- 1Programa de Pós-graduação em Ecologia e Conservação da Biodiversidade, Universidade Estadual de Santa Cruz, Ilhéus, Brazil
- 2Departamento de Ciências Biológicas, Universidade Estadual de Santa Cruz, Ilhéus, Brazil
- 3Herpetology Section, Zoologisches Forschungsmuseum Alexander Koenig, Bonn, Germany
- 4Department of Physiology and Behavior, Laboratory of Bioacoustics (LaB) and EcoAcoustic Research Hub (EAR-Hub) – Biosciences Center, Federal University of Rio Grande do Norte, Natal, Brazil
Acoustic communication is a way of information exchange between individuals, and it is used by several animal species. Therefore, the detection, recognition and correct understanding of acoustic signals are key factors in effective communication. The priority of acoustic communication is effectiveness rather than perfection, being effective avoids affecting the sound-based communication system of the species. One of the factors that can affect effective communication is the overlap in time and frequency during signal transmission, known as signal masking. One type of sound that can cause masking is anthropogenic noise, which is currently increasing due to urban growth and consequently motorized transportation and machinery. When exposed to anthropogenic noise, animals can use compensatory mechanisms to deal with sound masking, such as the modification of acoustic parameters of their acoustic signal. Here, we performed a meta-analysis investigating whether different taxa have a general tendency for changes in acoustic parameters due to anthropogenic noise, we used taxa and acoustic parameters available in the literature that met the minimum criteria to perform a meta-analysis. We hypothesized that animals exposed to anthropogenic noise use compensation mechanisms, such as changes in dominant, maximum or minimum frequencies, call duration, note duration and call rate to deal with masking. We performed a meta-analysis, which synthesized information from 73 studies comprising 82 species of three taxa: insects, anurans and birds. Our results showed that in the presence of anthropogenic noise, insects did not change the acoustic parameters, while anurans increased call amplitude and birds increased dominant frequency, minimum and maximum frequencies, note duration and amplitude of their songs. The different responses of the groups to anthropogenic noise may be related to their particularities in the production and reception of sound or to the differences in the acoustic parameters considered between the taxa and also the lack of studies in some taxa.
Introduction
Acoustic communication is widely used by animals to exchange information among individuals. It is accomplished through an acoustic signal generated by a sender, propagated through the environment and received by a receptor (Wiley and Richards, 1978; Ryan and Kime, 2003). Acoustic signals can reach long distances and carry information such as the identity, location and sexual status of the sender (Gerhardt and Huber, 2002). Their use is relevant in many contexts, such as attracting partners for breeding, territorial defense and danger alert (Gerhardt and Huber, 2002). When the communication process is not effective from emission to signal reception, interactions among communicating individuals are compromised.
Anthropogenic noise is a type of sound that can be considered as a kind of environmental pollution and that can interfere with the acoustic communication of animals (Harding et al., 2019). Usually, the anthropogenic noise energy is in the range from 1 to 4 kHz (Job et al., 2016), but for example a travelling truck can occupy a noise range from 1 to 8.4 kHz (Duarte et al., 2019). Several negative effects have been attributed to anthropogenic noise, such as decreased species richness and abundance (Francis et al., 2009; Benítez-López et al., 2010; McClure et al., 2013), altered biotic interactions (Shannon et al., 2016; Phillips et al., 2019), and physiological effects on individuals such as stress due to exposure to noise (Tennessen et al., 2016).
When there is an overlap in the time and frequency spectrum of the noise and the sound produced by species, masking effect occurs, which can inhibit the perception of acoustic signals of animals (Patterson and Green, 1978; Brumm and Slabbekoorn, 2005; Wiley, 2006). To avoid masking effects in noisy environments, animals can also alter calling behavior patterns, since individuals of many species modify calling activity periods (Sousa-Lima and Clark, 2008; Dominoni et al., 2016). Some animals can also adjust the properties of the acoustic signal, according to their phenotypic flexibility (Piersma and Drent, 2003), this phenomenon can even be transmitted from one generation to the next one, resulting in evolutionary changes (Brumm and Slabbekoorn, 2005). Masking caused by biotic or abiotic noise can influence the ecology and evolution of various sound communication systems in animals (Brumm and Slabbekoorn, 2005; Slabbekoorn and Ripmeester, 2008).
Anthropogenic noise occupies a sound frequency band similar to that used by some species of insects, anurans and birds. Studies suggest that a strategy to deal with this is altering the acoustic parameters (Hu and Cardoso, 2010; Lampe et al., 2012; McCarthy et al., 2013; Montague et al., 2013). Understanding whether changes in acoustic parameters caused by noise are responses to phenotypic or evolutionary plasticity has been investigated for biotic and abiotic noise (Brumm and Slabbekoorn, 2005; Slabbekoorn and Ripmeester, 2008). In insects, a study found that Grasshoppers raised under noisy conditions produced songs with higher maximum-frequency as adults, suggesting plasticity in this spectral property of sound (Lampe et al., 2014). In anurans, the frequency of call is conditioned by morphological constraints, such as body size, and is considered a parameter conserved between generations, nevertheless, individuals that live in environments with constant abiotic noise call at higher dominant frequencies than expected, suggesting evolutionary changes (Goutte et al., 2016; Röhr et al., 2016). In birds, evolutionary changes are also reported, proposing that biotic noise has shaped certain bird sound (Dubois and Martens, 1984; Brumm and Slabbekoorn, 2005), but changes are also suggested due to vocal plasticity (Gross et al., 2010; Bermúdez-Cuamatzin et al., 2012). Modification of acoustic parameters to avoid masking can compromise signal reception and affect its function (Patricelli and Blickley, 2006). The level of sound masking caused by anthropogenic noise and the ability to compensate for it may vary depending on the group of animals (Brumm and Slabbekoorn, 2005; Raboin and Elias, 2019).
Some review studies have investigated the effect of anthropogenic noise, evaluating changes in frequency (Roca et al., 2016), experimental studies (Kunc and Schmidt, 2021) or changes in the ocean soundscape (Duarte et al., 2021). Here, we performed a meta-analysis of the general patterns of each acoustic parameter that met the minimum criteria for a meta-analysis, investigating their changes as a consequence of anthropic noise in three groups. We hypothesize that animals exposed to noise will use compensation mechanisms to avoid masking. For some insects, anurans, and birds, sound emission is in the same frequency band as anthropogenic noise, so we predicted similar effects in the three investigated taxa. Specifically, we expected: (i) increase in the dominant, minimum and maximum frequencies, to avoid overlapping the same frequency range of the noise (Nemeth and Brumm, 2009; Lampe et al., 2014; Grenat et al., 2019); (ii) increase in call/song duration, note duration and call/song rate, to increase the chances of being detected in the presence of noise (Kaiser and Hammers, 2009; Roca et al., 2016); and (iii) increase in amplitude, to be heard in an environment with anthropogenic noise (Zollinger and Brumm, 2011; Zhao et al., 2018).
