- 1Institute of Avian Research, Wilhelmshaven, Germany
- 2Centre d’Etudes Biologiques de Chizé, CNRS-La Rochelle Université, UMR-7372, Villiers-en-Bois, France
- 3Centre for Ecology and Conservation, University of Exeter, Penryn, United Kingdom
The quality of the environment individuals experience during development is commonly regarded as very influential on performance in later life. However, studies that have experimentally manipulated the early-life environment and subsequently measured individual performance in all components of fitness over the complete life course are scarce. In this study, we incubated fertile eggs of Japanese quail (Coturnix japonica) at substandard and standard incubation temperature, and monitored growth, survival, and reproduction throughout the complete life course. While embryonic development was slower and hatching success tended to be lower under substandard incubation temperature, the prenatal treatment had no effect on post-hatching growth, survival to sexual maturity, or age at first reproduction. In adulthood, body mass and investment in individual egg mass peaked at middle age, irrespective of the prenatal treatment. Individual reproduction rate declined soon after its onset, and was higher in females that lived longer. Yet, reproduction, and its senescence, were independent of the prenatal treatment. Similarly, adult survival over the complete lifespan was not affected. Hence, we did not find evidence for effects on performance beyond the developmental period that was manipulated. Our results suggest that effects of unfavorable developmental conditions on individual performance later in life could be negligible in some circumstances.
Introduction
Quality differences in the environment individuals experience during development may have long-term effects on phenotype, survival, and reproduction, thereby profoundly impacting individual life histories and fitness (e.g., Kruuk et al., 1999; Metcalfe and Monaghan, 2001; Lummaa and Clutton-Brock, 2002; Reid et al., 2003; van de Pol et al., 2006; Nussey et al., 2007; Hamel et al., 2009; Hayward et al., 2013; Spagopoulou et al., 2020). Yet, whether negative fitness consequences of a poor developmental environment are universal, and at what life stage they are primarily incurred, remains difficult to infer (Cooper and Kruuk, 2018; Vedder et al., 2021). This difficulty partly stems from the scarcity of studies that combined experimental manipulation of the developmental environment with complete monitoring of individual lifelong performance. While experimental manipulation is required to establish an effect of the developmental environment per se, complete monitoring is required to avoid the problem of “the invisible fraction” (Grafen, 1988). This term refers to the fraction of individuals that does not survive, or recruit, to the pool of individuals of which fitness is measured. This fraction can be substantial due to mortality during individual development, or dispersal, and, if this fraction is non-random, can bias conclusions regarding the importance of the environment during development for complete fitness and life histories (Mojica and Kelly, 2010; Goodrich and Roach, 2013; Garratt et al., 2015; Vedder et al., 2021).
In this study, we experimentally manipulated the quality of the environment during early development in Japanese quail (Coturnix japonica), and monitored all age-specific components of fitness that compose total fitness: embryonic survival, survival to reproduction, age at onset of reproduction, lifelong age-specific reproduction and adult survival. Because effects of developmental conditions on fitness may be mediated by body mass differences in the wild (Plard et al., 2015; Ronget et al., 2018), we also monitored juvenile growth and adult body mass throughout life. Japanese quail are a popular avian model for research on physiology and senescence (Ottinger, 2001), in which all abovementioned components of fitness can be quantified. Moreover, it exhibits a very fast life history with a lifespan that rarely exceeds 3 years (Woodward and Abplanalp, 1971). To manipulate the quality of the early developmental environment, we artificially incubated fertile eggs at different incubation temperatures.
Low incubation temperatures in birds generally lead to slower embryonic development and reduced hatching success (Decuypere and Michels, 1992; DuRant et al., 2013). In addition, effects of incubation temperature on post-hatching phenotype are frequently reported, which may carry through to adulthood (DuRant et al., 2013). For example, in wood ducks (Aix sponsa) a low incubation temperature led to slower post-hatching growth, increased corticosterone levels, poorer locomoter performance, and a lower probability of recruitment to the breeding population (DuRant et al., 2010; Hopkins et al., 2011; Hepp and Kennamer, 2012). In blue tits (Cyanistes caeruleus) a low incubation temperature increased resting metabolic rate in the nestling stage (Nord and Nilsson, 2011), but intermediate incubation temperatures were associated with the highest adult body mass in the 3% of nestlings that were sampled in adulthood (Nord and Nilsson, 2016). An experiment in captive zebra finches (Taeniopygia guttata) found lower survival in adulthood in response to low incubation temperature, with survival being monitored until 77% of hatchlings were no longer alive (Berntsen and Bech, 2016). Yet, this does not necessarily imply that lower incubation temperatures negatively affect total fitness. Indeed, in common terns (Sterna hirundo) and Japanese quail a lower incubation temperature reduced telomere attrition during embryonic development (Vedder et al., 2018; Stier et al., 2020). Since increased telomere attrition may mechanistically link early-life adversity to decreased survival later in life-life (e.g., Heidinger et al., 2012; Eastwood et al., 2019), this illustrates the need to measure performance during an individual’s entire life.
In this study, we randomly assigned fertile Japanese quail eggs to incubation at standard and substandard temperature, and subsequently monitored individual performance in terms of growth, reproduction, and survival. We specifically monitored adult performance of females over their complete lifespan, to also test for senescence effects that only occur late in life. To our knowledge, this represents the most detailed study on the effects of incubation temperature on lifelong performance in any bird species to date.
Materials and Methods
Experimental Procedures and Data Collection
The study was performed in 2018 in a captive population of Japanese quail maintained at the Institute of Avian Research in Wilhelmshaven, Germany. In four breeding rounds, a total of 96 pairs were housed in breeding cages (122 cm × 50 cm × 50 cm). All individuals were of similar age (1 year old). They spent 8 days together in the breeding cages, and eggs were collected at a daily basis. Eggs were marked with an indelible marker and weighed, to the nearest 0.01 g, at the day of collection. Females were isolated from males, at least 10 days prior to pairing, to prevent stored sperm of previous males to fertilize any eggs (see Birkhead and Fletcher, 1994). The collected eggs were stored at 12°C and artificially incubated in four rounds, always within 7 days of laying. Excluding the egg that was laid on the first day after pairing (which was always undeveloped) for each pair, we collected a total of 604 eggs.
For the first 7 days, incubation of these eggs was performed at 37.7°C and 50% relative humidity in two identical fully automatic incubators (Grumbach, ProCon automatic systems GmbH & Co., KG, Mücke, Germany) that turned eggs every hour. After 7 days of incubation, eggs were candled. 525 of 604 eggs (87%) from 93 parent pairs contained a live embryo, of which 263 randomly selected eggs were further incubated at 37.7°C, whereas the other 262 eggs containing an embryo were further incubated at 36.0°C. The specific temperatures of the experimental groups were chosen as 37.7°C represents a standard incubation temperature that maximizes hatching success (Stier et al., 2020), while 36.0°C represents a considerable reduction in temperature that still results in viable hatchlings (Ben-Ezra and Burness, 2017). There was no difference in fresh egg mass between the two treatment groups (mean = 11.64 g for both groups, χ2 = 0.35, Δdf = 1, P = 0.552). The subsequent incubation was performed in the same identical incubators as in the first week, but with one incubator set to 36.0°C. The incubator with the lower incubation temperature was alternated between rounds. After 14 days of incubation, the eggs were placed in marked individual compartments to allow the hatchling to be linked to the egg it hatched from. Further incubation until hatching was done at the same temperatures, but with 70% relative humidity and no egg turning, in two hatching incubators (Favorit, HEKA Brutgeräte, Rietberg, Germany). From 15 days of incubation onward, both hatching incubators were checked every 6 h to monitor hatching until no viable embryos were left (after around 20 days of incubation). This allowed us to measure the total incubation duration that each hatchling required with a resolution of 0.25 days.
Once a day, all chicks that had hatched in the previous 24 h were taken out of the incubators, marked with a numbered plastic leg ring, weighed to the nearest 0.01 g, and placed in heated rearing cages (109 × 57 × 25 cm, Kükenaufzuchtbox Nr 4002/C, HEKA Brutgeräte, Rietberg, Germany), with maximally 30 individuals of mixed incubation treatment per cage. In the rearing cages, water and food were provided ad libitum, with a 16-8 h light-dark cycle. The rearing diet contained 21.0% protein and had a caloric value of 11.4 MJ/Kg (GoldDott, DERBY Spezialfutter GmbH, Münster, Germany). The temperature of the rearing cages was set at 37.0°C at hatching and gradually lowered to room temperature (20-25°C) over the course of two weeks. After 14 days, the plastic leg rings were replaced with uniquely numbered aluminum rings, and the chicks transferred to outdoor aviaries in mixed treatment groups. Here, they were kept on an adult diet with 19.0% protein and a caloric value of 9.8 MJ/Kg (GoldDott, DERBY Spezialfutter GmbH, Münster, Germany), and received a minimum of 16 h of light per day.
