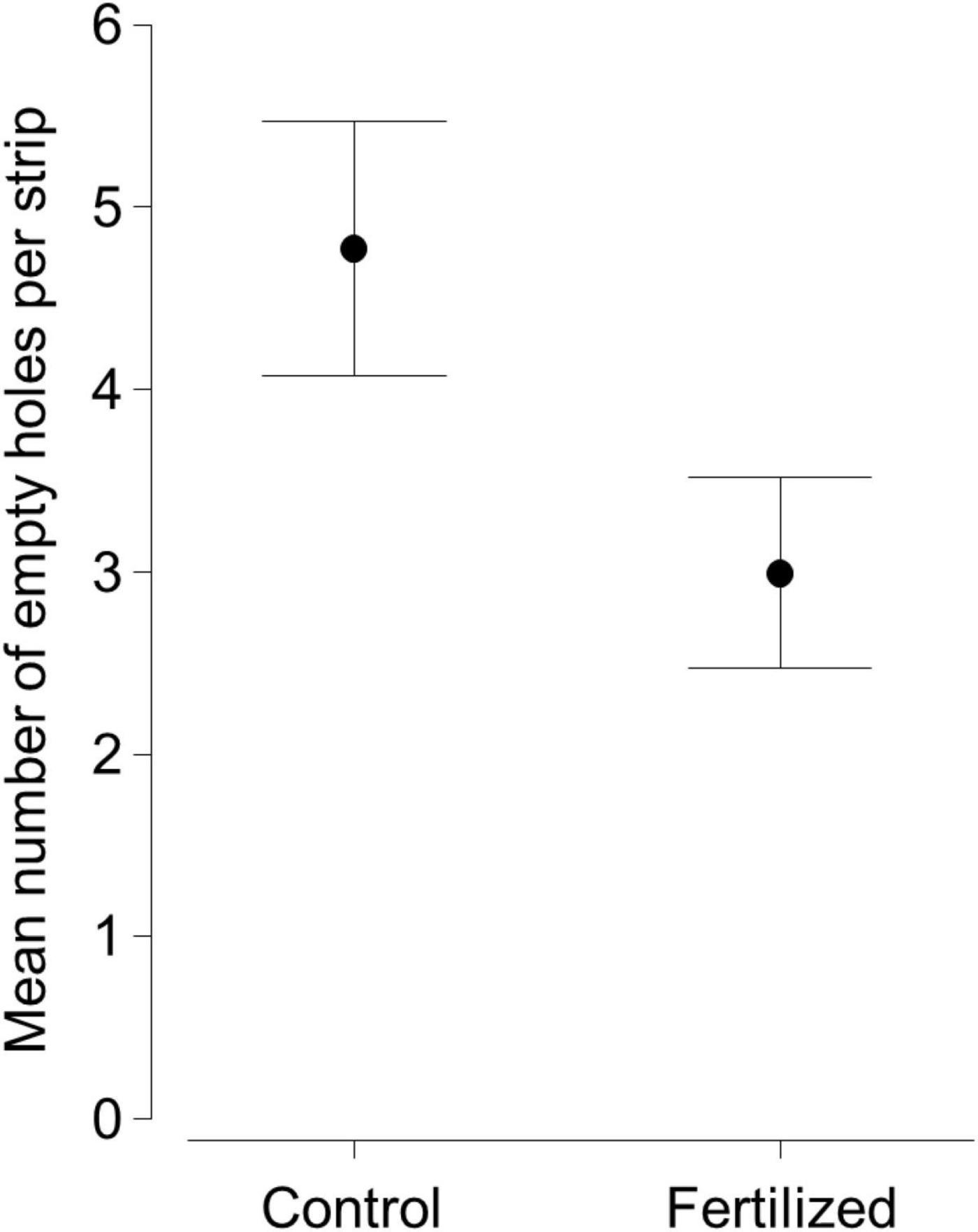
Figure 1. Mean feeding activity as the mean number of empty holes per bait-lamina strip with 95% confidence intervals for the number of empty holes per bait strip in subplots without (Control; N = 72) and with (Fertilized, N = 72) experimental fertilization (Pseudo-F140,4 = 18.21, P < 0.001).
Taxonomic Richness and Abundance
The long-term nutrient management strategy had a significant effect on the taxonomic richness of all soil faunal groups studied (Tables 4A–C), while experimental fertilization did not affect taxonomic richness. Nematoda (Pearson correlation R = −0.166, P = 0.047; Supplementary Figure 2A) and Oribatida (R = −0.327, P < 0.001; Supplementary Figure 2A) richness and Oribatida abundance (R = −0.210, P = 0.012; Supplementary Figure 2B) were negatively correlated to the long-term average fertilizer amount. Collembola richness was positively correlated to the livestock density (R = 0.169, P = 0.043; Supplementary Figure 3). Seasonal differences were significant in Nematoda and Collembola richness, and Nematoda richness was further negatively affected by soil organic carbon content (Table 4A). Nematoda communities on average had two (AEG, SEG) or three (HEG) genera fewer in autumn than in spring. Oribatida communities on average had two more species in spring in AEG, one fewer in spring in SEG, with no differences observed for HEG. Collembola abundance was only significantly affected by location (Pseudo-F141,3 = 28.65, Prop. = 0.29, P < 0.001) and Oribatida abundance was significantly and negatively affected by long-term nutrient management (Pseudo-F139,5 = 35.25, Prop. = 0.08, P = 0.003), but not by experimental fertilization.
