- 1Yale School of the Environment, Yale University, New Haven, CT, United States
- 2The New York Botanical Garden, Bronx, NY, United States
- 3Smithsonian Tropical Research Institute, Balboa, Panama
- 4Facultad de Ciencias, Departamento de Biología, Pontificia Universidad Javeriana, Bogotá, Colombia
- 5School of Biology and Ecology, University of Maine, Orono, ME, United States
- 6Department of Natural Resources Management, Texas Tech University, Lubbock, TX, United States
- 7Natural Science Research Laboratory, Museum of Texas Tech University, Lubbock, TX, United States
Evenness is a key community property that provides insights into resource acquisition and ecosystem functioning. However, it is unclear how other community properties influence evenness after integrating the effect of environmental gradients. Using 49 neotropical bat communities, we tested the hypothesis that evenness increases in communities that have low species richness and low biomass because the negative effect of richness and biomass on evenness is regulated by environmental seasonality. We selected among path models to determine how temperature seasonality, the most important gradient across study sites, affected richness and biomass as drivers of evenness. Employing three indices of evenness, we found that more seasonal climate reduces species richness, and lower richness increases evenness. Moreover, a decline in biomass with increasing seasonality also increases evenness. A decrease in resource specialization and rarity as sites become more seasonal may explain the negative relationship between seasonality, richness and evenness. Moreover, the negative effect of biomass on richness and evenness may be due to an expansion of niche space and a positive effect of smaller body size on diversification rates, which may allow more species packing and greater richness. We believe our results bring us closer to a unified theory of which factors control evenness in a community.
Introduction
Evenness and richness are the two components of diversity (Hurlbert, 1971; Pielou, 1975). Richness describes the number of species in a community, whereas evenness indicates how similar are proportional abundances of those species in a community. Most of our understanding of diversity stems from examination of richness among communities, and in particular how it varies along environmental gradients (Rosenzweig, 1995). For example, it is well established that richness increases with productivity and climatic stability, except in cases of eutrophication, and it is usually lower with decreasing temperature (Mittelbach and Mcgill, 2019). In comparison, our understanding of diversity using evenness is not as thorough, probably because abundance data, which are harder to acquire, is necessary to calculate evenness. Therefore, in comparison to species richness, the relationships between evenness and other community properties, and between evenness and environmental gradients are not as well understood (Tuomisto, 2012; Su, 2018).
Evenness has a complex relationship with other community properties. First, evenness can decrease with richness because rich communities often have many rare species, and rare species make a disproportional contribution to richness but a negligible contribution to abundance (Stirling and Wilsey, 2001; Stevens and Willig, 2002). However, evenness can also increase with richness in contexts where resource acquisition is not monopolized by a few species (Stirling and Wilsey, 2001), or when there are many generalists across guilds within an assemblage (Perez-Torres, 2004). Second, evenness can decrease as community biomass increases in cases where a few species monopolize resource acquisition or if those species also withstand a greater variety of environmental conditions (Hillebrand et al., 2008). For example, in some marine systems, biomass has a negative effect on evenness because generalists at high trophic levels become larger and monopolize most resources (Maureaud et al., 2019). In contrast, evenness can increase with community biomass when additional species added to a community have complementary niches compared to the species already present (Drobner et al., 1998). However, the effect of biomass on evenness weakens and the effect of richness on evenness becomes greater when more species with similar niches are added to the community (Hillebrand et al., 2008). Therefore, the relationships between evenness and other community properties are not only complex, they also depend on multiple interactions among community properties.
The ways in which evenness is affected by environmental gradients is not straight forward either because gradients can also affect richness and community biomass simultaneously. At sites where temperature and rainfall have small fluctuations year-round (i.e., low seasonality-low stress), productivity is usually higher, more species coexist, and communities can accumulate more biomass (Weiher and Keddy, 1999). For example, richness and biomass across primate and bird communities increase with lower seasonality (Terborgh et al., 1990; Hanya et al., 2011). In turn, sites with higher richness and high biomass due to low seasonality usually show low evenness because rare species can make a disproportional effect on evenness, or because few species dominate and make up the largest proportion of the community (Mulder et al., 2004). Conversely, at sites where temperature and rainfall have large fluctuations year-round (i.e., high stress-high seasonality), a smaller number of species is able to withstand environmental fluctuations (Tello and Stevens, 2010; Steudel et al., 2012), and those species can have low biomass because resources are temporarily patchy (Rotenberry and Wiens, 1980; Rosenzweig, 1995). However, sites with lower richness and lower biomass due to high seasonality can show high evenness because differences in abundance among the few resistant species could be small (Drobner et al., 1998). For example, in neotropical bat communities, evenness and richness were negatively related because species richness reflects differences in resource availability across sites, whereas changes in evenness reflect variation in environmental stability (Stevens and Willig, 2002). Furthermore, other neotropical bat communities have showed that richness and biomass were negatively related to evenness because hotter and drier sites harbored less and smaller species, but abundances were less variable across those species (Estrada-Villegas et al., 2012). Even though environmental seasonality has a direct effect on richness and biomass, the direct and indirect effects of seasonality on evenness are poorly understood, and it is unclear how richness and biomass across different degrees of seasonality contribute to evenness.
To clarify how community properties affect evenness after accounting for the effect of environmental seasonality, we tested the hypothesis that evenness increases in communities that have low species richness and low biomass because the negative effect of richness and biomass on evenness is ultimately regulated by seasonality. Areas that experience stronger fluctuations in temperature have less rich communities (Stevens, 2013) and have lower biomass (Hanya et al., 2011). Therefore, we expect that community evenness is not only controlled by the interrelationship with richness and biomass, but ultimately by environmental seasonality. Testing this hypothesis brings us closer to more fully understanding how diversity changes across space, and specifically how evenness is related to other community properties and ultimately shaped by large scale environmental drivers.
