- 1Northeast Institute of Geography and Agroecology, Chinese Academy of Sciences, Changchun, China
- 2University of Chinese Academy of Sciences, Beijing, China
- 3Key Laboratory of Geographical Processes and Ecological Security in Changbai Mountains, Ministry of Education, School of Geographical Sciences, Northeast Normal University, Changchun, China
- 4CAS Key Laboratory of Forest Ecology and Management, Institute of Applied Ecology, Chinese Academy of Sciences, Shenyang, China
Sustaining ecosystem services in alpine regions is a pressing global challenge given future accelerating environmental changes. Understanding how future climate change and land use/cover change (LUCC) drive ecosystem service will be important in this challenge. However, few studies have considered the combined effects of future climate change and LUCC on ecosystem services. We assessed water yield and soil retention services and their drivers in the Changbai mountains region (CBMR) from the 2020 to 2050s using the Integrated Valuation of Ecosystem Services and Trade-offs (InVEST) model and factor control experiments. Water yield decreased by 2.80% and soil retention increased by 6.14% over the 30 years. Climate change decreased water yield and increased soil retention, while LUCC decreased both water yield and soil retention. The interactive effects between climate change and LUCC had relatively small inhibitory effects on water yield and large facilitation effects on soil retention. Changes in water yield were mainly attributed to climate change, while soil retention was largely influenced by interaction. Our study highlights the individual and interactive contributions of future climate change and land use to ecosystem service in the mountains region, which can provide important information for informed future land management and policy making for sustaining diverse ecosystem services.
Introduction
Ecosystem services are benefits that people derive from natural ecosystems, and they act as an important bridge between natural ecosystems and human well-being (Steffen et al., 2015; Gomes et al., 2021). Since the twentieth century, the warming climate has triggered extreme weather, and population expansion and food security have led to urban and cropland expansion. These changes have seriously affected ecosystem sustainability, and further led to the degradation and loss of ecosystem services (Buitenwerf et al., 2018). Therefore, a greater understanding of future global change trajectories and their effects on ecosystem services provided an important basis for future ecosystem management and decision-making.
Climate change and land use are direct drivers of many ecosystem services and can affect ecosystem distribution and thus determine ecosystem services supply (Kuglerová et al., 2020). Climate change also increases the frequency and severity of extreme events, such as high-winds and extreme temperature fluctuations than can alter ecosystem biophysical processes, and therefore ecosystem services. These events are expected to become even more serious threat in the next decade (Fezzi et al., 2015; Daneshi et al., 2021). Land use/cover change (LUCC) by human-induced directly affects ecosystem composition and configuration and ultimately their ability to provide ecosystem services (Fu et al., 2017; Augustynczik and Yousefpour, 2021). For instance, in alpine regions LUCC significantly affects ecosystem services through changing carbon balance and water flow (Lawler et al., 2014). Spatial changes in land use also have a significant impact on the future provision and location of ecosystem services (Ricke et al., 2018). Many studies have used LUCC as a proxy or visual representation of ecosystem service change to convey potential future development and assess environmental change (Ladouceur et al., 2021; Zambon et al., 2021). Numerous modeling studies on changes in ecosystem services and their contributing factors have been conducted, but typically they have focused on the independent effects of climate change or LUCC. Few studies have considered the combined impacts of climate and land use change on ecosystem services under future climate change conditions (Runting et al., 2017; Guo et al., 2020).
Mountains cover 22% of the earth’s surface, are home to 915 million people, and provide a range of important ecosystem services, such as timber, water supply, and wildlife habitat (Romeo et al., 2015). Mountains experience higher rates of temperature rise than lowlands, therefore they are more sensitive to the effects of climate change, and they are also very susceptible to land use changes impacts due to their high elevation and slope, which in turn affect ecosystem function and services (Kim et al., 2017). Thus, more assessment and monitoring of ecosystem services in mountain areas are needed to maintain ecosystem health under increasing environmental change (Egan and Price, 2017; Bai et al., 2019). The Changbai mountains region (CBMR) is a dormant active volcano and provides a variety of ecological services to the region, such as water provision, habitat, tourism, and carbon sink. It is also the source of the Songhua River, the largest river in Northeast, and precipitation runoff from the mountains also provides important recharge for other local rivers (e.g., Yalu River and Heilongjiang River) (Song et al., 2020). However, the CBMR environment has experienced unprecedented climate change (warming and drying) and human disturbances (e.g., urbanization, reclamation, deforestation), which have profoundly altered ecosystem structure and function. Historical climate data and projections indicate that warming and drying of the CBMR region will become more pronounced in the future and will be accompanied by more frequent extreme weather events (Zhang et al., 2021). Additionally, tourism development and food security-induced urban expansion have changed the natural trajectory of the LUCC, creating a series of land and environmental problems such as land degradation and water pollution (Wang et al., 2021).
To achieve a balance between regional development and environmental protection in the CBMR, the Chinese government implemented a series of measures to improve the fragmented ecological environment [e.g., establishment of a National Nature Reserve in (1986) (Guo et al., 2014)], and then enacted several ecological restoration projects [e.g., Grain for Green Project in (1999) (Cao et al., 2009) and Natural Forest Protection Project in (2000) (Wei et al., 2014)]. Recent studies have shown that these land use decisions significantly enhanced the CBMR carbon sink, and reduced wind-sand erosion (Mao et al., 2019). Some studies also found increased woodlands cover reduced surface runoff, which in turn affected downstream water use patterns (Feng et al., 2022). However, these studies generally focused on past-to-present changes in ecosystem services, or the effects of ecological projects on a particular ecosystem service, and do not provide a comprehensive assessment on the combined impacts of climate change and land use decisions on ecosystem services under an uncertain future. Thus, a deeper understanding of how climate change and policy-driven LUCC affect ecosystem services and quantifying these drivers can inform future land use decisions and natural resource management.
We focus on two types ecosystem services in CBMR: water yield and soil retention services, the quality of them is an important criterion for assessing the ecological health of alpine regions. CBMR has high vegetation cover, requires considerable water for growth, and is highly susceptible to soil erosion from abundant summer precipitation and steep slope. To better understanding the combine effects of future climate change and LUCC on ecosystem services. We first simulated climate change and LUCC for CBMR in 2050, and then used the InVEST model to assess water yield and soil retention under future climate and land use scenarios. We address the following questions in the CBMR: (1) how do climate and land use change under high emission scenarios SSP 8.5 and environmental protection policies, (2) how do ecosystem services change spatially and temporally, and (3) what are the individual and interactive effects of future climate and land use changes on water yield and soil retention?
Materials and Methods
Study Area
Changbai mountain region (CBMR) is located in Northeast China (126.49°E to 129.64°E, 41.18°N to 43.70°N). It includes Helong, Linjiang, Fusong, Antu, and Changbai Counties and is dominated by alpine terrain, with the highest peak in Northeast China, Baiyun Peak (2, 666 m above sea level). The CBMR land area is approximately 2.42 × 104 km2, accounting for 13% of Jilin Province, China (Figure 1). CBMR is characterized by a typical temperate continental monsoonal climate with hot and rainy summers, and cold and dry winters. Over the past 30 years, the average annual temperature was 4.38°C, and the average annual precipitation was 742 mm (Wang et al., 2020).