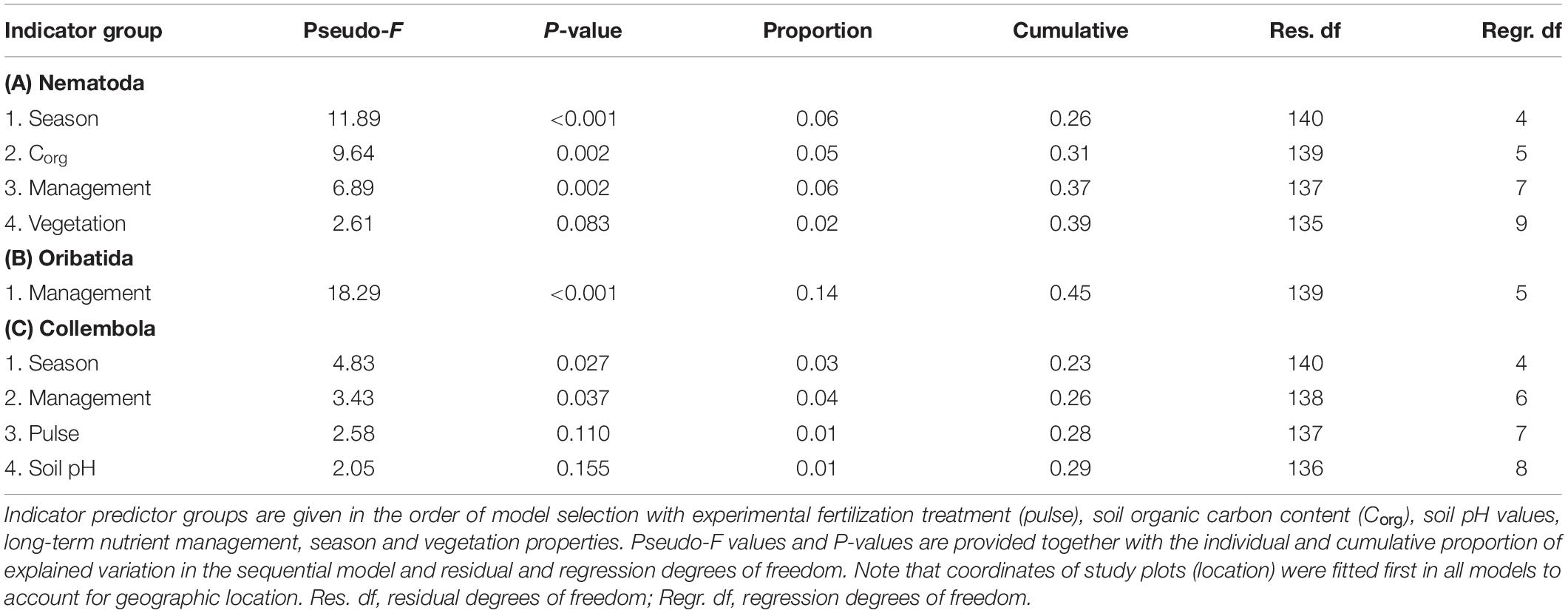
Table 4. Results of distance-based linear models (DistLMs) testing for effects of indicator groups (Table 2) as predictors for (A) Nematoda genus, (B) Oribatida species, and (C) Collembola species richness.
Community Composition
Nematode communities differed markedly between locations and between spring and autumn samples (Figure 2). Nematode communities in HEG were characterized by higher abundances of Helicotylenchus and Amplimerlinius. SEG plots had higher abundances of Tylenchorhynchidae and Eucephalobus, while representatives of Prodorylaimus were more abundant in grassland soils in AEG and HEG. Soil organic carbon content and long-term nutrient management were selected in the final model (Table 3B), with higher abundances of Tylenchorhynchidae and Eucephalobus with increasing long-term fertilization (Figure 2). Experimental fertilization neither significantly affected Nematoda community composition in the final model nor in marginal tests for individual predictors.
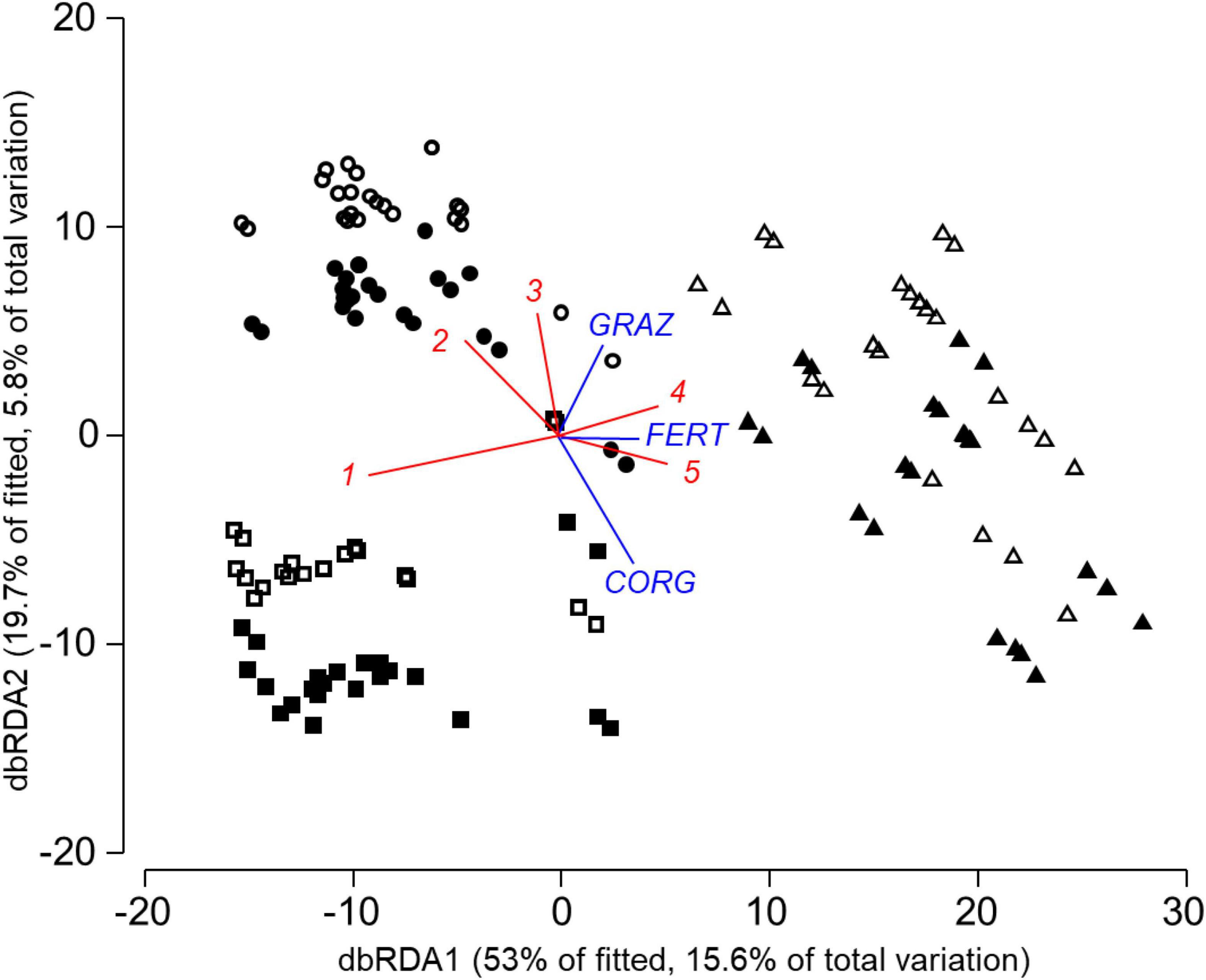
Figure 2. Distance-based RDA plot of study plots based on Nematode community data and the model in Table 3B from AEG (◼), HEG (•) and SEG (▲) with samples from spring (solid) and autumn (open) 2008. Individual predictor variables from selected indicator groups of the model in Table 3B are superimposed (CORG, soil organic carbon content; GRAZ, average livestock density 2006–2008; FERT, average fertilizer amount 2006–2008). Nematoda genera with correlation coefficients >0.3 between axis scores of sites and abundances are superimposed with (1) Prodorylaimus, (2) Helicotylenchus, (3) Amplimerlinius, (4) Tylenchorhynchidae, and (5) Eucephalobus.