Materials and Methods
Site Selection
We selected 49 bat communities from the ATLANTIC BATS data base (Muylaert et al., 2017; Figure 1). The Atlantic Forest spans more than 3,000 km from north to south in eastern South America, and transitions into the Chaco and Pantanal in the southwest portion of its range in Paraguay and Pampas to the south in Uruguay. Most of the selected sites are from forested areas of the Atlantic Forest sensu stricto, and only four sites are in the transition between the Atlantic Forests and the Cerrado (Supplementary Material 1). All bat surveys were conducted using mist nets in forested areas, either national parks, research stations, public forest reserves, or private forested land. Thus, data are comparable, and adequately represent the structure of local bat assemblages from forested areas across the Atlantic Forest. For details please see Muylaert et al. (2017).
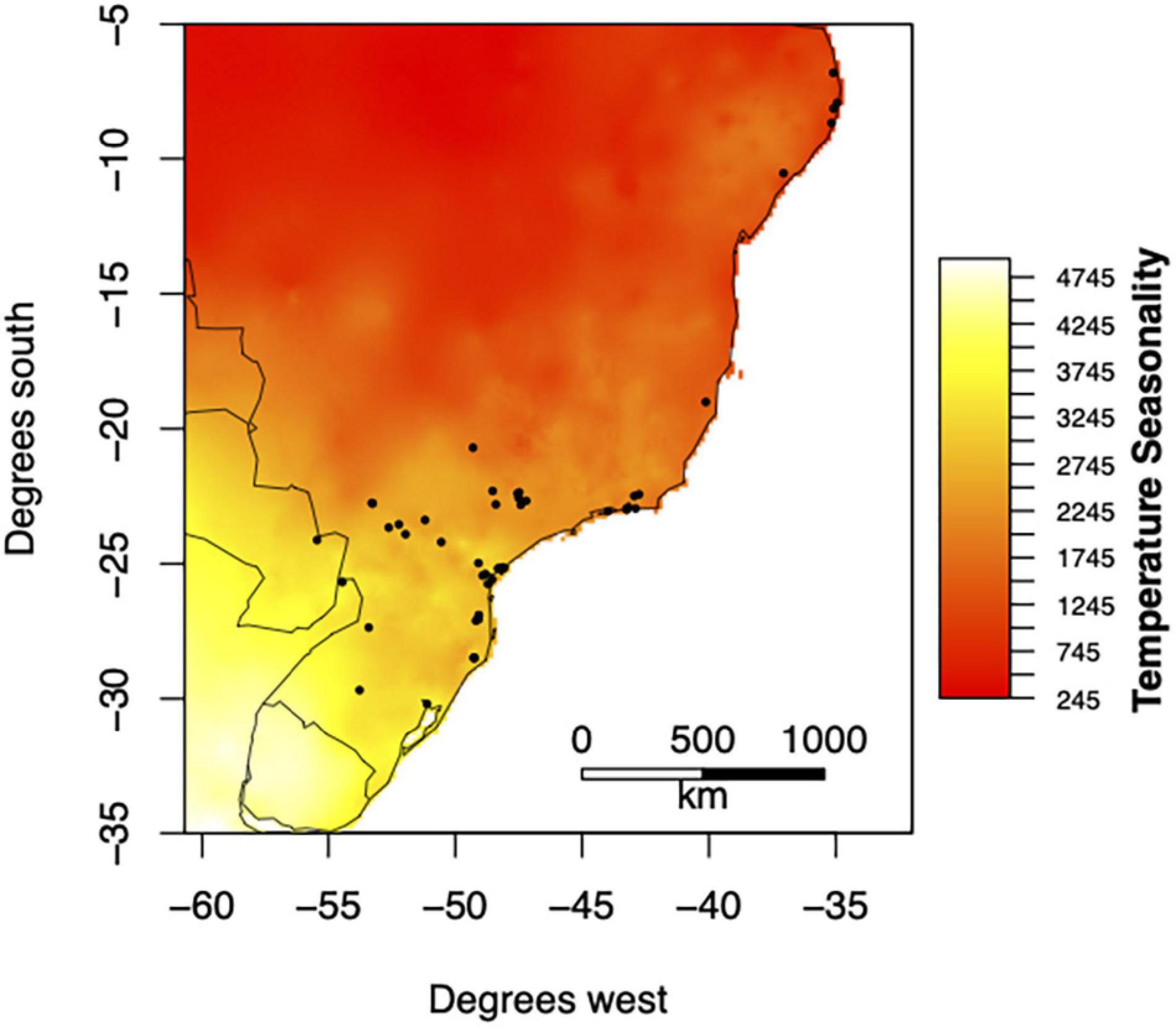
Figure 1. Geographic distribution of temperature seasonality across 49 sites from the ATLANTIC BATS data base (Muylaert et al., 2017). Temperature seasonality (SD X100) was obtained from WorldClim (Fick and Hijmans, 2017). Temperature seasonality, along with other variables of temperature and precipitation, were used to calculate the effect of environmental seasonality, richness, and biomass on community evenness.
We used the following criteria to select the aforementioned 49 communities. First, communities had to be surveyed during wet and dry seasons, as indicated in the ATLANTIC BATS data set (Muylaert et al., 2017). Second, sites had to be below 1,000 m.a.s.l. Third, the sampling effort to assess community structure had to be greater than 10,000 m2 h (sampling effort follows Straube and Bianconi, 2002). Fourth, we calculated sampling coverage according to Chao and Jost (2012), and selected communities that had a coverage greater than 0.8. Using coverage allowed us to select communities with a low probability of finding a new species given the sampled community (Chao and Jost, 2012). We acknowledge that other methods could have been used to select bat communities from the ATLANTIC BATS data set, however, selecting communities with high coverage, relatively high sampling effort, and sampled in both climatic seasons in forests below 1,000 m.a.s.l., allowed us enough replication without compromising reliability and representativeness.
