- 1Estación Científica La Malinche, Centro Tlaxcala de Biología de la Conducta (CTBC), Universidad Autónoma de Tlaxcala, Tlaxcala, Mexico
- 2Department of Evolutionary Zoology and Human Biology, University of Debrecen, Debrecen, Hungary
- 3Juhász-Nagy Pál Doctoral School of Biology and Environmental Sciences, University of Debrecen, Debrecen, Hungary
- 4Department of Biology, University of Turku, Turku, Finland
- 5Univ Lyon, Université Claude Bernard Lyon 1, CNRS, ENTPE, UMR 5023 LEHNA, Villeurbanne, France
- 6Institut Pluridisciplinaire Hubert Curien, UMR 7178, CNRS, Université de Strasbourg, Strasbourg, France
- 7School of Biological Sciences, University of Aberdeen, Aberdeen, United Kingdom
Hormonal pathways have been proposed to be key at modulating how fast individuals grow and reproduce and how long they live (i.e., life history trajectory). Research in model species living under controlled environment is suggesting that insulin-like growth factor 1 (IGF-1), which is an evolutionarily conserved polypeptide hormone, has an important role in modulating animal life histories. Much remains, however, to be done to test the role played by IGF-1 in shaping the phenotype and life history of animals in the wild. Using a wild long-lived bird, the Alpine swift (Tachymarptis melba), we show that adults with higher levels of IGF-1 had longer wings and shorter telomeres. Hence, telomeres being a proxy of lifespan in this species, our results support a potential role of IGF-1 at shaping the life-history of wild birds and suggest that IGF-1 may influence the growth-lifespan trade-off.
Introduction
How fast an individual grows and reproduces and how long it lives are referred to as its life history trajectory (Flatt and Heyland, 2011). Although life history traits exhibit a wide range of variation among species, consistent patterns of covariation between traits are widespread (Gaillard et al., 1989). Such patterns of covariation are thought to be regulated by competitive allocation of limited resources (Stearns, 1992), although other proximate mechanisms have also been suggested (Harshman and Zera, 2007). Accordingly, hormonal pathways have been proposed to play a key role in regulating life-history trade-offs (Ricklefs and Wikelski, 2002). One candidate hormone is insulin-like growth factor 1 (IGF-1), which is an evolutionarily conserved polypeptide known to have effects on three key life-history traits: growth, reproduction, and survival (Swanson and Dantzer, 2014; Lodjak and Verhulst, 2020). Moreover, IGF-1 is suspected to be involved in the regulation of the trade-offs among these three life-history traits, but this hypothesis has only been scarcely tested in free-living animals (Swanson and Dantzer, 2014; Lodjak and Verhulst, 2020).
In laboratory models (i.e., worms, flies, and mice), IGF-1 signaling and circulating levels are linked to the growth of somatic (Musarò et al., 2001; Yakar et al., 2002) and reproductive structures (Kaaks et al., 2000), gonadal function regulation (Bartke et al., 2013), and reproductive performance (Li et al., 2011). Moreover, the inhibition of IGF-1 signaling is associated with increased lifespan (Holzenberger et al., 2003), but the proximate mechanisms underlying this association are less well understood. Although IGF-1 levels are highly repeatable within individuals, 0.41–0.85 (Roberts et al., 1990; Yuan et al., 2009), they respond to environmental fluctuations on nutritional resource availability and temperature (Gabillard et al., 2003; Sparkman et al., 2009; Regan et al., 2020). Therefore, studies on the role played by IGF-1 on growth, reproduction, and survival, in natural settings may be particularly relevant. In free-living animals, ecological conditions have been shown to influence the direction of the links between IGF-1 and life-history traits. For example, in free-ranging garter snakes (Thamnophis elegans) the presence of a positive association between IGF-1 levels and adult body size is dependent on the habitat type (Sparkman et al., 2009). So far, general conclusions reached in laboratory models, on the positive association between IGF-1 with growth have been (at least partially) confirmed in several non-model species (inter-specific comparison, Lodjak et al., 2018). Moreover, although the number of studies evaluating the link between IGF-1 and lifespan in non-model species has been very limited, they mostly confirmed the negative association between IGF-1 and lifespan reported in model organisms, both at the interspecific (Swanson and Dantzer, 2014; Lodjak et al., 2018) and at the intraspecific level (Lewin et al., 2017, but see Chaulet et al., 2012; Lendvai et al., 2020). Still, the nature of the proximate mechanisms implicated remains elusive, and one of the putative candidates is oxidative stress (Holzenberger et al., 2003), which is known to accelerate the shortening of telomeres (Reichert and Stier, 2017), one of the hallmarks of aging (López-Otín et al., 2013).
