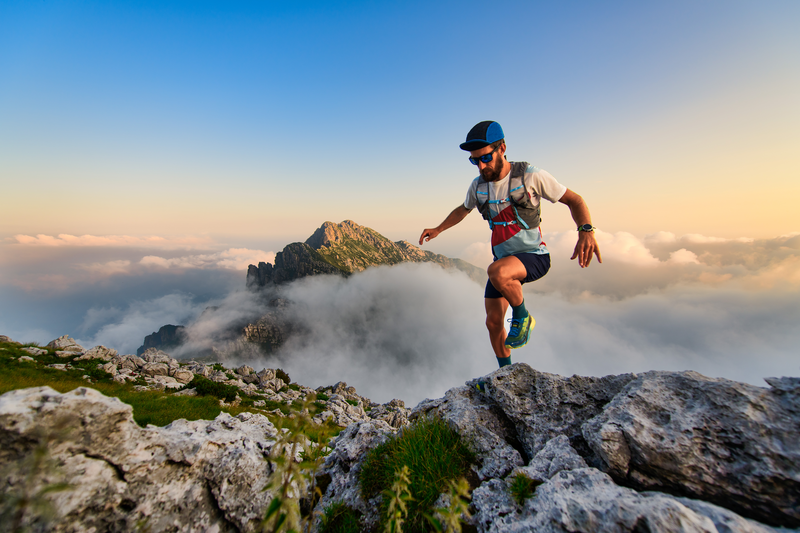
95% of researchers rate our articles as excellent or good
Learn more about the work of our research integrity team to safeguard the quality of each article we publish.
Find out more
ORIGINAL RESEARCH article
Front. Ecol. Evol. , 03 June 2022
Sec. Evolutionary Ecology of Social Behaviour
Volume 10 - 2022 | https://doi.org/10.3389/fevo.2022.813777
This article is part of the Research Topic Social Invertebrates as Models for Non-Kin Cooperation View all 12 articles
Evolutionary transitions from solitary to group-living are ubiquitous in animal systems. While the fitness consequences of group size changes are often investigated, the long-standing debate on whether kinship is a prerequisite of sociality is still ongoing. In the current study, we used kleptoparasitic spiders Argyrodes miniaceus (subfamily Argyrodinae, Theridiidae) as a model system to assess the role of group size on the foraging payoffs of kin and non-kin groups. We set up laboratory-manipulated kin and non-kin foraging groups and used feeding occurrence and duration as proxies for foraging benefits and feeding latency and the number of host attacks as estimates of foraging costs. Compared to solitary individuals, feeding durations of successfully fed individuals in groups was not significantly different from that of solitary foragers in both kin and non-kin groups. The occurrences of feeding decreased significantly in group sizes two and above, in non-kin groups, and in group sizes three and above, in kin groups. In kin groups, groups size two had significantly shorter feeding latencies compared to other group sizes, even though feeding duration did not change systematically with group size. Similarly, the number of attacks from the hosts were highest in non-kin groups with more than two individuals and in kin groups with more than three individuals. The juxtaposition of kin and non-kin group showed that A. miniaceus enjoyed the highest foraging payoffs when being solitary or in small groups (group size two). However, host attacks appeared to hamper feeding occurrences in kin groups, which was not observed in non-kin groups. Our results contrast sharply with the feeding benefits of kinship recorded in kin-based groups of sub-social species present in related subfamilies in the Theridiidae.
The evolutionary pathways from solitary to sociality in a given taxonomic group have been investigated with a wide range of approaches, including theoretical (West et al., 2007), physiological (Oliveira et al., 2015; Kingwell et al., 2021), genetic (French, 2016; Warner et al., 2019; Yan and Liebig, 2021), and experimental (Schneider and Bilde, 2008; Gow et al., 2019; Abdi et al., 2020a,b). During the early stages of sociality, i.e., when group-living and cooperation facultatively occurred in populations, it is clear that group size, a parameter related to social complexity (reviewed in Taborsky, 2021), could influence the fitness outcomes (Korb and Heinze, 2016; Brandell et al., 2021). While predictions of the theoretical models of group size effects on fitness have long been established (Giraldeau and Caraco, 2000), and the role of kinship (Giraldeau and Caraco, 1993; review of Platt and Bever, 2009) and other stochastic ecological effects, e.g., dispersal (Brown, 2016; Fernandez-Fournier and Avilés, 2018), have been discussed, it is important to apply these theories to the empirical animal systems. In group-living spiders, group size is an important determinant of fitness. Studies have reported that transitioning from solitary to certain group size would lead to broader dietary niches (Majer et al., 2018), weaker individual hunting ability (Harwood and Avilés, 2018), and the capture of larger prey (Guevara et al., 2011; Dumke et al., 2018). However, the importance of kinship in foraging has rarely been tested in group-living spiders (but see Auletta and Rayor, 2011; Yip and Rayor, 2013). Here, we used a group-living kleptoparasitic spider to test the foraging payoffs in different group sizes and to study the foraging outcomes in kin and non-kin groups depending on group size.
