- 1Programa de Pós-Graduação em Biodiversidade e Conservação, Universidade Federal do Pará, Altamira, Brazil
- 2Laboratório de Ecologia, Departamento de Meio Ambiente e Desenvolvimento, Universidade Federal do Amapá, Macapá, Brazil
- 3Programa de Pós-Graduação em Biodiversidade e Meio Ambiente, Universidade Federal da Grande Dourados, Dourados, Brazil
- 4School of Science, Engineering and the Environment, University of Salford, Salford, United Kingdom
Over a century after Wallace’s proposition of the riverine barrier hypothesis, the role of rivers in the diversification of species remains a matter of interest in Amazon biogeography. Amazonian rivers, in particular those large and fast flowing rivers, are widely recognized to act as barriers to the dispersal of some organisms. However, the extent to which primate species changes across interfluves (β-diversity) in response to river features remains to be explicitly tested. In this study, we examine how river characteristics affect the variation in taxonomic and phylogenetic β-diversity to elucidate the ecological processes behind the differences in primate assemblages between neighboring interfluves in the Brazilian Amazonia. We compiled International Union for Conservation of Nature (IUCN) distribution maps of 80 river-bounded primate species in 10 interfluves separated by major rivers throughout the Brazilian Amazonia. We assessed both the taxonomic (disregarding phylogenetic relationships between species) and phylogenetic β-diversities. We applied multiple linear models to evaluate whether annual discharge, sinuosity, and reflectance (as a proxy for amount of sediments) in each river or river section that separates neighboring interfluves make rivers effective barriers to primates. We found that mean discharge has a positive effect, while both sinuosity and amount of sediments have negative effects on primate β-diversity. These variables have significant effects on total taxonomic and phylogenetic β-diversity between neighboring interfluves, and their species turnover components. River features, however, have no effect on species richness differences. Genera are capable of traversing almost all interfluves, but species are replaced by others in opposite interfluves. Discharge affected both small- and large-sized primate total β-diversity, but sinuosity only affected large-sized primate assemblages in neighboring interfluves. Our results indicate that although Amazonian rivers act as barriers for many primate species, this barrier effect seems limited to the short/medium time scales, as primate lineages are able to cross them over long-time scales.
Introduction
Vicariant events such as the emergence of large rivers or uplift of mountain chains can favor allopatric speciation (Hoorn et al., 2010; Dal Vechio et al., 2019; Mendez-Camacho et al., 2021). In particular, large Amazonian rivers have been considered the most important engines of speciation by vicariance in this biome. Wallace (1852) was the first to notice that large Amazonian rivers delimit the distribution of species and in particular of primates, thus acting as barriers to dispersal in an otherwise featureless landscape. More recently, several authors refined this idea, which became known as the riverine barrier hypothesis (Patton et al., 1994; Gascon et al., 2000). The main prediction of this hypothesis is that congeneric species are separated by large rivers and their evolution was a result of the formation of said river which severed the original population in two which with time, speciate by vicariance (Patton et al., 1994). The riverine barrier hypothesis, which has been extensively tested in the last decades, is currently accepted as an important mechanism of speciation in Amazonia. It was confirmed in several taxonomic groups, including birds, mammals, and some reptiles (Ayres and Clutton-Brock, 1992; Peres et al., 1996; Vallinoto et al., 2006; Naka, 2011; Ribas et al., 2012; Boubli et al., 2015; Lynch Alfaro et al., 2015; Dal Vechio et al., 2019; Fordham et al., 2020). Although the diversification of Amazonian primates has been largely attributed to allopatric speciation by vicariance produced by rivers (Hershkovitz, 1977; Peres et al., 1996; van Roosmalen et al., 2002; Vallinoto et al., 2006; Boubli et al., 2015; Lynch Alfaro et al., 2015), some studies have shown that rivers historically considered as geographic barriers do not isolate primate populations living in their opposite banks (Craul et al., 2008; Link et al., 2015). In addition, little attention is taken on the physical and chemical characteristics of rivers that do act as barriers promoting primate diversification (e.g., Ayres and Clutton-Brock, 1992; Fordham et al., 2020).
The Amazon basin is a transcontinental drainage that holds some of the largest and highest-discharge rivers in the world (Latrubesse et al., 2005; Wohl, 2007). Amazonian rivers are quite distinct and dynamic, showing distinct features, namely river size (i.e., discharge and width), sinuosity, and sediment load (Ayres and Clutton-Brock, 1992; Latrubesse et al., 2005, 2010; Boubli et al., 2015), which can influence species dispersal chances. However, while river size is frequently evaluated as a driver for primate diversification (Ayres and Clutton-Brock, 1992; Fordham et al., 2020), the effects of other variables such as sinuosity and amount of sediments remain elusive. Consequently, additional factors responsible for the differential filtering of species by rivers are still poorly known. For instance, the continuous accumulation of sediments in some river stretches over time can create islands, that even temporarily, act as stepping stones, narrowing river width and ultimately facilitating animal crossing between riverbanks, mainly by those species with lesser swimming abilities (Ayres and Clutton-Brock, 1992; Peres et al., 1996; Sillero et al., 2018). Riverbank erosion can also promote sediment transport between riverbanks causing changes in river form and width, and in the location of islands (Hooke, 2003; Peixoto et al., 2009; Constantine et al., 2014). Sinuosity grows rapidly in sediment-rich rivers, making them more susceptible to such changes (Constantine et al., 2014; Ahmed et al., 2019). Sinuosity may also facilitate the dispersal of individuals between riverbanks given that meander cutoffs can physically transfer masses of land between opposite riverbanks, including their associated fauna and flora (Ayres and Clutton-Brock, 1992; Gascon et al., 2000). Recent accumulating evidences on the formation of paleo-channels throughout Amazonia indicate changes in direction and form of many rivers over time (Cremon et al., 2016; Ruokolainen et al., 2019; Rossetti et al., 2021). All these aforementioned processes can increase opportunities for passive lateral dispersal of individuals between riverbanks (Rabelo et al., 2014; Lynch Alfaro et al., 2015).
Several analytic tools that were recently developed can be used to investigate how large rivers can promote changes in β-diversity on a macrogeographic scale. One of these tools is the partitioning of β-diversity in two components: turnover and nestedness (Baselga, 2010; Carvalho et al., 2012). Turnover represents the replacement of species between communities, while nestedness represents the loss or gain of species between communities (Baselga, 2010; Carvalho et al., 2012). β-diversity partitioning allows the incorporation of phylogenetic trees, which improves our interpretation of the effects of different drivers on biodiversity (Graham and Fine, 2008; Cardoso et al., 2014). Taken together, those approaches that take into account more than one dimension of diversity allow us to generate more robust conclusions about how different biogeographic issues (e.g., barriers to dispersal) act on different processes (e.g., speciation, changes in species composition) or patterns (e.g., species distribution) (Graham and Fine, 2008; Miller et al., 2018). For example, disentangling the nestedness and turnover components of the β-diversity allows us to verify if the barriers completely preclude movements of some lineages, or if they are somewhat permeable in the geologic time scale. The comparison of the taxonomic and phylogenetic β-diversity may also give insights about the timescale in which the barriers preclude dispersal. For instance, while taxonomic β-diversity can inform about changes occurring at short timescales (changes in species occupying different riverbanks), phylogenetic β-diversity can provide information at long timescales (changes at higher taxonomic levels, with greater barriers separating more distant lineages within the genera, and smaller barriers separating closely related species). Barriers that impede movement at longer timescales cause higher effects on the phylogenetic β-diversity, thus generating more contrasting assemblages. Such analytic approaches are still uncommon in biogeographic analysis in Amazonia (e.g., Fluck et al., 2020; Carvalho et al., 2021). Most studies so far have focused only on the taxonomic component of β-diversity, without considering the phylogenetic component or the partitioning of β-diversity (e.g., Ayres and Clutton-Brock, 1992; Dambros et al., 2017, 2020; Fordham et al., 2020).