Methods
Literature Search
We searched for studies which investigated changes in signals through acoustic parameters modified by the action of anthropogenic sounds. We performed a systematic review following PRISMA protocol (Page et al., 2021). Searches were implemented on the Scopus and Web of Science platforms for all available years through January 2022. Searches were performed using the following keywords: (“noise*” OR “masking”) AND (“traffic*” OR “road*” OR “urban” OR “anthropic” OR “anthropogenic”) AND (“signal*” OR “call*” OR “vocalization*” OR “song*”). These keywords were searched in the title, abstract and keywords of the studies. The number of records obtained in the systematic review is presented in a flow chart (Figure 1 and Supplementary Table 1).
Criteria for Study Inclusion
We selected studies according to the following criteria: (i) investigated the effect of anthropogenic sounds on the acoustic parameter, with control (not exposed to anthropogenic sounds) and treatment (exposed to anthropogenic noise), conducted through experiment or in nature; (ii) studies with one or more acoustic parameters evaluated (e.g., dominant frequency, call duration, call rate); (iii) terrestrial animals; and (iv) studies with information on: mean, standard deviation and number of individuals sampled for each treatment (control and noise), or studies with sufficient statistical information to allow calculation of effect size.
In our study, we had to deal with measurement errors, such as bias generated when extracting minimum and maximum frequency measurements visually from spectrograms. Beecher (1988) and Zollinger et al. (2012) reported similar problems for studying birdsong in noisy environments, for example, two sounds identical in frequency and amplitude can look markedly different on a spectrogram if there is some other higher amplitude sound in the background of a recording that is not present in the other, also noise can make it difficult to detect the minimum frequency of the signal in the spectrogram. To avoid measurement errors, it is recommended to use power or amplitude spectra (Zollinger et al., 2012). From the power spectra, the minimum and maximum frequency can be reliably measured (Zollinger et al., 2012; Brumm et al., 2017). We chose to include in our study only data from minimum and maximum frequencies measured in power spectrum or studies of experiments that controlled measurement errors. Therefore, we excluded from our database 82 individual responses that did not meet this criterion.
We built a database containing seven acoustic parameters: (i) dominant frequency (the frequency that contains more sound energy); (ii) minimum frequency (lowest frequency of sound), (iii) maximum frequency (highest frequency of sound); (iv) call duration (length from start to end of sound in insects and anurans; in birds the studies measure song duration (the start of the first element to the end of the last element of a song); (v) note duration (length from start to end of a note of the song); (vi) call/song rate (number of calls/songs emitted over of time); and (vii) call amplitude (sound pressure). Not all acoustic parameters were found in the three classes of animals that conform our database. The data obtained for insects includes the orders orthoptera and hemiptera, and in the literature we found the acoustic parameters dominant frequency and maximum frequency tested for anthropogenic noise. In anurans, we found only dominant frequency, call duration, call rate and call amplitude. For birds, we obtained data on all acoustic parameters, dominant frequency, maximum frequency, minimum frequency, song duration, note duration, song rate and song amplitude.
Effect Sizes
For each study that we extracted the mean, standard deviation and number of individuals sampled for each treatment (control and noise), we calculated the effect size using Hedges’ g statistic, using the “metafor” package (Viechtbauer, 2010). We extracted data found only in figures using the Get Data Graph Digitizer program (Get Data Graph Digitizer, 2021). Data available only in figures with median and interquartile range had their mean and standard deviation estimated according to the method of Hozo et al. (2005). For studies that did not report the mean and standard deviation, we used the “esc” package (Lüdecke, 2019) to convert statistical test results into Hedges’ g effect size measurements (Supplementary Table 2). The analyses were performed in R environment (R Core Team, 2021).
Controlling for Phylogenetic Signal
Our samples include species from three taxonomic groups, then it was necessary to control the non-independence of the species, because the evolutionary history of these species can be shared. With the species of our dataset, we created a tree with the phylogenetic and taxonomic information obtained in Tree of Life Web Project website (Tree of Life Web Project, 2007), these data were obtained using the package “rotl” (Michonneau et al., 2016). The lengths of the branches of the phylogenetic tree were calculated using the method of Grafen (1989) and a correlation matrix of phylogenetic relatedness among species was constructed for our dataset, these steps were performed using the package “ape” (Paradis and Schliep, 2019), the analyses were performed in the R environment (R Core Team, 2021; Supplementary Figure 1). This correlation matrix of phylogenetic was inserted in our meta-analysis as a random variable (see section “Meta-Analysis”).
Meta-Analysis
We tested the effect size of the data assuming that they were heterogeneous and performed a random-effects meta-analysis. We used a multilevel meta-analytical model to control non-independence between effect sizes using random variables in the model (Nakagawa and Santos, 2012; Nakagawa et al., 2017). We included “species” and “phylogenetic signal” as random variables in our model, species was included as a random variable to control for the effect of several studies investigating the same species. We built the model using the function rma.mv, and we adjusted it by using restricted maximum likelihood (REML). We included acoustic parameter as moderator in the model, for our result to be obtained for each acoustic parameter by taxon. The analyzes were performed in the “metafor” package (Viechtbauer, 2010), in the R environment (R Core Team, 2021).
We also investigated the presence of outliers as they may affect the validity and robustness of the meta-analysis (Viechtbauer and Cheung, 2010), we detected their presence through Cook’s distance. We removed data from our analysis with Cook’s distance above five, leading to the exclusion of eight potential outliers from our data base (see result with outliers in Supplementary Table 3).
Publication Bias
To test the publication bias we used the Egger regression test (Egger et al., 1997). We maintained the same model structure used to evaluate the effect of anthropogenic noise for the acoustic parameters, but we changed the moderator that was the acoustic parameter by the sample variance. When the regression intercept significantly deviates from zero it is considered that the data are asymmetric and biased toward publication bias (Sterne and Egger, 2006). We considered the result with a tendency for publication bias when the intercept differed from zero at p < 0.1 (Egger et al., 1997). Additionally, we measured the level of heterogeneity of the meta-analysis using I2, which describes the percentage of variation across the studies due to data heterogeneity (Higgins et al., 2003).
Results
We found a total of 73 studies (Figure 1), published between 2006 to January 2022 (Figure 2). The number of studies were conducted in 34 countries and varied for insects, anurans and birds (Figure 3). The most evaluated anthropogenic noise in the studies was urban and car traffic, but we also found noise generated by aircraft and gas compressor. From the studies we obtained 286 effect sizes, with individual responses distributed in the three taxa, where we found data for different acoustic parameters (Table 1). We obtained data from four insect species, 22 anuran species and 56 bird species, for a total of 82 species evaluated. The Egger’s regression model was not significant, showing that our results are unaffected by the publication bias (intercept = 0.0438; C.I. = −0.37 to 0.46; p = 0.8372). Additionally, we observed a high level of heterogeneity (I2 = 92.99%).