Communities of oribatid mites differed between locations and between spring and autumn samples (Figure 3). Oribatid communities in autumn were characterized by higher abundances of Malaconothrus monodactylus. Communities in AEG and some communities in SEG had high abundances of Eupelops acromios and Achipteria coleoptrata, whereas communities in HEG and some communities in SEG were characterized by high abundances of Tectocepheus velatus. Subplots with higher soil organic carbon content and pH values, but lower long-term fertilization had higher abundances of A. nitens, A. coleoptrata, and E. acromios and lower abundances of T. velatus. Experimental fertilization neither affected Oribatida community composition significantly in the final model nor in marginal tests for individual predictors (Table 3C).
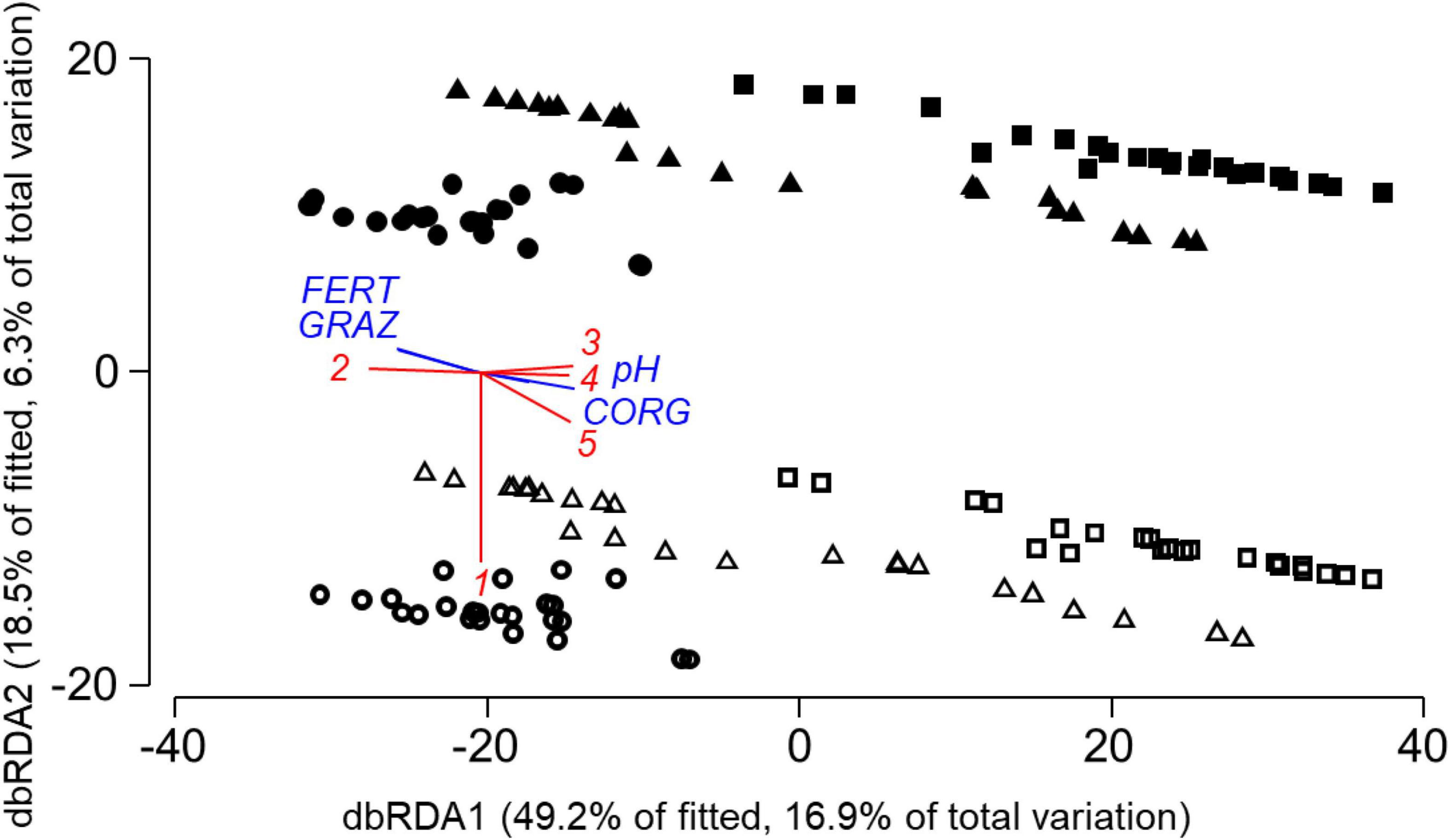
Figure 3. Distance-based RDA plot of study plots based on oribatid mite community data and the model in Table 3C from AEG (◼), HEG (•) and SEG (▲) with samples from spring (solid) and autumn (open) 2009. Individual predictor variables from selected indicator groups of the model in Table 3C are superimposed (CORG, soil organic carbon content; pH, soil pH value; GRAZ, average livestock density 2006–2008; FERT, average fertilizer amount 2006–2008). Oribatida species with correlation coefficients >0.3 between axis scores of sites and abundances are also superimposed with (1) Malaconothrus monodactylus, (2) Tectocepheus velatus, (3) Eupelops acromios, (4) Achipteria coleoptrata, and (5) A. nitens.