In this study, we investigated the role of large Amazonian rivers as effective physical barriers to primates, which have limited dispersal abilities (Schloss et al., 2012; Sales et al., 2019). Specifically, we investigated the characteristics of rivers (i.e., discharge, load of sediments, and sinuosity) as drivers of isolation for primate communities distributed among 10 major Amazonian interfluves. To do that, we estimated both taxonomic and phylogenetic β-diversity and their partitioning, turnover and nestedness, between pairs of neighboring interfluves, relating them with the characteristics of the rivers that separate these interfluves. If the hypothesis of rivers as barriers for primates is correct, we expect less similarity (great taxonomic and phylogenetic β-diversity) across riverbanks (Ayres and Clutton-Brock, 1992). Thus, we expect to find a positive relationship between the dissimilarity of primate communities and river discharge (Ayres and Clutton-Brock, 1992; Fordham et al., 2020), and negative relationships with sediment load and river sinuosity (Ayres and Clutton-Brock, 1992; Gascon et al., 2000). Furthermore, considering the phylogenetic dimension, we expect that rivers that act as strong geographic barriers, preclude the crossing of some lineages (i.e., genera), leading to a stronger effect of lineage loss (nestedness) than lineage sharing (turnover). Additionally, assuming the lower chances and lower frequency of crossing in rivers that act as stronger geographic barriers, we expect that lineages on opposite banks of larger rivers will diverge more, and consequently, that the effect of large rivers as geographic barriers will be stronger for phylogenetic β-diversity than for taxonomic β-diversity. Finally, considering the swimming ability of large-sized primates, we expect a lower effect of rivers on them, implying they are less limited by rivers and, consequently, more widely distributed (i.e., occupying more interfluves) than small- and mid-sized species (Ayres and Clutton-Brock, 1992).
Materials and Methods
Study Area
The Amazonia is divided by several large rivers that delimit large interfluvial areas (i.e., landmasses between major rivers) which are often considered as areas of endemism for birds and primates (Cracraft, 1985; da Silva and Oren, 1996; da Silva et al., 2005; Naka, 2011; Ribas et al., 2012). The composition of species within these interfluves have provided interesting opportunities to study spatial patterns of species distribution. Here, we used interfluves to investigate the effects of large rivers on the patterns of primate β-diversity in Brazilian Amazonia. We restricted our study to the Brazilian Amazonia because both its primate fauna and river characteristics are well known and freely available (Ayres and Clutton-Brock, 1992; Paglia et al., 2012). Although limited in geographical scope, the Brazilian Amazonia covers over 60% of the entire Amazon basin and all interfluves but Napo have most of their extensions within Brazil (Juen and De Marco, 2012). Additionally, our study area encompasses the mid and lower parts of all major rivers that form this basin, presumably the region where we would expect to see the greatest effect of rivers as barriers for primate dispersal. In contrast, their headwaters are known to have a limited role in isolating species (Ayres and Clutton-Brock, 1992; Peres et al., 1996; Fordham et al., 2020).
We selected interfluves based on previous studies with terrestrial vertebrates, which identified 10 large biogeographic regions separated by major rivers in Amazonia (da Silva et al., 2005; Naka, 2011; Borges and Da Silva, 2012; Ribas et al., 2012; Silva et al., 2019), as follows (Figure 1): Guiana (between the Amazon, lower Negro, and Branco rivers), Pantepui-Duida (between Branco and mid and upper Negro rivers), Imeri (between Negro and Uaupés rivers), Napo (between Uaupés, Negro, Uneiuxi, Pucabi, Juami, Içá, Japurá, and Napo rivers), Jaú (between Napo/Solimões, Negro, Uneiuxi, Pucabi, Juami, and Içá rivers), Inambari (between Napo/Solimões, Amazonas, and Madeira rivers), Rondônia (between Madeira and Tapajós rivers), Tapajós (between Tapajós and Xingu rivers), Xingu (between Xingu and Tocantins rivers), and Belém (between Tocantins and Amazon rivers). Our sample units were the pairs of neighboring interfluves distributed along the study area (N = 17, Table 1).
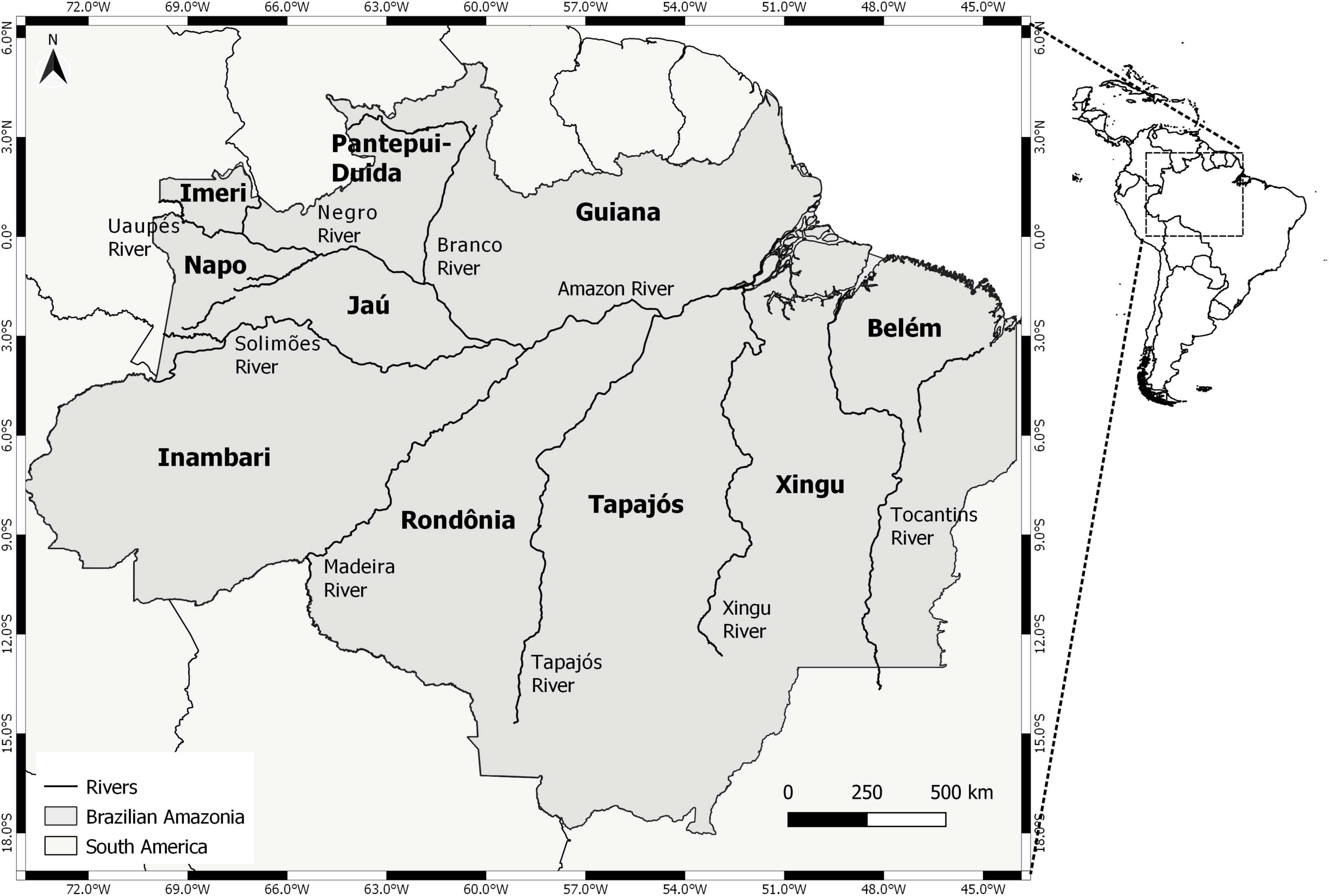
Figure 1. Major interfluves of the Brazilian Amazonia, recognized as different biogeographic provinces.
Data Collection
We included only primate species occurring in the Brazilian Amazonia. Their taxonomy followed Paglia et al. (2012), which was based on previous taxonomic studies of Neotropical primates (Rylands et al., 2000; Rylands and Mittermeier, 2009). However, we also consider recent species descriptions and taxonomic changes (Ferrari et al., 2010, 2014; Boubli et al., 2012; Gualda-Barros et al., 2012; Lynch Alfaro et al., 2012; Dalponte et al., 2014; Byrne et al., 2016; Rylands et al., 2016; Silva et al., 2018; Serrano-Villavicencio et al., 2019). Our database included 80 (65%) out of the 124 primates with confirmed occurrence in the Amazonia (Primate Specialist Group website, 2021) and available in the updated International Union for Conservation of Nature’s (IUCN) red list1 (Supplementary Table 1). Although distribution maps such as those obtained by IUCN are not reliable to infer about the presence/absence of species in specific points (Herkt et al., 2017; Fluck et al., 2020), we used them only to obtain species occurrence within a given interfluve, and not to assume their occurrence in specific sites within the interfluves or to run species distribution models. Then, we are confident that their use in this study was appropriate, minimizing possible distribution errors.