Although understanding variation in lifespan is a major focus of evolutionary ecology, accurately measuring lifespan in the wild can be logistically challenging (Nussey et al., 2008). Remarkably, telomere length has been shown to be linked to life expectancy in non-model species (Wilbourn et al., 2018). Telomeres are non-coding sequences of repetitive DNA located at the end of linear chromosomes that shorten with each cellular replication (Backburn and Epel, 2012), in response to cellular stressors including oxidative stress (Reichert and Stier, 2017), and under increased metabolic demand (Casagrande and Hau, 2019). Both, IGF-1 and telomere length, have been shown to diminish with age (Moverare-Skrtic et al., 2009) and are linked to longevity (Deelen et al., 2013). The association between IGF-1 and telomeres has mainly been explored in laboratory animals and human patients exhibiting pathological disorder of their somatotropic axis as well as cancer cell lines (Aulinas et al., 2013; Matsumoto et al., 2015). While cross-sectional and correlative studies in humans and laboratory animals showed that IGF-1 and telomere length are positively related (Barbieri et al., 2009; Moverare-Skrtic et al., 2009; Yeap et al., 2019), studies conducted in cell lines found a negative association between these variables (Matsumoto et al., 2015; Matsumoto and Takahashi, 2016). Hence, the direction and strength of association between IGF-1 and telomeres is still equivocal, and much remains to be done to understand whether telomere dynamics is part of the mechanisms underlying the covariation observed between IGF-1 and lifespan.
The Alpine swift (Tachymarptis melba) is an insectivorous migratory bird that live up to 26 years of age and has a slow pace of life (i.e., late age at sexual maturity, only one reproductive attempt per year, and small clutch size per breeding attempt) (Bize et al., 2009). The fact that adult Alpine swifts with longer telomeres have higher survival rate (Bize et al., 2009) makes them a good model for exploring the links between IGF-1 and telomere length. We propose that, if IGF-1 is a proximate mechanism underlying trade-offs between growth and lifespan, then IGF-1 should show a positive association with body size and a negative relationship with telomere length. As effects of IGF-1 on size can be tissue specific (Sharma et al., 2012), and thus may affect the growth of different body parts in dissimilar ways, we measured wing length, sternum length and body mass as proxies of body size.
Methods
Fieldwork was carried out in 2018 in two Alpine swift colonies located in Bienne and Solothurn, Switzerland, where nestlings have been ringed each year since at least 1968 and adults have been subjected to an individual based study since 2000 (Bize et al., 2009). Forty-three adult Alpine swifts were captured on their nests or roosting during the breeding season. After capture, each adult was weighed with a digital scale to the nearest 0.1 g, the same person (PB) measured their wing with a ruler to the nearest mm and their sternum with a caliper to the nearest 0.1 mm. Wing length was measured as the distance on the closed wing from the foremost extremity of the carpus to the tip of the longest primary feathers; the wing being flattened and straightened to give maximal wing length. Sternum was measured from one end to the other of the keel. We collected a 200 μL blood sample from the foot vein using heparinized microvette tubes (Sarstedt, Germany) to quantify telomere length and IGF-1 levels. Blood samples were kept on ice in the field before being centrifuged at 10 000 g × 10 min to separate plasma from red blood cells, and then stored at –80°C until laboratory analyses. The Alpine swift is a monomorphic bird species, and thus adults were genetically sexed (Bize et al., 2005). The exact chronological age was known for 38 birds that had been ringed as nestlings (range: 2–17 years).
Telomere Length
Multiplex quantitative PCR was used to quantify relative telomere length (RTL). Full protocols for genomic DNA extraction using nucleated red blood cells and of multiplex qPCR description adapted for swifts are available in Criscuolo et al. (2017). The concentration and purity (presence of residual proteins or solvents) of the extracted DNA were checked with a Nano-Drop ND-1000 spectrophotometer (Thermo Fisher Scientific, MA, United States). The quality and the integrity of DNA were confirmed by gel electrophoresis on 1% agarose gels stained with ethidium bromide (checking for the absence of DNA smears corresponding to degraded DNA). Our 43 adults’ RTL were measured within a batch of chick and adult samples (total 109 samples) measured in duplicates over one 384 wells plate. Amplification efficiencies of control and telomere sequences were of 99.6% (identical for both sequences) and r2 of dilution curves of 0.97 and 0.98, respectively. Based on duplicates within plates, intra-class correlation coefficient for final RTL values was 0.689.
Insulin-Like Growth Factor-1
IGF-1 concentrations were measured from 10 μl of plasma using an enzyme-linked immunosorbent assay (for further details see Mahr et al., 2020). We used chicken plasma in quadruplicates to determine intra- (5.3%) and inter-assay coefficient of variation (10.4%). We validated the assay for the Alpine swifts by showing that serially diluted plasma samples were parallel with the standard curve.
Statistical Analyses
Statistical analyses were performed in R version 4.0.0, using the R package lmerTest (Kuznetsova et al., 2017; R Core Team, 2021). A preliminary analysis exploring the effect of sampling date, hour, and breeding site on IGF-1 levels showed that only sampling date accounted for variation in IGF-1 levels; sampling date was therefore retained as a covariate when analyzing variation in IGF-1. Firstly, we tested for an association between IGF-1 and wing length, sternum length and/or body mass by fitting a general linear model with IGF-1 as response variable and all three biometric traits, plus sampling date, as explanatory variables (model 1). Variance inflation factors in this first model were below 1.24 indicating no collinearity issues between biometric traits. Secondly, we investigated how IGF-1 (response variable) was related to telomere length by including as explanatory variables telomere length while controlling for possible confounding effect of chronological age and traits identified as significant in our model 1. Sample sizes vary across analyses due to missing values in some of the traits.