All chicks were weighed at an age of 7, 14, 28, and 42 days after individual hatching, to the nearest 0.01 g at age 7 d, and to the nearest g at all later ages. Chicks were sexed based on plumage characteristics, which are distinctly different between males and females from the age of 4 weeks. All chicks that died prematurely were molecularly sexed (following Becker and Wink, 2003), except one. From 5 weeks onward, the chicks were individually checked for the onset of reproductive activity every 2-3 days. For males this was done by checking for the production of cloacal foam, which is a good indicator for sexual activity (Sachs, 1969). For females this was done by checking for the presence of an egg in the oviduct, which can be easily established by palpation. Each individual was weighed again at its age of onset of reproduction (hereafter referred to as “age at sexual maturity”). At the onset of reproduction, each female was temporarily housed in a breeding cage until she laid at least 4 eggs. This way, her laying rate was established and her eggs were weighed to the nearest 0.01 g on the day of laying. Afterward, the females were moved back into the outdoor aviaries, with a minimum of 16 h of light per day to ensure the continuation of reproductive activity (Kovach, 1974). All mortality was monitored on a daily basis, and all females were subsequently weighed and monitored for reproductive investment (laying rate and egg mass across 7 days) at approximately 0.5 year intervals. Due to logistic challenges of keeping large numbers of males, and the difficulty of testing individual male reproductive performance independent of partner performance, we did not monitor male adult performance.
Statistical Analyses
The effect of the treatment (as a categorical fixed effect) on hatching success, and post-hatching survival to sexual maturity, were analyzed using generalized linear mixed models with a binominal error distribution and a logit link function. Treatment effects on incubation duration, body mass at hatching and subsequent growth (i.e. mass at day 7, day 14, day 28, day 42, and sexual maturity), and age at sexual maturity, were analyzed using linear mixed models with normal error distributions. In the model for body mass growth, age was treated as a categorical fixed effect, such that the effect of the treatment on body mass was tested at all ages in a single model (i.e., the interaction effect between treatment and age in Table 1). All models included parent pair identity as a random effect, and the model for body mass growth additionally included individual identity, nested within parent pair, as a random effect. For the analyses on growth, post-hatching survival to sexual maturity, and age at sexual maturity, sex (and its interaction with age) was included as a fixed effect to account for potential sex differences in development and survival to sexual maturity.
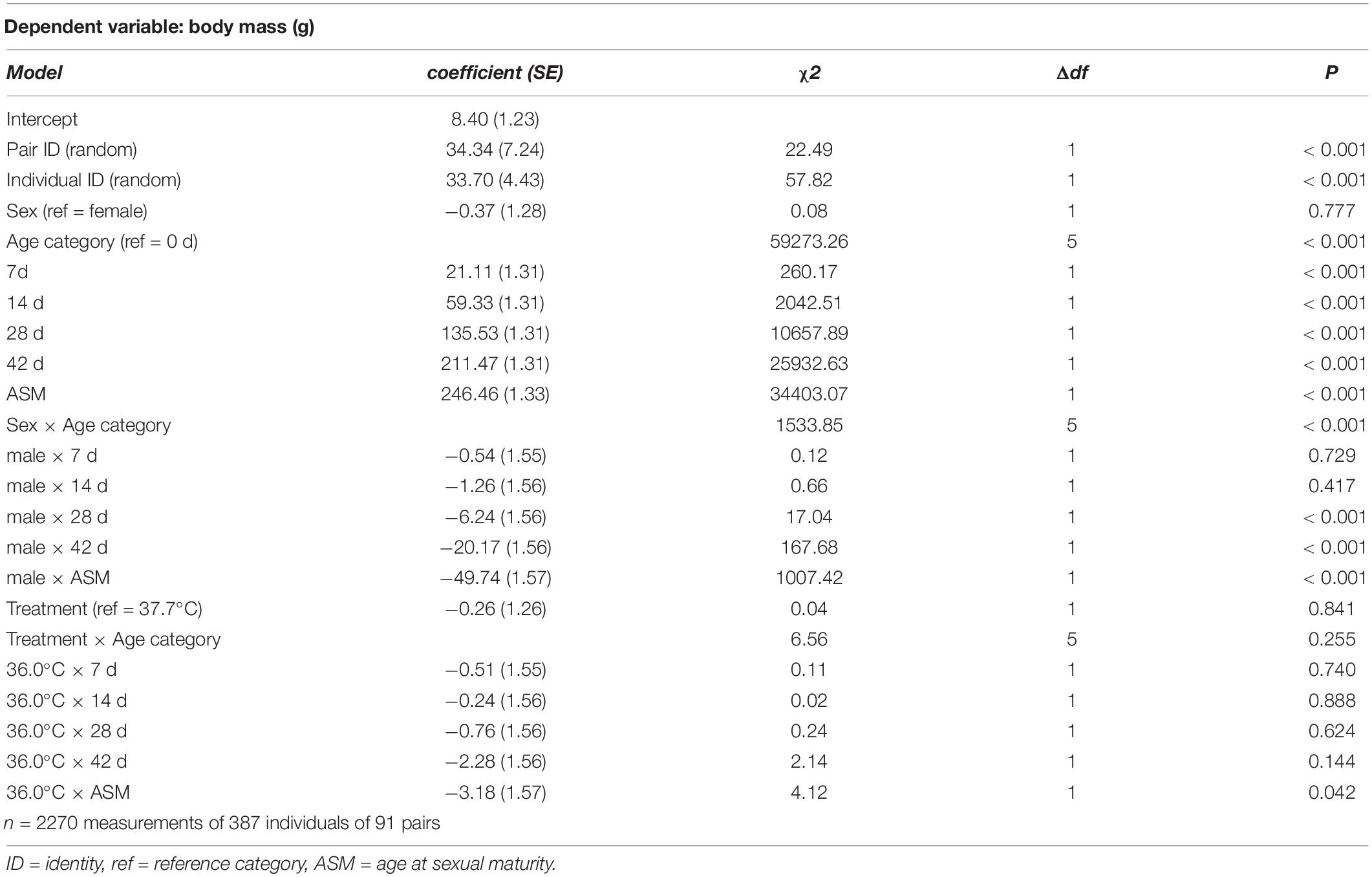
Table 1. Summary of a linear mixed model testing for effects of incubation treatment on age- and sex-specific body mass in Japanese quail chicks.
The adult females were, on average, weighed and monitored for reproductive performance (see above) at an age of 58, 243, 404, 591, 863, 1,012, 1,134, and 1,224 days, if they reached that age. Effects of the incubation treatment on adult body mass, laying rate, and egg mass, were analyzed together with age (in days) as a continuous variable. To account for non-linear effects of age, we also always added the quadratic term of age. We also included age at last sampling per individual (following van de Pol and Verhulst, 2006) to assure that the effect of age represents the within-individual pattern, unconfounded by individuals with non-random phenotypes (with respect to the dependent variable) not surviving to later sampling ages. To test whether the incubation treatment had an effect on the rate of senescence (i.e., a within-individual decline in performance with age) we also tested for the interaction between treatment and age. These models included parent pair identity, and individual identity (nested within parent pair) as random effects. The models for adult body mass and egg mass assumed a normal error distribution, and the model for laying rate a binominal error distribution with a logit link function. All aforementioned models were run in MLwiN (version 2.22; Rasbash et al., 2004) and significance (P < 0.05) was assessed using the Wald statistic.
Breakpoint models may better describe non-linear effects of age, can pinpoint the age of peak performance, and assess whether a decline in performance (senescence) occurs after the peak (e.g., Berman et al., 2009). However, in our case, they have the disadvantage that age should be treated as a discrete variable. We therefore repeated the analyses in which a trait peaked at middle age with breakpoint models, for which we binned our continuous age data to discrete ages representing the average age of all individuals in the 8 abovementioned measurement periods (see Supplementary Information).
We tested whether adult survival depended on the incubation treatment with a mixed-effects Cox model (Ripatti and Palmgren, 2000; Therneau et al., 2003; Nenko et al., 2018). This model included the treatment as explanatory variable and survival as response variable using the “coxme” function in the “coxme” R package (Therneau, 2018) implemented in R 3.5.3 (R Core Team, 2014). The identity of the parent pair was added as a random effect. The data were encoded with a zero as starting point for all individuals and with the lifespan until death (in days, max. = 1365, n = 180) as stop (Therneau, 2018).
Results
Early-Life Performance
Hatching success tended to decrease with incubation temperature, from 77.6% at 37.7°C to 70.2% at 36.0°C (χ2 = 3.60, Δdf = 1, P = 0.057). Lower temperature also delayed hatching, by roughly one day, due to longer development time (18.23 days vs. 17.16 days, coefficient ± SE = 1.07 ± 0.04 d, χ2 = 626.12, Δdf = 1, P < 0.001).
There was no evidence for body mass at hatching and subsequent growth to be affected by the incubation treatment (Figure 1 and Table 1). 96.6% of hatchlings survived to sexual maturity, and there was no indication for hatchling survival to be different between the incubation treatments (Table 2), nor was the age at sexual maturity different between the incubation treatments (Figure 1 and Table 2).
