- Department of Biology, Misericordia University, Dallas, PA, United States
Studies on non-native epibionts typically focus on the organismal-level impacts of epibiosis on basibionts, rather than community-level impacts of this relationship. The purpose of our study was to evaluate if non-native basibionts in general facilitate invasions through epibiosis in Maine compared to native basibiont species. We collected 64 basibiont assemblages including replicate samples of 10 different basibiont taxa on the central Maine coast in October 2019. Each basibiont and associated epibionts were identified to genus, classified as native or non-native to the region where they were collected, and weighed. We found that while there was no association between invasion status of the epibiont and the basibiont, native basibionts had a significantly higher Shannon Diversity Index than non-native basibionts. Although diversity of epibionts was greater on native basibionts, the percentage of invaders varied across basibiont taxa. Specific basibiont taxon characteristics may be more important than status because different taxa have different surface topographies, resulting in varying settlement among epibiont species. Our study indicates that there is differential settlement of epibiont taxa across basibiont taxa, which may help predict, based on surface characteristics, which species support more epibiont taxa. This study, as a snapshot of floating dock fouling communities within a 10 km radius, may indicate that non-native basibionts play a role in changing community structure. Expanding the scope of this initial study to include a wider taxonomic and geographic range should help determine if epibiosis is truly a facilitative process in invasions.
Introduction
Novel ecosystems are the product of new combinations of native and non-native species, resulting in potential changes in ecosystem functions. These are the result of anthropogenic activity – intentional or inadvertent – and result from degradation or invasion of native ecosystems (Hobbs et al., 2006). Non-native species are a threat to marine biodiversity, and invasions have been found in over 84% of marine ecoregions (Molnar et al., 2008). Regions with low diversity may be at a higher risk for invasion, as well as regions with high numbers of transport vectors or disturbances (Cohen and Carlton, 1998).
A potential facilitative mechanism for invasions that has not been directly examined in the literature is epibiosis. Epibiosis occurs when basibionts, organisms that provide a habitable surface, are colonized by epibionts, the organisms that settle on basibiont surfaces (Wahl, 1989). In the Gulf of Maine, a non-native epibiont Membranipora membranacea indirectly facilitates the invasion by Codium fragile as the epibiont causes decreased growth and increased mortality in native kelp, a competitor for space, on which the bryozoan settles (Levin et al., 2002). Floerl et al. (2004) described the entrainment of propagules of other species by a non-native bryozoan on the hull of a boat covered in antifouling paint. The bryozoans provided an additional habitable space not otherwise available on the boat surface. Prenter et al. (2004) suggest that such facilitative interspecific interactions increase the success and impact of non-native species. However, there are few studies that look at the community-wide impacts of epibiosis, particularly whether epibiosis is a mechanism for facilitating invasion.
The purpose of this study is to analyze the impact of non-native species in marine ecosystems by focusing on invasion status, taxonomy, and frequency of associated epibionts on basibiont specimens at one location on the central coast of Maine. We aim to determine if facilitation via epibiosis is impacting community structure in a marine ecosystem in the Gulf of Maine. We ask if the invasion status of the basibiont impacts the frequency of settled non-native epibionts and if there are any differences in epibiont diversity on native and non-native basibionts. Our predictions are that, if non-native basibionts directly facilitate further invasion, we expect to see an increased frequency of non-native epibiont settlement on non-native basibionts. Because patterns in marine communities suggest there is a relationship between invasion and diversity (Stachowicz et al., 2002), we also predict that there may be a difference in species diversity on non-native basibionts versus native basibionts.
Materials and Methods
Study Sites
The samples (n = 64) used in this study were collected from floating docks at three sites in the Damariscotta estuary of Maine on October 11, 2019: a scallop farm at Peter’s Island (43°54′32.68″ N, 69°34′05.05″ W), South Bristol Fishermen’s Co-op (43°51′50.07″ N, 69°33′16.67″ W), and the Darling Marine Center (43°56′3.16″ N, 69°34′46.41″ W; Figure 1).
