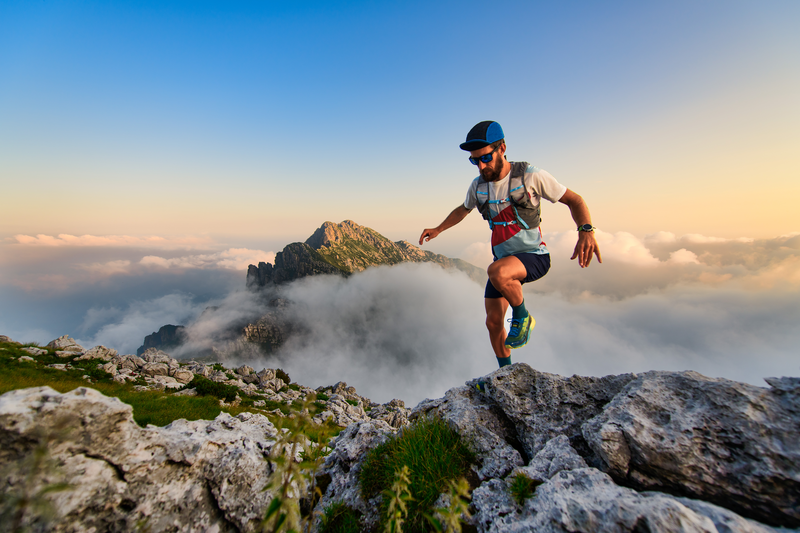
94% of researchers rate our articles as excellent or good
Learn more about the work of our research integrity team to safeguard the quality of each article we publish.
Find out more
ORIGINAL RESEARCH article
Front. Ecol. Evol. , 15 April 2022
Sec. Behavioral and Evolutionary Ecology
Volume 10 - 2022 | https://doi.org/10.3389/fevo.2022.834074
This article is part of the Research Topic Optimal Bird Migration: Implications for Navigation, Physiology, and Stopover Ecology View all 8 articles
Many migratory species have advanced their migration timing as a response to advanced breeding conditions. While data on arrival timing to breeding grounds in Europe is plentiful, information from the African departure sites are scarce. Here we investigated changes in arrival timing of four long-distance migratory passerines to a stopover site in Israel and potential links to Enhanced Vegetation Index (EVI) at the species-specific African pre-departure sites and local temperatures at the stopover site. We found that Lesser whitethroat (Curruca curruca) and Eastern Bonelli’s warbler (Phylloscopus orientalis) advanced arrival to the stopover site. The arrival timing of Thrush nightingale (Luscinia luscinia) and Olive-tree warbler (Hippolais olivetorum) did not change and was associated with mean EVI at the pre-departure site in Africa during the pre-migratory period. Additionally, temperatures at the stopover site affected the arrival timing of Lesser whitethroat only. This is probably because this species breed at higher northern latitudes and fine-tune their migration timing to match local conditions. Our results show that spring migration can be influenced by exogenous cues such as weather condition and food availability, and the level of response is species-specific. Moreover, some species show flexibility and fine-tuned migration speed in response to local conditions en route. While flexibility seems advantageous, dependence on multiple sites with varying conditions may ultimately limit advanced arrival to the breeding ground and result in mismatch with optimal conditions.
Migratory birds must time their migration to synchronize with resource availability en-route and most importantly on the breeding site. Early arrival is advantageous because the first individuals can take advantage of optimal nesting locations and mate choices (Kokko, 1999). A major challenge for long-distance migratory birds is that they often rely on multiple sites along their migration route, frequently on a latitudinal gradient, with spatial variability in conditions (Newton, 2008). The extensive, and asymmetrical pace of global changes, with higher rate of change in higher latitudes (Flato and Boer, 2001; Burrows et al., 2011), poses a risk of mismatch between arrival timing and optimal condition of food abundance and temperatures and may have detrimental consequences for populations (Møller et al., 2008; Jones and Cresswell, 2010; Saino et al., 2011; Mayor et al., 2017; Burgess et al., 2018). It is therefore not surprising that the adaptation of migration phenology in relation to climatic changes has attracted great attention in recent years (e.g., Jonzén et al., 2006; Tøttrup et al., 2008, 2012; Lawrence et al., 2021). Many long-distance migratory species show advanced arrival to their breeding grounds, potentially to match advanced onset of breeding season across the northern hemisphere (e.g., Tryjanowski et al., 2002; Sparks et al., 2005; Jonzén et al., 2006; Horton et al., 2020). The cues these long-distance migrants use to start migration, and what mechanism generates adjustment of migration timing, to match conditions in a distant breeding site, remain poorly understood, especially in passerine species.
The timing of migration has been long known to be predominantly controlled by genetic and intrinsic cues (Gwinner and Wiltschko, 1978; Gwinner, 1996), but also by external local conditions. These include photoperiod (Marra et al., 2005; Bradshaw and Holzapfel, 2008; Åkesson et al., 2021), wind assistance to reduce flight costs (e.g., Arizaga et al., 2011; Sjöberg et al., 2015) and environmental conditions, such as temperature, rain and different vegetation indices which can be used as a proxy for food availability at the pre-departure sites (Saino et al., 2004; Shamoun-Baranes et al., 2006; Tøttrup et al., 2012; Aharon-Rotman et al., 2021; Lawrence et al., 2021). The latter likely affect migration decision, the physical condition of the birds, their fattening rates, and hence the timing of departure (Marra et al., 1998; Arizaga et al., 2011; Aharon-Rotman et al., 2016; Aloni et al., 2019).
However, the advanced arrival of some long-distance migrants to the breeding ground, often thousands of kilometres away from departure sites, suggest that endogenous cues and local external conditions at the non-breeding (wintering) ground are not solely responsible for driving migration decisions. Two possible mechanisms have been suggested: (a) climate-driven evolutionary changes in the onset of spring migration through selection for early arrivals over a short period (Pulido et al., 2001; Jonzén et al., 2006; Gienapp et al., 2007) or a shift through generational changes as a results of new recruits to the population arriving earlier (Gill et al., 2014), and (b) Phenotypic plasticity in response to year-to-year variation in local conditions encountered en route (Ahola et al., 2004; Tøttrup et al., 2010; Bussière et al., 2015; Haest et al., 2020). It is possible that the generally advanced trend in migratory birds is a result of phenotypic plasticity and micro-evolutionary forces acting in concert.
Our current understanding of phenological changes in passerines migrating between Africa and Europe is largely based on data collected at the European breeding grounds, where many migrants advanced their arrival (e.g., Saino et al., 2004; Rubolini et al., 2007; Thorup et al., 2007), or at the western part of the migration route, where birds migrate from wintering sites in western Africa through Gibraltar to various breeding locations across Europe (e.g., Haest et al., 2020; Bell et al., 2021; Lawrence et al., 2021). The main wintering and pre-departure sites for many long-distance species in the eastern part of the route, along the Eastern-Mediterranean flyway, are in the Afrotropical zone, where extreme climatic events have previously been correlated with declines in European breeding populations (Payevsky et al., 2003; Newton, 2004; Ockendon et al., 2014; Vickery et al., 2014). Despite their presumed importance, we still lack critical information on species-specific wintering ranges (Walther and Rahbek, 2002; Bairlein, 2003), and only few studies on passerine species have examined species-specific phenological changes together with their possible drivers, such as response to changing conditions at these sites (Altwegg et al., 2012; Bussière et al., 2015; Haest et al., 2020; Aharon-Rotman et al., 2021).