Kleptoparasitism refers to the behavior when an individual steals resources from other individuals (the same or different species). Kleptoparasitism reduces the amount of energy expended on foraging and has been reported in insects, spiders, birds, and mammals (reviewed in Iyengar, 2008). In the spider subfamily of Argyrodinae (Theridiidae), fewer than 20 of the named species (thus about 10% of the species) are group-living kleptoparasites (Whitehouse, 2011; Su and Smith, 2014). The group-living Argyrodes spiders use a variety of strategies to exploit the resources of their host. For the most part, these species live on the webs of their hosts (Su and Smith, 2014) or build a support web connected to the host’s web, as in the case of A. antipodiana (Whitehouse, 1986). These kleptoparasitic animals feed on prey captured by the hosts, as well as the silk of the host web. In a few instances, Argyrodes species have been shown to prey on their hosts or the host spiderlings (Silveira and Japyassú, 2012). Nonetheless, specific foraging strategies depend on ecological conditions and vary among species (Whitehouse, 2011). Some group-living Argyrodes species use a “creep-up-and-share” strategy, in which they approach the food that a host is feeding and consume prey partially digested by the host, thereby eliminating the need to produce digestive enzymes by themselves (Whitehouse, 1997; Whitehouse and Lubin, 2005). This feeding strategy presumably maximizes food resource intake in terms of quantity and quality across all feeding tactics (Whitehouse, 1997) and could thus potentially be used to quantify food intake. However, the role of group size in their foraging payoffs has not been tested. Some researchers have considered the tolerance of Argyrodes individuals when conducting “creep-up-and-share” feeding behavior as a form of cooperative foraging (Whitehouse, 2011). The Argyrodinae is a subfamily in the Theridiidae, which contains a number of sub-social species (e.g., genus Anelosimus) (Agnarsson, 2004) where sociality could have been driven by kin selection (Su and Smith, 2014; Liu et al., 2016). Kleptoparasitic behavior undoubtedly brings resource-driven foraging dynamics into play in the evolution of sociality (Su et al., 2021). In nature, Argyrodes has been shown to form kleptoparasitic groups with kin as well as non-kin individuals (Su et al., 2018); these species therefore provide excellent opportunities to simultaneously test the role of group size and the contribution of kinship in the foraging payoffs during the early-staged evolution of sociality.
Cooperative behaviors ranging from reciprocal mutualism in non-kin groups to caste-differentiated eusocial colonies (Wilson, 1975). However, the evolutionary processes leading to the transitioning from solitary to sociality remain at the center of ongoing debates (Nowak et al., 2010, 2017; Abbot et al., 2011; Herre and Wcislo, 2011; Birch, 2017). Most researchers are in agreement that cooperative behavior has produced a diversity of biological innovations; however, the issue of whether kinship is a necessary evolutionary driver of the development and maintenance of an early-staged cooperation has yet to be conclusively determined. Furthermore, researchers have yet to conclude whether inclusive fitness theory (Hamilton, 1964) is applicable to general cases of sociality or whether it is simply a special instance of natural selection (Nowak et al., 2010, 2017). Researchers have developed several theories to address this controversy (e.g., Garcia and De Monte, 2013; Liao et al., 2015; Nowak et al., 2017); however, there is a lack of empirical evidence testing the role of kinship in early-stage evolution of sociality in nature.
Our focal system, the group-living Argyrodes miniaceus and its host Nephila pilipes is an ideal natural system to study the evolution of cooperative behavior and test hypotheses pertaining to the role of kinship in an early evolutionary form of cooperative behavior and sociality (Whitehouse, 2011). In the current study, we sought to determine whether foraging payoffs differ across members of different group sizes. Specifically, we measured feeding duration, feeding latency, and attacks from hosts as three variables to quantify foraging payoffs. We predicted that, if the group-living behavior in A. miniaceus were of a cooperative nature, the highest foraging payoffs of this form of kleptoparasitism would occur at group size larger than one. Secondly, we compared the trends of foraging outcomes in kin and non-kin groups. We predicted that if kin selection played a role in cooperative foraging in A. miniaceus, then the optimal size of foraging groups would be greater for kin groups than for non-kin groups. Absent of the latter pattern would suggest that kin selection might not be important in the evolution of group living in these spiders.
Argyrodes miniaceus (Doleschall, 1857) is a group-living kleptoparasitic spider species specialized in the invasion and exploitation of the webs created by female orb-weaving hosts (Su et al., 2018), such as Nephila sp., including Nephila pilipes and Trichonephila clavata in Taiwan (personal observations of Yu in Namaxia, Kaohsiung, Taiwan). Nephila pilipes build two-dimensional orb webs where A. miniaceus forage, mate, and reproduce (personal observations of Yu); the orb web is made of frame threads as the basic structure, radial threads stretching from the central area of orb web (termed hub) to frame threads, and spiral threads connecting between radial threads (Wijerathna, 2016). In general, N. pilipes stay at hub to wait for prey, while A. miniaceus stay around the capture area composed of radial threads and spiral threads. Female A. miniaceus produce individual egg sacs in nearby vegetation or branches close to the host web. Male and female A. miniaceus both undergo four instars prior to maturation. In the field, it is common to find overlapping generations of A. miniaceus living in a web, i.e., adults, sub-adults, and juveniles of both sexes (first to third instars, Su et al., 2018) and the average group size is 4.8 ± 8.2 (Su et al., 2021). Argyrodes miniaceus utilizes four techniques when foraging on host webs: silk consumption, catching small insects, stealing wrapped food bundles from the host, and feeding with the hosts at the same time by creeping up to the food bundles (termed “creep-up-and-share”). Creep-up-and-share is their primary tactic among juvenile and adult A. miniaceus (usually two to three participants when conducting this feeding tactic, Su et al., 2018), indicating tolerance toward conspecifics during feeding; however, intraspecific aggression can still manifest in foraging as well as mating contexts. This unique form of group-living kleptoparasitism enables manipulation of kinship among group members in a laboratory setting.
We collected A. miniaceus (Araneae: Theridiidae) (referred as Argyrodes hereafter) and their hosts, female Nephila pilipes (Araneae: Nephilidae) (referred as Nephila hereafter), in Namaxia District of Kaohsiung in Taiwan (N 23° 16′ 17.9″, E 120° 43′ 33.3″), in which both species are commonly found. Juvenile and adult Argyrodes have been shown to share food with conspecifics; however, we focused exclusively on adult females for our experiments, due to the fact that foraging behavior is less observable in males. Adult female Argyrodes obtained from multiple host webs (i.e., minimal probability of sharing kinship) were used to assemble non-kin groups (Su et al., 2018). Kin groups were assembled by collecting egg sacs of Argyrodes in the field as well as those of spiders that had mated in the lab. Hatching involved attaching egg sacs to the side of 50 ml centrifuge tubes, at the bottom of which was placed moist cotton with a few drops of potable water for humidity. The tube opening was blocked using dry cotton to maintain suitable ventilation. Each egg sac contained 30–100 eggs, which hatched at room temperature after roughly 3 weeks (personal observations of Yu). The spiderlings were held in individual containers to prevent social interactions. At intervals of 2–3 days, the spiderlings were fed crickets that were partially digested by Nephila. After the Argyrodes spiderlings reached the third or fourth instar, they were reared in the web of Nephila in cages individually (BugDorm-1: 30 cm × 30 cm × 30 cm, BugDorm-6M1020 and 6E1020: 103 cm × 103 cm × 204 cm, MegaView Science Co., Ltd.) until they reached adulthood.