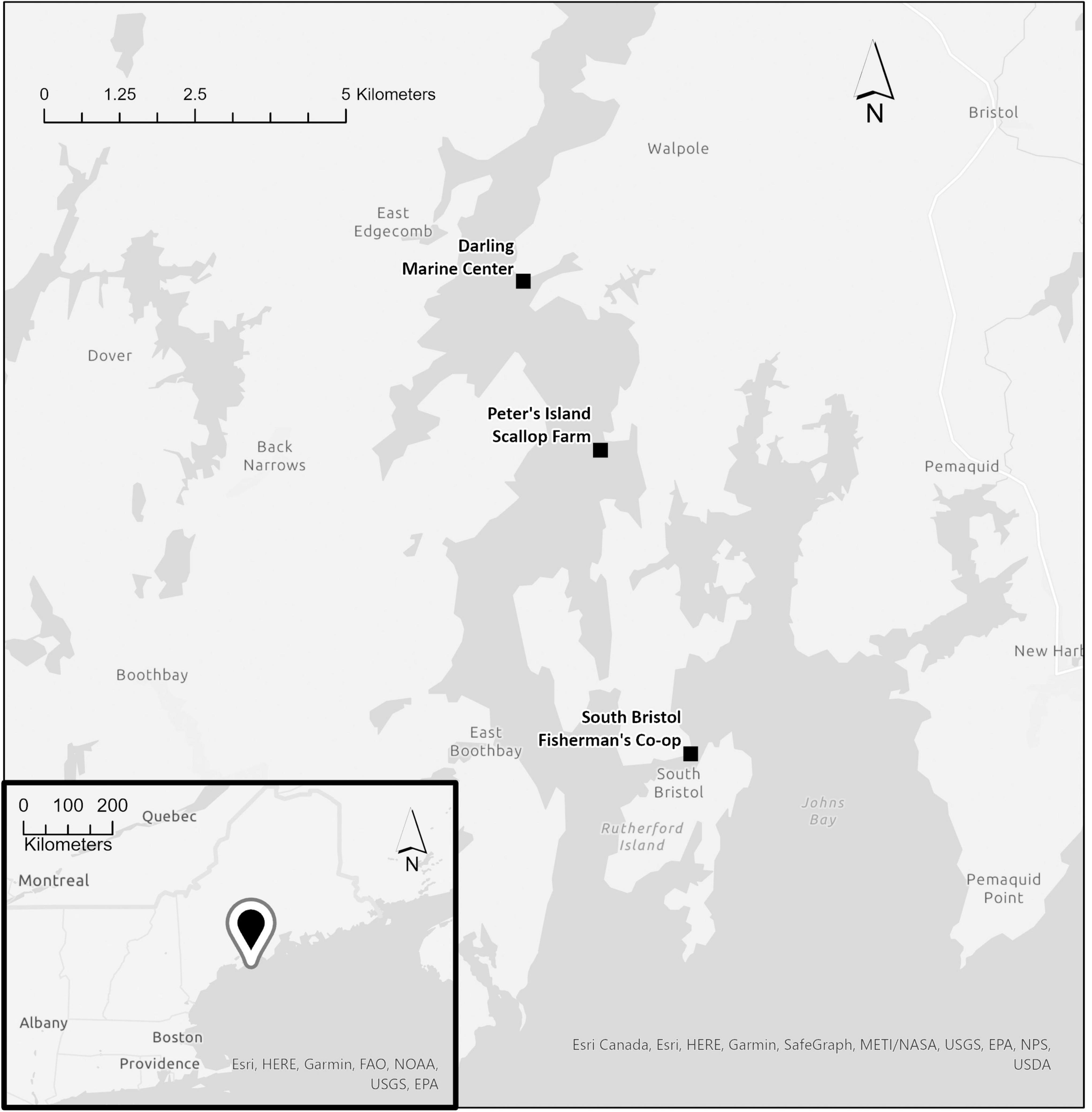
Figure 1. Map of study sites in the Damariscotta Estuary. Inset map shows the general location of the sites in the Gulf of Maine, indicated by the pointer symbol.
