- 1School of Biosciences, Cardiff University, Cardiff, United Kingdom
- 2Road Ecology Center, Department of Environmental Science and Policy, University of California, Davis, Davis, CA, United States
- 3The Endangered Wildlife Trust – Wildlife and Transport Programme, Johannesburg, South Africa
- 4SARChI Chair on Biodiversity Value and Change, School of Mathematical and Natural Sciences, University of Venda, Thohoyandou, South Africa
The SARS-CoV-2 pandemic severely reduced many human activities. So pronounced was the change, it has given rise to the term “anthropause”: the considerable alteration of modern human activities. Among these was surface transportation, with prolonged traffic reductions, in excess, of 50% in many countries. Roads and traffic are responsible for functionally fragmenting ecosystems, wildlife populations, and species interactions. The unintentional “dialing-down” of traffic has given continuous monitoring systems of wildlife-vehicle conflict a unique opportunity to study the consequences of perturbing this source of wildlife disturbance and mortality. Experimental manipulation of traffic at the global scale would not have been possible without mitigation responses to SARS-CoV-2. Such a perturbation allows robust empirical investigation into wildlife responses to traffic, including changes in mortality, behavior, genetic connectivity, and knock-on ecosystem effects, the responses to which can be replicated across a global network of wildlife-vehicle conflict monitoring systems. We review the extent to which these extensive data-collection systems provide the primary source of data to study many of these responses, providing the raw material to understand some striking wildlife consequences of the anthropause.
Introduction
Major roads split the Earth’s terrestrial surface into ∼ 600,000 patches, of which more than half are < 1 km2 in area (Ibisch et al., 2016). Wildlife-vehicle conflicts, including fatal collisions, are an inevitable consequence of this dense infrastructure. Despite many countries having mega datasets of wildlife-vehicle collisions, (WVC) (Shilling et al., 2020), as an attempt to quantify and therefore understand the where and why a WVC event occurs, these observational data do not fully address a central question, “what is the impact to biodiversity of roads and vehicles?”
Second to legal hunting, WVCs are estimated to be the largest source of anthropogenic mortality for vertebrates, globally (Hill et al., 2019). Estimates in terms of numerical loss of vertebrates in any given country are in the millions per annum (Schwartz et al., 2020). Globally, there are ∼1.4 billion vehicles and such high numbers begs the question, “what effect(s) do cars have on vertebrate ecology?” This is hard to answer because, as Paine’s classic experiments have taught us, to determine the nature of ecological interactions either the system or interactions must be perturbed (Paine, 1966). Paine removed top predator, Pisaster spp. from rocky shores in Makkaw (Washington, United States) and in doing so revealed ecological dynamics [keystone species (Paine, 1966) and trophic cascades (Paine, 1969)] that are a crucial part of species interactions, but which were not visible without perturbation. Similarly, simple numerical counts of WVCs will not inform whether traffic is altering the persistence, fitness and behavior of species and populations (van der Ree et al., 2011), or how roads may alter connectivity. To determine the ecological impact of WVCs on wildlife, we would need to perform a wide-scale field manipulation with replicates (Johnson, 2002); in other words, remove or reduce traffic across multiple countries. The global emergence of SARS-CoV-2 (the pathogen causing COVID-19) has provided such an experimental perturbation.
Experimental Perturbation: SARS-CoV-2 Gives Rise to the Anthropause
The SARS-CoV-2 outbreak was declared a global health emergency in January 2020 (Sohrabi et al., 2020) and a pandemic in March 2020 by the World Health Organization (WHO). In the face of this newly emerged and rapidly spreading zoonotic pathogen, attempts at disease control focused on reducing contact rates, termed “lockdowns” or “stay-at-home orders,” the strictest of which confined people to their homes (Hale et al., 2021). At peak restrictions (April 2020) over half of humanity, some 4.4 billion people worldwide, were under some form of lockdown (Bates et al., 2021). Control of SARS-CoV-2 subsequently led to a significant reduction in human activity and mobility globally, termed the “anthropause” (Rutz et al., 2020). So profound was the reduction in human mobility that it resulted in a “global quietening”; the longest and most prominent global anthropogenic seismic noise reduction since records began (Lecocq et al., 2020). Pronounced effects of the anthropause included a wide-scale reduction in vehicle use, as corroborated by Apple’s mobility data (Figure 1), and corresponding significant reductions in vehicle emissions: NO2 and particulate matter (Chauhan and Singh, 2020; Zambrano-Monserrate et al., 2020). At the height of mobility restrictions, vehicle use dropped by over 50% in many countries and, to date, is represented by several troughs in vehicle use compared to time periods of “normal” mobility (Figure 1). The anthropause enabled a unique opportunity for the greatest natural experiment of our time in terms of assessing anthropogenic effects on ecological systems (Bates et al., 2020), and so provides the chance to examine how traffic affects wildlife, using fundamental principles of experimental ecology. Here, we review evidence and discuss the opportunities provided by the anthropause to determine the ecological effects of traffic on wildlife.