Water is considered to pose high resistance to primate dispersal (Rabelo et al., 2019). However, although uncommon, primates occasionally swim to cross rivers (Chaves and Stoner, 2010; Gonzalez-Socoloske and Snarr, 2010; Nunes, 2014). Body size should be a key characteristic influencing the ability of primates to disperse across rivers (Ayres and Clutton-Brock, 1992). In fact, all the available reports come from large-sized atelids, and we are not aware of any report on small- or mid-sized species swimming in the wild. We thus considered primate dispersal ability across rivers as limited and species-specific (Ayres and Clutton-Brock, 1992; Schloss et al., 2012; Rabelo et al., 2019). We thus compiled the average body mass (kg) of the species from Smith and Jungers (1997), classifying them according to their body masses as small- (<1.5 kg), mid- (1.5–4.99 kg), or large-sized species (≥5.0 kg).
River Characteristics
We estimated mean annual river discharge by averaging the values recorded in all measuring stations for a given river obtained from the Global Runoff Data Centre (GRDC, Germany2) (Fordham et al., 2020), which provides in situ annual river discharge times series data for several rivers worldwide. Since the GRDC does not have data for all sections of the Amazon River, the discharge of missing sections were estimated through a simple linear regression between the discharge and the distance from the river beginning (bifurcation between Solimões and Negro rivers) and its estuary (Fordham et al., 2020). Relatively small rivers such as Uneiuxi, Pucabi, and Juami (that separate Napo and Jaú interfluves) have no annual discharge data available were arbitrarily set to 1000 m3/s.
We established a river sinuosity index (SI) by measuring the straight distance between river headwaters and mouths (D1), and the total length of the rivers (D2) using the length function in the QGIS 3.16 software (QGIS Development Team, 2022). Then, we calculated the SI through the following formula: SI = 1 − (D1/D2). The SI values ranged from 0.064 to 0.306, with higher values representing rivers that are more sinuous. We obtained the sediment load of the rivers based on the empirical correlation between the red light reflectance and the sediment load in sampling stations, provided by Fassoni-Andrade and Paiva (2019) as a high resolution raster file with reflectance values in 250-m pixels. Then, we averaged the sediment load of five points along each river (or river section), following Laranjeiras et al. (2021). River characteristics are described in Supplementary Table 2.
Analysis
Based on the species distribution overlaid on a map of the major Amazonian rivers, we built a matrix of primate occurrence (presence/absence) in each interfluve (Supplementary Table 1). Since our goal was to evaluate the effect of large rivers on the pattern of β-diversity among Amazonian primates, we disregarded those species that occurred only in the central portion of the interfluve, i.e., those species whose distribution was not limited by any river. Our reasoning was that factors other than rivers, such as vegetation and edaphic variation (habitat type), relief, and/or distance per se must limit these species (Emmons, 1984; Gascon et al., 2000). From the occurrence matrix, we calculated the total taxonomic β-diversity (βtotal.tax) for each pair of interfluves, and their components, turnover (i.e., replacement of one species by another – βturn.tax) and nestedness (i.e., richness difference due to gain or loss of species – βrich.tax) (Carvalho et al., 2012). We also calculated the total phylogenetic β-diversity (βtotal.phy) and its components, turnover (i.e., lineage replacement – βturn.phy) and nestedness (i.e., gain or loss of lineages – βrich.phy). To calculate phylogenetic β-diversity, we built a phylogenetic tree with all the species included in our analysis from recent phylogenies of all the genus with more than two species (Supplementary Table 3 and Supplementary Figure 1). For Pithecia, for which we could not find any phylogeny, and for those genera for which we found two or more inconsistent phylogenies, we used a conservative approach, disregarding any hierarchy for the species/clades involved in the inconsistencies. The phylogenetic relationships of the genera was based on molecular data provided by Perelman et al. (2011). For both dimensions of the β-diversity (taxonomic and phylogenetic) we used the Jaccard distances with 100 resamples. β-diversity was calculated through the function beta using the package BAT (Cardoso et al., 2021), in R 3.6.3 (R Core Team, 2020).
The β-diversity (both taxonomic and phylogenetic) and its components (βtotal.tax, βturn.tax, βrich.tax, βtotal.phy, βturn.phy, βrich.phy, βtax.small, βtax.mid, βtax.large) between neighboring interfluves were used as response variables to infer which river characteristics made them effective biogeographic barriers using multiple linear regressions. We used mean discharge, sinuosity and sediment load as predictor variables. River discharge was log-transformed for normality. We standardized all predictor variables to zero mean and unit variance. We selected the most parsimonious models based on their Akaike Information Criterion corrected for small samples (AICc). We checked model residuals for spatial autocorrelation using Moran’s I. Since our sampling units in the linear models were pairs of interfluves, we built a “distance class connectivity matrix” (Fortin and Dale, 2005) by accessing the shorter connections between pairs of interfluves, considering the number of rivers between them +1. We discarded only one model for which the residuals showed spatial autocorrelation (βtax.large). We checked the residuals of the models for normality through Shapiro–Wilk’s test. Significance level was set at 0.05.
Results
Over half of the 80 Amazonian primate species evaluated (N = 46; 58%) were restricted to only 1 of the 10 interfluves defined in this study (Table 2). Conversely, eight species (10%) were widely distributed (≥4 interfluves), with Sapajus apella occupying nine interfluves (Table 2 and Supplementary Table 1). Contrary to our expectations, only three of the eight species with wider distributions were large-sized (Alouatta seniculus, Ateles chamek, Lagothrix lagotricha, Supplementary Table 1).
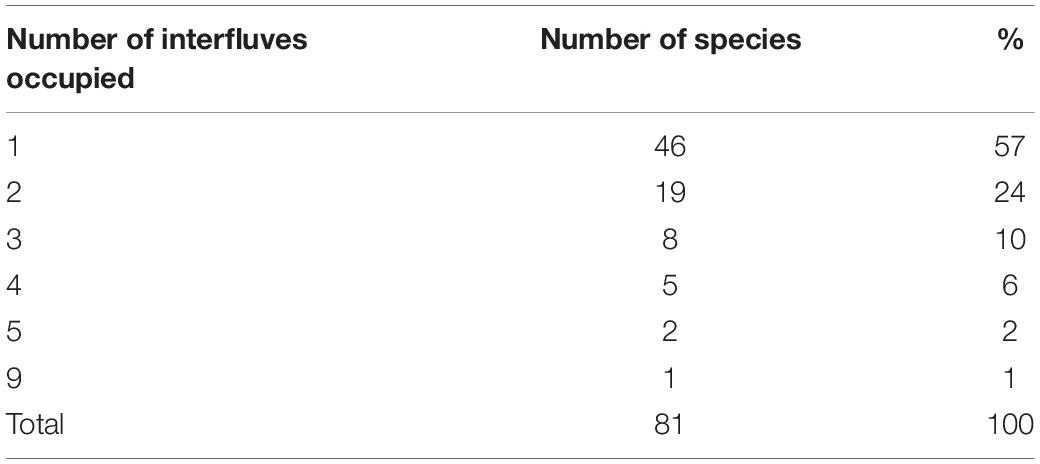
Table 2. Frequency of occurrence of primate species in the major interfluves of the Brazilian Amazonia.
The Inambari interfluve presented the greatest primate species richness in the Brazilian Amazonia (N = 26 species), followed by the Rondônia interfluve (N = 23). The other interfluves presented from 7 to 18 species (Supplementary Table 1). The average dissimilarity between interfluves in the study area was 0.821 (range: 0.333–1.000). We identified three distinct groups of interfluves sharing species among themselves. They can be roughly divided between the northern and southern (plus Imeri) interfluves, with the southern group divided in western and eastern groups (Figure 2). Napo and Jaú interfluves presented the lowest dissimilarity (0.333), while the highest dissimilarity was observed between Pantepui-Duida and all southern interfluves (Belém, Xingu, Tapajós, Rondônia, Inambari). Indeed, given the high endemism, most of the interfluve pairs presented high dissimilarity among them (>0.7, Supplementary Table 4). Rondônia (N = 13) and Inambari (N = 9) interfluves presented the highest number of endemic species, followed by Guiana (N = 7). Other interfluves presented from zero to three endemic species (Supplementary Table 1).
