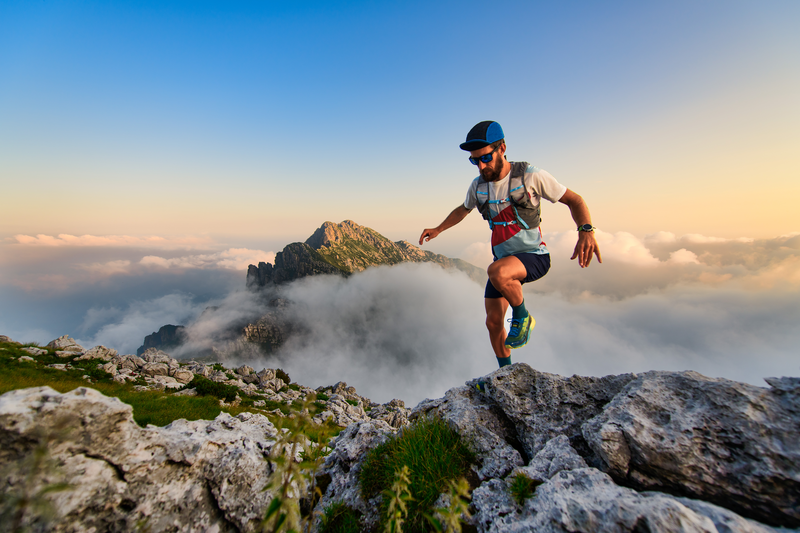
94% of researchers rate our articles as excellent or good
Learn more about the work of our research integrity team to safeguard the quality of each article we publish.
Find out more
ORIGINAL RESEARCH article
Front. Ecol. Evol. , 03 May 2022
Sec. Conservation and Restoration Ecology
Volume 10 - 2022 | https://doi.org/10.3389/fevo.2022.855547
Clarifying the adaptation mechanism of alpine plants to climate or habitat under the alpine environmental gradient on the Qinghai–Tibet Plateau is substantially important to understand the their geography in alpine regions and their responses to future climate change. The spatial distribution characteristics of functional traits in leaves and current-year twigs of Quercus aquifolioides on five consecutive altitudinal gradients in Southeastern Tibet were analyzed. The relationship between the functional traits and habitat factors (topographic and soil factors) was explored. Key results: the functional traits of leaves and current-year twigs of Quercus aquifolioides in Southeastern Tibet showed significant linear variations along the altitudinal gradients (p < 0.001). Quercus aquifolioides at low altitudes tended to have shorter current-year twigs and less leaves with larger LA (leaf area) and higher RWC (relative water content) than those at high altitudes. Strong trade-off and coordination relationship were found between the functional traits of leaves and those of current-year twigs, respectively. SL (slope) and TN (total nitrogen) contributed the most to leaf functional traits (p < 0.05); AL (altitude) was the main determinant of functional traits in current-year twigs of Quercus aquifolioides in southeast Tibet. In conclusion, our observation demonstrate that the ecological adaptation strategy of Quercus aquifolioides was formed through the trade-off mechanism among various functional traits, the variation of hydrothermal conditions and soil environmental factors caused by altitude in the alpine region lead to differences in functional traits of Quercus aquifolioides along an altitudinal gradient in southeast Tibet.
Plant functional traits reflect their survival, growth, and reproduction strategies adapted to the external environment during the evolutionary process, and they exert a strong influence on the structure and function of ecosystems (Faucon et al., 2017; Fu et al., 2020). Meanwhile, plant functional traits have mutual adaptability and co-evolution with their habitats; they could not only reflect the ability of plants to acquire, utilize, and conserve resources but also closely link the environment, the plant individual, and the biological system structure, process, and function (Funk et al., 2017; Bruelheide et al., 2018; Eller et al., 2020). Therefore, investigating the variation characteristics of plant functional traits in different habitats under global change, including plant ecological strategies (Wang et al., 2019; Balazs et al., 2020), community assembly processes (Zirbel and Brudvig, 2020), and mechanisms of species coexistence (Siefert et al., 2015; Kunstler et al., 2016; Guo et al., 2020), has become a key issue in recent years for global ecology. Leaves are important organs for the photosynthesis of plants and the hubs for energy conversion of primary producers (Read et al., 2014). Meanwhile, they are most sensitive to environmental changes, and the effects of environmental changes on plants are often reflected through leaf traits. Therefore, leaf functional traits are crucial to understand the differences in external phenotypes of plant leaves and the mutual adaptation between internal physiological dynamics and environment (Wei et al., 2020). Current-year twigs are the most dynamic part of the plant because of their high internal nutrient flow and transfer, and they reflect the response of the plant to the environment better than the whole plant (Wang et al., 2019). Different environmental factors, such as climate, topography, and soil, could affect plant growth by influencing ecological factors, such as light intensity, temperature, precipitation, and soil traits, and then change and adjust plant functional traits (Fu et al., 2015; Fu and Shen, 2016, 2017; Kunstler et al., 2016; Niu et al., 2020). Altitude, as a comprehensive environmental factor, makes environmental factors such as temperature, precipitation, and illumination, change extensively in a small range of vertical gradients, thus affecting plant stress tolerance and adaptability. Therefore, altitude gradient is an ideal factor to study plant adaptation to environmental changes (Aiba et al., 2020; Peng et al., 2020). Studies have shown that with the increase in altitude, specific leaf area (SLA) and leaf phosphorus content showed a linear upward trend, while leaf thickness (LT), leaf area (LA), leaf potassium content, and length-to-width ratio (L/W) decreased. Leaf relative water content (RWC), leaf nitrogen content (LNC), and leaf nitrogen: phosphorus (N:P) ratio showed a curve with the increase in altitude (first decreased and then increased), while leaf dry matter content (LDMC) first increased and then decreased (Aiba et al., 2020; Niu et al., 2020; Peng et al., 2020). Plants at higher altitudes tend to invest more in current-year twigs and stems, and thicker stems could support heavier reproduction and photosynthetic construction and withstand greater winds (Sun et al., 2019). However, plants at low altitude tend to have longer twig length, which is beneficial for plants to capture light energy, improve flower pollination efficiency and seed diffusion (Zhu et al., 2019). Meanwhile, the special external environment, such as strong wind and heavy snow in high altitude area restricts the random overgrowth of twigs; thus, the twig stem structure has certain trade-off characteristics in longitudinal and radial direction (Sun et al., 2019).