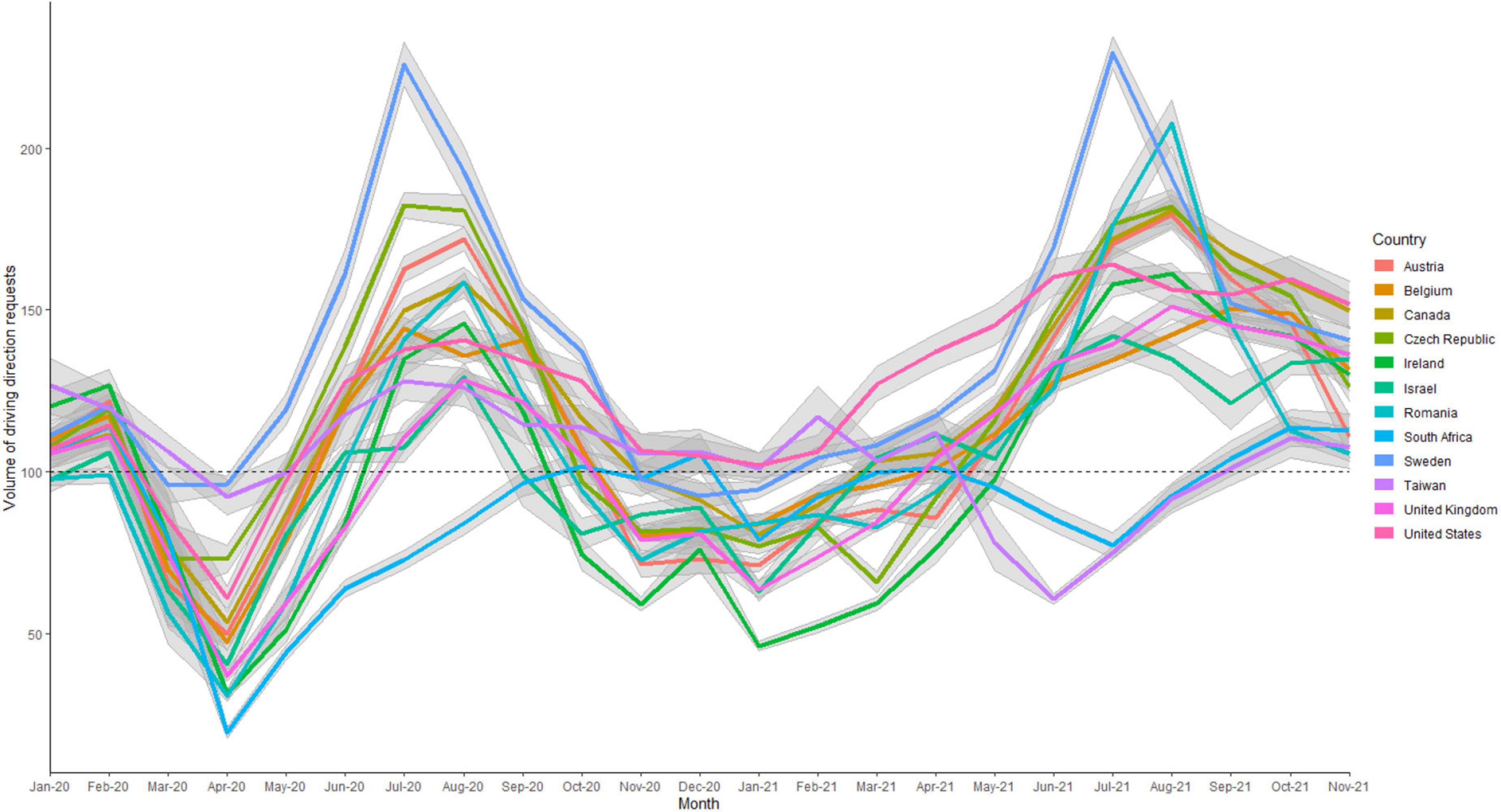
Figure 1. Country-specific changes in monthly mean aggregated requests for directions in Apple maps, representing a proxy for traffic activity during the SARS CoV-2 pandemic in 2020/21 relative to a baseline “norm” on 13th Jan 2020. Countries with data shown are those with National WVC schemes (after Shilling et al., 2020). Data source; https://covid19.apple.com/mobility.
An Anthropause-Mediated Reduction in Wildlife-Vehicle Collisions
We know that high traffic volume translates into high numbers of WVCs (Litvaitis and Tash, 2008). Therefore, one obvious outcome of reduced traffic due to the anthropause could be a corresponding reduction in collisions. Indeed, research to date, largely corroborates this hypothesis: In the United States, the number of large wild animals killed per day on state highways was reduced by 34% (Shilling et al., 2021). WVC reductions of 19% were observed in South Korea (Bates et al., 2021) and reductions ranged from 0–40% across 11 European countries (Bil et al., 2021), with the null effects occurring in countries where SARS-CoV-2 mitigation was minimal. A reduction of 48% in WVC was observed on one continuously monitored location during Australia’s anthropause (Driessen, 2021), although the sample size was small. In similar constant effort studies, a drop of 50% in WVC of the northern white-breasted hedgehog (Erinaceus roumanicus) was seen (Łopucki et al., 2021). In contrast, others have noted increases in WVC over the duration of the pandemic (Abraham and Mumma, 2021), although decreases were apparent in the time periods where the human mobility was most restricted. Species-specific effects linked to ecological traits are likely hidden within these patterns. The most dramatic reductions in traffic (Figure 1), coincided with a period of high mobility for many species in the northern hemisphere: the breeding season and migration period. Amphibians, the most seriously declining taxa on Earth, normally suffer huge road mortality due to site philopatry (Beebee, 2013), but the largest reduction in traffic coincided with their peak period of movement, which appears to have translated into significantly reduced amphibian mortality in some countries (Manenti et al., 2020; Bates et al., 2021; LeClair et al., 2021).