Results
Plasma levels of IGF-1 in adult Alpine swifts were positively associated with wing length (Figure 1A and Table 1A) but not related to body mass and sternum, those results being obtained after controlling for the date of sampling (Table 1A). Adults sampled later in the season had lower IGF-1 levels (Table 1). Hence, wing length and sampling date were included as covariates in the follow-up analysis. Adult birds with higher IGF-1 levels had shorter telomeres (Figure 1B and Table 1B), after controlling for the date of sampling, wing length, and chronological age (Table 1B).
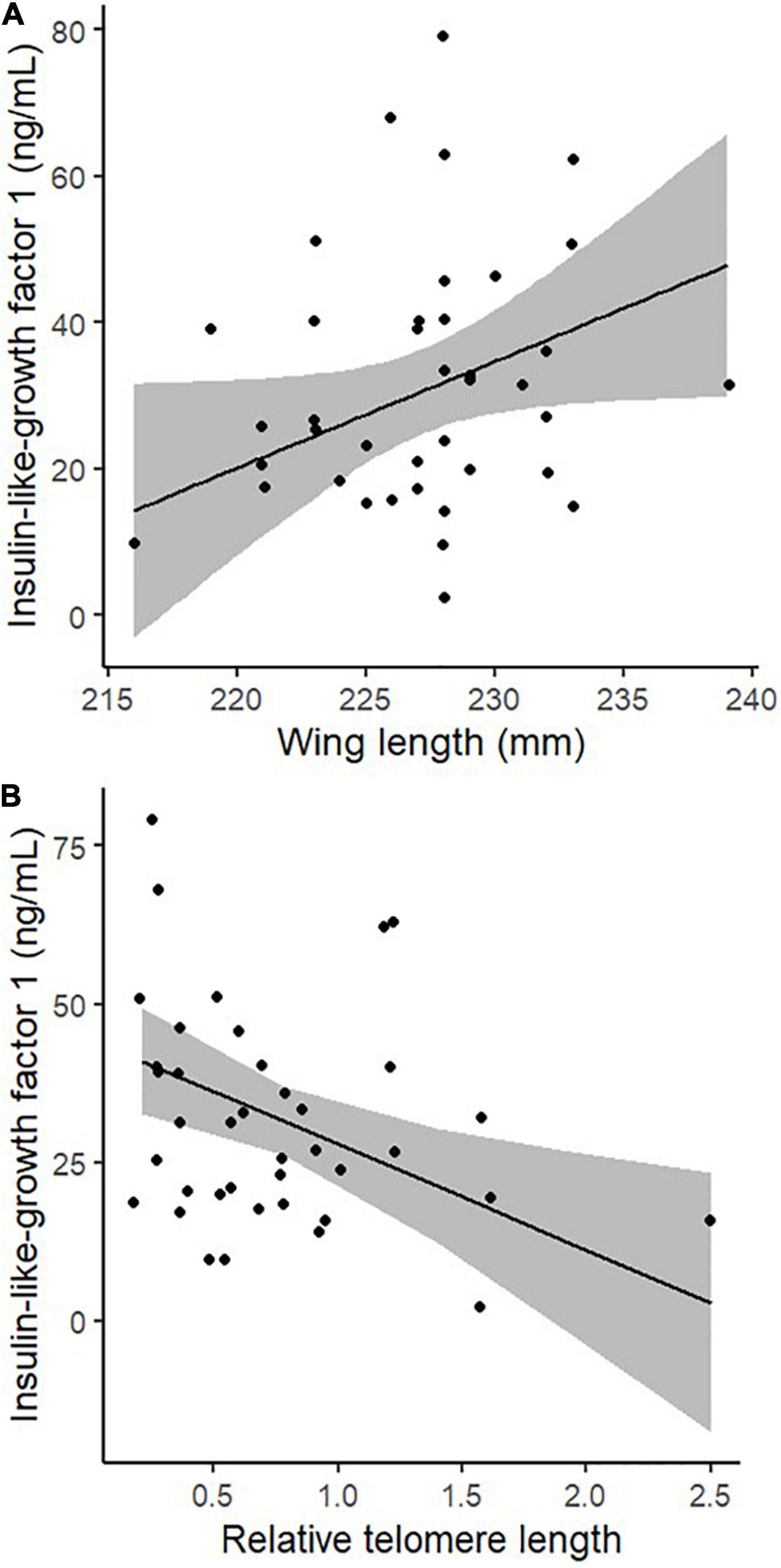
Figure 1. Panel (A) shows the relationship between wing length and plasma levels of insulin-like growth factor 1, in adult Alpine swifts. Panel (B) presents the association of relative telomere length and plasma levels of insulin-like growth factor 1, in adult Alpine swifts.