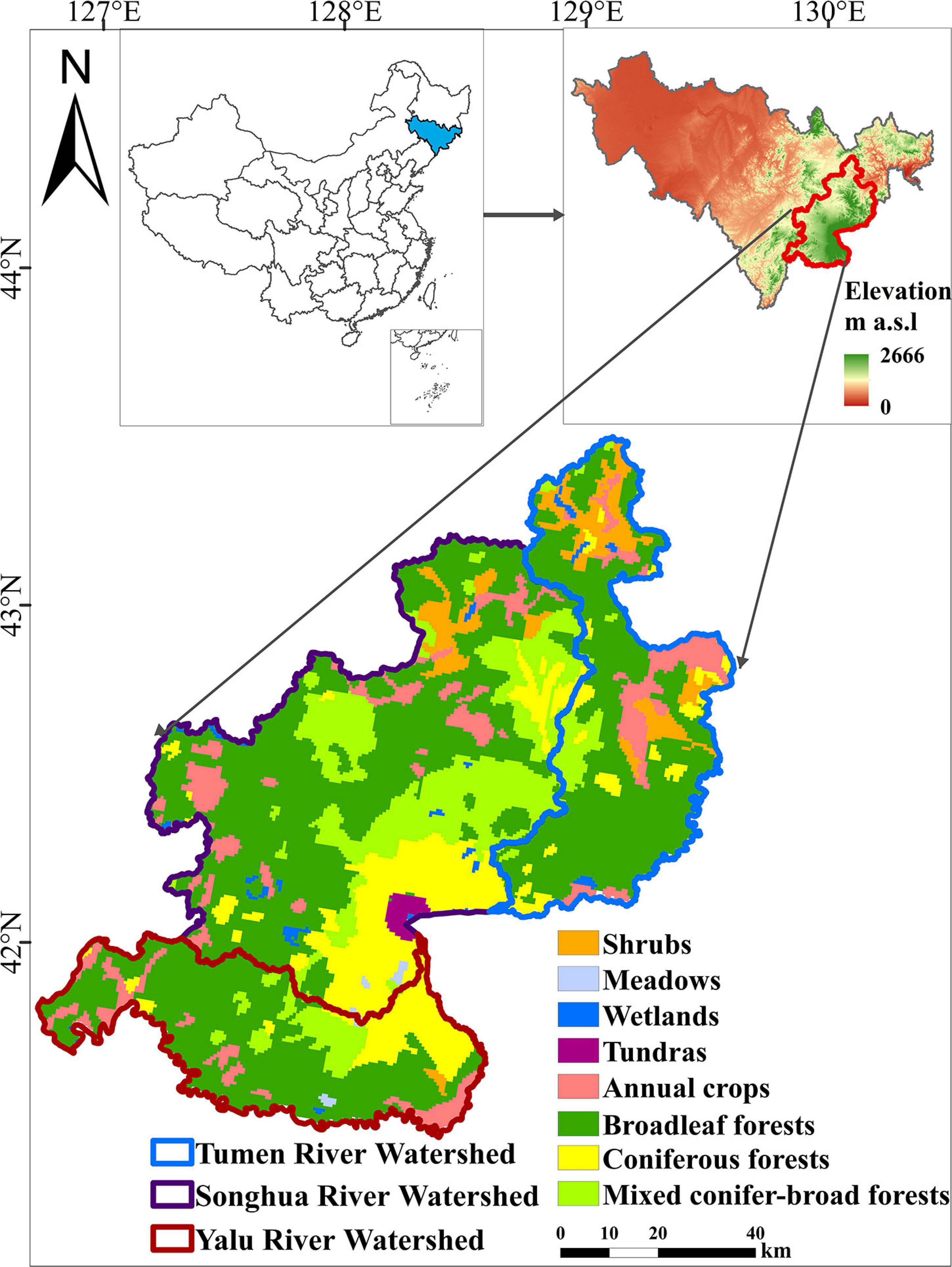
Figure 1. Location, digital elevation model (DEM) and vegetation types (2010) of the Changbai mountains region (CBMR).
Changbai mountain region can be divided into three watersheds based on terrain and catchment area (Figure 1). The Songhua River Watershed (SRW) is the largest (about 53.52%), and its main vegetation types are broadleaf, mixed conifer-broadleaf, and coniferous forests. The Yalu River Watershed (YRW) is the smallest (about 24.54%), and its main vegetation types are broadleaf and coniferous forests. The main vegetation types in the Tumen River basin (TRW) are broadleaf forests and annual cropland (Figure 1).
Current Climate and Land Use Data
The meteorological data were obtained from the China Meteorological Data Sharing Service,1 and included daily temperature, precipitation, and solar radiation for 2016–2020 at 1 km resolution. Land use data at 30 m resolution for 2020 was obtained from the National Earth System Science Data Center.2 Digital elevation model (DEM) data were obtained from the U.S. Geological Survey3 at 90 m resolution.
Future Climate and Land Use Change Scenarios
We simulated future climate and land use scenarios for the 2050s (2045–2050). Since the high emission scenario (SSP 8.5) assumes a business-as-usual-high-end emissions scenario by the end of the century (Jacob et al., 2014), we used it to assess future climate change effects. The CLUE-S model was used to determine future land use based on historical trends, in which ecological protection policy was the main driving factor.
Future Climate Data
Future climates for the period 2045–2050 were obtained from the Coupled Model Intercomparison Project (Phase 6).4 We assembled daily precipitation, minimum/maximum temperature, and radiation data according to predictions of the CESM1-BGC, CCSM4, CNRM-CM5, FGOALS-g2, MIROC5, and MRI-CGCM3 global models under the SSP 8.5 scenario, and chosen these six models because they have been validated to have good applicability in the Changbai Mountains (Wang et al., 2019). Then, we regionalized it to 1 km resolution for CBMR using the WFR-ARW regional dynamical model.
Land Use/Cover Change Projections
The CLUE-S (The Conversion of Land Use and its Effects at Small Region Extent) model was used to simulate land use/cover in 2050. CLUE-S is a spatially explicit land use model that simulates dynamic competition between different land use types. It is based on high-resolution (usually better than 1 km) spatial image data and is suitable for simulating land use change at small and medium spatial scales. The model includes spatial and non-spatial modules. The non-spatial module calculates land use demand based on the analysis of natural, social and economic factors. The spatial module uses a raster system to translate these demands into land use changes based on probabilities and rules for different land use types (Verburg et al., 2002).
The model requires three main types of input data: location suitability, spatial policies and restrictions, and conversion settings. First, location suitability was determined by quantifying the relationship between land use/cover patterns and constraints (Land Management Policies) through logistic regression (Verburg et al., 2002). Secondly, spatial constraints were used to limit land use/cover variables by setting woodlands and wetlands as environmental constraints. Finally, conversion settings were determined by a land use conversion matrix of past time series. A detailed description of CLUE-S can be found in Verburg and Overmars (2009).
We validated model accuracy by comparing simulation results for 2000, 2010, and 2020 with satellite remote sensing data. The Kappa index was used to assess the consistency between the simulation results and remote sensing data (Vliet et al., 2011). The Kappa index was 0.8554, 0.8827, and 0.8814 in 2000, 2010, and 2020, respectively, which demonstrated that the CLUE-S simulation results were reliable, selected indexes and parameters conformed to requirements, and future simulation results were in accordance with development trends and had practical significance.
Integrated Valuation of Ecosystem Services and Trade-Offs Model Parameterization
We used the Water Yield module and the Sediment Delivery Ratio module of the InVEST model to estimate ecosystem services under current and future scenarios. The Water Yield module is based on the Budyko curve and the Sediment Delivery Ratio module is based on the Revised Universal Soil Loss Equation (RUSLE), which are described in Appendix 1 (Supplementary Material). The InVEST model is mainly based on spatial data (land use data and climate data) and assesses the value of ecosystem services through empirical models. Due to its ease of accessibility and rich functionality, the model has been widely used in ecological and hydrological studies (Luetzenburg et al., 2020; Morán-Ordóez et al., 2021). All models were parameterized and tested at the CBMR (Xiao et al., 2002; Yu et al., 2018; Li et al., 2019). Table 1 briefly describes the data sets used to assess the two ecosystem services. Details of the model parameterization can be found in Appendix 2 (Supplementary Material). The precipitation, temperature, and reference evapotranspiration datasets were spatially interpolated for all sites using the ArcGIS 10.3 platform with a spatial resolution of 1,000 m. A detailed description of the InVEST model can be found in Sharp et al. (2018).