The Anthropause as a Perturbation Experiment: Ecological Effects
While the obvious direct effect of traffic reduction is reduced wildlife mortality across multiple species (described above) by reviewing current wildlife-vehicle conflict research, we may be able to posit some additional ecological effects (Figure 2). Roadkill risk is species-specific (Benítez-López et al., 2010; Alamgir et al., 2017) with slow-moving and wide-ranging species, for example, at a high risk of road mortality as a function of their life-history traits (González-Suárez et al., 2018). The positive effects of the anthropause could therefore be expected to be greatest for those species that are most prone to WVCs, but also those whose population sizes are most impacted; typically those with low reproductive rates (Rytwinski and Fahrig, 2012). Reduced traffic may lead to loss of “barrier effects,” isolation and/or, fragmentation of local populations which, in the long-term could be manifested as changes in gene flow and improved fitness (Figure 2; Benítez-López et al., 2010). Pollution events could be expected to reduce because even low concentrations of contaminants (associated with vehicle tires) can cause significant die-offs in fish (Tian et al., 2021).
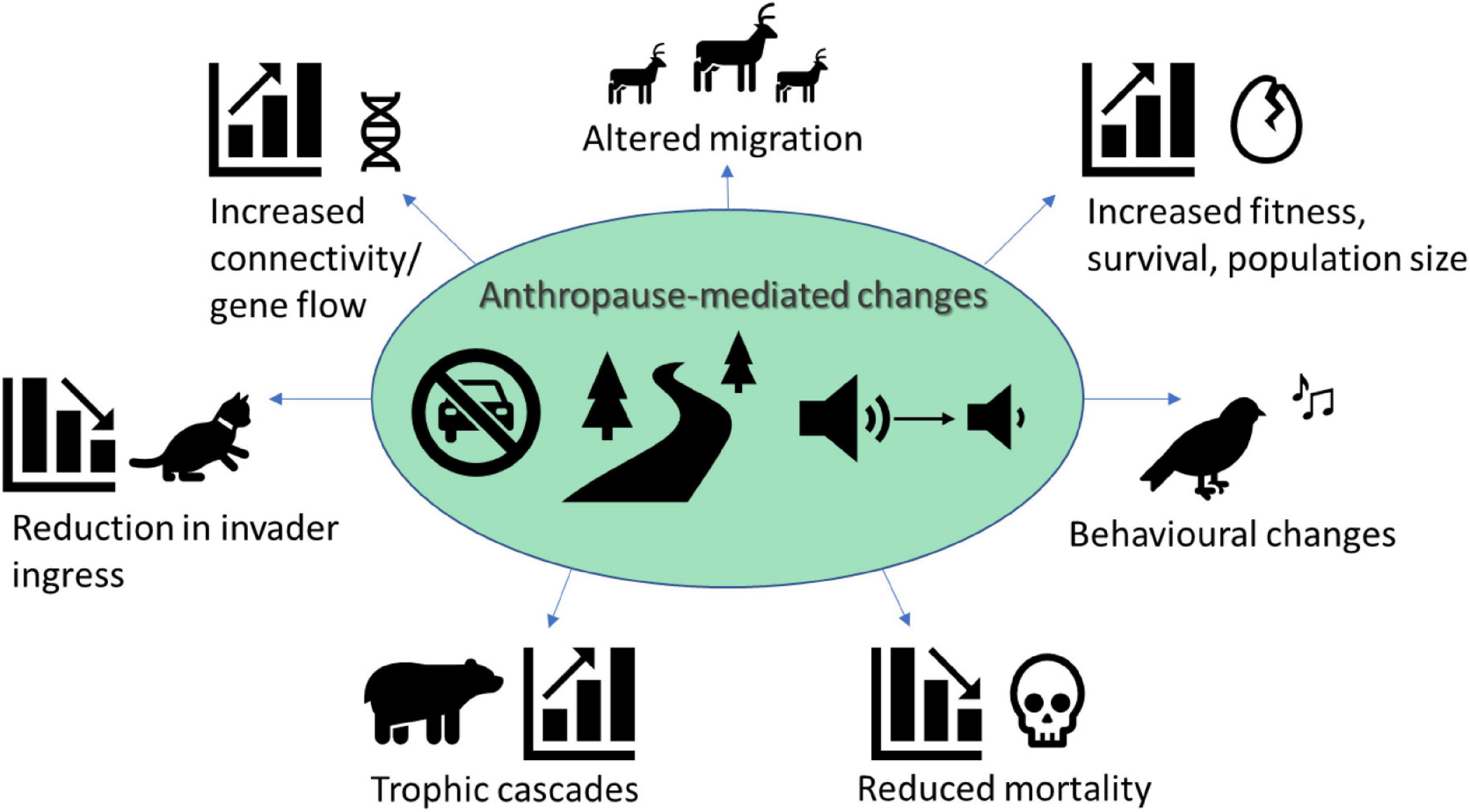
Figure 2. Overview of the anthropause-mediated changes (reduced traffic and noise) and the posited and realized ecological effects of the anthropause on wildlife-vehicle conflicts (WVC). To date, reductions in mortality (Manenti et al., 2020; Bates et al., 2021; Bil et al., 2021; Driessen, 2021; LeClair et al., 2021; Shilling et al., 2021; Łopucki et al., 2021) and behavioral changes (Derryberry et al., 2020) have been observed due to anthropause mediated reductions in traffic volume and traffic noise, respectively.