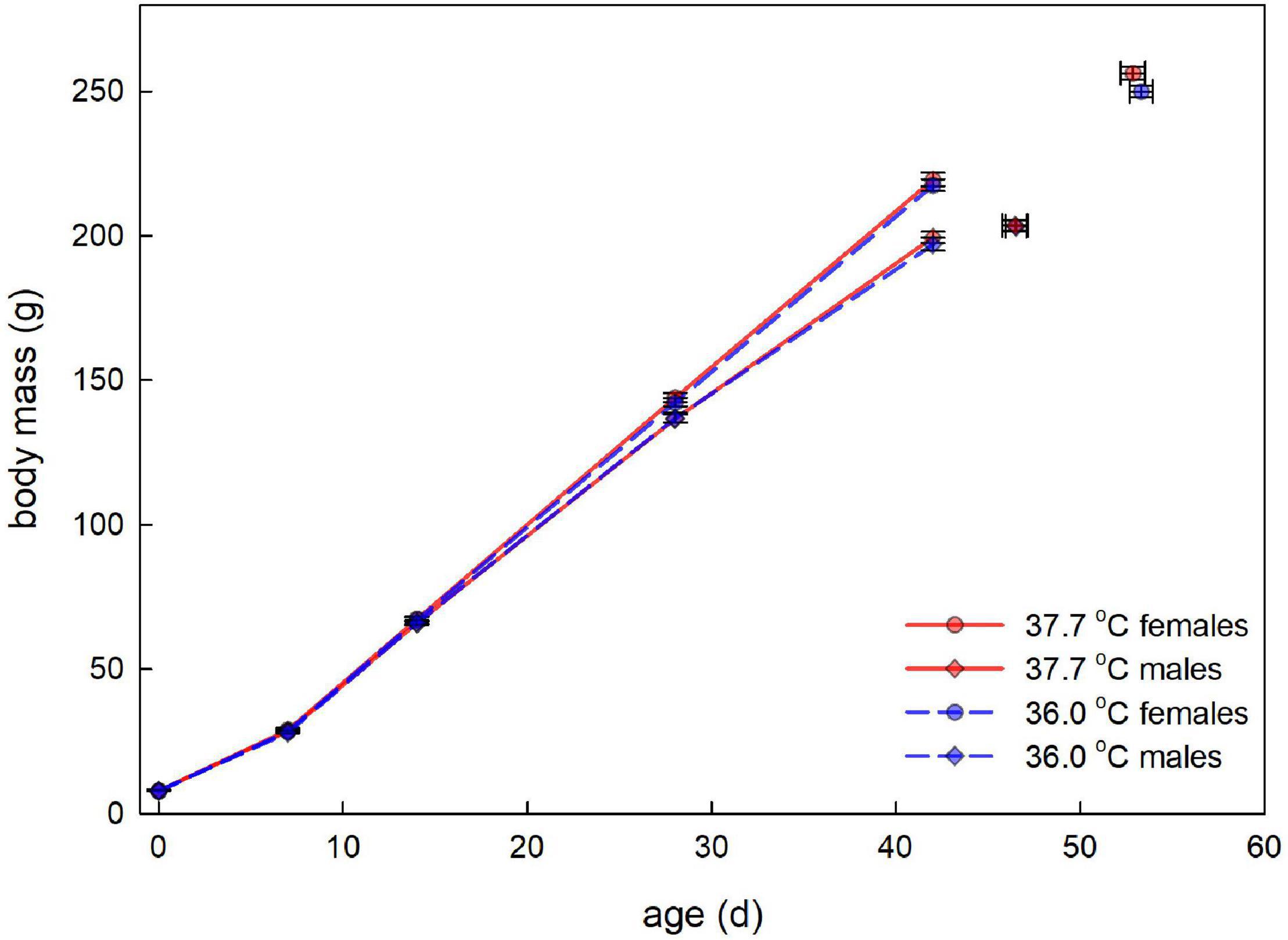
Figure 1. Post-hatching body mass (average ± SE) plotted against age for male and female Japanese quail incubated at 37.7°C (n = 203 hatchlings) or 36.0°C (n = 184 hatchlings). The lines represent the growth in the first six weeks after hatching. Although growth between 7 and 42 days old may appear linear, effects of the treatment were analyzed with age as a categorical variable (see Table 1). The final data points (with bi-directional error bars) represent the average age (± SE) and average mass (± SE) at sexual maturity, for each category.
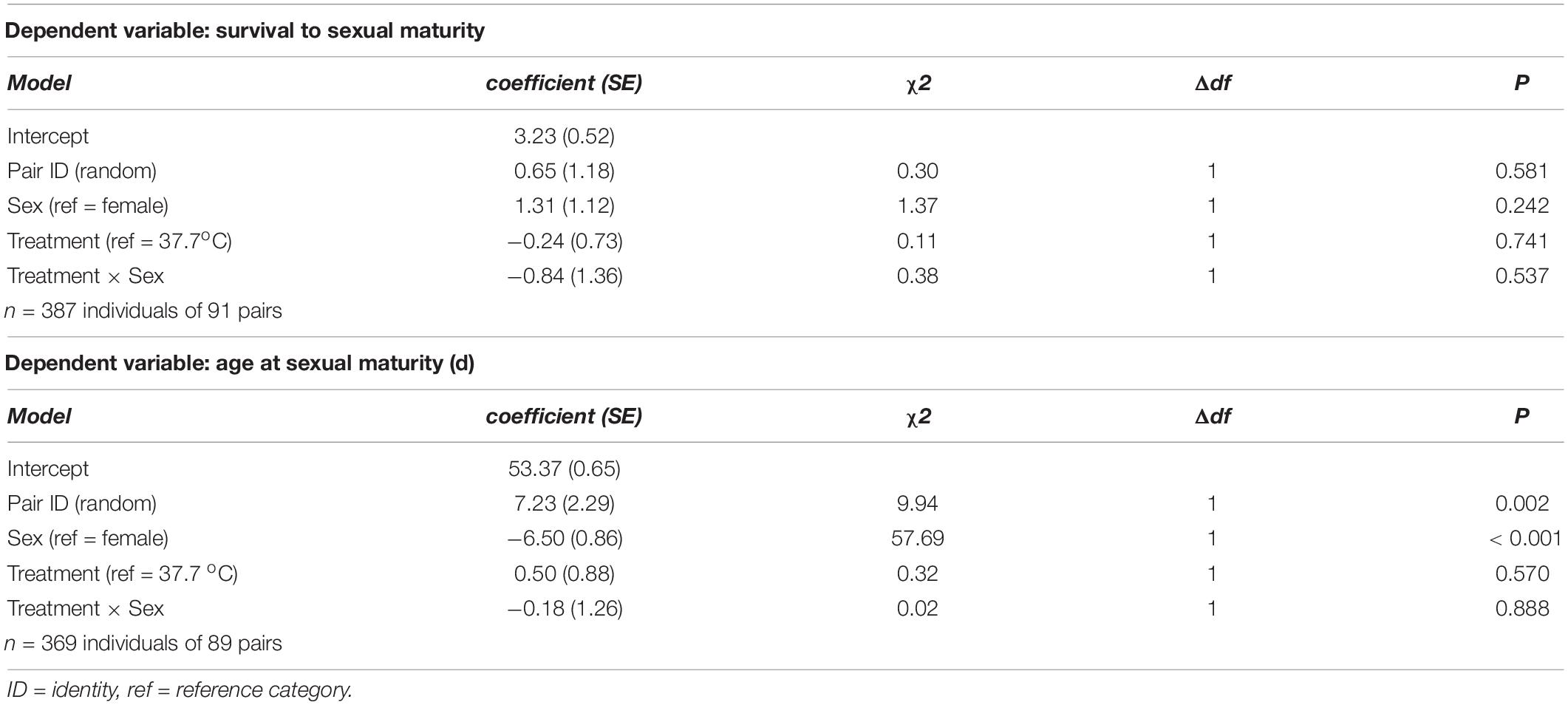
Table 2. Summary of (generalized) linear mixed models testing for effects of incubation treatment on sex-specific survival to sexual maturity, and age at sexual maturity, in Japanese quail chicks.
Adult Performance
Adult body mass peaked at middle age, as evidenced by a quadratic effect of age (Figure 2 and Table 3). Breakpoint modeling indicated this peak to occur around 404 days of age, after which body mass declined (Supplementary Figure 1A and Supplementary Table 1A). There was, however, no effect of the incubation treatment on adult body mass, or on how it changed with age (Figure 2 and Table 3). There was also no selective mortality in relation to body mass, as the last sampling age to which individuals survived was not related to body mass (Table 3).