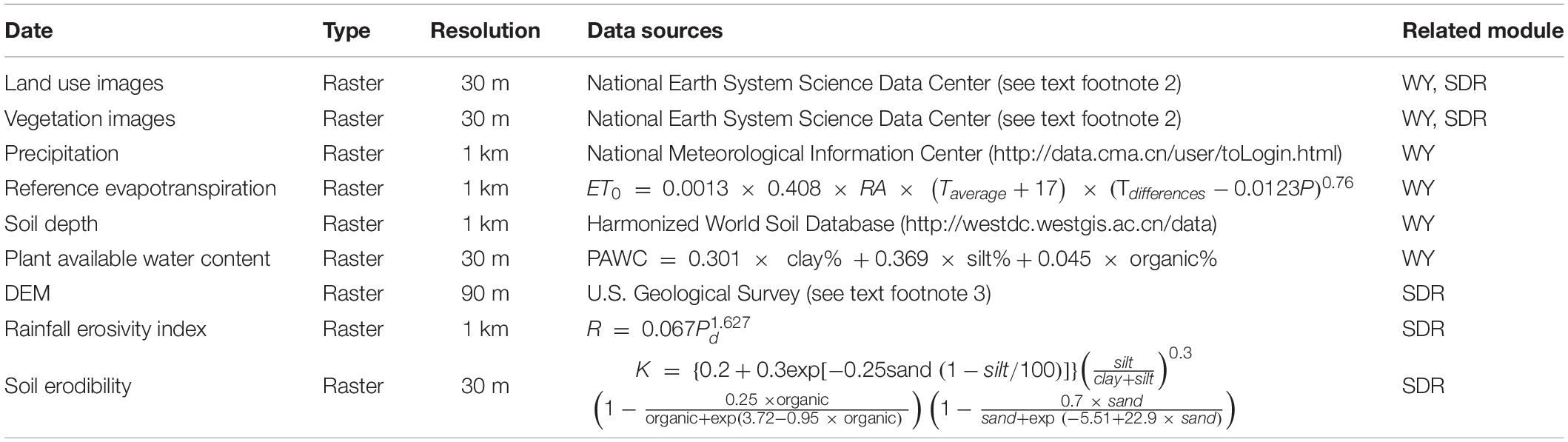
Table 1. Data requirement for the integrated valuation of ecosystem services and trade-offs (InVEST) model (WY, Water Yield module; SDR, Sediment Delivery Ratio module).
Experimental Design
We designed a factor control analysis through controlling two influential factors of climate and land use to assess climate change, LUCC and their interactive effects on ecosystem services. We generated four simulation scenarios by integrating climate and land use scenarios at 2020s (2016–2020) and 2050s (2045–2050) (Table 2). Then we used the InVEST model to assess water yield and soil retention under the four simulated scenarios.
We determined the magnitude of effects of climate change and land use by the difference between the baseline scenario (Scenario 1) and the climate change only (Scenario 3) or LUCC only (Scenario 2) scenarios. We determine the combined climate and land use effects by the difference between the 2050s simulation scenario (Scenario 4) and the baseline scenario (Scenario 1), and then determined the interactive effects by subtracting the individual effects of climate change and LUCC from the combined effects.
Results
Future Climate and Land Use Change
The CBMR climate became warmer and drier from the 2020 to 2050s, with 92% of the area warming, and about 56% showing a drying trend. Under the high emissions scenario, annual mean temperature increased from 5.25°C in the 2020s to 5.57°C in the 2050s, an increase of 6.10%, with the most pronounced warming in the SRW. A decreasing annual precipitation trend was significant, lowering from 773 mm in the 2020s to 691 mm in the 2050s, a decrease of 10.65%. The decrease occurred mainly in TRW and SRW (Figures 2A,B).
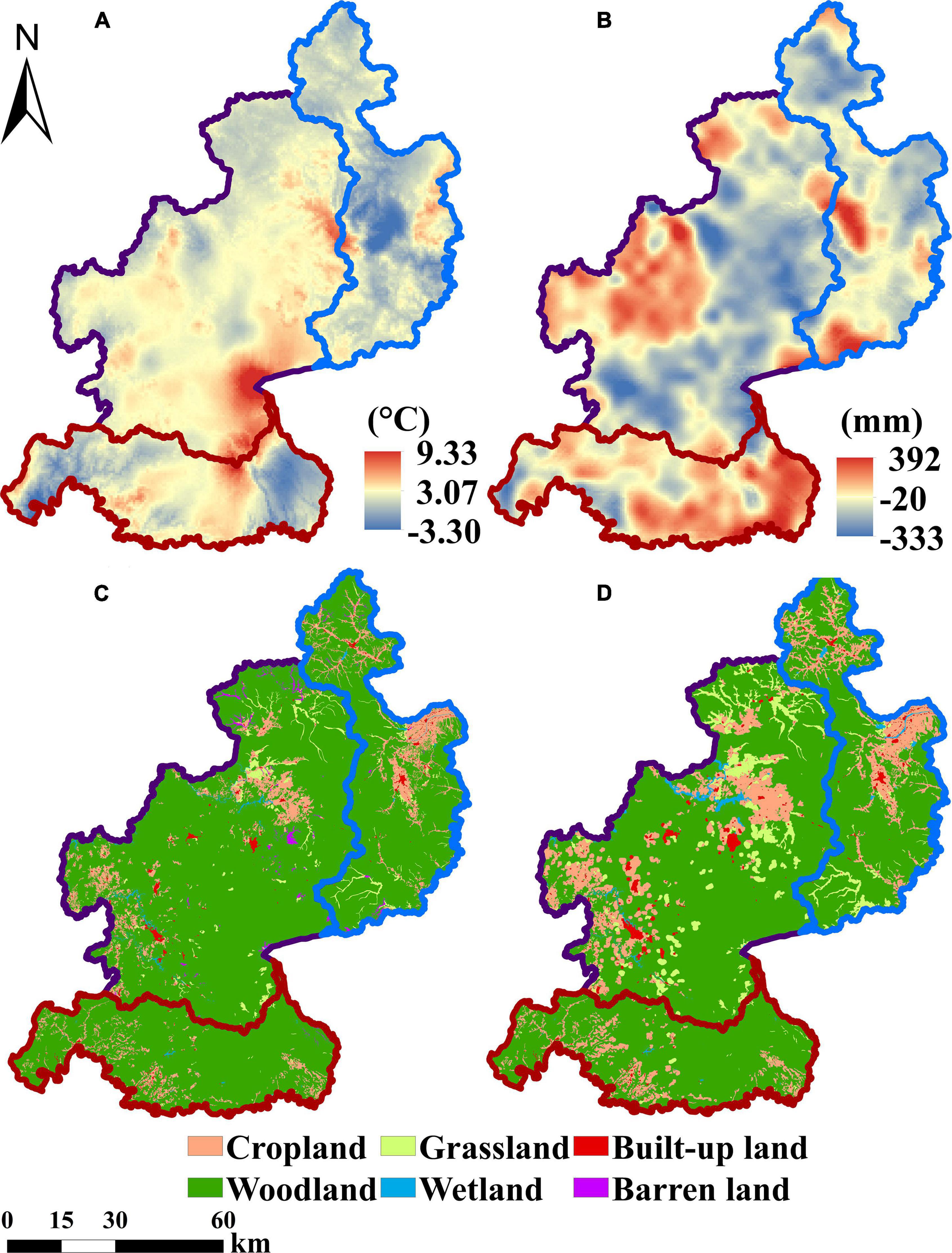
Figure 2. Spatial changes in annual average temperature (A) and annual precipitation (B) and during 2020–2050s, spatial distributions of land use for year 2020 (C) and year 2050 (D).
Although the limits of environmental protection constraints such as the Natural Forest Protection Project in (2000) (Wei et al., 2014) and the Grain for Green Project in (1999) (Cao et al., 2009) were considered in the future land use simulation, significant changes occurred in the CBMR due to human disturbances. Woodlands, the dominant land cover type would decrease by 7.79% (1.87 × 103 km2) and be converted mainly to cropland and grassland, with a significant decrease in SRW. Croplands, converted from woodland and barren land, would increase the most at 4.10% (0.98 × 103 km2), mainly in SRW and YRW. Grasslands, built-lands and wetlands (natural and artificial) experienced varying degrees of increase, with 2.64% (0.64 × 103 km2), 0.69% (0.16 × 103 km2) and 0.63% (0.15 × 103 km2), respectively (Figures 2C,D).