Female Nephila hosts were held in cages (103 cm × 103 cm × 204 cm, BugDorm-6M1020 and BugDorm-6E1020, MegaView Science Co., Ltd.) with sufficient space to build orb-webs. We removed Nephila individuals that were unable to complete a web after 1 day. Non-kin and kin groups of Argyrodes were assembled into groups of one to five individuals. Prior to initiating the experiments, Argyrodes were starved for at least for 48 h prior to be used in experiments but were fed ad libitum otherwise. For the sake of convenience in observation, individual Argyrodes were anesthetized using CO2 and then marked with paint splotches of various colors (Sharpe and Avilés, 2016; Luminous Powder Kit #1162A, BioQuip Products, Inc.). Experiment groups were assigned to cages randomly.
Experiments on non-kin groups were conducted from January 2019 to November 2020. Experiments on kin groups were conducted in March, April, and July of 2021. All experiments were conducted during the day. Each experimental session involved placing a live cricket (Gryllus bimaculatus) weighing ∼ 0.3 g (Robinson and Robinson, 1973) within the capture area of the Nephila host web. After capturing the prey, Nephila generally returned to the central area of the web (i.e., the hub) to manipulate the prey into food bundles. As soon as the Nephila began handling the prey, we started recording the feeding session using video cameras (Sony FDR-AX40 and HDR-PJ675, China). For each Argyrodes, we documented the feeding duration, feeding latency, and the number of attacks from Nephila, based on observations of the recordings. The variables were as follows:
1. Feeding duration: Feeding behavior of each Argyrodes was defined as the amount of time in which the mouthpart of Argyrodes was in direct contact with food bundles of Nephila. The length of feeding duration was used as a proxy for foraging benefit gained based on the “creep-up-and-share” strategy, wherein feeding duration using creep-up-and-share strategy is an important indicator of foraging benefit to gain body mass, and presumably contributes to later probalility of reproduction (see Whitehouse, 1997).
2. Feeding latency: Feeding latency was defined as the duration between the point at which Nephila began handling the prey and the point at which the mouthpart of each Argyrodes first came into contact with the prey. Feeding latency was used to estimate the foraging cost associated with the “creep-up-and-share” strategy. If a spider did not feed throughout the experiment, we assigned the latency a value of 20 min (the longest possible duration of the non-feeding category given the duration of the observations, see data analysis).
3. Number of host attacks: When Nephila detected the movements of Argyrodes, they either vibrated the web with their legs in a menacing manner (referred to as vibration hereafter) to expel Argyrodes away or captured Argyrodes directly (referred to as capture hereafter). We recorded the occurrences of vibration and capture as the number of attacks throughout the feeding session as another aspect of foraging cost associated with the “creep-up-and-share” strategy.
All statistical analyses were performed in R (v 4.0.2; R Core Team, 2020). Prior to any analyses, we square-root transformed feeding duration and cube-root transformed feeding latency. We further transformed raw feeding latency as the absolute difference from the maximum value (20 min). The transformed feeding latencies were zero-inflated but otherwise continuous, a structure best described by the Tweedie distribution (Tweedie, 1984). After the transformation, a value of 0 denoted maximum latency (i.e., an Argyrodes did not feed), and smaller values corresponded to longer raw latency. Data for feeding duration shared the same feature. We therefore used generalized linear models (GLMs) with Tweedie distributions (glmmTMB package; Magnusson et al., 2021) to perform subsequent analyses. We constructed separate linear models for feeding duration and feeding latency in non-kin and kin groups, respectively. In each linear model, we began by including group size, initial distance of each Argyrodes from the hub, the sum of vibrations and captures from the host (hereafter “attacks”), and ambient temperature as predictors. We did not detect significant collinearity among predictors based on visual inspection of data and variance inflation factor values (Supplementary Figure 1 and Supplementary Table 1; Zuur et al., 2008). We eliminated each predictor in turn and used Akaike Information Criteria (AIC) score difference from the full model (≤2) to evaluate the contribution of each predictor. We also tested models with two-way interactions, but those models were inferior in all cases (Model 6, Supplementary Tables 2, 3). Using GLMs with the Tweedie distribution, we constructed and tested two models: a zero-inflated model and a conditional model. In the zero-inflated model, the GLM examined the effect of each predictor in causing more zeros in the response variable. In the conditional model, the GLM tested the relationship of each predictor with non-zero values of the response variable, equivalent to a regular GLM.
When testing the effect of group size on vibrations and captures from the host, we employed generalized linear mixed models (GLMMs) with suitable distributions (Poisson, generalized Poisson, or negative binomial (Supplementary Tables 4A,C, 5A,C) using package lme4 and glmmTMB (Bates et al., 2015; Magnusson et al., 2021). In each GLMM, we included group size as a fixed variable and the identity of the Nephila host (host ID) as a random variable. We did not individually mark Nephila hosts in the earlier experiments, so we were unable to keep track of host identity in those experiments (Supplementary Table 7). Therefore, to gauge the influence of missing host IDs on statistical outcomes, we performed statistical analyses considering the identity of unidentifiable hosts in two extreme scenarios: (1) all unidentifiable hosts were assumed to be a sample of identified hosts. Under this scenario, we randomly assigned host IDs to unidentifiable Nephila hosts 100 times. (2) all unidentifiable hosts represented different individuals that did not overlap with any identified hosts. If the 101 models from the two scenarios were qualitatively similar in statistical outcomes, we would conclude that the influence of missing host ID did not affect the results.
For non-kin groups, the optimal model included all predictors except attacks from hosts (Model 5, Supplementary Table 2). Longer distance to the hub, lower temperatures, and a group size larger than one (with the exception of group size of four) were all significantly associated with zero feeding duration (Table 1A). However, none of the predictors explained the length of non-zero feeding duration in non-kin groups (Figure 1A and Table 1B).
