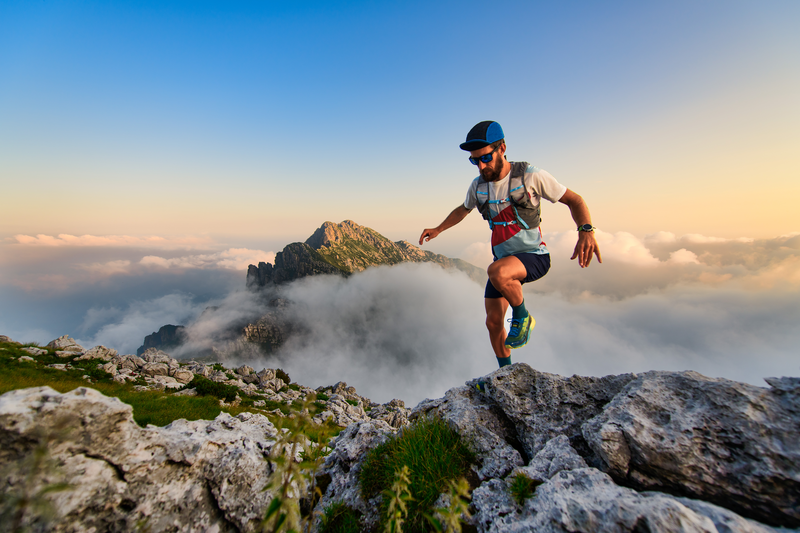
95% of researchers rate our articles as excellent or good
Learn more about the work of our research integrity team to safeguard the quality of each article we publish.
Find out more
BRIEF RESEARCH REPORT article
Front. Ecol. Evol. , 01 March 2022
Sec. Behavioral and Evolutionary Ecology
Volume 10 - 2022 | https://doi.org/10.3389/fevo.2022.837790
This article is part of the Research Topic Optimal Bird Migration: Implications for Navigation, Physiology, and Stopover Ecology View all 8 articles
Refueling performance is the primary currency of a successful migration as birds must maintain energy stores to achieve an optimal travel schedule. Migrating birds can anticipate heightened energy demand, not to mention increased uncertainty that energy demands will be satisfied, especially within an urbanizing landscape following long-distance flights. We tested the expectation that refueling performance of songbirds is reduced as densities increase at stopover sites in an urbanizing coastline of the Gulf of Mexico. We measured the density of migrating birds, their refueling performance, and arthropod abundance in two large tracts of contiguous forest paired with two small isolated patches embedded within residential settings throughout spring migration over the course of 2 years. Refueling performance declined with increasing migrant densities, even though the overall daily densities of birds stopping in these landscapes were relatively low and arthropod densities were low throughout. Habitat patch size alone did not account for differences in refueling performance, but smaller habitat patches more often concentrated migrants in higher densities where they experienced reduced refueling performance. We found support for density-dependent refueling performance during spring migration through a region where overall passage and stopover densities are low; suggesting that larger contiguous forest tracks within urban landscapes provide higher quality habitat for refueling and that effect is likely even more pronounced in landscapes within higher density migratory corridors. The nutritional challenges encountered during migration influence the overall pace of migration and changes in access to food resources due to increasing urbanization may ultimately impact optimal travel schedules.
How migratory birds are responding to urbanizing landscapes during passage has received surprisingly little attention, despite the fact that long-distance, intercontinental migratory birds are unlikely to be able to avoid urbanizing landscapes during passage (Rodewald and Matthews, 2005; Matthews and Rodewald, 2010; Seewagen et al., 2010, 2011). Moreover, migrating birds are traveling through increasingly light−polluted skies that disrupt visual cues, attracting them to urban areas where they stopover in high densities (La Sorte et al., 2017; Van Doren et al., 2017; McLaren et al., 2018; Horton et al., 2019). Suitable stopover habitat in urban areas may be highly fragmented into small patches (McKinney, 2002) wherein migrants may experience elevated competitor density with negative consequences for meeting energy demands (Moore and Yong, 1991). These conditions are magnified when habitat is within an urbanizing landscape along the edge of an ecological barrier or imbedded within inhospitable terrain (Shochat et al., 2002; Cohen et al., 2017). Coastlines, for example, are generally characterized by a fragmented landscape and reduced habitat quality (Simons et al., 2000; Buler and Moore, 2011), increased predation pressure (Lindstrom, 1990; Aborn, 1994; Cimprich et al., 2005; Zenzal et al., 2013; McCabe and Olsen, 2015), and increased competition for resources because edges often concentrate energy-constrained migrants (Buler and Moore, 2011); not to mention the increased possibility of being displaced into an inhospitable landscape where survival probability shrinks (Ward et al., 2018).
The objective of this study was to measure refueling performance of migratory songbirds stopping over in forested patches within an urbanizing landscape along the northern coast of the Gulf of Mexico during spring passage. The Gulf of Mexico is a prominent feature of the Nearctic-Neotropical migration system, with millions of birds stopping in habitats along the northern gulf coast during both spring and fall passage (Cohen et al., 2021). Although distribution patterns in this region suggest that migratory birds select hardwood forest during both spring and fall (Buler et al., 2007; Cohen et al., 2021), where fuel deposition rates are expected to be positively correlated with amount of forested cover in the landscape (Rodewald and Brittingham, 2007; Ktitorov et al., 2008; Cohen et al., 2014), spring migrants are under strong extrinsic constraints after crossing the Gulf of Mexico (i.e., energetic demand and adverse weather) and stop in high densities in close proximity to the coast (Lafleur et al., 2016; Clipp et al., 2020; Cohen et al., 2021). Although large forested patches may provide more suitable habitat than smaller isolated patches for most long-distance migrants, en route migrant-habitat associations within fragmented, urbanizing landscapes are poorly understood, particularly during initial stopover when birds are energetically constrained and opportunity for habitat selection is limited.
