- 1Department of Evolutionary and Environmental Biology, Institute of Evolution, University of Haifa, Haifa, Israel
- 2Department of Entomology and Wildlife Ecology, University of Delaware, Newark, DE, United States
Stopping-over is critical for migrating birds. Yet, our knowledge of bird stopover distributions and their mechanisms near wide ecological barriers is limited. Using low elevation scans of three weather radars covering 81,343 km2, we quantified large-scale bird departure patterns during spring and autumn (2014–2018) in between two major ecological barriers, the Sahara Desert and Mediterranean Sea. Boosted Regression Tree models revealed that bird distributions differed between the seasons, with higher densities in the desert and its edge, as well as inland from the sea, during spring and a predominantly coastal distribution in the autumn. Bird distributions were primarily associated with broad-scale geographic and anthropogenic factors rather than individual fine-scale habitat types. Notably, artificial light at night strongly correlated with high densities of migrants, especially in the autumn. Autumn migrants also selected sites located close to water sources. Our findings substantially advance the understanding of bird migration ecology near ecological barriers and facilitate informed conservation efforts in a highly populated region by identifying a few high-priority stopover areas of migrating birds.
Introduction
Migratory birds have been found to steeply decline in recent decades (Berthold et al., 1998; Sokolov et al., 2001; Sanderson et al., 2006; Rosenberg et al., 2019). Research and conservation efforts have mostly focused on protecting breeding habitats or wintering grounds and only rarely on stopover habitats used by birds during migration (Hutto, 2000; Marra et al., 2015; Becciu et al., 2019) although migration is the period when the risk of mortality is the highest (Sillett and Holmes, 2002; Newton, 2006). Since most of the migration time is spent on refueling and resting rather than in active migratory flight (Alerstam, 2003; Wikelski et al., 2003), the ability of the birds to accomplish the journey, as well as their survival and breeding output following migration, depend on the availability and the quality of stopover sites (Moore et al., 1995; Calvert et al., 2009; McGowan et al., 2011).
Stopover areas adjacent to ecological barriers are critically important for the migrants that have just completed or are preparing for a cross-barrier flight (Petit, 2000). These areas are usually characterized by high densities of migrants that may compete for limited resources (Moore and Yong, 1991; Petit, 2000). Most of the birds that migrate between Europe and sub-Saharan Africa face two wide ecological barriers: the Mediterranean Sea (about 550 km wide, but its width varies substantially between different areas) and the Saharo-Arabian desert belt (about 2000 km wide) which they need to cross or detour twice a year. Israel is located at the edges of these two barriers. The Negev Desert in the south of Israel is located at the northern part of the Saharo-Arabian desert belt and an approximately 200 km long north-south coastline in the west of the country borders the eastern part of the Mediterranean Sea. Although bird migration occurs across the whole width of the Mediterranean Sea, densities are highest at its western and eastern edges, where sea-crossing is short or avoided through flight over land bridges (Bruderer and Liechti, 1999; Newton, 2008), resulting in high densities and diversity of birds migrating throughout Israel (Shirihai, 1996). Understanding how birds negotiate these ecological barriers is important for our understanding of migration biology and for the conservation of stopover sites used by many bird species during migration before and after barrier crossing.
The choice of a stopover site might be affected by various factors at different spatial scales (Moore et al., 2005; Chernetsov, 2006). Large-scale geographic factors extrinsic to the habitat patch include the distance of the site from seas or rivers, the site’s latitude, longitude and altitude, as well as various climatic parameters. Local-scale factors intrinsic to the habitat patch may include land use, vegetation indices and other habitat features (Hutto, 1985; Moore and Aborn, 2000). While intrinsic factors that determine the quality of the habitat may be important and could be critical near ecological barriers where site quality may affect bird survival and physiological conditions, the extent to which habitat properties influence the spatial distribution of the birds and their stopover site use is unclear (Buler and Moore, 2011). Studies of stopover habitat use, specifically in the Middle East region, typically focused on local-scale analysis at single or several isolated sites (Simons et al., 2000; Shochat et al., 2002; Sapir et al., 2004; Domer et al., 2018), as methodological limitations did not permit studying large-scale distribution patterns of migratory birds, as has been done using weather radars in North America (Buler et al., 2007; Buler and Dawson, 2014; Sieges et al., 2014; Lafleur et al., 2016; Cohen et al., 2021). The present study is the first to use low elevation scans of weather radars outside North America to study, at a national scale, the spatial distributions of migrating birds that depart for migration from stopover sites. Shedding light on the factors that affect bird distributions among stopover areas near large ecological barriers is fundamental for our understanding of stopover site selection mechanisms and is urgent for the conservation of important areas that support large populations of migratory birds from diverse species.
The Mediterranean Sea is located in-between the breeding and the over-wintering areas of many Palearctic-Afrotropical migratory birds. When migrating birds encounter large water bodies such as the Mediterranean Sea, three possible responses may take place: direct crossing of the sea (Bruderer and Liechti, 1998; Bradley et al., 2014; Deppe et al., 2015; Horton et al., 2019; Ferretti et al., 2021), bypassing the sea by following the coast (Bruderer and Liechti, 1998; Bradley et al., 2014; Gesicki et al., 2019), or interrupting the sea crossing by starting to fly across the sea and at some point along the way changing their flight course toward the shore (Alerstam and Pettersson, 1977; Alfiya, 1995; Fortin et al., 1999). Many of the Palearctic migrants that will choose either of the last two options when reaching the eastern part of the Mediterranean Sea will encounter the Mediterranean coast of Israel on their route. Importantly, in Israel, like in many other areas in the world, human population density in coastal areas is very high (Kummu et al., 2016) with a density of about 3000 people per km2 (Central Bureau of Statistics, 2018). Coastal areas are the most populated in the country, and more than 50% of the population of Israel lives within about 20 km of the Mediterranean coast. Consequently, this region is characterized by high anthropogenic development that includes widespread urbanization and intense agriculture, as well as fragmentation and degradation of natural habitats.
The Saharo-Arabian desert belt has little or no food resources for Palearctic migrating birds that have to cross it in order to reach their over-wintering grounds, often under challenging weather (Moreau, 1961; Schmaljohann et al., 2007). These harsh conditions affect the survival of the birds and, especially in the case of juveniles, the mortality associated with crossing the Sahara can be as high as half the total annual mortality (Strandberg et al., 2010). Therefore, it is important to understand the factors affecting spatial stopover site distributions of migrants that just crossed or are about the cross this wide desert. Due to the tendency of en route migrants to stop-over at the edges of wide ecological barriers, including near the shore (Moore et al., 1990; Lafleur et al., 2016) and at the margins of deserts (Fry et al., 1970; Frannsson et al., 2006), analyzing what affects their spatial distribution in these areas is crucial for understanding fundamental habitat use relationships during migration, their potential fitness implications, and, consequently how they may influence migrants conservation.
The use of weather surveillance radars is the only method allowing broad-scale investigation of stopover site use, including characterizing the factors responsible for migrant spatial distribution. So far, such analyses were made only in North America (Buler et al., 2007; Buler and Dawson, 2014; Sieges et al., 2014; Lafleur et al., 2016; Cohen et al., 2021) and the present study is the first of its kind outside North America to use weather surveillance radars over large spatial scales. Furthermore, this work is pivotal in addressing major knowledge gaps regarding broad, nation-wide stopover distributions within unique geographic settings that include two wide ecological barriers. We quantify migrant spatial distributions using three radars at the finest spatial resolution attempted to date and explore both spring and autumn migrations. We cover large parts of Israel, which is characterized by a wide range of climatic conditions and ecoregions, including Mediterranean-type scrub, Mediterranean Sea coast, semi-arid steppe and harsh desert, over a relatively small area (∼22,000 km2). We modeled the variability in bird densities in relation to 30 different covariates including geographic and weather conditions (i.e., factors extrinsic to the habitat patch), as well as site properties such as vegetation type and land use (i.e., intrinsic to the habitat patch). Over recent years, studies from North America suggested that attraction of birds to Artificial Light At Night (ALAN) cause high bird mortality due to collision with man-made structures and dramatically alters stopover use patterns of nocturnal bird migrants (Van Doren et al., 2017; McLaren et al., 2018). Yet, to the best of our knowledge, no other studies from elsewhere in the world were undertaken to explore this serious anthropogenic factor. We consequently examined if, and in what ways, ALAN may influence spatial distributions of migrating birds through Israel.
Our goal was to reveal the factors affecting the distribution of migrating birds at stopover sites located next to wide ecological barriers which the birds encounter in both migration seasons when crossing Israel. Similar to Buler and Moore (2011) in the case of crossing the Gulf of Mexico, we expect that extrinsic factors will have a large impact on bird distribution patterns in both migration seasons as a result of the response of birds to large-scale changes in environmental conditions when they are found near the Saharo-Arabian desert belt and the Mediterranean Sea. We consequently expect to find high densities of birds at the edges of the barriers immediately following their crossing (Petit, 2000). Also, since precipitation is rare during bird migration seasons in Israel, we predict that bird spatial densities will be affected by the attraction of birds to various fresh water resources. Following findings from studies in North America (Van Doren et al., 2017; McLaren et al., 2018), we additionally expect that ALAN will affect bird distribution such that the migrants will broadly concentrate near sources of artificial light at night in both seasons. We also expect a greater impact of the intrinsic habitat factors during the autumn when the birds prepare for crossing the desert. This is because the selection of stopover sites is presumably based on food availability to maximize lipid deposition rates and thereby migration speed (Lindström et al., 2019). This is in contrast to spring when birds do not face major barriers after crossing Israel and thus do not need to accumulate high lipid stores. The results of these analyses can be used to facilitate data-driven conservation decisions to help protect migrating birds and their stopover sites.