Table 1. The statistical results of feeding duration in response to group size, kinship, distance to the hub, ambient temperature, and host attacks for kin and non-kin groups.
Figure 1. Feeding duration (in seconds, square root transformed) as a foraging benefit in different group sizes of (A) non-kin and (B) kin laboratory assembled groups. Results shown that under the conditional model, where the zeros were removed, the feeding duration for the successfully fed Argyrodes showed nonsignificant difference among group sizes, including with the largest group size five. The data in non-kin groups showed a trend toward a peak in feeding duration in group size two, whereas kin groups do not have this trend. The samples sizes were: Group size one: n = 12; group size two: n = 8 for non-kin group and n = 19 for kin group; group size three: n = 8 for non-kin group and n = 7 for kin group; group size four: n = 12 for non-kin group and n = 4 for kin group; group size five: n = 13 for non-kin group and n = 7 for kin group.
For kin groups, the full model and the model excluding distance to the hub were equally optimal, thus we report results from the full model here (Model 1, Supplementary Table 2). Similar to non-kin groups, longer distance to the hub, lower temperature, and a group size larger than two led to more zero feeding duration, even though the effect of distance was not significant (p = 0.065, Table 1C). The confidence interval of the parameter for distance suggested the non-significance was likely the result of a smaller effect, rather than an uncertainty of parameter estimation (Table 1C). Fewer attacks from the host were also associated with more zero feeding duration. This result in kin groups reflected the fact that attacks resulted from foraging activities of Argyrodes (i.e., non-zero feeding duration), during which they were in closer proximity to the host and incurred more attacks. Once the Argyrodes began feeding, more attacks from the host resulted in shorter feeding duration (Table 1D). Feeding duration of group sizes two to five was not significantly different to that of group size one in both kin and non-kin groups (Figure 1B and Table 1D).
For non-kin groups, the model excluding attacks from the host was the optimal model (Model 5, Supplementary Table 3). As in feeding duration, longer distance, lower temperature, and a group size larger than one (with the exception of group size of four) were significantly associated with maximal latency (i.e., no feeding; Table 2A). Higher temperature was also significantly associated with shorter feeding latencies (Table 2B). Feeding latencies of group size two to five were not significantly different from that of group size one (Figure 2A and Table 2B).
Table 2. Statistical results of feeding latency in response to group size, kinship, distance to the hub, ambient temperature, and host attacks for kin and non-kin groups.
Figure 2. Feeding latency as a foraging cost of (A) non-kin and (B) kin groups in different group sizes. Latencies in the figure are expressed as the absolute difference from the maximum latency (20 min) after taking the cube-root of the raw data). Values of zero on the y-axis denote a raw latency of 20 min; larger values of transformed latency denote shorter raw latency. Results shown that under the conditional model, where cases of no feeding during the observation period (maximum latency) were excluded. While most of the comparisons of feeding latency across group sizes were not significant, group size two in kin groups showed longer feeding latencies, thus higher costs, than other group sizes. Because the data were cube transformed and further transformed raw feeding latency as the absolute difference from the maximum value, larger values here represent shorter latency. The sample sizes were: Group size one: n = 12; group size two: n = 8 for non-kin group and n = 19 for kin group; group size three: n = 8 for non-kin group and n = 7 for kin group; group size four: n = 12 for non-kin group and n = 4 for kin group; group size five: n = 13 for non-kin group and n = 7 for kin group (exclude the data of transformed feeding latency = 0). ** means significant difference between group size one and two at p < 0.01.
For kin groups, the full model and the model without distance were equally optimal, thus we report results from the full model (Model 1, Supplementary Table 3). In this conditional model, group size of two had shorter feeding latencies comparing to group size one (Figure 2B and Table 2C), again, no significance (p = 0.064, Table 2C) of distance was more likely due to a smaller effect. Higher temperature caused shorter feeding latencies. Groups consisting of two Argyrodes kin also had significantly shorter feeding latencies compared to other group sizes (Figure 2B and Table 2C).
In non-kin groups, the consensus from the 101 GLMMs showed that group sizes larger than two induced significantly more web vibrations from the host (the expelling behavior) than group size one (Supplementary Table 4B). Similarly, group sizes larger than three received significantly more web vibrations from the host in kin groups (Supplementary Table 4D). Group sizes did not have an effect on the number of captures by the host in both non-kin and kin groups (Supplementary Tables 5B,D). The results of the number of host attacks are summarized in Figure 3.
Figure 3. Numbers of Nephila attacks (the sum of vibrations and captures) as a foraging cost of (A) non-kin and (B) kin groups in different group sizes. Two kinds of attacks are included in the analyses. The vibration, which is a tactic for Nephila to chase Argyrodes away, occurred more frequently. The number of this kind of attacks increased when group size > 2 in non-kin groups (see also Supplementary Table 4B) and when group size > 3 in kin groups (Supplementary Table 4D). The number of captures, a tactic to kill the Argyrodes, occurred in very low frequency and showed no difference across group sizes (see also Supplementary Table 5). Group size one: n = 14; group size two: n = 8 for non-kin group and n = 13 for kin group; group size three: n = 6 for non-kin group and n = 6 for kin group; group size four: n = 4 for non-kin group and n = 6 for kin group; group size five: n = 5 for non-kin group and n = 5 for kin group.