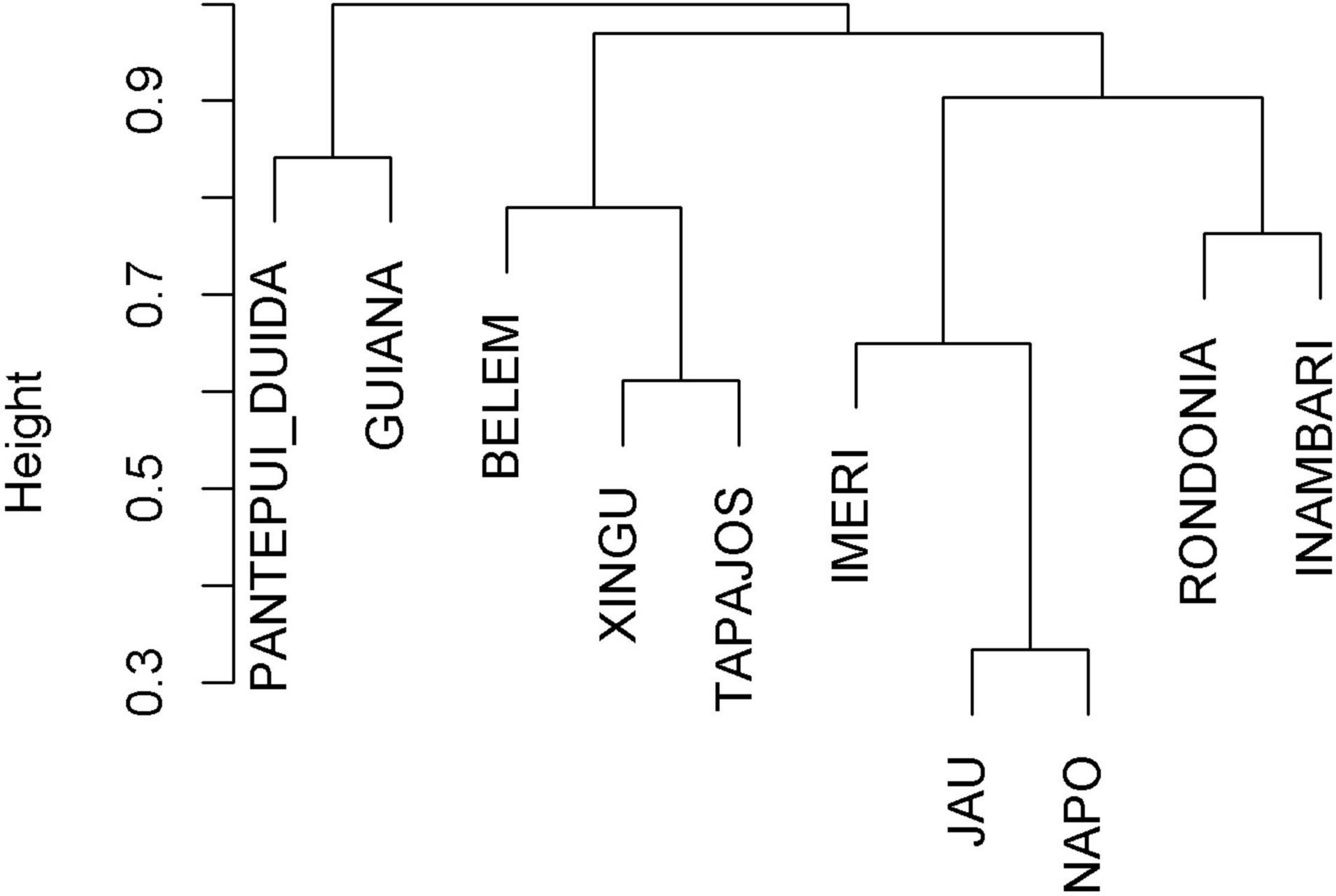
Figure 2. Cluster analysis of the major interfluves in the Brazilian Amazonia based on the primate species composition.
Primate communities were more similar in interfluves separated by sinuous rivers. Conversely, rivers with greater discharge resulted in less similar communities across their banks. The effect of the sediment load in the role of rivers as barriers was observed only for βtotal.tax and rivers with lower sediment load separated more dissimilar assemblages (Table 3).
As expected, phylogenetic β-diversity (R2 = 0.794) was better explained by river characteristics than taxonomic β-diversity (R2 = 0.762), although the difference was relatively small. Mean discharge had the strongest effect on both taxonomic and phylogenetic components of primate β-diversity in the Brazilian Amazon basin, followed by sinuosity (Table 3). Moreover, we found that river characteristics explained better the β-diversity of small-sized (R2 = 0.601) than large-sized primates (R2 = 0.542), although we did not detect any effect for mid-sized primates. Furthermore, river characteristics had an effect on the turnover component of the β-diversity, but they were not good predictors of the nestedness component of the β-diversity at all, a pattern that was consistent for both the taxonomic and phylogenetic β-diversity (Table 3).
Discussion
The diversification of Amazonian species is complex (Hoorn et al., 2010; Mendez-Camacho et al., 2021), resulting from multiple mechanisms of vicariance (e.g., Andes uplift, emergence or redirection of large rivers, changes in the vegetation caused by climate change) and non-vicariance (e.g., expansion of species distribution, habitat gradients, human-driven barrier transposition), acting independently or interactively (da Rocha and Kaefer, 2019). Our results support the role of rivers as key agents in spatially structuring Amazonian primate diversity. Confirming our hypothesis, we found significant evidence for a positive effect of mean annual discharge and a negative effect of sinuosity on primate β-diversity. While previous studies have already showed the role of rivers as barriers for Amazonian primates (mainly because of annual discharge: Ayres and Clutton-Brock, 1992; Fordham et al., 2020), our study goes beyond, showing both discharge and sinuosity as the main drivers of changes in primate β-diversity in Amazonia.
Rivers with higher annual discharge have a greater positive effect on primate composition changes between opposite riverbanks, meaning that higher discharge leads to less similar primate communities. It makes sense that discharge act more effectively to prevent primate flux across opposite riverbanks. On one hand, wide, low discharge rivers favor animals crossing using rocks (i.e., stepping stones), islands and/or fallen trees, especially during drier periods (Mamalis et al., 2018). In fact, the resistance caused by discharge to the dispersal of animals is lower during the dry season (Prevedello and Vieira, 2009; Rabelo et al., 2019). On the other hand, high discharge rivers tend to keep higher volumes of water in their main channel even during the driest periods, thus inhibiting primates and other terrestrial animals to cross between riverbanks. Other studies have used width as a measure of river size (Ayres and Clutton-Brock, 1992; Fordham et al., 2020). However, river discharge is probably stronger than river width as a feature promoting the isolation of primates in the Amazonia because width is highly variable (Fordham et al., 2020). Moreover, there are so far many dams built along several Amazonian rivers (Lees et al., 2016), which can artificially input biases on the measurement of river width and, consequently, on the influence of this variable on primate speciation.
Previous studies did not investigate (Ayres and Clutton-Brock, 1992) or find significant results on the role of sinuosity in primate diversification (Fordham et al., 2020). However, we claim that a simple comparison of rivers draining different watersheds, as tested by Fordham et al. (2020), is not appropriate to test this issue because rivers within different watersheds may show similar dynamics (Supplementary Table 5 and Supplementary Figure 2). In fact, mean sinuosity among black water (mean ± SD = 0.819 ± 0.09) and white water rivers (0.817 ± 0.07) are quite similar. For instance, river stretches within the Negro River watershed, which have predominantly black water, are as sinuous as those draining the predominantly white water rivers of the Solimões watershed (see Supplementary Table 2). Nevertheless, our results indicate that, independently of basin, sinuous rivers seem to be less effective barriers for primates than straight rivers. The main explanation for the greater permeability of sinuous rivers is the meandric dynamic. Meandering rivers can show relatively frequent cutoffs (Hooke, 2003; Constantine et al., 2014), that increase opportunities for lateral passive dispersal of individuals between opposed riverbanks (Peres et al., 1996). Additionally, recent reports of paleo-channels in Amazonia indicate they were produced by changes in the direction of some large rivers (Ruokolainen et al., 2019; Rossetti et al., 2021). These changes transferred large landmasses from one riverbank to the opposite side together with all animals and plants living there. Moreover, the dynamics of sedimentation and erosion in islands are also greater in sinuous rivers (Peixoto et al., 2009; Ahmed et al., 2019), partly contributing to their filtering effect on primates.
The effects of sediment load on the diversification of primate communities is somewhat controversial and limited to the (total) taxonomic β-diversity (Table 3). Although the general idea that sediment load can be related to the sedimentation and erosion dynamics (mainly in islands and riverbanks), thus creating stepping stones that can facilitate species crossing over rivers (Rabelo et al., 2014; Lynch Alfaro et al., 2015), this dynamic can also be related to river sinuosity (Constantine et al., 2014; Ahmed et al., 2019). However, in some cases, sediment load is stronger than river sinuosity per se (see Table 3). For instance, the high sediment load associated with the long-term dynamic of island formation can be important in the Amazonas River mouth, where some mid- and large-sized species such as Aotus azarae and Alouatta belzebul, occur in both riverbanks. The Amazon River mouth is characterized by multiple, non-shifting channels with high sediment load, subjected to high and complex sedimentation and erosion dynamics in its islands and banks, thus promoting permeability (Ayres and Clutton-Brock, 1992; Gensac et al., 2016). Although there are no evidence that the Madeira River, which have the second higher sediment load among the large Amazonian rivers, act as an effective barrier for many taxa (Santorelli et al., 2018), it split out several primate species occupying the Inambari and Rondônia interfluves, including Callibella humilis, Cebuella pygmaea, Mico rondoni, Saguinus labiatus, Sapajus apella, Cebus unicolor, and several species of Plecturocebus (P. baptista, P. bernhardi, P. caligatus, P. cinerascens, P. dubius).