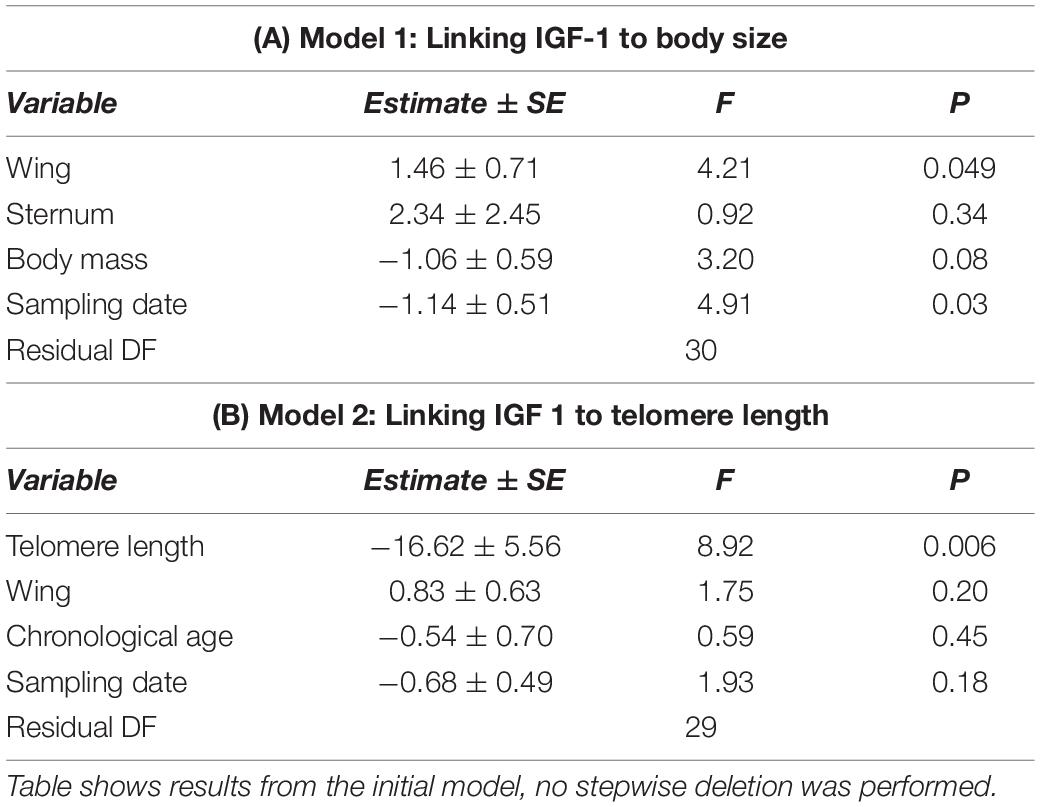
Table 1. Linear models showing positive association between plasma levels of insulin-like growth factor 1 and wing length (model 1), and negative relation between plasma levels of insulin-like growth factor 1 and relative telomere length (model 2), in adult Alpine swifts.
Discussion
Overall, as predicted, we found that circulating levels of IGF-1 were positively associated with wing length and negatively to telomere length, in adult Alpine swifts. Those opposite associations of IGF-1 with body size and telomere length support the idea that IGF-1 may regulate, at least partially, the life-history of this long-lived bird species.
Our results show that adult birds with higher levels of IGF-1 had longer wings, which matches recent findings in adult bearded reedlings (Panurus biarmicus) showing a positive association of between IGF-1 levels and tail feather length (Mahr et al., 2020) and in nestling pied flycatchers (Ficedula hypoleuca) showing a positive association between IFG-1 and growth and size at fledging (Lodjak et al., 2016). The positive association between IGF-1 and wing length in adult swifts may be caused either by the effects of IGF-1 on wing bone growth early in life and/or primary feather growth. An experimental elevation of IGF-1 in adult bearded reedlings had no effects on feather growth, but increased moult intensity (Lendvai et al., 2021), whereas an experimental administration of IGF-1 to nestling pied flycatchers positively affected the growth of their tarsi but not of their wings (Lodjak et al., 2017). Hence, the present finding in adult Alpine swifts of a link between IGF1-1 and wing length is likely to be dependent on wing bones length rather than primary feathers length. A positive effect of IGF-1 on bone growth has been reported on numerous occasions [e.g., Yakar et al., 2002; Lodjak et al., 2016; Lendvai et al., 2021]. The effect of IGF-1 on bone growth appears, however, to be stronger on short than long bones, at least in laboratory models (Wang et al., 2006). It may explain why we only observed an association between IGF-1 with wing bones length (carpals and metacarpals) but not with sternum length, with the carpals and metacarpals being short boned whereas the sternum is one of the longest bones in swifts. The contrasting finding between IGF-1 and wing length in experimentally manipulated nestling pied flycatchers and adult swifts may come from differences between species in the influence of IGF-1 on wing bone growth, considering that Alpine swifts spend most of their lifetime aloft (Liechti et al., 2013). Hence, one hypothesis is that the positive association between IGF-1 and wing length in adult swifts comes from early-life allocation of resources into wing bone growth. This hypothesis also assumes that IGF-1 should be repeatable through the individual’s life, from the nestling to adult stage, which has been previously reported (Roberts et al., 1990; Yuan et al., 2009). In this study, IGF-1 was found to vary with sampling date, and thus IGF-1 may potentially change during the breeding season. Yet, variation in IGF-1 levels among individuals can still be higher that those within individuals, making the trait flexible but repeatable. Many examples of this can be found in the literature regarding different physiological traits such as glucose levels (Montoya et al., 2018, 2020), antioxidant defenses (Récapet et al., 2019) or mitochondrial function (Stier et al., 2019). Further studies are now needed in swifts and other bird species to examine the repeatability through individual’s life in IGF-1 and test for effects early in life of IGF-1 on the growth of short (e.g., carpals and metacarpals) and long (tarsi, sternum) bones.