Ecosystem Service Changes
The CBMR showed a slight decreasing trend in water yield, from 617 mm/km2 in the 2020s to 600 mm/km2 in the 2050s, a decrease of 2.80%. The most pronounced decrease was in the eastern mountains, while the southern mountains showed a slight increase (Figures 3, 4). Sub-regionally, TRW and SRW showed decreases in water yield (due to decreased precipitation), of 29 mm/km2 (5.50%) and 37 mm/km2 (5.91%) respectively, while YRW showed an increase, of 38 mm/km2 (5.53%) (Figures 3, 4).
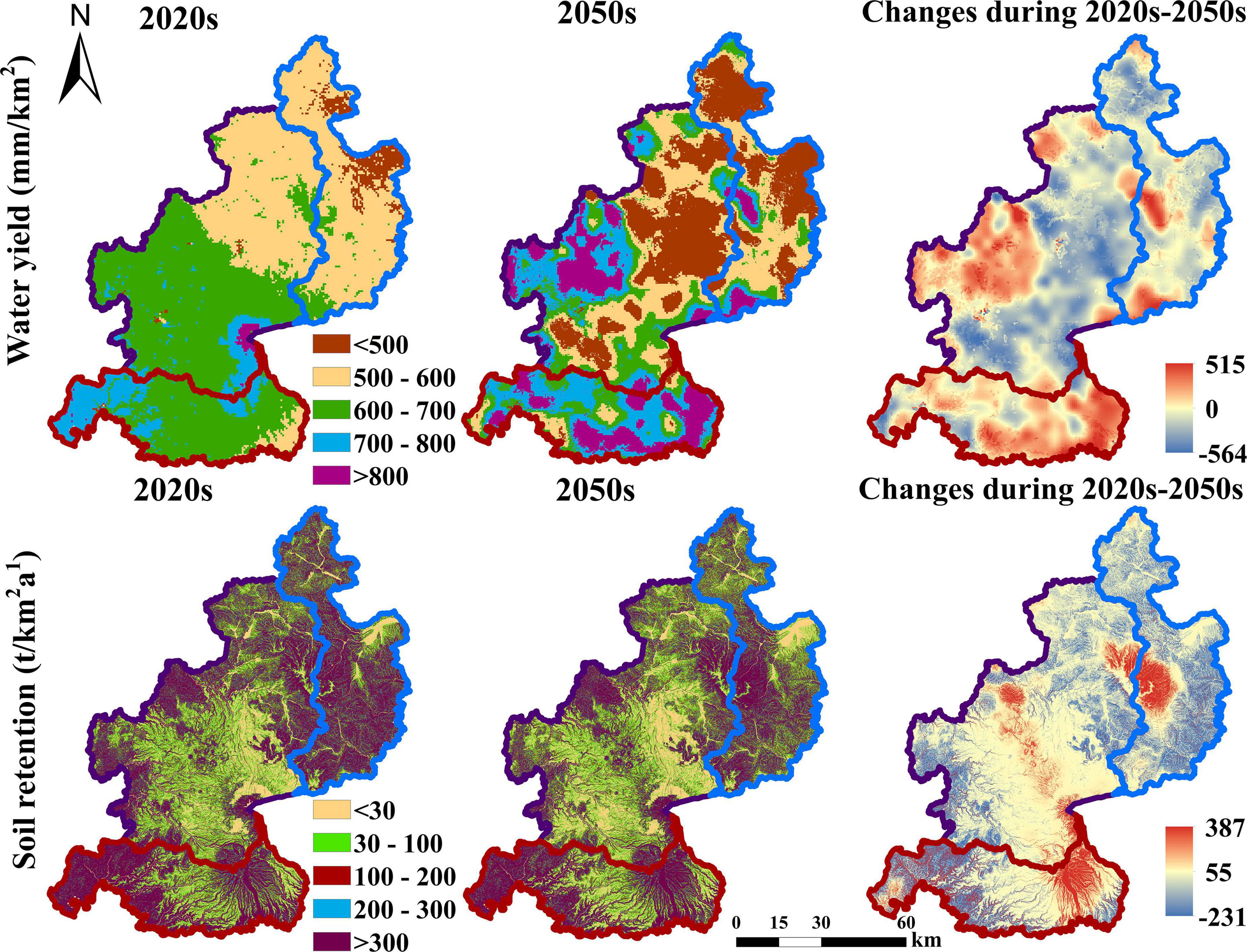
Figure 3. Spatial distributions of water yield and soil retention in 2020 and 2050s and changes during 2020–2050s.
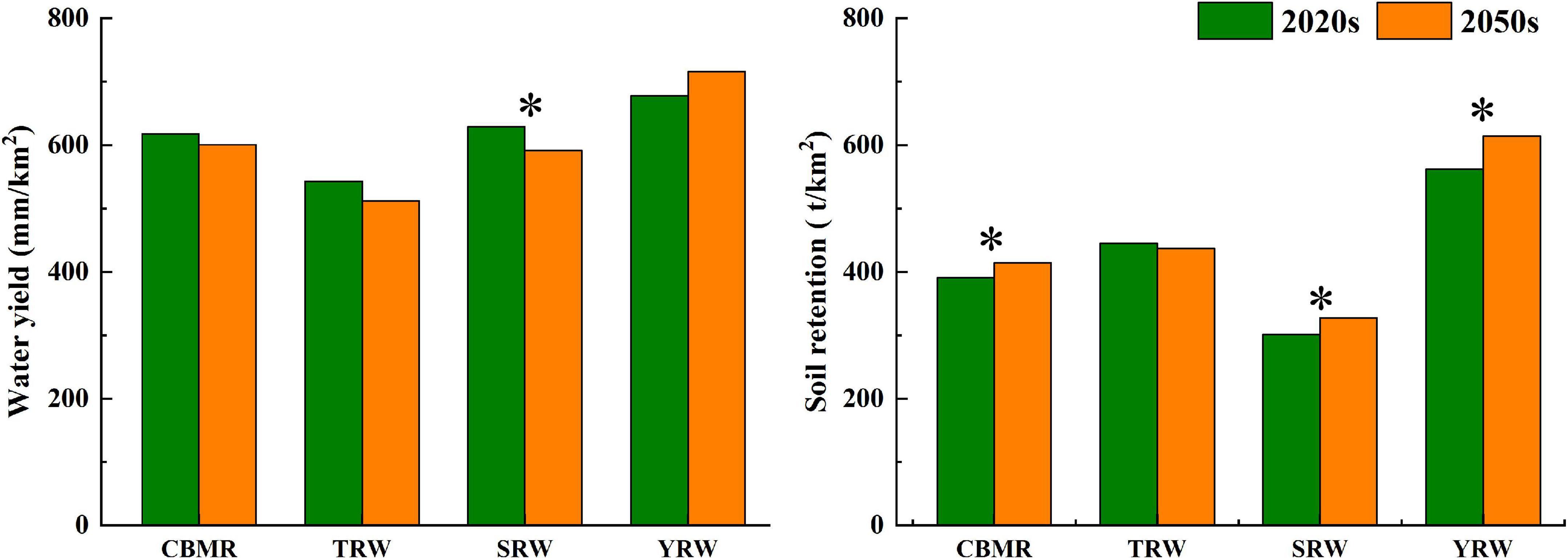
Figure 4. Water yield and soil retention in 2020 and 2050s for the Changbai mountains region (CBMR) and three watersheds (the sign “*” indicated significant differences at the 0.05 level).
Soil retention in the CBMR significantly increased (due to the decreased precipitation and interactive effects), from 390 t/km2 in the 2020s to 414 t/km2 in the 2050s, an increase of 6.14%. The increases were mainly in the eastern mountains, while decreases occurred in the northern hills (Figures 3, 4). The largest increase occurred in the YRW, of 42.98 t/km2 (7.66%), followed by SRW, of 26.08 t/km2 (8.66%). In the TRW there was a significant decrease, of 8.23 t/km2 (1.85%) (Figures 3, 4).
Effects of Climate and Land Use
Climate change was the dominant factor affecting water yield changes in the CBMR, followed by interactive effects and LUCC. Interactive effects were the most significant driving factor for soil retention changes, followed by climate change and LUCC.