Materials and Methods
Mapping Landbird Stopover Locations Using Weather Surveillance Radar Observations
To understand the spatio-temporal variability of migratory birds in Israel, we used the measured reflectivity factor (a relative measure of bird density) from three weather radars to map the onset of birds departing from their stopover locations. We obtained raw data from a radar station operated by the Israel Meteorological Service (IMS) at Bet Dagan (32.007°N, 34.814°E) in Central Israel, and from two stations operated by the meteorology unit of the Israeli Airforce at Meron (MER; 32.996°N, 35.416°E) and Ramon (RAM; 30.652°N, 34.780°E) in the north and south of the country, respectively. Due to the proximity of these latter two stations to international borders, MER radar also covered some areas in Lebanon and Syria while the range of RAM radar also included parts of the Egyptian Sinai Peninsula (Figure 1). Radar data were collected during several spring and autumn migration seasons between the years 2014–2018, with the exception of autumn data from 2016 and 2017 that were missing from the MER and RAM archive. Additionally, the RAM data in years prior to 2017 were too sparse to interpret bird movement. We were consequently able to use data from five springs and five autumns for IMS, five springs and three autumns for MER, and two springs and one autumn for RAM.
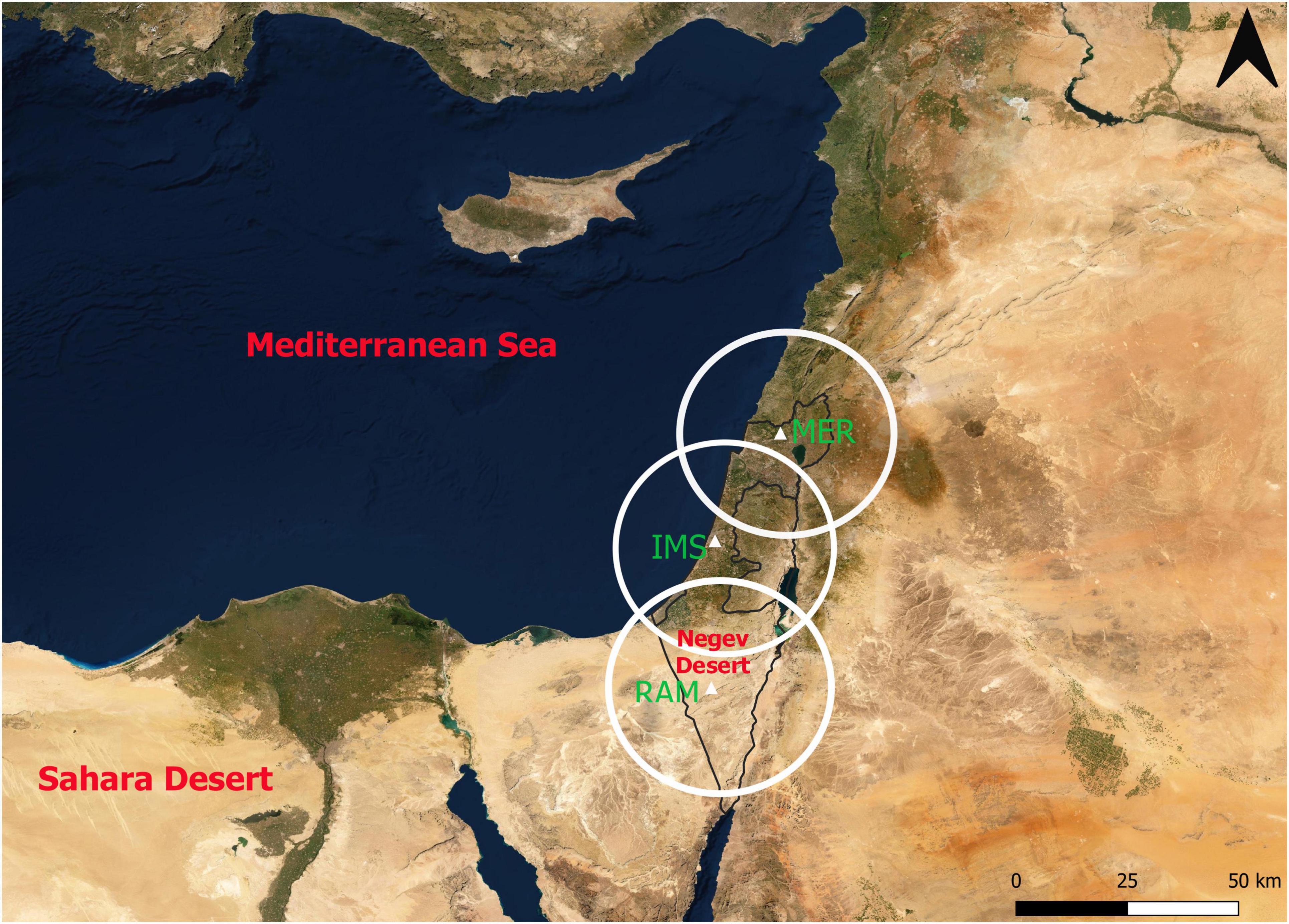
Figure 1. Satellite imagery of the study area with the weather radars (white triangles), their 100-km-radius coverage (white circles) and international borders (black lines). The ecological barriers in the study area are the Mediterranean Sea to the west of Israel and the northern fringes of the Saharo-Arabian desert belt that includes the Negev Desert in the southern part of country. Please note the transition from a desert environment in the south of the country (sandy colors in the image) to Mediterranean-type scrub vegetation in the center and northern parts of the country that is greener in color.
The radars emit C-band electromagnetic waves and record returned signals, known as reflectivity, from objects in the atmosphere at a polar resolution of ∼125 m in range and 1° in azimuth (except for MER radar in which sampling resolution was ∼62.5 m in range during 2017). The radars scan the atmosphere at varying tilt angles and the tilt angle closest to horizontal is the most useful for detecting birds departing from stopover sites to commence their flight (Table 1, Supporting Information). Scan data were collected through a two-stage process. At the first stage, we visually screened radar data at this tilt angle around the time of evening civil twilight when birds, primarily migrating passerines, depart daytime stopover locations. Nights with low reflectivity (i.e., few birds that departed), precipitation, anomalous propagation or other types of contamination cannot be used for mapping bird distributions and were subsequently eliminated from further analysis. In total, after the filtering processes, we analyzed 199 days for the autumn and 222 days for the spring from all three radars.
As expected, we found that birds initiate nocturnal migratory flights en masse in an abrupt exodus closely synchronized to the position of the sun (Åkesson et al., 1996). In a second stage, we capitalize on this phenomenon by interpolating radar data to an empirically determined sun angle at the point of maximum rate increase in mean total reflectivity among scans near the onset of nocturnal flight exodus for each of the remaining nights following McLaren et al. (2018). For nights with sparse data (from MER and RAM), we determined the sampling sun angle from the average angle among all the sampling days we used for the analysis from all the radars (9.8 degrees below the horizon). This represents a refinement to the method of Buler and Dawson (2014) that accounts for nightly variability in the exact timing of flight exodus. Our approach also accounts for temporal sampling bias between radars and nights, and minimizes the displacement of birds aloft from their ground sources (Buler and Diehl, 2009).
We constructed a radial grid shapefile for each radar to display data and for extracting georeferenced data, including measures of ground elevation, land cover, and other landscape metrics used for modeling bird distributions. Individual sample volumes of radar data grids match the resolution of the radar data: 125 m in range × 1° azimuth. Within 100 km of each radar, we assessed radar beam blockage and areas of persistent clutter by determining the frequency of detection of reflectivity among individual radar scans for the lowest tilt angle (for details see Buler and Diehl, 2009). In addition, the data were filtered to only include volumes that sampled more than 10% of the nightly vertically-integrated radar reflectivity (VIR) across at least 75% of sampling nights. We mapped the standardized probability of reflectivity detection for each sample volume to identify areas of (1) persistent ground clutter, where sample-mean magnitudes of measured reflectivity exceeding 1,000 Z occurred at a high frequency, and (2) partial beam blockage, where reflectivity was infrequently measured regardless of magnitude. Data from these sample volumes were excluded from analyses.
We computed mean seasonal VIR across sampling nights and years at the lowest tilt angle (Supplementary Table 1). The VIR represents an approximation of the mean reflectivity (i.e., cross-sectional area of energy reflected from birds back to the radar) from the ground to an elevation of 1.5 km within the column of airspace over the two-dimensional “footprint” of an individual sampling volume (Buler and Dawson, 2014) that is measured in units of cm2/ha. To aid in visualizing the results, we characterized bird stopover use across the study period by the mean VIR (MN) and the mean coefficient of variation of VIR (CV) across all nights. We classified stopover use following Buler and Dawson (2014). “Important” stopover sites were identified as those areas with high mean (≥85th percentile) VIR, and further categorized as “consistently high”, “moderately variable” or “highly variable” for CV ≤25th percentile, between the 25th and 75th percentile and greater than the 75th percentile, respectively. Stopover areas of “Medium” and “Low” use were classified as so according to MN between the 50th and 85th percentile, and below the 50th percentile, respectively. Areas classified as having consistently-high bird density are potentially important stopover sites for landbirds in the region.