Confirming our prediction, rivers characteristics affected more the phylogenetic β-diversity than the taxonomic β-diversity, indicating that the taxa separated by the bulkier and less sinuous rivers are more phylogenetically distant than taxa separated by small and more sinuous rivers. The small difference in the explanatory power of the models can be explained by a possible mismatch in the temporal scale. Rivers act as barriers for primates species or even for divergent populations of some species (e.g., Vallinoto et al., 2006) at shorter temporal scales, while a considerable part of phylogenetic β-diversity occurs at longer temporal scales (i.e., changes at higher taxonomic levels, with greater barriers separating more distant lineages within the genera, and smaller barriers separating closely related species).
The temporal scale can also explain why we do not observe differences in the nestedness component of the β-diversity. In fact, many primate genera are widely distributed across several Amazonian interfluves, indicating that rivers are rarely an insurmountable barrier for some lineages. Although the low, wide river stretches located next to river mouths can be considered as barriers to primate dispersal (but see the case of the Amazon River mouth discussed above), it is unlikely that river headwaters (i.e., high, narrow stretches) fulfill the same role. For instance, some primates occur in both riverbanks, and there is a low genetic divergence between populations living in riverheads, as compared to those located near river mouths (Peres et al., 1996; Vallinoto et al., 2006). Stretches considerably narrow and shallow can allow river crossings, thus increasing dispersal chances. This can be the case of Mico emiliae, which appears to have crossed the headwaters of the Iriri River, thus allowing the extension of its distribution as far to the western bank of the Xingu River (Andrade et al., 2018). Considering that most Platyrrhini genera had their origin estimated to at least 6 million years ago (Schneider et al., 2001; Lynch Alfaro et al., 2012; Byrne et al., 2018), there was enough time for them to cross through river waterheads, thus expanding across several Amazonian interfluves in some cases.
Considering that the species of primates are not homogeneously distributed across the Amazonia, the role of rivers as barriers has implications for the conservation of species. The Amazonia, which has the highest primate diversity in the world (Rylands et al., 2000; Rylands and Mittermeier, 2009), also suffers a spatially biased pressure from deforestation. For instance, while the Belém, Xingu, Tapajós, and Rondônia are located at the arc of deforestation, where forest clearance advances at an accelerated pace, other interfluves, such as Guiana, Imeri and Napo are still kept relatively well preserved (da Silva et al., 2005; Fearnside, 2005; Soares-Filho et al., 2006; Broadbent et al., 2008; Alves, 2010; Kalamandeen et al., 2018). Considering the high endemism level in some interfluves (e.g., Rondônia), the loss of forests cannot be compensated by forest maintenance in other interfluves, under penalty of loss of unique endemics. Even in areas with low endemism there is a real risk of loss of the few endemic species present in some highly deforested interfluves, such as Belém and Xingu.
Our results point out to the existence of at least three distinct primate groups in the Brazilian Amazonia, based on the similarity among the communities occurring in each interfluve. The dissimilarity between the northern and southern groups can be explained by the clear geomorphological differences between the Guiana (nutrient-poor sandy soils drained by black water) and Brazilian (rich-nutrient alluvial soils drained by white and clear waters) shields, divided by the Amazon River. On the other hand, the dissimilarity between the western and eastern groups is expected due to marked differences in past geological and climatic events in the Old Amazon basin (da Silva and Oren, 1996; Hoorn et al., 2010; Wesselingh et al., 2010; Mendez-Camacho et al., 2021), coupled with present differences in ecological conditions where the west (west of the Madeira river, is characterized by high rainfall rain, high habitat diversity, eutrophic soils from the Andes runoff (Archer, 2005; Ferrari, 2005; Hoorn et al., 2010; Wesselingh et al., 2010; Juen and De Marco, 2012). In summary, the reconfiguration of the Amazonian landscape in the Neogene, associated with spatial heterogeneity in current ecological conditions across the Amazonian basin may explain the observed differences across these three regions (Ribas et al., 2012; Lynch Alfaro et al., 2015; Lima et al., 2017; Byrne et al., 2018).
Although there is no evidence to accept the generality of the riverine barrier hypothesis to other taxa (Gascon et al., 2000; Santorelli et al., 2018), rivers are important biogeographic barriers for species with limited dispersal ability, such as primates (Ayres and Clutton-Brock, 1992; Boubli et al., 2015; Lynch Alfaro et al., 2015; Santorelli et al., 2018; Fordham et al., 2020; this study). The patterns of β-diversity described here are partly driven by the ability of primates to actively or passively cross rivers. While some primates have limited mobility across rivers, the large atelids, for example, are fully able to cross rivers swimming (Chaves and Stoner, 2010; Gonzalez-Socoloske and Snarr, 2010; Benchimol and Venticinque, 2014; Nunes, 2014). This may partly explain why rivers affected the large-sized primates less than small-sized ones. Rivers can limit primate dispersal, favoring speciation over longer timescales (Ayres and Clutton-Brock, 1992), with several accumulating evidences of rivers acting as effective barriers that promote population isolation and diversification in primates (Peres et al., 1996; Vallinoto et al., 2006; Boubli et al., 2015; Lynch Alfaro et al., 2015). In fact, populations separated by large rivers tend to be divergent. For instance, previous studies showed genetically divergent populations of Saguinus niger occurring in opposite banks of Tocantins River, between Belém and Xingu interfluves (Vallinoto et al., 2006), and Saguinus fuscicollis occurring in opposite banks of Juruá River (Peres et al., 1996). The isolation promoted by rivers favors the emergence of clades divergent to the original, ultimately favoring speciation. More genetic studies are necessary to disclose the role of rivers as effective barriers for primates and other animals (Vallinoto et al., 2006; Boubli et al., 2015; Lynch Alfaro et al., 2015; Dal Vechio et al., 2019; Naka and Pil, 2020).
Data Availability Statement
The original contributions presented in the study are included in the article/Supplementary Material, further inquiries can be directed to the corresponding author.
Ethics Statement
Ethical review and approval was not required for the animal study because no animal was captured or manipulated.
Author Contributions
ÍM and RH designed the study, collected the data, and wrote the manuscript draft. ÍM, RH, and WC performed the analyses. All authors reviewed, edited, and approved the final version of the manuscript.
Funding
This study was supported by a scholarship from the Coordenação de Aperfeiçoamento de Pessoal de Nível Superior (PNPD/CAPES, Brazil) granted through the Programa de Pós-Graduação em Biodiversidade e Conservação (PPGBC-UFPA) to ÍM. JPB was supported by a UKRI Grant NERC (NE/T000341/1).
Conflict of Interest
The authors declare that the research was conducted in the absence of any commercial or financial relationships that could be construed as a potential conflict of interest.
Publisher’s Note
All claims expressed in this article are solely those of the authors and do not necessarily represent those of their affiliated organizations, or those of the publisher, the editors and the reviewers. Any product that may be evaluated in this article, or claim that may be made by its manufacturer, is not guaranteed or endorsed by the publisher.
Acknowledgments
ÍM is grateful to Leandro Sousa and Eder Mileno for their valuable advice on QGIS tools and map design. We also thank Rafael Rabelo and Ubirajara Oliveira for the insightful comments and criticisms that greatly improved our article.