Individual Effects of Climate and Land Use
Climate change decreased water yield by 9.60 mm/km2 for the whole region (1.55%) from the 2020s to 2050s, with most pronounced effects in the southern mountainous area (Figures 5A, 6). Climate change decreased water yield by 3.50 and 3.63% in the TRW and the SRW, respectively, and increased water yield by 4.10% in the YRW. Climate change increased soil retention in the CBMR by 11.17 t/km2 (2.86%) from the 2020 to 2050s, mainly in the northern hills (Figures 5B, 6). Climate change increased soil retention in TRW and SRW by 1.85 and 6.71%, respectively, while in the YRW decreased by 1.08%.
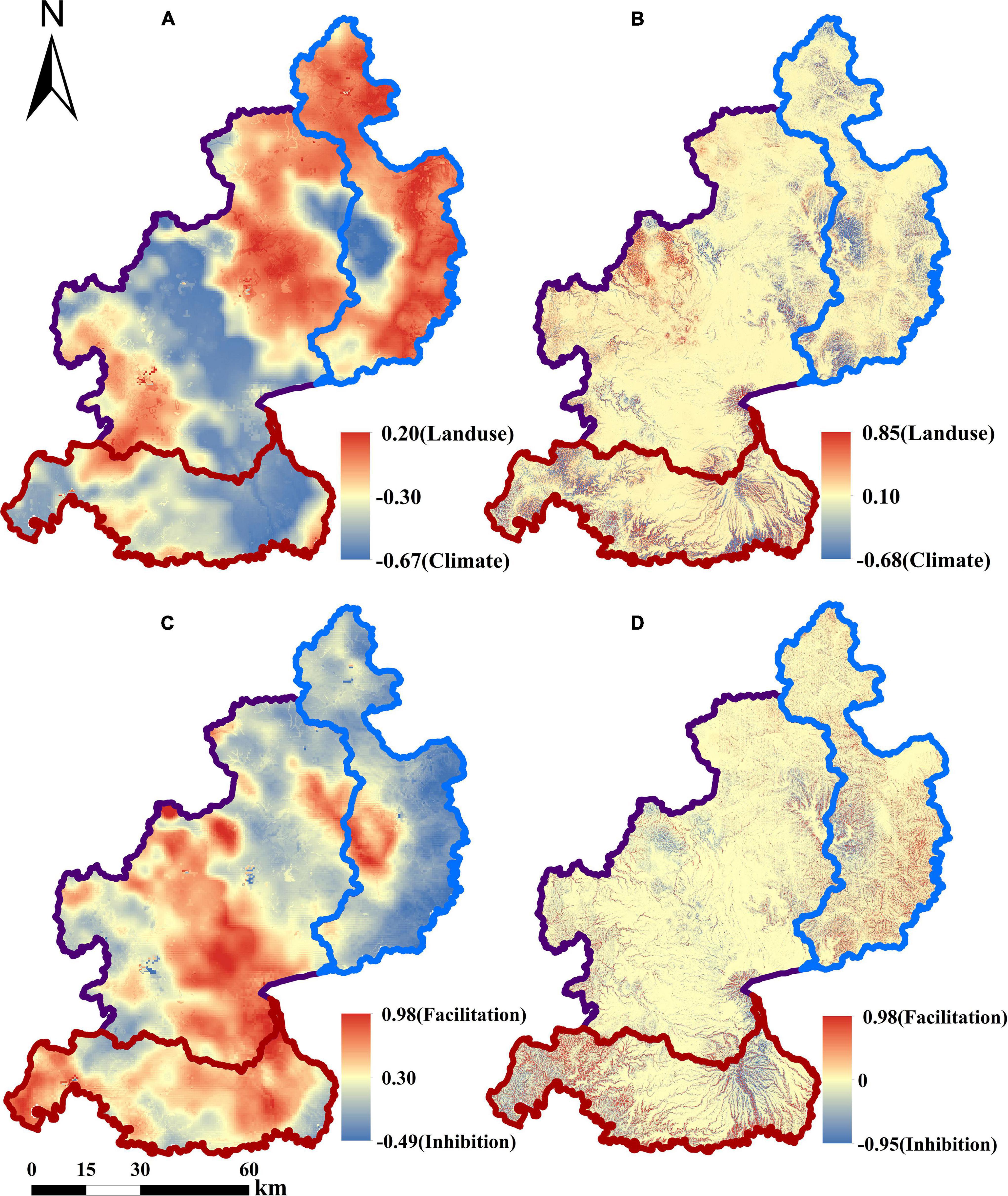
Figure 5. Spatial distributions of the individual effects (A: Water yield; B: Soil retention) and interactive effects (C: Water yield; D: Soil retention) of LUCC and climate change.
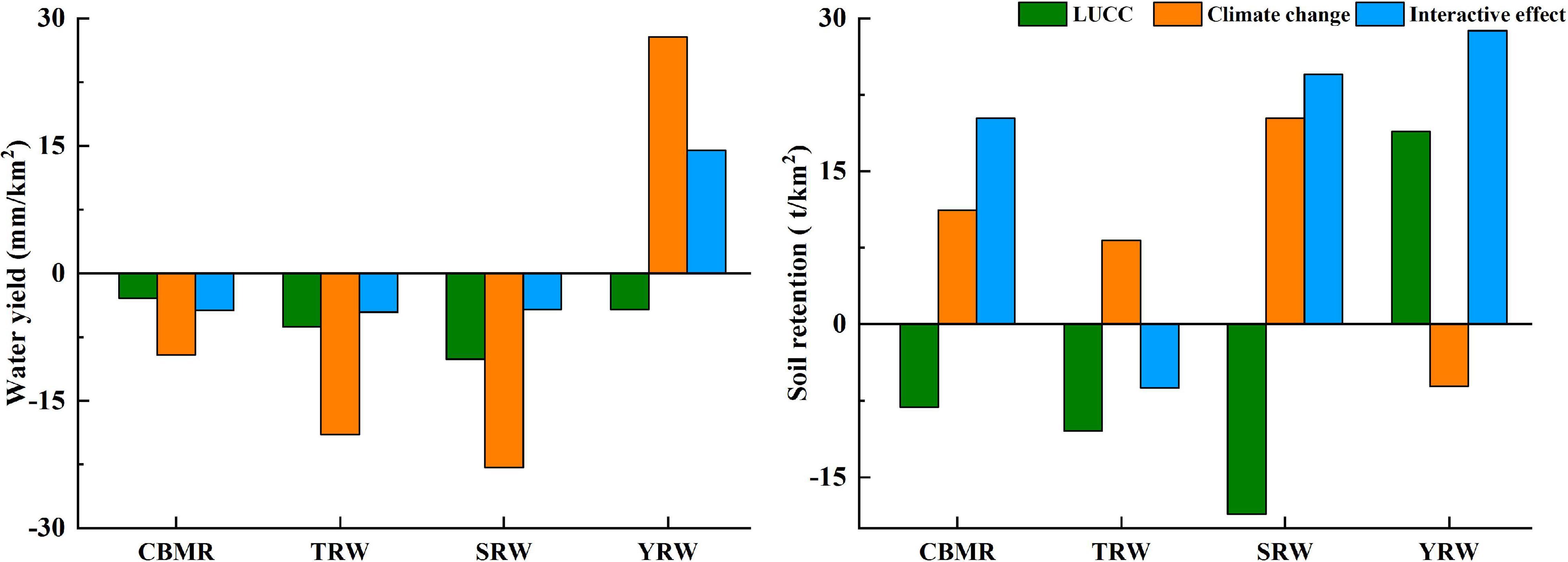
Figure 6. The effect sizes of climate change, land use/cover change (LUCC) and their interaction on changes in water yield and soil retention during 2020–2050s for the Changbai mountains region (CBMR) and three watersheds.
Land use decreased water yield in the CBMR by 2.90 mm/km2 (0.50%) from the 2020 to 2050s, mainly in the northern hills (Figures 5A, 6). Land use had negative effects on water yield for all three watersheds, decreasing by 1.16, 1.60, and 0.63% in the TRW, SRW, and YRW, respectively. Land use also decreased soil retention in the CBMR by 8.14 t/km2 (2.08%), mainly in the southern mountainous region (Figures 5B, 6). Land use effects decreased soil retention by 2.35 and 6.18% in the TRW and SRW, respectively, while increasing it by 3.36% in the YRW.