Community Properties
For every community, we calculated three indices of evenness using Hill numbers. We calculated the Shannon-Weiner evenness J′ using q = 1 (Pielou, 1975; Jost, 2010), 1E, using q = 1 (Simpson, 1949; Tuomisto, 2012), and the inverse of Simpson dominance index D, denoted as 2E, using q = 2 (Simpson, 1949; Tuomisto, 2012). By using 1E and 2E, we calculated evenness by emphasizing abundance of common species, and abundance of the very abundant species, respectively (Tuomisto, 2012; Chao et al., 2014). We picked these three indices because they capture different ways in which evenness is related to richness and species’ relative abundances, and because they have a long history in ecology (e.g., Buzas and Hayek, 1996; Smith and Wilson, 1996; Weiher and Keddy, 1999; Wilsey and Stirling, 2007; Jost, 2010; Tuomisto, 2012). Finally, for every community we also calculated species richness (S) and average community biomass (M). Richness was calculated using the Hill number q = 0. We calculated average biomass per community by summing the multiplications of the abundances and the biomass of all the sampled species, and then dividing such multiplication by the total number of individuals per community. By using average instead of total biomass, we decoupled the effects of abundance from body size. We used species biomass (mass in grams) reported in the literature (Wilson and LaVal, 1974; Mies et al., 1996; Reid, 1997; Bernard, 2001; Santos et al., 2003; Smith et al., 2003; Haynes and Lee, 2004; Canals et al., 2005; Ortega and Alarcón-D, 2008; Jones et al., 2009; Oprea et al., 2009; Tavares and Velazco, 2010; Moratelli et al., 2011; Tejedor, 2011; Nogueira et al., 2012; Barquez et al., 2017; Verde et al., 2017; Cardoso, 2018; Velazco and Patterson, 2019; Giménez and Schiaffini, 2020) or from individuals captured in some of the selected communities (Pillatti, Esberard, and Moratelli, personal communication). We used the R package vegetarian to calculate all evenness indices.
Temperature Seasonality
To calculate environmental seasonality across sites, we used an approach similar to Stevens (2013). We first obtained 19 layers from WorldClim (Fick and Hijmans, 2017), and then calculated a principal component analysis (PCA) after scaling and centering all variables. After using the broken-stick stopping rule (Jackson, 1993), we concluded that the first four axes explained most of the variation across all climatic variables. We then employed a varimax rotation after constraining the analyses to four axes, retained the PCA scores for all sampling sites, and then selected the axis that explained most of the variance. We correlated the original values of the layers with the four axes after the varimax rotation to interpret the axes, and confirmed that the axis with the highest loading explained 47.1% of the variation across climatic variables. Moreover, this first axis was positively and highly correlated with annual temperature range, temperature seasonality and mean diurnal temperature range (Supplementary Material 2). In contrast, the second axis explained only 21% of the variance, and its correlation with precipitation variables was not as evident as the correlation between the first axis and temperature variables. We know that environmental seasonality, in particular temperature, accounts for a significant portion of the variation in species richness across the Atlantic Forest (Stevens, 2013). Therefore, we want to use a similar approach to determine how much environmental seasonality (i.e., temperature) regulates community evenness after accounting for the variation of community richness and biomass. To obtain and process WorldClim data we used the R packages raster, sp, gdalUtils and rgdal.
Statistical Analyses
We used piecewise structural equation modeling to evaluate the effect of environmental seasonality (Env), richness (S), and biomass (M) on evenness (J, 1E, and 2E). Piecewise structural equation modeling (Lefcheck, 2016) is an ideal method to evaluate our hypothesis because it maintains the causal relationships among variables from observational data, tests for the effect of an explanatory variable on a response variable, and accounts for the variation of more than one explanatory variable on the response variable (Lefcheck, 2016). Following the strategy of Estrada-Villegas et al. (2012), we created a set of models that incorporated different alternatives in which richness and biomass affect evenness after accounting for the effect of seasonality on richness and biomass. The first two models proposed sequential effects of uncorrelated explanatory variables while changing the order of the relationship between the variables. The third model included two simultaneous direct effects on evenness after accounting for the effect of seasonality on richness and biomass. The fourth and fifth models proposed chained effects of uncorrelated exploratory variables where richness affects biomass and vice versa. The second term in the fourth model included the expected negative correlation between richness and biomass (Isaac et al., 2005), and the second term in the fifth model included the expected positive correlation between biomass and richness (Wilsey and Potvin, 2000). The sixth and seventh models proposed an indirect effect of richness on biomass, and vice versa, before testing for a direct effect of richness and biomass on evenness. These seven models cover the most common theoretical expectations between richness, biomass, and evenness (Drobner et al., 1998; Stirling and Wilsey, 2001; Stevens and Willig, 2002; Mulder et al., 2004; Estrada-Villegas et al., 2012; Su, 2018; Maureaud et al., 2019), and account for the expected negative effects of environmental seasonality on richness and biomass (Terborgh et al., 1990; Hanya et al., 2011; Stevens, 2013). For comparison, we also calculated a “null” path model with the direct effect of environmental seasonality on evenness.