Unlike nematode and oribatid communities, the composition of Collembola communities differed between locations, but not between spring and autumn sample dates (Figure 4). Folsomia quadrioculata, Lepidocyrtus lanuginosus, and Parisotoma notabilis were more abundant in AEG and HEG than in SEG. The abundances of L. lanuginosus and P. notabilis increased with soil pH values, F. quadrioculata and L. lanuginosus were positively related to livestock density (Table 3D). Experimental fertilization neither affected Collembola community composition significantly in the final model nor in marginal tests for individual predictors.
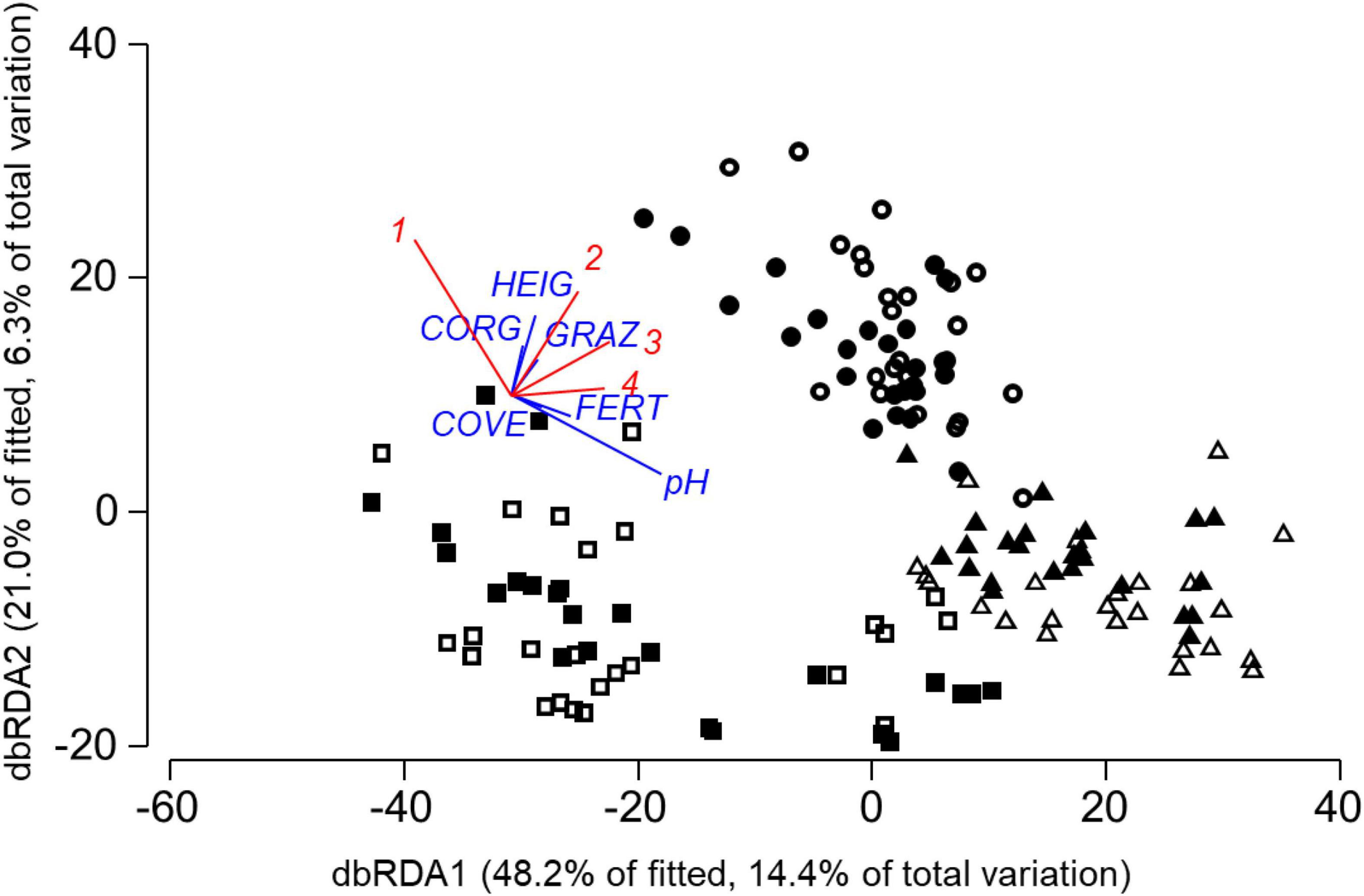
Figure 4. Distance-based RDA plot of study plots based on Collembola community data and the model in Table 3D from AEG (◼), HEG (•) and SEG (▲) with samples from spring (solid) and autumn (open) 2009. Individual predictor variables from selected indicator groups of the model in Table 3D are superimposed (CORG, soil organic carbon content; pH, soil pH value; GRAZ, average livestock density 2006–2008; FERT, average fertilizer amount 2006–2008; COVE, vegetation cover; HEIG, vegetation height). Collembola species with correlation coefficients >0.3 between axis scores of sites and abundances are also superimposed with (1) Isotoma viridis, (2) Folsomia quadrioculata, (3) Lepidocyrtus lanuginosus, and (4) Parisotoma notabilis.