Quercus aquifolioides, as the most widely and highest distributed hard-leaf evergreen broad-leaved forest climax, is of great importance to maintain the stability of the high-altitude fragile ecosystem and soil and water conservation; it also plays a vital role in the ecological security barrier of Qinghai–Tibet plateau (Li et al., 1998, 2007; Shen et al., 2016). At present, studies on Quercus aquifolioides were mainly concentrated in Zheduo Mountain in Ganzi Prefecture of Sichuan Province (Zhu et al., 2010, 2012; Xie et al., 2014), Balang Mountain in Wolong of Sichuan Province (Li et al., 2009; Liu et al., 2013; Zhou et al., 2015), Yulong Mountain in Yunnan Province (Huang et al., 2015), and Changbai Mountain in Jilin Province (Cong et al., 2018), which mainly focused on the supply and accumulation of nutrient elements (Li et al., 2007, 2009; Zhu et al., 2010, 2012; Xie et al., 2014; Cong et al., 2018), photosynthesis and respiration, and other physiological characteristics (Huang et al., 2015). Only a few studies could be found on the spatial distribution pattern (Shen et al., 2016) and genetic diversity (Li et al., 1998) of Quercus aquifolioides in Southeastern Tibet. Few studies on the altitudinal distribution of functional traits of leaves and current-year twigs of Quercus aquifolioides in Southeastern Tibet were also available. The present study aimed to clarify the following three issues by analyzing the spatial distribution characteristics of the founctional traits of leaves and current-year twigs of Quercus aquifolioides under five consecutive altitude gradients: (1) to explore the altitudinal distribution of functional traits in leaves and current-year twigs of Quercus aquifolioides in Southeastern Tibet; (2) to investigate the trade-off mechanism of functional traits in leaves and current-year twigs of Quercus aquifolioides in the process of adaptation and acclimation to alpine environment; and (3) to clarify the relationships between the functional traits and environmental factors.
The research area is located in Gongbu Nature Reserve, Gongbu Jiangda County, Nyingchi City, Tibet Autonomous Region (E93°13′–94°50′, N28°40′–30°20′). The annual average temperature is above 7°C, and the frost-free period is more than 150 days. The annual average precipitation and evaporation are 500–700 mm and 1,300–1,700 mm, respectively. The annual average relative humidity is 50–75%, the annual sunshine hours are more than 1,900 h. The area has a large altitudinal difference (2,300–6,060 m), with an average altitude of more than 3,500 m, and the vertical distribution of plants are obvious. The main trees distributed in the region include dark coniferous forest dominated by Abies georgei var. smithii (Viguie et Gaussen) Cheng et L. and Picea likiangensis var. linzhiensis Cheng et L.F. Fu, bright coniferous forest dominated by Pinus densata, evergreen broadleaf hard forest dominated by Quercus aquifolioides Rehd. et Wils., and deciduous broad-leaved forest dominated by Betula platyphylla Suk. and Populus davidiana Dode.
Samples were collected according to the standardized measurement manual of plant functional traits (Cornelissen et al., 2003; Pérez-Harguindeguy et al., 2013; Moretti et al., 2016). At the end of the growing season for alpine plants on the Tibetan plateau in September 2020, we established sample plots in mature forests at altitudes of 3,186, 3,291, 3,417, 3,519, and 3,620 m above sea level. The general information of each sample plot along altitude gradient is shown in Table 1. Three 20 m × 20 m quadrates were built at each altitude, 15 quadrates in total. The altitude, location, slope (SL), slope aspect (SP), vegetation coverage, and soil temperature, soil moisture, soil bulk density of each quadrates were measured. In each quadrates, three healthy mature individuals of Quercus aquifolioides with similar DBH and stand age were selected. Four well-grown, unshaded branches from the middle of the tree were collected, followed by five mature leaves and three current year twigs from each branch. A total of 180 mature leaves and 108 current year twigs were collected at a single altitude. Samples were marked, placed into plastic bags, and stored in a 4°C incubator, and taken to the laboratory to complete the determination of functional traits within 24 h.
The collected leaves were soaked in distilled water to a constant weight, then removed and wiped dry. The saturated fresh weight (SW) was weighed with an electronic balance (0.0001 g). Then, the leaf length (LL), the leaf width at the widest part in the middle of the leaves (LW), and the leaf thickness (LT) at the place away from the main vein was measured from the tip, middle and bottom of leaf then get the mean value with a vernier caliper (0.01 mm). Afterward, the length–width ratio (LL/LW) and leaf circumference (LPM) were calculated. The leaf area (LA) was scanned by LI-3000A leaf area analyzer (LI-COR, Lincoln, NE, United States), and then the leaf volume (LV) was calculated as follows: LV = LA × LT. The fresh weight (FW) and dry weight (DW) of leaves were measured using an electronic balance (0.0001 g). Lastly, leaf dry-matter content (LDMC), specific leaf area (SLA), leaf density (LD), and relative water content (RWC) were calculated as follows: LDMC = DW/SW; SLA = LA/DW; LD = DW/LV; and RWC = (FW–DW)/(SW–DW) × 100%. All the abbreviations of leaf functional traits were defined in Table 2.
The number of leaves on each current-year twig was recorded, every leaf area (LA -single) was scanned by LI-3000A leaf area analyzer (LI-COR, Lincoln, NE, United States), and then the total leaf area (TLA) on each twig was calculated. The twig diameter (D) and length (TL) of the twig were measured with a vernier caliper (accuracy of 0.01 mm), and then the cross-sectional sapwood area (TSA) of the twig was measured. The volume (TV) of the twig was measured by drainage method. The twigs were dried at 80°C to constant weight, and the twig dry weight (TDW) was measured using an electronic balance (0.0001 g). Then, the twig tissue density (TD), leaf emergence intensity (LEI), leaf spreading efficiency (LSE), twig leafing intensity (TLD), and Huber value (HV) were calculated as follows: TD = TDW/TV; LEI = TLN/TL; LSE = TLA/TL; TLD = TLN/TDW; HV = TSA/TLA (Schumann et al., 2019). All the abbreviations of current-year twig functional traits were defined in Table 2.