Modeling Bird Response to Geographic, Habitat, Atmospheric and Anthropogenic Covariates
We calculated stopover densities at a 120-m resolution grid covering the land area within the effective range of detection of 100 km of each radar to model the effects of 30 different geographic, habitat, atmospheric and anthropogenic covariates on the densities of birds at stopover sites (Table 1). The number of cells used for the analysis was 74784 cells in the autumn and 69446 cells in the spring. From previous work (e.g., Schaub et al., 2004; Sjöberg et al., 2015; Lafleur et al., 2016; McLaren et al., 2018; Zenzal et al., 2018), we identified several environmental factors that may predict bird habitat use and obtained datasets for use as covariates in modeling. These included several geographic and corrective variables (i.e., spatial coordinates, distance to coast, distance to the nearest radar, rivers, lakes, fish ponds, artificial water sources, natural water bodies, relative elevation and bright ALAN), weather covariates [i.e., precipitation, temperature, zonal (u) and meridional (v) components of wind, velocity and geopotential height at 500 hPa], and landscape variables (i.e., land cover composition, forest cover type), soil wetness index and Soil Adjusted Vegetation Index (SAVI; see below). For the variables Distance from fish ponds and Distance from rivers, we obtained fish pond data from the Israeli Ministry of Agriculture GIS repository1, and river data from the Survey of Israel2. For the landscape variable we used the national land cover classification produced by Israel’s National Ecosystem Assessment Program (HaMa’arag3), with the exception of areas managed by the KKL-JNF (Keren Kayemeth LeIsrael – Jewish National Found), for which we used a land cover/vegetation dataset provided by the KKL-JNF (available from the KKL-JNF upon request).
Other data were freely available and downloaded from their sources. We obtained weather variables from ECMWF (European Centre for Medium-Range Weather Forecasts) and ALAN from VIIRS (Visible Infrared Imaging Radiometer Suite) Corrected Reflectance Imagery4. For weather covariates, we calculated seasonal means for each year. Due to sparse data in the VIIRS imagery in some months, we combined data across the spring and autumn and computed yearly means. We classified bright ALAN as light ≥5 times brighter than the natural level (digital number value > 26) following McLaren et al. (2018). We computed seasonal mean SAVI and soil wetness index for each year using data from the MODIS (MODerate-resolution Imaging Spectroradiometer) satellite obtained from the USGS LPDAAC (United States Geological Survey Land Processes Distributed Active Archive Center). SAVI is used to correct NDVI for the influence of soil brightness in areas where vegetation cover is low. We calculated SAVI using the formula [(NIR-red)/(NIR + red + L) × (1 + L)] (Huete, 1988). We obtained NIR and red surface reflectance bands from MODIS data product MYD13Q1 v006 which is produced at 16 days intervals at a spatial resolution of 250 m, and used a correction factor (L) of 0.5 (Huete, 1988). We computed soil wetness index from the MODIS reflectance data product MCD43A4 v005 (Nadir BRDF-Adjusted Reflectance) which is produced every 16 days at 500 m resolution and contains seven reflectance bands. We calculated a mean wetness index using the tasseled cap transformation for MODIS data (Lobser and Cohen, 2007), by the tasseledCap function (MODIS R package). Pairwise correlation among predictors were examine and in the spring model, the soil wetness index covariate was exclude as a result of high correlation with the northing variable (r = 0.9). The rest of the covariates correlations were less than 0.85 and generally low.
We ran separate model for each season and used the log of the arithmetic mean VIR (hereafter referred to as “lmean”) from our summarized radar data as the response variable. To model the relationships between lmean and the covariates, we used Boosted Regression Trees (BRT) models with the gbm.step function in R package “dismo” (Elith et al., 2008). The models had a Gaussian error distribution, 10-fold cross validation, tree complexity of 10, learning rate of 0.1, bag fraction of 0.5 and minimum number of trees of 25 per step. We ran the model 20 times for each season (with different seed for every model), averaged the results and evaluated model fit with an assessment of the total deviance explained of training data and a mean cross-validation correlation. Notably, BRT models use only a random subset of the data to fit each new tree (Friedman, 2002). Consequently, spatial and temporal correlation of the data is low. When models are intended only for interpolation (predicting the response variable values within the same spatial and temporal ranges by which they were trained), random cross-validation yields fair error estimates (Roberts et al., 2017). All analyses were conducted using R version 4.0.3 (R Core Team).
Results
The spring and autumn models share the same top 13 covariates (out of 30) that affect stopover densities with some difference in the order of importance (Table 2). The only covariate that is not shared is the soil wetness index which was excluded from the spring model as a result of high correlation with the northing covariate. All of the top 13 covariates are geographic and anthropogenic and neither relate to atmospheric factors nor to habitat properties. The combined relative importance of all the rest of the covariates used in the models, which include habitat and atmospheric covariates (17 different covariates) is less than 15%. The models had a mean cross-validation correlation of 0.805 ± 0.0001 for spring and 0.801 ± 0.0003 for autumn.
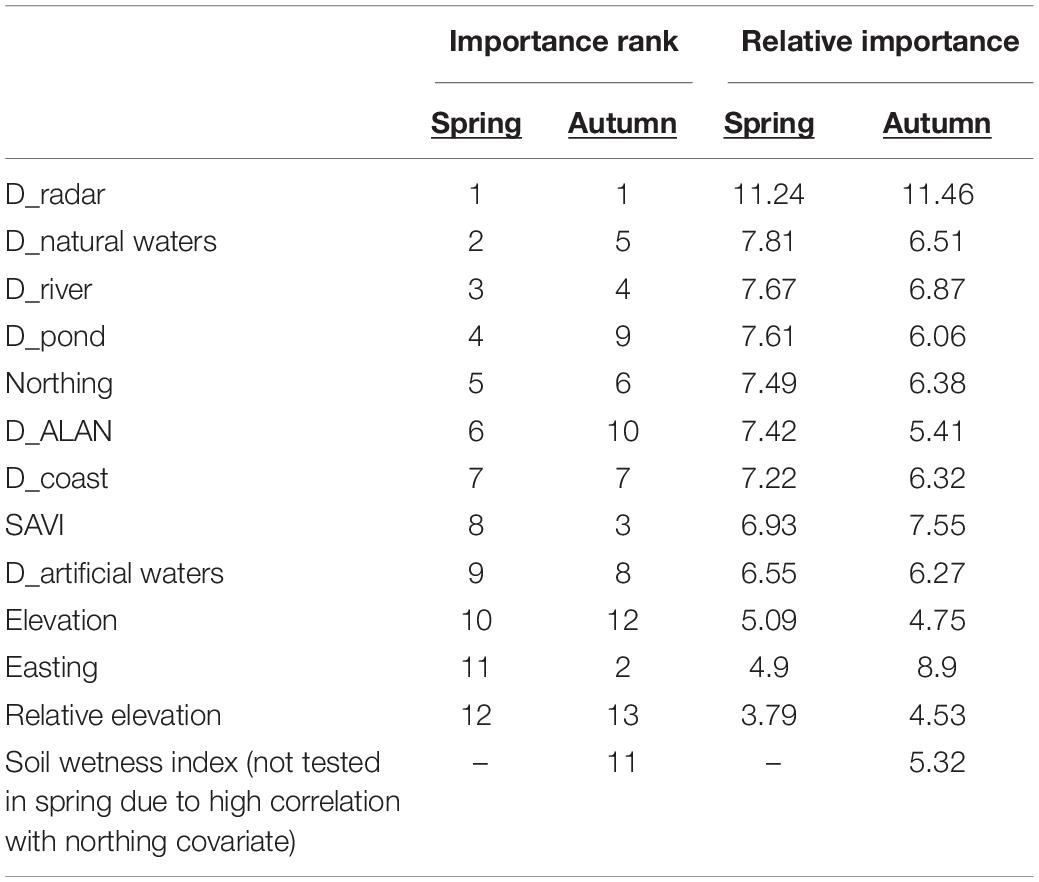
Table 2. Top 13 influencing covariates in the spring and the autumn as found in Boosted Regression Tree models. Relative importance of covariates is based on the number of times a variable is selected for splitting, weighted by the squared improvement of the model, averaged over all trees and scaled so that the sum adds to 100 (Friedman and Meulman, 2003). Covariate descriptions are given in Table 1.