Supplementary Material
The Supplementary Material for this article can be found online at: https://www.frontiersin.org/articles/10.3389/fevo.2022.857920/full#supplementary-material
Footnotes
References
Ahmed, J., Constantine, J. A., and Dunne, T. (2019). The role of sediment supply in the adjustment of channel sinuosity across the Amazon Basin. Geology 47, 807–810. doi: 10.1130/G46319.1
Alves, D. S. (2010). Space-time dynamics of deforestation in Brazilian Amazônia. Int. J. Remote Sens. 23, 2903–2908. doi: 10.1080/01431160110096791
Andrade, R. P., Mourthe, I., Saccardi, V., and Hernández-Ruz, E. J. (2018). Eastern extension of the geographic range of Mico emiliae. Acta Amaz. 48, 257–260. doi: 10.1590/1809-4392201704392
Archer, A. W. (2005). Review of amazonian depositional systems. Int. Assoc. Sedimentol. Spec. Publ. 35, 17–39. doi: 10.1002/9781444304350.ch2
Ayres, J. M., and Clutton-Brock, T. H. (1992). River boundaries and species range size in Amazonian primates. Am. Nat. 140, 531–537. doi: 10.1086/285427
Baselga, A. (2010). Partitioning the turnover and nestedness components of beta diversity. Glob. Ecol. Biogeogr. 19, 134–143. doi: 10.1111/j.1466-8238.2009.00490.x
Benchimol, M., and Venticinque, E. M. (2014). Responses of primates to landscape change in Amazonian land-bridge islands-a multi-scale Analysis. Biotropica 46, 470–478. doi: 10.1111/btp.12122
Borges, S. H., and Da Silva, J. M. C. (2012). A new area of endemism for Amazonian birds in the Rio Negro basin. Wilson J. Ornithol. 124, 15–23. doi: 10.1676/07-103.1
Boubli, J. P., Ribas, C., Lynch Alfaro, J. W., Alfaro, M. E., da Silva, M. N., Pinho, G. M., et al. (2015). Spatial and temporal patterns of diversification on the Amazon: a test of the riverine hypothesis for all diurnal primates of Rio Negro and Rio Branco in Brazil. Mol. Phylogenet. Evol. 82, 400–412. doi: 10.1016/j.ympev.2014.09.005
Boubli, J. P., Rylands, A. B., Farias, I. P., Alfaro, M. E., and Alfaro, J. L. (2012). Cebus phylogenetic relationships: a preliminary reassessment of the diversity of the untufted capuchin monkeys. Am. J. Primatol. 74, 381–393. doi: 10.1002/ajp.21998
Broadbent, E. N., Asner, G. P., Keller, M., Knapp, D. E., Oliveira, P. J. C., and Silva, J. N. (2008). Forest fragmentation and edge effects from deforestation and selective logging in the Brazilian Amazon. Biol. Conserv. 141, 1745–1757. doi: 10.1016/j.biocon.2008.04.024
Byrne, H., Lynch Alfaro, J. W., Sampaio, I., Farias, I., Schneider, H., Hrbek, T., et al. (2018). Titi monkey biogeography: parallel pleistocene spread by Plecturocebus and Cheracebus into a post-Pebas Western Amazon. Zool. Scr. 47, 499–517. doi: 10.1111/zsc.12300
Byrne, H., Rylands, A. B., Carneiro, J. C., Alfaro, J. W., Bertuol, F., da Silva, M. N., et al. (2016). Phylogenetic relationships of the New World titi monkeys (Callicebus): first appraisal of taxonomy based on molecular evidence. Front. Zool. 13:10. doi: 10.1186/s12983-016-0142-4
Cardoso, P., Mammola, T., Rigal, F., and Carvalho, J. (2021). BAT: Biodiversity Assessment Tools. R package version 2.5.0. Available online at: https://CRAN.R-project.org/package=BAT (accessed February 25, 2021).
Cardoso, P., Rigal, F., Borges, P. A. V., Carvalho, J. C., and Faith, D. (2014). A new frontier in biodiversity inventory: a proposal for estimators of phylogenetic and functional diversity. Methods Ecol. Evol. 5, 452–461. doi: 10.1111/2041-210x.12173
Carvalho, J. C., Cardoso, P., and Gomes, P. (2012). Determining the relative roles of species replacement and species richness differences in generating beta-diversity patterns. Glob. Ecol. Biogeogr. 21, 760–771. doi: 10.1111/j.1466-8238.2011.00694.x
Carvalho, W. D., Mustin, K., Farneda, F. Z., de Castro, I. J., Hilário, R. R., Martins, A. C. M., et al. (2021). Taxonomic, functional and phylogenetic bat diversity decrease from more to less complex natural habitats in the Amazon. Oecologia 197, 223–239. doi: 10.1007/s00442-021-05009-3
Chaves, ÓM., and Stoner, K. (2010). River crossings by Ateles geoffroyi and Alouatta pigra in southern Mexico: a preliminary report. Rev. Chil. Hist. Nat. 83, 435–442.
Constantine, J. A., Dunne, T., Ahmed, J., Legleiter, C., and Lazarus, E. D. (2014). Sediment supply as a driver of river meandering and floodplain evolution in the Amazon Basin. Nat. Geosci. 7, 899–903. doi: 10.1038/ngeo2282
Cracraft, J. (1985). Historical biogeography and patterns of differentiation within the South American avifauna: areas of endemism. Ornithol. Monogr. 36, 49–84. doi: 10.2307/40168278
Craul, M., Radespiel, U., Rasolofoson, D. W., Rakotondratsimba, G., Rakotonirainy, O., Rasoloharijaona, S., et al. (2008). Large rivers do not always act as species barriers for Lepilemur sp. Primates 49, 211–218. doi: 10.1007/s10329-008-0092-3
Cremon, ÉH., Rossetti, D. D. F., Sawakuchi, A. D. O., and Cohen, M. C. L. (2016). The role of tectonics and climate in the late Quaternary evolution of a northern Amazonian River. Geomorphology 271, 22–39. doi: 10.1016/j.geomorph.2016.07.030
da Rocha, D. G., and Kaefer, I. L. (2019). What has become of the refugia hypothesis to explain biological diversity in Amazonia? Ecol. Evol. 9, 4302–4309. doi: 10.1002/ece3.5051
da Silva, J. M. C., and Oren, D. C. (1996). Application of parsimony analysis of endemicity in Amazonian biogeography: an example with primates. Biol. J. Linn. Soc. 59, 427–437. doi: 10.1111/j.1095-8312.1996.tb01475.x
da Silva, J. M. C., Rylands, A. B., and da Fonseca, G. A. B. (2005). The fate of the amazonian areas of endemism. Conserv. Biol. 19, 689–694. doi: 10.1111/j.1523-1739.2005.00705.x
Dal Vechio, F., Prates, I., Grazziotin, F. G., Zaher, H., Graboski, R., Rodrigues, M. T., et al. (2019). Rain forest shifts through time and riverine barriers shaped the diversification of South American terrestrial pit vipers (Bothrops jararacussu species group). J. Biogeogr. 47, 516–526. doi: 10.1111/jbi.13736
Dalponte, J. C., Silva, F. E., and Souza e Silva, J. (2014). New species of titi monkey, genus Callicebus Thomas, 1903 (Primates, Pitheciidae), from southern Amazonia, Brazil. Pap. Avulsos. Zool. 54, 457–472. doi: 10.1590/0031-1049
Dambros, C., Zuquim, G., Moulatlet, G. M., Costa, F. R. C., Tuomisto, H., Ribas, C. C., et al. (2020). The role of environmental filtering, geographic distance and dispersal barriers in shaping the turnover of plant and animal species in Amazonia. Biodivers. Conserv. 29, 3609–3634. doi: 10.1007/s10531-020-02040-3
Dambros, C. S., Morais, J. W., Azevedo, R. A., and Gotelli, N. J. (2017). Isolation by distance, not rivers, control the distribution of termite species in the Amazonian rain forest. Ecography 40, 1242–1250. doi: 10.1111/ecog.02663
Emmons, L. H. (1984). Geographic variation in densities and diversities on non flying mammals in Amazonia. Biotropica 16, 210–222. doi: 10.2307/2388054
Fassoni-Andrade, A. C., and Paiva, R. C. D. (2019). Mapping spatial-temporal sediment dynamics of river-floodplains in the Amazon. Remote Sens. Environ. 221, 94–107. doi: 10.1016/j.rse.2018.10.038
Fearnside, P. M. (2005). Deforestation in Brazilian Amazonia: history, fates, and consequences. Conserv. Biol. 19, 680–688. doi: 10.1111/j.1523-1739.2005.00697.x
Ferrari, S. F. (2005). “Biogeography of amazonian primates,” in A Primatologia no Brasil, eds S. L. Mendes and A. G. Chiarello (Vitória: IPEMA), 101–122.