Discussion
Soil fauna feeding activity responded to experimental fertilization with a plant-sourced organic fertilizer rapidly, within 14 days after application. This response was independent of the changes in taxonomic community composition, richness and total abundance associated with long-term nutrient management. Thus, in terms of immediate response, edaphic mesofauna communities do not appear to adapt to external inputs. Considering the impact of nitrogen availability on priming effects in the root system (Dijkstra et al., 2013), this may have serious consequences, e.g., for the carbon storage in grassland ecosystems and should therefore caution relevant actors (e.g., Manolache et al., 2020) involved in the development of future grassland management strategies.
The almost immediate reduction of the feeding activity does not confirm results from previous studies with other organic fertilizers (Pfotzer and Schüler, 1997) nor our first hypothesis. It might be explained by an increase in soil microbial biomass from experimental fertilizer pulses (Ali et al., 2021) and the manufacturer of the AGROBIOSOL® organic fertilizer claims that the application “activates” soil life. Microbes provide an alternative protein-rich food source for soil invertebrates and their increased availability may have drastically reduced the attractiveness of protein-poor bait lamina substrates (Li et al., 2020). The rapid response of the mesofauna reported here is consistent with the fast negative effect of chemical fertilizer application on soil fauna feeding activity in tropical oil palm agroecosystems compared to the application of plant residues (Tao et al., 2016). The generality and permanence of organic and chemical fertilizer effects on soil mesofauna in grassland soils nevertheless needs to be investigated in the future. Wahyuningsih et al. (2019), for example, even reported an initial positive response of feeding activity to the application of chemical nitrogen fertilizer within a few days after application. Alternatively, the application of organic fertilizer may have rapidly induced a certain level of feeding inactivity in soil fauna communities, but this explanation does not seem very probable based on our existing knowledge.
Soil invertebrate species composition, taxonomic richness and overall abundance were significantly altered by long-term nutrient management for all taxa. This confirms our second hypothesis and proves that the management regime strongly influences the structure of belowground communities (see also Degtyarev et al., 2019; Korobushkin et al., 2019; Saifutdinov et al., 2020). Given the duration of the meso- and microfauna life cycles (Hopkin, 1997; Khan and Kim, 2007) and the comparatively small-scale fertilizer application (see section “Materials and Methods”), immediate community changes due to demographic responses or to lethal and repulsive effects are highly unlikely. However, our study suggests that rapid changes in feeding activity must not be accompanied by structural changes of the soil community. The evaluation of management effects on grassland soils must thus consider both short-term and long-term responses of edaphic biota. The fact that the species richness of Oribatida was only affected by long-term nutrient management confirms that this taxon is less sensitive to pulse stress (sensu Bengtsson, 2002) and rather reflect conditions over longer temporal scales (Zaitsev et al., 2013). Land-use intensity is known to vary between years in the studied grasslands (Allan et al., 2014) and soil taxa such as Oribatida may rather respond to this inter-annual variation.
Our results indicate that the response to fertilization and to local variation of soil properties is dominated by a relatively small number of species in each of the taxonomic groups. The remaining species form a species pool that varies locally from region to region and, to a lesser extent, from season to season. As in other studies (Bongers and Bongers, 1998; Yeates, 1999), organic fertilization increased the relative density of some bacterivorous nematodes (e.g., Eucephalobus in Figure 2). This suggests that the experimental fertilization not only promoted microbial growth, but also shifted the soil fungal to bacteria ratio toward bacteria (see also De Vries et al., 2006). Interestingly, as previously indicated also for forests (Zaitsev et al., 2013), only Oribatida species with rapid generation time like the non-specialized T. velatus responded to experimental fertilization pulses. Larger sexually reproducing, fungivorous and herbifungivorous species in the genus Achipteria rather responded to soil conditions which generally reflect land-use history. Compared, to Oribatida, the weaker response of Collembola species to experimental fertilization may be best explained by the higher vertical and horizontal mobility in comparison to the other soil taxa in this study (Hopkin, 1997; Meyer et al., 2021). Very strong seasonal variation of Oribatida communities highlights the need for multiple sampling campaigns over the year to realistically determine soil microarthropod diversity and community composition.
Conclusion
The rapid change in feeding activity proves the sensitivity of meso- and microfauna in permanent grassland soils to organic fertilizer application. This response is probably due to the stimulation of microbial growth, especially that of bacteria, in organically fertilized soils. Several studies based on food web analysis support this assumption by showing a shift from the fungal and to the bacterial channel in fertilized grassland soils (Bardgett et al., 1996; De Vries et al., 2006; Denef et al., 2009). However, detecting changes in nutrient or energy fluxes through soil food web analysis requires massive structural changes in the community, as these methods derive estimates of matter fluxes based on abundance or biomass changes. Therefore, these approaches cannot detect immediate changes in feeding activity that are not accompanied by changes in community structure, as was the case in our study. We hypothesize that food web analyses underestimate the ecological consequences of intensive management because they do not account for animal behavior. Since short-term and small-scale processes play a key role in the rhizosphere (Philippot et al., 2013), there is an urgent need to develop nutrient management strategies for permanent grasslands that take into account both the conservation of the edaphic community and changes of ecosystem functions caused by rapid responses of the soil mesofauna to fertilizer inputs. These considerations are fundamentally important as a more sustainable management of agricultural permanent grasslands is a key component of ongoing discussions about further developing the common agricultural policy in the EU (Peeters et al., 2020; Montanarella and Panagos, 2021).
Data Availability Statement
The datasets presented in this article are not readily available because datasets are stored as non-public data in the Information System of the Biodiversity Exploratories (BEXIS id’s 31171, 31174, 31176, and 31181), but are available upon request. Requests to access the datasets should be directed to KB, S2xhdXMuQmlya2hvZmVyQGItdHUuZGU=.
