- 1Evolutionary Zoology, Department of Environment and Biodiversity, University of Salzburg, Salzburg, Austria
- 2Department of Geoecology, Institute of Geosciences and Geography, Faculty of Natural Sciences III, Martin Luther University Halle-Wittenberg, Halle, Germany
- 3Department of Ecology and Biogeography, Nicolaus Copernicus University Toruń, Toruń, Poland
- 4Department of Biological Sciences, Pwani University, Kilifi, Kenya
Ecosystem functions are important for the resilience of ecosystems and for human livelihood quality. Intact habitats and heterogeneous environments are known to provide a large variety of ecosystem functions. Natural and near natural ecosystems surrounding agroecosystems may positively support crop growing conditions and thus facilitate crop yields. In contrast, monocultures of crops and trees as well as degraded landscapes are known to provide less ecosystem functions. The Taita Hills in southern Kenya are part of the Eastern Afromontane biodiversity hotspot, and represent a habitat mosaic consisting of largely intact cloud forests, agroecosystems and plantations of exotic trees. In this region, subsistence farmers rely on ecosystem functions provided by natural ecosystems. In this study, we analyze three proxies of biodiversity and ecosystem functions, namely pollination activity, predation rates, and arthropod diversity in tree canopies. We set study plots along forest-agroecosystem-gradients, covering cloud forest, forest edge and agricultural fields, as well as plantations of exotic trees. We assessed environmental conditions, to evaluate the extent to which local environmental factors influence ecosystem functions. Based on these data we investigate potential spill over of ecosystem functions from forest into adjoining agroecosystems. For predation rates we found trends of spill over effects from forest interior into the agroecosystem. The expression of ecosystem functions differed among habitat types, with comparatively high predation rates in the forest, high pollinator activity in the open agricultural areas, and highest arthropod diversity along the forest edge. Eucalyptus plantations showed reduced ecosystem functions and lowest arthropod diversity. Local factors such as vegetation cover and flower supply positively influence pollinator activity. Our study show that natural ecosystems may positively contribute ecosystem functions such as predation, while the homogenization of biota through planting of invasive exotic tree species significantly reduce biodiversity and ecosystem functions. Transition habitats such as forest margins, and small-scale ecological enhancement positively influences biodiversity and ecosystem functions.
Introduction
Natural ecosystems provide valuable habitats for numerous animal and plant species and ecosystem functions (Ebeling et al., 2018). Ecosystem functions are of high relevance to the resilience of ecosystems, and for human well-being (Naeem et al., 2010). Human livelihood quality rely on intact nature and landscapes (Millennium Ecosystem Assessment, 2005; IPBES, 2019). In the meanwhile, the value of ecosystem functions has been estimated and quantified in monetary terms in various studies (Dainese et al., 2019). For example, it is estimated that the annual pollination of crops by various insects alone creates US$ 195 billion to US$ 387 billion United States dollars in value (Klein et al., 2008; Porto et al., 2020). Studies evidenced that landscape homogenization in agroecosystems lead to a significant reduction of ecosystem functions and services, such as pollinator activity and pest control (Steffan-Dewenter et al., 2006; Witt et al., 2018; Dainese et al., 2019). Thus, natural and near-natural ecosystem interspersed throughout landscape may significantly increase crop yields in neighboring fields through positive spill over effects (Kollmann et al., 2016; Barrios et al., 2018; Dawson et al., 2019; Tamburini et al., 2020; Tschartke et al., 2021). This was evidenced for pollinators (Garibaldi et al., 2016) and predators like birds which are of high relevance for pest control (Benjamin et al., 2016; Karp et al., 2018; Tela et al., 2021).
The distribution of biodiversity and ecosystem functions become particularly evident for the highly degraded cloud forest fragments of Taita Hills, southern Kenya. This mountain range is part of the Eastern Afromontane biodiversity hotspot (Burgess et al., 2007; Mittermeier et al., 2011). These mountains were originally covered by cloud forest, but become almost completely deforested in the wake of human settlement (Maeda et al., 2010). Diverse and species riche cloud forest has been almost completely transformed into eucalyptus plantations and agricultural fields, interspersed by some few cloud forest remnants (Teucher et al., 2020), with negative effects on (endemic) flora and fauna (Omoro et al., 2010; Norfolk et al., 2017) as well as ecosystem functions (e.g., soil fertility, soil erosion, Michelsen et al., 1996; Scott et al., 2005; Baohanta et al., 2012; water availability, Hohenthal, 2018; Castro-Díez et al., 2019). For instance, many of the original local springs dried up during the past decades in the wake of large-scale planting of eucalyptus trees (Hohenthal et al., 2015). Other ecosystem functions such as pollination and pest control (among others, see Lamarque, 2009; Pellikka et al., 2013) might similarly negatively affected from ecosystem destruction.
In our work we measured different proxies of ecosystem functions in parallel, on identical plots and along gradients to test for potential spill over effects (from forest interior into the surrounding agroecosystems). Apart from measuring ecosystem functions on the ground, we also considered arthropod diversity of tree canopies, which harbor an essential part of biodiversity in tropical forests (see Nadkarni, 1994). And, in addition to potential effects and trends at a landscape scale (comparing different ecosystems), we also measured potential effects of the local environment for each study plot. For our analyses we considered natural cloud forest, forest edge (transition zone between cloud forest and agricultural land), agricultural fields (open land), and eucalyptus plantations. Ecosystem functions were measured at 10 × 10 m study plots, which were mainly set along gradients from forest interior, transgressing the forest edge, and ranging into the agricultural fields (perpendicular to the forest edge) (see Habel and Ulrich, 2020). We measured three proxies to quantify biodiversity and ecosystem functions: pollination activity, predation rates [according the Rapid Ecosystem Function Assessment (REFA) protocol, Meyer et al., 2015; Meyer et al., 2017], as well as arthropod diversity in tree canopies using flight interception traps (see Habel et al., 2021). We chose these proxies as they are considered to play a key-role for successful agriculture (Meyer et al., 2015) and represent the ecological performance of a landscape. In addition, we measured environmental parameters for study plots to account for potential local effects of environmental conditions on ecosystem functions. Based on these data and the results obtained, we will answer the following questions:
1. Are there significant differences in arthropod diversity and ecosystem functions between the different habitat types studied?
2. Do arthropod diversity and ecosystem functions differ between native forest and exotic tree plantations?
3. Do positive spill over effects of diversity and ecosystem functions exist from forest interior into the agricultural landscape?
4. What can we derive from our results for practical forest conservation and landscape management?
Materials and Methods
Study Area
The Taita Hills represent the northern most section of the Eastern Arc Mountain range (Newmark, 1998; Burgess et al., 2007) and host a large variety of endemic (endangered) plant and animal species (Bytebier, 2001). Therefore, the Taita Hills are part of the Eastern Afromontane Biodiversity hotspot (Mittermeier et al., 2011). Pre-colonial human settling started the transformation of natural cloud forest into agroecosystems for smallholder farming (Hohenthal, 2018), and forest replacement by exotic tree plantations (Teucher et al., 2020). This transformation of ecosystems also affects species diversity until today (Mulwa et al., 2021) and species composition (Schmitt et al., 2020). These changes of ecosystems also impact various ecosystem functions which are important for the resilience and recovering of ecosystems, and human livelihood quality. Studies showed that the expansion of eucalyptus trees caused the drying out of major parts of Taita Hills, and subsequently the vanishing of most springs (Hohenthal et al., 2015; unpublished data). That is problematic as water is one of the key pre-requisites for successful smallholder farming. We assume that other ecosystem functions have been negatively affected by these fundamental changes of ecosystems.
Study Set-Up
For the measuring of ecosystem functions, we applied a standardized, low-tech, and easy-repeatable technique – the REFA (Meyer et al., 2015). We measured pollination activity and predation rates. These proxies are of high relevance to conduct successful smallholder farming (Tscharntke et al., 2012). Data collection was performed at the end of the dry season (3–24 September 2021). One study plot (10 × 10 m) consisted of three colored pan traps (yellow, blue, and white) set in the plot center, and 10 artificial caterpillar dummies out of green plasticine (spread across the study plot, with 10 cm minimum distance between single dummies, fixed on a small brown paper pad). In total we established 160 study plots along gradients from dense cloud forest interior into open agroecosystems, and additional 20 plots within exotic plantations. Gradients were set perpendicularly to the border of the forest. For each gradient, we established two study plots inside the forest (8 m and about 200 m distant from forest edge), one plot at the forest border (0 m), and five study plots across the agricultural land (with 8, +16, +32, +64, +128 m distance from the previous study plot, following a logarithmic scale). The aim of integrating gradients in our study was to test for potential spillover effects of ecosystems functions from natural forest into the adjoining agroecosystems (see section “Introduction”). In parallel, we used these data to compare levels of ecosystem functions for the different habitats. For this comparative approach we used data from the following habitat types and study plots: Cloud forest (200 m distance from forest edge, N = 20 plots), agricultural land (248 m distance from forest edge, N = 20 plots), forest border (N = 20 plots), and eucalyptus tree plantations (N = 20 plots). The exact location of each study plot was measured with a handheld GPS Device (Garmin GPSMAP 64s), and visualized in Figure 1. In the following, we describe the two proxies of ecosystem function assessed based on the REFA method, and the collection of arthropods in tree canopies using flight interception traps.