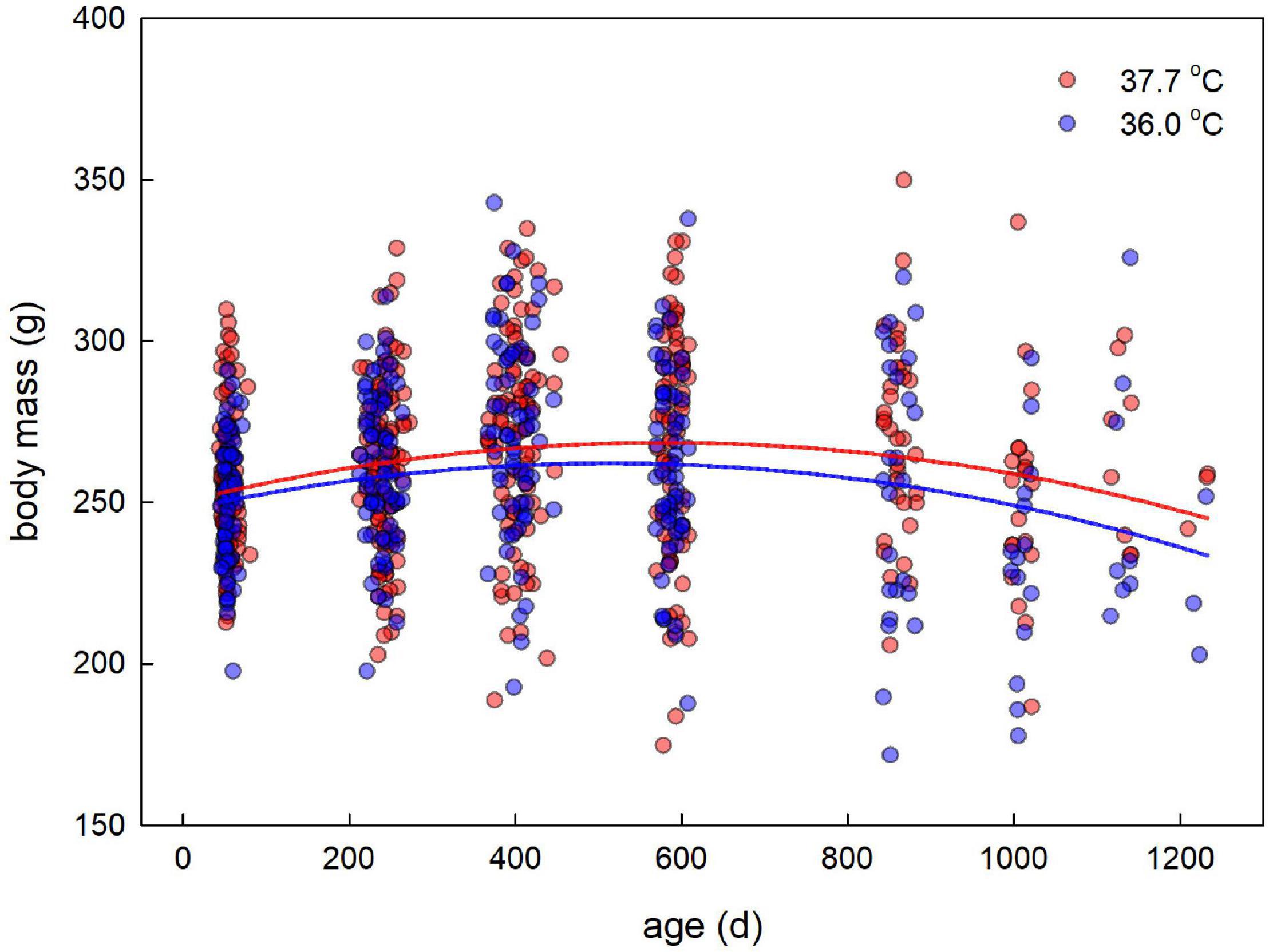
Figure 2. Adult body mass plotted against age for female Japanese quail incubated at 37.7°C (n = 99 females) or 36.0°C (n = 79 females). The lines represent the model prediction (see Table 3) for each incubation temperature.
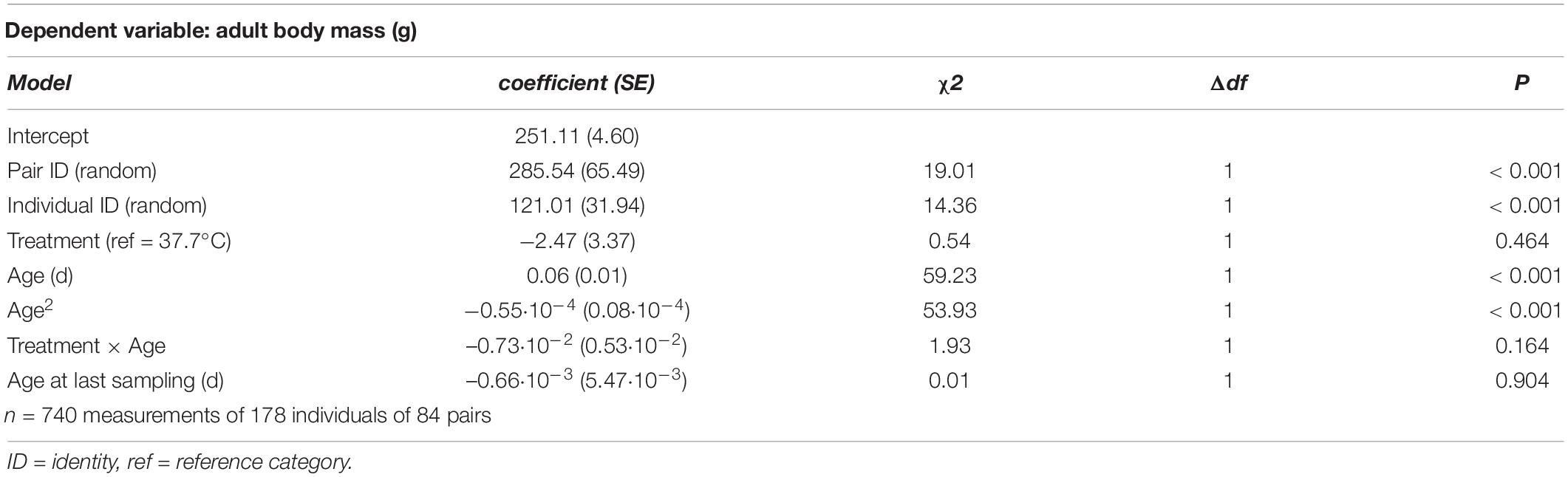
Table 3. Summary of a linear mixed model testing for effects of incubation treatment on age-specific adult body mass in Japanese quail females.
There was rapid senescence in reproductive performance, with laying rate strongly declining with age within individual females (Figure 3 and Table 4). Females that survived to later sampling ages had overall better reproductive performance (Figure 3 and Table 4), indicating selective mortality of poor layers. There was no evidence for the incubation treatment to have an effect on laying rate, or the change in laying rate with age (Figure 3 and Table 4). Investment in individual egg mass peaked at middle age, but also was not affected by the incubation treatment, or related to the last sampling age to which individuals survived (Figure 4 and Table 5). Breakpoint modeling indicated the peak in individual egg mass to occur around 243 days of age, after which there was relatively little change with age (Supplementary Figure 1B and Supplementary Table 1B).
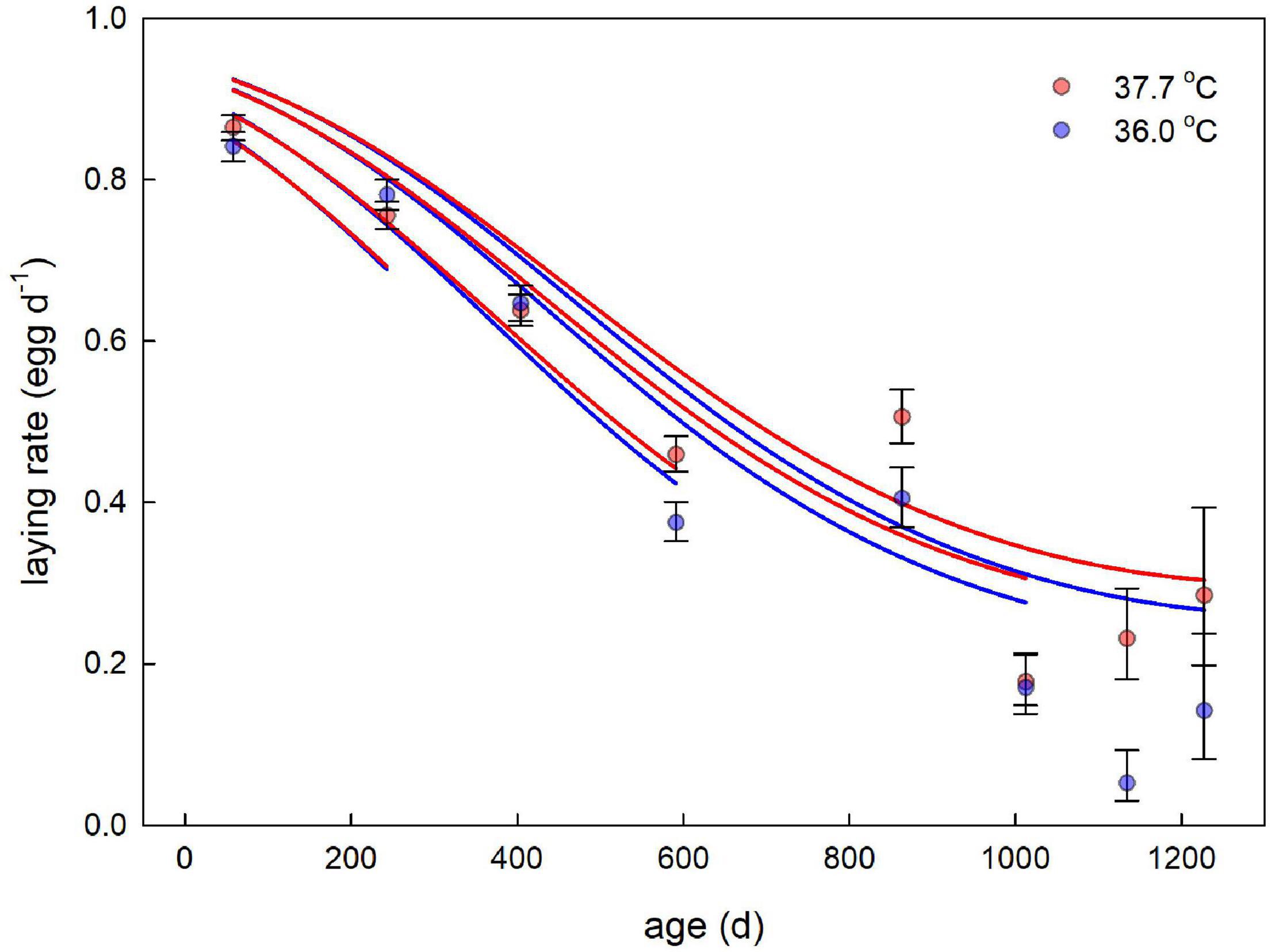
Figure 3. Egg laying rate plotted against age for female Japanese quail incubated at 37.7°C (n = 99 females) or 36.0°C (n = 80 females). Data points and error bars represent averages (± SE) based on the raw data. The lines represent the model predictions (see Table 4) for each incubation temperature, and several ages of last sampling, to illustrate the selective mortality of poor layers.