The model selection results indicated that group size consistently contributed substantially to the occurrence of feeding (i.e., the results of zero-inflation model), feeding duration, and feeding latency (i.e., the results under conditional model) in both kin or non-kin groups. Other factors, i.e., Argyrodes distance to the hub, ambient temperature, and attacks of hosts, were also important. Our results demonstrated that the occurrences of feeding in Argyrodes depended on group size in both kin and non-kin groups (Tables 1A,C) but host attacks only hampered feeding occurrences in kin groups. Compared to solitary feeding, the occurrences of feeding decreased in group size two to five in non-kin groups (except group size four) and group size three to five in kin groups (Tables 1A,C). Among individuals that got to feed, their feeding duration and latency were not significantly affected by group sizes, with the exception that Argyrodes in group size two of kin groups did not wait as long before commencing feeding (Tables 1, 2 and Figure 2). In addition to group size, Argyrodes feeding activities were hampered by lower ambient temperature and a longer distance from the hub. The risks of being chased away by Nephila, i.e., the number of vibrations, the primary technique of host attack, increased in group size three to five in non-kin groups and in group size four to five in kin groups (Supplementary Tables 4B,D). In general, our results showed an interesting pattern that the occurrences of feeding in A. miniaceus decreased in larger groups. Once an Argyrodes individual started feeding, feeding duration did not differ with respect to group size. Accordingly, Argyrodes enjoyed the highest foraging payoffs when solitary compared to any group size. The only notable exceptions were individuals in non-kin groups of size four and kin groups of size two, which had similar foraging payoffs to those of solitary individuals (Tables 1A,C).
We demonstrated experimentally that the per capita foraging payoffs of Argyrodes decreased with groups of any size compared to solitary individuals. Since resource size was fixed in our experiments, per capita foraging payoffs decreased when there were more foragers in a group. This result aligned with the field observations where the resource size was a determinant of group size in Argyrodes (Su et al., 2021). In natural populations, the average group size of A. miniaceus is 4.8 ± 8.2 (Su et al., 2021), yet we showed that individuals foraging in groups would suffer lower foraging payoffs (Table 1D) and more host attacks (Supplementary Table 4D). Therefore, the results of our experimental setup using the average size of food resource (0.3 g of food, Robinson and Robinson, 1973) did not correspond to the observed average group size in the natural populations. There are several possible mechanisms that would maintain an average group size of ∼5 individuals in the field. The first mechanism is the limited dispersal model, in which group-living results as a consequence of a potentially high cost of dispersal as in some social spiders (reviewed in Whitehouse and Lubin, 2005; Avilés and Guevara, 2017). For Argyrodes spiders, Nephila webs represent rare resource patches (Su et al., 2021). Spiders born on the same host web might therefore be forced to tolerate one another, even though foraging payoffs may be lower than when spiders are solitary on a web. Under this scenario, creep-up-and-share dynamic might evolve such that each member on the web takes turns distracting the host and allowing other members to feed more safely. The producer-scrounger model could be an alternative explanation for such group-living behavior in spiders (i.e., crab spiders, Dumke et al., 2016). This model predicts a group-living outcome because each individual may have the opportunity of being the producer (i.e., the first Argyrodes to locate and feed on the trapped prey on the host’s web), which enjoys higher feeding payoffs than the scroungers. In our experiments, spiders that fed first did have longer feeding duration both in kin and non-kin groups, even though the difference did not reach statistical significance (feeding duration in kin group: 25.59 vs. 21.35, t = 1.24, df = 26.07, p = 0.23; non-kin group 28.00 vs. 22.64, t = 1.95, df = 29.37, p = 0.06; Supplementary Figure 2). Further tests on dispersal costs, as well as the interactions between Argyrodes spiders and their hosts would help assess the validity of these hypotheses.
Group size in Argyrodes species tends to be positively correlated with food abundance (Cangialosi, 1990a,b; Agnarsson, 2003, 2011; Su et al., 2021). However, the contribution of kinship in promoting group-living has been inconclusive. Although living with kin can sometimes be beneficial (reviewed in Taborsky et al., 2021), the opposite has also been observed in a wide range of taxa (e.g., Zöttl et al., 2013; Dunn et al., 2014; Foster and Briffa, 2014; Thompson et al., 2017; Schweinfurth and Taborsky, 2018). In Argyrodes spiders, individuals sharing a web in the field could have higher relatedness than what would be expected by chance (Su et al., 2018), which could support the hypothesis that forming foraging groups with kin further increases fitness. Our results revealed that, regardless of kinship, groups of any size suffered higher foraging costs than solitary individuals, and that relatedness among members did not lead to larger optimal group size (Figure 3 and Supplementary Tables 4B,D). Argyrodes spiders are belong to the same family to the sub-social Theridiidae spiders (Agnarsson, 2004), and it has been hypothesized that Argyrodes spiders may represent a very early stages of sub-sociality (Whitehouse, 2011). However, our findings suggested that kin selection hypotheses, the explanation for some social spiders (see Schneider and Bilde, 2008; Yip and Rayor, 2013; Ruch et al., 2014; review in Yip and Rayor, 2014), may not apply in our system.
We used feeding duration, feeding latency, and risk of being attacked by hosts to estimate the foraging payoffs of Argyrodes. Overall, our results indicated that group living may not provide much (if any) foraging benefit, and kinship did not affect group size-foraging payoff dynamics. From our field observation, if an adult female A. miniaceus fed successfully using creep-up-and-share tactic in a feeding trial, it could have enough reproductive energy to produce egg sacs (Yu personal observation). It would be necessary to conduct further work on other group-living Argyrodinae species to verify the appropriateness of using foraging payoffs as a fitness proxy. Moreover, due to the experimental design our non-kin and kin groups might also differ in aspects other than kinship (e.g., prior social experience), which prevented us from statistically examining the effect of kinship on group formation. However, the fact that group size negatively influenced foraging payoffs in both kin and non-kin groups was still noteworthy. Further experiments using individuals with the same social experiences and in similar development stages are required to fully test the role of kinship in facilitating or deterring group-living in these spiders. We also advocate Argyrodes spiders to be a tractable model system with which to test more hypotheses regarding the origin of sociality, e.g., sibling cooperation and parent-offspring competition, that are not often considered in empirical studies (see example of earwigs, Kramer et al., 2015 and review in Kramer and Meunier, 2019).
The original contributions presented in the study are included in the article/Supplementary Material, further inquiries can be directed to the corresponding author.
C-NY conducted fieldwork, lab work, and wrote the methods and results. Y-CS designed and supervised the project, acquired the facility, and conducted the writing. C-YK designed and conducted the data analyses and conducted writing of the methods and results. H-CL assisted the analyses and edited manuscript. All authors contributed to the article and approved the submitted version.