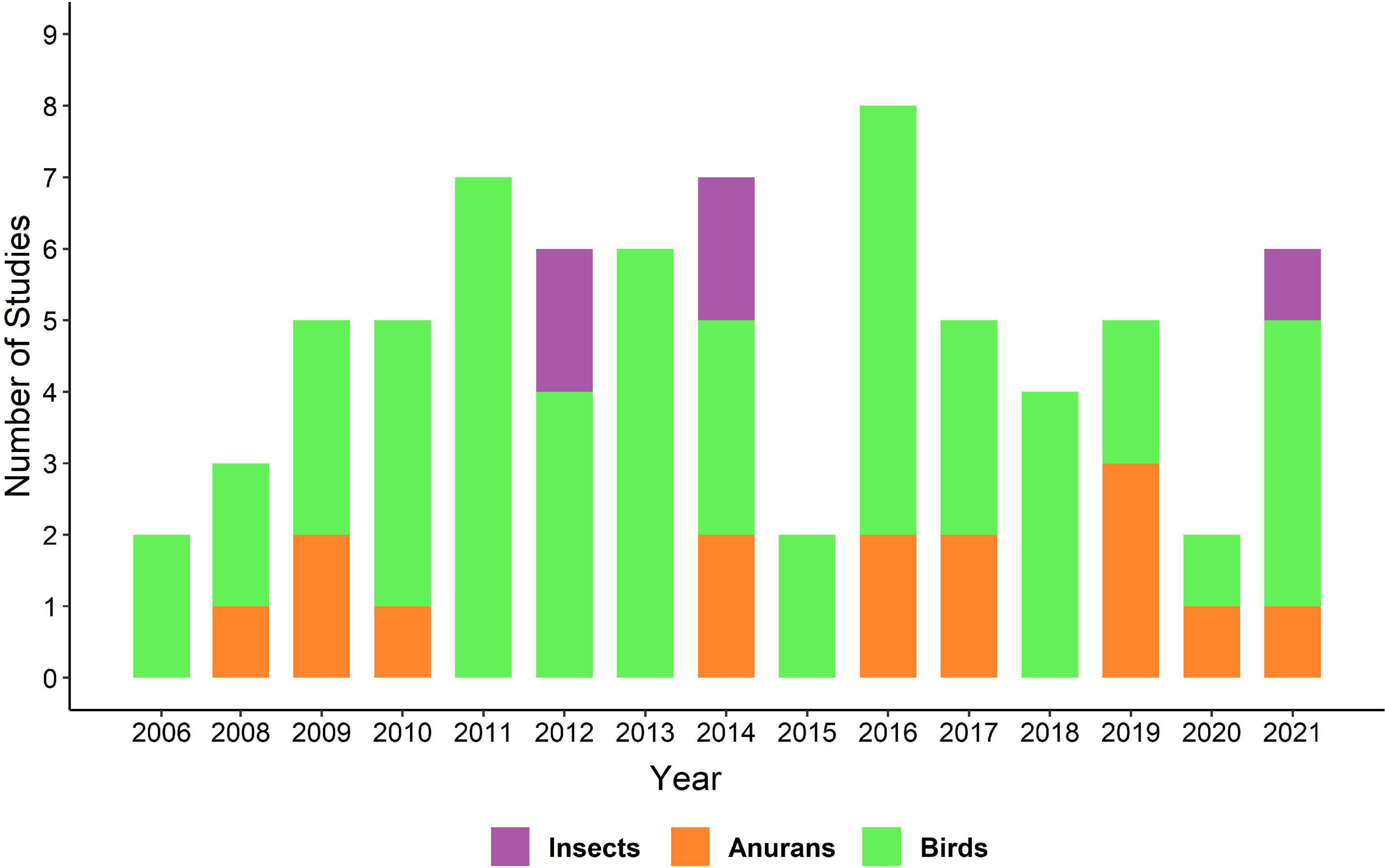
Figure 2. Studies included in the meta-analysis. Number of studies present in our database, separated by year and color-coded taxon.
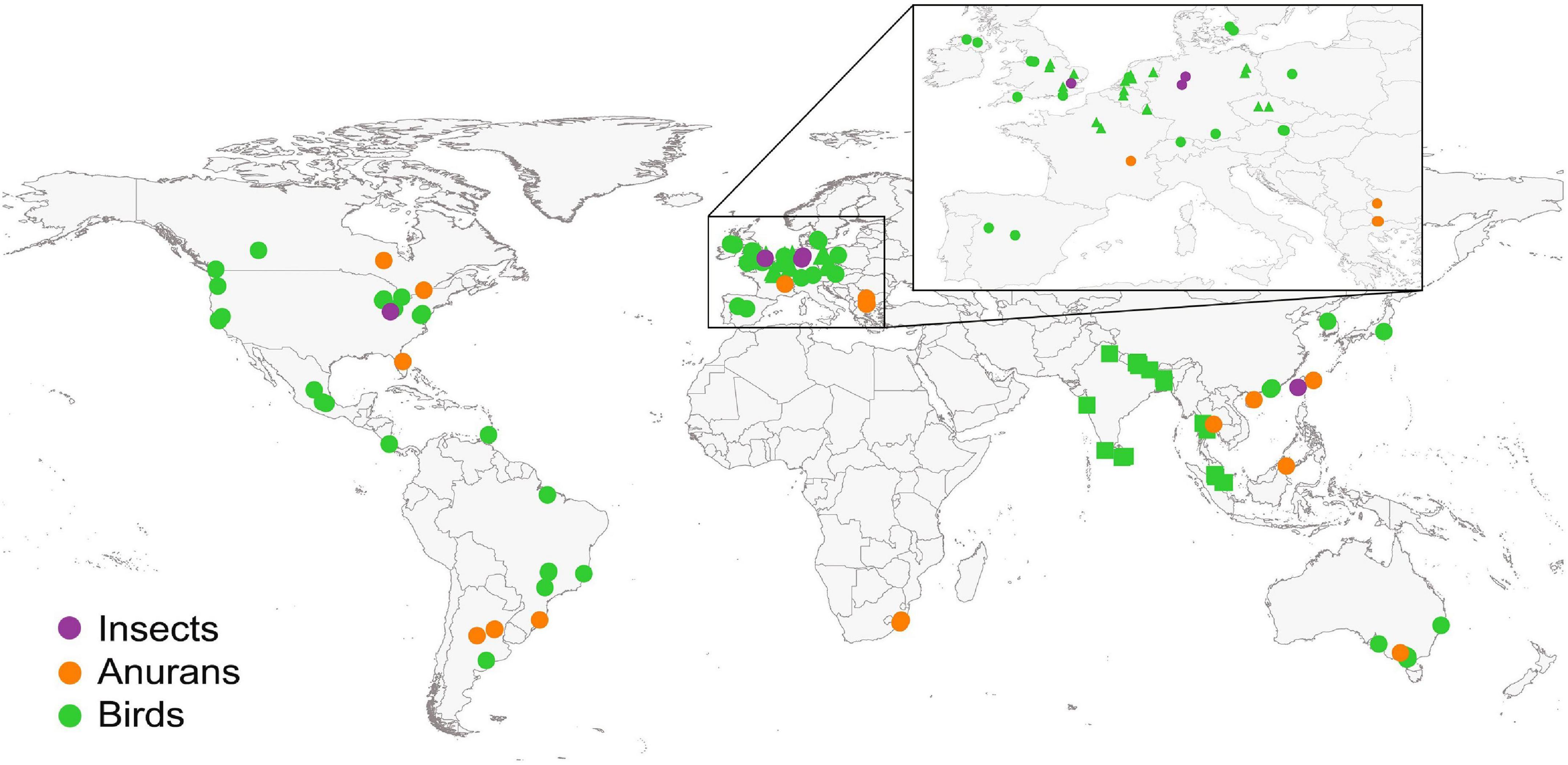
Figure 3. Geographic distribution of studied sites included in the meta-analysis. Color-coded circles represent different taxon, solid circles represent studies that had one or two sampling sites, and each different shape (square and triangle) represent a study that had more than two sampling sites.