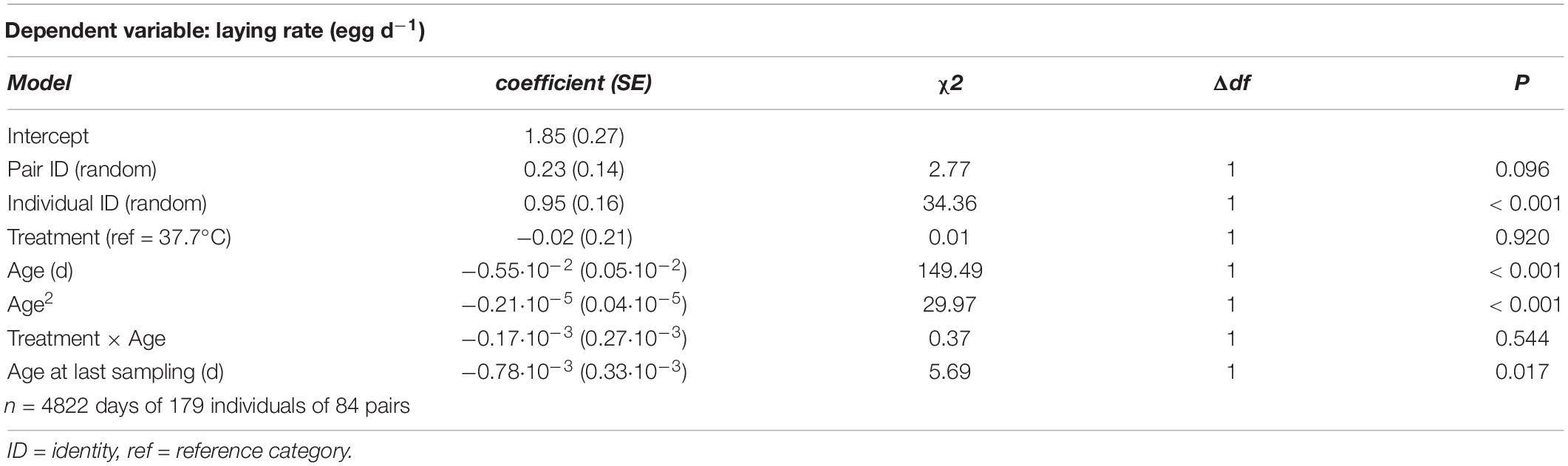
Table 4. Summary of a generalized linear mixed model testing for effects of incubation treatment on age-specific laying rate in Japanese quail females.
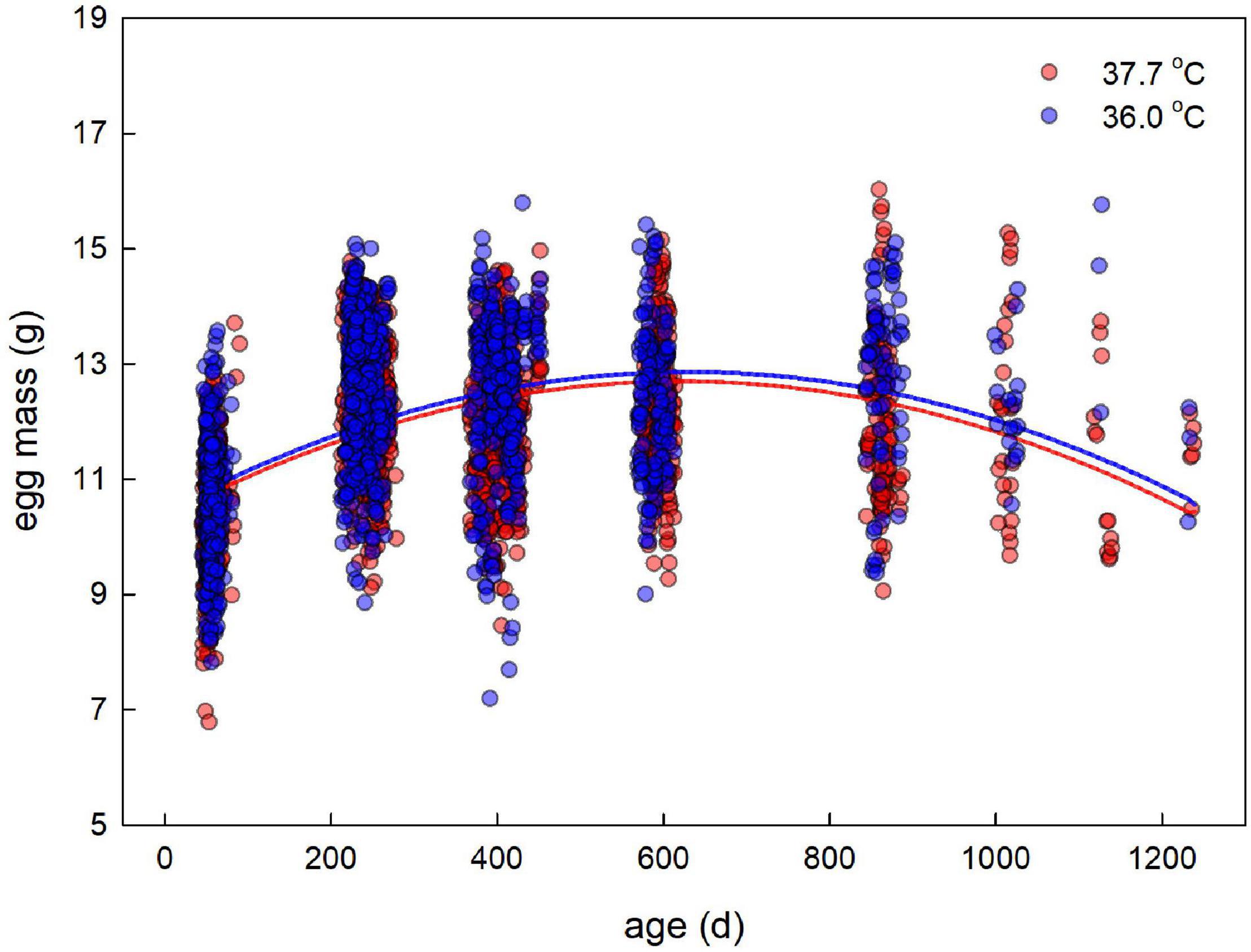
Figure 4. Mass of individual eggs laid plotted against age for female Japanese quail incubated at 37.7°C (n = 97 females) or 36.0°C (n = 79 females). The lines represent the model prediction (see Table 5) for each incubation temperature.