Three cutting-ring soil samples and 200 g bulk soil samples were collected near the each sample tree. A total of nine cutting-ring soil samples and nine bulk samples were collected at a single altitude. The soil temperature (ST) and soil moisture content (SWC) of soil samples were measured in situ (20 cm underground) by using the portable soil quick-measuring kit (WET Kit, delta-T, United Kingdom). Soil pH was measured by potentiometric method (Starter 300, United States), soil bulk density (SBD) was measured by cutting-ring method, soil organic carbon (TOC) was measured by K2Cr2O7 external heating method, soluble organic carbon (DOC) was measured by Total Organic Carbon Analyzer (TOC-VCPH/CPN, Japan). Micro-Kjeldahl method was developed to determine total nitrogen (TN). Soil total phosphorus (TP) was determined by HCLO4-H2SO4 digestion combined with molybdenum antimony resistance spectrophotometric method, soil available phosphorus (AP) was determined by molybdenum antimony method after 0.5 M NaHCO3 extraction, soil total potassium (TK) was determined by HF-HCLO4 digestion combined flame spectrum method, and soil available potassium (AK) was determined by flame spectrum method after extraction with 1 M NH4OAc.
All the statistics were calculated using SPSS software (v.25.0, IBM Corp., Armonk, NY, United States) and plotting was performed on Origin 2021b (Origin lab, Northampton, Massachusetts, United States). A p-value < 0.05 was considered for assessing significant statistical effects. One-way ANOVA and least significant difference method (LSD) were used to analyze the significance of the differences in functional traits among different altitude gradients. Only the functional indicators with significant differences among altitudes were selected, the relationship between them and altitudes were analyzed by regression, and the optimal regression model was selected in accordance with the regression coefficient. Pearson correlation analysis was performed to explore the relationships between functional traits. The functional traits of each leaf and current-year twig were selected as data of species. Three topographical factors [altitude (AL), slope (SL), and slope aspect (SP)] and 11 soil factors (pH, SBD, ST, SW, TN, TP, TK, AP, AK, TOC, DOC) were used as environmental data. Redundancy analysis (RDA) was performed using Canoco 5.0 (Microcomputer Power, United States) to screen the main functional traits of the leaves and current-year twigs of Quercus aquifolioides and evaluate the effects of environmental factors on the functional traits of Quercus aquifolioides populations. Prior to RDA, the significance of the effect of each variable was assessed using a Monte Carlo permutation test.
The variation characteristics of leaf functional traits (LL/LW, LA, SLA, LV, LD, and RWC) along altitude gradient are shown in Figure 1. All the functional traits were significantly different (p < 0.001, Table 3) among altitudes to some extent, and they showed linear variation with the altitude. LL/LW and LD linearly increased with the altitude (p < 0.001), whereas LA, SLA, LV, and RWC linearly decreased (p < 0.001). The variation characteristics of the functional traits of current-year twigs (TL, TV, TD, ILG, DGT, and HV) along altitude gradients are shown in Figure 2. All the functional traits were significantly different (p < 0.05), and they showed linear variation with altitude. TL (p < 0.05), TV (p < 0.001), and LEI (p < 0.05) linearly increased with the increase in altitude, whereas TD, TLD, and HV linearly decreased (p < 0.05).
Figure 1. Altitudinal distribution of leaf functional traits of Quercus aquifolioides in Southeastern Tibet. (A) Altitudinal distribution of leaf length-width ratio (LL/LW); (B) altitudinal distribution of leaf area (LA); (C) altitudinal distribution of specific leaf area (SLA); (D) altitudinal distribution of leaf volume (LV); (E) altitudinal distribution of leaf density (LD); and (F) altitudinal distribution of relative water content (RWC).
Table 3. One-way analysis of variance of functional traits of Quercus aquifolioides between altitude.
Figure 2. Altitudinal distribution of twigs functional traits of Quercus aquifolioides in Southeastern Tibet. (A) Altitudinal distribution of twig length (TL); (B) altitudinal distribution of twig volume (TV); (C) altitudinal distribution of twig tissue density (TD); (D) altitudinal distribution of intensity of leaf growth based on twigs (LEI); (E) altitudinal distribution of Huber value (HV); and (F) altitudinal distribution of leafing intensity (TLD).
Pearson correlation analysis results showed that among the indicators of leaf functional traits, LA had a very significant positive correlation with SLA (r = 0.361, p < 0.001) (Figure 3), but this significantly positive relationship just existed in 3,186 and 3,620 m (Figure 4 and Supplementary Table 2). LA was significantly negatively correlated with LDMC (r = −0.362, p < 0.001) and LD (r = −0.901, p < 0.001) (Figure 3), which were only existed in low altitude (Figure 4 and Supplementary Table 2). SLA showed a significant positive correlation with LA (r = 0.361, p < 0.001), LW (r = 0.391, p < 0.001) and LPM (r = 0.320, p < 0.001) (Figure 3), meanwhile, showed significantly negatively relationship with LDMC (r = −0.437, p < 0.001) and LD (r = −0.518, p < 0.001). These significantly negatively relationship existed at five altitudinal gradients except for 3,519 m (Figure 4 and Supplementary Table 2). LDMC showed significant negative correlation with LA (r = −0.275, p < 0.001) and LV (r = −0.160, p = 0.016) and significant positive correlation with LD (r = 0.291, p < 0.001) and RWC (r = 0.383, p < 0.001).
Figure 4. The scaling relationships between leaf functional traits at different altitudinal gradient. (A) The scaling relationship between leaf area (LA) and specific leaf area (SLA); (B) the scaling relationship between leaf area (LA) and leaf density (LD); (C) the scaling relationship between leaf area (LA) and leaf dry-matter content (LDMC); (D) the scaling relationship between specific leaf area (SLA) and leaf volume (LV); (E) the scaling relationship between specific leaf area (SLA) and leaf density (LD); and (F) the scaling relationship between specific leaf area (SLA) and leaf dry-matter content (LDMC).
TLN was positively correlated with TLA (r = 0.767, p < 0.001) at five altitudinal gradients (Figures 5, 6 and Supplementary Table 2), but negatively correlated with TLD (r = −0.310, p < 0.001). It was found in the Pearson correlation analysis between functional traits of all current-year twigs that there was no relationship between TLA and HV (Figure 5), but TLA was significantly positively related with HV at each altitude (Figure 6 and Supplementary Table 2). LA-single only showed positive correlation with HV (r = 0.560, p < 0.001). LSE was positively correlated with TLA (r = 0.743, p < 0.001), LEI (r = 0.658, p < 0.001) (Figure 5). TLD was negatively correlated with TL (r = −0.625, p < 0.001), TBD (r = −0.629, p < 0.001), TV (r = −0.559, p < 0.001), TDW (r = −0.518, p < 0.001) and TLA (r = −0.343, p < 0.001), respectively (Figure 5).