To fully understand phenological changes in migratory species, it is necessary to consider other factors rather than only the mean or median arrival day. Analysing only the first or mean arrival day does not comprehensively describe phenological dynamics or sex and age differences (Spina et al., 1994; Izhaki and Maitav, 1998; Tøttrup and Thorup, 2008), and may fail to identify trends (Kovács et al., 2011; Miles et al., 2017). Therefore, other population measures should be considered. Further, different phenological changes may occur among spatially-segregated populations. To address this issues, wing length measurements may be considered, as wing length could serve as a proxy measure to differentiate between the sexes and among spatially-segregated populations in migratory passerines (Milá et al., 2008; Förschler and Bairlein, 2011). This is based on the correlation between wing length and migration distance or phenology at breeding destinations in many species (Fiedler, 2005; Nowakowski et al., 2014; Hahn et al., 2016; Toews et al., 2017).
Our aim was to study the relationship between spring migration phenology and environmental factors at the African pre-departure sites and at a stopover site in four long distance migratory passerine species. To this end, we first investigated any phenological changes in the arrival of these species to an important stopover site in Israel between 2000 and 2021. Next, we tested if environmental conditions at the departure site in Africa, measured as Enhanced Vegetation Index (EVI), and local temperatures at the stopover site can explain variation in arrival timing. We also analysed changes in wing length as a proxy for migration distance, to identify potentially different populations within the individuals stopping in Israel or age-biased differences. We hypothesised that high EVI at the departure site and higher spring temperatures at the stopover site will facilitate earlier departure due to fast fuelling and flexibility to fine-tune migration speed as a response to conditions en route.
The Nili and David Jerusalem Bird Observatory (JBO) in Israel (31.780°N, 35.206°E) is an important stopover site for species that migrate between the Afrotropical wintering grounds and breeding grounds in the Palearctic region. The JBO is located in the city of Jerusalem within a large area of parks surrounding the Israeli Parliament building. The elevation is 805 m above sea level and the climate is Mediterranean, with an average annual rainfall of approximately 550 mm. Low trees and bushes typical of the Judean mountains dominate the vegetation. Typical plants are Pistacia palaestina, Rhamnus alaternus, Prunus dulcis (almond), and Olea europea (olive).
We selected four long-distance migratory passerines. All four species spend the main non-breeding season in Africa, depart for spring migration from sub-Sahara East Africa to breeding sites in Europe and Western Asia, and stop in Israel during spring migration. Our selected species were Lesser whitethroat (Sylvia curruca), Eastern Bonelli’s warbler (Phylloscopus orientalis), Thrush nightingale (Luscinia luscinia) and Olive-tree warbler (Hippolais olivetorum).
Olive-tree warbler and Thrush nightingale both winter in southern Africa (Figure 1; Underhill et al., 1998; Tøttrup et al., 2012). However, before leaving Africa on spring migration towards the breeding grounds, Thrush nightingale stop in the Horn of Africa for the pre-migratory period (Tøttrup et al., 2012), and it is highly likely that Olive-tree warbler do the same. The wintering ranges of Lesser whitethroat and Eastern Bonelli’s warbler are located in the Afrotropical zone. The main wintering area of Eastern Bonelli’s warbler is within Sudan, while the wintering area of Lesser whitethroat extends to Chad (BirdLife International, 2019; Figure 1).
Figure 1. Main Non-breeding (wintering) distribution maps of the study species along the Eastern-Mediterranean flyway. The area of Horn of Africa was used as the pre departure area for Lesser whitethroat, Thrush nightingale and Olive-tree warbler, where they are likely to spend the months prior to migration departure. GIS data downloaded from BirdLife International data zone (http://www.birdlife.org/). Enhanced Vegetation Index (EVI) was calculated for each site to be analysed against arrival timing of species to stopover site in Israel.
The breeding grounds of Thrush nightingale and Lesser whitethroat expand over vast areas in Europe and Asia, while the breeding areas of Eastern Bonelli’s warbler and Olive-tree warbler are concentrated in southeast Europe and northeast Mediterranean, stretching from the eastern Adriatic and Balkan Peninsula to western and southern Turkey and north Israel (BirdLife International, 2019). Olive-tree warbler inhabit mainly coastal areas and islands (Kralj et al., 2012).
Birds were caught using mist-nets positioned in permanent locations throughout the study period. We considered only individuals captured during the main spring migration season (March-May), as over 96% of captured individuals were recorded on passage during these months in the study species. Each bird was aged, if possible, and measured by experienced ringers (wing length, fat score and weight), and date of capture was recorded. Age could be identified in the hand only in Lesser whitethroat and Thrush nightingale. We extracted spring arrival dates in Israel as the first ordinal date of capture (days from January 1st). For each individual we only considered the first capture in a migration season. Recaptures during the same season were excluded from the analysis.
We calculated the monthly normalized EVI during the pre-migratory period (prior to the birds’ departure; February/March) for the period 2000–2020 in the species-specific pre-departure areas (Figure 1). The EVI can be used as a proxy measure for the actual ecological conditions of vegetation phenology (e.g., food availability; Houborg et al., 2007; Papeş et al., 2012). The EVI was developed to determine photosynthetic activity in high biomass regions (Huete et al., 2002). For Lesser whitethroat and Eastern Bonelli’s warbler, this area was based on wintering distribution maps from BirdLife International (2019). Using QGIS 3.6.0 (QGIS Development Team, 2021,1), we selected the wintering ranges based on the parameter “season” of the attribute table of the dataset. For Lesser whitethroat we followed Wisz et al. (2007), which further restricted the area based on bioclimatic models. This resulted in two separate areas for Lesser whitethroat: (1) around Chad and Sudan (2) the Horn of Africa. For Thrush nightingale and Olive-tree warbler, we calculated EVI during the same period in the Horn of Africa to be used as pre-departure areas. These two species winter in Southern Africa, and it is therefore likely that before crossing the Sahara desert on northward migration to the breeding ground, they stop in the Horn of Africa and may use cues in this region to initiate migration (e.g., Tøttrup et al., 2012). For all species, we clipped the final African ranges to remove areas of bare ground (e.g., rocks and desert) which were unlikely to provide viable habitat for migratory birds using the European Space Agency’s global land cover map from 20092 at 2.5 minutes resolution.
We used Google Earth Engine (GEE), a cloud-based remote-sensing platform, to calculate mean EVI across the wintering range for each species. We used NASA’s MODIS Terra Vegetation Indices product (Didan, 2015), a global collection of 16-day composite images at 1km spatial scale which provides Normalized Vegetation Density Index (NDVI) and EVI. In GEE, we calculated the mean EVI across the wintering ranges for each species and for the Horn of Africa. We repeated this process for all the images during 2000–2020. Then we calculated the monthly mean EVI for each of the pre-migratory months February and March over the years for each species. This produced a data set with monthly means of EVI for February and March for each year from 2000 to 2020 for each area.
Local mean temperatures at Giv’at Ram weather station in Jerusalem, the closest station to the JBO, were downloaded for the main spring migration period from the Israel Meteorological Service.3 Daily temperatures were averaged to monthly means.
We estimated trends in the migration phenology of each species (2000–2021) using four population measures: the 5th, 50th (median) and 95th percentile dates, as well as the mean arrival day. In the Eastern Bonelli, due to small sample size, we analyse phenology data between 2004 and 2021 only. For this purpose, we fitted separate regression lines using generalised linear mixed models (GLMM) for each of the quantile specified. Arrival day (in each of the quantiles) or mean arrival day were set as the response variable, with age (second year or adults; if known) and year of capture as explanatory variables as well as the interactions between year and age. Note that accounting for age was possible only in two species: Lesser whitethroat and Thrush nightingale. We accounted for age in the statistical models because some species show differences in arrival timing between age groups (Smith and Moore, 2005). Year was included as both fixed and random effect to account for pseudo-replication. Negative and positive slopes depict that migration was advanced or delayed, respectively.