Author Contributions
KB, TD, and VW contributed to the conception and design of the study. KB and TD performed the field work. AZ coordinated the identification of Acari and Collembola samples. KB and DB performed the statistical analysis. KB wrote the first draft of the manuscript. DB, TD, AZ, and VW wrote sections of the manuscript. All authors contributed to manuscript revision, read, and approved the submitted version.
Funding
This work has been funded by the DFG Priority Program 1374 “Biodiversity Exploratories.” Field work permits were issued by the responsible state environmental offices of Baden-Württemberg, Thüringen, and Brandenburg. Identification of oribatid mites and trait allocation was partially supported by the RFBR project 18-29-25067.
Conflict of Interest
The authors declare that the research was conducted in the absence of any commercial or financial relationships that could be construed as a potential conflict of interest.
Publisher’s Note
All claims expressed in this article are solely those of the authors and do not necessarily represent those of their affiliated organizations, or those of the publisher, the editors and the reviewers. Any product that may be evaluated in this article, or claim that may be made by its manufacturer, is not guaranteed or endorsed by the publisher.
Acknowledgments
We appreciate the comments of two referees and the editor that helped to improve the manuscript. We thank the managers of the three Exploratories, Konstans Wells, Swen Renner, Sonja Gockel, and Andreas Hemp for their work in maintaining the plot and project infrastructure; Simone Pfeiffer and Maren Gleisberg for giving support through the central office; Jens Nieschulze for managing the central data base; and Markus Fischer, Eduard Linsenmair, Dominik Hessenmöller, Daniel Prati, Ingo Schöning, François Buscot, Ernst-Detlef Schulze, Wolfgang W. Weisser, and the late Elisabeth Kalko for their role in setting up the Biodiversity Exploratories project. We also thank the administration of the Hainich National Park, UNESCO Biosphere Reserve Swabian Alb, and UNESCO Biosphere Reserve Schorfheide-Chorin as well as all land owners for the excellent collaboration.
Supplementary Material
The Supplementary Material for this article can be found online at: https://www.frontiersin.org/articles/10.3389/fevo.2022.864470/full#supplementary-material
References
Ali, S., Dongchu, L., Jing, H., Ahmed, W., Abbas, M., Qaswar, M., et al. (2021). Soil microbial biomass and extracellular enzymes regulate nitrogen mineralization in a wheat-maize cropping system after three decades of fertilization in a Chinese Ferrosol. J. Soils Sediments 21, 281–294. doi: 10.1007/s11368-020-02770-5
Allan, E., Bossdorf, O., Dormann, C. F., Prati, D., Gossner, M. M., Tscharntke, T., et al. (2014). Interannual variation in land-use intensity enhances grassland multidiversity. Proc. Natl. Acad. Sci. U.S.A. 111, 308–313. doi: 10.1073/pnas.1312213111
Anderson, M., Gorley, R., and Clarke, K. P. (2008). PRIMER: Guide to Software and Statistical Methods. Plymouth: Primer-e.
Anderson, M. J. (2014). Permutational Multivariate Analysis of Variance (PERMANOVA). Hoboken, NJ: Wiley.
Bardgett, R. D., Hobbs, P. J., and Frostegård, Å (1996). Changes in soil fungal: bacterial biomass ratios following reductions in the intensity of management of an upland grassland. Biol. Fertil. Soils 22, 261–264. doi: 10.1007/bf00382522
Bardgett, R. D., and Van Der Putten, W. H. (2014). Belowground biodiversity and ecosystem functioning. Nature 515, 505–511. doi: 10.1038/nature13855
Bengtsson, J. (2002). Disturbance and resilience in soil animal communities. Eur. J. Soil Biol. 38, 119–125. doi: 10.1016/s1164-5563(02)01133-0
Birkhofer, K., Bezemer, T. M., Bloem, J., Bonkowski, M., Christensen, S., Dubois, D., et al. (2008). Long-term organic farming fosters below and aboveground biota: implications for soil quality, biological control and productivity. Soil Biol. Biochem. 40, 2297–2308. doi: 10.1016/j.soilbio.2008.05.007
Birkhofer, K., Bezemer, T. M., Hedlund, K., and Setälä, H. (2012). “Community composition of soil organisms under different wheat farming systems,” in Microbial Ecology in Sustainable Agroecosystems. Advances in Agroecology, eds T. Cheeke, D. C. Coleman, and D. H. Wall (Boca Raton, FL: CRC Press).