For invertebrates, there was no change in the acoustic parameters when exposed to anthropogenic sounds (Table 1 and Figure 4A). Anurans increased their call amplitude due to anthropogenic sounds (Table 1 and Figure 4B). For birds the acoustic parameters dominant frequency, minimum frequency, maximum frequency, note duration and call amplitude increased in sites of anthropogenic noise (Table 1 and Figure 4C).
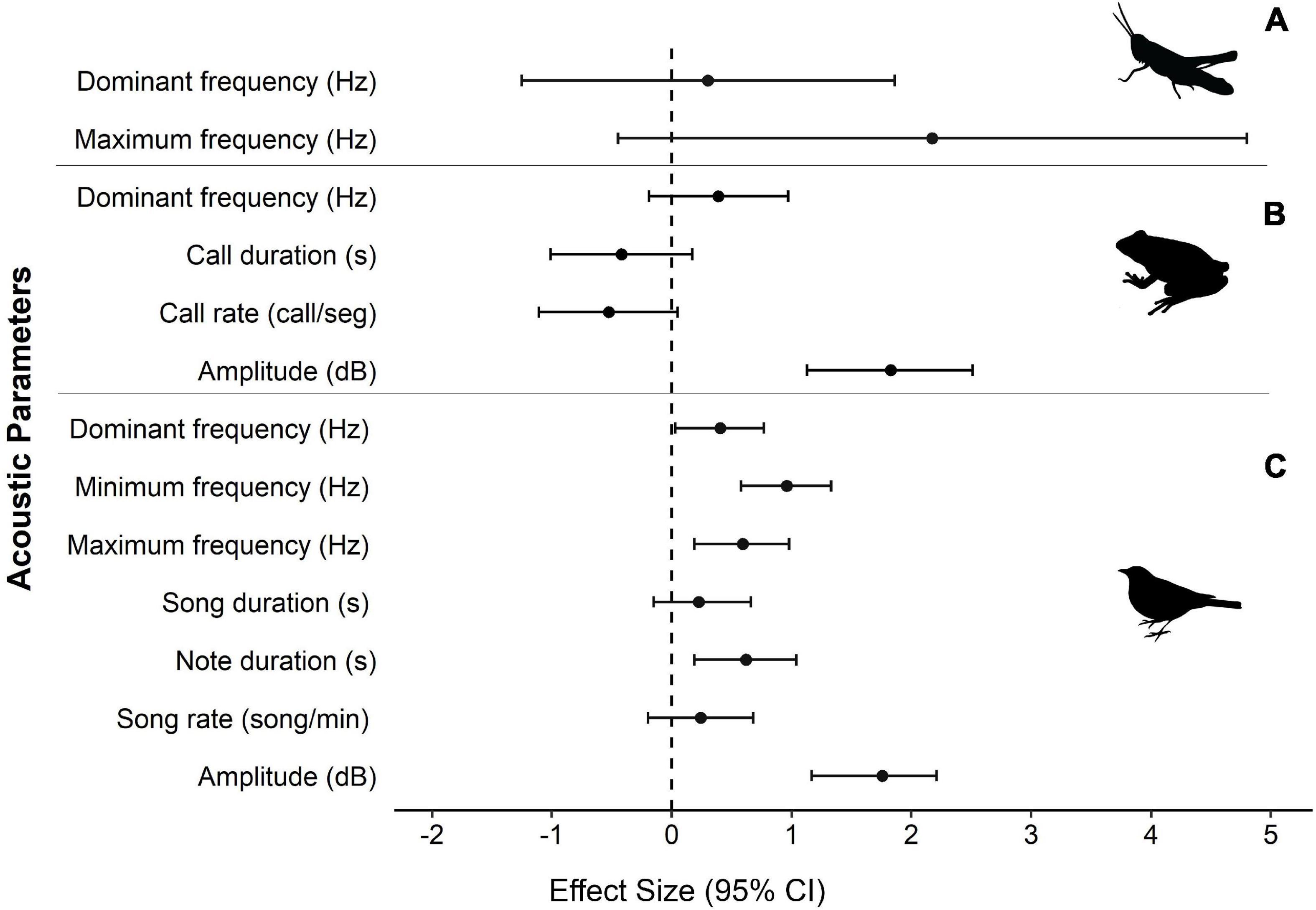
Figure 4. Forest plot representing effect of anthropogenic sounds for acoustic parameters. The mean effect size Hedges’ g (represented by black circles) and confidence interval values of 95% (bars) for each acoustic parameter, separated by taxon, (A) Insects, represented by the orders Orthoptera and Hemiptera, (B) Anurans and (C) Birds.
Discussion
Our study detected a general pattern for some acoustic parameters to change in response to anthropogenic noise, with different results for the three taxa studied. Birds show a general pattern to change several acoustic parameters, such as dominant frequency, minimum and maximum frequencies, note duration and song amplitude. Anurans suggest a general pattern to change only the amplitude of the call, for insects there were no changes in the general pattern for none of the investigated acoustic parameters.
For insects the number of studies that evaluated noise interference for acoustic communication was small, compared to the other groups, and this is reflected in the confidence interval of the results, which showed the need for more studies for this taxon. Despite the small number of studies for the group, we observed a tendency for frequencies to increase in the presence of noise, but our result was not significant. Other strategies can also be used by insects, Duarte et al. (2019) showed that Gryllus sp. reduced its sounds emission by 90% in the presence of truck noise, but it was not possible to test the generality of that acoustic parameter, because this parameter has not been tested in studies that allowed performing a meta-analysis. To decrease vocal activity can be a strategy to avoid masking, as individuals spend a lot of energy to emit an acoustic signal, calling more implies higher energy expenditure (Prestwich and Walker, 1981). However, we do not know how this affects the interactions mediated by acoustic communication.
Anurans increased call amplitude in the presence of noise. Our meta-analysis showed that this was the only acoustic parameter that responded to anthropogenic noise for anurans, showing that anurans can use the strategy of call with high intensity to avoid masking. Increased level of vocalization intensity according to noise, known as the “Lombard effect” (Brumm and Zollinger, 2011), has also been reported in studies with anurans that vocalize next to noisy environments, as streams (Halfwerk et al., 2016; Shen and Xu, 2016), although, some studies did not find evidence for the Lombard effect (Love and Bee, 2010; Zhao et al., 2018). Even though our results suggest the effect of anthropogenic noise on call amplitude, we interpret our results with caution, due to the low number of studies that evaluated this acoustic parameter and the difficulty in measuring the amplitude, which can be influenced by several factors, such as the direction of the signal emitter, among others.
Studies have shown how masking can affect anurans. For example, in several species that vocalize in sites with anthropogenic noise, the frequency of calls has increased (Parris et al., 2009; Cunnington and Fahrig, 2010; Grenat et al., 2019), although this response is not a general pattern for the group, as shown in our meta-analysis. Some studies that did not investigate a change in frequency due to noise, suggest an increase in the call rate, to increase chances of detection (Kaiser and Hammers, 2009; Roca et al., 2016). Our study showed an inverse tendency, with a decrease in the call rate, but the result was not significant, this tendency could be confirmed in the future with the increase in the number of studies.