We applied a GLMM to describe any temporal changes in wing length over the study period. We also hypothesised that wing length varies with season, due to sex or age-biased migration phenology, or because individuals may come from different populations and their wing length may correspond to their flight distance (Fiedler, 2005; Nowakowski et al., 2014; Toews et al., 2017). To account for this variation, we divided our bird capture data from the entire migration season into early and late arrival phases. While arbitrary, by using a cut off at the 30th percentile of each end of the arrival period we can capture a substantial proportion of early and late individuals arriving during the migration season. Annual mean wing length was set as a response variable with age (second year and adults; where known), arrival group (early or late) and year of capture as explanatory variables, as well as the interactions between all variables to account for any differences in the slopes between the age and arrival groups. Year was also set as a random effect. We accounted for age in the statistical model because some species show morphological differences in wing length between age groups (Kiat and Sapir, 2018). Post hoc test was performed when interaction was significant using estimated marginal means of the linear trends to evaluate differences between the groups.
For Eastern Bonelli’s warbler, due to low sample size (Supplementary Table 1), we ran a GLMM for the individual wing length across the study period (to avoid dividing the dataset into early and late phases and lose individual measurements). Wing length was set as a response variable, with year and arrival day as explanatory variables, and year of capture set as a random effect.
We used linear models to analyse the relationship between arrival dates to the stopover site in Israel and environmental conditions on the wintering ground and stopover site in each species. For Olive-tree warbler and Eastern Bonelli’s warbler, we set the mean annual arrival day as the response variable with average EVI in February and March, and temperatures in Jerusalem in March, April and May as explanatory variables. For Lesser whitethroat and Thrush nightingale, we used GLMM to also account for variation between age groups. To this end, we include age as an explanatory variable and interaction terms between age and each of the explanatory variables to account for different slopes for each age group. Finally, we added year as a random effect to account for pseudo-replication. Models were selected based on Akaike Information Criterion (AIC) multi-model approach. First, all possible models within 95% cumulative AIC weight were averaged (Symonds and Moussalli, 2011), than variables were scored based on the sum of Akaike weights (sw) to identify the most important variables. Variable is considered relatively more important as its sw approaching 1. We report both standardized and non-standardized coefficients for ease of interpretation of the relative effect of the explanatory variables.
All statistical analyses were conducted using R version 3.6.0 (R Development Core Team). R-function lme in R package nlme (Pinheiro et al., 2020) and R functions lm and lmer from the R package lme4 were used for the linear and mixed effect models, R-function emtrend in the R package emmeans (Lenth, 2020) was used to perform the post hoc test and estimate the marginal means of the linear trend. Variables in the arrival trend models were excluded based on a threshold significance level set to P = 0.05. Models analysing the relationship between arrival timing and environmental conditions were ranked by their AICc using the R function dredge and the importance of each variable in the average model were determined using the importance function. Both functions are in the R package MuMIn (Barton, 2020).
We found that two of our study species advanced arrival to stopover site in Israel throughout the study period. Lesser whitethroat advanced arrival by 0.30–0.46 days per year in all four population measurements (Figure 2 and Table 1). The interaction between year and age was significant in the model, and a post hoc test revealed that the advancement in arrival of the 95th percentile occurred in adults, but not in the second year group (Table 1). Eastern Bonelli’s warbler advanced arrival by 0.70–0.85 days a year in all population measurements, except the 95th percentile (Table 1). Thrush nightingale and Olive-tree warbler did not change their arrival time.
Figure 2. Spring migration phenology (mean first arrival day) of the four study species migrating from wintering grounds in Africa to stopover sites in Israel across 22 years (2000–2021). Lines were fitted using GLMM and shown only where slope was significant (P < 0.05) with confidence intervals shaded in grey. In Lesser whitethroat and Thrush nightingale data depict adults (triangles and a dashed line) and second year individuals (solid circles and a solid line).
Table 1. Summary of the changes in arrival timing (days per year) of the first 5th percentile, median, 95th percentile and mean arrival of the four studied species to stopover site in Israel, estimated by generalized linear mixed effect models, and the difference between age groups (Adults and second year; SY) where age could be identified.
The average wing length of Lesser whitethroat increased slightly throughout the study period in both adults and second year birds (β = 0.03, P = 0.01, Table 2). A significant interaction between year of capture and age implies a different slope for second year and adult birds (Interaction term year × age: t = −1.95, P = 0.05). A post hoc test revealed that the slopes were significantly different (0.07, SE < 0.01, df = 60, t = 1.95, P = 0.05). We did not detect any long-term trend in average wing length in the other species.
Table 2. Results of linear mixed-effect model testing the effect of year, arrival phase (early or late; “Group”) and Age (Second year or adults; where known), with Year also as a random effect, on the average wing length of the studied species during spring migration in Jerusalem, Israel during the study period (2000–2021).
In both Lesser whitethroat and Thrush nightingale, the only two species where age could be identified in the field, adults had longer wings than second year birds, and in all species early arrivals had longer wings than late arrivals (Figure 3 and Table 2). This difference was strongest in Eastern Bonelli’s warbler, where the average wing length in the first 30th percentile was 68.2 mm in compared to an average wing length of 65.0 in the 70th percentile. Note that sex was not identified in our species and these differences may be a result of inter-sexual differences (will be discussed). The standard deviation of the random effect (year) was small and close to zero in the case of Olive-tree warbler, indicating that the variability between individuals is small (Table 2).
Figure 3. Wing length differences between arrival phases in the four migratory passerines species caught at a stopover site in Israel during spring migration (2000–2021). Box-and-whisker describing the mean yearly wing length for each arrival group on the x-axis, and black stripes depict the median. Early and late arrivals were calculated as the first and last 30% of individuals on their first capture. For Lesser whitethroat and Thrush nightingale, data is separated into adults (white boxes) and second year individuals (dark grey boxes). Due to limited sample size (Supplementary Table 1), data for Eastern Bonelli’s warbler were not divided into arrival phases, but the whole data set was used to analyse trends in wing length against arrival day. For the purpose of representation, boxes in this plot show mean wing length of the first and last 30% (“Early” and “Late”), as well as of the core arrivals (“Mid”). Statistical models are detailed in Table 2.
The average model of all possible models within 95% cumulative AIC weight for Lesser whitethroat (39 models, Supplementary Table 2) included EVI in March in the Horn of Africa and temperatures in Jerusalem in both March and April. The importance score suggested that temperatures in March has relatively strong effect on the mean arrival day of Lesser whitethroat to the stopover site (sw = 0.94; Table 3 and Figure 4).
Table 3. Coefficients of the average model of all possible models within 95% cumulative AIC weight, testing the effect of environmental variables on the mean arrival day of migratory species, and the sum of Akaike weights (sw) describing the importance of each variable (N containing models in brackets).
Figure 4. The relationship between mean arrival day and average Enhanced Vegetation Index (EVI) on the Horn of Africa and temperatures in March at the stopover site in Jerusalem. Predicted lines were drawn from a linear model and only variables considered important by the multi-model approach are shown. Black points represent raw data and the 95% confidence interval is shown in grey. The lines in each plot are derived from different species-specific linear models outlined in the methods.
Both EVI in March in the Horn of Africa and March temperatures in Jerusalem were included in the average model of Thrush nightingale (based on 47 models, Supplementary Table 3). Model results and importance score suggested a stronger effect of greenness at the pre-departure site on the mean arrival day (sw = 0.81) than temperatures in March (Table 3 and Figure 4).
The average model for Olive-tree warbler (based on 49 models, Supplementary Table 4) included only EVI in the Horn of Africa in March. The importance score suggested a relatively strong effect of EVI on the mean arrival day (sw = 0.82; Table 3 and Figure 4).
The average model for Eastern Bonelli’s warbler (based on 51 models; Supplementary Table 5) was the null model (no predictors) and the sw of each of the variables were <0.39.
In thrush nightingale and Lesser whitethroat, the only two species where age could be identified, age was not significant in the models, suggesting a similar effect of EVI and temperatures on both second year and adult birds. We therefore present the models where age is not included.