Collection and Processing of Samples
Each sample consisted of a single basibiont and all associated epibionts. The basibionts were haphazardly selected and removed from the floating docks by hand or by gently scraping the dock surface with a net (mesh size = 4.8 mm). Epibionts were considered any sessile species attached to the basibiont at time of collection. Each sample was placed in a numbered 50 mL polystyrene vial and preserved initially in 99% isopropyl alcohol with menthol crystals for relaxation. In the laboratory, the samples were placed in 70% ethanol for longer preservation. For each sample, all basibiont and epibiont specimens were identified to genus using dichotomous keys (e.g., Weiss, 1995) and classified by invasion status (native, including cryptogenic taxa if present, or non-native). The blotted wet weight of each basibiont and associated epibionts were individually determined, in addition to the number of epibionts in each sample. We used blotted wet weight as it is a reliable method for estimating size of most common invertebrates (Ricciardi and Bourget, 1998). To ensure reliability of our measurements, we repeated blotting and weighing until the weights of each sample were consistent. We calculated the Shannon Diversity Index (SDI) of the epibiont assemblages on each basibiont, using weight to measure abundance of the organisms as it applies to both colonial and solitary organisms.
Statistical Methods
In our statistical analyses, we pooled the data across all three sites, because these sites were in close proximity (all within 10 km of one another) and had similar fouling communities. We used a chi-square test to determine if there was an association between epibiont and basibiont status using the proportion of non-native organisms in each sample. A t-test was conducted to determine if the percentage of epibionts that were non-native differed among epibionts by their basibiont host status. An additional t-test was used to compare the mean number of epibiont taxa per basibiont genus on native and non-native basibionts to determine if different genera of basibionts supported varying number of taxa.
Four analysis of variance (ANOVA) tests were used to compare across basibiont taxa. First, we compared the epibiont-basibiont weight ratio among basibiont phyla. The second and third ANOVA tests were used to compare the percentage of epibionts that were non-native among basibiont phyla and basibiont genera, respectively. Finally, we compared the SDI of epibionts (by weight) across basibiont phyla. Significant values (P < 0.05) were further examined with a Tukey’s test.
Finally, we used an analysis of covariance to test the combined effects of basibiont weight and status on each of the following: the percentage of epibionts that were non-native on each basibiont, and the total epibiont weight on each basibiont host. All statistical analyses were conducted in R (R Project for Statistical Computing, SCR_001905).
Results
A total of 64 basibiont assemblages were collected across all sites; 26 basibionts were non-native species, and 38 basibionts were native. The basibionts and epibionts, as well as their associations, are shown in Table 1.
Does Invasion Status Matter?
We tested the hypothesis that there is an overall general difference in epibiont characteristics between native and non-native basibionts. Non-native epibionts were equally distributed among native and non-native basibionts (chi-squared = 1.934, df = 1, p = 0.1643). On native basibionts, there were 63 occurrences of non-native epibionts and 37 occurrences of native epibionts. Overall, there were 27 occurrences of each non-native and native epibionts on non-native basibionts (Figure 2). Of non-native epibionts, 30% settled on non-native basibionts, and 70% settled on native basibionts.