Figure 6. The scaling relationships between functional traits of current-year twigs at different altitudinal gradient. (A) The scaling relationship between Total leaf area on each twig (TLA) and Twig cross-sectional sapwood area (TSA); (B) the scaling relationship between Total leaf area on each twig (TLA) and Total number of leaves on each twig (TLN); (C) the scaling relationship between Total leaf area on each twig (TLA) and Huber value (HV); (D) the scaling relationship between Huber value (HV) and Twig cross-sectional sapwood area (TSA); (E) the scaling relationship between Huber value (HV) and intensity of leaf growth based on the twigs (LEI); and (F) the scaling relationship between Huber value (HV) and leaf spreading efficiency (LSE).
The cumulative percentage of species-environment relationship between axis 1 and axis 2 was 98.29 and 99.9%, respectively, indicating that there was a high correlation between functional traits and environmental factors, which could clearly reflect the influence degree of dominant factors on leaf and twig functional traits (Table 4). The total contribution value of SL, TN, SBD, pH and DOC to 14 leaf functional traits reached 99.9% (Table 5). SL and TN contributed the most to leaf functional traits and had significant correlation (p < 0.05). The total contribution value of AL, SBD, SL, ST and pH to 14 functional traits of current-year twigs reached 99.9% (Table 5), and AL had the highest contribution value to functional traits of current-year twigs and had significant correlation (p < 0.05). Figure 7 clearly showed the correlation between leading factors and functional traits (factors with contribution rate < 0.1 and p value > 0.9 were not shown in Figure 7). In Figure 7A, LT and LDMC are located in the upper left corner of the RDA ranking diagram, and are positively correlated with DOC, but negatively correlated with pH. LD and LL/LW were located at the left end of axis 1, which were positively correlated with SL and negatively correlated with TN. The other leaf functional traits were located in the upper right corner of the sequence diagram, and were positively correlated with TN and SBD, but negatively correlated with SL. In Figure 7B, LA-single and TLD are located in the upper left corner of the RDA ranking diagram, and are negatively correlated with AL and SL. TD, LSE and HV at the left end of axis 1 were positively correlated with ST and SBD, but negatively correlated with pH. Other functional traits of current year twigs were located in the lower right corner of the sequence diagram, and were positively correlated with AL and SL.
Table 4. RDA analysis of functional traits of leaf and current-year twigs and environmental factors.
Figure 7. RDA analysis of leaf functional traits (A), functional traits of current-year twigs (B) and environmental factors.
The present study showed that LL/LW and LD increased with altitude, whereas LA, SLA, and RWC decreased (Figure 1). The increase in LL/LW indicated that leaves gradually became longer, and the photosynthates of Quercus aquifolioides were used for the longitudinal extension of LA. The reason for the decrease in LA with the increase in altitude may be that the efficiency of light energy acquisition ability of leaves decreased under low-temperature environment, and leaves needed to reduce the rate of respiration and transpiration by reducing LAs (Craine and Lee, 2003). Meanwhile, high altitude and low temperature make the growth rate of trees slow down, LA decreases, photosynthetic rate decreases, net growth decreases, plant cell volume decreases, cell wall thickens, plant leaves become harder, and more photosynthates accumulate to prepare for the winter and the next year’s growth (Hölscher et al., 2002; Wright et al., 2002). SLA decreased with increasing altitude, indicating that in high-altitude areas, Quercus aquifolioides has a better ability to retain the acquired resources and could better adapt to resource-poor high-altitude habitats, which is an adaptation to limited resources (Niu et al., 2020). Combined with higher speed winds at high altitude, it is likely to cause damage to the plants, and the reduced LA makes SLA smaller. In low-altitude areas with relatively abundant resources, high SLA and small LD lead to a large light capture area of the leaves. Thus, plants have a higher production capacity. RWC is not only closely related to photosynthesis, but it also reflects the water and heat conditions of the plant’s living environment to a certain extent (Siefert et al., 2015; Wang et al., 2019). In the present study, RWC decreased linearly with the altitude gradient, which is closely related to the unique and drastic change trend of the altitude gradient of the Qinghai–Tibet Plateau. The water stress caused by the high altitude and low temperature produced lower soil water content, while the low RWC was one of the strategies of adaptation of Quercus aquifolioides to external water and heat conditions in Tibetan plateau.
TL (p < 0.05), TV (p < 0.001), and LEI (p < 0.05) increased linearly with the altitude, whereas TD, TLD, and HV decreased linearly (p < 0.05) (Figure 2). With the increase in altitude, the increase in the length of the twigs is needed for the upper competition resources. The spatial position of the plant branches in the community determines its competitiveness and the ability to obtain resources. A longer TL helps increase light acquisition and reproduction effectiveness and further improves the ability to withstand the harsh environment of high altitude (Sun et al., 2019; Wang et al., 2019). The present study revealed that as the altitude increased, TL increased, which led to an increase in TV. With the increase in TL, TDW and TV also increased. The LSE, TLD, and TD of the current-year twigs of Quercus aquifolioides at high altitude were lower than those in low-altitude areas. Studies have shown that while branchlets grow longitudinally, they also need radial growth to enhance their mechanical support, thereby enhancing the transport of water and nutrients (Pérez-Harguindeguy et al., 2013; Sun et al., 2019; Zhu et al., 2019). Thus, along altitude gradient, TSA increased slowly than TLA (Supplementary Table 1 and Supplementary Figure 1), resulting in a decrease in HV in high-altitude areas. Although the altitude distribution of twig functional traits of woody plants in mountainous areas of China have been widely discussed, no consistent conclusion is available. Zhu et al. (2019) found that the biomass of stems in mixed forests at mid-altitude levels was smaller than that of evergreen and broad-leaved forests at other altitudes in Wuyi Mountain. In the study on the response of stem and leaf traits of Rhododendron przealskii to altitude in Southeastern Qinghai–Tibet Plateau, no significant differences were found in the stem length, stem thickness, specific stem length, and stem biomass of each branch at different altitudes (p > 0.05) (He et al., 2021). In the present study, all twig functional traits demonstrated significant differences between altitudes. The reason is that the mountains in Southeast Tibet have obvious characteristics of mountain vertical belts, which are directly facing the Yarlung Zangbo River water vapor channel and are extremely sensitive to the Indian monsoon response. The correlation of temperature and precipitation between altitudes is significant (Spicer et al., 2003). Thus, the gradient changes in environmental factors lead to the heterogeneity of plant population development and distribution patterns.