Interactive Effects
Interactive effects between climate change and LUCC had significant impacts on CBMR future ecosystem services, with inhibitory effects on water yield and facilitation effects on soil retention. Interactive effects decreased water yield by 4.36 mm/km2 (0.71%) from the 2020 to 2050s in the CBMR, with the most pronounced inhibitory effects occurring in the southern and eastern mountainous region (Figures 5C, 6). Interactive effects increased soil retention by 20.22 t/km2 (5.17%) from the 2020 to 2050s in the CBMR, mainly in the eastern and southern mountainous areas (Figures 5D, 6).
Discussion
Water yield changes were mainly attributed to climate change, while soil retention was largely influenced by interactions. Recent studies noted that precipitation patterns directly determine water yield, especially in mountainous areas, where precipitation is the main recharge (Braun et al., 2019). For example, Yin et al. (2022) found that water yield changes in North China were more sensitive to precipitation than temperature, and the changes demonstrated a trend consistent with the precipitation change from 1995 to 2015. Tirupathi and Shashidhar (2020) found that a 9.5% precipitation increase led to a doubling in water yield during 2010–2040 under the Representative Concentration Pathways scenario 8.5 in the Krishna River basin of India. Meanwhile, increasing temperature can also alter evapotranspiration to exacerbate decreasing water yield (Bai et al., 2020). However, soil retention in mountainous areas was more susceptible to precipitation intensity due to the high altitude and slope effects (Soto et al., 2021). Decreased precipitation can diminish slope erosion and reduce soil scouring by affecting runoff (Qiu and Turner, 2013; O’Connor et al., 2019). Although the CBMR has a temperate monsoonal continental climate, it is significantly influenced by topography and humid oceanic air flow. Precipitation is abundant throughout the year, especially from July to September (Li et al., 2019). Therefore, future warming and drying climates will continue to lead to increased soil retention in the CBMR. This is consistent with southern Europe, where Mediterranean (Ebro), Alpine (Adige), and continental (Sava) basins are expected to experience a 20% increase in soil retention by 2050 due to a 40% decrease in water availability caused by climate change and irrigation (Jorda-Capdevila et al., 2019).
In our future land use simulation (2020–2050s), CBMR land use conversion area reached 18.20% despite the implementation of ecological restoration projects. Some studies have found that land use directly determined vegetation type, which further influenced surface runoff and evapotranspiration (Khorchania et al., 2020). The greatest land use changes predicted in our study were in the TRW and SRW, with a significant shift from woodlands to grasslands and croplands. These changes reduced watershed water yield because woodlands have a greater water storage capacity than other vegetation type (Grimm et al., 2013). Furthermore, some studies have found that the expanded croplands, especially an increase in paddy fields led a greater demand for irrigation, which would directly affect evaporation and runoff, in turn decreasing water yield (Cord et al., 2017). In the TRW and SRW watersheds, future LUCC will similarly decrease soil retention. This is because in most regions, forest canopies have a stronger precipitation interception capacity than grasslands and annual crops, especially in mountainous areas, while the complex root system of woodlands also increases soil stability (Felipe-Lucia et al., 2020). For example, compared to our study, Xia et al. (2021) found that the GFGP project in the Liaohe River basin of China resulted in soil loss of 4.33 × 105–2.94 × 105 t/year from 2007 to 2015. In mountainous areas of Spain, the revegetation of Aragón between the mid-20th and early 21st centuries led to a significant increase in water supply (>1,000%) and soil retention (>400%) (Brunoa et al., 2021).
In the CBMR, climate change interacts with LUCC to significantly impact ecosystem services, especially soil retention on the landscape. Predicted future land use change-induced soil retention primarily occurred in the relatively low elevation of western and northern flanks, which were most vulnerable to climate change. Although woodland loss reduced soil retention, decreases in precipitation-based erodibility interact with the reduction of surface runoff to enhance soil retention (Morán-Ordóez et al., 2020). For water yield, future warmer and drier climates and decreased water holding capacity by ecosystems due to declining woodlands will exacerbate decreasing water yield (Krueger et al., 2019). For example, Clerici et al. (2019) used IPCC-LUCC scenarios to analyze the effects of LUCC and climate change on water provision and carbon storage in Colombian Andes from 2016 to 2046. The results showed that climate change scenarios had a greater impact on water availability than LUCC scenarios, and the interactive between climate change and LUCC significantly reduced the water provision.
It should be noted that there were several limitations in our study. First, our simulations of future climate did not consider the effects of mountain microclimates, which can increase climate uncertainty (Bagstad et al., 2013). Secondly, land use data resolution was 30 m, while the downloaded and simulated climate data was 1 km resolution, which may increase uncertainty of the simulation results (Zhang et al., 2019). However, our simulations predicted the climate and land use conditions in the CBMR over the next 30 years, and quantitatively assessed through factor control experiments the effects of climate and land use individually, interactively and in combination with ecosystem service changes. Our study can provide important information for government land use decisions and natural resource management in response to future environmental changes.
Conclusion
In the CBMR, we assessed water yield, soil retention and the factors controlling them from the 2020 to 2050s using the InVEST model and factor control analyses. Water yield decreased and soil retention increased significantly over the three decades. Climate change decreased water yield and increased soil retention, while LUCC decreased both water yield and soil retention. The interactive effects of climate change and LUCC had relatively small inhibitory effects on water yield and largely facilitated on soil retention. Changes in water yield were mainly attributed to climate change, while soil retention was largely influenced by interactions. Our results highlight the driver impacts of climate and land use on ecosystem services and provide important information for governments to develop land-use and ecological management policies to tackle future environmental changes.
Data Availability Statement
The original contributions presented in the study are included in the article/Supplementary Material, further inquiries can be directed to the corresponding author.
Author Contributions
HW and WW: conceptualization, writing, and editing. HW, WW, and ZL: methodology. HW, LW, SM, YZ, WZ, and MJ: data curation and analysis. All authors contributed to the article and approved the submitted version.
Funding
This study was jointly funded by the Development and Application of National Key Research and Development Plan (2019YFC0409102), the National Natural Science Foundation of China (U19A2023, 41871045, 41922006, and 41801081), the Chinese Academy of Sciences (Y7H7031001), the Natural Science Foundation of Jilin Province, China (20200201047JC), and the Key Laboratory of Geographical Processes and Ecological Security in Changbai Mountains, Ministry of Education.
Conflict of Interest
The authors declare that the research was conducted in the absence of any commercial or financial relationships that could be construed as a potential conflict of interest.
Publisher’s Note
All claims expressed in this article are solely those of the authors and do not necessarily represent those of their affiliated organizations, or those of the publisher, the editors and the reviewers. Any product that may be evaluated in this article, or claim that may be made by its manufacturer, is not guaranteed or endorsed by the publisher.