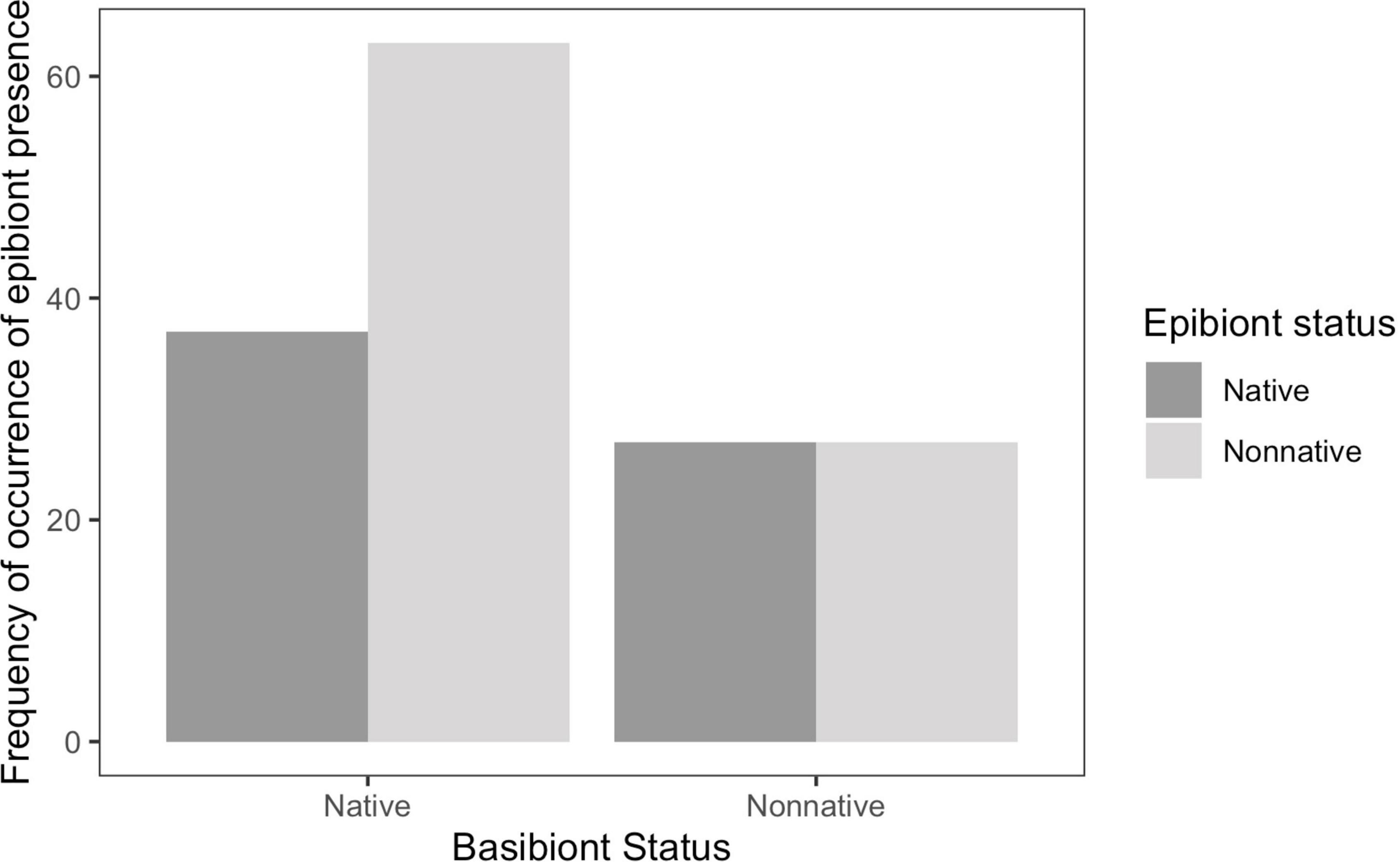
Figure 2. Frequency of occurrence by basibiont status. Non-native epibionts have a higher abundance on native basibionts.
Quantity, by weight, of non-native epibionts on non-native basibionts (68.988 ± 8.48%) and on native basibionts (69.303 ± 6.09%) were not significantly different in our study (t = 0.03, df = 48.6, P = 0.976; Figure 3A). Native basibiont genera yielded significantly greater epibiont diversity (SDI: 0.296 ± 0.064) than on non-native basibiont genera (SDI: 0.540 ± 0.054; t = –2.9081, df = 54.658, P < 0.01; Figure 3B).