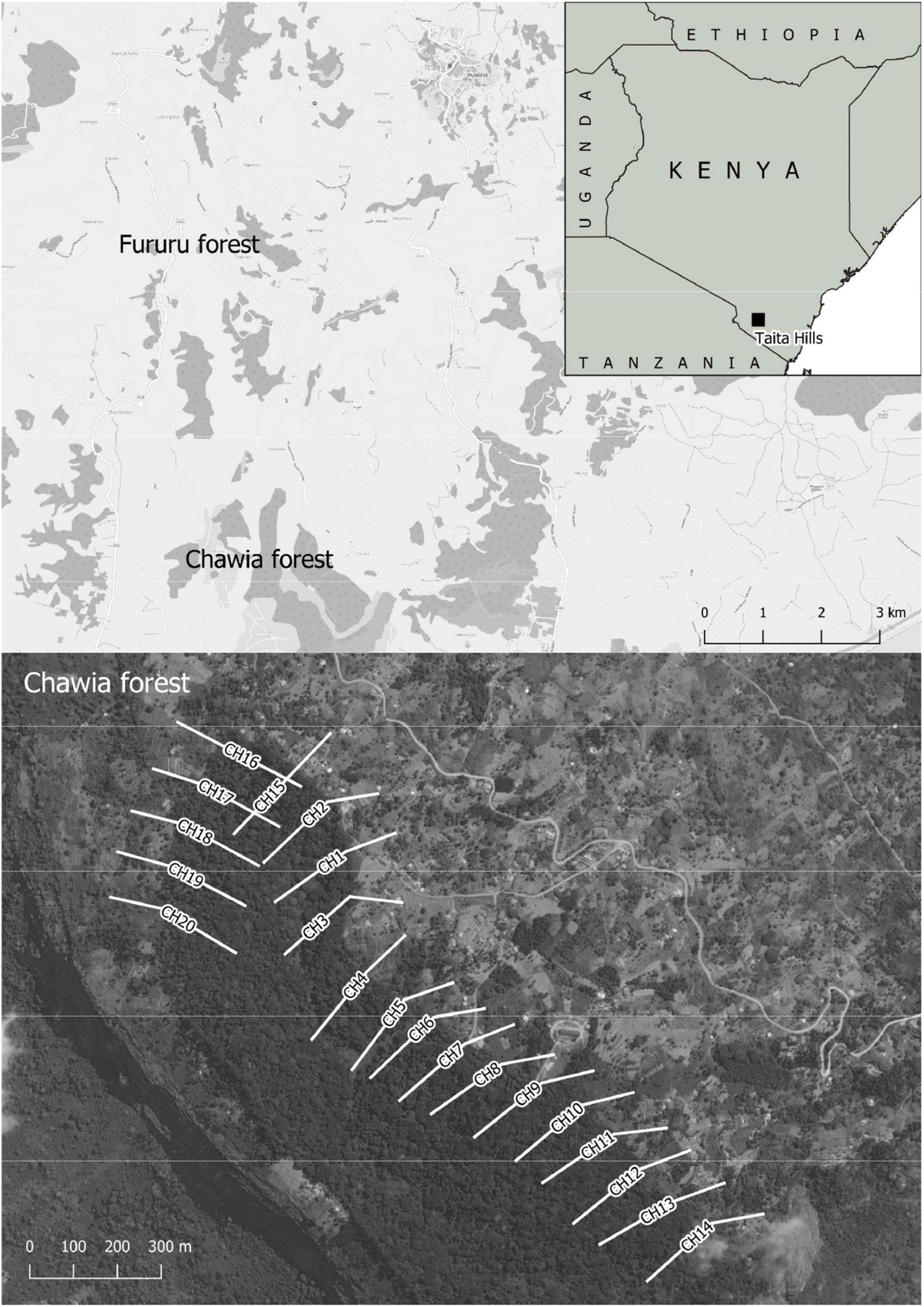
Figure 1. The Taita Hills study area in Kenya (small inlet map), with the two forest patches, Fururu forest (plantation) and Chawia cloud forest, surrounded by open agricultural land. Gradients are shown in the large map below.
Rapid Ecosystem Function Assessment
Pollination activity is crucial for successful agriculture and high crop yields (Klein et al., 2008). We measured pollination activity based on the total number of insects caught with yellow, white and blue pan traps. Several studies have shown that these colors exhibit highest sampling efficiencies across a wide array of different taxa of flying insects (Campbell and Hanula, 2007; Westphal et al., 2008; Wilson et al., 2008; Nuttman et al., 2011). Pan traps were filled with saturated salt solution with dish washer and placed at a height of about one meter above ground level. Three traps were positioned in the center of each study plot. The pan traps were activated for a total time span of 7 h, from morning till late afternoon. We subsequently sorted the material according to orders Hymenoptera (excluding Formicidae), Coleoptera, Diptera, and Lepidoptera. In addition, we considered representatives of the family Formicidae.
Natural pest control may significantly increase agricultural yields. Therefore, we measured the level of pest control by measuring predation rates. For this, we counted attacks on artificial caterpillars made out of green plasticine (Loiselle and Farji-Brener, 2002; Koh and Menge, 2005; Ruiz-Guerra et al., 2012). This method allows to differentiate among predator groups (in our case we differentiated among insects, birds, rodents, and snails) by respective bite marks on the green plasticine (cf. Howe et al., 2009). We used 10 × 2 cm long caterpillar dummies at each study plot. The caterpillars were exposed for 24 h to attract both, diurnal as well as nocturnal predators. Subsequently, we assessed all bite marks and calculated the proportion of dummies with at least one bite mark. As one predator may cause several bite marks, we did not consider bite frequency per dummy. Thus, more than one bite mark per species would be recorded as 1 record per dummy. Vanished dummies were classified as predated, without any further information on the group of predator (cf. Meyer et al., 2017).
Flight Interception Traps in Tree Canopies
Apart from the two proxies of ecosystem functions, we also assessed arthropod diversity in canopies of trees, across all four habitat types. For data collection, we used light traps equipped with blue, green, and UV LEDs, which were attached to four panes (10 × 30 cm each) of acrylic glass. A collecting jar underneath was filled with 70% Ethanol. A power bank provided electricity for two nights (>48 h). We installed light traps in the lower canopies about 4 m above ground. Sampling was done at five locations for each of the four habitat types (inside the cloud forest Chawia, along the forest edge of Chawia bordering open agricultural land, open agricultural land, and plantations of exotic eucalyptus trees, in total 20 sampling sites). Arthropod sampling was repeatedly conducted for 2 days and nights at each sampling site. Each trap was activated the whole 48 h to utilize the passive effect of the flight interception traps during the day, and the attractive effect during night. We emptied the traps every second day at 5 p.m., changed the batteries and relocated them. Each trap was set at least 1 h before sunset. Sampling was conducted during a 10-day sampling campaign (13-23 September 2021). Some of the UV LEDs inside the Chawia cloud forest were placed in the dense leaf canopy. Therefore, the radiant power of the LEDs was limited in space. Distances among traps were at least 150 m, and the UV LEDs were placed so that the light of the neighboring lamp was not visible to reduce spatial autocorrelation (see Horak, 2013).
Environmental Parameters
Local environmental conditions might impact biodiversity and ecosystem functions. Thus, we measured the following environmental parameters for single study plots: Estimated cover of leaf litter (no, little, medium, and high); estimated cover of herbs, shrubs, and canopy-closure (all with this scale 0–25, 25–50, 50–75, 75–100%); estimated flower availability (no, little, medium, and high).
Statistics
We used generalized linear modeling (GLM) to link pollination activity, predation rates, and biodiversity measures (response variables) to the above mentioned environmental parameters (categorical predictors), and to the distance from forest edge (metric parameter). As the response variables were based on count data, we used a Poisson error structure and a linear link function (O’Hara and Kotze, 2010; Little, 2013). Single effects of habitat structure were assessed from one-way ANOVA and post hoc Tukey tests. We estimated the degree of habitat variability from the coefficient of variation CV = σ/μ of the two REFA variables within each habitat type, where σ and μ are the SD and the mean variable expressions. Calculations were done with Statistics 12.0. Errors refer to standard errors and bootstrapped confidence limits.
Results
The four habitat types (cloud forest, forest edge, agricultural land, and exotic tree plantations) differed with respect to predation rate and pollination activity (Table 1 and Figure 2). Predation rate was highest in the cloud forest and pollination activity was highest at the forest edge (Figure 2). Pollination activity, i.e., Hymenoptera, Coleoptera, and Diptera abundances were lowest in the plantations and highest in the agricultural land (Table 1). Trapping with flight-interception traps in canopies returned very similar results (Figure 3). Forest edges and agricultural land were richest in total insect numbers (Figure 3A) and major insect orders (Figure 3B). The plantations were in both cases devoid of insects (Figure 3). The GLM returned significant differences in pollination activity with respect to habitat characteristics (Table 2). Pollination activity was lowest in places without herb cover (Figure 4A) and low flower numbers (Figure 4D). Predation rates increased with increased shrub cover (Figure 4C). Leaf litter cover did not significantly influence predation and pollination activity (Figure 4B).