Species-specific differences in mortality risk or fitness leads back to Paine’s work (Paine, 1969, 1966), with trophic cascades a possible outcome. Some raptor species, for example, are attracted to roads (Benítez-López et al., 2010), potentially due to the availability of roadkill for scavenging (Schwartz et al., 2018). Any significant loss of biomass, in the form of roadkill, may lead to prey-switching, a biotic interaction that is acknowledged in the rewilding and invasive species literature to create ecosystem imbalance and alter evolving responses (Lurgi et al., 2018). Moose (Alces alces) have been shown to use roads to shield their vulnerable young from their main predator, the road-averse black bear (Ursus arctos), by basing their birth sites close to roads (Berger, 2007). Loss of the predator protection associated with roads could thus reduce prey fitness. Finally, the anthropause could offer a positive, but brief, respite from invasive species ingress and habitat loss due to road construction. While previous studies inform us that numerous ecological impacts due to traffic occur (Figure 2) the anthropause-mediated reprieve may be too short to observe some effects, for example, changes in gene flow.
Ecological Effects of the Anthropause: The Soundscape
Road traffic is a pervasive source of anthropogenic noise that has the potential to influence anti-predator behavior through perceived risk, or the “ecology of fear,” that could lead to reduced fitness through lost foraging opportunities. The theory posits that the costs of avoiding predation may reduce prey fecundity and survival to such an extent that it exceeds the reduction in prey numbers due to predation. Empirical evidence finds traffic noise alone can alter physiological stress and reduce offspring size (Zollinger et al., 2019). “Phantom road” experiments, where traffic-noise playback is introduced into a previously undisturbed and roadless area have shown a 25% decline in bird abundance, and complete avoidance of noisy sites by some species (McClure et al., 2013). Foraging, vigilance and resting behavior all significantly reduced in the prairie dog, Cynomys ludovicianus (Shannon et al., 2014), and activity was reduced across five bat species (Finch et al., 2020). Some species do not respond to traffic noise with avoidance behavior, but still show and impact of noise with reduced body condition potentially due to a reduction in foraging time (Ware et al., 2015). Combined, the previously published literature suggests traffic noise induces behavioral changes.
In Spring 2020, the SARS CoV-2 outbreak caused a global human quietus, much of which was due to reduced traffic volume (Lecocq et al., 2020). Loss of fear, due to traffic reductions could therefore be expected to create an ecological release, i.e., an increase in population size and/or fecundity and changes in behavior (Figure 2). Lockdowns resulted, at least anecdotally, in changes in animal distributions, notably large species frequenting urban areas (Rabou, 2020; Bates et al., 2021) and it is noted that reductions in large animal WVC were seen in the United States (Shilling et al., 2021). A rapid change in behavior was observed in response to anthropause-reduced traffic noise; urban white-crowned sparrows, Zonotrichia leucophrys, shifted their song frequency as they no longer needed to compete with traffic noise to be heard, so their song traveled further (Derryberry et al., 2020). The effect was greater than expected and could translate into fitness benefits (Halfwerk, 2020).
The Global Wildlife-Vehicle Collisions Observatory
There are ∼15 known national or regional-extent WVC reporting systems that continuously collect observations from government and volunteer sources (Shilling et al., 2020; Figure 1). Collectively, these systems document the spatial and temporal occurrences of thousands of species, millions of observations and span 120 sampling years (Shilling et al., 2020). Crucially, each scheme includes a period before, during, and will (eventually) include post-anthropause data. In our opinion, the global WVC observatory provides one of the largest continuous sets of species occurrence data for many terrestrial vertebrates and certainly the largest for WVC. In terms of determining the effects of the anthropause on WVC, each system represents a replicate across multiple countries, providing a rare opportunity in ecology for meta-replication: statistically powerful replication across studies (Johnson, 2002). We are not aware of a similar continuous, large-extent monitoring system for wildlife on each continent. This positions the WVC observatory dataset as a primary source of data for understanding both transportation impacts on wildlife and for the effect of the anthropause on wildlife occurrence.