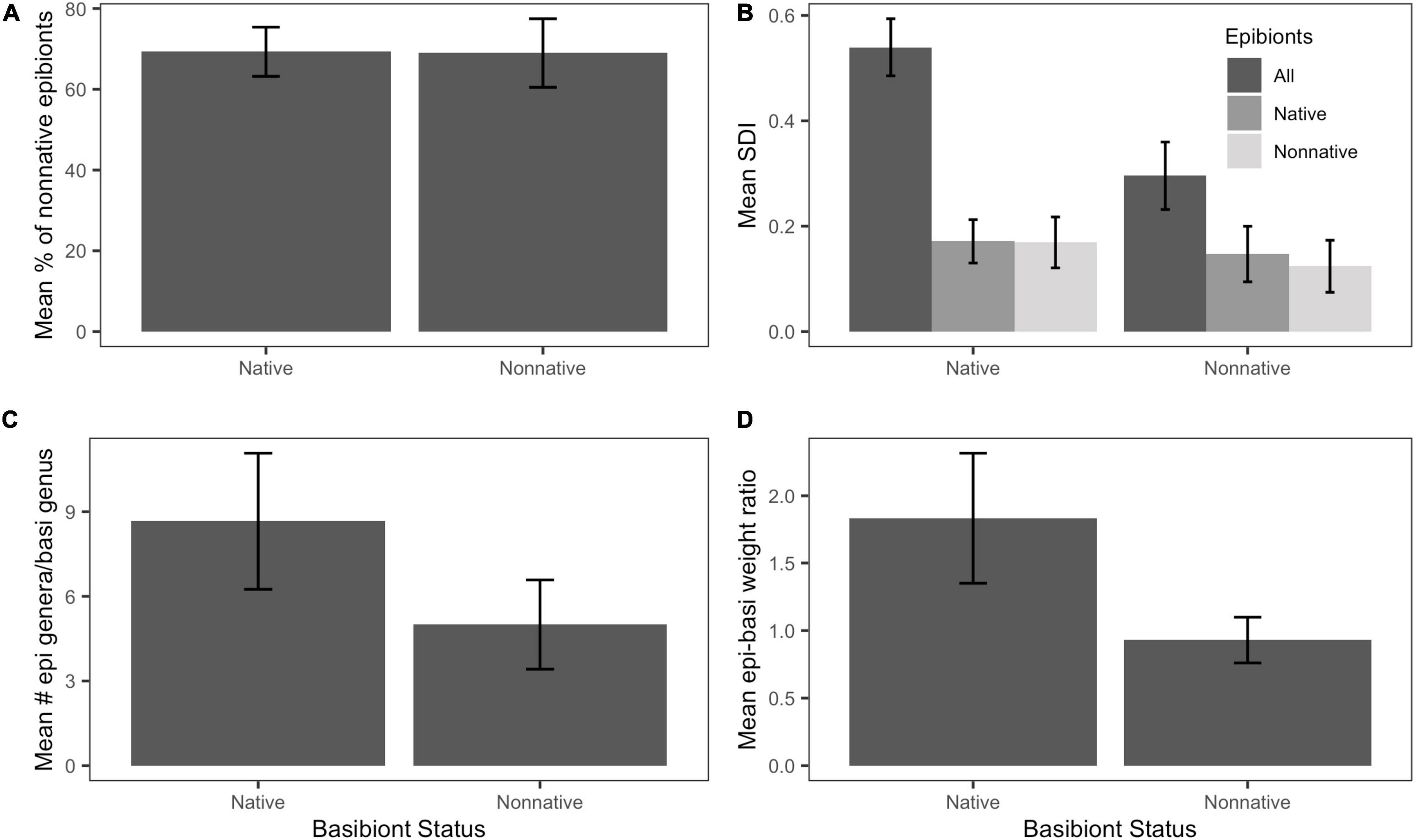
Figure 3. Distribution and diversity of epibionts by basibiont status. Error bars indicate standard error. (A) Mean percentage of non-native epibionts by weight. There was no significant difference in percentage of non-native epibionts between native and non-native basibionts (t = 1.37, df = 49.6, P = 0.17). (B) Mean Shannon Diversity Index (SDI). There was a significantly higher SDI on native basibionts than non-native basibionts (t = –2.91, df = 54.66, P < 0.01). (C) Mean number of epibiont genera per basibiont genus. There was no significant difference between native and non-native basibionts (t = –1.27, df = 7.81, P = 0.241). (D) Mean epibiont-basibiont weight ratio. There was no significant difference between basibiont groups (t = –1.77, df = 45.66, p = 0.08).