In this study, using a combination of long-term biological observations and remotely-sensed environmental monitoring, we show intra-specific differences in spring migration phenology and in the response to environmental cues. While Lesser whitethroat and Eastern Bonelli’s warbler advanced arrival to the stopover site, the arrival of Thrush nightingale and Olive-tree warbler did not change throughout the study period and was associated with mean EVI on the pre-departure site in the Horn of Africa during the period before departure. Importantly, we show that for Lesser whitethroat, a species with northern breeding areas, temperatures at the stopover site were correlated to arrival timing, suggesting fine-tuning of stopover arrival to match conditions en-route.
Clearly, there are many additional variables at multiple locations along the migration routes that potentially affect migration decisions (e.g., Saino et al., 2004; Arizaga et al., 2011; Sjöberg et al., 2015). Hence, a more comprehensive suite of variables may be more appropriate to make conclusions on the triggers to depart on migration. However, while our study suggest that conditions in the Horn of Africa potentially affect the migration decision of some species, a lack of accurate information on the departure site and routes taken, currently limit this kind of analysis with our data. Before we can apply a more elaborate testing of multiple variables, we need fundamental studies on the wintering locations and on migration routes. This could be done via stable isotope analysis to reveal the likely departure sites (e.g., Raz et al., Submitted)4 and the use of tracking devices (e.g., Tøttrup et al., 2012). Still, our study highlights the potential of such studies in measuring more accurately the variables that play a role in the decision making by migratory species before departure.
We found that individuals arriving later had significantly shorter wings than early arrivals in all study species. This trend has multiple potential explanations: (1) sexual dimorphism (2) age differences, or (3) separate populations. Sexual dimorphism has been suggested in Lesser whitethroat (Loskot, 2005) and Thrush nightingale (Csörgő et al., 2018), hence, the early and late arrival phases may represent males and females, depicting different migration phenology. However, Kováts (2012) argues that the difference found in wing length in immature Thrush nightingale were not attributed to sex-biased migration timing. Adults may also have longer wings (as we show in Lesser whitethroat and Thrush nightingale). While our results show that the trend of decreasing wing length with season persists in both adults and second year birds, we do not have a record of age in Olive-tree warbler and Eastern Bonelli, and therefore cannot rule out that the differences in wing length may be attributed to age-biased migration phenology. Finally, the differences in wing length between arrival phases may be attributed to different populations. The longer wings suggest that the early arrivals may head to breeding sites farther from the wintering grounds than the later arrivals, based on association between wing length and migration distance (Fiedler, 2005; Nowakowski et al., 2014; Toews et al., 2017). Indeed, ring recoveries show that early arriving individuals of Lesser whitethroat are heading towards breeding sites in West Europe, while individuals that breed in central and Eastern Europe, which are geographically closer to Israel, arrive later. This explanation may also hold for Eastern Bonelli’s warbler, because the same trend has been recorded at a stopover site in Greece (Barboutis et al., 2013). Moreover, multiple populations of Eastern Bonelli’s warbler may also explain the advanced arrival trend shown only in the early and median arrival phases, but not in the late arrival phase, as they may be responding and adapting to different conditions at separated sites.
The average wing length of Lesser whitethroat increased throughout the study period, and possibly suggest that changes had taken place in the population structure and/or in migration behaviour. Such a change in the morphology of a species may result from selection pressure for individuals with longer wings, because long wings are more energetically efficient for long flights and may facilitate the adjustment to changing conditions along the migration route (Yom-Tov et al., 2006; Hahn et al., 2016). In addition, increased wing length could potentially result from increased breeding success in the western breeding ranges (the early arrival population) as a result of improved conditions from warming climate, as suggested for Garden warbler (Sylvia borin; Kovács et al., 2011). Nonetheless, the differences in wing length between the arrival groups and with years, for Lesser whitethroat, were very small (< 2mm) in three out of our four species, and could be attributed to measurements error. Interpretation of these differences should be therefore done with caution.
Our results show that the arrival of Lesser whitethroat to stopover site in Israel advanced in the last 20 years and was correlated to temperatures at the stopover site. EVI at a wintering site in Horn of Africa had only marginal effect on arrival timing, maybe due to the area from which we calculated EVI not accurately representing the population of Lesser whitethroat passing through Israel, or that changes occurred in the population, as also suggested by the increased wing length (discussed above). These observations suggest that further studies need to investigate the main African wintering and pre-departure site of Lesser whitethroat to gain a holistic understanding of the forces acting on the migration decisions of this species.
In support of our results, Lesser whitethroat has also been advancing its arrival timing in the last 60 years to breeding and stopover sites in northern Europe (Hüppop and Hüppop, 2003; Jonzén et al., 2006; Miles et al., 2017). Importantly, its arrival timing has also been correlated to conditions (precipitation) at the Horn of Africa wintering ground (Aloni et al., 2017) and to local temperatures in Europe and to North Atlantic Oscillation (NAO) index (Hüppop and Hüppop, 2003; Jonzén et al., 2006; but see statistical limitations in the use of NAO; Haest et al., 2018). These correlations suggest not only that conditions at the pre-migratory site affect migration timing, but also that Lesser whitethroat are able to adjust migration timing en route depending on local conditions, as previously found in other species (e.g., Tøttrup et al., 2010; Haest et al., 2020).
In contrast to our results, the arrival timing of Lesser whitethroat to breeding ground in Poland did not change between 1983 and 2003. However, the authors suggested that the lack of trend was attributed to changes to the population size (Tryjanowski et al., 2005). In addition, the median arrival of Lesser whitethroat to Eilat (Israel), a stopover site located about 250 km south of our study site in Jerusalem, has been delayed between 1984 and 2004, as a response to high NDVI values at the wintering ground (Tøttrup et al., 2008). The discrepancies may be a result of both the time period analysed and the area and period from which the index was calculated. Tøttrup et al. (2008) averaged NDVI over both wintering areas of Lesser whitethroat (Horn of Africa and Chad and Sudan), over the period December to February, while we separated the two areas due to their different climate and only accounted for the months before departure (February–March) due to annual variability of EVI in Africa (Adole et al., 2018).
Our results suggest no trend in arrival phenology of Thrush nightingale to the stopover site in Israel, but dependence on conditions at the African pre-departure site. As EVI is a proxy for food availability, it is likely that high and low EVI determine fuelling rates and either allow advanced departure and arrival to the stopover site (high EVI), or limit the birds ability to gain enough fat before departure, particularly in north Africa, before crossing ecological barrier such as the Sahara desert. For example, low and high EVI in the Horn of Africa in 2000 and 2020 (Supplementary Figures 1, 2) are likely the cause behind the relatively late and early arrival of Thrush nightingale, respectively (Figure 2).
In contrast to Lesser whitethroat, which is also a northern breeding species, the arrival of Thrush nightingale was not correlated to local temperatures at the stopover site in Israel. This may be explained by the relatively late arrival of Thrush nightingale to the stopover site, probably because individuals arrive from further wintering locations in Southern Africa. Therefore, they may be time-constrained in this migration leg and their departure decision is dominated by feeding conditions at the pre-departure site in Africa.
Previous studies found that Thrush nightingale advanced its arrival to the Island of Christianso in the Baltic Sea during 1976–1997 (Tøttrup et al., 2006), to Sweden, in correlation with high NAO index from 1980 to 2004 (Jonzén et al., 2006) and to its breeding ground in Poland between 1983 and 2003 (Tryjanowski et al., 2005). While these studies took place in earlier time periods, they still suggest that while Thrush nightingale did not advance arrival to Israel on northward migration, it may still show flexibility and adjust migration speed en route as a response to local conditions, and ultimately advanced arrival to Europe.