Supplementary Material
The Supplementary Material for this article can be found online at: https://www.frontiersin.org/articles/10.3389/fevo.2022.854497/full#supplementary-material
Footnotes
- ^ http://data.cma.cn/
- ^ http://www.geodata.cn/
- ^ http://www.usgs.gov/
- ^ https://esgf-node.llnl.gov/projects/cmip6/
References
Augustynczik, A., and Yousefpour, R. (2021). Assessing the synergistic value of ecosystem services in European beech forests. Ecosyst. Serv. 49:101264. doi: 10.1016/j.ecoser.2021.101264
Bagstad, K. J., Semmens, D. J., Waage, S., and Winthrop, R. (2013). A comparative assessment of decision-support tools for ecosystem services quantification and valuation. Ecosyst. Serv. 5, 27–39. doi: 10.1016/j.ecoser.2013.07.004
Bai, P., Liu, X., Zhang, Y. Q., and Liu, C. M. (2020). Assessing the impacts of vegetation greenness change on evapotranspiration and water yield in China. Water Resour. Res. 56:e2019WR027019. doi: 10.1029/2019WR027019
Bai, Y., Ochuodho, T. O., and Yang, J. (2019). Impact of land use and climate change on water-related ecosystem services in Kentucky, USA. Ecol. Indic. 102, 51–64. doi: 10.1016/j.ecolind.2019.01.079
Braun, D., Jong, R., Schaepman, M. E., Furrer, R., Hein, L., Kienast, F., et al. (2019). Ecosystem service change caused by climatological and non-climatological drivers: a Swiss case study. Ecol. Applic. 29:e01901. doi: 10.1002/eap.1901
Brunoa, D., Sorandoab, R., Álvarez-Farizoa, B., Castellanoa, C., Céspedes, V., Gallardo, B., et al. (2021). Depopulation impacts on ecosystem services in Mediterranean rural areas. Ecosyst. Serv. 52:101369. doi: 10.1016/j.ecoser.2021.101369
Buitenwerf, R., Sandel, B., Normand, S., Mimet, A., and Svenning, J. C. (2018). Land surface greening suggests vigorous woody regrowth throughout European semi-natural vegetation. Glob. Change Biol. 24, 5789–5801. doi: 10.1111/gcb.14451
Cao, S. X., Chen, L., and Liu, Z. D. (2009). An investigation of Chinese attitudes toward the environment: case study using the Grain for Green Project. Ambio 38, 55–64. doi: 10.1579/0044-7447-38.1.55
Clerici, N., Cote-Navarro, F., Escobedo, F. J., Rubiano, K., and Villegas, J. C. (2019). Spatio-temporal and cumulative effects of land use-land cover and climate change on two ecosystem services in the Colombian Andes. Sci. Total Environ. 685, 1181–1192. doi: 10.1016/j.scitotenv.2019.06.275
Cord, A. F., Brauman, K. A., Chaplin-Kramer, R., Huth, A., Ziv, G., and Seppelt, R. (2017). Priorities to advance monitoring of ecosystem services using earth observation. Trends Ecol. Evol. 32, 416–428. doi: 10.1016/j.tree.2017.03.003
Daneshi, A., Brouwer, R., Najafinejad, A., Panahi, M., and Maghsood, F. F. (2021). Modelling the impacts of climate and land use change on water security in a semi-arid forested watershed using InVEST. J. Hydrol. 593:125621. doi: 10.1016/j.jhydrol.2020.125621
Egan, P., and Price, M. F. (2017). Mountain Ecosystem Services and Climate Change. Paris: The United Nations Educational, Scientific and Cultural Organization.
Felipe-Lucia, M. R., Soliveres, S., Penone, C., Fischer, M., Ammer, C., Boch, S., et al. (2020). Land-use intensity alters networks between biodiversity, ecosystem functions, and services. Proc. Natl. Acad. Sci. U.S.A. 117, 28140–28149. doi: 10.1073/pnas.2016210117
Feng, M. M., Zhang, W. G., Zhang, S. Q., Sun, Z. Y., Li, Y., Huang, Y. Q., et al. (2022). The role of snowmelt discharge to runoff of an alpine watershed: evidence from water stable isotopes. J. Hydrol. 604:127209. doi: 10.1016/j.jhydrol.2021.127209
Fezzi, C., Harwood, A. R., Lovett, A. A., and Bateman, I. J. (2015). Erratum: the environmental impact of climate change adaptation on land use and water quality. Nat. Climate Change 5, 255–260. doi: 10.1038/nclimate2585
Fu, Q., Li, B., Hou, Y., Bi, X., and Zhang, X. S. (2017). Effects of land use and climate change on ecosystem services in Central Asia’s arid regions: a case study in Altay Prefecture, China. Sci. Total Environ. 607-608, 633–646. doi: 10.1016/j.scitotenv.2017.06.241
Gomes, E., Inácio, M., Bogdzevi, K., Kalinauskas, M., Karnauskaitë, D., and Pereira, P. (2021). Future land use changes and its impacts on terrestrial ecosystem services: a review. Sci. Total Environ. 781:147716. doi: 10.1016/j.scitotenv.2021.146716
Grimm, N. B., Chapin, F. S., Bierwagen, B., Gonzalez, P., Groffman, P. M., Luo, Y., et al. (2013). The impacts of climate change on ecosystem structure and function. Front. Ecol. Environ. 11:474–482. doi: 10.1890/120282
Guo, X. Y., Zhang, H. Y., Wang, Y. Q., and Clark, J. (2014). Mapping and assessing typhoon-induced forest disturbance in Changbai mountain national nature reserve using time series landsat imagery. J. Mt. Sci. 12, 404–406. doi: 10.1007/s11629-014-3206-y
Guo, Y. H., Fang, G. H., Xu, Y. P., Tian, X., and Xie, J. K. (2020). Identifying how future climate and land use/cover changes impact streamflow in Xinanjiang Basin, East China. Sci. Total Environ. 710:136275. doi: 10.1016/j.scitotenv.2019.136275
Jacob, D., Petersen, J., Eggert, B., Alias, A., Christensen, O. B., Bouwer, L. M., et al. (2014). EURO-CORDEX: new high-resolution climate change projections for European impact research. Reg. Environ. Change 14, 563–578. doi: 10.1007/s10113-013-0499-2
Jorda-Capdevila, D., Gampe, D., García, V. H., Ludwig, R., Sabater, S., Vergoñós, L., et al. (2019). Impact and mitigation of global change on freshwater-related ecosystem services in Southern Europe. Sci. Total Environ. 651, 895–908. doi: 10.1016/j.scitotenv.2018.09.228
Khorchania, M., Nadal-Romeroa, E., Tague, C., Lasantaa, T., Zabalzaa, J., Lana-Renaultc, N., et al. (2020). Effects of active and passive land use management after cropland abandonment on water and vegetation dynamics in the Central Spanish Pyrenees. Sci. Total Environ. 717:137160. doi: 10.1016/j.scitotenv.2020.137160
Kim, I., Arnhold, S., Ahn, S., Le, Q. B., Kim, S. J., Park, S. J., et al. (2017). Land use change and ecosystem services in mountainous watersheds: predicting the consequences of environmental policies with cellular automata and hydrological modeling. Environ. Model. Softw. 122:103982. doi: 10.1016/j.envsoft.2017.06.018
Krueger, E., Rao, P. S. C., and Borchardt, D. (2019). Quantifying urban water supply security under global change. Glob. Environ. Change 56, 66–74. doi: 10.1016/j.gloenvcha.2019.03.009
Kuglerová, L., Jyvsjrvi, J., Ruffing, C., Muotka, T., Jonsson, A., Andersson, A., et al. (2020). Cutting edge: a comparison of contemporary practices of riparian buffer retention around small streams in Canada, Finland, and Sweden. Water Resour. Res. 56:e2019WR026381. doi: 10.1029/2019WR026381
Ladouceur, E., Mcgowan, J., Huber, P., Possingham, H., Scridel, D., Klink, R. V., et al. (2021). An objective-based prioritization approach to support trophic complexity through ecological restoration species mixes. J. Appl. Ecol. 59, 394–407. doi: 10.1111/1365-2664.13943
Lawler, J. J., Lewis, D. J., Nelson, E., Plantinga, A. J., Polasky, S., Withey, J. C., et al. (2014). Projected land-use change impacts on ecosystem services in the United States. Proc. Natl. Acad. Sci. U.S.A. 111, 7492–7497. doi: 10.1073/pnas.1405557111
Li, X. H., Farooqi, T. J. A., Jiang, C., Liu, S. R., and Sun, J. X. (2019). Spatiotemporal variations in productivity and water use efficiency across a temperate forest landscape of Northeast China. Forest Ecosyst. 6:22. doi: 10.1186/s40663-019-0179-x
Luetzenburg, G., Bittner, M. J., Calsamiglia, A., Renschler, C. S., Estrany, J., and Poeppl, R. (2020). Climate and land use change effects on soil erosion in two small agricultural catchment systems Fugnitz- Austria, CanRevull-Spain. Sci. Total Environ. 704:135389. doi: 10.1016/j.scitotenv.2019.135389
Mao, D. H., He, X. Y., Wang, Z. M., Tian, Y. L., Xiang, H. X., Yu, H., et al. (2019). Diverse policies leading to contrasting impacts on land cover and ecosystem services in Northeast China. J. Clean. Prod. 240:117961. doi: 10.1016/j.jclepro.2019.117961
Morán-Ordóez, A., Ameztegui, A., Cáceres, M. D., de-Miguelb, S., Lefèvref, F., Brotons, L., et al. (2020). Future trade-offs and synergies among ecosystem services in Mediterranean forests under global change scenarios. Ecosyst. Serv. 45:101174. doi: 10.1016/j.ecoser.2020.101174
Morán-Ordóez, A., Ramsauer, J., Coll, L., Brotons, L., and Ameztegui, A. (2021). Ecosystem services provision by Mediterranean forests will be compromised above 2°C warming. Glob. Change Biol. 27, 4210–4222. doi: 10.1111/gcb.15745
O’Connor, J., Santos, M. J., Rebel, K. T., and Dekker, S. C. (2019). The influence of water table depth on evapotranspiration in the Amazon arc of deforestation. Hydrol. Earth Syst. Sci. 23, 3917–3931. doi: 10.5194/hess-2019-47
Qiu, J. X., and Turner, M. G. (2013). Spatial interactions among ecosystem services in an urbanizing agricultural watershed. Proc. Natl. Acad. Sci. U.S.A. 110, 12149–12154. doi: 10.1073/pnas.1310539110
Ricke, K., Drouet, L., Caldeira, K., and Tavoni, M. (2018). Country-level social cost of carbon. Nat. Climate Change 8, 895–900. doi: 10.1038/s41558-018-0282-y
Romeo, R., Vita, A., Testolin, R., and Hofer, T. (2015). Mapping the Vulnerability of Mountain Peoples to Food Insecurity. Rome: FAO.