Fuel deposition rate (hereafter, refueling performance) is the primary currency of a successful migration as birds must efficiently utilize and maintain energy stores to maximize speed of migration and achieve an optimal travel schedule (Alerstam and Lindström, 1990; Alerstam, 2011). In spring, migrants often arrive in “waves” along the northern gulf coast with large numbers of energy-depleted birds distributed among available habitats (Cohen et al., 2017) where they have little information about habitat quality (Németh and Moore, 2007, 2014). Smaller, isolated forest fragments may concentrate higher densities of migrants than larger patches (Packett and Dunning, 2009; Keller and Avery, 2014), and these higher densities may intensify competition, which may reduce opportunity for a migrant to replenish depleted energy stores (Moore and Yong, 1991; Kelly et al., 2002; Moore et al., 2003). We tested the expectation that refueling performance declines with migrant density in an urbanizing coastal landscape. We tested this expectation with measures of refueling performance from individual migrants, the density of migrants, and arthropod occurrence and abundance within paired small and large forested habitat patches along the Mississippi coastline.
We assessed density dependent refueling at four study areas along the Mississippi coast. Two similarly sized, large tracts of contiguous forest were paired with two small forest patches embedded within residential settings (Figure 1). The larger sites are the Davis Bayou Area (DAV) of the Gulf Islands National Seashore (30° 23′ 31.17″, 88° 47′ 27.50″) and Shepard State Park (SHE) near the city of Gautier (30° 22′ 31.32″, 88° 37′ 57.12″). Small sites are approximately 0.5- hectare woodlots at Hellmers Lane (HEL) in the town of Ocean Springs (30° 24′ 26.46″, 88° 49′ 27.98″) and “Don’s Woods” in the town of Pascagoula (30° 20′ 51.49″, 88° 33′ 4.31″; Figure 1). All of the sites were wooded with similar composition of the same abundant tree species, including oaks (Quercus spp.), pines (Pinus spp.), sweetgum (Liquidambar styraciflua), and magnolia (Magnolia spp.), and shrub species including gallbery (Ilex glabra) and yaupon (Ilex vomitoria). Developed land (NLCD 2006) comprised more of the 1-km area surrounding the smaller patches (78.68 and 96.15% HEL and DON, respectively) as compared to the larger patches (38.23 and 65.31% DAV and SHE, respectively) while forested and woody wetland comprised more of area around the larger patches (22.10 and 16.91% DAV and SHE, respectively) as compared to the smaller patches (11.46 and 1.51% HEL and DON, respectively). The canopy cover was > 85% at all sites and higher at the large sites (90.5 ± 1.18 and 91.23 ± 0.63% DAV and SHE, respectively) as compared to the small sites (86.21 ± 1.46 and 88.76 ± 1.59% HEL and DON, respectively). Similarly, the canopy height was > 18 m at all sites and higher at the larger sites (24.99 ± 2.46 and 23.77 ± 1.27 m DAV and SHE, respectively) as compared to the smaller sites (18.99 ± 1.76 m, 18.07 ± 0.20 m HEL and DON, respectively). The tree size did not differ between the large and small sites (diameter at breast height; 17.28 ± 1.43 cm, 22.92 ± 1.15 cm, 20.17 ± 2.29 cm, 18.28 ± 1.54 cm DAV, SHE, HEL, and DON, respectively).
Figure 1. Map of coastal Mississippi with paired study sites in small forested habitat patches [Hellmer’s Lane (HEL), Don’s Woods (DON)] and large contiguous forested areas [Davis Bayou area of the Gulf Islands National Seashore (DAV), Shepard State Park (SHE)].
We used circulating plasma metabolite concentrations as a measure of refueling performance for birds captured during spring migratory stopover. Plasma metabolite levels are highly correlated with changes in body mass, and measurement of circulating metabolite concentrations (particularly triglycerides) is an effective method for assessing differences in habitat quality as reflected in refueling performance with a single capture (Schaub and Jenni, 2001; Guglielmo et al., 2002, 2005; Seaman et al., 2006). Plasma metabolites (triglycerides) were sampled for migrating birds throughout the spring at three habitat patches in 2011 (1 April–15 May, HEL, SHE, and DON) and sampling at the fourth (DAV) was added in 2012 (23 March–12 May, Davis Bayou). Nets were operated for approximately 5 h from sunrise and only in weather safe for netting with morning temperatures similar throughout the season and among sites (19.39 ± 3.66°C, 20.37 ± 3.89°C at large and small sites, respectively). Cycling metabolites are known to deteriorate and lose potential to reflect feeding within 20 min (Guglielmo et al., 2005; Zajac et al., 2006). To increase likelihood of detecting metabolite profiles associated with recent foraging activity within each site, the time between net checks was no more than 15 min and the capture to bleeding time was 12.69 ± 4.0 min.
Upon capture, each bird was fitted with a USGS aluminum band, morphometric measurements were recorded, and a blood sample (<1% body mass) was taken from the brachial vein prior to release. Blood samples were kept cool in capillary tubes until return to processing facilities on the afternoon of the capture day. Plasma was separated from red blood cells using a microhematocrit centrifuge and extracted from capillary tubes and frozen at −80°C until analysis using endpoint assay kits (Guglielmo et al., 2002, 2005). Circulating triglyceride concentration (mmol L–1) was calculated by subtracting free glycerol from total triglyceride. Because body condition can also influence triglyceride levels, we calculated condition for each individual using a principal component analysis on size-corrected mass (mass divided by wing chord), fat score (Helms and Drury, 1960; 0–5 scale), and muscle score (Bairlein, 1985; 0–3 scale). The first PCA axis (the only axis with an eigenvalue greater than 1) explained over 40% of the total variation; therefore, this principal component score for each individual was included in refueling performance analyses.