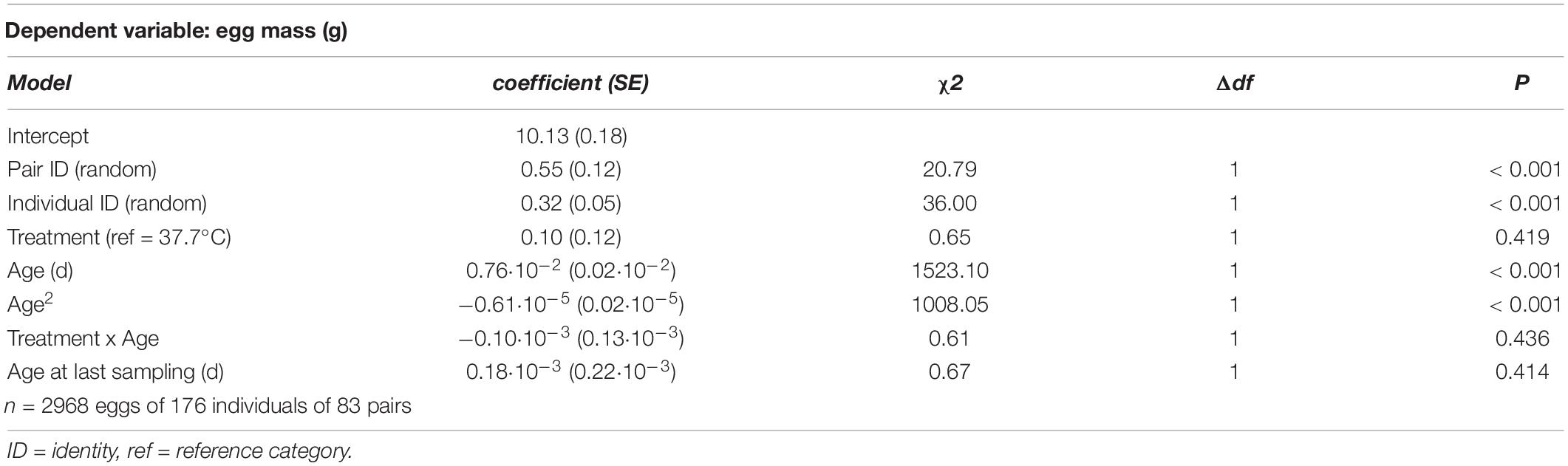
Table 5. Summary of a linear mixed model testing for effects of incubation treatment on age-specific egg mass in Japanese quail females.
Adult survival did not differ between the incubation treatments (Figure 5, Hazard ratio ± SE = 0.94 ± 0.18, Z-value = −0.36, P = 0.720).
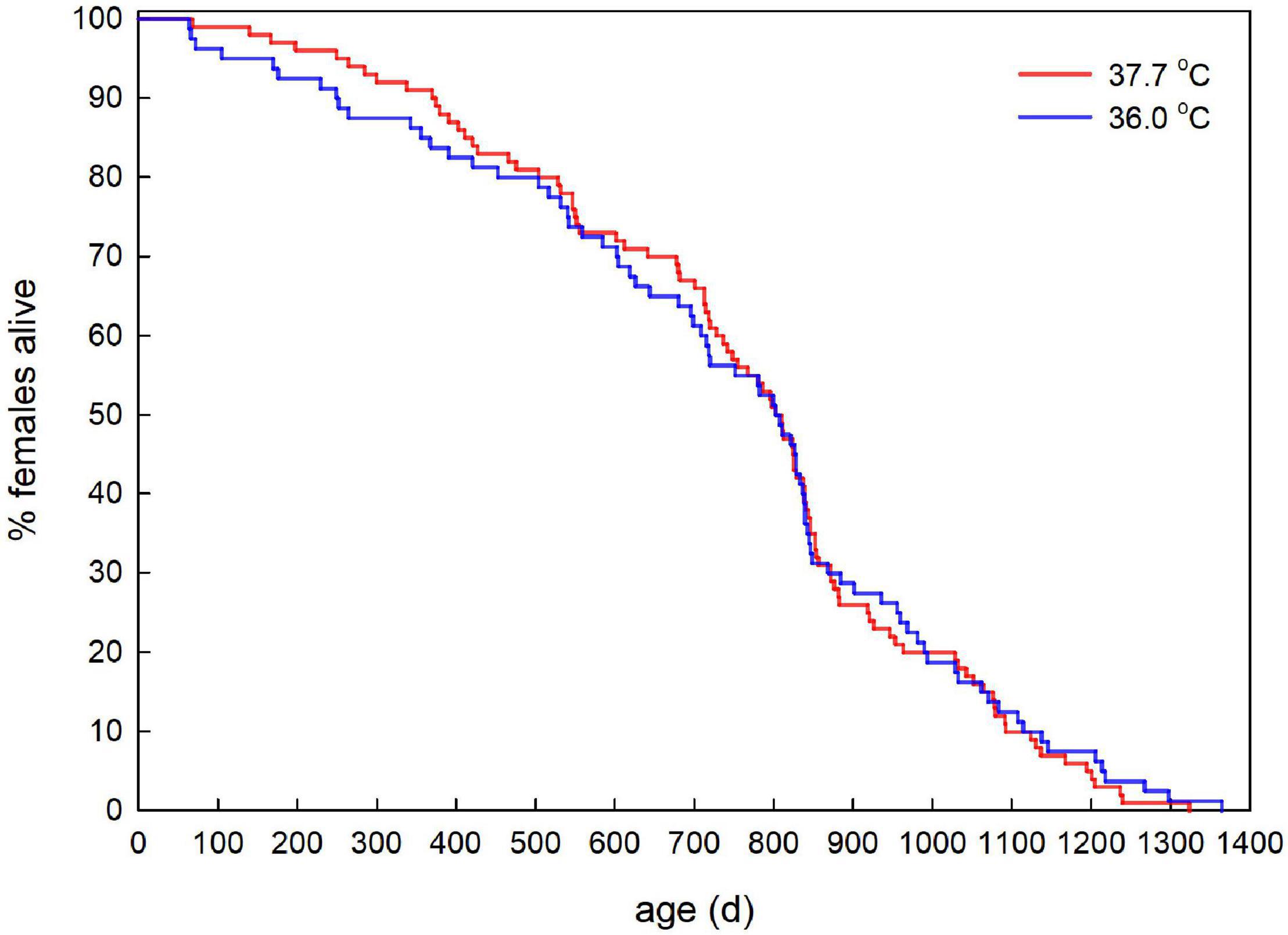
Figure 5. Age-specific survival of adult female Japanese quail incubated at 37.7°C (n = 100) or 36.0°C (n = 80).
Discussion
Our unique life-long assessment on the performance of Japanese quail exposed to experimentally manipulated conditions during embryonic development did not provide evidence for effects on performance beyond the period that was manipulated. The lower incubation temperature clearly slowed embryonic development, and reduced the viability of embryos from the first week of development to hatching. Despite the latter effect being just short of statistical significance, both results are in line with general findings in birds (Decuypere and Michels, 1992; DuRant et al., 2013). Since we were specifically interested in long-term effects of our incubation treatment, we did not aim to severely depress hatching success with an even lower incubation temperature. The general pattern of slower and less successful embryonic development with lower incubation temperature can be physically explained by lower temperature providing less energy to fuel biochemical reactions (Gillooly et al., 2002). As such, a lower incubation temperature will represent a resource limitation for the developing embryo, and can be conceptually compared to other types of stressful conditions experienced during development (e.g., decreased food availability, or increased requirement for immunocompetence) in the context of life-history theory.
As life-history theory is contingent on the existence of resource-based trade-offs between investment in growth, reproduction and (long-term) self-maintenance (Williams, 1966; Kirkwood, 1977; Stearns, 1992), it predicts that a reduced availability of resources to invest in these traits should reduce performance in one or more of these life-history traits. From an evolutionary perspective, it could be hypothesized that late-life performance should get compromised the most by resource limitation during development, because the strength of selection declines with age (Medawar, 1952; Hamilton, 1966). In other words, reduced performance at old age would cause a lesser reduction in fitness than reduced performance at young age. The ability to defer the cost of resource limitation in early life to later in life should thus be favored by natural selection. While we did find a reduced performance during embryonic development, in contrast with expectation, this effect did not carry through, or exacerbate, to post-hatching growth, adult body mass, reproduction and survival.
The absence of effects beyond the period that was manipulated may be explained by the physiological traits that underlie post-hatching performance in growth, survival and reproduction not being sensitive to thermal stress during embryonic development, limiting deferred fitness consequences. Alternatively, the relatively benign conditions under which our study animals lived (e.g., ad libitum food, no predation pressure) may have provided them with the opportunity to repair any damage incurred during embryonic development (Omholt and Kirkwood, 2021). Research on livestock selected on extreme productivity, as also relevant to Japanese quail, is equivocal in whether productivity is limited by resource acquisition, or by resource-based trade-offs with somatic maintenance (Douhard et al., 2021). The possibility to repair damage may occur only under favorable environmental conditions, which could be less frequent in nature and explain why long-term adverse effect are frequently reported among animals living in the wild (e.g., Kruuk et al., 1999; Reid et al., 2003; van de Pol et al., 2006; Nussey et al., 2007; Hamel et al., 2009; Spagopoulou et al., 2020). In the wild the acquisition of resources for somatic repair may be more constrained, either directly due to food limitation, or due to a trade-off between foraging and predation risk, causing further trade-offs between repair and late-life performance (Omholt and Kirkwood, 2021). Yet, studies in the wild can often not determine the environmental trait of interest a priori and establish causality experimentally. Moreover, no apparent long-term effects of poor early-life environment have been reported also in the wild (Wilkin and Sheldon, 2009; Vedder and Bouwhuis, 2018).