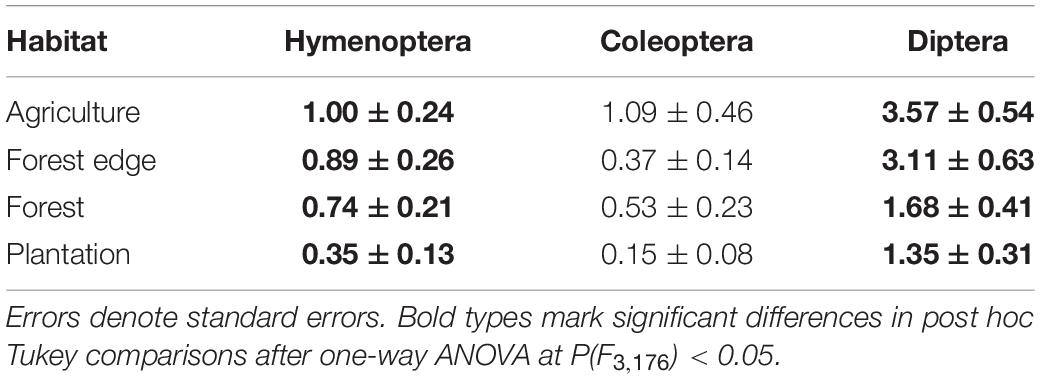
Table 1. Average values of major pollinator abundances (blue, yellow, and white traps combined) in the four habitat types.
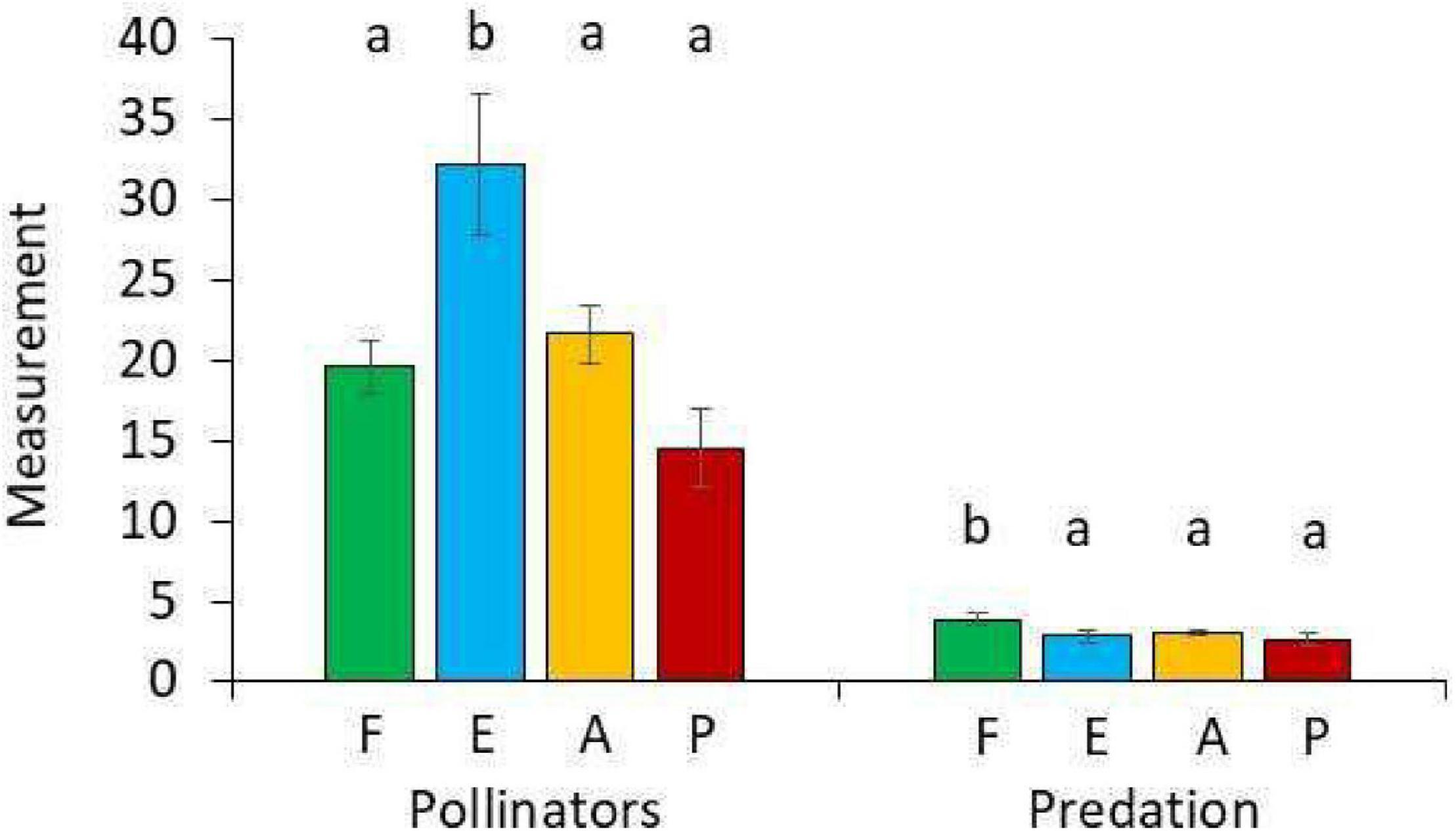
Figure 2. Average numbers of pollinators (all color traps combined) and bite marks (arthropod predation) in the forest (F, green), the forest edge (E, blue), the agricultural fields (A, orange), and the tree plantation (P, red). Different letters above the bars denote statistically significant differences in pair-wise U-tests after one-way Kruskal–Wallis ANOVA at P < 0.05. Error bars denote standard errors.
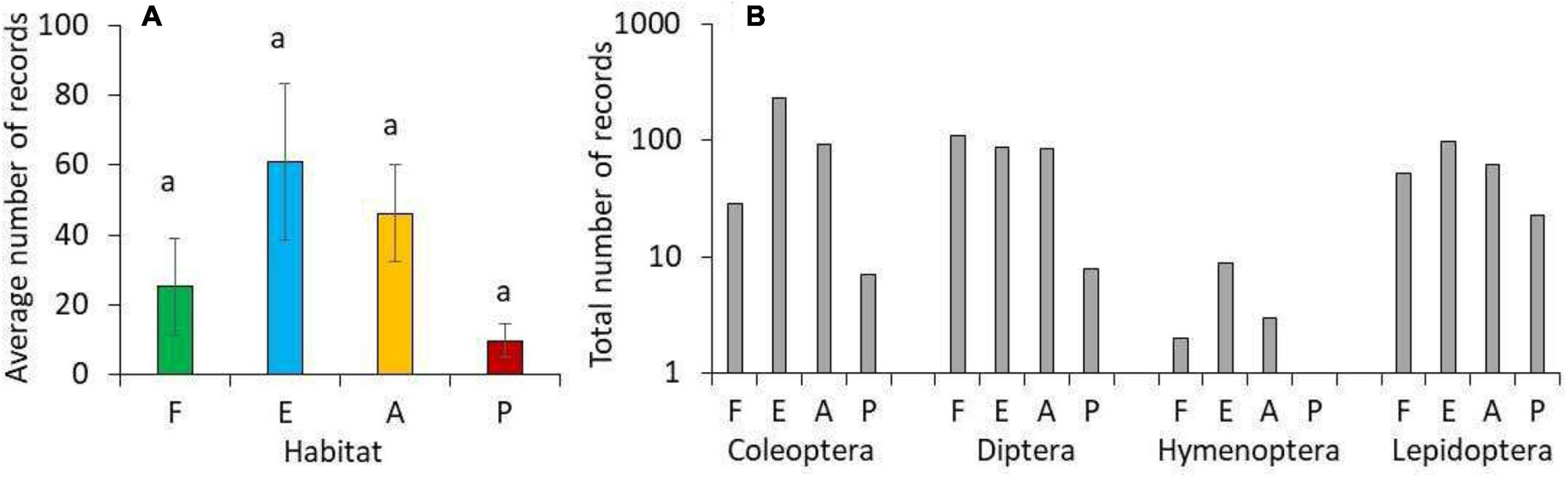
Figure 3. (A) Average numbers of insects recorded in light traps in the forest (F, green), the forest edge (E, blue), the agricultural fields (A, orange), and the tree plantation (P, red). Identical letters in (A) above the error bars indicate lack of statistically significant differences in pair-wise U-tests after one-way Kruskal–Wallis ANOVA at P < 0.05. Error bars denote standard errors. (B) Respective numbers of four major insect orders.