We used two approaches to select the model that best described our data; we first calculated AIC for each model, and then calculated a test of goodness-of-fit based on “tests of directed separation” (Lefcheck, 2016). The conditional independence of the proposed relationships is tested with a combined Fischer’s C test, and its corresponding P-value. P-values greater than 0.05 indicate that the model provides a good fit to the data (Lefcheck, 2016). Therefore, we selected the model that had the lowest AIC, and a P-value greater than 0.05. Moreover, we calculated standardized coefficients to determine the direction and magnitude of the direct and indirect effects onto evenness. Standardized coefficients are necessary because the units among the PCA scores, richness, biomass and evenness are not the same. To calculate piecewise structural equation models we used the functions even_psem from the package piecewiseSEM (Lefcheck, 2016). Following the “t-rule,” the “null” path model has an equal number of unknown and known parameters. Thus the “null” path model is saturated with zero degrees of freedom, and no Fisher’s C score and P-value could be calculated.
Results
Community evenness significantly increased with environmental seasonality for two out of three indices of evenness (Supplementary Material 3). While there was not a significant relationship between J′ and environmental seasonality, 1E and 2E significantly increased with environmental seasonality (Supplementary Material 3). The most parsimonious models across all measures of evenness indicate that greater environmental seasonality reduced richness, and in two out of the three best models, we found that an increase in richness elicited a reduction in community evenness (Table 1). Our results for J′ indicate a weak effect of richness on evenness, whereas richness had a significantly negative effect on 1E and 2E (Table 1). Environmental seasonality always had a negative significant effect on richness. The second most parsimonious model across all measures of evenness was not always the same. For J′, greater environmental seasonality decreased biomass, although not significantly, but greater biomass significantly decreased evenness (Table 1). However, for 1E, there was an independent significant negative effect of richness on evenness, after accounting for a significant negative effect of environmental seasonality on richness and biomass, and a significant negative indirect effect of biomass on richness (Table 1). In other words, when environmental seasonality increased, both richness and biomass decreased, and lower richness produced an increase in evenness. These results coincide with those found for the best models for J′. Finally, the second-best model for 2E also indicates that richness had a direct and negative effect on evenness, with biomass having an indirect effect on evenness given its direct and negative effect on richness (Table 1). Again, where environmental seasonality was higher, richness declined and evenness increased.
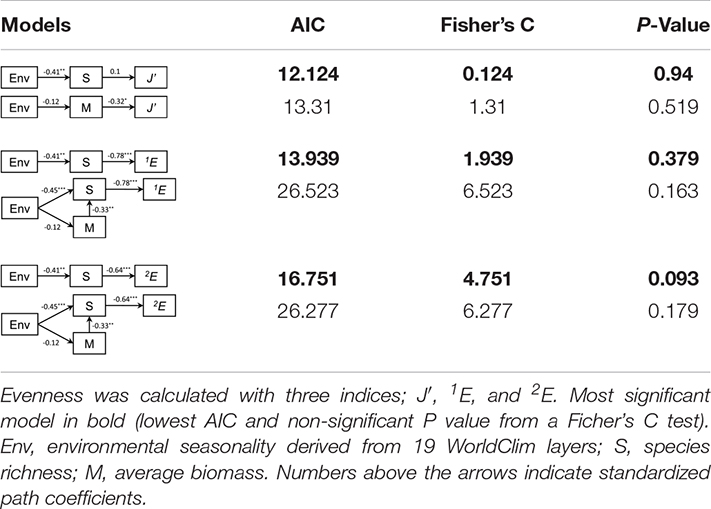
Table 1. Best path models to explain the effect of environmental seasonality, species richness, and average biomass on community evenness.
Discussion
Our results show that environmental seasonality has an important effect on evenness and supports the hypothesis that evenness is driven by richness and biomass. More importantly, our results indicate that evenness is ultimately controlled by the negative effect of environmental seasonality on richness and biomass. Community evenness is higher in sites that have low species richness and low biomass because greater seasonal fluctuations in climate, particularly temperature, seem to reduce richness and biomass (Weiher and Keddy, 1999; Stevens and Willig, 2002; Hanya et al., 2011; Stevens, 2013; Alroy, 2019). The best models across all three measures of evenness indicate that more seasonal climate reduces richness, and lower species richness increases evenness. The models with the second-best support suggest that a decline in biomass with increasing seasonality also increases evenness. We concur with Passy et al. (2017) that the relationship between richness and evenness is driven by environmental factors, where an increase in environmental stress (seasonality in our case) eliminates less tolerant species, leaving only generalists that are able to persist in more stressful environments. Our findings help clarify the complex relationships between evenness and other community properties, and how evenness changes across environmental gradients at local (Weiher and Keddy, 1999; Wilsey and Potvin, 2000) and regional scales (Estrada-Villegas et al., 2012).
Our results contrast with Tramer (1969), who predicted that communities from seasonal environments will vary in diversity according to their relative abundance distributions (i.e., evenness), whereas communities in less seasonal environments will vary in terms of richness. Instead, we have shown that environmental seasonality will elicit variation in richness, and this elicits variation in evenness. Our findings also contrast with the results from Rotenberry (1978), who showed that environmental seasonality decreased evenness in temperate bird communities, with no significant change in richness along the same gradient. However, the gradient that Rotenberry (1978) evaluated is less steep (≈7° of latitudinal change) compared to the bat communities across the Atlantic Forests (28.15° of latitudinal change). Other studies of bird communities also contrast with our findings; in a comparison between temperate and tropical bird communities, Karr (1971) found that the abundance of the two most common species, when compared to the abundance of rest of the community, was greater in communities in more seasonal climates than in communities in less seasonal climates, suggesting greater dominance (i.e., lower evenness) in more seasonal sites. However, Karr (1971) showed that the number of rare species in more seasonal environments was lower than in less seasonal environments, a result that concurs with our study.