Transect surveys were conducted throughout spring migration (23 March–17 May 2011 and 18 March–14 May 2012) to determine the densities of migrating birds within the four habitat patches. Transect surveys were conducted twice a day (morning and afternoon) every other day in 2011 and every day in 2012 with morning surveys beginning 15 min after sunrise and afternoon surveys beginning after 1500 h and concluding before sunset. Migrant densities were calculated as birds ha–1 from standardized counts along 300-m transects at the two smaller sites and 500-m transect at the two larger sites. Survey lines were flagged in 25 m increments, and the surveyor maintained a constant pace along the transect lines of 1-km h–1. All migratory birds encountered by sight and/or sound within 25-m of each side of the transect line were recorded. Detection probabilities were not expected to differ greatly between species within 25-m of the transect during migration in coastal Mississippi habitats with similar vegetation composition and structure (Buler et al., 2007).
Many of the nocturnally migratory bird species that stopover in abundance in this region have overlapping breeding ranges in southern Mississippi. To ensure that the counted and sampled individuals were migratory, we did not sample from individuals captured after the first nesting dates for that species (Billerman et al., 2020). We classified the species captured in nets and detected on surveys by foraging strata according to Barrow et al. (2000) (Supplementary Table 1) and further categorized species for analyses into either foliage (understory, canopy, sub-canopy) or ground foraging.
Arthropod sampling was conducted during each week of spring migration to characterize the abundance of food available to migrants at each site. Foliage arthropods were counted and identified from branch clippings along the transects of randomly selected Yaupon holly (Ilex vomitoria) or oak tree (Quercus spp.), chosen for their ubiquity across all sites (Cohen et al., 2012; Lain et al., 2017). One 12″ branch 3–4 feet above ground level with no less than 40 leaves was clipped into a bag weekly at six points along the transects in each study site (Cooper and Whitmore, 1990). All arthropods in each sample were measured for length and identified to order. Using the length-weight regression equation described by Rogers et al. (1976) for North American arthropods, arthropod order and length was used to determine biomass per gram of clipped vegetation. Weekly sampling for ground arthropods occurred only during 2012 at three locations along the transects in each study site. Leaf litter arthropods were counted, identified to order, and measured for length within a 0.25 m2 quadrat. The length-weight relationships were used to estimate total biomass in the leaf litter.
We tested for differences in migrant density among habitat patch sizes and years with a generalized linear mixed effects model including the day of season as a random effect with a varying intercept weighted by the number of sampling days in the “lme4” R package (Bates et al., 2015). We tested for the influence of the density of migrants, site size, year, and the individual bird’s condition on refueling performance, as measured by plasma metabolite concentrations (triglycerides), in a generalized linear mixed effects model (with a log link to keep estimates positive) and including the species as a random effect with a varying intercept. We ran the same model with only canopy foraging species and for all foraging groups with the density of all birds, resident and migrant species, as the bird density predictor. To test for the influence of patch size alone on refueling performance, we ran the same model excluding density of migrants. We used a similarly specified model to test for the influence of foliage arthropod density on refueling performance for foliage foraging species (understory, canopy, sub-canopy).
Because foliage arthropod counts contained many zeros, we used two-part models with a hurdle component for modeling the occurrence of zeroes and a count component to model abundance (Zuur et al., 2009). We used a Poisson distribution with log link in the “pscl” R package to test for effect of habitat patch size, sampling week, and year, on arthropod occurrence and density (Zeileis et al., 2008). We tested for significance of model terms with likelihood ratio tests for mixed models in the “lmerTest” R package (Kuznetsova et al., 2017) and for two-part models in the “lmtest” (Zeileis and Hothorn, 2002). All analyses were conducted in R version 3.6.3 (R Core Team, 2020). We report mean ± se throughout.
We sampled the community of Nearctic-Neotropical birds along the coast of Mississippi during spring migration of 2011 and 2012 and detected 45 species of migrating ground, understory, sub-canopy, and canopy foraging bird species during surveys and netting in paired small and large forested habitat patches (Supplementary Tables 1, 2). Migrating birds stopped in higher densities at the smaller sites as compared to the larger (0.54 ± 0.06 birds ha–1 in small and 0.35 ± 0.04 birds ha–1 in large, χ2 = 512.95, p < 0.001, n = 651) (Figure 2) and this difference was reflected in netting capture rates that were also higher at the small sites (Supplementary Table 2). Among sites, migrants occurred in marginally higher densities on afternoon surveys, as compared to the morning (0.40 ± 0.04 birds ha–1in the morning and 0.51 ± 0.06 birds ha–1 in the afternoon, χ2 = 3.37, p = 0.07, n = 651), suggesting most migrants depart these coastal habitats on the evening of arrival (Figure 2). Migrating bird densities peaked in early April when differences in density between the large and small sites were most apparent (Figure 3). There were no annual differences in the density of all migrants (χ2 = 0.83, p = 0.36) or of foliage foraging understory, sub-canopy, and canopy migrants (χ2 = 0.10, p = 0.75) while densities of ground foraging migrants were higher in 2012 than in 2011 (0.08 ± 0.01 birds ha–1 in 2012 and 0.04 ± 0.01 birds ha–1in 2011, χ2 = 4.07, p = 0.04, n = 651), when both of the larger sites were surveyed.
Figure 2. Morning and afternoon densities of all migratory birds, foliage foraging (canopy, sub-canopy, or understory) migratory birds, and ground foraging migratory birds throughout spring migration of 2011 and 2012 at forested stopover sites in coastal Mississippi.
Figure 3. Seasonal variability in the density of migratory birds and between foliage (canopy, sub-canopy, or understory) and ground foraging birds throughout spring migration (Day of Year 80 = 21 March) of 2011 and 2012 at two sets of paired large (red and orange) and small (light and dark blue) forested stopover sites in coastal Mississippi.