This work was supported by the Ministry of Science and Technology (MOST), Taiwan to Y-CS (funding numbers MOST 107-2311-B-037-004-MY3; 110-2621-B-037-001-MY3). The fieldwork also supported by the University Social Responsibility (conducted in Namaxia, Kaohsiung, Taiwan) funding to Kaohsiung Medical University, Taiwan.
The authors declare that the research was conducted in the absence of any commercial or financial relationships that could be construed as a potential conflict of interest.
All claims expressed in this article are solely those of the authors and do not necessarily represent those of their affiliated organizations, or those of the publisher, the editors and the reviewers. Any product that may be evaluated in this article, or claim that may be made by its manufacturer, is not guaranteed or endorsed by the publisher.
We thank the members of Ecology and Evolutionary Genomics laboratory at the Kaohsiung Medical University for assistance in this research. We also thank Namaxia District Office, Kaohsiung, Taiwan to provide the facility for behavioral experiments.
The Supplementary Material for this article can be found online at: https://www.frontiersin.org/articles/10.3389/fevo.2022.813777/full#supplementary-material
Abbot, P., Abe, J., Alcock, J., Alizon, S., Alpedrinha, J. A., Andersson, M., et al. (2011). Inclusive fitness theory and eusociality. Nature 471, E1–E4. doi: 10.1038/nature09831
Abdi, M. K., Lupi, D., Jucker, C., and Hardy, I. C. W. (2020a). Kinship effects in quasi-social parasitoids I: co-foundress relatedness and host dangerousness interactively affect host exploitation. Biol. J. Linn. Soc. Lond. 130, 627–641. doi: 10.1093/biolinnean/blaa046
Abdi, M. K., Hardy, I. C. W., Jucker, C., and Lupi, D. (2020b). Kinship effects in quasi-social parasitoids II: co-foundress relatedness and host dangerousness interactively affect host exploitation. Biol. J. Linn. Soc. Lond. 130, 642–660. doi: 10.1093/biolinnean/blaa047
Agnarsson, I. (2003). Spider webs as habitat patches—the distribution of kleptoparasites (Argyrodes, Theridiidae) among host webs (Nephila, Tetragnathidae). J. Arachnol. 31, 344–349. doi: 10.1636/s02-21
Agnarsson, I. (2004). Morphological phylogeny of cobweb spiders and their relatives (Araneae, Araneoidea, Theridiidae). Zool. J. Linn. Soc. 141, 447–626. doi: 10.1111/j.1096-3642.2004.00120.x
Agnarsson, I. (2011). Habitat patch size and isolation as predictors of occupancy and number of argyrodine spider kleptoparasites in Nephila webs. Sci. Nat. 98, 163–167. doi: 10.1007/s00114-010-0750-3
Auletta, A., and Rayor, L. S. (2011). Preferential prey sharing among kin not found in the social huntsman spider, Delena cancerides (Araneae: Sparassidae). J. Arachnol. 39, 258–262. doi: 10.1086/709106
Avilés, L., and Guevara, J. (2017). “Sociality in spiders,” in Comparative Social Evolution, eds D. R. Rubenstein and P. Abbot (Cambridge: Cambridge University Press), 188–223.
Bates, D., Mächler, M., Bolker, B., and Walker, S. (2015). Fitting linear mixed-effects models using lme4. J. Stat. Softw. 67, 1–48. doi: 10.18637/jss.v067.i01
Birch, J. (2017). The Philosophy of Social Evolution. Oxford: Oxford University Press. doi: 10.1093/oso/9780198733058.001.0001
Brandell, E. E., Dobson, A. P., Hudson, P. J., Cross, P. C., and Smith, D. W. (2021). A metapopulation model of social group dynamics and disease applied to Yellowstone wolves. Proc. Natl. Acad. Sci. U.S.A. 118:e2020023118. doi: 10.1073/pnas.2020023118
Brown, C. R. (2016). The ecology and evolution of colony-size variation. Behav. Ecol. Sociobiol. 70, 1613–1632.
Cangialosi, K. R. (1990a). Life cycle and behavior of the kleptoparasitic spider, Argyrodes ululans (Araneae, Theridiidae). J. Arachnol. 18, 347–358.
Cangialosi, K. R. (1990b). Social spider defense against kleptoparasitism. Behav. Ecol. Sociobiol. 27, 49–54.
Doleschall, L. (1857). Bijdrage tot de kennis der arachniden van den indischen archipel. Nat. Tijdschr. Voor Neder. Ind. 13, 339–434.
Dumke, M., Herberstein, M. E., and Schneider, J. M. (2016). Producers and scroungers: feeding-type composition changes with group size in a socially foraging spider. Proc. Biol. Sci. 283:20160114. doi: 10.1098/rspb.2016.0114
Dumke, M., Herberstein, M. E., and Schneider, J. M. (2018). Advantages of social foraging in crab spiders: groups capture more and larger prey despite the absence of a web. Ethology 124, 695–705.
Dunn, J., Dunn, D. W., Strand, M. R., and Hardy, I. C. (2014). Higher aggression towards closer relatives by soldier larvae in a polyembryonic wasp. Biol. Lett. 10:20140229. doi: 10.1098/rsbl.2014.0229
Fernandez-Fournier, P., and Avilés, L. (2018). Environmental filtering and dispersal as drivers of metacommunity composition: complex spider webs as habitat patches. Ecosphere 9:e02101.
Foster, N. L., and Briffa, M. (2014). Familial strife on the seashore: aggression increases with relatedness in the sea anemone Actinia equina. Behav. Processes 103, 243–245. doi: 10.1016/j.beproc.2014.01.009
French, J. A. (2016). Genes, dopamine pathways, and sociality in primates. Proc. Natl. Acad. Sci. U.S.A. 113, 6325–6327. doi: 10.1073/pnas.1605972113
Garcia, T., and De Monte, S. (2013). Group formation and the evolution of sociality. Evol. Int. J. Org. Evol. 67, 131–141. doi: 10.1111/j.1558-5646.2012.01739.x
Giraldeau, L. A., and Caraco, T. (1993). Genetic relatedness and group size in an aggregation economy. Evol. Biol. 7, 429–438.