A decrease in resource specialization coupled with a decrease in rarity can help explain the negative relationship between seasonality, richness, and evenness. Species in variable environments use a large spectrum of resources, whereas species in more constant environments use a smaller spectrum (MacArthur, 1972). When resource availability is more consistent across time, like in areas with more stable climate, more species can specialize on a particular subset of resources (MacArthur, 1972; Terborgh, 1973). Specialists, by the very nature of specializing on a narrow range of resources, are rare (i.e., few number of individuals, Gaston, 1994). In more seasonal areas, however, resources are patchy temporally and only the species that use a wider spectrum of resources are able to thrive. By contrast, seasonality promotes fluctuations in abundance of populations of specialists (i.e., rare species) ultimately contributing to their local extinction (MacArthur, 1972; Gaston, 1994). Therefore, as seasonality increases, species richness decreases, and evenness increases because specialist and/or rare species are filtered out. In fact, we observe a reduction in rarity as seasonality increases in our data set. When we inspect the matrix of species occurrences across sites ordered by their environmental seasonality value, we see that more seasonal sites tend to have fewer species, and those rare ones are in less seasonal sites (Supplementary Material 4).
By extension, a decrease in rarity due to higher seasonality should be evidenced across guilds because some species may be specialized to smaller subset of resources within guilds (Winemiller and Pianka, 1990). Indeed, this seems to be case in our data. Further inspection of the occurrence matrix suggests that a decrease in rarity also occurs across guilds (Supplementary Material 4). For example, species from the large frugivorous guild, like Vampyrodes caraccioli and Chiroderma villosum, seemed to be filtered out from more seasonal sites. The same seems to occur for smaller frugivores (e.g., Rhinophylla pumilio and Artibeus cinereus), smaller gleaners (e.g., Lampronycteris brachyotis and Lophostoma brasiliense), larger gleaners (e.g., Mimon crenulatum and Tonatia saurophila), nectarivores (e.g., Lonchophylla peracchii), and sanguivorous (e.g., Diaemus youngii). We acknowledge that a more thorough analysis would be necessary to clarify how rarity decreases with seasonality across guilds, but these preliminary observations suggest that more seasonal environments filter out rare species within guilds, supporting the inference that a decrease in richness elicits an increase in evenness in more seasonal sites.
Finally, niche space and diversification rates may partially explain why average biomass decreases evenness directly or indirectly via a reduction in species richness across communities. Greater diversification rates lead to greater species richness (Scholl and Wiens, 2016); a pattern evidenced in mammals, including bats (Rolland et al., 2014), and South American frugivorous bats (Rojas et al., 2012). Moreover, average biomass (i.e., body size) has been found to be negatively related to species richness (Isaac et al., 2005; Rojas et al., 2012), as we found in our study, suggesting that sites with high species richness thanks to high diversification rates also harbor many species of smaller size. In our case, not only does higher seasonality have a negative effect on species richness, which decreases evenness due to resources specialization and rarity, but sites that have higher biomass have less bat species, which increases evenness. Lastly, we propose that the negative effect of biomass on evenness can be due to an expansion of niche space, which in turn allows more species to be added to communities. Using biomass as a dimension of the niche, larger niche space may allow greater niche separation among species and greater species richness. As a result, communities can have more rare species, some with larger average biomass but many with smaller body sizes, thus the negative relationship between average biomass and richness. In sum, the relationship between evenness and biomass, both in terms of total community biomass (Maureaud et al., 2019) or average biomass (our study) seems to be negative, as suggested by other studies with plants and birds (Cotgreave and Harvey, 1994; Drobner et al., 1998). However, other studies have found the opposite trends (Wilsey and Potvin, 2000; Magurran et al., 2013). Therefore, future analyses should decouple the effect of species abundances on richness and biomass to elucidate further why evenness decreases with biomass, either average or total, across changes of environmental seasonality.
In conclusion, environmental seasonality is a major driver of richness, biomass and evenness in bat communities across the Atlantic Forests. Evenness of neotropical bat communities seems to be controlled by environmental seasonality via a reduction in species richness and a decrease in average biomass. Our study contributes to the clarification of how community properties change across large spatial scales and broad environmental gradients, and brings us closer to a unified theory of evenness. Moreover, our results show how community properties affect evenness, and clearly indicates that environmental seasonality ultimately drives community evenness by controlling species richness and biomass.
Data Availability Statement
Publicly available datasets were analyzed in this study. This data can be found here: https://doi.org/10.1002/ecy.2007.
Author Contributions
SE-V designed the study, performed statistical analyzes, and wrote the manuscript. JP-T, BM, and RS provided substantial contributions to the interpretation of the results and helped to wrote portions of the manuscript. All authors contributed to the article and approved the submitted version.
Funding
This project was funded by the Cullman Fellowship from the Yale School of the Environment and the New York Botanical Garden awarded to SE-V.
Conflict of Interest
The authors declare that the research was conducted in the absence of any commercial or financial relationships that could be construed as a potential conflict of interest.
Publisher’s Note
All claims expressed in this article are solely those of the authors and do not necessarily represent those of their affiliated organizations, or those of the publisher, the editors and the reviewers. Any product that may be evaluated in this article, or claim that may be made by its manufacturer, is not guaranteed or endorsed by the publisher.
Acknowledgments
We thank Patricia Pillatti, Carlos Esberard, and Ricardo Moratelli for biomass data on Lonchophylla peracchii. We also thank Renata Muylaert and her co-authors for compiling the ATLANTIC BATS data set. Joe LaManna provided constructive criticisms to the manuscript.
Supplementary Material
The Supplementary Material for this article can be found online at: https://www.frontiersin.org/articles/10.3389/fevo.2022.839384/full#supplementary-material
Supplementary Material 1 | List of study sites and their descriptors. We selected sites that had a sampling effort greater than 10,000 m2 h (sampling effort calculated following Straube and Bianconi, 2002) and a sampling coverage > 0.8 according to Chao and Jost (2012). For site IDs and descriptors, please see the ATLANTIC BATS data base (Muylaert et al., 2017) (https://doi.org/10.6084/m9.figshare.16688848.v2).