Given that multiple traits are often coordinated with one another, plant functional traits could not be viewed and analyzed in isolation, and the trade-off and combination of plant functional traits determines the plant’s life history strategy (Siefert et al., 2015; Funk et al., 2017; Wang et al., 2019; Aiba et al., 2020). The present study found that LA was significantly positively correlated with LV and SLA but significantly negatively correlated with LDMC and LD. Many previous studies have confirmed the co-variation relationship between leaf size and other plant functional traits, and that the formation of leaf size was related to leaf energy balance (Wright et al., 2002; Craine and Lee, 2003; Read et al., 2014; Wang et al., 2019). The possible reason is that in high-altitude and low-temperature habitats, the time for leaves to receive light energy and assimilate carbon dioxide is shortened. Plants may increase the nitrogen content of leaves to stabilize carbon capture capacity, while the cost of respiration and transpiration of leaflets is lower; thus, a low metabolic rate could be better maintained (Zhang et al., 2012). To a certain extent, LDMC represents the plant’s ability to use a certain environmental resource and indicates how much leaves are built (Read et al., 2014). Species with high construction cost per unit LA usually have thicker leaves and higher leaf tissue density (Li and Bao, 2014), especially in high-altitude and low-temperature environments, due to the increase in mesophyll cells and the thickening of cell walls, especially epidermal cell walls and thick and small leaf formats that reduce the internal water loss of plants. Thus, SLA decreases, and the time required for the leaves to repay the initial construction cost through the carbohydrates formed by their own photosynthesis production is longer (Hikosaka et al., 2002).
In the present study, the supported LA per twig cross-sectional sapwood area (HV) was positively correlated with LSE (supported LA per branch length). The results showed that with the increase in the length of the current-year twig, the cross-section diameter of the twig and the number of supporting leaves and LA on the twig increased. Increasing studies have shown that the trade-off between leaf size and leaf emergence is a trade-off shared by woody plants, herbaceous plants, and even a single angiosperm (Wright et al., 2002). Studies have shown that under low temperature, low nutrient soil, and high-altitude stress environment, the biomass allocated to stems at the level of twigs is usually higher, and the leaves have higher LA per unit mass (Wright et al., 2002; Sun et al., 2006). The present study also found a very significant positive correlation between TL of and TD of the current-year twigs, and this finding was related to the trade-off between the longitudinal growth and the radial growth of the plant. The longitudinal growth of the shoots is related to the efficiency of light capture and the spread of seeds; meanwhile, the shoots need to grow radially while growing longitudinally to increase their mechanical support and increase the transportation of water and nutrients (Xiang et al., 2008; Sun et al., 2019). Most studies have shown that current-year twigs, as a metabolically active component, has much greater longitudinal growth rate than radial growth (Xiang et al., 2008; Pérez-Harguindeguy et al., 2013; Zhu et al., 2019). The present study found no significant correlation between TD and other functional traits of the current-year twigs. However, previous studies have shown that low wood density is usually related to the rapid growth of stem diameter and volume, and species with low wood density usually have high LA per branch mass (Wright et al., 2006). In the research on wood density and environmental factors, wood density is strongly related to leaf water potential. Species with high xylem density are usually easier to survive in drier soil and have a lower risk of duct occlusion (Hacke et al., 2001). The twig tissue density of the current-year twigs of Quercus aquifolioides decreased with altitude. In addition, the twigs showed decreased twig tissue density in harsher external habitat, indicating the plant’s increased leaf water potential and deepened roots that could resist high altitude, high winds, and low temperatures in high-altitude areas.
The present study found SL (slope gradient) and TN contributed the most to leaf functional traits and had significant correlation (p < 0.05), which was consistent with Wang’s study on leaf functional traits of apricot (Prunus armeniaca L.) (Wang et al., 2019), they found slope exerted significant effects on SLA (F = 5.578, p = 0.004), LDMC (F = 7.017, p = 0.001), LWC (F = 9.144, p = 0.000), LA (F = 4.797, p = 0.008). As important topographic factors, slope aspect and slope affect plant growth and leaf functional traits by regulating the combination of water and heat. However, we did not find evidence of slope aspect was the main topographic determinant of leaf functional traits. A study in a tropical dry forest landscape in the Balsas river basin in S-central Mexico showed evidence of filtration processes of plant functional strategies to deal with drought and heat stress, operating in the dry forest mostly along topographic positions but not much along the gradient generated by slope aspect (Méndez-Toribio et al., 2017). A number of studies has showed soil nitrogen content was response for the changes in plant functional traits and functional diversity (Jia et al., 2011; Roa-Fuentes et al., 2015; Shovon et al., 2019). In nitrogen-poor sites, changes in functional diversity were mainly attributable to the presence of fast-growing species in sites with higher soil moisture (Poorter and Evans, 1998) and in dry but nitrogen-rich sites, the NUE (nitrogen use effectiveness) of fast-growing species is compromised by moisture stress (Gong et al., 2011). Meanwhile, the present study also found AL was the main determinant of functional traits in current-year twigs of Quercus aquifolioides in southeast Tibet. Previous studies had showed a lot of evidence of altitude is the main topographical factor affecting the functional traits of dominant species (He et al., 2020; Zhang et al., 2021), specially in the Himalayas, stem and branch traits that integrate multiple plant organs and functions are better predictors of species’ altitudinal distributions than leaf traits along the extreme environmental gradient (Maharjan et al., 2021).