In birds, effects of incubation temperature on post-hatching phenotype are frequently reported, both in captivity and the wild (DuRant et al., 2010, 2012; Hopkins et al., 2011; Nord and Nilsson, 2011, 2021; Ben-Ezra and Burness, 2017; Vedder et al., 2018; Stier et al., 2020). However, studies that tested whether such effects translated to fitness consequences are rare (Hepp and Kennamer, 2012; Berntsen and Bech, 2016). Our study is unique in estimating all components of fitness (i.e., survival to reproduction, age at onset of reproduction, age-specific reproductive performance, and adult survival), without an ‘invisible fraction’ (Grafen, 1988). Since we did not find an effect of incubation temperature on fitness components beyond hatching success, we caution against over-interpreting the evolutionary relevance of effects on aspects of the phenotype without a clear link to fitness. In addition, we recommend studies that artificially incubate eggs in a conservation context to aim for incubation temperatures that maximize hatching success, as we did not find evidence for antagonistic effects on late-life fitness that could overrule the immediate benefit of surviving the embryonic stage. Nevertheless, we cannot rule out that fitness costs are transferred to the next generation, for instance if the offspring of the mothers that received the low incubation temperature would show impaired survival (Lemaître and Gaillard, 2017).
We did find that individuals that lived longer had a higher age-specific reproductive rate. A positive correlation between reproduction and survival is also frequently found in the wild, and indicative of heterogeneity in individual quality (Wilson and Nussey, 2010; Vedder and Bouwhuis, 2018). It has been hypothesized that such individual heterogeneity may stem from the quality of the early-life environment positively affecting both reproduction and survival (Vedder and Bouwhuis, 2018). However, our current study shows that heterogeneity in quality is not restricted to the wild. Furthermore, because post-hatching conditions were kept equal between individuals, and the experimentally manipulated incubation temperature had no effect on adult performance, we show that environmental variation may not be required to cause heterogeneity in individual quality. Other sources of individual variation (e.g., genetic or pre-natal maternal effects) may therefore be more promising candidates for future research aiming to explain heterogeneity in quality. For example, inbreeding has strong negative effects on various components of early-life fitness in Japanese quail (Sittmann et al., 1966) and our future research aims to include late-life performance, and estimate the effects of inbreeding over the complete life course. Although, the current experiment did not involve variation in parental age, the scope for transgenerational effects of aging and stress is increasingly recognized (Monaghan and Metcalfe, 2019) and also poses a promising research avenue into understanding the causes of heterogeneity in individual quality.
In conclusion, despite a very comprehensive study to the effects of experimentally manipulated embryonic conditions on performance in growth, survival, and reproduction over the complete life course of a precocial bird, we could not find evidence for effects that occurred beyond the period that was manipulated. We therefore suggest that long-term fitness consequences of the quality of the early-life environment may be less universal than often acclaimed, and caution against translating short-term phenotypic effects to fitness consequences, without knowledge on age-specific reproduction and survival.
Data Availability Statement
The raw data supporting the conclusions of this article will be made available by the authors, without undue reservation.
Ethics Statement
All procedures complied with current German law and did not require ethical review and approval.
Author Contributions
OV and BT: conceptualization and experimental design. OV: data collection. OV and CB: data analysis. OV: writing with feedback from BT and CB. All authors contributed to the article and approved the submitted version.
Funding
The study was funded by grant no. 428800869 from the German Research Foundation (DFG, Deutsche Forschungsgemeinschaft) to OV.
Conflict of Interest
The authors declare that the research was conducted in the absence of any commercial or financial relationships that could be construed as a potential conflict of interest.
Publisher’s Note
All claims expressed in this article are solely those of the authors and do not necessarily represent those of their affiliated organizations, or those of the publisher, the editors and the reviewers. Any product that may be evaluated in this article, or claim that may be made by its manufacturer, is not guaranteed or endorsed by the publisher.
Acknowledgments
We thank Chiti Arvind and Anna Kersten for help with the measurements, Adolf Völk for the husbandry of the quail, and Götz Wagenknecht for molecularly sexing the chicks that died prematurely. Sandra Bouwhuis and two reviewers provided comments on the manuscript.
Supplementary Material
The Supplementary Material for this article can be found online at: https://www.frontiersin.org/articles/10.3389/fevo.2022.834433/full#supplementary-material
References
Becker, P. H., and Wink, M. (2003). Influences of sex, sex composition of brood and hatching order on mass growth in common terns Sterna hirundo. Behav. Ecol. Sociobiol. 54, 136–146. doi: 10.1007/s00265-003-0605-4
Ben-Ezra, N., and Burness, G. (2017). Constant and cycling incubation temperatures have long-term effects on the morphology and metabolic rate of Japanese quail. Phys. Biochem. Zool. 90, 96–105. doi: 10.1086/688383
Berman, M., Gaillard, J. M., and Weimerskirch, H. (2009). Contrasted patterns of age-specific reproduction in long-lived seabirds. Proc. Royal Soc. B 276, 375–382. doi: 10.1098/rspb.2008.0925
Berntsen, H. H., and Bech, C. (2016). Incubation temperature influences survival in a small passerine bird. J. Avian Biol. 47, 141–145. doi: 10.1111/jav.00688
Birkhead, T. R., and Fletcher, F. (1994). Sperm storage and the release of sperm from the sperm storage tubules in Japanese quail Coturnix japonica. Ibis 136, 101–105. doi: 10.1111/j.1474-919x.1994.tb08137.x
Cooper, E. B., and Kruuk, L. E. B. (2018). Ageing with a silver-spoon: A meta-analysis of the effect of developmental environment on senescence. Evol. Lett. 2, 460–471. doi: 10.1002/evl3.79
Decuypere, E., and Michels, H. (1992). Incubation temperature as a management tool: a review. Worlds Poult. Sci. J. 48, 28–38. doi: 10.1079/wps19920004
Douhard, F., Douhard, M., Gilbert, H., Monget, P., Gaillard, J. M., and Lemaître, J. F. (2021). How much energetic trade-offs limit selection? Insights from livestock and related laboratory model species. Evol. Appl. 14:2726. doi: 10.1111/eva.13320
DuRant, S. E., Hepp, G. R., Moore, I. T., Hopkins, B. C., and Hopkins, W. A. (2010). Slight differences in incubation temperature affect early growth and stress endocrinology of wood duck (Aix sponsa) ducklings. J. Exp. Biol. 213, 45–51. doi: 10.1242/jeb.034488
DuRant, S. E., Hopkins, W. A., Hepp, G. R., and Walters, J. R. (2013). Ecological, evolutionary, and conservation implications of incubation temperature-dependent phenotypes in birds. Biol. Rev. 88, 499–509. doi: 10.1111/brv.12015
DuRant, S. E., Hopkins, W. A., Wilson, A. F., and Hepp, G. R. (2012). Incubation temperature affects the metabolic cost of thermoregulation in a young precocial bird. Funct. Ecol. 26, 416–422. doi: 10.1111/j.1365-2435.2011.01945.x
Eastwood, J. R., Hall, M. L., Teunissen, N., Kingma, S. A., Hidalgo Aranzamendi, N., Fan, M., et al. (2019). Early-life telomere length predicts lifespan and lifetime reproductive success in a wild bird. Mol. Ecol. 28, 1127–1137. doi: 10.1111/mec.15002
Garratt, M., Lemaitre, J.-F., Douhard, M., Bonenfant, C., Capron, G., Warnant, C., et al. (2015). High juvenile mortality is associated with sex-specific adult survival and lifespan in wild roe deer. Curr. Biol. 25, 759–763. doi: 10.1016/j.cub.2014.11.071
Gillooly, J. F., Charnov, E. L., West, G. B., Savage, V. M., and Brown, J. H. (2002). Effects of size and temperature on developmental time. Nature 417, 70–73. doi: 10.1038/417070a
Goodrich, S. H., and Roach, D. A. (2013). Effects of early-life environment on phenotype and selection in Agrostemma githago. Int. J. Plant Sci. 174, 877–885. doi: 10.1086/670366
Grafen, A. (1988). “On the use of data on lifetime reproductive success,” in Reproductive success: studies of individual variation in contrasting breeding systems, ed. T. H. Clutton-Brock (Chicago: University of Chicago Press), 454–471.