We found that there is a substantial difference in the stopover distributions of birds between the spring and the autumn with higher densities in the desert (southern areas) and inland during the spring whereas birds were concentrated near the coast during the autumn (Figure 2). While bird densities were overall slightly higher in the autumn in the northern half of Israel, spring densities were much higher in the southern half of the country (Figure 3a). In addition, bird density decreased with distance from fresh water sources (rivers and artificial water reservoir) only during the autumn while no such effect was found during the spring (Figures 3b,c). Also, we found a positive effect of the soil wetness index on the stopover densities in the autumn (Table 2). Thus, birds tended to concentrate near water sources and in humid areas only during the autumn. Interestingly, the ground elevation covariate demonstrates a marked contrast between the spring and the autumn. Migrant densities were high at lower elevations during autumn while during spring densities were the greatest at higher elevations. In elevations near and below sea level, densities were much higher during the autumn compared to spring, while between sea level and about 1000 m above mean sea level (AMSL), densities in both seasons were similar, with slightly higher autumn densities. Above 1000 AMSL, there was a sharp contrast between the seasons, with very low autumn and very high spring densities (Figure 3d).
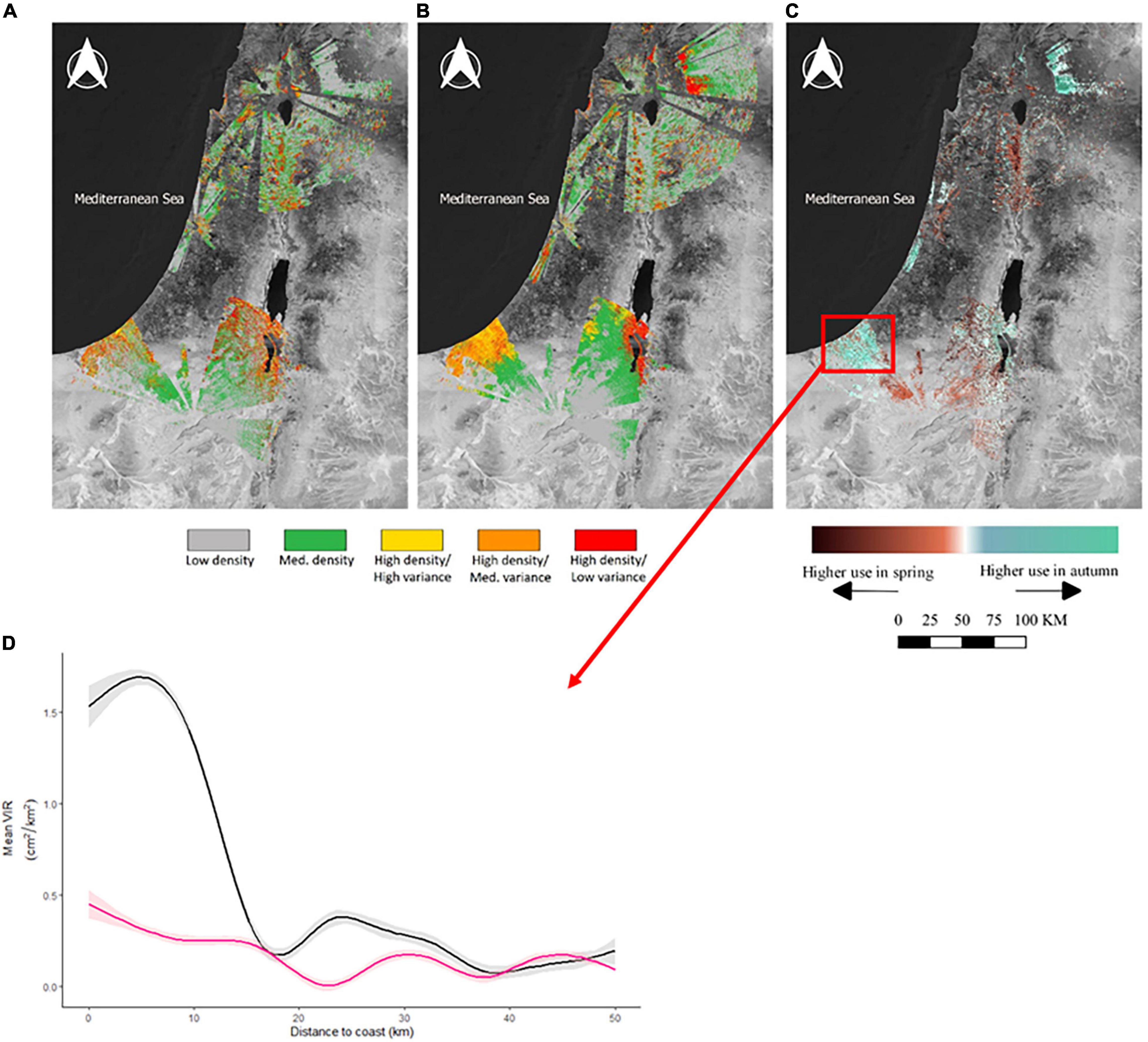
Figure 2. Spatial mapping of bird stopover densities classified by seasonal mean and coefficient of variation during (A) spring and (B) autumn. Classes are divided by seasonal mean stopover density as “low density” (<50th percentile), “medium density” (50th–85th percentile), and “high density” (≥85th percentile), and high density areas are further classified by the coefficient of variation as “high variance” (>75th percentile), “medium variance” (25th–75th percentile), and “low variance” (≤25th percentile). (C) Seasonal differences in mean reflectivity between the autumn and the spring. (D) Example of the change in mean vertically integrated bird reflectivity (VIR; cm2/km2) by distance to coast from Ramon radar in autumn (black line and a gray error area) and in spring (dark pink line and light pink error area).
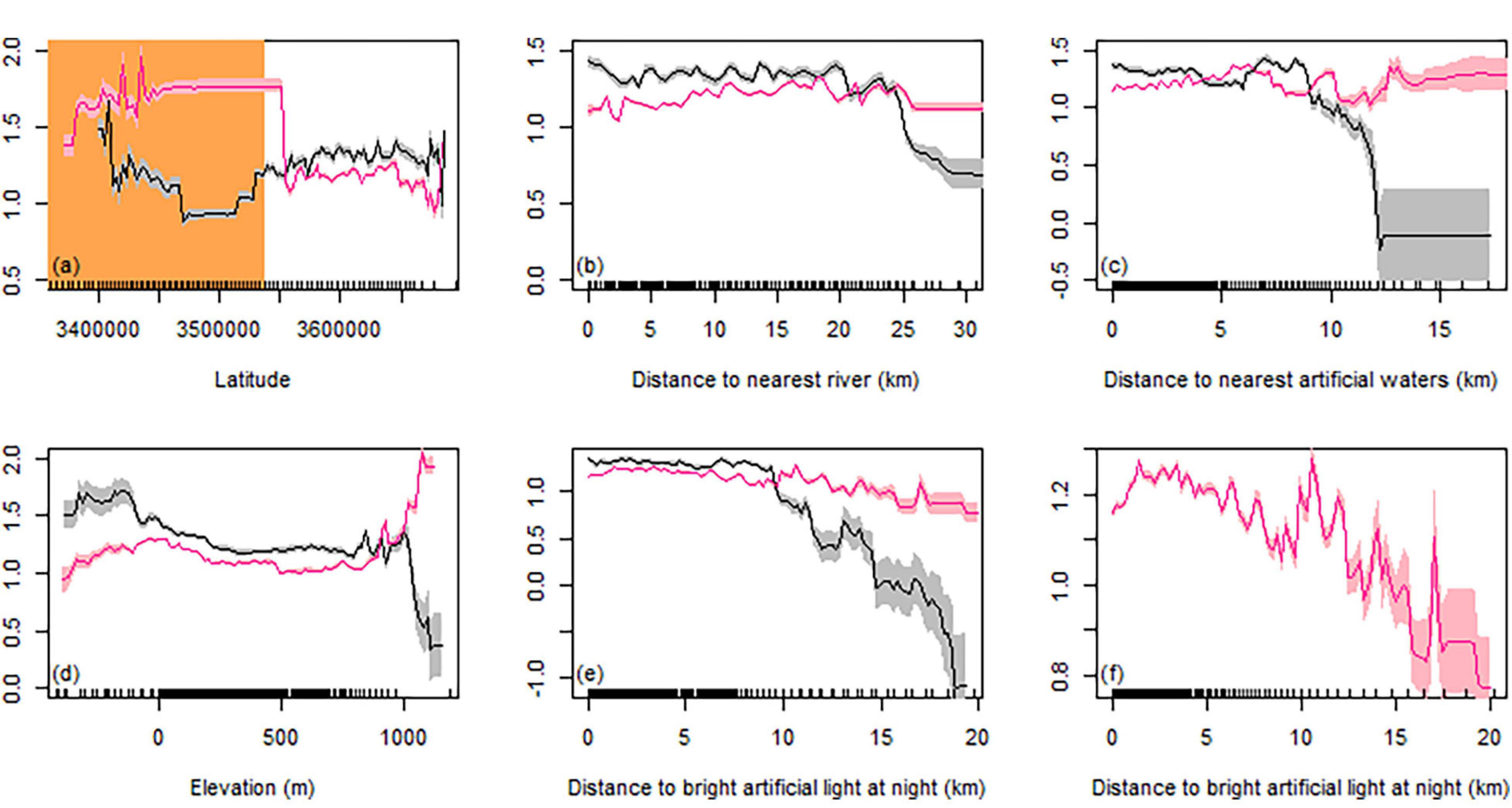
Figure 3. Marginal responses of mean vertically integrated bird reflectivity (VIR; cm2/km2) based on boosted regression tree (BRT) models in relation to (a) northing, (b) distance to rivers, (c) distance to artificial water reservoirs, (d) ground elevation, (e) distance to bright artificial light at night (ALAN) for both autumn and spring, (f) distance to bright ALAN only in the spring (note the change in the scale compared with e). Autumn is depicted by a black line and a gray error area, representing 95% confidence intervals among ensemble of 20 BRT models. Spring is depicted by a dark pink line and a light pink error area. In (a), the orange area represents the desert region latitudes, which encompass the southern half of Israel.