The functional traits of leaves and current-year twigs of Quercus aquifolioides in Southeastern Tibet showed significant linear changes within an altitude range suitable for its widespread distribution of a single species. The Quercus aquifolioides species at low altitude tend to have shorter current-year twigs, a smaller amount of leaves with larger LA, and higher relative water than those at high altitude. A strong mutual balance and coordination relationships were also found between the functional traits of the leaves and current-year twigs, respectively. SL (slope) and TN contributed the most to leaf functional traits and AL (altitude) was the main topographic determinant of functional traits in current-year twigs. The ecological adaptation strategy of Q. aquifolioides was formed through the trade-off mechanism among various functional traits to adapt to the changes of hydrothermal conditions and soil environmental factors caused by altitude gradient in the alpine region.
The original contributions presented in the study are included in the article/Supplementary Material, further inquiries can be directed to the corresponding author/s.
XZ: software, methodology, and writing original draft. CW: methodology and writing original draft. CZ: methodology and conceptualization. All authors contributed to the article and approved the submitted version.
This work was supported by the National Natural Science Foundation of China (31960256) and the central government guides local projects of China (XZ202101YD0016C).
The authors declare that the research was conducted in the absence of any commercial or financial relationships that could be construed as a potential conflict of interest.
All claims expressed in this article are solely those of the authors and do not necessarily represent those of their affiliated organizations, or those of the publisher, the editors and the reviewers. Any product that may be evaluated in this article, or claim that may be made by its manufacturer, is not guaranteed or endorsed by the publisher.
The Supplementary Material for this article can be found online at: https://www.frontiersin.org/articles/10.3389/fevo.2022.855547/full#supplementary-material
Aiba, M., Kurokawa, H., Onoda, Y., Nakashizuka, T., and Roxburgh, S. (2020). Trait–abundance relationships in tree communities along temperature and successional gradients. J. Veg. Sci. 31, 551–560. doi: 10.1111/jvs.12878
Balazs, K. R., Kramer, A. T., Munson, S. M., Talkington, N., Still, S., and Butterfield, B. J. (2020). The right trait in the right place at the right time: matching traits to environment improves restoration outcomes. Ecol. Appl. 30:e02110. doi: 10.1002/eap.2110
Bruelheide, H., Dengler, J., Purschke, O., Lenoir, J., Jimenez-Alfaro, B., Hennekens, S. M., et al. (2018). Global trait-environment relationships of plant communities. Nat. Ecol. Evol. 2, 1906–1917. doi: 10.1038/s41559-018-0699-8
Cong, Y., Wang, A., He, H. S., Yu, F. H., Tognetti, R., Cherubini, P., et al. (2018). Evergreen Quercus aquifolioides remobilizes more soluble carbon components but less N and P from leaves to shoots than deciduous Betula ermanii at the end-season. iForest Biogeosci. Forest. 11, 517–525. doi: 10.3832/ifor2633-011
Cornelissen, J. H. C., Lavorel, S., Garnier, E., Díaz, S., Buchmann, N., Gurvich, D. E., et al. (2003). A handbook of protocols for standardised and easy measurement of plant functional traits worldwide. Austr. J. Bot. 51:45. doi: 10.1071/bt02124
Craine, J. M., and Lee, W. G. (2003). Covariation in leaf and root traits for native and non-native grasses along an altitudinal gradient in New Zealand. Oecologia 134, 471–478. doi: 10.1007/s00442-002-1155-6
Eller, C. B., Meireles, L. D., Sitch, S., Burgess, S. S. O., and Oliveira, R. S. (2020). How climate shapes the functioning of Tropical Montane cloud forests. Curr. Forest. Rep. 6, 97–114. doi: 10.1007/s40725-020-00115-6
Faucon, M. P., Houben, D., and Lambers, H. (2017). Plant functional traits: soil and ecosystem services. Trends Plant Sci. 22, 385–394. doi: 10.1016/j.tplants.2017.01.005
Fu, D., Wu, X., Duan, C., Smith, A. R., and Jones, D. L. (2020). Traits of dominant species and soil properties co-regulate soil microbial communities across land restoration types in a subtropical plateau region of Southwest China. Ecol. Eng. 153:105897. doi: 10.1016/j.ecoleng.2020.105897
Fu, G., and Shen, Z. X. (2016). Response of alpine plants to nitrogen addition on the Tibetan Plateau: a meta-analysis. J. Plant Growth Regul. 35, 974–979. doi: 10.1007/s00344-016-9595-0
Fu, G., and Shen, Z. X. (2017). Effects of enhanced UV-B radiation on plant physiology and growth on the Tibetan Plateau: a meta-analysis. Acta Physiol. Plant. 39:85. doi: 10.1007/s11738-017-2387-8
Fu, G., Shen, Z. X., Sun, W., Zhong, Z. M., Zhang, X. Z., and Zhou, Y. T. (2015). A meta-analysis of the effects of experimental warming on plant physiology and growth on the Tibetan Plateau. J. Plant Growth Regul. 34, 57–65. doi: 10.1007/s00344-014-9442-0
Funk, J. L., Larson, J. E., Ames, G. M., Butterfield, B. J., Cavender-Bares, J., Firn, J., et al. (2017). Revisiting the Holy Grail: using plant functional traits to understand ecological processes. Biol. Rev. Camb. Philos. Soc. 92, 1156–1173. doi: 10.1111/brv.12275
Gong, X. Y., Chen, Q., Lin, S., Brueck, H., Dittert, K., Taube, F., et al. (2011). Tradeoffs between nitrogen and water use efficiency in dominant species of the semiarid steppe of Inner Mongolia. Plant Soil 34, 227–238. doi: 10.1007/s11104-010-0525-9
Guo, Y., Zhao, P., Zhou, Z., Yi, J., Chai, Y., and Yue, M. (2020). Coexistence of three common species in a temperate mixed forest: Linking seedling microhabitats and functional traits. For. Ecol. Manag. 465:118057. doi: 10.1016/j.foreco.2020.118057
Hacke, U. G., Sperry, J. S., Pockman, W. T., Davis, S. D., and McCulloh, K. A. (2001). Trends in wood density and structure are linked to prevention of xylem implosion by negative pressure. Oecologia 126, 457–461. doi: 10.1007/s004420100628
He, J. L., Wang, J. N., Zhou, T. Y., Song, Y. K., Shi, N., Naudiyal, N., et al. (2020). Effects of growth stage and altitude on twig functional traits and biomass allocation of Rhododendron przewalskii in the headwater region of Minjiang River, China. Chin. J. Appl. Ecol. 31, 4027–4034. doi: 10.13287/j.1001-9332.202012.001
He, J. L., Wang, J. N., Zhou, T. Y., Song, Y. K., Zha, L., Naudiyal, N., et al. (2021). Responses of stem and leaf traits of Rhododendron przewalskii to altitude in the southeastern Qinghai-Tibet Plateau. Acta. Ecologica. Sinica. 41, 2349–2358. doi: 10.5846/stxb202004230968
Hikosaka, K., Nagamatsu, D., Ishii, H. S., and Hirose, T. (2002). Photosynthesis-nitrogen relationship in species at different altitudes on Mount Kinabalu, Malaysia. Ecol. Res. 17, 305–313. doi: 10.1046/j.1440-1703.2002.00490.x
Hölscher, D., Schmitt, S., and Kupfer, K. (2002). Growth and leaf traits of four broad-leaved tree species along a hillside gradient. Forstw. Cbl. 121, 229–239. doi: 10.1046/j.1439-0337.2002.02031.x
Huang, X. X., Feng, C. C., Jiang, Y. L., Tang, T., and Cheng, X. M. (2015). Physiological and biochemical characteristics of Quercus aquifolioides in response to different altitudes in Yulong Snow Mountain of Yunnan. J. West China Forest. Sci. 44:6.