Birkhofer, K., Diekötter, T., Boch, S., Fischer, M., Müller, J., Socher, S., et al. (2011). Soil fauna feeding activity in temperate grassland soils increases with legume and grass species richness. Soil Biol. Biochem. 43, 2200–2207. doi: 10.1016/j.soilbio.2011.07.008
Birkhofer, K., Fliessbach, A., Gavín-Centol, M. P., Hedlund, K., Ingimarsdóttir, M., Jørgensen, H. B., et al. (2021). Conventional agriculture and not drought alters relationships between soil biota and functions. Sci. Rep. 11, 1–12. doi: 10.1038/s41598-021-03276-x
Blüthgen, N., Dormann, C. F., Prati, D., Klaus, V. H., Kleinebecker, T., Hölzel, N., et al. (2012). A quantitative index of land-use intensity in grasslands: integrating mowing, grazing and fertilization. Basic Appl. Ecol. 13, 207–220. doi: 10.1016/j.baae.2012.04.001
Bongers, T., and Bongers, M. (1998). Functional diversity of nematodes. Appl. Soil Ecol. 10, 239–251. doi: 10.1016/s0929-1393(98)00123-1
Chaneton, E. J., Lemcoff, J. H., and Lavado, R. S. (1996). Nitrogen and phosphorus cycling in grazed and ungrazed plots in a temperate subhumid grassland in Argentina. J. Appl. Ecol. 33, 291–302. doi: 10.2307/2404751
De Vries, F. T., Hoffland, E., van Eekeren, N., Brussaard, L., and Bloem, J. (2006). Fungal/bacterial ratios in grasslands with contrasting nitrogen management. Soil Biol. Biochem. 38, 2092–2103. doi: 10.1016/j.soilbio.2006.01.008
Degtyarev, M. I., Korobushkin, D. I., Gongalsky, K. B., and Zaitsev, A. S. (2019). Enchytraeid Community (Annelida, Clitellata, Enchytraeidae) and its dependence on edaphic conditions in rice agroecosystems in Russia. Russian J. Ecol. 50, 384–390. doi: 10.1134/s1067413619040064
Delgado-Baquerizo, M., Reich, P. B., Trivedi, C., Eldridge, D. J., Abades, S., Alfaro, F. D., et al. (2020). Multiple elements of soil biodiversity drive ecosystem functions across biomes. Nat. Ecol. Evol. 4, 210–220. doi: 10.1038/s41559-019-1084-y
Denef, K., Roobroeck, D., Wadu, M. C. M., Lootens, P., and Boeckx, P. (2009). Microbial community composition and rhizodeposit-carbon assimilation in differently managed temperate grassland soils. Soil Biol. Biochem. 41, 144–153. doi: 10.1016/j.soilbio.2008.10.008
Dijkstra, F. A., Carrillo, Y., Pendall, E., and Morgan, J. A. (2013). Rhizosphere priming: a nutrient perspective. Front. Microbiol. 4:216. doi: 10.3389/fmicb.2013.00216
Ding, J., Zhu, D., Chen, Q.-L., Zheng, F., Wang, H.-T., and Zhu, Y.-G. (2019). Effects of long-term fertilization on the associated microbiota of soil collembolan. Soil Biol. Biochem. 130, 141–149. doi: 10.1016/j.soilbio.2018.12.015
Eisenbeis, G., Lenz, R., Dogan, H., Schuler, G., and Pfadenhauer, J. (1996). Zur biologischen Aktivitäit von Nadelwaldböden: messung der tierischen Frassaktivität mit dem Köderstreifen-Test sowie Bestimmung yon Streuabbauraten mit dem Minicontainer-Test. Verhandlungen Gesellschaft für Ökologie 26, 305–311.
Eisenhauer, N., Antunes, P. M., Bennett, A. E., Birkhofer, K., Bissett, A., Bowker, M. A., et al. (2017). Priorities for research in soil ecology. Pedobiologia 63, 1–7. doi: 10.1016/j.pedobi.2017.05.003
Fischer, M., Bossdorf, O., Gockel, S., Hänsel, F., Hemp, A., Hessenmöller, D., et al. (2010). Implementing large-scale and long-term functional biodiversity research: the biodiversity exploratories. Basic Appl. Ecol. 11, 473–485. doi: 10.1016/j.baae.2010.07.009
Forge, T. A., Bittman, S., and Kowalenko, C. G. (2005). Responses of grassland soil nematodes and protozoa to multi-year and single-year applications of dairy manure slurry and fertilizer. Soil Biol. Biochem. 37, 1751–1762. doi: 10.1016/j.soilbio.2004.11.013
Geissen, V., and Brümmer, G. (1999). Decomposition rates and feeding activities of soil fauna in deciduous forest soils in relation to soil chemical parameters following liming and fertilization. Biol. Fertil. Soils 29, 335–342. doi: 10.1007/s003740050562
Haynes, R. J., and Williams, P. H. (1993). Nutrient cycling and soil fertility in the grazed pasture ecosystem. Adv. Agron. 49, 119–199. doi: 10.1016/s0065-2113(08)60794-4
Hopkin, S. P. (1997). Biology of the Springtails (Insecta: Collembola). Oxford: Oxford University Press.
Hristov, J., Clough, Y., Sahlin, U., Smith, H. G., Stjernman, M., Olsson, O., et al. (2020). Impacts of the EU’s Common Agricultural Policy “Greening” reform on agricultural development, biodiversity, and ecosystem services. Appl. Econ. Perspect. Policy 42, 716–738. doi: 10.1002/aepp.13037
Huhta, V., Hyvönen, R., Koskenniemi, A., Vilkamaa, P., Kaasalainen, P., and Sulander, M. (1986). Response of soil fauna to fertilization and manipulation of pH in coniferous forests. Acta Forest. Fennica 195:7641.
Kempson, D., Lloyd, M., and Ghelardi, R. (1963). A new extractor for woodland litter. Pedobiologia 3, 1–21.
Khan, Z., and Kim, Y. H. (2007). A review on the role of predatory soil nematodes in the biological control of plant parasitic nematodes. Appl. Soil Ecol. 35, 370–379. doi: 10.1016/j.apsoil.2006.07.007
Korobushkin, D. I., Butenko, K. O., Gongalsky, K. B., Saifutdinov, R. A., and Zaitsev, A. S. (2019). Soil nematode communities in temperate rice-growing systems. Eur. J. Soil Biol. 93:103099. doi: 10.1016/j.ejsobi.2019.103099
Kratz, W. (1998). The bait-lamina test: general aspects, applications and perspectives. Environ. Sci. Pollut. Res. 5, 94–96. doi: 10.1007/BF02986394
Lazcano, C., Gómez-Brandón, M., Revilla, P., and Domínguez, J. (2013). Short-term effects of organic and inorganic fertilizers on soil microbial community structure and function. Biol. Fertil. Soils 49, 723–733. doi: 10.1007/s00374-012-0761-7
Li, J., Zhang, X. C., Luo, J. F., Lindsey, S., Zhou, F., Xie, H. T., et al. (2020). Differential accumulation of microbial necromass and plant lignin in synthetic versus organic fertilizer-amended soil. Soil Biol. Biochem. 149:107967. doi: 10.1016/j.soilbio.2020.107967
Manolache, S., Nita, A., Hartel, T., Miu, I. V., Ciocanea, C. M., and Rozylowicz, L. (2020). Governance networks around grasslands with contrasting management history. J. Environ. Manag. 273:111152. doi: 10.1016/j.jenvman.2020.111152
McArdle, B. H., and Anderson, M. J. (2001). Fitting multivariate models to community data: a comment on distance-based redundancy analysis. Ecology 82, 290–297. doi: 10.1890/0012-9658(2001)082[0290:fmmtcd]2.0.co;2
Meyer, S., Kundel, D., Birkhofer, K., Fliessbach, A., and Scheu, S. (2021). Soil microarthropods respond differently to simulated drought in organic and conventional farming systems. Ecol. Evol. 11, 10369–10380. doi: 10.1002/ece3.7839
Montanarella, L., and Panagos, P. (2021). The relevance of sustainable soil management within the European Green Deal. Land Use Policy 100:104950. doi: 10.1016/j.landusepol.2020.104950
Peeters, A., Lefebvre, O., Balogh, L., Barberi, P., Batello, C., Bellon, S., et al. (2020). A Green Deal for implementing agroecological systems: reforming the common agricultural policy of the European Union. J. Sustain. Organ. Agric. Syst. 70, 83–93.