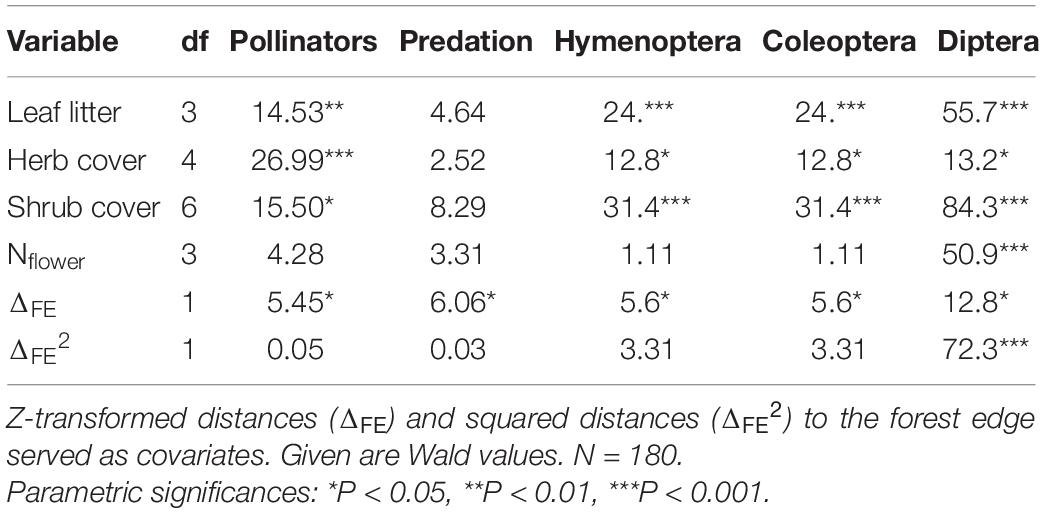
Table 2. Generalized linear modeling (Poisson error structure, linear link function) detected significant influences of important categorical habitat structures (leaf litter, herb, and shrub cover) on pollinator abundances but not on predation pressure (number of bite marks).
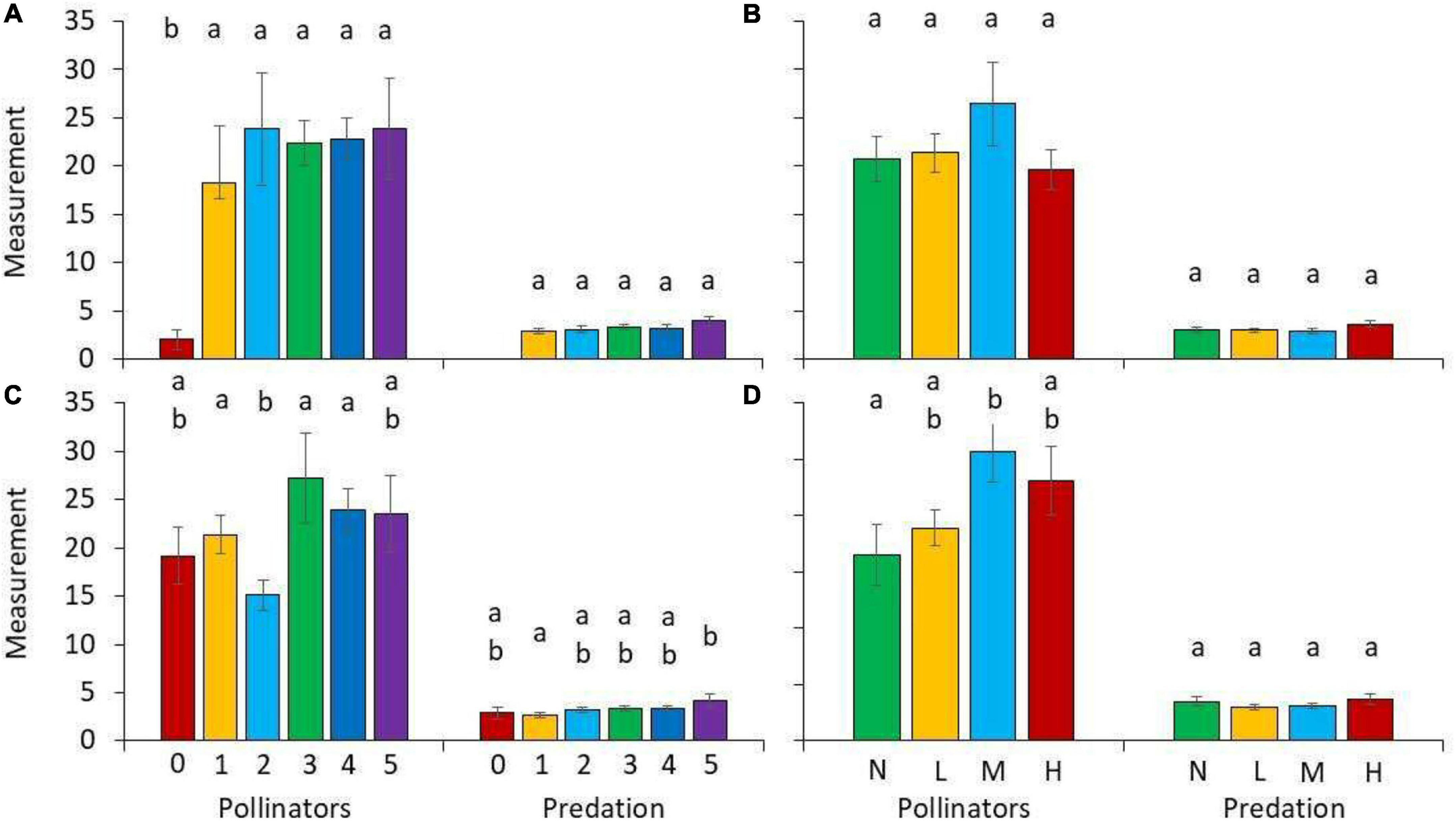
Figure 4. Average numbers of pollinator activity (all color traps combined) and arthropod predation (bite marks) in dependence on the fraction of (A) herb cover, (B) leaf litter, (C) shrub cover (0: 0%, 1: 1–24%, 2: 25–49%, 3: 50–74%, 4: 75–99%, and 5: 100%), and (D) number of blossoms (none, low, medium, high). Different letters above the bars denote statistically significant differences in pair-wise U-tests after one-way Kruskal–Wallis ANOVA at P < 0.05. Error bars denote standard errors.
We further looked at the variability of the measured function within each habitat type (Figure 5). We found relatively low coefficients of variation of CV < 1 indicating a lower within habitat variability than expected from a Poisson distribution. For the two measures (pollination and predation), variability was highest within the cloud forest and lowest in the exotic tree plantations (Figure 5). All raw data (pollinator activity, predation rate, flight interception traps, and environmental parameters) are given in Supplementary Appendix 1–4.
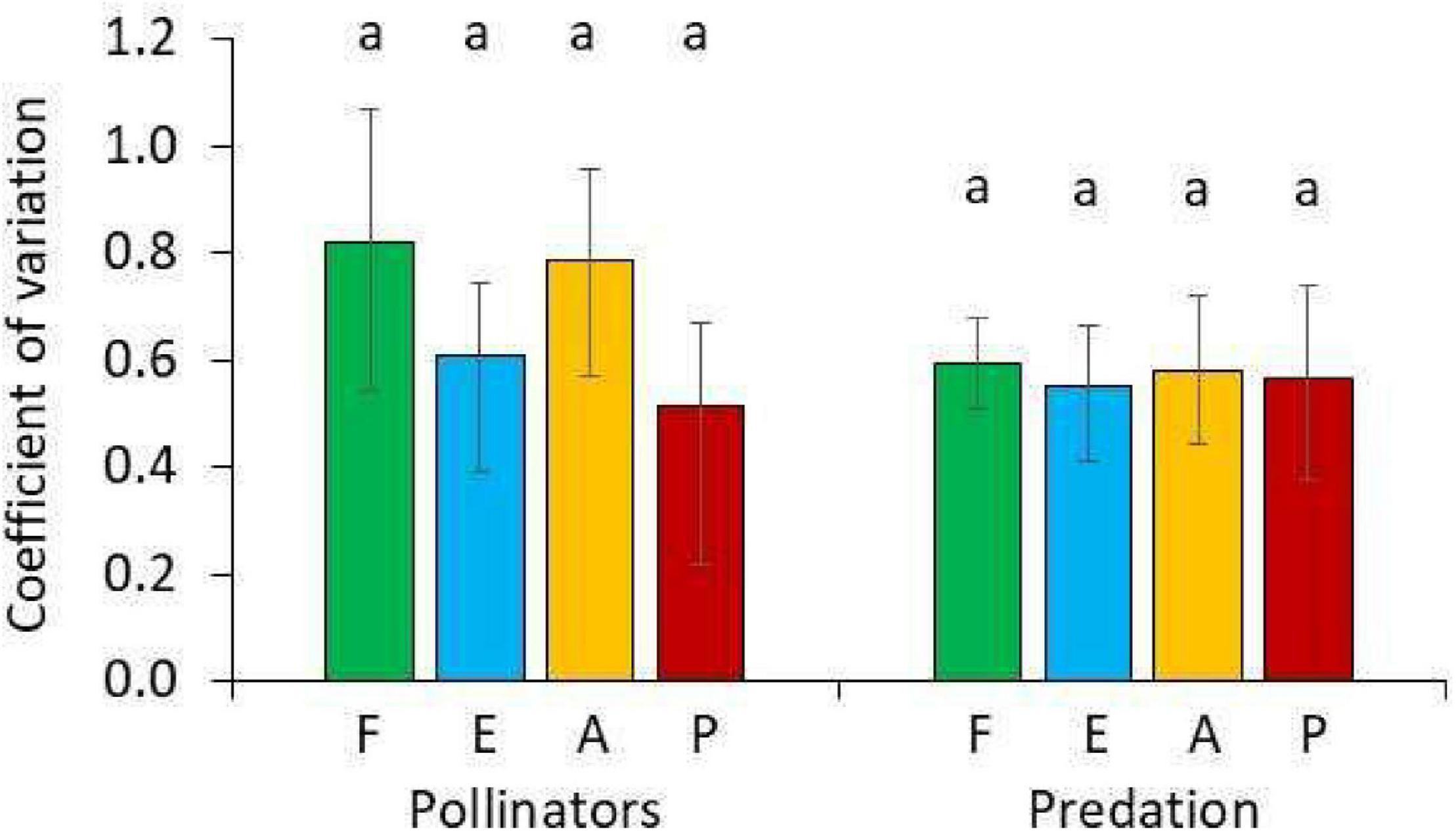
Figure 5. Coefficients of the variability of pollinator activity (all color traps combined) and arthropod predation (bite marks) in the forest (F, green), the forest edge (E, blue), the agricultural fields (A, orange), and the tree plantation (P, red). Identical letters in above the bars indicate lack of statistically significant differences in pair-wise U-tests after one-way Kruskal–Wallis ANOVA at P < 0.05. Error bars denote bootstrapped 95% confidence limits.