Hamel, S., Gaillard, J. M., Festa-Bianchet, M., and Cote, S. D. (2009). Individual quality, early-life conditions, and reproductive success in contrasted populations of large herbivores. Ecology 90, 1981–1995. doi: 10.1890/08-0596.1
Hamilton, W. D. (1966). Moulding of senescence by natural selection. J. Theor. Biol. 12, 12–45. doi: 10.1016/0022-5193(66)90184-6
Hayward, A. D., Rickard, I. J., and Lummaa, V. (2013). Influence of early-life nutrition on mortality and reproductive success during a subsequent famine in a preindustrial population. Proc. Natl. Acad. Sci. U.S.A. 110, 13886–13891. doi: 10.1073/pnas.1301817110
Heidinger, B. J., Blount, J. D., Boner, W., Griffiths, K., Metcalfe, N. B., and Monaghan, P. (2012). Telomere length in early life predicts lifespan. Proc. Natl. Acad. Sci. U.S.A. 109, 1743–1748. doi: 10.1073/pnas.1113306109
Hepp, G. R., and Kennamer, R. A. (2012). Warm is better: incubation temperature influences apparent survival and recruitment of wood ducks (Aix sponsa). PLoS One 7:e47777. doi: 10.1371/journal.pone.0047777
Hopkins, B. C., DuRant, S. E., Hepp, G. R., and Hopkins, W. A. (2011). Incubation temperature influences locomotor performance in young wood ducks (Aix sponsa). J. Exp. Zool. A Ecol. Genet. Physiol. 315, 274–279. doi: 10.1002/jez.673
Kovach, J. K. (1974). The behaviour of Japanese quail: Review of literature from a bioethological perspective. Appl. Anim. Ethol. 1, 77–102. doi: 10.1016/0304-3762(74)90010-8
Kruuk, L. E. B., Clutton-Brock, T. H., Rose, K. E., and Guinness, F. E. (1999). Early determinants of lifetime reproductive success differ between the sexes in red deer. Proc. Royal Soc. B 266, 1655–1661. doi: 10.1098/rspb.1999.0828
Lemaître, J. F., and Gaillard, J. M. (2017). Reproductive senescence: new perspectives in the wild. Biol. Rev. 92, 2182–2199. doi: 10.1111/brv.12328
Lummaa, V., and Clutton-Brock, T. (2002). Early development, survival and reproduction in humans. Trends Ecol. Evol. 17, 141–147. doi: 10.1016/s0169-5347(01)02414-4
Metcalfe, N. B., and Monaghan, P. (2001). Compensation for a bad start: grow now, pay later? Trends Ecol. Evol. 16, 254–260. doi: 10.1016/s0169-5347(01)02124-3
Mojica, J. P., and Kelly, J. K. (2010). Viability selection prior to trait expression is an essential component of natural selection. Proc. Royal Soc. B 277, 2945–2950. doi: 10.1098/rspb.2010.0568
Monaghan, P., and Metcalfe, N. B. (2019). The deteriorating soma and the indispensable germline: gamete senescence and offspring fitness. Proc. Royal Soc. B 286:20192187. doi: 10.1098/rspb.2019.2187
Nenko, I., Hayward, A. D., Simons, M. J. P., and Lummaa, V. (2018). Early-life environment and differences in costs of reproduction in a preindustrial human population. PLoS One 13:e0207236. doi: 10.1371/journal.pone.0207236
Nord, A., and Nilsson, J. A. (2011). Incubation temperature affects growth and energy metabolism in blue tit nestlings. Am. Nat. 178, 639–651. doi: 10.1086/662172
Nord, A., and Nilsson, J. A. (2016). Long-term consequences of high incubation temperature in a wild bird population. Biol. Lett. 12:20160087. doi: 10.1098/rsbl.2016.0087
Nord, A., and Nilsson, J. A. (2021). Low incubation temperature slows the development of cold tolerance in a precocial bird. J. Exp. Biol. 224:jeb237743. doi: 10.1242/jeb.237743
Nussey, D. H., Kruuk, L. E. B., Morris, A., and Clutton-Brock, T. H. (2007). Environmental conditions in early life influence ageing rates in a wild population of red deer. Curr. Biol. 17, R1000–R1001. doi: 10.1016/j.cub.2007.10.005
Omholt, S. W., and Kirkwood, T. B. (2021). Aging as a consequence of selection to reduce the environmental risk of dying. Proc. Natl. Acad. Sci. U.S.A. 118:e2102088118. doi: 10.1073/pnas.2102088118
Ottinger, M. A. (2001). Quail and other short-lived birds. Exp. Gerontol. 36, 859–868. doi: 10.1016/s0531-5565(00)00246-1
Plard, F., Gaillard, J. M., Coulson, T., Hewison, A. J. M., Douhard, M., Klein, F., et al. (2015). The influence of birth date via body mass on individual fitness in a long-lived mammal. Ecology 96, 1516–1528. doi: 10.1890/14-0106.1
Rasbash, J. R., Steele, F. A., Browne, W. J., and Prosser, B. (2004). A user’s guide to MLwiN version 2.0. London: Centre for Multilevel Modelling, University of Bristol.
Reid, J. M., Bignal, E. M., Bignal, S., McCracken, D. I., and Monaghan, P. (2003). Environmental variability, life-history covariation and cohort effects in the red-billed chough Pyrrhocorax pyrrhocorax. J. Anim. Ecol. 72, 36–46. doi: 10.1046/j.1365-2656.2003.00673.x
Ripatti, S., and Palmgren, J. (2000). Estimation of multivariate frailty models using penalized partial likelihood. Biometrics 56, 1016–1022. doi: 10.1111/j.0006-341x.2000.01016.x
Ronget, V., Gaillard, J. M., Coulson, T., Garratt, M., Gueyffier, F., Lega, J. C., et al. (2018). Causes and consequences of variation in offspring body mass: Meta-analyses in birds and mammals. Biol. Rev. 93, 1–27. doi: 10.1111/brv.12329
Sachs, B. D. (1969). Photoperiodic control of reproductive behavior and physiology of the male Japanese quail (Coturnix coturnix japonica). Horm. Behav. 1, 7–24. doi: 10.1016/0018-506x(69)90002-6
Sittmann, K., Abplanalp, H., and Fraser, R. A. (1966). Inbreeding depression in Japanese quail. Genetics 54:371. doi: 10.1093/genetics/54.2.371
Spagopoulou, F., Teplitsky, C., Chantepie, S., Lind, M. I., Gustafsson, L., and Maklakov, A. A. (2020). Silver-spoon upbringing improves early-life fitness but promotes reproductive ageing in a wild bird. Ecol. Lett. 23, 994–1002. doi: 10.1111/ele.13501
Stier, A., Metcalfe, N. B., and Monaghan, P. (2020). Pace and stability of embryonic development affect telomere dynamics: an experimental study in a precocial bird model. Proc. Royal Soc. B 287:20201378. doi: 10.1098/rspb.2020.1378
Therneau, T. M. (2018). coxme: Mixed Effects Cox Models. Available online at: https://CRAN.R-project.org/package=coxme (accessed date 2020-01-14).
Therneau, T. M., Grambsch, P. M., and Pankratz, V. S. (2003). Penalized survival models and frailty. J. Comput. Graph. Stat. 12, 156–175. doi: 10.1198/1061860031365
van de Pol, M., Bruinzeel, L. W., Heg, D., van der Jeugd, H. P., and Verhulst, S. (2006). A silver spoon for a golden future: long-term effects of natal origin on fitness prospects of oystercatchers (Haematopus ostralegus). J. Anim. Ecol. 75, 616–626. doi: 10.1111/j.1365-2656.2006.01079.x
van de Pol, M., and Verhulst, S. (2006). Age-dependent traits: a new statistical model to separate within-and between-individual effects. Am. Nat. 167, 766–773. doi: 10.1086/503331
Vedder, O., and Bouwhuis, S. (2018). Heterogeneity in individual quality in birds: overall patterns and insights from a study on common terns. Oikos 127, 719–727. doi: 10.1111/oik.04273
Vedder, O., Pen, I., and Bouwhuis, S. (2021). How fitness consequences of early-life conditions vary with age in a long-lived seabird: A Bayesian multivariate analysis of age-specific reproductive values. J. Anim. Ecol. 90, 1505–1514. doi: 10.1111/1365-2656.13471
Vedder, O., Verhulst, S., Zuidersma, E., and Bouwhuis, S. (2018). Embryonic growth rate affects telomere attrition: an experiment in a wild bird. J. Exp. Biol. 221:jeb181586. doi: 10.1242/jeb.181586
Wilkin, T. A., and Sheldon, B. C. (2009). Sex differences in the persistence of natal environmental effects on life histories. Curr. Biol. 19, 1998–2002. doi: 10.1016/j.cub.2009.09.065
Williams, G. C. (1966). Natural selection costs of reproduction and a refinement of Lack’s principle. Am. Nat. 100, 687–690. doi: 10.1086/282461
Wilson, A. J., and Nussey, D. H. (2010). What is individual quality? An evolutionary perspective. Trends Ecol. Evol. 25, 207–214. doi: 10.1016/j.tree.2009.10.002
Keywords: aging, birds, developmental conditions, incubation, lifespan, lifetime reproductive success, senescence, silver spoon effect
Citation: Vedder O, Bichet C and Tschirren B (2022) The Effect of Manipulated Prenatal Conditions on Growth, Survival, and Reproduction Throughout the Complete Life Course of a Precocial Bird. Front. Ecol. Evol. 10:834433. doi: 10.3389/fevo.2022.834433
Received: 13 December 2021; Accepted: 09 March 2022;
Published: 14 April 2022.
Edited by:
Jean-Francois Lemaitre, UMR 5558 Biométrie et Biologie Evolutive (LBBE), FranceReviewed by:
Jack Thorley, University of Cambridge, United KingdomRémi Fay, Swiss Ornithological Institute, Switzerland
Copyright © 2022 Vedder, Bichet and Tschirren. This is an open-access article distributed under the terms of the Creative Commons Attribution License (CC BY). The use, distribution or reproduction in other forums is permitted, provided the original author(s) and the copyright owner(s) are credited and that the original publication in this journal is cited, in accordance with accepted academic practice. No use, distribution or reproduction is permitted which does not comply with these terms.
*Correspondence: Oscar Vedder, b3NjYXIudmVkZGVyQGlmdi12b2dlbHdhcnRlLmRl