To our knowledge, our study is the first to analyse trends in migration phenology of the Olive-tree warbler. We show that, similar to Thrush nightingale, the arrival of Olive-tree warbler to Israel did not change, and was correlated with EVI at the pre-departure site in the Horn of Africa. The migration route and phenology of Olive-tree warbler, at least at the African part of the migration route, is similar to that of Thrush nightingale. Indeed, the year-to-year fluctuation in arrival timing of Olive-tree warbler was also correlated to that of Thrush nightingale (Supplementary Figure 3). This is not surprising as both species winter in Southern Africa, stop at the Horn of Africa before crossing the Sahara desert on north migration, and are relatively late arrivals to the Israeli stopover site. The correlation between the arrival timing of these species suggest that they experience similar conditions en route and use a similar spring migration strategy.
The arrival of Olive-tree warbler was independent of local temperatures at the stopover site. Similar to Thrush nightingale, Olive-tree warbler winter in Southern Africa, and individuals have a longer distance to cover during the annual migration. It is therefore likely that the departure decisions of Olive-tree warbler are less flexible and predominantly based on suitable departure conditions at the pre-departure site. A second explanation may be the proximity of the breeding grounds of Olive-tree warbler in southeast Europe and northeast Mediterranean, to the Israeli stopover site. This proximity means that the remaining journey from Israel to the breeding ground is short, and probably does not require long and intensive preparation. It may therefore allow for some flexibility in the length of stay at the stopover site in Israel, and adjustment of arrival at the breeding grounds when optimal breeding conditions occur. Therefore, they are likely less time-restricted, and do not have to fine-tune their migration timing to match multiple other stops.
We are also not aware of previous studies on changes to the migration phenology of Eastern Bonelli’s warbler. Our results show that, similarly to Lesser whitethroat, Eastern Bonelli’s warbler advanced arrival to the Israeli stopover site in the last 20 years. Indeed, the arrival timing of Eastern Bonelli’s warbler was correlated with year-to-year fluctuation in arrival timing of Lesser whitethroat (Supplementary Figure 3), supporting similar conditions en route and similar spring migration strategy. However, the arrival of Eastern Bonelli’s warbler was independent of spatial and temporal availability of resources during the pre-migratory period (measured as EVI) at its wintering site. While the small sample size of Eastern Bonelli’s warbler (average number of individuals per year: 24.8 ± 16.3) require interpreting the results with caution, we are confident that the presumed wintering area from which we calculated EVI represent the population flying through Israel. This is because previous studies show that the autumn temperature at the same wintering ground affected the body condition of Eastern Bonelli’s warbler during spring migration (Aloni et al., 2019). Instead, we suggest that the migration decisions of Eastern Bonelli’s warbler could be primarily dominated by endogenous cues and photoperiod (Ramenofsky, 2012; Stanley et al., 2012; Gersten and Hahn, 2016), which stay fixed as the earth’s climate changes, or environmental variables other than vegetation growth (Haest et al., 2020). Thus, the advanced arrival of Eastern Bonelli’s warbler to the stopover site, potentially corresponding to advanced conditions at the breeding ground, could be a result of increasing selection for individuals which migrate earlier (Both and Visser, 2001; Jonzén et al., 2006), or a generational shift towards early arrivals as a result of advances in arrival dates of new recruits (Gill et al., 2014).
Moreover, the advanced arrival of Eastern Bonelli’s warbler was recorded only for the early, mean and median phases of migration, and not for the late phase. This could be a result of phenological changes which vary between populations depending on the cues birds are responding to along their migration route and breeding/non-breeding conditions. It has also been suggested that birds in the earliest migration phases in several trans-Saharan species are experiencing greater change in spring phenology and therefore they are responding strongly to cues along the migration route, compared to the later migration phases which may rely on endogenous control (Tøttrup et al., 2010). In our case, the differences in migration phases may also be related to sex-biased migration timing, or to young, inexperienced individuals relying on endogenous cues, while adults are more flexible to respond to external cues.
Similar to Olive-tree warbler, the arrival of Eastern Bonelli’s warbler was independent of local temperatures at the stopover site in Israel. This is again likely due to the proximity of their breeding grounds to the Israeli stopover site, which allow them to use the Israeli stopover site until optimal breeding conditions occur (discussed above).
Generally, the decision when to depart on migration is strongly dependent on endogenous rhythms and photoperiods (Gwinner, 1996; Bradshaw and Holzapfel, 2008). However, spring departure can also be influenced by exogenous cues such as weather condition and food availability, and the level of response is species-specific. Here, we suggest that some species are able to show flexibility and fine-tuned migration speed in response to local conditions en route (Hüppop and Hüppop, 2003; Tøttrup et al., 2008, 2010). This flexibility may be a result of phenotypic plasticity, but we lack individual data to support this argument. Thus, individuals are likely using constant decision making processes based on local weather and body condition throughout the migration route in order to match optimal breeding conditions.
Still, selection and micro-evolutionary processes probably play a role and may shift departure times earlier (Pulido et al., 2001; Jonzén et al., 2006; Gienapp et al., 2007), and possibly explain the advanced arrival of Eastern Bonelli’s warbler in our study. While advanced arrival is advantageous in the current warming climate, selection for early individuals may suggests less adaptability to year-to-year variation in local and en route conditions (e.g., Hüppop and Hüppop, 2003). Moreover, while many migratory birds may shift to earlier departure and reduced time in Africa, this shift may be possible only if conditions, such as food availability for high fuelling rates, permit, and within the framework in which individuals are able to respond. For example, a drought event in 2011 in the Horn of Africa, an area which potentially affect migration decision in three out of our four studied species, caused substantial delay of several species, including Thrush nightingale, to the breeding and stopover sites (Tøttrup et al., 2012; This study). Limiting conditions at the pre-departure and stopover sites of long distance migratory species may have consequences for reproduction success and survival as a result of mismatched arrival and “thermal delay” (Saino et al., 2011), emphasizing the challenges to long-distance migratory species in the race to match global changes to ensure their survival.
The raw data supporting the conclusions of this article will be made available by the authors, without undue reservation.
Ethical review and approval was not required for the animal study because the study is based on long term data from a ringing station. All work at the ringing station is approved by the relevant authorities.
YA-R and GP formulated the idea. YK and GP performed the fieldwork. JM and TR extracted and calculated all EVI and mapping data. YA-R and JM performed the data analysis. YA-R wrote the manuscript with input from all other authors. All authors contributed to the article and approved the submitted version.
YA-R was funded by the University of New England-PDF program.
The authors declare that the research was conducted in the absence of any commercial or financial relationships that could be construed as a potential conflict of interest.
All claims expressed in this article are solely those of the authors and do not necessarily represent those of their affiliated organizations, or those of the publisher, the editors and the reviewers. Any product that may be evaluated in this article, or claim that may be made by its manufacturer, is not guaranteed or endorsed by the publisher.
We thank all the staff and bird-ringers at the JBO, in particular Yotam Lehnardt and Alena Kacal.
The Supplementary Material for this article can be found online at: https://www.frontiersin.org/articles/10.3389/fevo.2022.834074/full#supplementary-material
Supplementary Figure 1 | Example of Enhanced Vegetation Index (EVI) in 2000 (low values) and 2020 (high values) in the Horn of Africa. Data sources: NASA’s MODIS Terra Vegetation Indices product (Didan, 2015), Google, Digital Globe.
Supplementary Figure 2 | Mean Enhanced Vegetation Index (EVI) in March in the Horn of Africa (Fig. S1) from 2000 to 2020. The trend line is not significant (p > 0.05).
Supplementary Figure 3 | Correlation between the year-to-year variations in arrival timing of species. The plot show the results of Pearson correlation between the first arrival day of the different species at the stopover site in Israel. Red asterisk show significant correlation: *<0.05, **<0.01, ***<0.001.