The occurrence of foliage arthropods (i.e., zero vs. non-zero counts), did not differ among years (χ2 = 4.18, p = 0.12) or by patch size (χ2 = 2.82, p = 0.24) but varied by sampling week (χ2 = 17.89, p < 0.001, n = 657). Where foliage arthropods occurred, (i.e., among the positive counts) biomass density was similar among sampling weeks (χ2 = 0.12, p = 0.94), years (χ2 = 3.50, p = 0.17), and patch sizes (χ2 = 1.20, p = 0.55). The analysis of leaf litter arthropod biomass was limited by sample size but, unlike foliage arthropods, arthropods were detected in all samples and biomass increased throughout the season (χ2 = 15.62, p < 0.001, n = 31) but did not vary among site sizes (χ2 = 1.41, p = 0.07).
We characterized refueling performance of migrating birds with circulating plasma metabolite concentrations and, in support of our prediction of density-dependent refueling, performance declined with increasing migrant densities (χ2 = 5.08, p = 0.02) (Figure 4). Refueling performance did not vary with condition of the individual bird (χ2 = 2.33, p = 0.13) but was higher in 2012 as compared to 2011 (1.22 ± 0.10 mmol L–1 in 2011 and 1.90 ± 0.16 mmol L–1 in 2012, χ2 = 21.44, p < 0.01, n = 101) (Figure 4), when sampling was added at the second large site. The size of the habitat patch alone did not influence refueling performance (1.54 ± 0.19 mmol L–1 in large and 1.39 ± 0.10 mmol L–1 in small, χ2 = 1.84, p = 0.18) nor did the influence of the density of all birds, migrants and residents combined, (χ2 = 1.44, p = 0.23, n = 63). The results for the analysis of canopy foraging birds alone was limited by sampling size but refueling performance declined marginally with increasing migrant densities (χ2 = 3.17, p = 0.08, n = 54). We tested for the influence of foliage arthropod density on refueling performance for foliage foraging species accounting for the individual condition (χ2 = 2.82, p = 0.09), year (χ2 = 15.22, p < 0.01), and site size (χ2 = 0.98, p = 0.32) and did not find a relationship (χ2 = 0.33, p = 0.56, n = 70). Sample sizes were insufficient for a similar analysis for ground foraging birds because leaf litter arthropod sampling did not sufficiently overlap with samples collected from ground foraging birds.
Figure 4. Estimated marginal means ± 95% CI of refueling performance of 101 individuals of 24 species of migrating birds, as measured by triglyceride concentrations, by the same-day density of migrating birds during 2011 and 2012 (random effect with a varying intercept).
Long-distance, intercontinental migratory birds invariably encounter urbanizing landscapes during passage where they may experience elevated competitor density with negative consequences for refueling performance. Upon arrival following movement across the Gulf of Mexico in spring, migratory songbirds in our study, approximately half of which were in depleted energetic condition, distributed themselves across both large and small patches of forested habitat along the coast (Buler and Moore, 2011). Although densities across our study landscape were low relative to other parts of the northern gulf coast (Cohen et al., 2021), migrants were more concentrated per unit area in small habitat patches than in the large contiguous forested areas. This concentrating effect in smaller, more isolated patches is consistent with models of landscape-scale migrant distributions in relationship to habitat patch sizes and non-ideal free distributions in the face of limited knowledge of surroundings (Shochat et al., 2002; Diehl et al., 2003; Pennington et al., 2008). Crowding may be especially pronounced at the edges of ecological barriers when migrants arrive and encounter an urbanizing landscape with fragmented habitat, minimal cues to habitat quality, pressure to move quickly to breeding grounds, and less latitude energetically to explore among available habitats (Simons et al., 2000; Cohen et al., 2017). Landing in poor-quality habitat extracts a search cost on refueling performance (Alerstam and Lindström, 1990; Alerstam and Hedenström, 1998), so quickly locating habitat with sufficient food resources at each stopover becomes an important determinant of a successful migration (Cohen et al., 2014).
The measure of refueling performance, triglyceride concentration, across this urbanizing coastal landscape fell within the range considered elevated for refueling (Seewagen et al., 2011). Although the diversity of migrating songbirds that stopped over at our study sites could expect to show a positive refueling performance, performance was density dependent, despite the rather low observed densities in our study. Density dependent effects can be thought of as scale-dependent, as with stopover habitat use (Moore et al., 2005). Within landscapes, for example, refueling performance can vary among habitats and by species due to dietary and vegetative differences (Smith and McWilliams, 2010) and this was the case within our focal landscape where values were higher at Shepard State Park, the large tract of contiguous forest, as compared to Don’s Woods. At the broader spatial scale, migrants may be concentrated within high passage corridors with high stopover-to-passage ratios (Cohen et al., 2021) where density-dependent effects would be high, and they may have greater impact as these landscapes urbanize, minimizing patch sizes. While the Mississippi coastline is urbanizing, it is not within a particularly high-volume passage area during spring migration (Cohen et al., 2021). Yet, we still found density-dependent effects even in an urbanizing area with a low stopover-to-passage ratio, suggesting that these effects may be even stronger elsewhere.
Arthropod abundances were low at all sites and we did not detect the expected differences among the patch sizes nor were we able to make a direct link between food abundance and refueling rate in this study. Sampling arthropods presents many challenges due to the diversity of substrates, plant associations, influence of phenology and weather and differences obtained from sampling methods (Cooper and Whitmore, 1990; Cooper et al., 2012). Weather and phenology were likely not overly influential in this study for three reasons: spring leaf-out occurs before peak spring migration on the Gulf Coast (Ault et al., 2015), there were no extreme weather events during the course of this study, and the local morning temperatures were similar throughout the season and among the habitat patches. Nevertheless, we did find that arthropod occurrence varied among sampling weeks and leaf litter arthropods increased throughout the season.
A community-based approach is entirely appropriate during stopovers when competition for resources or habitats are likely common because many species that share migratory pathways also overlap in food and habitat niches (Cohen and Satterfield, 2020). While sample sizes limit inference about food availability for the 45 species in this study, all of these species are primarily insectivorous generalists during migration making generalized measures of food availability likely the most appropriate for this field study. Foraging behavior and diet likely converge during stopover, especially among leaner migrants (Loria and Moore, 1990; Yong and Moore, 1997). Further, migrants with reduced fat reserves broadened their use of habitat and increased their foraging repertoire and this increased plasticity would lead to convergence among heterospecifics (Martin and Karr, 1990; Parrish, 2000).