Supplementary Material 2 | Correlations between original values from the 19 WorldClim layers (Fick and Hijmans, 2017) and the four axes selected from the PCA. PCA axis 1 explained 47.1% of the variation across climatic variables and shows a gradient of temperature seasonality across sampling sites throughout the Atlantic Forest (https://doi.org/10.6084/m9.figshare.16688848.v2).
Supplementary Material 3 | List of all path models for evenness indices J′, 1E, and 2E. Most significant model in bold (lowest AIC and non-significant P value from a Ficher’s C test). Env, environmental seasonality derived from 19 WorldClim layers; S, species richness; M, average biomass. Numbers above the arrows are standardized path coefficients. The “null” path model is saturated with zero degrees of freedom, and no Fisher’s C score and P-value could be calculated. Therefore, the “null” model at the bottom of the table for comparison (https://doi.org/10.6084/m9.figshare.16688848.v2).
Supplementary Material 4 | Matrix of species occurrences across sites. Sites are ordered by environmental seasonality; sites with less seasonable climate have more rare species (left side of the matrix) compared to sites with more seasonable climate (right side of the matrix). Env.var, Environmental seasonality; ID, Site ID according to Muylaert et al. (2017). Environmental seasonality is the first axis from a PCA that accounted for 47.1% of the variation across climatic variables. Higher values indicate sites that experience greater temperature seasonality, lower mean annual temperature, and warmest and coldest quarters (https://doi.org/10.6084/m9.figshare.16688848.v2).
References
Alroy, J. (2019). Latitudinal gradients in the ecology of New World bats. Glob. Ecol. Biogeogr. 28, 784–792. doi: 10.1111/geb.12892
Barquez, R. M., Miotti, M. D., Idoeta, F. M., and Díaz, M. M. (2017). Two new species of Myotis (Chiroptera: Vespertilionidae) for Argentina. Pap. Avuls. Zool. 57, 287–294. doi: 10.11606/0031-1049.2017.57.22
Bernard, E. (2001). Vertical stratification of bat communities in primary forests of Central Amazon. Brazil J. Trop. Ecol. 17, 115–126. doi: 10.1017/S0266467401001079
Buzas, M. A., and Hayek, L.-A. C. (1996). Biodiversity Resolution: An Integrated Approach. Biodiver. Lett. 3, 40–43. doi: 10.2307/2999767
Canals, M., Atala, C., Grossi, B., and Iriarte-Díaz, J. (2005). Relative size of hearts and lungs of small bats. Acta Chiropt. 7, 65–72. doi: 10.3161/1508110054527777
Cardoso, V. (2018). Morcegos do Parque Estadual Carlos Botelho, Sudeste da Mata Atlântica: Taxonomia e Saúde Ambiental. Brazil: Unviersidade Federal de São Carlos.
Chao, A., Chiu, C.-H., and Jost, L. (2014). Unifying Species Diversity, Phylogenetic Diversity, Functional Diversity, and Related Similarity and Differentiation Measures Through Hill Numbers. Annu. Rev. Ecol., Evol. Systemat. 45, 297–324. doi: 10.1146/annurev-ecolsys-120213-091540
Chao, A., and Jost, L. (2012). Coverage-based rarefaction and extrapolation: standardizing samples by completeness rather than size. Ecology 93, 2533–2547. doi: 10.1890/11-1952.1
Cotgreave, P., and Harvey, P. H. (1994). Evenness of Abundance in Bird Communities. J. Anim. Ecol. 63, 365–374. doi: 10.2307/5554
Drobner, U., Bibby, J., Smith, B., and Wilson, J. B. (1998). The relation between community biomass and evenness: what does community theory predict, and can these predictions be tested? Oikos 82, 295–302. doi: 10.2307/3546969
Estrada-Villegas, S., Mcgill, B. J., and Kalko, E. K. V. (2012). Determinants of species evenness in a neotropical bat ensemble. Oikos 121, 927–941. doi: 10.1111/j.1600-0706.2011.19837.x
Fick, S. E., and Hijmans, R. J. (2017). WorldClim 2: new 1-km spatial resolution climate surfaces for global land areas. Int. J. Climatol. 37, 4302–4315. doi: 10.1002/joc.5086
Giménez, A. L., and Schiaffini, M. I. (2020). Patagonian bats: new size limits, southernmost localities and updated distribution for Lasiurus villosissimus and Myotis dinellii (Chiroptera: Vespertilionidae). Mammalia 84, 150–161. doi: 10.1515/mammalia-2019-0024
Hanya, G., Stevenson, P., Van Noordwijk, M., Te Wong, S., Kanamori, T., Kuze, N., et al. (2011). Seasonality in fruit availability affects frugivorous primate biomass and species richness. Ecography 34, 1009–1017. doi: 10.1111/j.1600-0587.2010.06775.x
Haynes, M. A., and Lee, T. E. Jr. (2004). Artibeus obscurus (Chiroptera: Phyllostomidae). Mamm. Species 752, 1–5. doi: 10.1644/752
Hillebrand, H., Bennett, D. M., and Cadotte, M. W. (2008). Consequences of dominance: A review of evenness effects on local and regional ecosystem processes. Ecology 89, 1510–1520. doi: 10.1890/07-1053.1
Hurlbert, S. H. (1971). The Nonconcept of Species Diversity: A Critique and Alternative Parameters. Ecology 52, 577–586. doi: 10.2307/1934145
Isaac, N. J. B., Jones, K. E., Gittleman, J. L., and Purvis, A. (2005). Correlates of Species Richness in Mammals: Body Size, Life History, and Ecology. Am. Nat. 165, 600–607. doi: 10.1086/429148
Jackson, D. A. (1993). Stopping Rules in Principal Components Analysis: A Comparison of Heuristical and Statistical Approaches. Ecology 74, 2204–2214. doi: 10.2307/1939574
Jones, K. E., Bielby, J., Cardillo, M., Fritz, S. A., O’dell, J., Orme, C. D. L., et al. (2009). PanTHERIA: a species-level database of life history, ecology, and geography of extant and recently extinct mammals. Ecology 90, 2648–2648. doi: 10.1890/08-1494.1
Jost, L. (2010). The Relation between Evenness and Diversity. Diversity 2, 207–232. doi: 10.3390/d2020207
Karr, J. R. (1971). Structure of avian communities in selected Panama and Illinois habitats. Ecol Monogr. 41, 207–233. doi: 10.2307/1942366
Lefcheck, J. S. (2016). piecewiseSEM: Piecewise structural equation modelling in r for ecology, evolution, and systematics. Methods Ecol. Evol. 7, 573–579. doi: 10.1111/2041-210x.12512
MacArthur, R. H. (1972). Geographical ecology: patterns in the distribution of species. New Jersey: Princeton University Press.