Giraldeau, L. A., and Caraco, T. (2000). Social Foraging Theory. Princeton, NJ: Princeton University Press. doi: 10.2307/2680211
Gow, E. A., Arcese, P., Dagenais, D., Sardell, R. J., Wilson, S., and Reid, J. M. (2019). Testing predictions of inclusive fitness theory in inbreeding relatives with biparental care. Proc. R. Soc. B Biol. Sci. 286:20191933. doi: 10.1098/rspb.2019.1933
Guevara, J., Gonzaga, M. O., Vasconcellos-Neto, J., and Avilés, L. (2011). Sociality and resource use: insights from a community of social spiders in Brazil. Behav. Ecol. 22, 630–638. doi: 10.1093/beheco/arr022
Hamilton, W. D. (1964). The genetical evolution of social behaviour. I & II. J. Theor. Biol. 7, 1–52.
Harwood, G., and Avilés, L. (2018). The shortfall of sociality: group-living affects hunting performance of individual social spiders. Behav. Ecol. 29, 1487–1493.
Herre, E. A., and Wcislo, W. T. (2011). In defence of inclusive fitness theory. Nature 471, E8–E9. doi: 10.1038/nature09835
Iyengar, E. V. (2008). Kleptoparasitic interactions throughout the animal kingdom and a re-evaluation, based on participant mobility, of the conditions promoting the evolution of kleptoparasitism. Biol. J. Linn. Soc. Lond. 93, 745–762. doi: 10.1111/j.1095-8312.2008.00954.x
Kingwell, C., Böröczky, K., Steitz, I., Ayasse, M., and Wcislo, W. (2021). Cuticular and dufour’s gland chemistry reflect reproductive and social state in the facultatively eusocial sweat bee Megalopta genalis (Hymenoptera: Halictidae). J. Chem. Ecol. 47, 420–432. doi: 10.1007/s10886-021-01262-1
Korb, J., and Heinze, J. (2016). Major hurdles for the evolution of sociality. Annu. Rev. Entomol. 61, 297–316. doi: 10.1146/annurev-ento-010715-023711
Kramer, J., and Meunier, J. (2019). The other facets of family life and their role in the evolution of animal sociality. Biol. Rev. 94, 199–215. doi: 10.1111/brv.12443
Kramer, J., Thesing, J., and Meunier, J. (2015). Negative association between parental care and sibling cooperation in earwigs: a new perspective on the early evolution of family life? J. Evol. Biol. 28, 1299–1308. doi: 10.1111/jeb.12655
Liao, X., Rong, S., and Queller, D. C. (2015). Relatedness, conflict, and the evolution of eusociality. PLoS Biol. 13:e1002098. doi: 10.1371/journal.pbio.1002098
Liu, J., May-Collado, L. J., Pekár, S., and Agnarsson, I. (2016). A revised and dated phylogeny of cobweb spiders (Araneae, Araneoidea, Theridiidae): a predatory Cretaceous lineage diversifying in the era of the ants (Hymenoptera, Formicidae). Mol. Phylogenet. Evol. 94(Pt B), 658–675. doi: 10.1016/j.ympev.2015.09.023
Magnusson, A., Skaug, H., Nielsen, A., Berg, C., Kristensen, K., Maechler, M., et al. (2021). Generalized Linear Mixed Models using Template Model Builder. Available Online at: https://CRAN.R-project.org/package=glmmTMB (accessed January 22, 2022).
Majer, M., Holm, C., Lubin, Y., and Bilde, T. (2018). Cooperative foraging expands dietary niche but does not offset intra-group competition for resources in social spiders. Sci. Rep. 8:11828. doi: 10.1038/s41598-018-30199-x
Nowak, M. A., McAvoy, A., Allen, B., and Wilson, E. O. (2017). The general form of Hamilton’s rule makes no predictions and cannot be tested empirically. Proc. Natl. Acad. Sci. U.S.A. 114, 5665–5670. doi: 10.1073/pnas.1701805114
Nowak, M. A., Tarnita, C. E., and Wilson, E. O. (2010). The evolution of eusociality. Nature 466, 1057–1062. doi: 10.1038/nature09205
Oliveira, R. C., Oi, C. A., do Nascimento, M. M., Vollet-Neto, A., Alves, D. A., Campos, M. C., et al. (2015). The origin and evolution of queen and fertility signals in Corbiculate bees. BMC Evol. Biol. 15:254. doi: 10.1186/s12862-015-0509-8
Platt, T. G., and Bever, J. D. (2009). Kin competition and the evolution of cooperation. Trends Ecol. Evol. 24, 370–377.
R Core Team (2020). R: A Language and Environment for Statistical Computing. Vienna: R Foundation for Statistical Computing.
Robinson, M. H., and Robinson, B. (1973). Ecology and Behavior of the Giant Wood Spider Nephila maculata (Fabricius) in New Guinea. Washington, DC: Smithsonian Institution Press. doi: 10.5479/SI.00810282.149
Ruch, J., Herberstein, M. E., and Schneider, J. M. (2014). Families hunt more successfully: effect of group composition on hunting and communal feeding. Anim. Behav. 91, 171–178.
Schneider, J. M., and Bilde, T. (2008). Benefits of cooperation with genetic kin in a subsocial spider. Proc. Natl. Acad. Sci. U.S.A. 105, 10843–10846. doi: 10.1073/pnas.0804126105
Schweinfurth, M. K., and Taborsky, M. (2018). Relatedness decreases and reciprocity increases cooperation in Norway rats. Proc. R. Soc. B Biol. Sci. 285:20180035. doi: 10.1098/rspb.2018.0035
Sharpe, R. V., and Avilés, L. (2016). Prey size and scramble vs. contest competition in a social spider: implications for population dynamics. J. Anim. Ecol. 85, 1401–1410. doi: 10.1111/1365-2656.12559
Silveira, M. C., and Japyassú, H. F. (2012). Notes on the behavior of the kleptoparasitic spider Argyrodes elevatus (Theridiidae, Araneae). Rev. Etol. 11, 56–67.