Density-dependent effects may negatively impact a successful migration. For example, when migrants with similar nutritional requirements and heightened energy demand find themselves locally concentrated in forest fragments, competition could depress food resources and reduce the rate at which migrants restore essential fuel loads (Hansson and Pettersson, 1989; Moore and Yong, 1991; Kelly et al., 2002; Lindström, 2003). In this study, low prey availability suggest that competitive pressure may exist across coastal stopover habitats consistent with the idea that crowding of migrants into smaller patches impacts efficient fuel deposition. Even if food resources are not depressed, more migrants may lead to aggressive interactions, which may depress the rate at which migrants feed regardless of resource availability (Arizaga et al., 2011). Migrants might also alter foraging behavior in the presence of other migrants, which may negatively impact the acquisition of resources and refueling performance (Moore and Yong, 1991; Moore et al., 2003). Therefore, even where refueling efficiency is not directly related to resource depletion, behavioral interference can result in density-dependent refueling.
Migratory songbirds that stopped within forest fragments along the Mississippi gulf coast did not remain for extended periods as evidenced by higher migrant densities during afternoon surveys (presumptive day of arrival) than during surveys conducted the following morning. Given the constraints imposed by energetic demand and a tight spring migration schedule, not to mention density-dependent effects, the majority of migrants at our study sites are probably transient; that is, birds that stop to rest after crossing the Gulf of Mexico only to depart the night of arrival. Although movements of migrants in relation to this urbanizing coastal landscape are unknown, birds may exhibit localized exploratory movements to familiarize themselves with the area and to sample nearby resources (Aborn and Moore, 1997; Seewagen et al., 2010; Cohen et al., 2012) or make landscape level movements toward resource richer habitats just north of the immediate coast (Buler and Moore, 2011; Zenzal et al., 2021). Previous work suggests that habitat patch-level effects (e.g., resources, competition) do impact movements of en route migrants in both urban and non-urban environments, and those movements vary with energetic condition upon arrival, time since arrival, and habitat quality (Matthews and Rodewald, 2010; Cohen et al., 2012). Future investigation of movement patterns of individuals of known energetic condition within and away from these habitat fragments would shed light on the quality of this landscape for migrating birds (Simons et al., 2000; Cohen et al., 2014).
Finding habitat where a migrant can efficiently and safely refuel is a pressing challenge during passage and one of the key reasons to stopover in the first place. Energy constrained migrants stopping over in urbanizing coastal landscapes may find themselves crowded in habitat fragments where prey abundance is low. Crowding has a density-dependent impact on refueling performance, which translates into a reduced likelihood of meeting energetic demands and increased risk of predation not to mention delayed arrival at the destination and nutritional deficit upon arrival at destination if not addressed during the next stopover. Habitat patch size alone did not account for differences in refueling performance in our study but migrants were often more concentrated in the small patches and these concentrations reduced refueling performance, supporting the importance of large tracts of contiguous forest within urban landscapes. Although the suitability of stopover habitats encountered during a migratory journey likely vary considerably, the extent to which the resources birds encounter during migration carry over to limit populations remains uncertain (Newton, 2006; Cohen et al., 2017) as does the potential increased cost of encountering landscapes undergoing unprecedented anthropogenic impacts (Wilcove and Wikelski, 2008).
The raw data supporting the conclusions of this article will be made available by the authors, without undue reservation.
The animal study was reviewed and approved by the University of Southern Mississippi Institutional Animal Care and Use Committee protocol # 11092210 and by USGS for BBL Permit No. 21221.
JL and FM conceived the research idea, design, and developed the field methods. JL collected the data. EC analyzed the data. EC and FM wrote the manuscript. All authors contributed to review and editing of manuscript drafts.
Funding for this research was provided by the Eastern Bird Banding Association, Manomet Center for Conservation Sciences, and the USGS Gulf Coast Joint Venture.
The authors declare that the research was conducted in the absence of any commercial or financial relationships that could be construed as a potential conflict of interest.
All claims expressed in this article are solely those of the authors and do not necessarily represent those of their affiliated organizations, or those of the publisher, the editors and the reviewers. Any product that may be evaluated in this article, or claim that may be made by its manufacturer, is not guaranteed or endorsed by the publisher.
We thank the National Park Service, the Land Trust for the Mississippi Coastal Plain, the Mississippi Department of Wildlife, Fisheries, and Parks, and private landowners of Pascagoula, Mississippi for access to work on these properties. Three field technicians assisted us with this work, William Oakley, Nicholas Beauregard, and Josh Hodge.
The Supplementary Material for this article can be found online at: https://www.frontiersin.org/articles/10.3389/fevo.2022.837790/full#supplementary-material
Aborn, D. A. (1994). Correlation between raptor and songbird numbers at a migratory stopover site. Wilson Bull. 106, 150–154.