Challenges exist, however, in terms of merging disparate datasets and accounting for changes in observer bias (Crimmins et al., 2021; Hochachka et al., 2021). Decreases in WVCs, for example, have been attributed to changes in reporter effort (Dörler and Heigl, 2021). WVC recording systems investigating effects of the anthropause should therefore carefully consider how to account for reporter bias both in terms of reduced effort and shifts in spatial observations. Estimates could be made using a combination of the observations, frequency of sampling by observers (e.g., how often they drive a route), observation efficiency, or by comparing relative changes in species. Caution is also needed in interpreting species level effects, controlling for that fact that the period of year when the reductions in traffic mobility were greatest may disproportionality favor certain species, e.g., amphibians in the Northern hemisphere. In addition, as a perturbation experiment the anthropause may represent a rather nuanced one, because while traffic volume decreased there is empirical evidence that traffic speeds increased (Katrakazas et al., 2020) with obvious detrimental effects that may have especially negative consequences for slow-moving species.
The anthropause-provided opportunities to study the effects of traffic on wildlife are already paying dividends, with noted reductions in WVCs (Manenti et al., 2020; Abraham and Mumma, 2021; Bates et al., 2021; Bil et al., 2021; Driessen, 2021; LeClair et al., 2021; Shilling et al., 2021; Łopucki et al., 2021) and rapid behavioral shifts that may increase fitness (Derryberry et al., 2020). We further posit additional potential changes to species interactions, behavior, fitness, animal distributions and gene flow due to the global respite from traffic impacts (Figure 2). The global WVC observatory provides several important avenues to investigate impacts, including very large numbers of: (1) species both within and among all large-extent systems, (2) individual records among and within each system, (3) continuous and varied spatial and temporal locations, (4) replicates among and within the global systems, and (5) recorded metadata to assist in using each record. The SARS-CoV-2 pandemic is still in progress. New variants of concern continue to emerge1 and there exists a likelihood of further, fluctuating lockdown policies, locally or at a national level (Hale et al., 2021). As an unintentional perturbation experiment, this one is still ongoing, with travel precautions and thus impacts to travel likely still in place for some time, and potential long-term changes in transport use are possible (van Wee and Witlox, 2021).
Discussion—The Anthropause Perturbation
Biodiversity declines in the Anthropocene are severe, with even common species undergoing declines (Ceballos et al., 2017). Conservation of imperiled or even common wildlife species may have received an unprecedented “bump” during the anthropause because of reduced mortality or indirect ecological effects (Bates et al., 2021). Consequently, wildlife populations of individual species, or demographic groups may have increased during reduced traffic periods (which will vary amongst countries), providing both short- or long-term benefits. It is likely, however, that the reprieve from traffic is short-lived, although the anthropause has demonstrated the capacity for change.
Framing the reduction in WVCs in terms of biological conservation is important for future policy development at the intersection of transportation and ecology. The potential scale of the reduction also suggests a number of ideas and needs: (1) there may be too much traffic for wildlife to survive in many places; (2) continuous and widespread WVC surveillance is needed to track composition, numbers and losses of wildlife communities; (3) we need standardized reporting systems that track sampling effort, species, demographics, carcass loss rates, and other parameters to estimate true WVC rates; and, (4) loss of biodiversity and human incursion into wildlife ecosystems alters the probability of zoonotic spillover (Keesing et al., 2010; Rohr et al., 2020), exposing humanity to systemic harm, for example, a pandemic.
The SARS-CoV-2 pandemic may not be the only anthropause wildlife have experienced in terms of reduced traffic. If primary energy demand is a proxy for vehicle use then it has dropped substantially in the past; the 1918 flu pandemic, the great depression (1920s), World War II (1940s), the oil crisis/shock of the 70s/80s and the global financial crisis all resulted in significant reductions in energy demand (Ibn-Mohammed et al., 2021). Are there then other opportunities to revisit long-term datasets and address “was the impact of roads on biodiversity visible in previous anthropause periods?” Such effects would only be possible where long-term data collection exist. Such data, although not focused on WVCs, exist for birds, including the Common Birds Census (British Trust for Ornithology) in the United Kingdom between 1962 and 2000, the Christmas Bird Count (Audubon Society) in the United States between 1900 and present, and the South African Bird Atlas Project (University of Cape Town) between 1987 and present.
The reduction in WVCs may be fleeting, and, cynically, we could say it is not so much a welcome development but an indictment of the way things were before the anthropause. Isolation is not a novel solution in a post pandemic world, and we think that this difficult time could drive people to adopt new habits that reduce their ecological footprint and thereby help to safeguard biodiversity (Bavel et al., 2020). If we are successful in communicating the benefits of these new habits, a greener economy will eventually become a reality in an improved “Brave New World.” Virtual connectivity and social organization based on home-as-office are rapidly emerging, and technologies may help to recover the economy and decrease our carbon footprint, thus reducing our impact as a hyper-keystone species (Worm and Paine, 2016).