Our results also show that IGF-1 and telomere length were negatively related in adult swifts. Studies in laboratory models and humans indicate that IGF-1 has a positive effect on telomerase activity (Bayne and Liu, 2005), suggesting that IGF-1 and telomere length could be positively correlated (e.g., Barbieri et al., 2009; Moverare-Skrtic et al., 2009; Yeap et al., 2019, but see Matsumoto et al., 2015; Matsumoto and Takahashi, 2016). However, the experimental disruption of IGF-1 signaling pathway in laboratory animals has been found to extend lifespan (Holzenberger et al., 2003; Yuan et al., 2009), and the few studies performed in free-living animals corroborate such an inverse correlation between IGF-1 and lifespan (Lewin et al., 2017; Lodjak and Mägi, 2017) or in our study with a proxy of lifespan such as telomere length (Sirman, 2019). So, if the link between IGF-1 and telomere length is not due to telomerase, an alternative mechanism may be oxidative stress. Interestingly, reduced IGF-1 signaling has also been associated with increased in vivo resistance to oxidative stress in laboratory models (Holzenberger et al., 2003). Furthermore, administration of IGF-1 in wild birds was found to lead to greater oxidative damage (Lendvai et al., 2020; Vágási et al., 2020) and higher activity of glutathione peroxidase (Lodjak and Mägi, 2017; Lendvai et al., 2020), the later possibly reflecting up-regulated antioxidant activity in response to oxidative stress (Lodjak and Mägi, 2017; Lendvai et al., 2020). Variation in oxidative stress and telomere length have been linked to the aging process, with individuals with enhanced resistance to oxidative stress and/or longer telomeres having higher annual survival or better fitness (Bize et al., 2009), and importantly exposure to oxidative stress is thought to accelerate telomere shortening (Reichert and Stier, 2017; but see Pérez-Rodríguez et al., 2019). Therefore, a negative association between IGF-1 and telomere length as reported here in adult swifts and other previous studies (Barbieri et al., 2009; Moverare-Skrtic et al., 2009; Yeap et al., 2019) may come from cascading effects of IGF-1 on oxidative stress, affecting in turn telomere dynamics through direct or indirect pathways (Casagrande and Hau, 2019), an idea that requires now further empirical examination.
In conclusion, negative associations of IGF-1 with wing and telomere length found here suggest that IGF-1 may play a role in regulating life-histories, and welcome future studies exploring the association of IGF-1 with direct measurements of growth and lifespan in wild organisms.
Data Availability Statement
The raw data supporting the conclusions of this article will be made available by the authors, without undue reservation.
Ethics Statement
The animal study was reviewed and approved by Swiss Federal Food Safety and Veterinary Office (FSVO).
Author Contributions
BM, PB, and ÁL designed the study. BM, PB, and AS carried out the fieldwork. SZ and FC carried the laboratory analysis and interpretation of TL measures. ZT and ÁL carried the laboratory analysis and interpretation of IGF-1 measures. BM and PB carried out the data analyses. BM wrote the first draft. All authors critically revised the manuscript.
Funding
PB, FC, and AS were supported by a Carnegie Research Incentive Grant (RIG007773). BM was supported by a Godfrey Hewitt Mobility Award from the European Society for Evolutionary Biology. ÁL was supported by a grant from the National Research and Development Office (K139021).
Conflict of Interest
The authors declare that the research was conducted in the absence of any commercial or financial relationships that could be construed as a potential conflict of interest.
Publisher’s Note
All claims expressed in this article are solely those of the authors and do not necessarily represent those of their affiliated organizations, or those of the publisher, the editors and the reviewers. Any product that may be evaluated in this article, or claim that may be made by its manufacturer, is not guaranteed or endorsed by the publisher.