Another important influencing covariate was the distance to bright ALAN. In general, there were higher densities of birds near bright artificial light sources, and the densities started to decline at a distance of approximately 10 km from bright areas. Notably, the influence differed between the two migration seasons, and was stronger during the autumn (Figures 3e,f).
Discussion
In this study, we present the first broad-scale quantitative analysis of passerine stopover distributions outside North America in a unique geographic setting, testing how stopover bird distributions relate to two wide ecological barriers: a terrestrial barrier (the Saharo-Arabian desert belt which is one of the widest deserts in the world) and a marine barrier (the Mediterranean Sea). Our findings reveal complicated, season-specific effects of the ecological barriers on the stopover distribution of the migrants. These birds, which stage in Israel during their voyages between sub-Saharan Africa and Eurasia, use a wide variety of areas and habitats for stopover and tend to concentrate near the coast in the autumn and in the desert during the spring. In the desert, differences between the autumn and the spring are dramatic. The conditions in the desert differ substantially between the two seasons, as during the spring the desert is usually green in some areas, mainly along dry rivers beds, following the occasional winter rains. In contrast, during the autumn, following the hot summer and after many months with no precipitation, the desert is totally dry. Spring migrants that arrive to southern Israel, which is located at the northern fringes of the Saharo-Arabian desert belt after crossing about 2000 km of desert thus find there some food. Consequently, during spring, they stop-over at the desert fringes in high densities. During the autumn, birds that arrive from areas found north of the desert, mainly consisting food-rich Mediterranean-type scrub (Izhaki and Safriel, 1985), encounter very dry areas in the desert and appear to avoid stopping-over within it. Thus, the last place for birds to attempt refueling before the crossing of the desert is north of it, and not within it (Sapir et al., 2004; Domer et al., 2021).
How stopover densities all over Israel vary between the two migration seasons can also be explored by looking at the covariates distance to rivers and distance to artificial water reservoirs. In both covariates, there is a clear decrease in the density of birds with distance from fresh water sources during the autumn. During the spring, these covariates do not have a clear influence. Therefore, during the autumn, bird densities are high near wet areas of different sorts that may provide fresh water to the migrants. In spring, after the rainy winter season, no such effect is discernible, likely because access to water is less limited.
The reasons for which migrants concentrate near the coast during the autumn are not clear. One possible explanation for this pattern could be the loop migration hypothesis, which suggests a counterclockwise loop migration path of various bird species at the eastern part of the Mediterranean Sea, with a general pattern of a relatively western migration concentration during the autumn and a more easterly migration during spring, which takes place further inland, including east of Israel (Shirihai, 1996; Koleček et al., 2016; Briedis et al., 2018; Klvaňa et al., 2018). The higher inland densities of birds during the spring in northern Israel also support this explanation. The high bird densities in coastal areas in the autumn, which are evident throughout central and southern Israel, are likely a result of coastal landfall in this region. Alfiya (1995), who used a long-range scanning radar images from central Israel, reported that migrating birds flying over the Mediterranean Sea during autumn head south during the first part of the night, parallel to the coastline that has a general south-north direction. Whereas, after midnight, the birds turn toward a more easterly direction that brings them over land. This behavioral change between the start and end of the night has also been described elsewhere, for example in Lake Erie (Gesicki et al., 2019). Indeed, early morning observations of birders along the coastline often reported active migration heading inshore, sometimes at mass. For example, approximately 50,000 birds, mostly skylarks, were counted on 5 November 2014 at a single point on the coast near Tel-Aviv in central Israel, during 06:00–9:00 a.m. (Granit, personal communication). Our results suggest that many of these birds stop-over in sites close to the coastline. Noteworthy, higher densities were found very close to the coast, up to 20 km away from it in the most urbanized region of the country (see below) and not further inland. This pattern may indicate that the birds are not selective and stage in any habitat along the coast, just because it is found next to the shoreline. We note that we did not find any important effects of habitat properties or weather conditions on the stopover densities, and only extrinsic geographic and anthropogenic factors affected bird distribution, as found in Buler and Moore (2011).
Similar to findings from North America (McLaren et al., 2018; Cabrera-Cruz et al., 2020; Cohen et al., 2021), we found a strong correlation between proximity to artificial light at night and bird stopover densities. This broad scale pattern related to bright lights is the first documented evidence of this anthropogenic phenomenon outside the United States Northern and especially Central Israel are characterized by very high light pollution, whereas the desert, in the south of the country, has substantially fewer light sources (Falchi et al., 2016). While not a causal relationship per se, the relationship with ALAN was important even after controlling for potential confounds like amount of urban development and proximity to the coastline that is heavily developed. Therefore, the relationship of bird density with ALAN is consistent with behavioral attraction of migrating birds to artificial light (Gauthreaux and Belser, 2006; Van Doren et al., 2017). The possible consequences of staging in low quality stopover sites due to suboptimal selection of habitats as a result of this attraction is of heightened conservation concern. Optimal selection of stopover habitat is critical to migrating birds since stopover site quality may influence the rate of fuel accumulation (Kelly et al., 2002; Ktitorov et al., 2008; Schaub et al., 2008), which can affect the timing and speed of migration (McLaren et al., 2013; Lindström et al., 2019), as well as bird survival (Pfister et al., 1998) and breeding output (Sandberg and Moore, 1996; Drent et al., 2003). Consequently, selection of sub-optimal habitats due to attraction to light likely have negative fitness consequences (Gaston et al., 2015; McLaren et al., 2018; Sanders et al., 2020).
Moreover, light pollution could elicit staging in urban habitats (e.g., city parks) where cat predation is high and bird mortality is furthermore boosted through collision with tall buildings (Loss et al., 2013, 2014; Van Doren et al., 2021). The stronger correlation between stopover densities and ALAN during the autumn can be explained by the composition of the migrants that include more immature birds that migrate for the first time, compared with spring. These immature birds are known to attract more strongly to ALAN compared to adults (Gauthreaux and Belser, 2006). This overall higher impact of ALAN during the autumn could have substantial population level consequences (La Sorte et al., 2017). Thus, it is urgent to have a better understanding of the quality of these hot spot areas through measures of mass gain or stopover duration. High densities of birds, in low quality stopover sites, can increase the competition and as a result, may decrease the probability that the migrant birds will successfully refuel and survive the migration journey (Moore and Yong, 1991), especially during autumn when many of the birds are about to cross a wide desert following their stopover (Schmaljohann et al., 2007).
Our work uncovered the way ecological barriers affect the spatial and temporal distributions of migrating birds while stopping-over for refueling before and after they cross these massive barriers. Yet, one has to bear in mind that these environmental conditions may vary over time. Specifically, the Sahara Desert has expanded over the twentieth century by more than 10% (Thomas and Nigam, 2018). This expansion can significantly affect the survival of birds that migrate through this area and therefore knowledge of the properties of intensively-used stopover sites in the desert and on its edges is critically important. We identified a few high-priority areas for conservation of migrating passerines, which have been used differently in spring and autumn. The coastal region has very high densities of birds during the autumn while being the most populated region in Israel. In addition, this region is undergoing further expansion of urbanized areas at the expense of natural habitats. It is furthermore facing substantial, extensive future threats to migrating birds, including the development of large wind turbine facilities and gas pumping rigs that include bright light sources. The findings of our work suggest that habitat alteration due to anthropogenic development in and near the Israeli Mediterranean coastline, including infrastructure that may attract birds such as strong artificial lights, should be minimized and even completely avoided, as much as possible, especially in the central and south coastal regions in Israel. Any such alterations should be accompanied by environmental impact assessments and long-term monitoring to understand their implications for migrants as development in this sensitive area could have negative impacts on the survival of many migrating birds.
Our quantitative and spatially-explicit metrics of bird stopover intensities can be used by different stakeholders to minimize different current and future risks to migrating passerines. For example, we showed that during autumn, just before crossing the wide Saharo-Arabian desert belt, birds tend to concentrate in or near wet areas, including rivers and artificial water reservoirs. These areas are threatened from water pollution and intensive tourism (Herut et al., 2000; Barinova et al., 2010; Barinova and Krassilov, 2012). Furthermore, covering water reservoirs with solar panels is now rapidly expanding throughout the country, with little or no monitoring of this potentially serious threat to different animal groups and surrounding ecosystems (Mathijssen et al., 2020). Therefore, we encourage investigating habitat properties in and around water sources, including the vegetation features, water quality and reservoir size and shape. This can increase our understanding of the significance of water sources for birds and how existing and planned water sources could be improved to support migrating birds. In addition, we recommend considering our findings in the land-use planning policy at local, regional and national scales, to conserve migratory birds and their stopover areas at different times of the year. Specifically, we highly recommend keeping open, undisturbed natural habitats in coastal areas and prohibiting vegetation removal at the desert and its edges (e.g., for sand mining and altering natural drainage systems). We note that bird stopover distributions and the long-term trends of their densities (Rosenberg et al., 2019), which could be affected by anthropogenic actions (Shamoun-Baranes et al., 2011; Hüppop et al., 2019), are essential for informed conservation of migratory birds and their habitats. Unfortunately, due to policy changes, access to the radar data repositories that are essential for these analyses is becoming limited in Europe (Shamoun-Baranes et al., 2021), substantially hindering our ability to quantify biodiversity changes and identify their causes and consequences.