There was no significant difference between mean number of epibiont genera on each native basibiont genus (8.67 ± 2.42) than on non-native basibiont groups (5.00 ± 1.58; t = –1.269, df = 7.81, P = 0.241; Figure 3C). There was also no significant difference in the epibiont-basibiont weight ratio when comparing non-native (0.929 ± 0.169) to native (1.833 ± 0.483) basibionts (t = –1.77, df = 45.655, P = 0.084; Figure 3D).
Does the Basibiont Taxon Matter?
Cumulatively, molluscan basibionts supported the most epibiont phyla (n = 7). Following that were Chordata and Rhodophyta (n = 5 each), Ochrophyta (n = 4), and Bryozoa (n = 2). Only molluscan basibionts supported all of the observed epibiont phyla; and mollusks were the only substrate for arthropod and cnidarian epibionts. However, note that there were varying number of samples for each basibiont phylum (Table 1). Chordate epibionts dominated the percent occurrence on all basibiont phyla (n = 47.54 ± 8.85%), followed by molluscan epibionts (n = 28.40 ± 3.41%).
There was a significant difference in epibiont-basibiont weight ratio among basibiont phyla (F = 3.72; df = 4, 59; P < 0.01). Only Ochrophyta supported more than its own weight in epibionts (3.151 ± 0.905). The epibiont-basibiont weight ratio for Ochrophyta is significantly higher than that of Chordata (Tukey’s, P < 0.05) and Mollusca (Tukey’s, P < 0.05).
There was a significant difference in the percentage of epibionts that were non-native among basibiont phyla (F = 0.3.15; df = 4, 59; P < 0.05). Ochrophyta supported significantly more non-native epibionts (mostly bryozoans) than Phylum Mollusca (Tukey’s, P < 0.05). The only non-native basibiont phylum was Chordata. Interestingly, there is a significant difference in percent epibionts that are non-native among genera (F = 3.551; df = 9, 54; P < 0.05), in which Ciona harbored a higher percentage of non-native epibionts (98.093 ± 0.717%) than Styela (44.905 ± 13.677%), Mytilus (45.910 ± 11.416%), and Laminaria (89.845 ± 5.470%; Tukey’s, P < 0.05).
Shannon Diversity Index varied with basibiont phyla. There was a significant difference in SDI among phyla (F = 3.78; df = 4, 59; P < 0.01). Phylum Rhodophyta (mean SDI: 0.846 ± 0.116, n = 4) had a significantly higher epibiont SDI than Phylum Chordata (mean SDI: 0.296 ± 0.064, n = 26; Tukey’s, P < 0.05).
Does Size and Status Matter?
Due to the variation in size among the collected basibionts, we were interested in determining if size (approximated by blotted wet weight) and basibiont status were useful in predicting either percent of non-native epibionts present or total epibiont weight colonizing a basibiont. We tested the hypothesis that the effect of basibiont weight on the percentage of epibionts that are non-native depended on basibiont invasion status. We found no evidence for an interaction between basibiont weight and status on the percent of non-native epibionts (F = 0.0285; df = 1, 60; P = 0.867; Figure 4). We also tested the hypothesis that the effect of basibiont weight on total epibiont weight depended on the invasion status of the basibiont. We again found no interaction between basibiont weight and status on total epibiont weight (F = 0.401; df = 1, 60; P = 0.529; Figure 5). In both cases, the effects of basibiont and status were additive. All larger basibionts (weighing above 20 grams) were native.