After continuously removing Pisaster starfish from the rocky shore for 20 years, Paine’s swansong experiment was to let them recolonize. As predicted, the ecosystem bounced back, and lost species returned (Lafferty and Suchanek, 2016). Similarly, we expect the traffic-related effects of the anthropause to be ephemeral and with increased human mobility so the old normal may return. Regardless, the anthropause and Paine’s foundational study demonstrate how important it is to observe and learn from nature during ecological disruption, to understand processes and relationships and ultimately protect it. Although few positive outcomes await animals on roads as traffic returns, critically evaluating the impact of this widespread human activity on wildlife must be one of them.
Data Availability Statement
Publicly available datasets were analyzed in this study. This data can be found here: https://covid19.apple.com/mobility.
Author Contributions
SP, FS, and WC conceived the presented idea. All authors made substantial contributions to conception and design, drafting and critical revision of the article, and approved it for publication.
Conflict of Interest
The authors declare that the research was conducted in the absence of any commercial or financial relationships that could be construed as a potential conflict of interest.
Publisher’s Note
All claims expressed in this article are solely those of the authors and do not necessarily represent those of their affiliated organizations, or those of the publisher, the editors and the reviewers. Any product that may be evaluated in this article, or claim that may be made by its manufacturer, is not guaranteed or endorsed by the publisher.
Acknowledgments
We acknowledge the vast suffering that people and communities have endured during the pandemic and in recognizing the benefits that wildlife have received from reductions in traffic due to the pandemic are not trying to downplay this suffering. We want to thank the many thousands of WVC observers throughout the world whose work enables us to propose studying impacts to wildlife from traffic and traffic reductions.
Footnotes
- ^ https://www.who.int/news/item/26-11-2021-classification-of-omicron-(b.1.1.529)-sars-cov-2-variant-of-concern
References
Abraham, J. O., and Mumma, M. A. (2021). Elevated wildlife-vehicle collision rates during the COVID-19 pandemic. Sci. Rep. 11:20391. doi: 10.1038/s41598-021-99233-9
Alamgir, M., Campbell, M. J., Sloan, S., Goosem, M., Clements, G. R., Mahmoud, M. I., et al. (2017). Economic, socio-political and environmental risks of road development in the tropics. Curr. Biol. 27, R1130–R1140. doi: 10.1016/j.cub.2017.08.067
Bates, A. E., Primack, R. B., Biggar, B. S., Bird, T. J., Clinton, M. E., Command, R. J., et al. (2021). Global COVID-19 lockdown highlights humans as both threats and custodians of the environment. Biol. Conserv. 263:109175. doi: 10.1016/j.biocon.2021.109175
Bates, A. E., Primack, R. B., Moraga, P., and Duarte, C. M. (2020). COVID-19 pandemic and associated lockdown as a “Global human confinement experiment” to investigate biodiversity conservation. Biol. Conserv. 248:108665. doi: 10.1016/j.biocon.2020.108665
Bavel, J. J. V., Baicker, K., Boggio, P. S., Capraro, V., Cichocka, A., Cikara, M., et al. (2020). Using social and behavioural science to support COVID-19 pandemic response. Nat. Hum. Behav. 4, 460–471. doi: 10.1038/s41562-020-0884-z
Beebee, T. J. C. (2013). Effects of road mortality and mitigation measures on amphibian populations. Conserv. Biol. 27, 657–668. doi: 10.1111/cobi.12063
Benítez-López, A., Alkemade, R., and Verweij, P. (2010). The impacts of roads and other infrastructure on mammal and bird populations: a meta-analysis. Biol. Conserv. 143, 1307–1316. doi: 10.1016/j.biocon.2010.02.009
Berger, J. (2007). Fear, human shields and the redistribution of prey and predators in protected areas. Biol. Lett. 3, 620–623. doi: 10.1098/rsbl.2007.0415
Bil, M., Andrasik, R., Cicha, V., Arnon, A., Kruuse, M., Langbein, J., et al. (2021). COVID-19 related travel restrictions prevented numerous wildlife deaths on roads: a comparative analysis of results from 11 countries. Biol. Conserv. 256:109076. doi: 10.1016/j.biocon.2021.109076
Ceballos, G., Ehrlich, P. R., and Dirzo, R. (2017). Biological annihilation via the ongoing sixth mass extinction signaled by vertebrate population losses and declines. Proc. Natl. Acad. Sci. U.S.A. 114, E6089–E6096. doi: 10.1073/pnas.1704949114
Chauhan, A., and Singh, R. P. (2020). Decline in PM2.5 concentrations over major cities around the world associated with COVID-19. Environ. Res. 187:109634. doi: 10.1016/j.envres.2020.109634
Crimmins, T. M., Posthumus, E., Schaffer, S., and Prudic, K. L. (2021). COVID-19 impacts on participation in large scale biodiversity-themed community science projects in the United States. Biol. Conserv. 256:109017. doi: 10.1186/s12889-021-12007-w
Derryberry, E. P., Phillips, J. N., Derryberry, G. E., Blum, M. J., and Luther, D. (2020). Singing in a silent spring: birds respond to a half-century soundscape reversion during the COVID-19 shutdown. Science 370, 575–579. doi: 10.1126/science.abd5777
Dörler, D., and Heigl, F. (2021). A decrease in reports on road-killed animals based on citizen science during COVID-19 lockdown. PeerJ 9:e12464. doi: 10.7717/peerj.12464
Driessen, M. M. (2021). COVID-19 restrictions provide a brief respite from the wildlife Roadkill toll. Biol. Conserv. 256:109012. doi: 10.1016/j.biocon.2021.109012
Finch, D., Schofield, H., and Mathews, F. (2020). Traffic noise playback reduces the activity and feeding behaviour of free-living bats. Environ. Pollut. 263:114405. doi: 10.1016/j.envpol.2020.114405
González-Suárez, M., Ferreira, F. Z., and Grilo, C. (2018). Spatial and species-level predictions of road mortality risk using trait data. Glob. Ecol. Biogeogr. 27, 1093–1105.