Data Availability Statement
The data supporting the results is archived on OSF and is available in the attached link: https://osf.io/5h6kv/?view_only=d7f51ec0d43f444ab9d81cf8da106a41.
Ethics Statement
Ethical review and approval was not required for the animal study because we used remote sensing (radars) for this study.
Author Contributions
IS, JB, and NS conceived the study. IS, JB, and JS processed radar data and wrote software and performed model analysis. JS and DT collected and processed covariate data. IS and NS wrote the manuscript with input from all co-authors. All authors contributed to the article and approved the submitted version.
Funding
This study was supported by a KKL-JNF forestry department grant “Examining the effects of forest properties on migrating birds that utilize KKL-JNF forests using comprehensive radar scans” (grant no. 90-05-182-19). Additionally, IS was supported by the Minerva Center for Movement Ecology and NS was supported by the Israel Science Foundation (Grant 702/17). Funding was also provided from USDA NIFA Hatch (grant no. DEL-00774) to JB.
Conflict of Interest
The authors declare that the research was conducted in the absence of any commercial or financial relationships that could be construed as a potential conflict of interest.
Publisher’s Note
All claims expressed in this article are solely those of the authors and do not necessarily represent those of their affiliated organizations, or those of the publisher, the editors and the reviewers. Any product that may be evaluated in this article, or claim that may be made by its manufacturer, is not guaranteed or endorsed by the publisher.
Acknowledgments
We thank the meteorology unit of the Israel Air Force and the Israel Meteorology Service for providing the radar data used in this work. We thank Yaron Charka for coordinating our work with KKL-JNF. Additionally, we thank Klil Giladi and Susannah Halligan for screening the radar images.
Supplementary Material
The Supplementary Material for this article can be found online at: https://www.frontiersin.org/articles/10.3389/fevo.2022.822220/full#supplementary-material
Footnotes
- ^ https://data1-moag.opendata.arcgis.com/datasets/%D7%91%D7%A8%D7%99%D7%9B%D7%95%D7%AA-%D7%93%D7%92%D7%99%D7%9D
- ^ https://data.gov.il/dataset/rivers
- ^ https://www.hamaarag.org.il/content/product/%D7%9B%D7%99%D7%A1%D7%95%D7%99-%D7%A7%D7%A8%D7%A7%D7%A2-2016
- ^ https://earthdata.nasa.gov/earth-observation-data/near-real-time/download-nrt-data/viirs-nrt
References
Åkesson, S., Alerstam, T., and Hedenström, A. (1996). Flight initiation of nocturnal passerine migrants in relation to celestial orientation conditions at twilight. J. Avian Biol. 95–102. doi: 10.2307/3677138
Alerstam, T. (2003). “Bird migration speed,” in Avian Migration, eds P. Berthold E. Gwinner E. Sonnenschein (Berlin: Springer), 253–267. doi: 10.1007/978-3-662-05957-9_17
Alerstam, T., and Pettersson, S. G. (1977). Why do migrating birds fly along coastlines? J. Theor. Biol. 65, 699–712. doi: 10.1016/0022-5193(77)90016-9
Alfiya, H. (1995). Surveillance radar data on nocturnal bird migration over Israel, 1989-1993. Isr. J. Zool. 41, 517–522.
Barinova, S., and Krassilov, V. A. (2012). Algal Diversity and Bio-indication of Water Resources in Israel. Int. J. Environ. Resour. 1, 62–72.
Barinova, S., Tavassi, M., Glassman, H., and Nevo, E. (2010). Algal indication of pollution in the Lower Jordan River, Israe. Appl. Ecol. Environ. Res. 8, 19–38. doi: 10.15666/aeer/0801_019038
Becciu, P., Menz, M. H. M., Aurbach, A., Cabrera-Cruz, S. A., Wainwright, C. E., Scacco, M., et al. (2019). Environmental effects on flying migrants revealed by radar. Ecography 42, 942–955. doi: 10.1111/ecog.03995
Berthold, P., Fiedler, W., Schlenker, R., and Querner, U. (1998). 25-year study of the population development of Central European songbirds: a general decline, most evident in long-distance migrants. Naturwissenschaften 85, 350–353.
Bradley, D. W., Clark, R. G., Dunn, P. O., Laughlin, A. J., Taylor, C. M., Vleck, C., et al. (2014). Trans-Gulf of Mexico loop migration of tree swallows revealed by solar geolocation. Curr. Zool. 60, 653–659. doi: 10.1093/czoolo/60.5.653
Briedis, M., Kurlavičius, P., Mackevičienė, R., Vaišvilienė, R., and Hahn, S. (2018). Loop migration, induced by seasonally different flyway use, in northern European barn swallows. J. Ornithol. 159, 885–891. doi: 10.1007/s10336-018-1560-1
Bruderer, B., and Liechti, F. (1998). Flight behaviour of nocturnally migrating birds in coastal areas: crossing or coasting. J. Avian Biol. 29, 499–507. doi: 10.2307/3677169
Bruderer, B., and Liechti, F. (1999). “Bird migration across the Mediterranean,” in Proceedings of the 22nd International Ornithological Congress, eds N. J. Adams R. H. Slotow (Johannesburg: BirdLife South Africa), 1983–1999.
Buler, J. J., and Dawson, D. K. (2014). Radar analysis of fall bird migration stopover sites in the northeastern U.S. Condor 116, 357–370. doi: 10.1650/CONDOR-13-162.1
Buler, J. J., and Diehl, R. H. (2009). Quantifying bird density during migratory stopover using weather surveillance radar. IEEE Trans. Geosci. Remote Sens. 47, 2741–2751. doi: 10.1109/TGRS.2009.2014463
Buler, J. J., and Moore, F. R. (2011). Migrant-habitat relationships during stopover along an ecological barrier: extrinsic constraints and conservation implications. J. Ornithol. 152, (Suppl. 1), S101–S112. doi: 10.1007/s10336-010-0640-7
Buler, J. J., Moore, F. R., and Woltmann, S. (2007). A multi-scale examination of stopover habitat use by birds. Ecology 88, 1789–1802. doi: 10.1890/06-1871.1
Cabrera-Cruz, S. A., Cohen, E. B., Smolinsky, J. A., and Buler, J. J. (2020). Artificial Light at Night is Related to Broad-Scale Stopover Distributions of Nocturnally Migrating Landbirds along the Yucatan Peninsula, Mexico. Remote Sens. 12:395. doi: 10.3390/RS12030395
Calvert, A. M., Walde, S. J., Taylor, P. D., Calvert, A. M., Walde, S. J., and Taylor, P. D. (2009). Nonbreeding-season drivers of population dynamics in seasonal migrants: conservation parallels across taxa. Avian Conserv. Ecol. 4:5. Available online at: https://dalspace.library.dal.ca//handle/10222/29831 [Accessed June 17, 2021]
Central Bureau of Statistics (2018). Population Density by Natural Areas 2018, Central Bureau of Statistic, Israel. Available online at: http://ghdx.healthdata.org/organizations/central-bureau-statistics-israelCent.Bur.Stat [Accessed June 28, 2021]
Chernetsov, N. (2006). Habitat selection by nocturnal passerine migrants en route: mechanisms and results. J. Ornithol. 147, 185–191. doi: 10.1007/s10336-006-0064-6
Cohen, E. B., Horton, K. G., Marra, P. P., Clipp, H. L., Farnsworth, A., Smolinsky, J. A., et al. (2021). A place to land: spatiotemporal drivers of stopover habitat use by migrating birds. Ecol. Lett. 24, 29–49. doi: 10.1111/ele.13618
Deppe, J. L., Ward, M. P., Bolus, R. T., Diehl, R. H., Celis-Murillo, A., Zenzal, T. J., et al. (2015). Fat, weather, and date affect migratory songbirds’ departure decisions, routes, and time it takes to cross the Gulf of Mexico. Proc. Natl. Acad. Sci. U.S.A. 112, E6331–E6338. doi: 10.1073/pnas.1503381112
Domer, A., Ovadia, O., and Shochat, E. (2018). Energy for the road: influence of carbohydrate and water availability on fueling processes in autumn-migrating passerines. Auk 135, 534–546. doi: 10.1642/AUK-17-228.1
Domer, A., Vinepinsky, E., Bouskila, A., Shochat, E., and Ovadia, O. (2021). Optimal stopover model: a state-dependent habitat selection model for staging passerines. J. Anim. Ecol. 90, 2793–2805. doi: 10.1111/1365-2656.13581
Drent, R., Both, C., Green, M., Madsen, J., and Piersma, T. (2003). Pay-offs and penalties of competing migratory schedules. Oikos 103, 274–292. doi: 10.1034/j.1600-0706.2003.12274.x
Elith, J., Leathwick, J. R., and Hastie, T. (2008). A working guide to boosted regression trees. J. Anim. Ecol. 77, 802–813. doi: 10.1111/j.1365-2656.2008.01390.x
Falchi, F., Cinzano, P., Duriscoe, D., Kyba, C. C. M., Elvidge, C. D., Baugh, K., et al. (2016). The new world atlas of artificial night sky brightness. Sci. Adv. 2:e1600377. doi: 10.1126/sciadv.1600377
Ferretti, A., Maggini, I., and Fusani, L. (2021). How to recover after sea crossing: the importance of small islands for passerines during spring migration. Ethol. Ecol. Evol. 33, 307–320. doi: 10.1080/03949370.2021.1886181
Fortin, D., Liechti, F., and Bruderer, B. (1999). Variation in the nocturnal flight behaviour of migratory birds along the northwest coast of the Mediterranean Sea. Ibis 141, 480–488. doi: 10.1111/j.1474-919x.1999.tb04417.x
Frannsson, T., Jakobsson, S., Kullberg, C., Mellroth, R., and Pettersson, T. (2006). Fuelling in front of the Sahara desert in autumn–an overview of Swedish field studies of migratory birds in the eastern Mediterranean. Ornis Svecica 16, 74–83.