References
Aulinas, A., Ramírez, M. J., Barahona, M. J., Mato, E., Bell, O., Surrallés, J., et al. (2013). Telomeres and endocrine dysfunction of the adrenal and GH/IGF-1 axes. Clin. Endocrinol. 79, 751–759. doi: 10.1111/cen.12310
Backburn, E. H., and Epel, E. S. (2012). Telomeres and adversity: too toxic to ignore. Nature 490, 169–171. doi: 10.1038/490169a
Barbieri, M., Paolisso, G., Kimura, M., Gardner, J. P., Boccardi, V., Papa, M., et al. (2009). Higher circulating levels of IGF-1 are associated with longer leukocyte telomere length in healthy subjects. Mech. Ageing Dev. 130, 771–776. doi: 10.1016/j.mad.2009.10.002
Bartke, A., Sun, L. Y., and Longo, V. (2013). Somatotropic signaling: trade-offs between growth, reproductive development, and longevity. Physiol. Rev. 93, 571–598. doi: 10.1152/physrev.00006.2012
Bayne, S., and Liu, J. P. (2005). Hormones and growth factors regulate telomerase activity in ageing and cancer. Mol. Cell. Endocrinol. 240, 11–22. doi: 10.1016/j.mce.2005.05.009
Bize, P., Criscuolo, F., Metcalfe, N. B., Nasir, L., and Monaghan, P. (2009). Telomere dynamics rather than age predict life expectancy in the wild. Proc. R. Soc. B 276, 1679–1683. doi: 10.1098/rspb.2008.1817
Bize, P., Roulin, A., Tella, J. L., and Richner, H. (2005). Female-biased mortality in experimentally parasitized Alpine Swift Apus melba nestlings. Func. Ecol. 19, 405–413. doi: 10.1111/j.1365-2435.2005.00995.x
Casagrande, S., and Hau, M. (2019). Telomere attrition: metabolic regulation and signalling function? Biol. Lett. 15:20180885. doi: 10.1098/rsbl.2018.0885
Chaulet, A., Medesani, D. A., Freitas, J., Cervino, A., Cervino, N., and Rodríguez, E. M. (2012). Induction of somatic growth in juvenile crayfish Cherax quadricarinatus (Decapoda, Parastacidae), by ecdysone and insulin growth factor. Aquaculture 370, 1–6. doi: 10.1016/j.aquaculture.2012.09.026
Criscuolo, F., Zahn, S., and Bize, P. (2017). Offspring telomere length in the long lived Alpine swift is negatively related to the age of their biological father and foster mother. Biol. Lett. 13:20170188. doi: 10.1098/rsbl.2017.0188
Deelen, J., Uh, H. W., Monajemi, R., van Heemst, D., Thijssen, P. E., Böhringer, S., et al. (2013). Gene set analysis of GWAS data for human longevity highlights the relevance of the insulin/IGF-1 signaling and telomere maintenance pathways. Age 35, 235–249. doi: 10.1007/s11357-011-9340-3
Flatt, T., and Heyland, A. (eds) (2011). Mechanisms of life History Evolution: the Genetics and Physiology of Life History Traits and Trade-Offs. New York, NY: Oxford University press.
Gabillard, J. C., Weil, C., Rescan, P. Y., Navarro, I., Gutiérrez, J., and Le Bail, P. Y. (2003). Effects of environmental temperature on IGF1, IGF2, and IGF type I receptor expression in rainbow trout (Oncorhynchus mykiss). Gen. Comp. Endocr. 133, 233–242. doi: 10.1016/S0016-6480(03)00167-9)
Gaillard, J. M., Pontier, D., Allaine, D., Lebreton, J. D., Trouvilliez, J., and Clobert, J. (1989). An analysis of demographic tactics in birds and mammals. Oikos 56, 59–76. doi: 10.2307/3566088)
Harshman, L. G., and Zera, A. J. (2007). The cost of reproduction: the devil in the details. Trends Ecol. Evol. 22, 80–86. doi: 10.1016/j.tree.2006.10.008
Holzenberger, M., Dupont, J., Ducos, B., Leneuve, P., Géloën, A., Even, P. C., et al. (2003). IGF-1 receptor regulates lifespan and resistance to oxidative stress in mice. Nature 421, 182–187. doi: 10.1038/nature01298
Kaaks, R., Lukanova, A., and Sommersberg, B. (2000). Plasma androgens, IGF-1, body size, and prostate cancer risk: a synthetic review. Prostate Cancer Prostatic Dis. 3, 157–172. doi: 10.1038/sj.pcan.4500421
Kuznetsova, A., Brockhoff, P. B., and Christensen, R. H. (2017). lmerTest package: tests in linear mixed effects models. J. Stat. Softw. 82, 1–26. doi: 10.18637/jss.v082.i13
Lendvai, A. Z., Tóth, Z., Pénzes, J., Vogel-Kindgen, S., Gander, B. A., and Vágási, C. I. (2020). Insulin-like growth factor 1 induces oxidative damage, but does not affect survival in a songbird. BioRxiv. [preprint]. doi: 10.1101/2020.03.27.012120
Lendvai, ÁZ., Tóth, Z., Mahr, K., Osváth, G., Vogel Kindgen, S., and Gander, A. (2021). Effects of experimental increase in insulin-like growth factor 1 on feather growth rate, moult intensity and feather quality in a passerine bird. J. Exp. Biol. 224:jeb242481. doi: 10.1242/jeb.242481