Hale, T., Angrist, N., Goldszmidt, R., Kira, B., Petherick, A., Phillips, T., et al. (2021). A global panel database of pandemic policies (Oxford COVID-19 government response tracker). Nat. Hum. Behav. 5, 529–538. doi: 10.1038/s41562-021-01079-8
Hill, J. E., DeVault, T. L., and Belant, J. L. (2019). Cause-specific mortality of the world’s terrestrial vertebrates. Glob. Ecol. Biogeogr. 28, 680–689. doi: 10.1002/ecy.2865
Hochachka, W. M., Alonso, H., Gutiérrez-Expósito, C., Miller, E., and Johnston, A. (2021). Regional variation in the impacts of the COVID-19 pandemic on the quantity and quality of data collected by the project eBird. Biol. Conserv. 254:108974. doi: 10.1016/j.biocon.2021.108974
Ibisch, P. L., Hoffmann, M. T., Kreft, S., Pe’er, G., Kati, V., Biber-Freudenberger, L., et al. (2016). A global map of roadless areas and their conservation status. Science 354, 1423–1427. doi: 10.1126/science.aaf7166
Ibn-Mohammed, T., Mustapha, K. B., Godsell, J., Adamu, Z., Babatunde, K. A., Akintade, D. D., et al. (2021). A critical analysis of the impacts of COVID-19 on the global economy and ecosystems and opportunities for circular economy strategies. Resour. Conserv. Recycl. 164:105169. doi: 10.1016/j.resconrec.2020.105169
Johnson, D. H. (2002). The importance of replication in wildlife research. J. Wildl. Manag. 66, 919–932.
Katrakazas, C., Michelaraki, E., Sekadakis, M., and Yannis, G. (2020). A descriptive analysis of the effect of the COVID-19 pandemic on driving behavior and road safety. Transp. Res. Interdiscip. Perspect. 7:100186. doi: 10.1016/j.trip.2020.100186
Keesing, F., Belden, L. K., Daszak, P., Dobson, A., Harvell, C. D., Holt, R. D., et al. (2010). Impacts of biodiversity on the emergence and transmission of infectious diseases. Nature 468, 647–652. doi: 10.1038/nature09575
Lafferty, K. D., and Suchanek, T. H. (2016). Revisiting Paine’s 1966 sea star removal experiment, the most-cited empirical article in the American naturalist. Am. Nat. 188, 365–378. doi: 10.1086/688045
LeClair, G., Chatfield, M. W. H., Wood, Z., Parmelee, J., and Frederick, C. A. (2021). Influence of the COVID-19 pandemic on amphibian road mortality. Conserv. Sci. Pract. 3:e535. doi: 10.1111/csp2.535
Lecocq, T., Hicks, S. P., Noten, K. V., van Wijk, K., Koelemeijer, P., Plaen, R. S. M. D., et al. (2020). Global quieting of high-frequency seismic noise due to COVID-19 pandemic lockdown measures. Science 369, 1338–1343. doi: 10.1126/science.abd2438
Litvaitis, J. A., and Tash, J. P. (2008). An approach toward understanding wildlife-vehicle collisions. Environ. Manage. 42, 688–697. doi: 10.1007/s00267-008-9108-4
Łopucki, R., Kitowski, I., Perlińska-Teresiak, M., and Klich, D. (2021). How is wildlife affected by the COVID-19 pandemic? Lockdown effect on the road mortality of hedgehogs. Animals 11:868. doi: 10.3390/ani11030868
Lurgi, M., Ritchie, E. G., and Fordham, D. A. (2018). Eradicating abundant invasive prey could cause unexpected and varied biodiversity outcomes: the importance of multispecies interactions. J. Appl. Ecol. 55, 2396–2407.
Manenti, R., Mori, E., Di Canio, V., Mercurio, S., Picone, M., Caffi, M., et al. (2020). The good, the bad and the ugly of COVID-19 lockdown effects on wildlife conservation: insights from the first European locked down country. Biol. Conserv. 249:108728. doi: 10.1016/j.biocon.2020.108728
McClure, C. J. W., Ware, H. E., Carlisle, J., Kaltenecker, G., and Barber, J. R. (2013). An experimental investigation into the effects of traffic noise on distributions of birds: avoiding the phantom road. Proc. Biol. Sci. 280:20132290. doi: 10.1098/rspb.2013.2290
Paine, R. T. (1969). The pisaster-tegula interaction: prey patches, predator food preference, and intertidal community structure. Ecology 50, 950–961. doi: 10.2307/1936888
Rabou, A. F. N. A. (2020). How is the COVID-19 outbreak affecting wildlife around the world? Open J. Ecol. 10, 497–517.