Su, Y. C., Peng, P., Elgar, M. A., and Smith, D. R. (2018). Dual pathways in social evolution: population genetic structure of group-living and solitary species of kleptoparasitic spiders (Argyrodinae: Theridiidae). PLoS One 13:e0208123. doi: 10.1371/journal.pone.0208123
Su, Y. C., and Smith, D. R. (2014). Evolution of host use, group-living and foraging behaviours in kleptoparasitic spiders: molecular phylogeny of the Argyrodinae (Araneae : Theridiidae). Invertebr. Syst. 28, 415–431. doi: 10.1071/is14010
Su, Y. C., Wu, C. Y., Yang, C. H., Li, B. S., Moi, S. H., and Lin, Y. D. (2021). Machine learning data imputation and prediction of foraging group size in a kleptoparasitic spider. Mathematics 9:415. doi: 10.3390/math9040415
Taborsky, B. (2021). A positive feedback loop between sociality and social competence. Ethology 127, 774–789. doi: 10.1111/eth.13201
Taborsky, M., Cant, M. A., and Komdeur, J. (2021). The Evolution of Social Behaviof. Cambridge: Cambridge University Press.
Thompson, F. J., Cant, M. A., Marshall, H. H., Vitikainen, E. I., Sanderson, J. L., Nichols, H. J., et al. (2017). Explaining negative kin discrimination in a cooperative mammal society. Proc. Natl. Acad. Sci. U.S.A. 114, 5207–5212. doi: 10.1073/pnas.1612235114
Tweedie, M. C. K. (1984). “An index which distinguishes between some important exponential families,” in Proceedings of the Indian Statistical Institute Golden Jubilee International Conference, Statistics: Applications and New Directions, eds J. K. Ghosh and J. Roy (Calcutta: Indian Statistical Institute), 579–604. doi: 10.1016/j.pnpbp.2013.04.014
Warner, M. R., Qiu, L., Holmes, M. J., Mikheyev, A. S., and Linksvayer, T. A. (2019). Convergent eusocial evolution is based on a shared reproductive groundplan plus lineage-specific plastic genes. Nat. Commun. 10:2651. doi: 10.1038/s41467-019-10546-w
West, S. A., Griffin, A. S., and Gardner, A. (2007). Evolutionary explanations for cooperation. Curr. Biol. 17, R661–R672. doi: 10.1016/j.cub.2007.06.004
Whitehouse, M. E. A. (1986). The foraging behaviours of Argyrodes antipodiana (Theridiidae), a kleptoparasitic spider from New Zealand. N. Z. J. Zool. 13, 151–168. doi: 10.1080/03014223.1986.10422658
Whitehouse, M. E. A. (1997). The benefits of stealing from a predator: foraging rates, predation risk, and intraspecific aggression in the kleptoparasitic spider Argyrodes antipodiana. Behav. Ecol. 8, 665–667. doi: 10.1093/beheco/8.6.665
Whitehouse, M. E. A. (2011). “Kleptoparasitic spiders of the subfamily Argyrodinae: a special case of behavioural plasticity,” in Spider Behaviour: Flexibility and Versatility, ed. M. E. Herberstein (Cambridge: Cambridge Unversity Press), 348–386. doi: 10.1017/CBO9780511974496.011
Whitehouse, M. E. A., and Lubin, Y. (2005). The functions of societies and the evolution of group living: spider societies as a test case. Biol. Rev. 80, 347–361. doi: 10.1017/s1464793104006694
Wijerathna, T. (2016). An Investigation of the Variations in Morphometry and the Web Architecture of the Giant Wood Spider (Nephila pilipes) in Forest and Non-Forest Habitats in the Wet Zone of Sri Lanka. Master’s thesis. Colombo: University of Kelaniya. doi: 10.13140/RG.2.2.35074.27846
Wilson, E. O. (1975). Sociobiology: The New Synthesis. Cambridge, MA: Belknap Press of Harvard University Press.
Yan, H., and Liebig, J. (2021). Genetic basis of chemical communication in eusocial insects. Genes Dev. 35, 470–482. doi: 10.1101/gad.346965.120
Yip, E. C., and Rayor, L. S. (2013). The influence of siblings on body condition in a social spider: is prey sharing cooperation or competition? Anim. Behav. 85, 1161–1168.
Yip, E. C., and Rayor, L. S. (2014). Maternal care and subsocial behaviour in spiders. Biol. Rev. 89, 427–449. doi: 10.1111/brv.12060
Zöttl, M., Heg, D., Chervet, N., and Taborsky, M. (2013). Kinship reduces alloparental care in cooperative cichlids where helpers pay-to-stay. Nat. Commun. 4:1341. doi: 10.1038/ncomms2344
Keywords: group-living, optimal group size, foraging payoff, kleptoparasitism, kin selection
Citation: Yu C-N, Kuo C-Y, Lin H-C and Su Y-C (2022) Foraging Payoffs Change With Group Size in Kin and Non-kin Groups of an Argyrodinae Kleptoparasitic Spider, Argyrodes miniaceus. Front. Ecol. Evol. 10:813777. doi: 10.3389/fevo.2022.813777
Received: 12 November 2021; Accepted: 16 May 2022;
Published: 03 June 2022.
Edited by:
Leticia Aviles, University of British Columbia, CanadaReviewed by:
Michael Taborsky, University of Bern, SwitzerlandCopyright © 2022 Yu, Kuo, Lin and Su. This is an open-access article distributed under the terms of the Creative Commons Attribution License (CC BY). The use, distribution or reproduction in other forums is permitted, provided the original author(s) and the copyright owner(s) are credited and that the original publication in this journal is cited, in accordance with accepted academic practice. No use, distribution or reproduction is permitted which does not comply with these terms.
*Correspondence: Yong-Chao Su, eWNzdTUyN0BrbXUuZWR1LnR3, eWNzdTUyN0BnbWFpbC5jb20=
Disclaimer: All claims expressed in this article are solely those of the authors and do not necessarily represent those of their affiliated organizations, or those of the publisher, the editors and the reviewers. Any product that may be evaluated in this article or claim that may be made by its manufacturer is not guaranteed or endorsed by the publisher.
Research integrity at Frontiers
Learn more about the work of our research integrity team to safeguard the quality of each article we publish.