In anurans, the consequences of changes in acoustic parameters caused by anthropogenic noise can affect sexual selection, as calls are used by females to choose males (Duellman and Trueb, 1985; Wells and Schwartz, 2007). For females of the group the preference for specific frequencies has been reported (Gerhardt, 1987; Ryan et al., 1992; Márquez and Bosch, 1997). In addition, in the presence of chorus and traffic noise, females decrease orientation toward the acoustic signal (Bee and Swanson, 2007). Another feature already shown is that males exposed to noise decrease the number of days present in the chorus and the duration of the chorus (Kaiser et al., 2011). These behavior changes were not investigated in our study, but changes in behavior when exposed to anthropogenic noise are also a mechanism used by anurans.
We suggest that birds showed a general tendency to change a higher number of acoustic parameters to avoid sound masking by anthropogenic noise. These mechanisms can benefit species that are exposed to anthropogenic noise, which is important to highlight this for the group. The number of acoustic parameters tested was greater compared to the other groups. The increasing signal frequencies may be advantageous in anthropogenic noise environment, where it has been shown that high frequencies are easier to detect (Ripmeester et al., 2010b; Pohl et al., 2012). But this may decrease signal efficiency for sexual selection and defense (Halfwerk et al., 2011). Some studies suggest that frequency depends on body size, larger birds produce songs with lower frequencies, so females can use song frequency as an indicator of male size in sexual selection (Ryan and Brenowitz, 1985; Gil and Gahr, 2002), thus, changes in frequencies songs can influence the preference of females. In addition, high frequency sounds may indicate that the transmitter is not hostile (Morton, 1977), this type of information can be used by males who defend territory. Therefore, modifying these signals may decrease communication efficiency, and males may be perceived as less attractive to females or less effective in the defense of territory (Mockford and Marshall, 2009).
Birds also increased note duration, longer duration of song and note can increase the chances of sound detection in the presence of anthropogenic noise. In birds, long song durations have been reported as responses to territory defense (Ripmeester et al., 2010a; Narango and Rodewald, 2016), however, in our study we found a general pattern only for note duration. Our results also suggest that the call amplitude increases in the presence of anthropogenic noise. Similar results have been shown in the literature for biotic noise, where studies have reported that the Lombard effect is a mechanism widely used by birds to avoid sound masking (Lampe et al., 2010; Zollinger and Brumm, 2011; Dorado-Correa et al., 2018; Singh et al., 2019). Zollinger and Brumm (2015) showed that changes in amplitude can have several costs for birds, for example, affecting sexual selection, defense of territory, condition and energy. Modifying the acoustic parameters can generate costs for birds, this should generate a loss/gain relationship, and the emitter should produce the most advantageous signal.
We showed a large difference between the number of studies by groups, the group of insects was the most neglected. These differences made it difficult to investigate a general pattern by taxon and to compare the same parameter in different taxa. Dominant frequency was the only acoustic parameter evaluated in the three groups. Although some studies suggested changes in this signal, change in the dominant frequency was a general pattern only in birds. This result may have occurred because the dominant frequency is considered an acoustic parameter difficult to change in insects and anurans, due to morphological restrictions (Castellano and Giacoma, 1998; Raboin and Elias, 2019). In birds, changes in frequency have been suggested, due to evolutionary and plastic responses to environmental changes (Morton, 1975; Bermúdez-Cuamatzin et al., 2012), and we emphasize that birds have anatomical characteristics that enable them to modulate the frequency and amplitude in vocal production, generating different types of singing (Riede and Goller, 2010; Ladich and Winkler, 2017), which does not occur in the other investigated groups. This is reflected in our results, which showed a general pattern for birds, changing the dominant, minimum and maximum frequencies of the sound. For anurans we tested call duration, in birds we tested song duration and note duration, in both we tested call/song rate, and we found a general pattern only for longer note duration in response to anthropogenic noise in birds. For call amplitude, anurans and birds increased signal amplitude, this was the only common change between both groups, increasing sound intensity due to noise is a feature widely used in vertebrates (Brumm and Zollinger, 2011). Thus, the birds group presented the most general patterns of responses to changes in acoustic parameters. Our meta-analysis showed all taxa either changed some acoustic parameter or showed a tendency to do so in environments with anthropogenic noise.
Conclusion
This work investigated the general patterns of changes in acoustic parameters caused by anthropogenic noise in insects, anurans and birds. Our approach showed that birds was the taxon with the most parameter changes, with dominant frequency, minimum and maximum frequencies, note duration and amplitude song, affected by anthropogenic noise in most studies that tested this effect. For anurans, a general pattern in the studies was an increase amplitude due to anthropogenic noise. Therefore, amplitude was the only parameter that changed in two different taxonomic groups. Additionally, we emphasized that the group of insects had few studies, thus this can influence the lack of a general pattern for the group. Additionally, considering the changes in the acoustic parameters, it is necessary to understand the consequences of these changes for each species. Questions such as the influence of these changes on the interactions mediated by animal acoustic communication need to be investigated deeply. Therefore, we emphasize that understanding these effects can contribute to strategies that minimize the consequences of anthropogenic noise for animals.
Data Availability Statement
The original contributions presented in the study are included in the article/Supplementary Material, further inquiries can be directed to the corresponding author.
Author Contributions
LG and MS contributed to conception, design of the study, and organized the database. LG performed the statistical analysis and wrote the first draft of the manuscript. LG, MS, RS-L, and JB wrote sections of the manuscript. All authors contributed to manuscript revision, read, and approved the submitted version.
Conflict of Interest
The authors declare that the research was conducted in the absence of any commercial or financial relationships that could be construed as a potential conflict of interest.
Publisher’s Note
All claims expressed in this article are solely those of the authors and do not necessarily represent those of their affiliated organizations, or those of the publisher, the editors and the reviewers. Any product that may be evaluated in this article, or claim that may be made by its manufacturer, is not guaranteed or endorsed by the publisher.
Acknowledgments
We are grateful to Marina H. L. Duarte, Lucas R. Forti, and Robert J. Young for their contributions on the manuscript. LG thanks the Bahia State Research Support Foundation (FAPESB) for scholarships. RS-L thanks the Brazilian Council for Scientific and Technological Development (CNPq-Brazil) for her research fellowship (process number 312763/2019-0). MS also thanks CNPq (processes 304999/2015-6 and 309365/2019-8) for research grants. This study was financed in part by Coordenação de Aperfeiçoamento de Pessoal de Nível Superior - Brasil (CAPES) - Finance Code 001.