Supplementary Tables 2–5 | Model selection process for the linear models analysing the relationship between arrival dates to the stopover site in Israel and environmental conditions on the wintering ground and the stopover site in the four study species. The tables include all possible models within 95% cumulative AIC weight, from which an average model was calculated to identify the most important variables. The average model outputs are detailed in Table 3. Study species include: Lesser whitethroat (Supplementary Table 2), Thrush nightingale (Supplementary Table 3), Olive-tree warbler (Supplementary Table 4) and Eastern Bonelli’s warbler (Supplementary Table 5).
Adole, T., Dash, J., and Atkinson, P. M. (2018). Characterising the land surface phenology of Africa using 500 m MODIS EVI. Appl. Geogr. 90, 187–199. doi: 10.1016/j.apgeog.2017.12.006
Aharon-Rotman, Y., Bauer, S., and Klaassen, M. (2016). A chain is as strong as its weakest link: assessing the consequences of habitat loss and degradation in a long-distance migratory shorebird. Emu 116, 199–207. doi: 10.1071/mu15029
Aharon-Rotman, Y., Perlman, G., Kiat, Y., Raz, T., Balaban, A., and Iwamura, T. (2021). Limited flexibility in departure timing of migratory passerines at the East-Mediterranean flyway. Sci. Rep. 11:5184. doi: 10.1038/s41598-021-83793-x
Ahola, M., Laaksonen, T., Sippola, K., Eeva, T., Rainio, K., and Lehikoinen, E. (2004). Variation in climate warming along the migration route uncouples arrival and breeding dates. Glob. Change Biol. 10, 1610–1617. doi: 10.1111/j.1365-2486.2004.00823.x
Åkesson, S., Ilieva, M., and Bianco, G. (2021). Flexibility and control of circadian activity, migratory restlessness and fueling in two songbird migrants. Front. Ecol. Evol. 9:666176. doi: 10.3389/fevo.2021.666176
Aloni, I., Markman, S., and Ziv, Y. (2017). February precipitation in the wintering grounds of the lesser whitethroat, Sylvia curruca: is it a cue for migration onset? R. Soc. Open Sci. 4:160755. doi: 10.1098/rsos.160755
Aloni, I., Markman, S., and Ziv, Y. (2019). Autumn temperatures at African wintering grounds affect body condition of two passerine species during spring migration. PLoS One 14:e0217619. doi: 10.1371/journal.pone.0217619
Altwegg, R., Broms, K., Erni, B., Barnard, P., Midgley, G. F., and Underhill, L. G. (2012). Novel methods reveal shifts in migration phenology of barn swallows in South Africa. Proc. R. Soc. B Biol. Sci. 279, 1485–1490.
Arizaga, J., Belda, E. J., and Barba, E. (2011). Effect of fuel load, date, rain and wind on departure decisions of a migratory passerine. J. Ornithol. 152, 991–999.
Bairlein, F. (2003). The study of bird migrations–some future perspectives. Bird Study 50, 243–253. doi: 10.1080/00063650309461317
Barboutis, C., Evangelidis, A., Akriotis, T., and Fransson, T. (2013). Spring migration phenology and arrival conditions of the Eastern Bonelli’s Warbler and the Semi-collared Flycatcher at a small Greek island. Ring. Migr. 28, 39–42. doi: 10.1080/03078698.2013.811118
Barton, K. (2020). MuMIn: Multi-Model Inference. R Package Version 1.43.17. Available Online at: https://CRAN.R-project.org/package=MuMIn
Bell, F., Bearhop, S., Briedis, M., El Harouchi, M., Bell, S. C., Castelo, J., et al. (2021). Geolocators reveal variation and sex-specific differences in the migratory strategies of a long-distance migrant. Ibis 164, 451–467.
BirdLife International (2019). BirdLife International and Handbook of the Birds of the World. Bird Species Distribution Maps of the World. Cambridge: Birdlife International.
Both, C., and Visser, M. E. (2001). Adjustment to climate change is constrained by arrival date in a long-distance migrant bird. Nature 411, 296–298. doi: 10.1038/35077063
Bradshaw, W., and Holzapfel, C. (2008). Genetic response to rapid climate change: it’s seasonal timing that matters. Mol. Ecol. 17, 157–166. doi: 10.1111/j.1365-294X.2007.03509.x
Burgess, M. D., Smith, K. W., Evans, K. L., Leech, D., Pearce-Higgins, J. W., Branston, C. J., et al. (2018). Tritrophic phenological match–mismatch in space and time. Nat. Ecol. Evol. 2, 970–975. doi: 10.1038/s41559-018-0543-1
Burrows, M. T., Schoeman, D. S., Buckley, L. B., Moore, P., Poloczanska, E. S., Brander, K. M., et al. (2011). The pace of shifting climate in marine and terrestrial ecosystems. Science 334, 652–655. doi: 10.1126/science.1210288
Bussière, E. M., Underhill, L. G., and Altwegg, R. (2015). Patterns of bird migration phenology in South Africa suggest northern hemisphere climate as the most consistent driver of change. Glob. Change Biol. 21, 2179–2190.
Csörgő, T., Fehérvári, P., Karcza, Z., Ócsai, P., and Harnos, A. (2018). Exploratory analyses of migration timing and morphometrics of the thrush nightingale. Ornis Hung. 26, 149–170. doi: 10.1515/orhu-2018-0010
Didan, K. (2015). ”MOD13A2 MODIS/Terra Vegetation Indices 16-Day L3 Global 1km SIN Grid V006 [Data set]”, (ed.) N.E.L.P. Daac. (Accessed 2021-11-23 from https://doi.org/10.5067/MODIS/MOD13A2.006).*
Fiedler, W. (2005). Ecomorphology of the external flight apparatus of blackcaps (Sylvia atricapilla) with different migration behavior. Ann. N. Y. Acad. Sci. 1046, 253–263. doi: 10.1196/annals.1343.022
Flato, G., and Boer, G. (2001). Warming asymmetry in climate change simulations. Geophys. Res. Lett. 28, 195–198. doi: 10.1029/2000gl012121
Förschler, M. I., and Bairlein, F. (2011). Morphological shifts of the external flight apparatus across the range of a passerine (Northern Wheatear) with diverging migratory behaviour. PLoS One 6:e18732. doi: 10.1371/journal.pone.0018732
Gersten, A., and Hahn, S. (2016). Timing of migration in common redstarts (Phoenicurus phoenicurus) in relation to the vegetation phenology at residence sites. J. Ornithol. 157, 1029–1036. doi: 10.1007/s10336-016-1359-x
Gienapp, P., Leimu, R., and Merilä, J. (2007). Responses to climate change in avian migration time—microevolution versus phenotypic plasticity. Clim. Res. 35, 25–35. doi: 10.3354/cr00712
Gill, J. A., Alves, J. A., Sutherland, W. J., Appleton, G. F., Potts, P. M., and Gunnarsson, T. G. (2014). Why is timing of bird migration advancing when individuals are not? Proc. R. Soc. B Biol. Sci. 281:20132161. doi: 10.1098/rspb.2013.2161
Gwinner, E. (1996). Circannual clocks in avian reproduction and migration. Ibis 138, 47–63. doi: 10.1111/j.1474-919x.1996.tb04312.x
Gwinner, E., and Wiltschko, W. (1978). Endogenously controlled changes in migratory direction of the Garden Warbler, Sylvia borin. J. Comp. Physiol. 125, 267–273. doi: 10.1007/bf00656605
Haest, B., Hüppop, O., and Bairlein, F. (2018). Challenging a 15-year-old claim: the North Atlantic Oscillation index as a predictor of spring migration phenology of birds. Glob. Change Biol. 24, 1523–1537. doi: 10.1111/gcb.14023
Haest, B., Hüppop, O., and Bairlein, F. (2020). Weather at the winter and stopover areas determines spring migration onset, progress, and advancements in Afro-Palearctic migrant birds. Proc. Natl. Acad. Sci. U.S.A. 117, 17056–17062. doi: 10.1073/pnas.1920448117
Hahn, S., Korner Nievergelt, F., Emmenegger, T., Amrhein, V., Csörgő, T., Gursoy, A., et al. (2016). Longer wings for faster springs: wing length relates to spring phenology in a long distance migrant across its range. Ecol. Evol. 6, 68–77. doi: 10.1002/ece3.1862
Horton, K. G., La Sorte, F. A., Sheldon, D., Lin, T.-Y., Winner, K., Bernstein, G., et al. (2020). Phenology of nocturnal avian migration has shifted at the continental scale. Nat. Clim. Change 10, 63–68.