Pfotzer, G. H., and Schüler, C. (1997). Effects of different compost amendments on soil biotic and faunal feeding activity in an organic farming system. Biol. Agric. Hortic. 15, 177–183. doi: 10.1080/01448765.1997.9755192
Philippot, L., Raaijmakers, J. M., Lemanceau, P., and Van Der Putten, W. H. (2013). Going back to the roots: the microbial ecology of the rhizosphere. Nat. Rev. Microbiol. 11, 789–799. doi: 10.1038/nrmicro3109
Potapov, A. M., Beaulieu, F., Birkhofer, K., Bluhm, S. L., Degtyarev, M. I., Devetter, M., et al. (2022). Feeding habits and multifunctional classification of soil-associated consumers from protists to vertebrates. Biol. Rev. 97, 1057–1117. doi: 10.1111/brv.12832
Saifutdinov, R. A., Sabirov, R. M., and Zaitsev, A. S. (2020). Springtail (Hexapoda: Collembola) functional group composition varies between different biotopes in Russian rice growing systems. Eur. J. Soil Biol. 99:103208. doi: 10.1016/j.ejsobi.2020.103208
Shaw, E. A., Boot, C. M., Moore, J. C., Wall, D. H., and Baron, J. S. (2019). Long-term nitrogen addition shifts the soil nematode community to bacterivore-dominated and reduces its ecological maturity in a subalpine forest. Soil Biol. Biochem. 130, 177–184. doi: 10.1016/j.soilbio.2018.12.007
Tao, H.-H., Slade, E. M., Willis, K. J., Caliman, J.-P., and Snaddon, J. L. (2016). Effects of soil management practices on soil fauna feeding activity in an Indonesian oil palm plantation. Agric. Ecosyst. Environ. 218, 133–140. doi: 10.1016/j.agee.2015.11.012
Törne, E. V. (1990). Assessing feeding activities of soil-living animals. I. Bait-lamina-tests. Pedobiologia 34, 89–101. doi: 10.1111/mec.12579
Veen, P., Jefferson, R., De Smidt, J., and Van der Straaten, J. (2009). Grasslands in Europe: of High Nature Value. Leiden: BRILL.
Vogt, J., Klaus, V. H., Both, S., Fürstenau, C., Gockel, S., Gossner, M. M., et al. (2019). Eleven years’ data of grassland management in Germany. Biodivers. Data J. 7:e36387. doi: 10.3897/BDJ.7.e36387
Vorobeichik, E. L., and Bergman, I. E. (2021). Bait-lamina test for assessment of polluted soils: rough vs. Precise scales. Ecol. Indic. 122:107277. doi: 10.1016/j.ecolind.2020.107277
Wahyuningsih, R., Marchand, L., and Caliman, J. P. (2019). Impact of inorganic fertilizer to soil biological activity in an oil palm plantation. IOP Conf. Ser. Earth Environ. Sci. 336, 1–8.
Wolters, V., and Joergensen, R. G. (1991). Microbial carbon turnover in beech forest soils at different stages of acidification. Soil Biol. Biochem. 23, 897–902. doi: 10.1016/0038-0717(91)90103-q
Yeates, G. M. (1999). Effects of plants on nematode community structure. Annu. Rev. Phytopathol. 37, 127–149. doi: 10.1146/annurev.phyto.37.1.127
Zaitsev, A. S., Berg, M. P., and van Straalen, N. M. (2013). Landscape geological age explains large scale spatial trends in oribatid mite diversity. Landsc. Ecol. 28, 285–296. doi: 10.1007/s10980-012-9834-0
Zaitsev, A. S., Wolters, V., Waldhardt, R., and Dauber, J. (2006). Long-term succession of oribatid mites after conversion of croplands to grasslands. Appl. Soil Ecol. 34, 230–239. doi: 10.1016/j.apsoil.2006.01.005
Keywords: bait-lamina, ecosystem function, land-use intensity, nitrogen input, permanent grassland, Acari (mites), Collembola (springtails), Nematoda
Citation: Birkhofer K, Baulechner D, Diekötter T, Zaitsev A and Wolters V (2022) Fertilization Rapidly Alters the Feeding Activity of Grassland Soil Mesofauna Independent of Management History. Front. Ecol. Evol. 10:864470. doi: 10.3389/fevo.2022.864470
Received: 28 January 2022; Accepted: 05 May 2022;
Published: 23 May 2022.
Edited by:
Christopher Williams, Liverpool John Moores University, United KingdomReviewed by:
Katalin Szlavecz, Johns Hopkins University, United StatesAndreea Nita, University of Bucharest, Romania
Copyright © 2022 Birkhofer, Baulechner, Diekötter, Zaitsev and Wolters. This is an open-access article distributed under the terms of the Creative Commons Attribution License (CC BY). The use, distribution or reproduction in other forums is permitted, provided the original author(s) and the copyright owner(s) are credited and that the original publication in this journal is cited, in accordance with accepted academic practice. No use, distribution or reproduction is permitted which does not comply with these terms.
*Correspondence: Klaus Birkhofer, S2xhdXMuQmlya2hvZmVyQGItdHUuZGU=; Volkmar Wolters, dm9sa21hci53b2x0ZXJzQGFsbHpvb2wuYmlvLnVuaS1naWVzc2VuLmRl