Supplementary Material
The Supplementary Material for this article can be found online at: https://www.frontiersin.org/articles/10.3389/fevo.2022.827440/full#supplementary-material
References
Bee, M. A., and Swanson, E. M. (2007). Auditory masking of anuran advertisement calls by road traffic noise. Anim. Behav. 74, 1765–1776. doi: 10.1016/j.anbehav.2007.03.019
Beecher, M. D. (1988). Spectrographic analysis of animal vocalizations: implications of the “uncertainty principle.”. Bioacoustics 1, 187–208. doi: 10.1080/09524622.1988.9753091
Benítez-López, A., Alkemade, R., and Verweij, P. A. (2010). The impacts of roads and other infrastructure on mammal and bird populations: a meta-analysis. Biol. Conserv. 143, 1307–1316. doi: 10.1016/j.biocon.2010.02.009
Bermúdez-Cuamatzin, E., Ríos-Chelén, A. A., Gil, D., and Garcia, C. M. (2012). Experimental evidence for real-time song frequency shift in response to urban noise in a passerine bird. Biol. Lett. 8:320. doi: 10.1098/rsbl.2011.1225
Brumm, H., and Slabbekoorn, H. (2005). Acoustic communication in noise. Adv. Study Behav. 35, 151–209. doi: 10.1016/S0065-3454(05)35004-2
Brumm, H., and Zollinger, S. A. (2011). The evolution of the lombard effect: 100 years of psychoacoustic research. Behaviour 148, 1173–1198. doi: 10.1163/000579511X605759
Brumm, H., Zollinger, S. A., Niemelä, P. T., and Sprau, P. (2017). Measurement artefacts lead to false positives in the study of birdsong in noise. Methods Ecol. Evol. 8, 1617–1625. doi: 10.1111/2041-210X.12766
Castellano, S., and Giacoma, C. (1998). Stabilizing and directional female choice for male calls in the European green toad. Anim. Behav. 56, 275–287. doi: 10.1006/anbe.1998.0784
Cunnington, G. M., and Fahrig, L. (2010). Plasticity in the vocalizations of anurans in response to traffic noise. Acta Oecol. 36, 463–470. doi: 10.1016/j.actao.2010.06.002
Dominoni, D. M., Greif, S., Nemeth, E., and Brumm, H. (2016). Airport noise predicts song timing of European birds. Ecol. Evol. 6, 6151–6159. doi: 10.1002/ece3.2357
Dorado-Correa, A. M., Zollinger, S. A., and Brumm, H. (2018). Vocal plasticity in mallards: multiple signal changes in noise and the evolution of the Lombard effect in birds. J. Avian Biol 49:jav–01564. doi: 10.1111/jav.01564
Duarte, C. M., Chapuis, L., Collin, S. P., Costa, D. P., Devassy, R. P., Eguiluz, V. M., et al. (2021). The soundscape of the anthropocene ocean. Science 371, eaba4658. doi: 10.1126/science.aba4658
Duarte, M. H. L., Caliari, E. P., Scarpelli, M. D. A., Lobregat, G. O., Young, R. J., and Sousa-Lima, R. S. (2019). Effects of mining truck traffic on cricket calling activity. J. Acoust. Soc. Am. 146, 656–664. doi: 10.1121/1.5119125
Dubois, A., and Martens, J. (1984). A case of possible vocal convergence between frogs and a bird in Himalayan torrents. J. Ornithol 125, 455–463. doi: 10.1007/bf01640137
Duellman, W. E., and Trueb, L. (1985). Biology of Amphibians. Baltimore, MD: Johns Hopkins University Press.
Egger, M., Smith, G. D., Schneider, M., and Minder, C. (1997). Bias in meta-analysis detected by a simple, graphical test. Br. Med. J. 315, 629–634.
Francis, C. D., Ortega, C. P., and Cruz, A. (2009). Noise pollution changes avian communities and species interactions. Curr. Biol. 19, 1415–1419. doi: 10.1016/j.cub.2009.06.052
Gerhardt, H. C. (1987). Evolutionary and neurobiological implications of selective phonotaxis in the green treefrog, Hyla cinerea. Anim. Behav. 35, 581–595.
Gerhardt, H. C., and Huber, F. (2002). Acoustic Communication in Insects and Anurans: Common Problems and Diverse Solutions. Chicago, IL: The University of Chicago. doi: 10.1643/ot-03-039
Get Data Graph Digitizer (2021). Available online at: www.getdata-graph-digitizer.com. (accessed July, 2021).
Gil, D., and Gahr, M. (2002). The honesty of bird song: multiple constraints for multiple traits. Trends Ecol. Evol. 17, 133–141. doi: 10.1016/S0169-5347(02)02410-2
Goutte, S., Dubois, A., Howard, S. D., Marquez, R., Rowley, J. J. L., Dehling, J. M., et al. (2016). Environmental constraints and call evolution in torrent-dwelling frogs. Evolution 70, 811–826. doi: 10.1111/evo.12903
Grenat, P. R., Pollo, F. E., Ferrero, M. A., and Martino, A. L. (2019). Differential and additive effects of natural biotic and anthropogenic noise on call properties of Odontophrynus americanus (Anura, Odontophryinidae): implications for the conservation of anurans inhabiting noisy environments. Ecol. Indic. 99, 67–73. doi: 10.1016/j.ecolind.2018.12.014
Gross, K., Pasinelli, G., and Kunc, H. P. (2010). Behavioral plasticity allows short-term adjustment to a novel environment. Am. Nat. 176, 456–464. doi: 10.1086/655428
Halfwerk, W., Bot, S., Buikx, J., van der Velde, M., Komdeur, J., ten Cate, C., et al. (2011). Low-frequency songs lose their potency in noisy urban conditions. Proc. Natl. Acad. Sci. U.S.A. 108, 14549–14554. doi: 10.1073/pnas.1109091108
Halfwerk, W., Lea, A. M., Guerra, M. A., Page, R. A., and Ryan, M. J. (2016). Vocal responses to noise reveal the presence of the Lombard effect in a frog. Behav. Ecol. 27, 669–676. doi: 10.1093/beheco/arv204
Harding, H. R., Gordon, T. A. C., Eastcott, E., Simpson, S. D., and Radford, A. N. (2019). Causes and consequences of intraspecific variation in animal responses to anthropogenic noise. Behav. Ecol. 30, 1501–1511. doi: 10.1093/beheco/arz114
Higgins, J. P. T., Thompson, S. G., Deeks, J. J., and Altman, D. G. (2003). Measuring inconsistency in meta-analyses. BMJ 327, 557–560. doi: 10.1136/bmj.327.7414.557
Hozo, S. P., Djulbegovic, B., and Hozo, I. (2005). Estimating the mean and variance from the median, range, and the size of a sample. BMC Med. Res. Methodol. 5:13. doi: 10.1186/1471-2288-5-13
Hu, Y., and Cardoso, G. C. (2010). Which birds adjust the frequency of vocalizations in urban noise? Anim. Behav. 79, 863–867. doi: 10.1016/j.anbehav.2009.12.036
Job, J. R., Kohler, S. L., and Gill, S. A. (2016). Song adjustments by an open habitat bird to anthropogenic noise, urban structure, and vegetation. Behav. Ecol. 27:arw105. doi: 10.1093/beheco/arw105
Kaiser, K., and Hammers, J. L. (2009). The effect of anthropogenic noise on male advertisement call rate in the neotropical treefrog, Dendropsophus triangulum. Behaviour 146, 1053–1069.