Houborg, R., Soegaard, H., and Boegh, E. (2007). Combining vegetation index and model inversion methods for the extraction of key vegetation biophysical parameters using Terra and Aqua MODIS reflectance data. Remote Sens. Environ. 106, 39–58. doi: 10.1016/j.rse.2006.07.016
Huete, A., Didan, K., Miura, T., Rodriguez, E. P., Gao, X., and Ferreira, L. G. (2002). Overview of the radiometric and biophysical performance of the MODIS vegetation indices. Remote Sens. Environ. 83, 195–213. doi: 10.1016/s0034-4257(02)00096-2
Hüppop, O., and Hüppop, K. (2003). North Atlantic Oscillation and timing of spring migration in birds. Proc. R. Soc. Lond. B Biol. Sci. 270, 233–240. doi: 10.1098/rspb.2002.2236
Izhaki, I., and Maitav, A. (1998). Blackcaps Sylvia atricapilla stopping over at the desert edge; inter-and intra sexual differences in spring and autumn migration. Ibis 140, 234–243. doi: 10.1111/j.1474-919x.1998.tb04384.x
Jones, T., and Cresswell, W. (2010). The phenology mismatch hypothesis: are declines of migrant birds linked to uneven global climate change? J. Anim. Ecol. 79, 98–108. doi: 10.1111/j.1365-2656.2009.01610.x
Jonzén, N., Lindén, A., Ergon, T., Knudsen, E., Vik, J. O., Rubolini, D., et al. (2006). Rapid advance of spring arrival dates in long-distance migratory birds. Science 312, 1959–1961. doi: 10.1126/science.1126119
Kiat, Y., and Sapir, N. (2018). Life-history trade-offs result in evolutionary optimization of feather quality. Biol. J. Linn. Soc. 125, 613–624.
Kokko, H. (1999). Competition for early arrival in migratory birds. J. Anim. Ecol. 68, 940–950. doi: 10.1046/j.1365-2656.1999.00343.x
Kovács, S., Csörgő, T., Harnos, A., Fehérvári, P., and Nagy, K. (2011). Change in migration phenology and biometrics of two conspecific Sylvia species in Hungary. J. Ornithol. 152, 365–373. doi: 10.1007/s10336-010-0596-7
Kováts, D. (2012). Autumn migration of the thrush nightingale (Luscinia luscinia) in northern Hungary. Ring 34, 23–36. doi: 10.2478/v10050-012-0001-4
Kralj, J., Barišić, S., Ćiković, D., and Tutiš, V. (2012). Range expansion of the olive-tree warbler Hippolais olivetorum along the Croatian coast. Acrocephalus 33, 105–107.
Lawrence, K. B., Barlow, C. R., Bensusan, K., Perez, C., and Willis, S. G. (2021). Phenological trends in the pre-and post-breeding migration of long-distance migratory birds. Glob. Change Biol. 28, 375–389. doi: 10.1111/gcb.15916
Lenth, R. V. (2020). emmeans: Estimated Marginal Means, aka Least-Squares Means. R package version 1.5.2-1. https://CRAN.R-project.org/package=emmeans
Loskot, V. (2005). Morphological variation and taxonomic revision of five south-eastern subspecies of Lesser Whitethroat Sylvia curruca (L.)(Aves: Sylviidae). Zool. Meded. 79, 157–165.
Marra, P. P., Francis, C. M., Mulvihill, R. S., and Moore, F. R. (2005). The influence of climate on the timing and rate of spring bird migration. Oecologia 142, 307–315. doi: 10.1007/s00442-004-1725-x
Marra, P. P., Hobson, K. A., and Holmes, R. T. (1998). Linking winter and summer events in a migratory bird by using stable-carbon isotopes. Science 282, 1884–1886. doi: 10.1126/science.282.5395.1884
Mayor, S. J., Guralnick, R. P., Tingley, M. W., Otegui, J., Withey, J. C., Elmendorf, S. C., et al. (2017). Increasing phenological asynchrony between spring green-up and arrival of migratory birds. Sci. Rep. 7:1902. doi: 10.1038/s41598-017-02045-z
Milá, B., Wayne, R. K., and Smith, T. B. (2008). Ecomorphology of migratory and sedentary populations of the Yellow-rumped Warbler (Dendroica coronata). Condor 110, 335–344. doi: 10.1525/cond.2008.8396
Miles, W. T., Bolton, M., Davis, P., Dennis, R., Broad, R., Robertson, I., et al. (2017). Quantifying full phenological event distributions reveals simultaneous advances, temporal stability and delays in spring and autumn migration timing in long distance migratory birds. Glob. Change Biol. 23, 1400–1414. doi: 10.1111/gcb.13486
Møller, A. P., Rubolini, D., and Lehikoinen, E. (2008). Populations of migratory bird species that did not show a phenological response to climate change are declining. Proc. Natl. Acad. Sci. U.S.A. 105, 16195–16200. doi: 10.1073/pnas.0803825105
Newton, I. (2004). Population limitation in migrants. Ibis 146, 197–226. doi: 10.1111/j.1474-919x.2004.00293.x
Nowakowski, J. K., Szulc, J., and Remisiewicz, M. (2014). The further the flight, the longer the wing: relationship between wing length and migratory distance in Old World reed and bush warblers (Acrocephalidae and Locustellidae). Ornis Fenn. 91, 178–186.
Ockendon, N., Johnston, A., and Baillie, S. R. (2014). Rainfall on wintering grounds affects population change in many species of Afro-Palaearctic migrants. J. Ornithol. 155, 905–917. doi: 10.1007/s10336-014-1073-5
Papeş, M., Peterson, A. T., and Powell, G. V. (2012). Vegetation dynamics and avian seasonal migration: clues from remotely sensed vegetation indices and ecological niche modelling. J. Biogeogr. 39, 652–664. doi: 10.1111/j.1365-2699.2011.02632.x
Payevsky, V. A., Vysotsky, V. G., and Zelenova, N. P. (2003). Extinction of a Barred Warbler Sylvia nisoria population in Eastern Baltic: long-term monitoring, demography, and biometry. Avian Ecol. Behav. 11, 89–105.