Ferrari, S. F., Guedes, P. G., Figueiredo-Ready, W. M. B., and Barnett, A. A. (2014). Reconsidering the taxonomy of the Black-Faced Uacaris, Cacajao melanocephalus group (Mammalia: Pitheciidae), from the northern Amazon Basin. Zootaxa 3866, 353–370. doi: 10.11646/zootaxa.3866.3.3
Ferrari, S. F., Sena, L., Schneider, M. P. C., and Júnior, J. S. S. (2010). Rondon’s Marmoset, Mico rondoni sp. n., from Southwestern Brazilian Amazonia. Int. J. Primatol. 31, 693–714. doi: 10.1007/s10764-010-9422-6
Fluck, I. E., Cáceres, N., Hendges, C. D., Brum, M. D. N., and Dambros, C. S. (2020). Climate and geographic distance are more influential than rivers on the beta diversity of passerine birds in Amazonia. Ecography 43, 860–868. doi: 10.1111/ecog.04753
Fordham, G., Shanee, S., and Peck, M. (2020). Effect of river size on Amazonian primate community structure: a biogeographic analysis using updated taxonomic assessments. Am. J. Primatol. 82, e23136. doi: 10.1002/ajp.23136
Fortin, M.-J., and Dale, M. (2005). Spatial Analysis – A Guide for Ecologists. Cambridge, MA: Cambridge University Press.
Gascon, C., Malcolm, J. R., Patton, J. L., da Silva, M. N., Bogart, J. P., Lougheed, S. C., et al. (2000). Riverine barriers and the geographic distribution of Amazonian species. Proc. Natl Acad. Sci. U.S.A. 97, 13672–13677. doi: 10.1073/pnas.230136397
Gensac, E., Martinez, J.-M., Vantrepotte, V., and Anthony, E. J. (2016). Seasonal and inter-annual dynamics of suspended sediment at the mouth of the Amazon river: the role of continental and oceanic forcing, and implications for coastal geomorphology and mud bank formation. Cont. Shelf Res. 118, 49–62. doi: 10.1016/j.csr.2016.02.009
Gonzalez-Socoloske, D., and Snarr, K. A. (2010). An incident of swimming in a large river by a mantled howling monkey (Alouatta Palliata) on the north coast of Honduras. Neotrop. Primates 17, 28–31. doi: 10.1896/044.017.0102
Graham, C. H., and Fine, P. V. (2008). Phylogenetic beta diversity: linking ecological and evolutionary processes across space in time. Ecol. Lett. 11, 1265–1277. doi: 10.1111/j.1461-0248.2008.01256.x
Gualda-Barros, J., Nascimento, F. O., and Amaral, M. K. (2012). A new species of Callicebus Thomas, 1903 (Primates, Pitheciidae) from the states of Mato Grosso and Pará, Brazil. Pap. Avulsos. Zool. 52, 261–279. doi: 10.1590/s0031-10492012002300001
Herkt, K. M. B., Skidmore, A. K., and Fahr, J. (2017). Macroecological conclusions based on IUCN expert maps: a call for caution. Glob. Ecol. Biogeogr. 26, 930–941. doi: 10.1111/geb.12601
Hershkovitz, P. (1977). Living New World Monkeys (Platyrrhini). Chicago, IL: The University of Chicago Press.
Hooke, J. (2003). River meander behaviour and instability: a framework for analysis. Trans. Inst. Br. Geogr. 28, 238–253. doi: 10.1111/1475-5661.00089
Hoorn, C., Wesselingh, F. P., Steege, H. T., Bermudez, M. A., Mora, A., Sevink, J., et al. (2010). Amazonia through time: andean uplift, climate change, landscape evolution, and biodiversity. Science 330, 927–931. doi: 10.1126/science.1194585
Juen, L., and De Marco, P. (2012). Dragonfly endemism in the Brazilian Amazon: competing hypotheses for biogeographical patterns. Biodivers. Conserv. 21, 3507–3521. doi: 10.1007/s10531-012-0377-0
Kalamandeen, M., Gloor, E., Mitchard, E., Quincey, D., Ziv, G., Spracklen, D., et al. (2018). Pervasive Rise of Small-scale Deforestation in Amazonia. Sci. Rep. 8:1600. doi: 10.1038/s41598-018-19358-2
Laranjeiras, T. O., Naka, L. N., Leite, G. A., and Cohn-Haft, M. (2021). Effects of a major Amazonian river confluence on the distribution of floodplain forest avifauna. J. Biogeogr. 48, 847–860. doi: 10.1111/jbi.14042
Latrubesse, E. M., Cozzuol, M., da Silva-Caminha, S. A. F., Rigsby, C. A., Absy, M. L., and Jaramillo, C. (2010). The Late Miocene paleogeography of the Amazon Basin and the evolution of the Amazon River system. Earth-Sci. Rev 99, 99–124. doi: 10.1016/j.earscirev.2010.02.005
Latrubesse, E. M., Stevaux, J. C., and Sinha, R. (2005). Tropical rivers. Geomorphology 70, 187–206. doi: 10.1016/j.geomorph.2005.02.005
Lees, A. C., Peres, C. A., Fearnside, P. M., Schneider, M., and Zuanon, J. A. S. (2016). Hydropower and the future of Amazonian biodiversity. Biodivers. Conserv. 25, 451–466. doi: 10.1007/s10531-016-1072-3
Lima, M. G. M., Buckner, J. C., Silva-Júnior, J. D. S. E., Aleixo, A., Martins, A. B., Boubli, J. P., et al. (2017). Capuchin monkey biogeography: understanding Sapajus Pleistocene range expansion and the current sympatry between Cebus and Sapajus. J. Biogeogr. 44, 810–820. doi: 10.1111/jbi.12945
Link, A., Valencia, L. M., Céspedes, L. N., Duque, L. D., Cadena, C. D., and Di Fiore, A. (2015). Phylogeography of the critically endangered brown spider monkey (Ateles hybridus): testing the riverine barrier hypothesis. Int. J. Primatol. 36, 530–547. doi: 10.1007/s10764-015-9840-6
Lynch Alfaro, J. W., Boubli, J. P., Olson, L. E., Di Fiore, A., Wilson, B., Gutiérrez-Espeleta, G. A., et al. (2012). Explosive Pleistocene range expansion leads to widespread Amazonian sympatry between robust and gracile capuchin monkeys. J. Biogeogr. 39, 272–288. doi: 10.1111/j.1365-2699.2011.02609.x
Lynch Alfaro, J. W., Boubli, J. P., Paim, F. P., Ribas, C. C., Silva, M. N., Messias, M. R., et al. (2015). Biogeography of squirrel monkeys (genus Saimiri): south-central Amazon origin and rapid pan-Amazonian diversification of a lowland primate. Mol. Phylogenet. Evol. 82, 436–454. doi: 10.1016/j.ympev.2014.09.004
Mamalis, L., Sousa da Silva, S., Bezerra Valerio, A., Norris, D., and Michalski, F. (2018). Stepping stones facilitate river crossings by Myrmecophaga tridactyla in the north-eastern Brazilian Amazon. Edentata 19, 30–34. doi: 10.2305/iucn.ch.2018.edentata-19-1.3.en
Mendez-Camacho, K., Leon-Alvarado, O., and Miranda-Esquivel, D. R. (2021). Biogeographic evidence supports the Old Amazon hypothesis for the formation of the Amazon fluvial system. PeerJ 9, e12533. doi: 10.7717/peerj.12533
Miller, J. T., Jolley-Rogers, G., Mishler, B. D., and Thornhill, A. H. (2018). Phylogenetic diversity is a better measure of biodiversity than taxon counting. J. Syst. Evol. 56, 663–667. doi: 10.1111/jse.12436
Naka, L. N. (2011). Avian distribution patterns in the Guiana Shield: implications for the delimitation of Amazonian areas of endemism. J. Biogeogr. 38, 681–696. doi: 10.1111/j.1365-2699.2010.02443.x
Naka, L. N., and Pil, M. W. (2020). Moving beyond the riverine barrier vicariant paradigm. Mol Ecol 29, 2129–2132. doi: 10.1111/mec.15465
Nunes, A. V. (2014). Report of a black spider monkey (Ateles chamek) swimming in a large river in central-western Brazil. Neotrop. Primates 21, 204–206. doi: 10.1896/044.021.0210
Paglia, A. P., da Fonseca, G. A. B., Rylands, A. B., Herrmann, G., Aguiar, L. M. S., Chiarello, A. G., et al. (2012). Annotated checklist of Brazilian Mammals. Occas. Pap. Conserv. Biol. 6, 1–76. doi: 10.1590/0001-3765202020191004
Patton, J. L., Da Silva, M. N., and Malcolm, J. R. (1994). Gene genealogy and differentiation among arboreal spiny rats (Rodentia: Echimyidae) of the Amazon basin: a test of the riverine barrier hypothesis. Evolution 48, 1314–1323. doi: 10.1111/j.1558-5646.1994.tb05315.x
Peixoto, J. M. A., Nelson, B. W., and Wittmann, F. (2009). Spatial and temporal dynamics of river channel migration and vegetation in central Amazonian white-water floodplains by remote-sensing techniques. Remote Sens. Environ. 113, 2258–2266. doi: 10.1016/j.rse.2009.06.015
Perelman, P., Johnson, W. E., Roos, C., Seuanez, H. N., Horvath, J. E., Moreira, M. A., et al. (2011). A molecular phylogeny of living primates. PLoS Genet. 7:e1001342. doi: 10.1371/journal.pgen.1001342
Peres, C. A., Patton, J. L., and Da Silva, M. N. (1996). Riverine barriers and gene flow in Amazonian saddle-back tamarins. Folia Primatol. 67, 113–124. doi: 10.1159/000157213
Prevedello, J. A., and Vieira, M. V. (2009). Does the type of matrix matter? A quantitative review of the evidence. Biodivers. Conserv. 19, 1205–1223. doi: 10.1007/s10531-009-9750-z
QGIS Development Team (2022). QGIS Geographic Information System. Open Source Geospatial Foundation Project. Available online at: http://qgis.osgeo.org (accessed February 24, 2022).