Jia, D., Qian, W., Hui, Y., and Shouren, Z. (2011). Effects of topographic variations and soil characteristics on plant func-tional traits in a subtropical evergreen broad-leaved forest. Biodiv. Sci. 19, 158–167. doi: 10.3724/sp.J.1003.2011.10312
Kunstler, G., Falster, D., Coomes, D. A., Hui, F., Kooyman, R. M., Laughlin, D. C., et al. (2016). Plant functional traits have globally consistent effects on competition. Nature 529, 204–207. doi: 10.1038/nature16476
Li, C., Wu, C., Duan, B., Korpelainen, H., and Luukkanen, O. (2009). Age-related nutrient content and carbon isotope composition in the leaves and branches of Quercus aquifolioides along an altitudinal gradient. Trees 23, 1109–1121. doi: 10.1007/s00468-009-0354-8
Li, F. L., and Bao, W. K. (2014). Elevational trends in leaf size of Campylotropis polyantha in the arid Minjiang River valley, SW China. J. Arid Environ. 108, 1–9. doi: 10.1016/j.jaridenv.2014.04.011
Li, J., Chen, K. Y., and Li, B. S. (1998). The variation of genetic diversity of Quercus aquifoliodes in different elevations. Acta Bot. Sin. 40:6. doi: 10.3321/j.issn:1672-9072.1998.08.012
Li, M. C., Liu, H. Y., Li, L. X., Yi, X. F., and Zhu, X. J. (2007). Carbon isotope composition of plants along altitudinal gradient and its relationship to environmental factors on the Qinghai-Tibet plateau. Polish J. Ecol. 55:11.
Liu, X., He, F., Fan, H., Pan, H., Li, M., and Liu, S. (2013). Leaf-form characteristics of plants in Quercus aquifolioides community along an elevational gradient on the Balang Mountain in Wolong Nature Reserve, Sichuan, China. Acta Ecol. Sin. 33, 7148–7156. doi: 10.5846/stxb20120718.1024
Maharjan, S. K., Sterck, F. J., Dhakal, B. P., Makri, M., and Poorter, L. (2021). Functional traits shape tree species distribution in the Himalayas. J. Ecol. 109, 3818–3834. doi: 10.1111/1365-2745.13759
Méndez-Toribio, M., Ibarra-Manríquez, G., Navarrete-Segueda, A., and Paz, H. (2017). Topographic position, but not slope aspect, drives the dominance of functional strategies of tropical dry forest trees. Environ. Res. Lett. 12:085002. doi: 10.1088/1748-9326/aa717b
Moretti, M., Dias, A. T. C., Bello, F., Altermatt, F., Chown, S. L., Azcárate, F. M., et al. (2016). Handbook of protocols for standardized measurement of terrestrial invertebrate functional traits. Funct. Ecol. 31, 558–567. doi: 10.1111/1365-2435.12776
Niu, K., Zhang, S., Lechowicz, M. J., and Perez Carmona, C. (2020). Harsh environmental regimes increase the functional significance of intraspecific variation in plant communities. Funct. Ecol. 34, 1666–1677. doi: 10.1111/1365-2435.13582
Peng, Y., Bloomfield, K. J., and Prentice, I. C. (2020). A theory of plant function helps to explain leaf-trait and productivity responses to elevation. New Phytol. 226, 1274–1284. doi: 10.1111/nph.16447
Pérez-Harguindeguy, N., Díaz, S., Garnier, E., Lavorel, S., Poorter, H., Jaureguiberry, P., et al. (2013). New handbook for standardised measurement of plant functional traits worldwide. Austr. J. Bot. 61, 167–234. doi: 10.1071/bt12225
Poorter, H., and Evans, J. R. (1998). Photosynthetic nitrogen-use efficiency of species that differ inherently in specific leaf area. Oecologia 116, 26–37. doi: 10.1007/s004420050560
Read, Q. D., Moorhead, L. C., Swenson, N. G., Bailey, J. K., Sanders, N. J., and Fox, C. (2014). Convergent effects of elevation on functional leaf traits within and among species. Funct. Ecol. 28, 37–45. doi: 10.1111/1365-2435.12162
Roa-Fuentes, L. L., Templer, P. H., and Campo, J. (2015). Effects of precipitation regime and soil nitrogen on leaf traits in seasonally dry tropical forests of the Yucatan Peninsula, Mexico. Oecologia 179, 585–597. doi: 10.1007/s00442-015-3354-y
Schumann, K., Leuschner, C., and Schuldt, B. (2019). Xylem hydraulic safety and efficiency in relation to leaf and wood traits in three temperate Acer species differing in habitat preferences. Trees 33, 1475–1490. doi: 10.1007/s00468-019-01874-x
Shen, Z. Q., Min, H., Dan, Q., Lu, J., and Fang, J. P. (2016). Spatial pattern analysis and associations of Quercus aquifolioides population at different growth stages in Southeast Tibet, China. Chin. J. Appl. Ecol. 27, 387–394.