Finally we tested for significant spill over effects of ecosystem functions, from the forest into the open agricultural lands. Plots of numbers of insect bites in the dummy larvae (Figure 6A) and numbers of individuals of flying arthropods in pan traps revealed a marked although statistically insignificant trend [ANOVA: P(F8,171) > 0.1] of increased bites and Hymenoptera individuals 8 and 16 m inside the agricultural land, and higher predation rates inside the forest than in open agroecosystems. Numbers of Diptera peaked around the forest edge while Coleoptera numbers increased toward the agricultural land (Figure 6B).
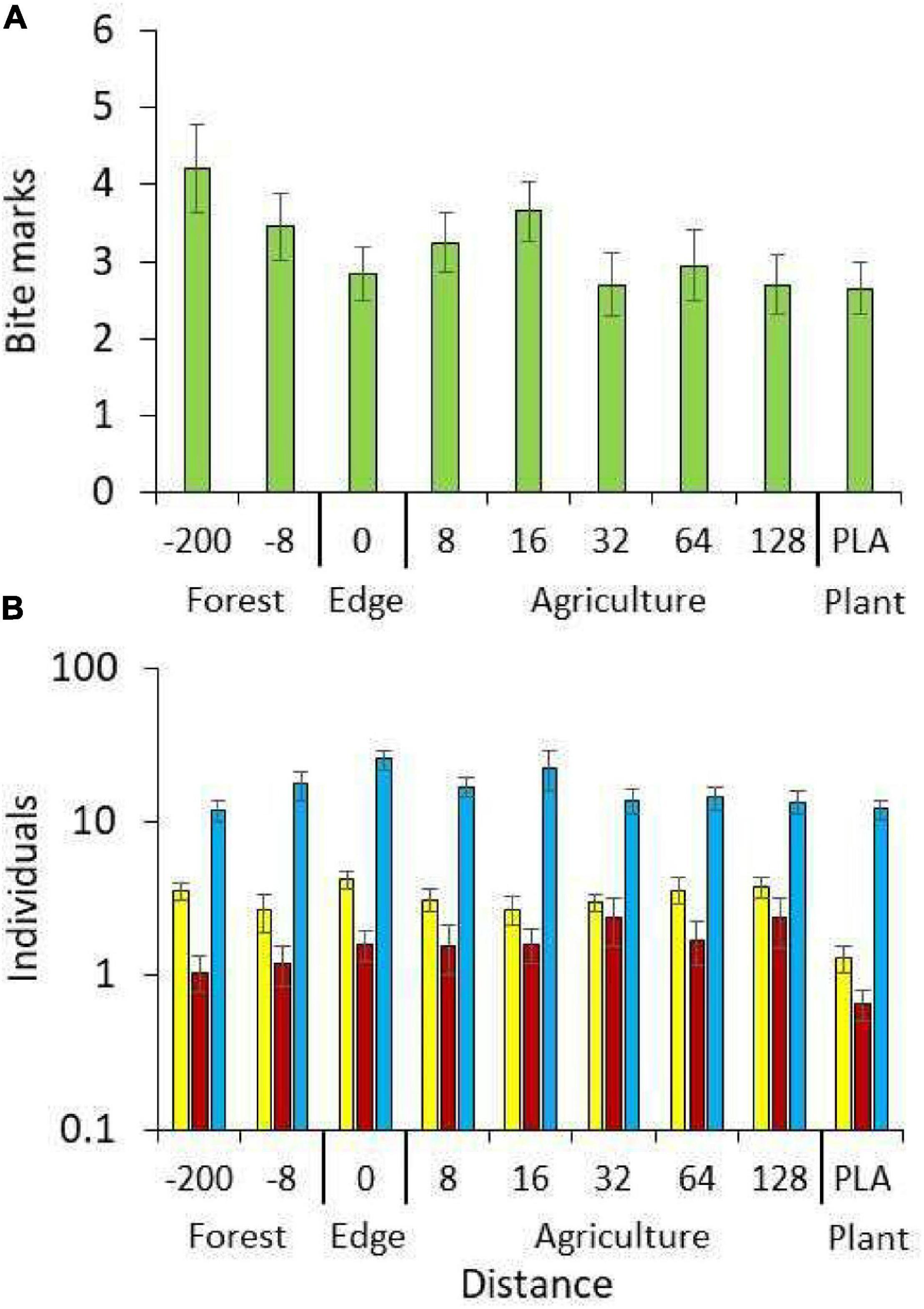
Figure 6. Mean numbers of dummy bitemarks (A) and individuals caught with pan traps (B: yellow: Hymenoptera, brown: Coleoptera, blue: Diptera) in dependence on distance to the forest. Plantation data (Plant) are shown separately. Error bars denote standard errors.
Discussion
We found a trend toward higher predation rates in heterogeneous and natural cloud forests than in the surrounding agroecosystems (with lowest rates in tree plantations). Bite marks were mainly from insects (ants), but also from small mammals (exclusively inside the forest). We conclude that the abundance of arthropods (mainly ants) is significantly higher inside natural forest than across the disturbed agricultural landscape. The obtained higher level of predation inside of forest indicate a potential source for spill over into the adjoining agroecosystems. This phenomenon was already shown in previous studies (Klein et al., 2003, 2007; Ricketts, 2004; Tscharntke et al., 2008; Calvet-Mir et al., 2012; Habel and Ulrich, 2020) and interpreted as a positive effect on agricultural productivity. Predation may support the regulation of potential pest outbreaks, and thus is of high relevance for smallholder farmers and food-security (cf. Olfert et al., 2002; Haddad et al., 2009). Therefore, it has been increasingly recommended that small forest islands remain during forest clearings, to guarantee a minimum of ecological pest control function, and thus to preserve the resilience of landscapes (Oliver et al., 2015). We found no trend of potential spill over of pollinator activity from forest into agroecosystems, but higher pollination activity throughout the agricultural landscape. Most pollinators depend on ample sunlight, and are therefore found in open landscapes with an adequate supply of flowers (see below). This is the case in the extensively and heterogeneous agricultural landscapes of Taita Hills, consisting of small fields, fallow land, and gardens around housings. These results underline that the co-existence of various ecosystems accelerate ecosystem functions, as long as farming remains rather is not too intense (Lichtenberg et al., 2017), as long as the cultivation of the fields is largely extensive and small-scale.
Ecosystem Functions in Different Ecosystems
Our data show that arthropod diversity and ecosystem functions vary among different types of ecosystems, and show partly contradictory trends. Previous studies showed that different ecosystems provide different habitat structures and resources, and thus are home of various plant and animal species, which subsequently provide ecosystem functions (Weisser et al., 2017). Arthropod activity such as pollinator activity is strongly influenced by solar radiation, and therefore pollination activity is greatly reduced in shaded and dense forest, but increases abruptly at the forest edge, as shown by our data (pan-traps and flight interception traps). This trend goes in line with other studies showing that flying arthropods accumulate particularly at sunny and flower-rich spots, which are more frequently found in open agroecosystems (Nicholls and Altieri, 2013; Isbell et al., 2017), as well as along ecotones (here the transition from forest into agroecosystem). We found that predation rates are comparatively high inside the forest. This finding goes in line with results from other studies (Schwab et al., 2021), indicating key preconditions such as microhabitat structures and microclimate accelerating the abundance of arthropods (Schwab et al., 2021). Ground-dwelling arthropods are adversely negatively affected by frequent disturbances through farming activities and thus may build up lower abundances in agroecosystems (Smith et al., 2008; Pardon et al., 2019), which might be a reason of reduced bite marks of dummy caterpillars in our study.