Pinheiro, J., Bates, D., Debroy, S., and Sarkar, D., and R Core Team (2020). nlme: Linear and Nonlinear Mixed Effects Models. R Package Version 3.1-139. Available Online at: https://CRAN.R-project.org/package=nlme
Pulido, F., Berthold, P., Mohr, G., and Querner, U. (2001). Heritability of the timing of autumn migration in a natural bird population. Proc. R. Soc. Lond. B Biol. Sci. 268, 953–959. doi: 10.1098/rspb.2001.1602
QGIS Development Team (2021). Open Source Geospatial Foundation Project. QGIS Geographic Information System. Available online at: http://qgis.osgeo.org
Ramenofsky, M. (2012). Reconsidering the role of photoperiod in relation to effects of precipitation and food availability on spring departure of a migratory bird. Proc. R. Soc. B Biol. Sci. 279, 15–16. doi: 10.1098/rspb.2011.1591
Rubolini, D., Møller, A. P., Rainio, K., and Lehikoinen, E. (2007). Intraspecific consistency and geographic variability in temporal trends of spring migration phenology among European bird species. Clim. Res. 35, 135–146. doi: 10.3354/cr00720
Saino, N., Ambrosini, R., Rubolini, D., Von Hardenberg, J., Provenzale, A., Hüppop, K., et al. (2011). Climate warming, ecological mismatch at arrival and population decline in migratory birds. Proc. R. Soc. B Biol. Sci. 278, 835–842. doi: 10.1098/rspb.2010.1778
Saino, N., Szép, T., Romano, M., Rubolini, D., Spina, F., and Møller, A. P. (2004). Ecological conditions during winter predict arrival date at the breeding quarters in a trans-Saharan migratory bird. Ecol. Lett. 7, 21–25. doi: 10.1046/j.1461-0248.2003.00553.x
Shamoun-Baranes, J., Van Loon, E., Alon, D., Alpert, P., Yom-Tov, Y., and Leshem, Y. (2006). Is there a connection between weather at departure sites, onset of migration and timing of soaring-bird autumn migration in Israel? Glob. Ecol. Biogeogr. 15, 541–552. doi: 10.1111/j.1466-8238.2006.00261.x
Sjöberg, S., Alerstam, T., Åkesson, S., Schulz, A., Weidauer, A., Coppack, T., et al. (2015). Weather and fuel reserves determine departure and flight decisions in passerines migrating across the Baltic Sea. Anim. Behav. 104, 59–68. doi: 10.1016/j.anbehav.2015.02.015
Smith, R. J., and Moore, F. R. (2005). Arrival timing and seasonal reproductive performance in a long-distance migratory landbird. Behav. Ecol. Sociobiol. 57, 231–239. doi: 10.1007/s00265-004-0855-9
Sparks, T., Bairlein, F., Bojarinova, J., Hüppop, O., Lehikoinen, E., Rainio, K., et al. (2005). Examining the total arrival distribution of migratory birds. Glob. Change Biol. 11, 22–30. doi: 10.1111/j.1365-2486.2004.00887.x
Spina, F., Massi, A., and Montemaggiori, A. (1994). Back from Africa: who’s running ahead? Aspects of differential migration of sex and age classes in Palearctic-African spring migrants. Ostrich 65, 137–150. doi: 10.1080/00306525.1994.9639676
Stanley, C. Q., Macpherson, M., Fraser, K. C., Mckinnon, E. A., and Stutchbury, B. J. (2012). Repeat tracking of individual songbirds reveals consistent migration timing but flexibility in route. PLoS One 7:e40688. doi: 10.1371/journal.pone.0040688
Symonds, M. R., and Moussalli, A. (2011). A brief guide to model selection, multimodel inference and model averaging in behavioural ecology using Akaike’s information criterion. Behav. Ecol. Sociobiol. 65, 13–21. doi: 10.1007/s00265-010-1037-6
Thorup, K., Tøttrup, A. P., and Rahbek, C. (2007). Patterns of phenological changes in migratory birds. Oecologia 151, 697–703. doi: 10.1007/s00442-006-0608-8
Toews, D. P., Heavyside, J., and Irwin, D. E. (2017). Linking the wintering and breeding grounds of warblers along the Pacific Flyway. Ecol. Evol. 7, 6649–6658. doi: 10.1002/ece3.3222
Tøttrup, A. P., Klaassen, R., Kristensen, M. W., Strandberg, R., Vardanis, Y., Lindström, Å., et al. (2012). Drought in Africa caused delayed arrival of European songbirds. Science 338, 1307–1307. doi: 10.1126/science.1227548
Tøttrup, A. P., Rainio, K., Coppack, T., Lehikoinen, E., Rahbe, E., Rahbek, C., and Thorup, K. (2010). Local temperature fine-tunes the timing of spring migration in birds. Integr. Comp. Biol. 50, 293–304. doi: 10.1093/icb/icq028
Tøttrup, A. P., and Thorup, K. (2008). Sex-differentiated migration patterns, protandry and phenology in North European songbird populations. J. Ornithol. 149, 161–167. doi: 10.1007/s10336-007-0254-x
Tøttrup, A. P., Thorup, K., and Rahbek, C. (2006). Changes in timing of autumn migration in North European songbird populations. Ardea 94, 527–536.
Tøttrup, A. P., Thorup, K., Rainio, K., Yosef, R., Lehikoinen, E., and Rahbek, C. (2008). Avian migrants adjust migration in response to environmental conditions en route. Biol. Lett. 4, 685–688. doi: 10.1098/rsbl.2008.0290
Tryjanowski, P., Kuźniak, S., and Sparks, T. (2002). Earlier arrival of some farmland migrants in western Poland. Ibis 144, 62–68. doi: 10.1046/j.0019-1019.2001.00022.x
Tryjanowski, P., Kuźniak, S., and Sparks, T. (2005). What affects the magnitude of change in first arrival dates of migrant birds? J. Ornithol. 146, 200–205. doi: 10.1007/s10336-005-0079-4
Underhill, L. G., Herremans, M., Navarro, R. A., and Harrison, J. A. (1998). Where do Palearctic migrant passerines concentrate in southern Africa during. Biol. Cons. Fauna 102, 168–174.
Vickery, J. A., Ewing, S. R., Smith, K. W., Pain, D. J., Bairlein, F., Škorpilová, J., et al. (2014). The decline of Afro-Palaearctic migrants and an assessment of potential causes. Ibis 156, 1–22. doi: 10.1111/ibi.12118
Walther, B. A., and Rahbek, C. (2002). Where do Palearctic migratory birds overwinter in Africa. Danks Orn Foren Tidsskr 96, 4–8. doi: 10.1371/journal.pone.0045005
Wisz, M. S., Walther, B., and Rahbek, C. (2007). Using potential distributions to explore determinants of Western Palaearctic migratory songbird species richness in sub-Saharan Africa. J. Biogeogr. 34, 828–841. doi: 10.1111/j.1365-2699.2006.01661.x
Keywords: arrival timing, biometrics, climate change, EVI, migration, flexibility, passerines, phenology
Citation: Aharon-Rotman Y, McEvoy JF, Kiat Y, Raz T and Perlman GY (2022) Time to Move On: The Role of Greenness in Africa and Temperatures at a Mediterranean Stopover Site in Migration Decision of Long-Distance Migratory Passerines. Front. Ecol. Evol. 10:834074. doi: 10.3389/fevo.2022.834074
Received: 13 December 2021; Accepted: 28 March 2022;
Published: 15 April 2022.
Edited by:
Simeon Lisovski, Alfred Wegener Institute Helmholtz Centre for Polar and Marine Research (AWI), GermanyReviewed by:
Birgen Haest, Swiss Ornithological Institute, SwitzerlandCopyright © 2022 Aharon-Rotman, McEvoy, Kiat, Raz and Perlman. This is an open-access article distributed under the terms of the Creative Commons Attribution License (CC BY). The use, distribution or reproduction in other forums is permitted, provided the original author(s) and the copyright owner(s) are credited and that the original publication in this journal is cited, in accordance with accepted academic practice. No use, distribution or reproduction is permitted which does not comply with these terms.
*Correspondence: Yaara Aharon-Rotman, WWFhcmEuQWhhcm9uLVJvdG1hbkB1bmUuZWR1LmF1
Disclaimer: All claims expressed in this article are solely those of the authors and do not necessarily represent those of their affiliated organizations, or those of the publisher, the editors and the reviewers. Any product that may be evaluated in this article or claim that may be made by its manufacturer is not guaranteed or endorsed by the publisher.
Research integrity at Frontiers
Learn more about the work of our research integrity team to safeguard the quality of each article we publish.