Aborn, D. A., and Moore, F. R. (1997). Pattern of movement by summer tanagers (Piranga rubra) during migratory stopover: a telemetry study. Behaviour 134, 1077–1100. doi: 10.1163/156853997X00412
Alerstam, T. (2011). Optimal bird migration revisited. J. Ornithol. 152, 5–23. doi: 10.1007/s10336-011-0694-1
Alerstam, T., and Hedenström, A. (1998). The development of bird migration theory. J. Avian Biol. 29, 343–369. doi: 10.2307/3677155
Alerstam, T., and Lindström, Å (1990). “Optimal bird migration: the relative importance of time, energy, and safety,” in Bird Migration, ed. E. Gwinner (Berlin: Springer), 331–351. doi: 10.1007/978-3-642-74542-3_22
Arizaga, J., Schmaljohann, H., and Bairlein, F. (2011). Stopover behaviour and dominance: a case study of the northern wheatear Oenanthe oenanthe. Ardea 99, 157–165. doi: 10.5253/078.099.0205
Ault, T. R., Schwartz, M. D., Zurita-Milla, R., Weltzin, J. F., and Betancourt, J. L. (2015). Trends and natural variability of spring onset in the coterminous United States as evaluated by a new gridded dataset of spring indices. J. Clim. 28, 8363–8378. doi: 10.1175/JCLI-D-14-00736.1
Bairlein, F. (1985). Body weights and fat deposition of Palaearctic passerine migrants in the central Sahara. Oecologia 66, 141–146. doi: 10.1007/BF00378566
Barrow, W. C. Jr., Chen, C.-C., Hamilton, R. B., Ouchley, K., and Spengler, T. J. (2000). Disruption and restoration of en route habitat, a case study: the chenier plain. Stud. Avian Biol. 20, 71–87.
Bates, D., Maechler, M., Bolker, B., and Walker, S. (2015). Fitting linear mixed-effects models using lme4. J. Stat. Softw. 67, 1–48.
Billerman, S. M., Keeney, B. K., Rodewald, P. G., and Schulenberg, T. S. (Eds). (2020). Birds of the World – Comprehensive life histories for all bird Species and Families. Ithaca, NY: Cornell Laboratory of Ornithology.
Buler, J. J., and Moore, F. R. (2011). Migrant–habitat relationships during stopover along an ecological barrier: extrinsic constraints and conservation implications. J. Ornithol. 152, 101–112. doi: 10.1007/s10336-010-0640-7
Buler, J. J., Moore, F. R., and Woltmann, S. (2007). A multi-scale examination of stopover habitat use by birds. Ecology 88, 1789–1802. doi: 10.1890/06-1871.1
Cimprich, D. A., Woodrey, M. S., and Moore, F. R. (2005). Passerine migrants respond to variation in predation risk during stopover. Anim. Behav. 69, 1173–1179. doi: 10.1016/j.anbehav.2004.07.021
Clipp, H. L., Cohen, E. B., Smolinsky, J. A., Horton, K. G., Farnsworth, A., and Buler, J. J. (2020). Broad-scale weather patterns encountered during flight influence landbird stopover distributions. Remote Sens. 12:565. doi: 10.3390/rs12030565
Cohen, E. B., Barrow, W. C. Jr., Buler, J. J., Deppe, J. L., Farnsworth, A., Marra, P. P., et al. (2017). How do en route events around the Gulf of Mexico influence migratory landbird populations? Condor 119, 327–343. doi: 10.1650/CONDOR-17-20.1
Cohen, E. B., Horton, K. G., Marra, P. P., Clipp, H. L., Farnsworth, A., Smolinsky, J. A., et al. (2021). A place to land: spatiotemporal drivers of stopover habitat use by migrating birds. Ecol. Lett. 24, 38–49. doi: 10.1111/ele.13618
Cohen, E. B., Moore, F. R., and Fischer, R. A. (2012). Experimental evidence for the interplay of exogenous and endogenous factors on the movement ecology of a migrating songbird. PLoS One 7:e41818. doi: 10.1371/journal.pone.0041818
Cohen, E. B., Pearson, S. M., and Moore, F. R. (2014). Effects of landscape composition and configuration on migrating songbirds: inference from an individual-based model. Ecol. Appl. 24, 169–180. doi: 10.1890/12-1867.1
Cohen, E. B., and Satterfield, D. A. (2020). ‘Chancing on a spectacle:’ co-occurring animal migrations and interspecific interactions. Ecography 43, 1657–1671. doi: 10.1111/ecog.04958
Cooper, N. W., Thomas, M. A., Garfinkel, M. B., Schneider, K. L., and Marra, P. P. (2012). Comparing the precision, accuracy, and efficiency of branch clipping and sweep netting for sampling arthropods in two Jamaican forest types. J. Field Ornithol. 83, 381–390. doi: 10.1111/j.1557-9263.2012.00388.x
Cooper, R. J., and Whitmore, R. C. (1990). Arthropod sampling methods in ornithology. Stud. Avian Biol. 13, 29–37.
Diehl, R. H., Larkin, R. P., and Black, J. E. (2003). Radar observations of bird migration over the great lakes. Auk 120, 278–290. doi: 10.1093/auk/120.2.278
Guglielmo, C. G., Cerasale, D. J., and Eldermire, C. (2005). A field validation of plasma metabolite profiling to assess refueling performance of migratory birds. Physiol. Biochem. Zool. 78, 116–125. doi: 10.1086/425198
Guglielmo, C. G., O’Hara, P. D., and Williams, T. D. (2002). Extrinsic and intrinsic sources of variation in plasma lipid metabolites of free-living western sandpipers (Calidris mauri). Auk 119, 437–445. doi: 10.1093/auk/119.2.437
Hansson, M., and Pettersson, J. (1989). Competition and fat deposition in goldcrests (Regulus regulus) at a migration stop-over site. Vogelwarte 35, 21–31.
Helms, C. W., and Drury, W. H. (1960). Winter and migratory weight and fat field studies on some North American buntings. Bird Band. 31, 1–40. doi: 10.2307/4510793
Horton, K. G., Nilsson, C., Doren, B. M. V., Sorte, F. A. L., Dokter, A. M., and Farnsworth, A. (2019). Bright lights in the big cities: migratory birds’ exposure to artificial light. Front. Ecol. Environ 17:209–214. doi: 10.1002/fee.2029
Keller, G. S., and Avery, J. D. (2014). Avian use of isolated cottonwood, tamarisk, and residential patches of habitat during migration on the high plains of New Mexico. Southwest. Nat. 59, 263–271. doi: 10.1894/F15-MLK-15.1
Kelly, J. F., DeLay, L. S., and Finch, D. M. (2002). Density-dependent mass gain by Wilson’s warblers during stopover. Auk 119, 210–213. doi: 10.1093/auk/119.1.210
Ktitorov, P., Bairlein, F., and Dubinin, M. (2008). The importance of landscape context for songbirds on migration: body mass gain is related to habitat cover. Landsc. Ecol. 23, 169–179. doi: 10.1007/s10980-007-9177-4
Kuznetsova, A., Brockhoff, P. B., and Christensen, R. H. (2017). lmerTest package: tests in linear mixed effects models. J. Stat. Softw. 82, 1–26.