Our data show that eucalyptus plantations provide lowest levels of biodiversity and ecosystem functions. The studied eucalyptus plantations are extremely homogeneous and of only little value to most native species. Thus, plantations of exotic eucalyptus represent only little basis for the development of most organisms (e.g. herbivorous arthropods, Zahn et al., 2009), and subsequently for other organisms at higher trophic levels, such as birds (Mulwa et al., 2021), and thus provide only little ecosystem functions (Pejchar and Mooney, 2009), as approved by our own data. Our results go in line with studies measuring the same proxies of ecosystem functions using the same techniques. The invasion of exotic plant species following ecosystem disturbance resulted in the homogenization of a once heterogeneous and species rich vegetation (Habel and Ulrich, 2021). Here, the spread of the exotic invasive Lantana camara shrub species along rivers in south-eastern Kenya resulted in collapse of biodiversity across all trophic levels (Habel et al., 2018), and negatively affect ecosystem functions (Habel and Ulrich, 2021). Similarly, in the case of Taita Hills, intense planting of eucalyptus trees caused shifts in abiotic conditions (such as hydrology and pH-value, see Bewket and Sterk, 2005; Chanie et al., 2013; Guzha et al., 2018), which may fundamentally modified species community structures (John and Kabigumila, 2007; Gardner et al., 2008; Martello et al., 2018; Allingham, 2020). Our results from the Taita Hills show that most plantations (especially when they consist of alien species) can result in drastic species loss (John and Kabigumila, 2007; Gardner et al., 2008), and severe reduction of ecosystem functions (Lemenih and Bongers, 2010; Omoro et al., 2010; Bayle, 2019). In consequence, the plantation of eucalyptus in Taita Hills does not represent suitable surrogate habitats for native plant and animal species.
Local Environmental Parameters and Ecosystem Functions
Our results show that local environmental conditions can influence and significantly increase local environmental functions. For example, pollination activity is positively influenced by the presence of vegetation and flowers. Some studies have already confirmed that landscape heterogeneity and the existence of flower rich sites and fallow land enhance ecosystem functions (cf. Isbell et al., 2017). In consequence, planting flowering plants (e.g., flowering areas and flowering strips) in agricultural landscapes is now a widely accepted tool to maintain and accelerate ecosystem function (e.g., pollination) which subsequently maximize crop yields (Garibaldi et al., 2014; Albrecht et al., 2020). In addition to attracting pollinators by offering flowers, it is necessary for numerous arthropods (including representatives from the groups of Hymenoptera and Diptera) to create fallow land for numerous arthropods with appropriate microhabitat structures (vegetation, bare soil) to support successful larval, i.e., species development.
From Theory to Practice
The results obtained and the correlations show evidence that the co-existence of various ecosystems represent different levels of biodiversity and provide different rates of ecosystem functions, and thus enhance the total value of ecosystem functions at the landscape level. Biodiversity and ecosystem functions can be specifically promoted by targeted ecological upgrading of small areas located in between agricultural land. Seeding flowering areas and flowering strips increases the attractiveness of landscapes, and the presence of pollinators – and thus may accelerate agricultural yields (Albrecht et al., 2020). In addition, the establishment of fallows (resting areas) as potential nurseries for numerous arthropods is essential for agroecosystems to be able to develop at all. The cultivation of exotic and invasive plant species (such as eucalyptus) do not represent a surrogate habitat for most forest specialist taxa and thus cause significant reductions in biodiversity and ecosystem functions, and the devastation of entire landscapes.
Data Availability Statement
The datasets presented in this study can be found in online repositories. The names of the repository/repositories and accession number(s) can be found in the article/Supplementary Material.
Ethics Statement
The animal study was reviewed and approved by NACOSTI.
Author Contributions
JH and MT developed ideas and the study set-up. TS, FM, FG, MT, and JH collected the data. WU performed statistical analyses. All authors contributed while data interpretation and writing.
Conflict of Interest
The authors declare that the research was conducted in the absence of any commercial or financial relationships that could be construed as a potential conflict of interest.
Publisher’s Note
All claims expressed in this article are solely those of the authors and do not necessarily represent those of their affiliated organizations, or those of the publisher, the editors and the reviewers. Any product that may be evaluated in this article, or claim that may be made by its manufacturer, is not guaranteed or endorsed by the publisher.
Acknowledgments
We thank Marianne Maghenda (Ngerenyi, Kenya) for project coordination and logistic support in the field. We thank the German Academic Exchange Service for funding (the Quality Network Biodiversity, Biocult). We are grateful for critical and fruitful comments by two reviewers on a draft version of this article.
Supplementary Material
The Supplementary Material for this article can be found online at: https://www.frontiersin.org/articles/10.3389/fevo.2022.816163/full#supplementary-material
References
Albrecht, M., Kleijn, D., Williams, N. M., Tschumi, M., Blaauw, B. R., Bommarco, R., et al. (2020). The effectiveness of flower strips and hedgerows on pest control, pollination services and crop yield: a quantitative synthesis. Ecol. Lett. 23, 1488–1498. doi: 10.1111/ele.13576
Allingham, S. (2020). The effects of habitat alteration on anuran diversity and assemblages on Mount Mulanje, Malawi. Afr. J. Wildlife Res. 50, 23–35.
Baohanta, R., Thioulouse, J., Ramanankierana, H., Prin, Y., Rasolomampianina, R., Baudoin, E., et al. (2012). Restoring native forest ecosystems after exotic tree plantation in Madagascar: combination of the local ectotrophic species Leptolena bojeriana and Uapaca bojeri mitigates the negative influence of the exotic species Eucalyptus camaldulensis and Pinus patula. Biol. Invasions 14, 2407–2421. doi: 10.1007/s10530-012-0238-5
Barrios, E., Valencia, V., Jonsson, M., Brauman, A., Hairiah, K., Mortimer, P. E., et al. (2018). Contribution of trees to the conservation of biodiversity and ecosystem services in agricultural landscapes. Int. J. Biodiv. Sci. Ecosys. Serv. Manage. 14, 1–16.
Bayle, G. K. (2019). Ecological and social impacts of eucalyptus tree plantation on the environment. J. Biodivers. Conserv. Bioresour. Manag. 5, 93–104. doi: 10.3329/jbcbm.v5i1.42189
Benjamin, A. O., Gibb, D. T., and Johnson, H. T. (2016). Effect of pests, pollinators and pest-control on crop yield within sub-Saharan Africa. Afr. J. Agri. 3, 221–233.
Bewket, W., and Sterk, G. (2005). Dynamics in land cover and its effect on stream flow in the Chemoga watershed, Blue Nile basin, Ethiopia. Hydrol. Process. 19, 445–458. doi: 10.1002/hyp.5542
Burgess, N. D., Butynski, T. M., Cordeiro, N. J., Doggart, N. H., Fjeldså, J., Howell, K. M., et al. (2007). The biological importance of the Eastern Arc Mountains of Tanzania and Kenya. Biol. Conservat. 134, 209–231. doi: 10.1016/j.biocon.2006.08.015
Calvet-Mir, L., Gómez-Baggethun, E., and Reyes-García, V. (2012). Beyond food production: Ecosystem services provided by home gardens. A case study in Vall Fosca, Catalan Pyrenees, Northeastern Spain. Ecol. Econ. 74, 153–160. doi: 10.1016/j.ecolecon.2011.12.011
Campbell, J. W., and Hanula, J. L. (2007). Efficiency of Malaise traps and colored pan traps for collecting flower visiting insects from three forested ecosystems. J. Insect. Conserv. 11, 399–408. doi: 10.1007/s10841-006-9055-4
Castro-Díez, P., Vaz, A. S., Silva, J. S., van Loo, M., Alonso, Á, Aponte, C., et al. (2019). Global effects of non-native tree species on multiple ecosystem services. Biol. Rev. Camb. Philos. Soc. 94, 1477–1501. doi: 10.1111/brv.12511
Chanie, T., Collick, A. S., Adgo, E., Lehmann, C. J., and Steenhuis, T. S. (2013). Eco-hydrological impacts of Eucalyptus in the semi humid Ethiopian Highlands: the Lake Tana Plain. J. Hydrol. Hydromech. 61, 21b–29b. doi: 10.2478/johh-2013-0004
Dainese, M., Martin, E. A., Aizen, M. A., Albrecht, M., Bartomeus, I., Bommarco, R., et al. (2019). A global synthesis reveals biodiversity-mediated benefits for crop production. Sci. Adv. 5:eaax0121. doi: 10.1126/sciadv.aax0121
Dawson, P. K., Park, S. E., Attwood, S. J., Jamnadass, R., Powell, W., Sunderland, T., et al. (2019). Contributions of biodiversity to the sustainable intensification of food production. Glob. Food Security 21, 23–37.