Rohr, J. R., Civitello, D. J., Halliday, F. W., Hudson, P. J., Lafferty, K. D., Wood, C. L., et al. (2020). Towards common ground in the biodiversity–disease debate. Nat. Ecol. Evol. 4, 24–33. doi: 10.1038/s41559-019-1060-6
Rutz, C., Loretto, M.-C., Bates, A. E., Davidson, S. C., Duarte, C. M., Jetz, W., et al. (2020). COVID-19 lockdown allows researchers to quantify the effects of human activity on wildlife. Nat. Ecol. Evol. 4, 1156–1159. doi: 10.1038/s41559-020-1237-z
Rytwinski, T., and Fahrig, L. (2012). Do species life history traits explain population responses to roads? A meta-analysis. Biol. Conserv. 147, 87–98. doi: 10.1016/j.biocon.2011.11.023
Schwartz, A. L. W., Shilling, F. M., and Perkins, S. E. (2020). The value of monitoring wildlife Roadkill. Eur. J. Wildl. Res. 66:18.
Schwartz, A. L. W., Williams, H. F., Chadwick, E., Thomas, R. J., and Perkins, S. E. (2018). Roadkill scavenging behaviour in an urban environment. J. Urban Ecol. 4:juy006.
Shannon, G., Angeloni, L. M., Wittemyer, G., Fristrup, K. M., and Crooks, K. R. (2014). Road traffic noise modifies behaviour of a keystone species. Anim. Behav. 94, 135–141.
Shilling, F., Collinson, W., Bil, M., Vercayie, D., Heigl, F., Perkins, S. E., et al. (2020). Designing wildlife-vehicle conflict observation systems to inform ecology and transportation studies. Biol. Conserv. 251:108797.
Shilling, F., Nguyen, T., Saleh, M., Kyaw, M. K., Tapia, K., Trujillo, G., et al. (2021). A reprieve from US wildlife mortality on roads during the COVID-19 pandemic. Biol. Conserv. 256:109013. doi: 10.1016/j.biocon.2021.109013
Sohrabi, C., Alsafi, Z., O’Neill, N., Khan, M., Kerwan, A., Al-Jabir, A., et al. (2020). World health organization declares global emergency: a review of the 2019 novel coronavirus (COVID-19). Int. J. Surg. 76, 71–76.
Tian, Z., Zhao, H., Peter, K. T., Gonzalez, M., Wetzel, J., Wu, C., et al. (2021). A ubiquitous tire rubber–derived chemical induces acute mortality in coho salmon. Science 371, 185–189.
van der Ree, R., Jaeger, J. A. G., van der Grift, E., and Clevenger, A. (2011). Effects of roads and traffic on wildlife populations and landscape function: road ecology is moving toward larger scales. Ecol. Soc. 16:48.
van Wee, B., and Witlox, F. (2021). COVID-19 and its long-term effects on activity participation and travel behaviour: a multiperspective view. J. Transp. Geogr. 95:103144. doi: 10.1016/j.jtrangeo.2021.103144
Ware, H. E., McClure, C. J. W., Carlisle, J. D., and Barber, J. R. (2015). A phantom road experiment reveals traffic noise is an invisible source of habitat degradation. Proc. Natl. Acad. Sci. U.S.A. 112, 12105–12109. doi: 10.1073/pnas.1504710112
Worm, B., and Paine, R. T. (2016). Humans as a hyperkeystone species. Trends Ecol. Evol. 31, 600–607. doi: 10.1016/j.tree.2016.05.008
Zambrano-Monserrate, M. A., Ruano, M. A., and Sanchez-Alcalde, L. (2020). Indirect effects of COVID-19 on the environment. Sci. Total Environ. 728:138813.
Keywords: anthropogenic disturbance, wildlife-vehicle conflict, perturbation, citizen science, traffic, COVID-19 quietus
Citation: Perkins SE, Shilling F and Collinson W (2022) Anthropause Opportunities: Experimental Perturbation of Road Traffic and the Potential Effects on Wildlife. Front. Ecol. Evol. 10:833129. doi: 10.3389/fevo.2022.833129
Received: 10 December 2021; Accepted: 16 February 2022;
Published: 07 March 2022.
Edited by:
Orsolya Valkó, Hungarian Academy of Science, HungaryReviewed by:
Tom Langen, Clarkson University, United StatesCopyright © 2022 Perkins, Shilling and Collinson. This is an open-access article distributed under the terms of the Creative Commons Attribution License (CC BY). The use, distribution or reproduction in other forums is permitted, provided the original author(s) and the copyright owner(s) are credited and that the original publication in this journal is cited, in accordance with accepted academic practice. No use, distribution or reproduction is permitted which does not comply with these terms.
*Correspondence: Sarah E. Perkins, cGVya2luc3NAY2FyZGlmZi5hYy51aw==