La Sorte, F. A., Fink, D., Buler, J. J., Farnsworth, A., and Cabrera-Cruz, S. A. (2017). Seasonal associations with urban light pollution for nocturnally migrating bird populations. Glob. Chang. Biol. 23, 4609–4619. doi: 10.1111/gcb.13792
Lafleur, J. M., Buler, J. J., and Moore, F. R. (2016). Geographic position and landscape composition explain regional patterns of migrating landbird distributions during spring stopover along the northern coast of the Gulf of Mexico. Landsc. Ecol. 31, 1697–1709. doi: 10.1007/s10980-016-0354-1
Lain, E. J., Zenzal, T. J. Jr., Moore, F. R., Barrow, W. C. Jr., and Diehl, R. H. (2017). Songbirds are resilient to hurricane disturbed habitats during spring migration. J. Avian Biol. 48, 815–826. doi: 10.1111/jav.01215
Lindstrom, Å (1990). The role of predation risk in stopover habitat selection in migrating bramblings, Fringilla montifringilla. Behav. Ecol. 1, 102–106. doi: 10.1093/beheco/1.2.102
Lindström, Å (2003). “Fuel Deposition Rates in Migrating Birds: Causes, Constraints and Consequences,” in Avian Migration, eds P. Berthold, E. Gwinner, and E. Sonnenschein (Berlin: Springer), 307–320. doi: 10.1007/978-3-662-05957-9_21
Loria, D. E., and Moore, F. R. (1990). Energy demands of migration on red-eyed vireos, Vireo olivaceus. Behav. Ecol. 1, 24–35. doi: 10.1093/beheco/1.1.24
Martin, T. E., and Karr, J. R. (1990). Behavioral plasticity of foraging maneuvers of migratory warblers: multiple selection periods for niches. Stud. Avian Biol. 13, 353–359.
Matthews, S. N., and Rodewald, P. G. (2010). Movement behaviour of a forest songbird in an urbanized landscape: the relative importance of patch-level effects and body condition during migratory stopover. Landsc. Ecol. 25, 955–965. doi: 10.1007/s10980-010-9475-0
McCabe, J. D., and Olsen, B. J. (2015). Tradeoffs between predation risk and fruit resources shape habitat use of landbirds during autumn migration. Auk 132, 903–913. doi: 10.1642/AUK-14-213.1
McKinney, M. L. (2002). Urbanization, biodiversity, and conservation: the impacts of urbanization on native species are poorly studied, but educating a highly urbanized human population about these impacts can greatly improve species conservation in all ecosystems. Bioscience 52, 883–890.
McLaren, J. D., Buler, J. J., Schreckengost, T., Smolinsky, J. A., Boone, M., and Emiel, et al. (2018). Artificial light at night confounds broad-scale habitat use by migrating birds. Ecol. Lett. 21, 356–364. doi: 10.1111/ele.12902
Moore, F., Mabey, S., and Woodrey, M. (2003). “Priority access to food in migratory birds: age, sex and motivational asymmetries,” in Avian Migration, eds P. Berthold, E. Gwinner, and E. Sonnenschein (Berlin: Springer), 281–292. doi: 10.1007/978-3-662-05957-9_19
Moore, F. R., Woodrey, M. S., Buler, J. J., Woltmann, S., and Simons, T. R. (2005). “Understanding the stopover of migratory birds: a scale dependent approach,” in Bird Conservation Implementation and Integration in the Americas: Proceedings of the Third International Partners in Flight Conference. 2002 March 20-24; Asilomar, California, Volume 2 Gen. Tech. Rep. PSW-GTR-191, eds C. J. Ralph and T. D. Rich (Albany, CA: US Dept. of Agriculture, Forest Service, Pacific Southwest Research Station), 684–689. doi: 10.3389/fphys.2017.00532
Moore, F. R., and Yong, W. (1991). Evidence of food-based competition among passerine migrants during stopover. Behav. Ecol. Sociobiol. 28, 85–90.
Németh, Z., and Moore, F. R. (2007). Unfamiliar stopover sites and the value of social information during migration. J. Ornithol. 148, 369–376. doi: 10.1007/s10336-007-0209-2
Németh, Z., and Moore, F. R. (2014). Information acquisition during migration: a social perspective. Auk 131, 186–194. doi: 10.1642/AUK-13-195.1
Newton, I. (2006). Can conditions experienced during migration limit the population levels of birds? J. Ornithol. 147, 146–166. doi: 10.1128/JVI.02305-15
Packett, D. L., and Dunning, J. B. Jr. (2009). Stopover habitat selection by migrant landbirds in a fragmented forest-agricultural landscape. Auk 126, 579–589. doi: 10.1525/auk.2009.08198
Parrish, J. D. (2000). Behavioral, energetic, and conservation implications of foraging plasticity during migration. Stud. Avian Biol. 20, 53–70.
Pennington, D. N., Hansel, J., and Blair, R. B. (2008). The conservation value of urban riparian areas for landbirds during spring migration: land cover, scale, and vegetation effects. Biol. Conserv. 141, 1235–1248. doi: 10.1016/j.biocon.2008.02.021
R Core Team (2020). R: A language and environment for statistical computing. Vienna: R Foundation for Statistical Computing.