Lewin, N., Swanson, E. M., Williams, B. L., and Holekamp, K. E. (2017). Juvenile concentrations of IGF-1 predict life-history trade-offs in a wild mammal. Funct. Ecol. 31, 894–902. doi: 10.1111/1365-2435.12808
Li, L., Fu, Y. C., Xu, J. J., Chen, X. C., Lin, X. H., and Luo, L. L. (2011). Caloric restriction promotes the reproductive capacity of female rats via modulating the level of insulin-like growth factor-1 (IGF-1). Gen. Comp. Endocrinol. 174, 232–237. doi: 10.1016/j.ygcen.2011.09.005
Liechti, F., Witvliet, W., Weber, R., and Bächler, E. (2013). First evidence of a 200-day non-stop flight in a bird. Nat. Commun. 4, 1–7. doi: 10.1038/ncomms3554
Lodjak, J., and Mägi, M. (2017). Crosstalk between growth and somatic maintenance in young animals. J. Avian Biol. 48, 1360–1363. doi: 10.1111/jav.01519
Lodjak, J., Mänd, R., and Mägi, M. (2018). Insulin-like growth factor 1 and life-history evolution of passerine birds. Funct. Ecol. 32, 313–323. doi: 10.1111/1365-2435.12993
Lodjak, J., Mägi, M., Sild, E., andMänd, R. (2017). Causal link between insulin-like growth factor 1 and growth in nestlings of a wild passerine bird. Funct. Ecol. 31, 184–191. doi: 10.1111/1365-2435.12679
Lodjak, J., Tilgar, V., and Mägi, M. (2016). Does the interaction between glucocorticoids and insulin-like growth factor 1 predict nestling fitness in a wild passerine? Gen. Comp. Endocrinol. 225, 149–154. doi: 10.1016/j.ygcen.2015.10.016
Lodjak, J., and Verhulst, S. (2020). Insulin-like growth factor 1 of wild vertebrates in a life-history context. Mol. Cell. Endocrinol. 518:110978. doi: 10.1016/j.mce.2020.110978
López-Otín, C., Blasco, M. A., Partridge, L., Serrano, M., and Kroemer, G. (2013). The hallmarks of aging. Cell 153, 1194–1217. doi: 10.1016/j.cell.2013.05.039
Mahr, K., Vincze, O., Tóth, Z., Hoi, H., Lendvai, and Áz. (2020). Insulin-like growth factor 1 is related to the expression of plumage traits in a passerine species. Behav. Ecol. Sociobiol. 74:39. doi: 10.1007/s00265-020-2821-6
Matsumoto, R., Fukuoka, H., Iguchi, G., Odake, Y., Yoshida, K., Bando, H., et al. (2015). Accelerated telomere shortening in acromegaly; IGF-I induces telomere shortening and cellular senescence. PLoS One 10:e0140189. doi: 10.1371/journal.pone.0140189
Matsumoto, R., and Takahashi, Y. (2016). Telomeres and Cellular Senescence in Metabolic and Endocrine Diseases. In Telomere: A Complex End of a Chromosome. 143. Croatia: Tech Publisher Rijeka.
Montoya, B., Briga, M., Jimeno, B., Moonen, S., and Verhulst, S. (2018). Baseline glucose level is an individual trait that is negatively associated with lifespan and increases due to adverse environmental conditions during development and adulthood. J. Comp. Physiol. B 188, 517–526. doi: 10.1007/s00360-017-1143-0
Montoya, B., Briga, M., Jimeno, B., and Verhulst, S. (2020). Glucose regulation is a repeatable trait affected by successive handling in zebra finches. J. Comp. Physiol. B 190, 455–464. doi: 10.1007/s00360-020-01283-4
Moverare-Skrtic, S., Svensson, J., Karlsson, M., Orwoll, E., Ljunggren, Ö, Mellström, D., et al. (2009). Serum Insulin-Like Growth Factor-1 concentration is associated with leukocyte telomere length in a population-based cohort of elderly men. J. Clin. Endocrinol. Metab. 94, 5078–5084. doi: 10.1210/jc.2009-1450
Musarò, A., McCullagh, K., Paul, A., Houghton, L., Dobrowolny, G., Molinaro, M., et al. (2001). Localized Igf-1 transgene expression sustains hypertrophy and regeneration in senescent skeletal muscle. Nat. Genet. 27, 195–200. doi: 10.1038/84839
Nussey, D. H., Coulson, T., Festa Bianchet, M., and Gaillard, J. M. (2008). Measuring senescence in wild animal populations: towards a longitudinal approach. Funct. Ecol. 22, 393–406. doi: 10.1111/j.1365-2435.2008.01408.x
Pérez-Rodríguez, L., Redondo, T., Ruiz-Mata, R., Camacho, C., Moreno-Rueda, G., and Potti, J. (2019). Vitamin E supplementation—but not induced oxidative stress—influences telomere dynamics during early development in wild passerines. Front. Ecol. Evol. 7:173. doi: 10.3389/fevo.2019.00173
R Core Team (2021). R: A Language and Environment for Statistical Computing. Vienna: R Foundation for Statistical Computing.