Kaiser, K., Scofield, D. G., Alloush, M., Jones, R. M., Marczak, S., Martineau, K., et al. (2011). When sounds collide: the effect of anthropogenic noise on a breeding assemblage of frogs in Belize, Central America. Behaviour 148, 215–232. doi: 10.1163/000579510X551660
Kunc, H. P., and Schmidt, R. (2021). Species sensitivities to a global pollutant: a meta-analysis on acoustic signals in response to anthropogenic noise. Glob. Chang. Biol. 27, 675–688. doi: 10.1111/gcb.15428
Ladich, F., and Winkler, H. (2017). Acoustic communication in terrestrial and aquatic vertebrates. J. Exp. Biol. 220, 2306–2317. doi: 10.1242/jeb.132944
Lampe, H. M., Balsby, T. J. S., Espmark, Y. O., and Dabelsteen, T. (2010). Does twitter song amplitude signal male arousal in redwings (Turdus iliacus)? Behaviour 147, 353–365. doi: 10.1163/000579509X12574305163567
Lampe, U., Reinhold, K., and Schmoll, T. (2014). How grasshoppers respond to road noise: developmental plasticity and population differentiation in acoustic signalling. Funct. Ecol. 28, 660–668. doi: 10.1111/1365-2435.12215
Lampe, U., Schmoll, T., Franzke, A., and Reinhold, K. (2012). Staying tuned: grasshoppers from noisy roadside habitats produce courtship signals with elevated frequency components. Funct. Ecol. 26, 1348–1354. doi: 10.1111/1365-2435.12000
Love, E. K., and Bee, M. A. (2010). An experimental test of noise-dependent voice amplitude regulation in Cope’s grey treefrog, Hyla chrysoscelis. Anim. Behav. 80, 509–515. doi: 10.1016/j.anbehav.2010.05.031
Lüdecke, D. (2019). ESC: Effect Size Computation for Meta Analysis (Version 0.5.0). Available online at: https://CRAN.R-project.org/package=esc (accessed 04 12, 2019)
Márquez, R., and Bosch, J. (1997). Male advertisement call and female preference in sympatric and allopatric midwife toads. Anim. Behav. 54, 1333–1345. doi: 10.1016/j.anbehav.2007.12.013
McCarthy, A. H., Potvin, D. A., Aslam, T., Bartlett, R., Beebe, S., Bennett, J., et al. (2013). Differences between the songs of rural and urban Australian magpies (Gymnorhina tibicen) and the potential consequences for territorial interactions. Notornis 60, 143–150.
McClure, C. J. W., Ware, H. E., Carlisle, J., Kaltenecker, G., and Barber, J. R. (2013). An experimental investigation into the effects of traffic noise on distributions of birds: avoiding the phantom road. Proc. R. Soc. B Biol. Sci. 280:20132290. doi: 10.1098/rspb.2013.2290
Michonneau, F., Brown, J. W., and Winter, D. J. (2016). rotl: an R package to interact with the Open Tree of Life data. Methods Ecol. Evol. 7, 1476–1481. doi: 10.1111/2041-210X.12593
Mockford, E. J., and Marshall, R. C. (2009). Effects of urban noise on song and response behaviour in great tits. Proc. R. Soc. B Biol. Sci. 276, 2979–2985. doi: 10.1098/rspb.2009.0586
Montague, M. J., Danek-Gontard, M., and Kunc, H. P. (2013). Phenotypic plasticity affects the response of a sexually selected trait to anthropogenic noise. Behav. Ecol. 24, 342–348. doi: 10.1093/beheco/ars169
Morton, E. S. (1975). Ecological sources of selection on avian sounds. Am. Nat. 109, 17–34. doi: 10.1016/j.heares.2015.05.009
Morton, E. S. (1977). On the occurrence and significance of motivation-structural rules in some bird and mammal sounds. Am. Nat. 111:855. doi: 10.1086/283219
Nakagawa, S., Noble, D. W. A., Senior, A. M., and Lagisz, M. (2017). Meta-evaluation of meta-analysis: ten appraisal questions for biologists. BMC Biol. 15:18. doi: 10.1186/s12915-017-0357-7
Nakagawa, S., and Santos, E. S. A. (2012). Methodological issues and advances in biological meta-analysis. Evol. Ecol. 26, 1253–1274. doi: 10.1007/s10682-012-9555-5
Narango, D. L., and Rodewald, A. D. (2016). Urban-associated drivers of song variation along a rural–urban gradient. Behav. Ecol. 27, 608–616. doi: 10.1093/beheco/arv197
Nemeth, E., and Brumm, H. (2009). Blackbirds sing higher-pitched songs in cities: adaptation to habitat acoustics or side-effect of urbanization? Anim. Behav. 78, 637–641. doi: 10.1016/j.anbehav.2009.06.016
Page, M. J., McKenzie, J. E., Bossuyt, P. M., Boutron, I., Hoffmann, T. C., Mulrow, C. D., et al. (2021). The PRISMA 2020 statement: an updated guideline for reporting systematic reviews. J. Clin. Epidemiol. 134, 178–189. doi: 10.1016/j.jclinepi.2021.03.001
Paradis, E., and Schliep, K. (2019). ape 5.0: an environment for modern phylogenetics and evolutionary analyses in R. Bioinformatics 35, 526–528. doi: 10.1093/bioinformatics/bty633
Parris, K. M., Velik-Lord, M., and North, J. M. A. (2009). Frogs call at a higher pitch in traffic noise. Ecol. Soc. 14:25.
Patricelli, G. L., and Blickley, J. L. (2006). Avian communication in urban noise: causes and consequences of vocal adjustment. Auk 123, 639–649. doi: 10.1642/0004-80382006123
Patterson, R. D., and Green, D. M. (1978). “Auditory masking,” in Hearing: Handbook of Perception, eds E. C. Carterette and M. P. Friedman (New York, NY: Academic Press), 337–361. doi: 10.1016/B978-0-12-161904-6.50016-6
Phillips, J. N., Ruef, S. K., Garvin, C. M., Le, M. L. T., and Francis, C. D. (2019). Background noise disrupts host–parasitoid interactions. R. Soc. Open Sci 6:190867. doi: 10.1098/rsos.190867
Piersma, T., and Drent, J. (2003). Phenotypic flexibility and the evolution of organismal design. Trends Ecol. Evol. 18, 228–233. doi: 10.1016/S0169-5347(03)00036-3
Pohl, N. U., Leadbeater, E., Slabbekoorn, H., Klump, G. M., and Langemann, U. (2012). Great tits in urban noise benefit from high frequencies in song detection and discrimination. Anim. Behav. 83, 711–721. doi: 10.1016/j.anbehav.2011.12.019
Prestwich, K. N., and Walker, T. J. (1981). Energetics of singing in crickets: effect of temperature in three trilling species (Orthoptera: Gryllidae). J. Comp. Physiol. 143, 199–212. doi: 10.1007/BF00797699
R Core Team (2021). R: A Language and Environment for Statistical Computing. Vienna: R Foundation for Statistical Computing.