Rodewald, P. G., and Brittingham, M. C. (2007). Stopover habitat use by spring migrant landbirds: the roles of habitat structure, leaf development, and food availability. Auk 124, 1063–1074. doi: 10.1093/auk/124.3.1063
Rodewald, P. G., and Matthews, S. N. (2005). Landbird use of riparian and upland forest stopover habitats in an urban landscape. Condor 107, 259–268. doi: 10.1093/condor/107.2.259
Rogers, L. E., Hinds, W. T., and Buschbom, R. L. (1976). A general weight vs. length relationship for insects1. Ann. Entomol. Soc. Am. 69, 387–389. doi: 10.1093/aesa/69.2.387
Schaub, M., and Jenni, L. (2001). Variation of fuelling rates among sites, days and individuals in migrating passerine birds. Funct. Ecol 15, 584–594. doi: 10.1046/j.0269-8463.2001.00568.x
Seaman, D. A. A., Guglielmo, C. G., Elner, R. W., and Williams, T. D. (2006). Landscape-scale physiology: site differences in refueling rates indicated by plasma metabolite analysis in free-living migratory sandpipers. Auk 123, 563–574. doi: 10.1093/auk/123.2.563
Seewagen, C. L., Sheppard, C. D., Slayton, E. J., and Guglielmo, C. G. (2011). Plasma metabolites and mass changes of migratory landbirds indicate adequate stopover refueling in a heavily urbanized landscape. Condor 113, 284–297. doi: 10.1525/cond.2011.100136
Seewagen, C. L., Slayton, E. J., and Guglielmo, C. G. (2010). Passerine migrant stopover duration and spatial behaviour at an urban stopover site. Acta Oecol. 36, 484–492. doi: 10.1016/j.actao.2010.06.005
Shochat, E., Abramsky, Z., Pinshow, B., and Whitehouse, M. E. A. (2002). Density-dependent habitat selection in migratory passerines during stopover: what causes the deviation from IFD? Evol. Ecol. 16, 469–488. doi: 10.1023/A:1020851801732
Simons, T. R., Pearson, S. M., and Moore, F. R. (2000). Application of spatial models to the stopover ecology of trans-gulf migrants. Stud. Avian Biol. 20, 4–14.
Smith, S. B., and McWilliams, S. R. (2010). Patterns of fuel use and storage in migrating passerines in relation to fruit resources at autumn stopover sites. Auk 127, 108–118. doi: 10.1525/auk.2009.09139
Van Doren, B. M., Horton, K. G., Dokter, A. M., Klinck, H., Elbin, S. B., and Farnsworth, A. (2017). High-intensity urban light installation dramatically alters nocturnal bird migration. Proc. Natl. Acad. Sci. U.S.A. 114, 11175–11180. doi: 10.1073/pnas.1708574114
Ward, M. P., Benson, T. J., Deppe, J., Zenzal, T. J., Diehl, R. H., Celis-Murillo, A., et al. (2018). Estimating apparent survival of songbirds crossing the Gulf of Mexico during autumn migration. Proc. R. Soc. B Biol. Sci. 285:20181747. doi: 10.1098/rspb.2018.1747
Wilcove, D. S., and Wikelski, M. (2008). Going, going, gone: is animal migration disappearing. PLoS Biol. 6:e188. doi: 10.1371/journal.pbio.0060188
Yong, W., and Moore, F. R. (1997). Spring stopover of intercontinental migratory thrushes along the northern coast of the Gulf of Mexico. Auk 114, 263–278. doi: 10.2307/4089167
Zajac, R. M., Cerasale, D. J., and Guglielmo, C. G. (2006). The rapid response of plasma metabolites to changes in feeding rate in a small passerine Wilsonia pusilla. J. Avian Biol 37, 405–408. doi: 10.1111/j.2006.0908-8857.03577.x
Zeileis, A., and Hothorn, T. (2002). Diagnostic checking in regression relationships. R News 2, 7–10.
Zeileis, A., Kleiber, C., and Jackman, S. (2008). Regression models for count data in R. J. Stat. Softw. 27, 1–25.
Zenzal, T. J., Fish, A. C., Jones, T. M., Ospina, E. A., and Moore, F. R. (2013). Observations of predation and anti-predator behavior of rubythroated hummingbirds during migratory. Southeast. Nat. 12:N21. doi: 10.1656/058.012.0416
Zenzal, T. J., Ward, M. P., Diehl, R. H., Buler, J. J., Smolinsky, J. A., Deppe, J. L., et al. (2021). Retreat, detour, or advance? Understanding the movement ecology of migrating birds confronting the Gulf of Mexico. Oikos 130, 669–819.
Keywords: stopover, migration, songbird, urbanization, Nearctic-Neotropical migrant birds, patch size, fuel deposition rate
Citation: Cohen EB, Lafleur JM and Moore FR (2022) Density Dependent Refueling of Migratory Songbirds During Stopover Within an Urbanizing Coastal Landscape. Front. Ecol. Evol. 10:837790. doi: 10.3389/fevo.2022.837790
Received: 17 December 2021; Accepted: 09 February 2022;
Published: 01 March 2022.
Edited by:
Nikita Chernetsov, Zoological Institute (RAS), RussiaReviewed by:
Ivan Maggini, University of Veterinary Medicine Vienna, AustriaCopyright © 2022 Cohen, Lafleur and Moore. This is an open-access article distributed under the terms of the Creative Commons Attribution License (CC BY). The use, distribution or reproduction in other forums is permitted, provided the original author(s) and the copyright owner(s) are credited and that the original publication in this journal is cited, in accordance with accepted academic practice. No use, distribution or reproduction is permitted which does not comply with these terms.
*Correspondence: Emily B. Cohen, ZW1pbHkuY29oZW5AdW1jZXMuZWR1
Disclaimer: All claims expressed in this article are solely those of the authors and do not necessarily represent those of their affiliated organizations, or those of the publisher, the editors and the reviewers. Any product that may be evaluated in this article or claim that may be made by its manufacturer is not guaranteed or endorsed by the publisher.
Research integrity at Frontiers
Learn more about the work of our research integrity team to safeguard the quality of each article we publish.