Friedman, J. H. (2002). Stochastic gradient boosting. Comput. Stat. Data Anal. 38, 367–378. doi: 10.1016/S0167-9473(01)00065-2
Friedman, J. H., and Meulman, J. J. (2003). Multiple additive regression trees with application in epidemiology. Stat. Med. 22, 1365–1381. doi: 10.1002/SIM.1501
Fry, C. H., Ash, J. S., and Ferguson-Lees, I. J. (1970). Spring weights of some Palaearctic migrants at Lake Chad. Ibis 112, 58–82. doi: 10.1111/j.1474-919X.1970.tb00076.x
Gaston, K. J., Visser, M. E., and Hölker, F. (2015). The biological impacts of artificial light at night: the research challenge. Philos. Trans. R. Soc. B Biol. Sci. 370:20140133. doi: 10.1098/RSTB.2014.0133
Gauthreaux, S. A., and Belser, C. G. (2006). “Effects of artificial night lighting on migrating birds,” in Ecological Consequences of Artificial Night Lighting, eds E. Rich and C. Longcore (Washington, DC: Island Press).
Gesicki, D. V., Cech, E. L., and Bingman, V. P. (2019). Detoured flight direction responses along the southwest coast of Lake Erie by night-migrating birds. Auk 136, 1–14. doi: 10.1093/auk/ukz018
Herut, B., Kress, N., and Hornung, H. (2000). Nutrient pollution at the lower reaches of Mediterranean coastal rivers in Israel. Water Sci. Technol. 42, 147–152. doi: 10.2166/WST.2000.0306
Horton, K. G., Van Doren, B. M., La Sorte, F. A., Cohen, E. B., Clipp, H. L., Buler, J. J., et al. (2019). Holding steady: little change in intensity or timing of bird migration over the Gulf of Mexico. Glob. Chang. Biol. 25, 1106–1118. doi: 10.1111/gcb.14540
Huete, A. R. (1988). A soil-adjusted vegetation index (SAVI). Remote Sens. Environ. 25, 295–309. doi: 10.1016/0034-4257(88)90106-x
Hüppop, O., Ciach, M., Diehl, R., Reynolds, D. R., Stepanian, P. M., and Menz, M. H. M. (2019). Perspectives and challenges for the use of radar in biological conservation. Ecography 42, 912–930. doi: 10.1111/ECOG.04063
Hutto, R. L. (1985). “Habitat selection by nonbreeding, migratory landbird,.” in Habitat Selection in Birds, ed. M. L. Cody (London: Academic Press)Available online at: https://www.google.com/books?hl=en&lr=&id=Uo_0h7bxkKcC&oi=fnd&pg=PA455&dq=Habitat+selection+by+nonbreeding,+migratory+landbirds&ots=oN0pSrhu8X&sig=_XLx92gYnIaxDaeUJ7HaUeqoog0 [Accessed June 28, 2021].
Hutto, R. L. (2000). On the importance of en-route periods to the conservation of migratory landbirds. Stud. Avian Biol. 20, 109–114.
Izhaki, I., and Safriel, U. N. (1985). Why do fleshy-fruit plants of the mediterranean scrub intercept fall-but not spring-passage of seed-dispersing migratory birds? Oecologia 1985 671, 40–43. doi: 10.1007/BF00378449
Kelly, J. F., DeLay, L. S., and Finch, D. M. (2002). Density-dependent mass gain by wilson’s warblers during stopover. Auk 119, 210–213. doi: 10.1093/auk/119.1.210
Klvaňa, P., Cepák, J., Munclinger, P., Michálková, R., Tomášek, O., and Albrecht, T. (2018). Around the Mediterranean: an extreme example of loop migration in a long-distance migratory passerine. J. Avian Biol. 49, 1–9. doi: 10.1111/jav.01595
Koleček, J., Procházka, P., El-Arabany, N., Tarka, M., Ilieva, M., Hahn, S., et al. (2016). Cross-continental migratory connectivity and spatiotemporal migratory patterns in the great reed warbler. J. Avian Biol. 47, 756–767. doi: 10.1111/jav.00929
Ktitorov, P., Bairlein, F., and Dubinin, M. (2008). The importance of landscape context for songbirds on migration: body mass gain is related to habitat cover. Landsc. Ecol. 23, 169–179. doi: 10.1007/s10980-007-9177-4
Kummu, M., De Moel, H., Salvucci, G., Viviroli, D., Ward, P. J., and Varis, O. (2016). Over the hills and further away from coast: global geospatial patterns of human and environment over the 20th-21st centuries. Environ. Res. Lett. 11:034010. doi: 10.1088/1748-9326/11/3/034010
La Sorte, F. A., Fink, D., Buler, J. J., Farnsworth, A., and Cabrera-Cruz, S. A. (2017). Seasonal associations with urban light pollution for nocturnally migrating bird populations. Glob. Chang. Biol. 23, 4609–4619. doi: 10.1111/gcb.13792
Lafleur, J. M., Buler, J. J., and Moore, F. R. (2016). Geographic position and landscape composition explain regional patterns of migrating landbird distributions during spring stopover along the northern coast of the Gulf of Mexico. Landsc. Ecol. 31, 1697–1709. doi: 10.1007/S10980-016-0354-1/FIGURES/8
Lindström, Å., Alerstam, T., and Hedenström, A. (2019). Faster fuelling is the key to faster migration. Nat. Clim. Chang. 9, 288–289. doi: 10.1038/s41558-019-0443-7
Lobser, S. E., and Cohen, W. B. (2007). MODIS tasselled cap: land cover characteristics expressed through transformed MODIS data. Int. J. Remote Sens. 28, 5079–5101. doi: 10.1080/01431160701253303
Loss, S. R., Will, T., Loss, S. S., and Marra, P. P. (2014). Bird–building collisions in the United States: estimates of annual mortality and species vulnerability. Condor 116, 8–23. doi: 10.1650/condor-13-090.1
Loss, S. R., Will, T., and Marra, P. P. (2013). The impact of free-ranging domestic cats on wildlife of the United States. Nat. Commun. 4:1396. doi: 10.1038/ncomms2380
Marra, P. P., Cohen, E. B., Loss, S. R., Rutter, J. E., and Tonra, C. M. (2015). A call for full annual cycle research in animal ecology. Biol. Lett. 11:20150552. doi: 10.1098/rsbl.2015.0552
Mathijssen, D., Hofs, B., Spierenburg-Sack, E., van Asperen, R., van der Wal, B., Vreeburg, J., et al. (2020). Potential impact of floating solar panels on water quality in reservoirs; pathogens and leaching. Water Pract. Technol. 15, 807–811. doi: 10.2166/WPT.2020.062
McGowan, C. P., Hines, J. E., Nichols, J. D., Lyons, J. E., Smith, D. R., Kalasz, K. S., et al. (2011). Demographic consequences of migratory stopover: linking red knot survival to horseshoe crab spawning abundance. Ecosphere 2:art69. doi: 10.1890/es11-00106.1
McLaren, J. D., Buler, J. J., Schreckengost, T., Smolinsky, J. A., Boone, M., Emiel van Loon, E., et al. (2018). Artificial light at night confounds broad-scale habitat use by migrating birds. Ecol. Lett. 21, 356–364. doi: 10.1111/ele.12902
McLaren, J. D., Shamoun-Baranes, J., and Bouten, W. (2013). Stop early to travel fast: modelling risk-averse scheduling among nocturnally migrating birds. J. Theor. Biol. 316, 90–98. doi: 10.1016/j.jtbi.2012.09.021
Moore, F. R., and Aborn, D. A. (2000). Mechanisms of en route habitat selection: How do migrants make habitat decisions during stopover? Stud. Avian Biol. 20, 34–42.
Moore, F. R. (1995). “Habitat requirements during migration: important link in conservation,” in Ecology and Management of Neotropical Migratory Birds, eds T.E. Martin and Finch, D.M. (New York, NY: Oxford University Press).