Shovon, T. A., Rozendaal, D. M. A., Gagnon, D., Gendron, F., Vetter, M., and Vanderwel, M. C. (2019). Plant communities on nitrogen-rich soil are less sensitive to soil moisture than plant communities on nitrogen-poor soil. J. Ecol. 108, 133–144. doi: 10.1111/1365-2745.13251
Siefert, A., Violle, C., Chalmandrier, L., Albert, C. H., Taudiere, A., Fajardo, A., et al. (2015). A global meta-analysis of the relative extent of intraspecific trait variation in plant communities. Ecol. Lett. 18, 1406–1419. doi: 10.1111/ele.12508
Spicer, R. A., Harris, N. B., Widdowson, M., Herman, A. B., Guo, S. X., Valdes, P. J., et al. (2003). Constant elevation of southern Tibet over the past 15 million years. Nature 421, 622–624. doi: 10.1038/nature.01356
Sun, J., Wang, M., Lyu, M., Niklas, K. J., Zhong, Q., Li, M., et al. (2019). Stem diameter (and Not Length) limits twig leaf biomass. Front Plant Sci. 10:185. doi: 10.3389/fpls.2019.00185
Sun, S., Jin, D., and Shi, P. (2006). The leaf size-twig size spectrum of temperate woody species along an altitudinal gradient: an invariant allometric scaling relationship. Ann. Bot. 97, 97–107. doi: 10.1093/aob/mcj004
Wang, X. Y., Cao, J. J., Zhang, X. F., Zhang, X. F., Kong, Y. Y., Tian, H., et al. (2019). Effects of topographic factors on leaf traits of apricot in the Loess Plateau, Northwest China. Chin. J. Appl. Ecol. 30, 2591–2599. doi: 10.13287/j.1001-9332.201908.027
Wei, M., Wang, S., Wu, B.-d, Jiang, K., Zhou, J.-w, and Wang, C.-y (2020). Variability of leaf functional traits of invasive tree Rhus typhina L. in North China. J. Central South Univ. 27, 155–163. doi: 10.1007/s11771-020-4285-2
Wright, I. J., Falster, D. S., Pickup, M., and Westoby, M. (2006). Cross-species patterns in the coordination between leaf and stem traits, and their implications for plant hydraulics. Physiol. Plant. 127, 445–456. doi: 10.1111/j.1399-3054.2006.00699.x
Wright, I. J., Westboy, M., and Reich, P. B. (2002). Convergence towards higher leaf mass per area in dry and nutrient-poor habitats has different consequences for leaf life span. J. Ecol. 90, 534–543. doi: 10.1046/j.1365-2745.2002.00689.x
Xiang, S., Wu, N., and Sun, S. (2008). Within-twig biomass allocation in subtropical evergreen broad-leaved species along an altitudinal gradient: allometric scaling analysis. Trees 23, 637–647. doi: 10.1007/s00468-008-0308-6
Xie, J., Wanze, Z., Zhou, P., Zhao, G., and Wang, Y. Y. (2014). Accumulation and biological cycling of nutrient elements in Quercus aquifolioides shrublands. Acta Bot. Boreali Occident. Sin. 34:6. doi: 10.7606/j.issn.1000-4025.2014.05.1032
Zhang, L., Luo, T., Liu, X., and Wang, Y. (2012). Altitudinal variation in leaf construction cost and energy content of Bergenia purpurascens. Acta Oecol. 43, 72–79. doi: 10.1016/j.actao.2012.05.011
Zhang, L., Khamphilavong, K., Zhu, H., Li, H., He, X., Shen, X., et al. (2021). Allometric scaling relationships of Larix potaninii subsp. chinensis traits across topographical gradients. Ecological Indicators 125:107492. doi: 10.1016/j.ecolind.2021.107492
Zhou, H., Xu, M., Pan, H., and Yu, X. (2015). Leaf-age effects on temperature responses of photosynthesis and respiration of an alpine oak, Quercus aquifolioides, in southwestern China. Tree Physiol. 35, 1236–1248. doi: 10.1093/treephys/tpv101
Zhu, G., Niklas, K. J., Li, M., Sun, J., Lyu, M., Chen, X., et al. (2019). Diminishing Returns” in the scaling between leaf area and Twig Size in three forest communities along an elevation gradient of Wuyi Mountain, China. Forests 10:1138. doi: 10.3390/f10121138
Zhu, W. Z., Cao, M., Wang, S. G., Xiao, W. F., and Li, M. H. (2012). Seasonal dynamics of mobile carbon supply in Quercus aquifolioides at the upper elevational limit. PLoS One 7:e34213. doi: 10.1371/journal.pone.0034213
Zhu, W. Z., Wang, S. G., and Hao, Y. Q. (2010). Dynamics of nutrient supply to sprouts from the roots and soil during sprouting of Quercus aquifoliodes shrublands, western Sichuan, China. Chin. J. Plant Ecol. 34:10. doi: 10.3773/j.issn.1005-264x.2010.10.007
Keywords: leaf traits, current-year twigs, altitude gradients, Quercus aquifolioides, Tibet
Citation: Zhang X, Wang C and Zhou C (2022) The Variation of Functional Traits in Leaves and Current-Year Twigs of Quercus aquifolioides Along an Altitudinal Gradient in Southeastern Tibet. Front. Ecol. Evol. 10:855547. doi: 10.3389/fevo.2022.855547
Received: 15 January 2022; Accepted: 13 April 2022;
Published: 03 May 2022.
Edited by:
Jun Zhou, University of Massachusetts Lowell, United StatesReviewed by:
Dongmei Yang, Zhejiang Normal University, ChinaCopyright © 2022 Zhang, Wang and Zhou. This is an open-access article distributed under the terms of the Creative Commons Attribution License (CC BY). The use, distribution or reproduction in other forums is permitted, provided the original author(s) and the copyright owner(s) are credited and that the original publication in this journal is cited, in accordance with accepted academic practice. No use, distribution or reproduction is permitted which does not comply with these terms.
*Correspondence: Chenni Zhou, Y2hlbm5pMjAxOEAxMjYuY29t
†These authors have contributed equally to this work and share first authorship
Disclaimer: All claims expressed in this article are solely those of the authors and do not necessarily represent those of their affiliated organizations, or those of the publisher, the editors and the reviewers. Any product that may be evaluated in this article or claim that may be made by its manufacturer is not guaranteed or endorsed by the publisher.
Research integrity at Frontiers
Learn more about the work of our research integrity team to safeguard the quality of each article we publish.