Runting, R. K., Bryan, B. A., Dee, L. E., Maseyk, F. J. F., Mandle, L., Hamel, P., et al. (2017). Incorporating climate change into ecosystem service assessments and decisions: a review. Glob. Change Biol. 23, 28–41. doi: 10.1111/gcb.13457
Sharp, R., Tallis, H. T., Ricketts, T., Guerry, A. D., Wood, S. A., Chaplin-Kramer, R., et al. (2018). InVEST 3.5.0 User’s Guide. Arlington, VA: The Nature Conservancy.
Song, F., Su, F., Mi, C., and Sun, D. (2020). Analysis of driving forces on wetland ecosystem services value change: a case in Northeast China. Sci. Total Environ. 751:141778. doi: 10.1016/j.scitotenv.2020.141778
Soto, D. P., Donoso, P. J., Elgueta, C. Z., Ríos, A. I., and Promis, A. (2021). Precipitation declines influence the understory patterns in Nothofagus pumilio old-growth forests in northwestern Patagonia. For. Ecol. Manag. 491:119169. doi: 10.1016/j.foreco.2021.119169
Steffen, W., Richardson, K., Rockström, J., Cornel1, S. E., Fetzer, I., Bennett, E. M., et al. (2015). Planetary boundaries: guiding human development on a changing planet. Science 347:1259855. doi: 10.1126/science.1259855
Tirupathi, C., and Shashidhar, T. (2020). Investigating the impact of climate and land-use land cover changes on hydrological predictions over the Krishna river basin under present and future scenarios. Sci. Total Environ. 721:137736. doi: 10.1016/j.scitotenv.2020.137736
Verburg, P. H., and Overmars, K. P. (2009). Combining top-down and bottom-up dynamics in land use modeling: exploring the future of abandoned farmlands in Europe with the Dyna-CLUE model. Landsc. Ecol. 24, 1167–1181. doi: 10.1007/s10980-009-9355-7
Verburg, P. H., Veldkamp, W., and Limpiada, R. (2002). Modeling the spatial dynamics of regional land use: the CLUE-S model. Environ. Manag. 30, 391–405. doi: 10.1007/s00267-002-2630-x
Vliet, J. V., Bregt, A. K., and Hagen-Zanker, A. (2011). Revisiting kappa to account for change in the accuracy assessment of land-use change models. Ecol. Model. 222, 1367–1375. doi: 10.1016/j.ecolmodel.2011.01.017
Wang, L. J., Ma, S., Zhao, Y. G., and Zhang, J. C. (2021). Ecological restoration projects did not increase the value of all ecosystem services in Northeast China. Forest Ecol. Manag. 495:119340. doi: 10.1016/j.foreco.2021.119340
Wang, L., Wang, W. J., Du, H. B., Wu, Z. F., Shen, X. J., and Ma, S. (2020). Decreasing precipitation occurs in daily extreme precipitation intervals across China in observations and model simulations. Climate Dyn. 54, 2597–2612. doi: 10.1007/s00382-020-05120-w
Wang, L., Wang, W. J., Wu, Z. F., Du, H. B., Zong, S. W., and Ma, S. (2019). Potential distribution shifts of plant species under climate change in Changbai Mountains, China. Forests 10:498. doi: 10.3390/f10060498
Wei, Y. W., Yu, D. P., Lewis, B. J., Zhou, L., Zhou, W. M., Fang, X. M., et al. (2014). Forest carbon storage and tree carbon pool dynamics under natural forest protection program in northeastern China. Chin. Geogr. Sci. 24, 397–405. doi: 10.1007/s11769-014-0703-4
Xia, H. J., Kong, W. J., Zhou, G., and Sun, O. J. X. (2021). Impacts of landscape patterns on water-related ecosystem services under natural restoration in Liaohe River Reserve, China. Sci. Total Environ. 792:20. doi: 10.1016/j.scitotenv.2021.148290
Xiao, X., Boles, S., Liu, J., Zhuang, D., and Liu, M. (2002). Characterization of forest types in Northeastern China, using multi-temporal SPOT-4 VEGETATION sensor data. Remote Sens. Environ. 82, 335–348. doi: 10.1016/s0034-4257(02)00051-2
Yin, G. D., Wang, G. Q., Zhang, X., Wang, X., Hu, Q. H., Shrestha, S., et al. (2022). Multi-scale assessment of water security under climate change in North China in the past two decades. Sci. Total Environ. 805:150103. doi: 10.1016/j.scitotenv.2021.150103
Yu, X. F., Ding, S. S., Zou, Y. C., Xue, Z. S., and Lv, X. G. (2018). Review of rapid transformation of floodplain wetlands in Northeast China: roles of human development and global environmental change. Chin. Geogr. Sci. 28, 114–124.
Zambon, N., Johannsen, L. L., Strauss, P., Dostal, T., Zumr, D., Cochrane, T. A., et al. (2021). Splash erosion affected by initial soil moisture and surface conditions under simulated rainfall. CATENA 196:104827. doi: 10.1016/j.catena.2020.104827
Zhang, J. J., Gao, G. Y., Fu, B. J., and Gupta, H. V. (2019). Formulating an elasticity approach to quantify the effects of climate variability and ecological restoration on sediment discharge change in the Loess Plateau, China. Water Resourc. Res. 55, 9604–9622. doi: 10.1029/2019WR025840
Keywords: water yield, soil retention, future climate change, land use and land cover change, interactive effects, InVEST model, CLUE-S model
Citation: Wang H, Wang WJ, Wang L, Ma S, Liu Z, Zhang W, Zou Y and Jiang M (2022) Impacts of Future Climate and Land Use/Cover Changes on Water-Related Ecosystem Services in Changbai Mountains, Northeast China. Front. Ecol. Evol. 10:854497. doi: 10.3389/fevo.2022.854497
Received: 14 January 2022; Accepted: 21 February 2022;
Published: 14 March 2022.
Edited by:
Fan Zhang, Institute of Geographic Sciences and Natural Resources Research (CAS), ChinaReviewed by:
Dragos George Zaharescu, Georgia Institute of Technology, United StatesFengping Li, Jilin University, China
Huakun Zhou, Key Laboratory of Restoration Ecology in Cold Regions, Northwest Institute of Plateau Biology (CAS), China
Copyright © 2022 Wang, Wang, Wang, Ma, Liu, Zhang, Zou and Jiang. This is an open-access article distributed under the terms of the Creative Commons Attribution License (CC BY). The use, distribution or reproduction in other forums is permitted, provided the original author(s) and the copyright owner(s) are credited and that the original publication in this journal is cited, in accordance with accepted academic practice. No use, distribution or reproduction is permitted which does not comply with these terms.
*Correspondence: Wen J. Wang, wangwenj@iga.ac.cn