Récapet, C., Arrivé, M., Doligez, B., and Bize, P. (2019). Antioxidant capacity is repeatable across years but does not consistently correlate with a marker of peroxidation in a free-living passerine bird. J. Comp. Physiol. B 189, 283–298. doi: 10.1007/s00360-019-01211-1
Regan, J. C., Froy, H., Walling, C. A., Moatt, J. P., and Nussey, D. H. (2020). Dietary restriction and insulin-like signalling pathways as adaptive plasticity: A synthesis and re-evaluation. Funct. Ecol. 34, 107–128. doi: 10.1111/1365-2435.13418
Reichert, S., and Stier, A. (2017). Does oxidative stress shorten telomeres in vivo? A review. Biol. Lett. 13:20170463. doi: 10.1098/rsbl.2017.0463
Ricklefs, R. E., and Wikelski, M. (2002). The physiology/life-history nexus. Trends Ecol. Evol. 17, 462–468. doi: 10.1016/S0169-5347(02)02578-8
Roberts, C. A., McCutcheon, S. N., Blair, H. T., Gluckman, P. D., and Breier, B. H. (1990). Developmental patterns of plasma insulin-like growth factor-1 concentrations in sheep. Domest. Anim. Endocrinol. 7, 457–463. doi: 10.1016/0739-7240(90)90003-I
Sharma, N., Castorena, C. M., and Cartee, G. D. (2012). Tissue-specific responses of IGF-1/insulin and mTOR signaling in calorie restricted rats. PLoS One 7:e38835. doi: 10.1371/journal.pone.0038835
Sirman, A. (2019). Biological Mechanisms Underpinning Growth and Ageing in Wild Birds. North Dakota, USA: North Dakota State University. [PhD thesis.]
Sparkman, A. M., Vleck, C. M., and Bronikowski, A. M. (2009). Evolutionary ecology of endocrine-mediated life-history variation in the garter snake Thamnophis elegans. Ecology 90, 720–728. doi: 10.1890/08-0850.1
Stier, A., Bize, P., Hsu, B.-Y., and Ruuskanen, S. (2019). Plastic but repeatable: rapid adjustments of mitochondrial function and density during reproduction in a wild bird species. Biol. Lett. 15:20190536. doi: 10.1098/rsbl.2019.0536
Swanson, E. M., and Dantzer, B. (2014). Insulin-like growth factor-1 is associated with life-history variation across Mammalia. Proc. R. Soc. B 281:20132458. doi: 10.1098/rspb.2013.2458)
Vágási, C. I., Tóth, Z., Pénzes, J., Pa, P. L., Ouyang, J. Q., Lendvai, et al. (2020). The Relationship between Hormones, Glucose, and Oxidative Damage Is Condition and Stress Dependent in a Free-Living Passerine Bird. Physiol. Biochem. Zool. 93, 466–476. doi: 10.1086/711957
Wang, Y., Nishida, S., Sakata, T., Elalieh, H. Z., Chang, W., Halloran, B. P., et al. (2006). Insulin-like growth factor-I is essential for embryonic bone development. Endocrinology 147, 4753–4761. doi: 10.1210/en.2006-0196
Wilbourn, R. V., Moatt, J. P., Froy, H., Walling, C. A., Nussey, D. H., and Boonekamp, J. J. (2018). The relationship between telomere length and mortality risk in non-model vertebrate systems: a meta-analysis. Philos. Trans. R. Soc. B 373:20160447. doi: 10.1098/rstb.2016.0447
Yakar, S., Rosen, C. J., Beamer, W. G., Ackert-Bicknell, C. L., Wu, Y., Liu, J. L., et al. (2002). Circulating levels of IGF-1 directly regulate bone growth and density. J. Clin. Investig. 110, 771–781. doi: 10.1172/JCI15463
Yeap, B. B., Hui, J., Knuiman, M., Chubb, S. A., Ho, K. K., Flicker, L., et al. (2019). Associations of plasma IGF1, IGFBP3 and estradiol with leucocyte telomere length, a marker of biological age, in men. Eur. J. Endocrinol. 182, 23–33. doi: 10.1530/EJE-19-0638
Keywords: aging, IGF-1, life-history, lifespan, pace-of-life syndrome, telomeres, trade-off
Citation: Montoya B, Tóth Z, Lendvai ÁZ, Stier A, Criscuolo F, Zahn S and Bize P (2022) Does IGF-1 Shape Life-History Trade-Offs? Opposite Associations of IGF-1 With Telomere Length and Body Size in a Free-Living Bird. Front. Ecol. Evol. 10:853674. doi: 10.3389/fevo.2022.853674
Received: 12 January 2022; Accepted: 28 February 2022;
Published: 18 March 2022.
Edited by:
Edward Narayan, The University of Queensland, AustraliaReviewed by:
Andrzej Bartke, Southern Illinois University School of Medicine, United StatesYufeng Zhang, University of Memphis, United States
Copyright © 2022 Montoya, Tóth, Lendvai, Stier, Criscuolo, Zahn and Bize. This is an open-access article distributed under the terms of the Creative Commons Attribution License (CC BY). The use, distribution or reproduction in other forums is permitted, provided the original author(s) and the copyright owner(s) are credited and that the original publication in this journal is cited, in accordance with accepted academic practice. No use, distribution or reproduction is permitted which does not comply with these terms.
*Correspondence: Bibiana Montoya, YmliaWFuYWMubW9udG95YWxAdWF0eC5teA==; Pierre Bize, cGllcnJlLmJpemVAYWJkbi5hYy51aw==