R Core Team (2020). “R: A Language and Environment for Statistical Computing”. Version 3.6.3 ed. Vienna: R Foundation for Statistical Computing.
Rabelo, R. M., Aragón, S., Bicca-Marques, J. C., and Nelson, B. W. (2019). Habitat amount hypothesis and passive sampling explain mammal species composition in Amazonian river islands. Biotropica 51, 84–92. doi: 10.1111/btp.12615
Rabelo, R. M., Silva, F. E., Vieira, T., Ferreira-Ferreira, J., Paim, F. P., Dutra, W., et al. (2014). Extension of the geographic range of Ateles chamek (Primates, Atelidae): evidence of river-barrier crossing by an amazonian primate. Primates 55, 167–171. doi: 10.1007/s10329-014-0409-3
Ribas, C. C., Aleixo, A., Nogueira, A. C., Miyaki, C. Y., and Cracraft, J. (2012). A palaeobiogeographic model for biotic diversification within Amazonia over the past three million years. Proc. R. Soc. B 279, 681–689. doi: 10.1098/rspb.2011.1120
Rossetti, D. F., Vasconcelos, D. L., Valeriano, M. M., and Bezerra, F. H. R. (2021). Tectonics and drainage development in central Amazonia: the Juruá River. Catena 206:105560. doi: 10.1016/j.catena.2021.105560
Ruokolainen, K., Moulatlet, G. M., Zuquim, G., Hoorn, C., and Tuomisto, H. (2019). Geologically recent rearrangements in central Amazonian river network and their importance for the riverine barrier hypothesis. Front. Biogeogr. 11:e45046. doi: 10.21425/f5fbg45046
Rylands, A. B., Heymann, E. W., Lynch Alfaro, J., Buckner, J. C., Roos, C., Matauschek, C., et al. (2016). Taxonomic review of the New World tamarins (Primates: Callitrichidae). Zool. J. Linn. Soc. 177, 1003–1028. doi: 10.1111/zoj.12386
Rylands, A. B., and Mittermeier, R. A. (2009). “The diversity of the NewWorld primates (Platyrrhini): an annotated taxonomy,” in South American Primates — Comparative Perspectives in the Study of Behavior, Ecology, and Conservation, eds P. A. Garber, A. Estrada, J. C. Bicca-Marques, E. W. Heymann, and K. B. Strier (Berlin: Springer), 23–54. doi: 10.1007/978-0-387-78705-3_2
Rylands, A. B., Schneider, H., Langguth, A., Mittermeier, R. A., Groves, C. P., and Rodríguez-Luna, E. (2000). An assessment of the diversity of new world primates. Neotrop. Primates 8, 61–93.
Sales, L. P., Ribeiro, B. R., Pires, M. M., Chapman, C. A., and Loyola, R. (2019). Recalculating route: dispersal constraints will drive the redistribution of Amazon primates in the Anthropocene. Ecography 42, 1789–1801. doi: 10.1111/ecog.04499
Santorelli, S. Jr., Magnusson, W. E., and Deus, C. P. (2018). Most species are not limited by an Amazonian river postulated to be a border between endemism areas. Sci. Rep. 8:2294. doi: 10.1038/s41598-018-20596-7
Schloss, C. A., Nunez, T. A., and Lawler, J. J. (2012). Dispersal will limit ability of mammals to track climate change in the Western Hemisphere. Proc. Natl Acad. Sci. U.S.A. 109, 8606–8611. doi: 10.1073/pnas.1116791109
Schneider, H., Canavez, F. C., Sampaio, I., Moreira, M. A., Tagliaro, C. H., and Seuanez, H. N. (2001). Can molecular data place each neotropical monkey in its own branch? Chromosoma 109, 515–523. doi: 10.1007/s004120000106
Serrano-Villavicencio, J. E., Hurtado, C. M., Vendramel, R. L., and Nascimento, F. O. (2019). Reconsidering the taxonomy of the Pithecia irrorata species group (Primates: Pitheciidae). J. Mammal. 100, 130–141. doi: 10.1093/jmammal/gyy167
Sillero, N., Biaggini, M., and Corti, C. (2018). Analysing the importance of stepping-stone islands in maintaining structural connectivity and endemicity. Biol. J. Linn. Soc. 124, 113–125. doi: 10.1093/biolinnean/bly033
Silva, F. E., Costa-Araújo, R., Boubli, J. P., Santana, M. I., Franco, C. L. B., Bertuol, F., et al. (2018). In search of a meaningful classification for Amazonian marmosets: should dwarf marmosets be considered Mico congenerics? Zool. Scr. 47, 133–143. doi: 10.1111/zsc.12278
Silva, S. M., Peterson, A. T., Carneiro, L., Burlamaqui, T. C. T., Ribas, C. C., Sousa-Neves, T., et al. (2019). A dynamic continental moisture gradient drove Amazonian bird diversification. Sci. Adv. 5:eaat5752. doi: 10.1126/sciadv.aat5752
Smith, R. J., and Jungers, W. L. (1997). Body mass in comparative primatology. J. Hum. Evol. 32, 523–559. doi: 10.1006/jhev.1996.0122
Soares-Filho, B. S., Nepstad, D. C., Curran, L. M., Cerqueira, G. C., Garcia, R. A., Ramos, C. A., et al. (2006). Modelling conservation in the Amazon basin. Nature 440, 520–523. doi: 10.1038/nature04389
Vallinoto, M., Araripe, J., Rego, P. S., Tagliaro, C. H., Sampaio, I., and Schneider, H. (2006). Tocantins river as an effective barrier to gene flow in Saguinus niger populations. Genet. Mol. Biol. 29, 215–219. doi: 10.1590/S1415-47572006000200005
van Roosmalen, M. G. M., van Roosmalen, T., and Mittermeier, R. A. (2002). A taxonomic review of the titi monkeys, genus Callicebus Thomas, 1903, with the description of two new species, Callicebus bernhardi and Callicebus stephennashi, from Brazilian Amazonia. Neotrop. Primates 10, 1–52.
Wesselingh, F. P., Hoorn, C., Kroonenberg, S. B., Antonelli, A., Lundberg, J. G., Vonhof, H. B., et al. (2010). “On the origin of Amazonian landscapes and biodiversity: a synthesis,” in Amazonia – Landscape and Species Evolution: A look into the Past, eds C. Hoorn and F. P. Wesselingh (Hoboken, NJ: Blackwell Publishing Ltd), 421–431. doi: 10.1016/j.ympev.2017.11.019
Keywords: Amazonian rivers, biogeography, dispersal, macroecology, species turnover, vicariance, Wallace’s riverine barrier hypothesis, β-diversity
Citation: Mourthé Í, Hilário RR, Carvalho WD and Boubli JP (2022) Filtering Effect of Large Rivers on Primate Distribution in the Brazilian Amazonia. Front. Ecol. Evol. 10:857920. doi: 10.3389/fevo.2022.857920
Received: 19 January 2022; Accepted: 21 April 2022;
Published: 09 May 2022.
Edited by:
Fernanda P. Werneck, National Institute of Amazonian Research (INPA), BrazilReviewed by:
Rafael M. Rabelo, Instituto de Desenvolvimento Sustentável Mamirauá, BrazilUbirajara Oliveira, Federal University of Minas Gerais, Brazil
Copyright © 2022 Mourthé, Hilário, Carvalho and Boubli. This is an open-access article distributed under the terms of the Creative Commons Attribution License (CC BY). The use, distribution or reproduction in other forums is permitted, provided the original author(s) and the copyright owner(s) are credited and that the original publication in this journal is cited, in accordance with accepted academic practice. No use, distribution or reproduction is permitted which does not comply with these terms.
*Correspondence: Ítalo Mourthé, imourthe@gmail.com; Jean P. Boubli, j.p.boubli@salford.ac.uk