Magurran, A. E., Queiroz, H. L., and Hercos, A. P. (2013). Relationship between evenness and body size in species rich assemblages. Biol. Lett. 9:20130856. doi: 10.1098/rsbl.2013.0856
Maureaud, A., Hodapp, D., Van Denderen, P. D., Hillebrand, H., Gislason, H., Dencker, T. S., et al. (2019). Biodiversity-ecosystem functioning relationships in fish communities: biomass is related to evenness and the environment, not to species richness. Proc. R. Soc. B. 286:20191189. doi: 10.1098/rspb.2019.1189
Mies, R., Kurta, A., and King, D. G. (1996). Eptesicus furinalis. Mamm. Species. 526, 1–7. doi: 10.2307/3504316
Moratelli, R., Peracchi, A. L., Dias, D., and De Oliveira, J. A. (2011). Geographic variation in South American populations of Myotis nigricans (Schinz, 1821) (Chiroptera, Vespertilionidae), with the description of two new species. Mamm. Biol. 76, 592–607. doi: 10.1016/j.mambio.2011.01.003
Mulder, C. P. H., Bazeley-White, E., Dimitrakopoulos, P. G., Hector, A., Scherer-Lorenzen, M., and Schmid, B. (2004). Species evenness and productivity in experimental plant communities. Oikos 107, 50–63. doi: 10.1111/j.0030-1299.2004.13110.x
Muylaert, R. D. L., Stevens, R. D., Esbérard, C. E. L., Mello, M. A. R., Garbino, G. S. T., Varzinczak, L. H., et al. (2017). ATLANTIC BATS: a data set of bat communities from the Atlantic Forests of South America. Ecology 98, 3227–3227. doi: 10.1002/ecy.2007
Nogueira, M. R., Lima, I. P., Peracchi, A. L., and Simmons, N. B. (2012). New Genus and Species of Nectar-Feeding Bat from the Atlantic Forest of Southeastern Brazil (Chiroptera: Phyllostomidae: Glossophaginae). Am. Museum Novit. 30, 1–30. doi: 10.1206/3747.2
Oprea, M., Aguliar, L. M. S., and Wilson, D. E. (2009). Anoura caudifer (Chiroptera: Phyllostomidae). Mamm. Species 844, 1–8. doi: 10.1644/844.1
Ortega, J., and Alarcón-D, I. (2008). Anoura geoffroyi (Chiroptera: Phyllostomidae). Mamm. Species 2008, 1–7. doi: 10.1644/818.1
Passy, S. I., Bottin, M., Soininen, J., and Hillebrand, H. (2017). Environmental filtering and taxonomic relatedness underlie the species richness–evenness relationship. Hydrobiologia 787, 243–253. doi: 10.1007/s10750-016-2968-3
Perez-Torres, J. (2004). Dinámica del ensamblaje de murciélagos en respuesta a la fragmentación en bosques nublados: un modelo de ecuaciones estructurales. [Ph.D.thesis]. Colombia: Pontificia Universidad Javeriana.
Reid, F. A. (1997). A field guide to the mammals of Central America and Southeast Mexico. New York, NY: Oxford University Press.
Rojas, D., Vale, Á, Ferrero, V., and Navarro, L. (2012). The role of frugivory in the diversification of bats in the Neotropics. J. Biogeogr. 39, 1948–1960. doi: 10.1111/j.1365-2699.2012.02709.x
Rolland, J., Condamine, F. L., Jiguet, F., and Morlon, H. (2014). Faster Speciation and Reduced Extinction in the Tropics Contribute to the Mammalian Latitudinal Diversity Gradient. PLoS Biol. 12:e1001775. doi: 10.1371/journal.pbio.1001775
Rosenzweig, M. L. (1995). Species Diversity in Space and Time. Cambridge: Cambridge University Press.