Ebeling, A., Hines, J., Hertzog, L. R., Lange, M., Meyer, S. T., Simons, N. K., et al. (2018). Plant diversity effects on arthropods and arthropod-dependent ecosystem functions in a biodiversity experiment. Basic Appl. Ecol. 26, 50–63. doi: 10.1016/j.baae.2017.09.014
Gardner, T. A., Hernández, M. I. M., Barlow, J., and Peres, C. A. (2008). Understanding the biodiversity consequences of habitat change: the value of secondary and plantation forests for neotropical dung beetles. J. Appl. Ecol. 45, 883–893. doi: 10.1111/j.1365-2664.2008.01454.x
Garibaldi, L. A., Carvalheiro, L. G., Leonhardt, S. D., Aizen, M. A., Blaauw, B. R., Isaacs, R., et al. (2014). From research to action: enhancing crop yield through wild pollinators. Front. Ecol. Environ. 12:439–447. doi: 10.1890/130330
Garibaldi L. A., Carvalheiro, L. G., Vaissière, B. E., Gemmill-Herren, B., Hipólito, J., Freitas, B. M., et al. (2016). Mutually beneficial pollinator diversity and crop yield outcomes in small and large farms. Science 351, 388–391. doi: 10.1126/science.aac7287
Guzha, A. C., Rufino, M. C., Okoth, S., Jacobs, S., and Nóbrega, R. L. B. (2018). Impacts of land use and land cover change on surface runoff, discharge and low flows: Evidence from East Africa. J. Hydrol. 15, 49–67. doi: 10.1016/j.ejrh.2017.11.005
Habel, J. C., and Ulrich, W. (2020). Ecosystem functions in natural and anthropogenic ecosystems across the East African coastal forest landscape. Biotropica 52, 598–607. doi: 10.1111/btp.12780
Habel, J. C., and Ulrich, W. (2021). Ecosystem functions in degraded riparian forests of southeastern Kenya. Ecol. Evol. 11, 12665–12675. doi: 10.1002/ece3.8011
Habel, J. C., Koc, E., Gerstmeier, R., et al. (2021). Insect diversity across an afro-tropical forest biodiversity hotspot. J. Insect Conserv. 25, 221–228.
Habel, J. C., Teucher, M., Ulrich, W., and Schmitt, T. (2018). Documenting the chronology of ecosystem health erosion along East African rivers. Rem. Sens. Ecol. Conserv. 4, 34–43. doi: 10.1002/rse2.55
Haddad, N. M., Crutsinger, G. M., Gross, K., Haarstad, J., Knops, J. M. H., and Tilman, D. (2009). Plant species loss decreases arthropod diversity and shifts trophic structure. Ecol. Lett. 12, 1029–1039. doi: 10.1111/j.1461-0248.2009.01356.x
Hohenthal, J. (2018). Local ecological knowledge in deteriorating water catchments : Reconsidering environmental histories and inclusive governance in the Taita Hills, Kenya. Helsinki: Helsingin yliopisto.
Hohenthal, J. M., Räsänen, M., Owidi, E., Andersson, B., Minoia, P., Pellikka, et al. (2015). Community and institutional perspectives on water management and environmental changes in the Taita Hills, Kenya. Helsinki: Helsingin yliopisto.
Horak, J. (2013). Effect of site level environmental variables, spatial autocorrelation and sampling intensity on Arthropod communities in an ancient temporate lowland woodland area. PLoS One 8:e81541. doi: 10.1371/journal.pone.0081541
Howe, A., Lövei, G. L., and Nachman, G. (2009). Dummy caterpillars as a simple method to assess predation rates on invertebrates in a tropical agroecosystem. Entomol. Exp. Applicata 131, 325–329. doi: 10.1111/j.1570-7458.2009.00860.x
IPBES (2019). “Global assessment report on biodiversity and ecosystem services of the Intergovernmental Science-Policy Platform on Biodiversity and Ecosystem Services,” in IPBES secretariat, eds E. S. Brondizio, J. Settele, S. Díaz, and H. T. Ngo (Bonn: IPBES), 1148. doi: 10.5281/zenodo.3831673
Isbell, F., Adler, P. R., Eisenhauer, N., Fornara, D., Kimmel, K., Kremen, C., et al. (2017). Benefits of increasing plant diversity in sustainable agroecosystems. J. Ecol. 105, 871–879. doi: 10.1111/1365-2745.12789
John, J. R. M., and Kabigumila, J. D. L. (2007). Impact of Eucalyptus plantations on the avian breeding community in the East Usambaras, Tanzania. Ostrich 78, 265–269. doi: 10.2989/OSTRICH.2007.78.2.23.103
Karp, D. S., Chaplin-Kramer, R., Meehan, T. D., Martin, E. A., DeClerck, F., Grab, H., et al. (2018). Crop pests and predators exhibit inconsistent responses to surrounding landscape composition. Proc. Nat. Acad. Sci. 115, E7863–E7870. doi: 10.1073/pnas.1800042115
Klein, A.-M., Cunningham, S. A., Bos, M., and Steffan-Dewenter, I. (2008). Advances in pollination ecology from tropical plantation crops. Ecology 89, 935–943. doi: 10.1890/07-0088.1
Klein, A.-M., Steffan-Dewenter, I., and Tscharntke, T. (2003). Pollination of Coffea canephora in relation to local and regional agroforestry management: Pollination of lowland coffee. J. Appl. Ecol. 40, 837–845.
Klein, A.-M., Vaissière, B. E., Cane, J. H., Steffan-Dewenter, I., Cunningham, S. A., Kremen, C., et al. (2007). Importance of pollinators in changing landscapes for world crops. Proc. Roy Soc. B 274, 303–313. doi: 10.1098/rspb.2006.3721
Koh, L. P., and Menge, D. N. L. (2005). Rapid Assessment of Lepidoptera Predation Rates in Neotropical Forest Fragments1. Biotropica 0:051207072004004. doi: 10.1111/j.1744-7429.2006.00114.x
Kollmann, J., Meyer, S. T., Bateman, R., Conradi, T., Gossner, M. M., Souza Mendonça, M., et al. (2016). Integrating ecosystem functions into restoration ecology-recent advances and future directions. Restor. Ecol. 24, 722–730. doi: 10.1111/rec.12422
Lamarque, F. (2009). Human-wildlife conflict in Africa: Causes, consequences and management strategies. Rome: Food and Agriculture Organization of the United Nations.
Lemenih, M., and Bongers, F. (2010). “The Role of Plantation Forests in Fostering Ecological Restoration: Experiences from East Africa,” in Degraded forests in Eastern Africa: Management and restoration, ed. F. Bongers (London: Earthscan).
Lichtenberg, E. M., Kennedy, C. M., Kremen, C., Batáry, P., Berendse, F., Bommarco, R., et al. (2017). A global synthesis of the effects of diversified farming systems on arthropod diversity within fields and across agricultural landscapes. Glob. Chang. Biol. 23, 4946–4957. doi: 10.1111/gcb.13714
Little, T. D. (ed.) (2013). The Oxford handbook of quantitative methods: Vol. 2: Statistical analysis. Oxford: Oxford University Press.
Loiselle, B. A., and Farji-Brener, A. G. (2002). What’s Up? An Experimental Comparison of Predation Levels between Canopy and Understory in a Tropical Wet Forest1. Biotropica 34, 327–330. doi: 10.1111/j.1744-7429.2002.tb00545.x
Maeda, E. E., Clark, B. J. F., Pellikka, P., and Siljander, M. (2010). Modelling agricultural expansion in Kenya’s Eastern Arc Mountains biodiversity hotspot. Agricult. Syst. 103, 609–620. doi: 10.1016/j.agsy.2010.07.004
Martello, F., Bello, F., de Morini, M. S. D. C., Silva, R. R., Souza-Campana, D. R., de Ribeiro, M. C., et al. (2018). Homogenization and impoverishment of taxonomic and functional diversity of ants in Eucalyptus plantations. Sci. Rep. 8:3266. doi: 10.1038/s41598-018-20823-1
Meyer, S. T., Koch, C., and Weisser, W. W. (2015). Towards a standardized Rapid Ecosystem Function Assessment (REFA). Trends Ecol. Evol. 30, 390–397. doi: 10.1016/j.tree.2015.04.006
Meyer, S. T., Leidinger, J. L. G., Gossner, M. M., and Weisser, W. W. (2017). Handbook of field protocols for using REFA methods to approximate ecosystem functions - Version 1.0. München: mediaTUM - Dokumenten- und Publikationsserver der Technischen Universität München.
Michelsen, A., Lisanework, N., Friis, I., and Holst, N. (1996). Comparisons of Understorey Vegetation and Soil Fertility in Plantations and Adjacent Natural Forests in the Ethiopian Highlands. J. Appl. Ecol. 33:627. doi: 10.2307/2404991
Millennium Ecosystem Assessment (2005). Ecosystems and human well-being: Synthesis. Washington, DC: Island Press.
Mittermeier, R. A., Turner, W. R., Larsen, F. W., Brooks, T. M., and Gascon, C. (2011). “Global Biodiversity Conservation: The Critical Role of Hotspots,” in Biodiversity Hotspots, eds F. E. Zachos and J. C. Habel (Heidelberg: Springer Berlin Heidelberg), 3–22.
Mulwa, M., Teucher, M., Ulrich, W., and Habel, J. C. (2021). Bird communities in a degraded forest biodiversity hotspot of East Africa. Biodivers. Conserv. 30, 2305–2318. doi: 10.1007/s10531-021-02190-y
Nadkarni, N. (1994). Diversity of species and interactions in the upper tree canopy of forest ecosystems. Am. Zool. 34, 70–78.
Naeem, S., Bunker, D. E., Hector, A., Loreau, M., and Perrings, C. (eds) (2010). Biodiversity, ecosystem functioning, and human wellbeing: An ecological and economic perspective. Oxford: Oxford Univ. Press.
Newmark, W. D. (1998). Forest Area, Fragmentation, and Loss in the Eastern Arc Mountains: Implications For the Conservation of Biological Diversity. J. East Afr. Nat. Hist. 87, 29–36.