Raboin, M., and Elias, D. O. (2019). Anthropogenic noise and the bioacoustics of terrestrial invertebrates. J. Exp. Biol. 222:jeb178749. doi: 10.1242/jeb.178749
Riede, T., and Goller, F. (2010). Peripheral mechanisms for vocal production in birds – differences and similarities to human speech and singing. Brain Lang. 115, 69–80. doi: 10.1016/j.bandl.2009.11.003
Ripmeester, E. A. P., Mulder, M., and Slabbekoorn, H. (2010b). Habitat-dependent acoustic divergence affects playback response in urban and forest populations of the European blackbird. Behav. Ecol. 21, 876–883. doi: 10.1093/beheco/arq075
Ripmeester, E. A. P., Kok, J. S., van Rijssel, J. C., and Slabbekoorn, H. (2010a). Habitat-related birdsong divergence: a multi-level study on the influence of territory density and ambient noise in European blackbirds. Behav. Ecol. Sociobiol. 64, 409–418. doi: 10.1007/s00265-009-0857-8
Roca, I. T., Desrochers, L., Giacomazzo, M., Bertolo, A., Bolduc, P., Deschesnes, R., et al. (2016). Shifting song frequencies in response to anthropogenic noise: a meta-analysis on birds and Anurans. Behav. Ecol. 27, 1269–1274. doi: 10.1093/beheco/arw060
Röhr, D. L., Paterno, G. B., Camurugi, F., Juncá, F. A., and Garda, A. A. (2016). Background noise as a selective pressure: stream-breeding anurans call at higher frequencies. Org. Divers. Evol. 16, 269–273. doi: 10.1007/s13127-015-0256-0
Ryan, M. J., and Brenowitz, E. A. (1985). The role of body size, phylogeny, and ambient noise in the evolution of bird song. Am. Nat. 126, 87–100. doi: 10.1086/284398
Ryan, M. J., and Kime, N. M. (2003). “Selection on long distance acoustic signals,” in Acoustic Communication, eds A. M. Simmons, R. R. Fay, and A. N. Popper (Berlin: Springer-Verlag), 225–274. doi: 10.1007/0-387-22762-8_5
Ryan, M. J., Perrill, S. A., and Wilczynski, W. (1992). Auditory tuning and call frequency predict population-based mating preferences in the cricket frog, Acris crepitans. Am. Nat. 139, 1370–1383. doi: 10.1086/285391
Shannon, G., McKenna, M. F., Angeloni, L. M., Crooks, K. R., Fristrup, K. M., Brown, E., et al. (2016). A synthesis of two decades of research documenting the effects of noise on wildlife. Biol. Rev. 91, 982–1005. doi: 10.1111/brv.12207
Shen, J.-X., and Xu, Z.-M. (2016). The Lombard effect in male ultrasonic frogs: regulating antiphonal signal frequency and amplitude in noise. Sci. Rep. 6:27103. doi: 10.1038/srep27103
Singh, M., Jaiswal, A., Ulman, Y., and Kumar, K. (2019). Vocal adjustments in purple sunbird (Cinnyris asiaticus) at noisy habitats. Acta Acust. U.Acust. 105, 294–300. doi: 10.3813/AAA.919312
Slabbekoorn, H., and Ripmeester, E. A. P. (2008). Birdsong and anthropogenic noise: implications and applications for conservation. Mol. Ecol. 17, 72–83. doi: 10.1111/j.1365-294X.2007.03487.x
Sousa-Lima, R. S., and Clark, C. W. (2008). Modeling the effect of boat traffic on the fluctuation of humpback whale singing activity in the Abrolhos National Marine Park, Brazil. Can. Acoust. Acoust. Can. 36, 174–181.
Sterne, J. A. C., and Egger, M. (2006). “Regression methods to detect publication and other bias in meta-analysis,” in Publication Bias in Meta–Analysis: Prevention, Assessment and Adjustments, eds H. R. Rothstein, A. J. Sutton, and M. Borenstein (Hoboken, NJ: Wiley), 99–110. doi: 10.1002/0470870168.ch6
Tennessen, J. B., Parks, S. E., and Langkilde, T. L. (2016). “Anthropogenic noise and physiological stress in wildlife,” in Advances in Experimental Medicine and Biology, eds A. N. Popper and A. Hawkins (New York, NY: Advances in Experimental Medicine and Biology), 1145–1148. doi: 10.1007/978-1-4939-2981-8_142
Tree of Life Web Project (2007). Available online at: http://tolweb.org (accessed September, 2021).
Viechtbauer, W. (2010). Conducting meta-analyses in R with the metafor. J. Stat. Softw. 36, 1–48. doi: 10.18637/jss.v036.i03
Viechtbauer, W., and Cheung, M. W.-L. (2010). Outlier and influence diagnostics for meta-analysis. Res. Synth. Methods 1, 112–125. doi: 10.1002/jrsm.11
Wells, K. D., and Schwartz, J. J. (2007). “The behavioral ecology of anuran communication,” in Hearing and Sound Communication in Amphibians, eds P. M. Narins, A. S. Feng, R. R. Fay, and A. N. Popper (New York: Springer), 44–86. doi: 10.1007/978-0-387-47796-1_3
Wiley, R. H. (2006). Signal detection and animal communication. Adv. Study Behav. 36, 217–247. doi: 10.1016/S0065-3454(06)36005-6
Wiley, R. H., and Richards, D. G. (1978). Physical constraints on acoustic communication in the atmosphere: implications for the evolution of animal vocalizations. Behav. Ecol. Sociobiol. 3, 69–94.
Zhao, L., Sun, X., Chen, Q., Yang, Y., Wang, J., Ran, J., et al. (2018). Males increase call frequency, not intensity, in response to noise, revealing no Lombard effect in the little torrent frog. Ecol. Evol. 8, 11733–11741. doi: 10.1002/ece3.4625
Zollinger, S. A., and Brumm, H. (2011). The lombard effect. Curr. Biol. 21, 614–615. doi: 10.1016/j.cub.2011.06.003
Zollinger, S. A., and Brumm, H. (2015). Why birds sing loud songs and why they sometimes don’t. Anim. Behav. 105, 289–295. doi: 10.1016/j.anbehav.2015.03.030
Keywords: sound production, auditory masking, animal communication, vocal adjustments, plasticity, anthropophony, terrestrial fauna
Citation: Gomes L, Solé M, Sousa-Lima RS and Baumgarten JE (2022) Influence of Anthropogenic Sounds on Insect, Anuran and Bird Acoustic Signals: A Meta-Analysis. Front. Ecol. Evol. 10:827440. doi: 10.3389/fevo.2022.827440
Received: 02 December 2021; Accepted: 09 February 2022;
Published: 28 February 2022.
Edited by:
Simon Ducatez, University of Cambridge, United KingdomReviewed by:
Gabriel Francescoli, Universidad de la República, UruguayYossi Yovel, Tel Aviv University, Israel
Copyright © 2022 Gomes, Solé, Sousa-Lima and Baumgarten. This is an open-access article distributed under the terms of the Creative Commons Attribution License (CC BY). The use, distribution or reproduction in other forums is permitted, provided the original author(s) and the copyright owner(s) are credited and that the original publication in this journal is cited, in accordance with accepted academic practice. No use, distribution or reproduction is permitted which does not comply with these terms.
*Correspondence: Lidiane Gomes, ZGlhbmUuZ29tZXNAeWFob28uY29tLmJy