Moore, F. R., Kerlinger, P., and Simons, T. R. (1990). Stopover on a Gulf coast barrier island by spring trans-Gulf migrants. Wilson Bull. 102, 487–500. doi: 10.1371/journal.pone.0148975
Moore, F. R., Woodrey, M. S., Buler, J. J., Woltmann, S., and Simons, T. R. (2005). “Understanding the stopover of migratory birds: a scale dependent approach,” in Bird Conservation Implementation and Integration in the Americas: Proceedings of the Third International Partners in Flight Conference 2002 March 20-24, Vol. 2, eds C. J. Ralph and T. D. Rich (Albany, CA: Pacific Southwest Research Station), 684–689. doi: 10.3389/fphys.2017.00532
Moore, F. R., and Yong, W. (1991). Evidence of food-based competition among passerine migrants during stopover. Behav. Ecol. Sociobiol. 28, 85–90. doi: 10.1007/BF00180984
Moreau, R. E. (1961). Problems of Mediterranean-saharan migration. Ibis 103, 580–623. doi: 10.1111/j.1474-919X.1961.tb02465.x
Newton, I. (2006). Can conditions experienced during migration limit the population levels of birds? J. Ornithol. 147, 146–166. doi: 10.1007/s10336-006-0058-4
Petit, D. R. (2000). Habitat use by landbirds along Nearctic-Neotropical migration routes: implications for conservation of stopover habitats. Stud. Avian Biol. 20, 15–33.
Pfister, C., Kasprzyk, M. J., and Harrington, B. A. (1998). Body-fat levels and annual return in migrating Semipalmated Sandpipers. Auk 115, 904–915. doi: 10.2307/4089509
Roberts, D. R., Bahn, V., Ciuti, S., Boyce, M. S., Elith, J., Guillera-Arroita, G., et al. (2017). Cross-validation strategies for data with temporal, spatial, hierarchical, or phylogenetic structure. Ecography 40, 913–929. doi: 10.1111/ECOG.02881
Rosenberg, K. V., Dokter, A. M., Blancher, P. J., Sauer, J. R., Smith, A. C., Smith, P. A., et al. (2019). Decline of the North American avifauna. Science 366, 120–124. doi: 10.1126/science.aaw1313
Sandberg, R., and Moore, F. R. (1996). Fat stores and arrival on the breeding grounds: reproductive consequences for passerine migrants. Oikos 77, 577–581. doi: 10.2307/3545949
Sanders, D., Frago, E., Kehoe, R., Patterson, C., and Gaston, K. J. (2020). A meta-analysis of biological impacts of artificial light at night. Nat. Ecol. Evol. 51, 74–81. doi: 10.1038/s41559-020-01322-x
Sanderson, F. J., Donald, P. F., Pain, D. J., Burfield, I. J., and van Bommel, F. P. J. (2006). Long-term population declines in Afro-Palearctic migrant birds. Biol. Conserv. 131, 93–105. doi: 10.1016/J.BIOCON.2006.02.008
Sapir, N., Abramsky, Z., and Shochat, E. (2004). Scale-dependent habitat selection in migratory frugivorous passerines. Naturwissenschaften 91, 544–547. doi: 10.1007/s00114-004-0564-2
Schaub, M., Jenni, L., and Bairlein, F. (2008). Fuel stores, fuel accumulation, and the decision to depart from a migration stopover site. Behav. Ecol. 19, 657–666. doi: 10.1093/beheco/arn023
Schaub, M., Liechti, F., and Jenni, L. (2004). Departure of migrating European robins. Erithacus rubecula, from a stopover site in relation to wind and rain. Anim. Behav. 67, 229–237. doi: 10.1016/j.anbehav.2003.03.011
Schmaljohann, H., Liechti, F., and Bruderer, B. (2007). Songbird migration across the Sahara: the non-stop hypothesis rejected! Proc. R. Soc. B Biol. Sci. 274, 735–739. doi: 10.1098/rspb.2006.0011
Shamoun-Baranes, J., Bauer, S., Chapman, J. W., Desmet, P., Dokter, A. M., Farnsworth, A., et al. (2021). Weather radars’ role in biodiversity monitoring. Science 372:248. doi: 10.1126/SCIENCE.ABI4680
Shamoun-Baranes, J., Dokter, A. M., Van Gasteren, H., Van Loon, E. E., Leijnse, H., and Bouten, W. (2011). Birds flee en mass from New Year’s Eve fireworks. Behav. Ecol. 22, 1173–1177. doi: 10.1093/beheco/arr102
Shirihai, H. (1996). “The white stork,” in The Birds of Israel, eds E. Dovrat and D. A. Christie (London: Academic Press).
Shochat, E., Abramsky, Z., Pinshow, B., and Whitehouse, M. E. A. (2002). Density-dependent habitat selection in migratory passerines during stopover: What causes the deviation from IFD? Evol. Ecol. 16, 469–488. doi: 10.1023/A:1020851801732
Sieges, M. L., Smolinsky, J. A., Baldwin, M. J., Barrow, W. C., Randall, L. A., and Buler, J. J. (2014). Assessment of bird response to the migratory bird habitat initiative using weather-surveillance radar. Southeast. Nat. 13, G36–G65. doi: 10.1656/058.013.0112
Sillett, T. S., and Holmes, R. T. (2002). Variation in survivorship of a migratory songbird throughout its annual cycle. J. Anim. Ecol. 71, 296–308. doi: 10.1046/j.1365-2656.2002.00599.x
Simons, T. R., Moore, F. R., and Gauthreaux, S. A. (2000). Mist netting trans-gulf migrants at coastal stopover sites: the influence of spatial and temporal variability on capture data. Stud. Avian Biol. 29, 135–143.
Sjöberg, S., Alerstam, T., Åkesson, S., Schulz, A., Weidauer, A., and Coppack, R. M. (2015). Weather and fuel reserves determine departure and flight decisions in passerines migrating across the Baltic Sea. Anim. Behav. 104, 59–68. doi: 10.1016/J.ANBEHAV.2015.02.015
Sokolov, L. V., Baumanis, J., Leivits, A., Poluda, A. M., Yefremov, V. D., Markovets, M. Y., et al. (2001). Comparative analysis of long-term monitoring data on numbers of passerines in nine European countries in the second half of the 20th century. Avian Ecol. Behav. 7, 41–74.
Strandberg, R., Klaassen, R. H. G., Hake, M., and Alerstam, T. (2010). How hazardous is the Sahara Desert crossing for migratory birds? Indications from satellite tracking of raptors. Biol. Lett. 6, 297–300. doi: 10.1098/rsbl.2009.0785
Thomas, N., and Nigam, S. (2018). Twentieth-century climate change over Africa: seasonal hydroclimate trends and sahara desert expansion. J. Clim. 31, 3349–3370. doi: 10.1175/JCLI-D-17-0187.1
Van Doren, B. M., Horton, K. G., Dokter, A. M., Klinck, H., Elbin, S. B., and Farnsworth, A. (2017). High-intensity urban light installation dramatically alters nocturnal bird migration. Proc. Natl. Acad. Sci. U.S.A. 114, 11175–11180. doi: 10.1073/pnas.1708574114
Van Doren, B. M., Willard, D. E., Hennen, M., Horton, K. G., Stuber, E. F., Sheldon, D., et al. (2021). Drivers of fatal bird collisions in an urban center. Proc. Natl. Acad. Sci. U.S.A. 118:e2101666118. doi: 10.1073/PNAS.2101666118
Wikelski, M., Tarlow, E. M., Raim, A., Diehl, R. H., Larkin, R. P., and Visser, G. H. (2003). Costs of migration in free-flying songbirds. Nature 423:704. doi: 10.1038/423704a
Keywords: biodiversity monitoring, bird conservation, East-Mediterranean flyway, light pollution, Palearctic-Afrotropical migratory birds, radar ornithology, stopover ecology, weather radars
Citation: Schekler I, Smolinsky JA, Troupin D, Buler JJ and Sapir N (2022) Bird Migration at the Edge – Geographic and Anthropogenic Factors but Not Habitat Properties Drive Season-Specific Spatial Stopover Distributions Near Wide Ecological Barriers. Front. Ecol. Evol. 10:822220. doi: 10.3389/fevo.2022.822220
Received: 25 November 2021; Accepted: 14 February 2022;
Published: 21 March 2022.
Edited by:
Cecilia Nilsson, Lund University, SwedenReviewed by:
Nathaniel E. Seavy, National Audubon Society, United StatesLin Zhang, Hubei University of Chinese Medicine, China
Piotr Matyjasiak, Cardinal Stefan Wyszyński University, Poland
Copyright © 2022 Schekler, Smolinsky, Troupin, Buler and Sapir. This is an open-access article distributed under the terms of the Creative Commons Attribution License (CC BY). The use, distribution or reproduction in other forums is permitted, provided the original author(s) and the copyright owner(s) are credited and that the original publication in this journal is cited, in accordance with accepted academic practice. No use, distribution or reproduction is permitted which does not comply with these terms.
*Correspondence: Inbal Schekler, goldinbal@gmail.com
†Present address: Jaclyn A. Smolinsky, Cherokee Nation System Solutions, United States Geological Survey, Wetland and Aquatic Research Center, Lafayette, LA, United States