Nicholls, C. I., and Altieri, M. A. (2013). Plant biodiversity enhances bees and other insect pollinators in agroecosystems. A review. Agron. Sustain. Dev. 33, 257–274. doi: 10.1007/s13593-012-0092-y
Norfolk, O., Jung, M., Platts, P. J., Malaki, P., Odeny, D., and Marchant, R. (2017). Birds in the matrix: the role of agriculture in avian conservation in the Taita Hills, Kenya. Afr. J. Ecol. 55, 530–540. doi: 10.1111/aje.12383
Nuttman, C. V., Otieno, M., Kwapong, P. K., Combey, R., Willmer, P., and Potts, S. G. (2011). The Utility of Aerial Pan-Trapping for Assessing Insect Pollinators Across Vertical Strata. J. Kansas Entomol. Soc. 84, 260–270. doi: 10.2317/JKES110319.1
O’Hara, R., and Kotze, J. (2010). Do not log-transform count data. Nat. Prec. 2010:1. doi: 10.1038/npre.2010.4136.1
Olfert, O., Johnson, G. D., Brandt, S. A., and Thomas, A. G. (2002). Use of Arthropod Diversity and Abundance to Evaluate Cropping Systems. Agron. J. 94, 210–216. doi: 10.2134/agronj2002.2100
Oliver, T. H., Heard, M. S., Isaac, N. J. B., Roy, D. B., Procter, D., Eigenbrod, F., et al. (2015). Biodiversity and Resilience of Ecosystem Functions. Trends Ecol. Evol. 30, 673–684. doi: 10.1016/j.tree.2015.08.009
Omoro, L. M. A., Pellikka, P. K. E., and Rogers, P. C. (2010). Tree species diversity, richness, and similarity between exotic and indigenous forests in the cloud forests of Eastern Arc Mountains, Taita Hills, Kenya. J. For. Res. 21, 255–264. doi: 10.1007/s11676-010-0069-0
Pardon, P., Reheul, D., Mertens, J., Reubens, B., Frenne, P., de, et al. (2019). Gradients in abundance and diversity of ground dwelling arthropods as a function of distance to tree rows in temperate arable agroforestry systems. Agricult. Ecosyst. Environ. 270-271, 114–128. doi: 10.1016/j.agee.2018.10.017
Pejchar, L., and Mooney, H. A. (2009). Invasive species, ecosystem services and human well-being. Trends Ecol. Evol. 24, 497–504. doi: 10.1016/j.tree.2009.03.016
Pellikka, P. K. E., Clark, B. J. F., Gosa, A. G., Himberg, N., Hurskainen, P., Maeda, E., et al. (2013). Agricultural Expansion and Its Consequences in the Taita Hills, Kenya. Kenya: Elsevier, 165–179.
Porto, R. G., Almeida, R. F., de, Cruz-Neto, O., Tabarelli, M., Viana, B. F., et al. (2020). Pollination ecosystem services: A comprehensive review of economic values, research funding and policy actions. Food Sec. 12, 1425–1442. doi: 10.1007/s12571-020-01043-w
Ricketts, T. H. (2004). Tropical forest fragments enhance pollinator activity in nearby coffee crops. Conserv. Biol. 18, 1262–1271. doi: 10.1111/j.1523-1739.2004.00227.x
Ruiz-Guerra, B., Renton, K., and Dirzo, R. (2012). Consequences of Fragmentation of Tropical Moist Forest for Birds and Their Role in Predation of Herbivorous Insects. Biotropica 44, 228–236. doi: 10.1111/j.1744-7429.2011.00795.x
Schmitt, T., Ulrich, W., Büschel, H., Bretzel, J., Gebler, J., Mwadime, L., et al. (2020). The relevance of cloud forest fragments and their transition zones for butterfly conservation in Taita Hills, Kenya. Biodivers. Conserv. 29, 3191–3207. doi: 10.1007/s10531-020-02017-2
Schwab, D., Wurz, A., Grass, I., Rakotomalala, A. A. N. A., Osen, K., Soazafy, M. R., et al. (2021). Decreasing predation rates and shifting predator compositions along a land-use gradient in Madagascar’s vanilla landscapes. J. Appl. Ecol. 58, 360–371. doi: 10.1111/1365-2664.13766
Scott, D. F., Bruijnzeel, L. A., and Mackensen, J. (2005). “The hydrological and soil impacts of forestation in the tropics,” in Forests, water, and people in the humid tropics: Past, present, and future hydrological research for integrated land and water management, eds M. Bonell and L. A. Bruijnzeel (Cambridge, UK: Cambridge University Press), 622–651.
Smith, J., Potts, S. G., Woodcock, B. A., and Eggleton, P. (2008). Can arable field margins be managed to enhance their biodiversity, conservation and functional value for soil macrofauna? J. Appl. Ecol. 45, 269–278. doi: 10.1111/j.1365-2664.2007.01433.x
Steffan-Dewenter, I., Klein, A. M., Alfert, T., Gaebele, V., and Tscharntke, T. (2006). “Bee diversity and plant-pollinator interactions in fragmented landscapes,” in Plant-pollinator interactions: from specialization to generalization, eds N. M. Waser and J. Ollerton (Chicago: University of Chicago Press), 387–407. doi: 10.1007/s004420050949
Tamburini, G., Bommarco, R., Wanger, T. C., Kremen, C., Van der Heijden, M. G. A., Liebman, M., et al. (2020). Agricultural diversification promotes multiple ecosystem services without compromising yield. Sci. Adv. 6, 1–8. doi: 10.1126/sciadv.aba1715
Tela, M., Cresswell, W., and Chapman, H. (2021). Pest-removal services provided by birds on subsistence farms in south-eastern Nigeria. PLoS One 16:e0255638. doi: 10.1371/journal.pone.0255638
Teucher, M., Schmitt, C. B., Wiese, A., Apfelbeck, B., Maghenda, M., Pellikka, P., et al. (2020). Behind the fog: Forest degradation despite logging bans in an East African cloud forest. Glob. Ecol. Conserv. 22:e01024. doi: 10.1016/j.gecco.2020.e01024
Tscharntke, T., Clough, Y., Wanger, T. C., Jackson, L., Motzke, I., Perfecto, I., et al. (2012). Global food security, biodiversity conservation and the future of agricultural intensification. Biol. Conserv. 151, 53–59. doi: 10.1016/j.biocon.2012.01.068
Tscharntke, T., Sekercioglu, C. H., Dietsch, T. V., Sodhi, N. S., Hoehn, P., and Tylianakis, J. M. (2008). Landscape constraints on functional diversity of birds and insects in tropical agroecosystems. Ecology 89, 944–951. doi: 10.1890/07-0455.1
Tschartke, T., Grass, I., Wanger, T. C., Westphal, C., and Batáry, P. (2021). Beyond organic farming – harnessing biodiversity-friendly landscapes. Trends Ecol. Evol. 36, 919–930. doi: 10.1016/j.tree.2021.06.010
Weisser, W. W., Roscher, C., Meyer, S. T., Ebeling, A., Luo, G., Allan, E., et al. (2017). Biodiversity effects on ecosystem functioning in a 15-year grassland experiment: Patterns, mechanisms, and open questions. Basic Appl. Ecol. 23, 1–73. doi: 10.1016/j.baae.2017.06.002
Westphal, C., Bommarco, R., Carré, G., Lamborn, E., Morison, N., Petanidou, T., et al. (2008). Measuring bee diversity in different European habitats and biogeographical regions. Ecol. Monogr. 78, 653–671. doi: 10.1890/07-1292.1
Wilson, J. S., Griswold, T., and Messinger, O. J. (2008). Sampling Bee Communities (Hymenoptera: Apiformes) in a Desert Landscape: Are Pan Traps Sufficient? J. Kansas Entomol. Soc. 81, 288–300. doi: 10.2317/JKES-802.06.1
Witt, A., Beale, T., and van Wilgen, B. W. (2018). An assessment of the distribution and potential ecological impacts of invasive alien plant species in eastern Africa. Transact. R. Soc. S Afr. 73, 217–236. doi: 10.1080/0035919X.2018.1529003
Keywords: pollination, predation, arthropod diversity, environmental conditions, ecosystem functions, habitat destruction, spill over, invasive exotic species
Citation: Seifert T, Teucher M, Ulrich W, Mwania F, Gona F and Habel JC (2022) Biodiversity and Ecosystem Functions Across an Afro-Tropical Forest Biodiversity Hotspot. Front. Ecol. Evol. 10:816163. doi: 10.3389/fevo.2022.816163
Received: 16 November 2021; Accepted: 04 January 2022;
Published: 14 February 2022.
Edited by:
Manuel B. Morales, Autonomous University of Madrid, SpainReviewed by:
Matteo Dainese, Eurac Research, ItalyStefan Siebert, North-West University, South Africa
Copyright © 2022 Seifert, Teucher, Ulrich, Mwania, Gona and Habel. This is an open-access article distributed under the terms of the Creative Commons Attribution License (CC BY). The use, distribution or reproduction in other forums is permitted, provided the original author(s) and the copyright owner(s) are credited and that the original publication in this journal is cited, in accordance with accepted academic practice. No use, distribution or reproduction is permitted which does not comply with these terms.
*Correspondence: Jan Christian Habel, amFuY2hyaXN0aWFuLmhhYmVsQHBsdXMuYWMuYXQ=