Rotenberry, J. T. (1978). Components of Avian Diversity Along a Multifactorial Gardient. Ecology 59, 693–699. doi: 10.2307/1938772
Rotenberry, J. T., and Wiens, J. A. (1980). Habitat Structure, Patchiness, and Avian Communities in North American Steppe Vegetation: A Multivariate Analysis. Ecology 61, 1228–1250. doi: 10.2307/1936840
Santos, M., Aguirre, L. F., Vázquez, L. B., and Ortega, J. (2003). Phyllostomus hastatus (Chiroptera: Phyllostomidae). Mamm. Species 722, 1–6. doi: 10.1644/0.722.1
Scholl, J. P., and Wiens, J. J. (2016). Diversification rates and species richness across the Tree of Life. Proc. R. Soc. B. 283:20161334. doi: 10.1098/rspb.2016.1334
Smith, B., and Wilson, J. B. (1996). A Consumer’s Guide to Evenness Indices. Oikos 76, 70–82. doi: 10.2307/3545749
Smith, F. A., Lyons, S. K., Ernest, S. K. M., Jones, K. E., Kaufman, D. M., Dayan, T., et al. (2003). Body mass of late quaternary mammals. Ecology 84, 3403–3403. doi: 10.1890/02-9003
Steudel, B., Hector, A., Friedl, T., Löfke, C., Lorenz, M., Wesche, M., et al. (2012). Biodiversity effects on ecosystem functioning change along environmental stress gradients. Ecol. Lett. 15, 1397–1405. doi: 10.1111/j.1461-0248.2012.01863.x
Stevens, R. D. (2013). Gradients of Bat Diversity in Atlantic Forest of South America: Environmental Seasonality, Sampling Effort and Spatial Autocorrelation. Biotropica 45, 764–770. doi: 10.1111/btp.12056
Stevens, R. D., and Willig, M. R. (2002). Geographical Ecology at the Community Level: Perspectives on the Diversity of New World Bats. Ecology 83, 545–560. doi: 10.1890/0012-96582002083[0545:GEATCL]2.0.CO;2
Stirling, G., and Wilsey, B. (2001). Empirical Relationships between Species Richness, Evenness, and Proportional Diversity. Am. Nat. 158, 286–299. doi: 10.1086/321317
Straube, F. C., and Bianconi, G. V. (2002). Sobre a grandeza e a unidade utilizada para estimar esforço de captura com utilização de redes-de-neblina. Chirop. Neotrop. 8, 150–152.
Su, Q. (2018). A relationship between species richness and evenness that depends on specific relative abundance distribution. PeerJ 6:e4951. doi: 10.7717/peerj.4951
Tavares, V. D. C., and Velazco, P. M. (2010). Platyrrhinus recifinus (Chiroptera: Phyllostomidae). Mamm. Species 42, 119–123. doi: 10.1644/859.1
Tejedor, A. (2011). Systematics of Funnel-Eared Bats (Chiroptera: Natalidae). Bull. Am. Museum Nat. Hist. 2011, 1–140. doi: 10.1206/636.1
Tello, J. S., and Stevens, R. D. (2010). Multiple environmental determinants of regional species richness and effects of geographic range size. Ecography 33, 796–808. doi: 10.1111/j.1600-0587.2009.05991.x
Terborgh, J., Robinson, S. K., Parker, T. A. III, Munn, C. A., and Pierpont, N. (1990). Structure and Organization of an Amazonian Forest Bird Community. Ecol Monogr 60, 213–238. doi: 10.2307/1943045
Tramer, E. J. (1969). Bird Species Diversity: Components of Shannon’s Formula. Ecology 50, 927–929. doi: 10.2307/1933715
Tuomisto, H. (2012). An updated consumer’s guide to evenness and related indices. Oikos 121, 1203–1218. doi: 10.1111/j.1600-0706.2011.19897.x
Velazco, P. M., and Patterson, B. D. (2019). Small Mammals of the Mayo River Basin in Northern Peru, with the Description of a New Species of Sturnira (Chiroptera: Phyllostomidae). Bull. Am. Museum Nat. Hist. 2019, 1–70. doi: 10.1206/0003-0090.429.1.1
Verde, R. D. S., Santos, F. C. B. D., Silva, R. D. C., and Calouro, A. M. (2017). First record of Lasiurus blossevillii Lesson & Garnot, 1826 (Chiroptera: Vespertilionidae) in the state of Acre, southwest of the Amazon. Brazil Oecol. Aust. 21, 191–196. doi: 10.4257/oeco.2017.2102.09
Weiher, E., and Keddy, P. A. (1999). Relative abundance and evenness patterns along diversity and biomass gradients. Oikos 87, 355–361.
Wilsey, B., and Stirling, G. (2007). Species richness and evenness respond in a different manner to propagule density in developing prairie microcosm communities. Plant Ecol. 190, 259–273. doi: 10.1007/s11258-006-9206-4
Wilsey, B. J., and Potvin, C. (2000). Biodiversity and ecosystem functioning: Importance of species evenness in an old field. Ecology 81, 887–892. doi: 10.1890/0012-96582000081[0887:BAEFIO]2.0.CO;2
Wilson, D. E., and LaVal, R. K. (1974). Myotis nigricans. Mammalian Species 39:1. doi: 10.2307/3503847
Keywords: Shannon-Weiner evenness, sampling coverage, climatic variability, Atlantic Forest, South America, Chiroptera, ATLANTIC BATS
Citation: Estrada-Villegas S, Pérez-Torres J, McGill BJ and Stevens RD (2022) Environmental Seasonality Regulates Community Evenness in Neotropical Bat Communities. Front. Ecol. Evol. 10:839384. doi: 10.3389/fevo.2022.839384
Received: 19 December 2021; Accepted: 23 February 2022;
Published: 22 March 2022.
Edited by:
Rubén G. Mateo, Autonomous University of Madrid, SpainReviewed by:
William Douglas Carvalho, Federal University of Grande Dourados, BrazilDaniel Pinero, National Autonomous University of Mexico, Mexico
Copyright © 2022 Estrada-Villegas, Pérez-Torres, McGill and Stevens. This is an open-access article distributed under the terms of the Creative Commons Attribution License (CC BY). The use, distribution or reproduction in other forums is permitted, provided the original author(s) and the copyright owner(s) are credited and that the original publication in this journal is cited, in accordance with accepted academic practice. No use, distribution or reproduction is permitted which does not comply with these terms.
*Correspondence: Sergio Estrada-Villegas, c2VyZ2lvLmVzdHJhZGF2aWxsZWdhc0B5YWxlLmVkdQ==