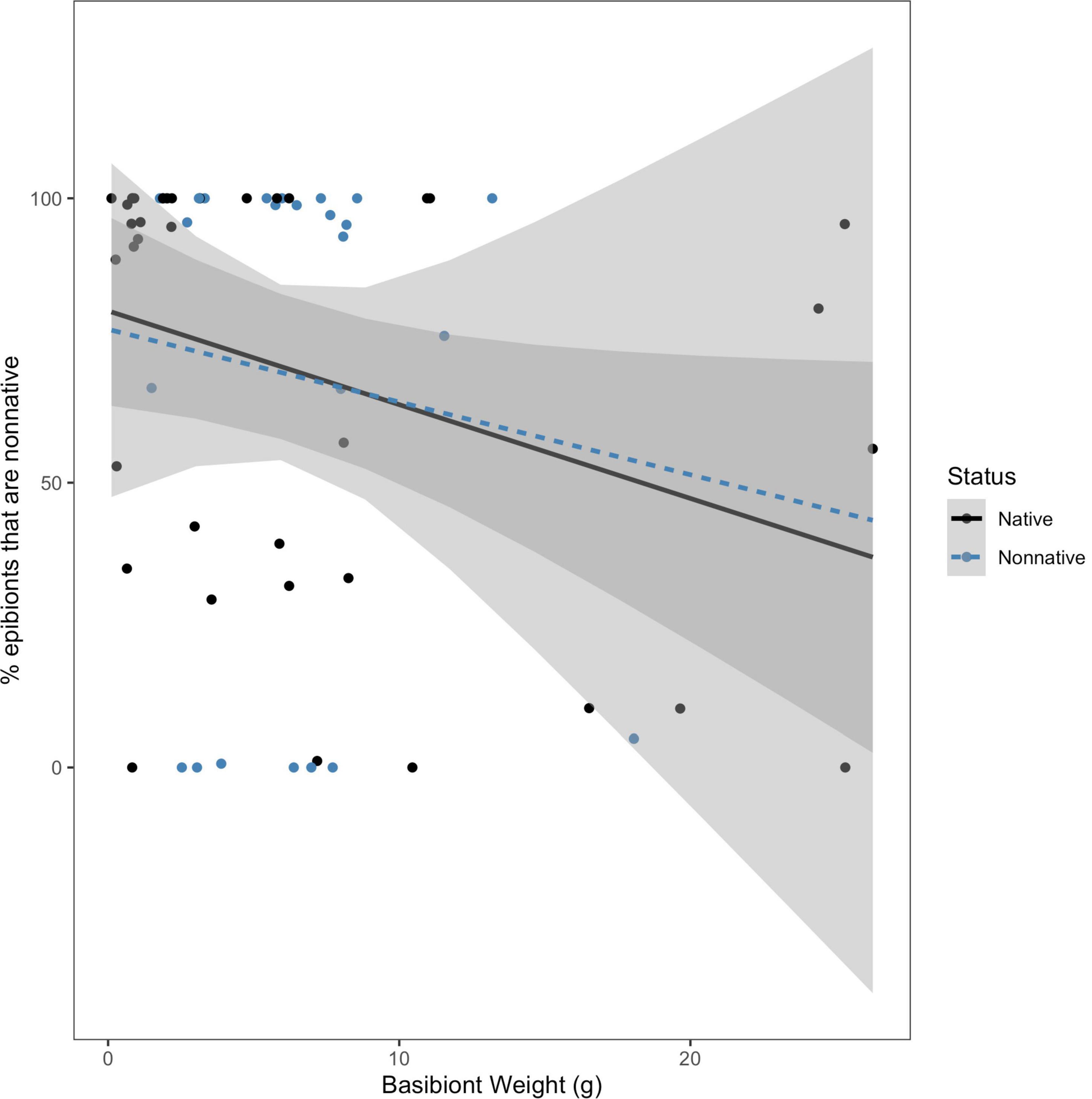
Figure 4. The effect of basibiont weight (g) on percent of epibionts that are non-native, by basibiont status. There was no interaction between status of the basibiont and basibiont weight in determining the percentage of non-native epibionts on each basibiont (F = 0.0285; df = 1, 60; p = 0.867).
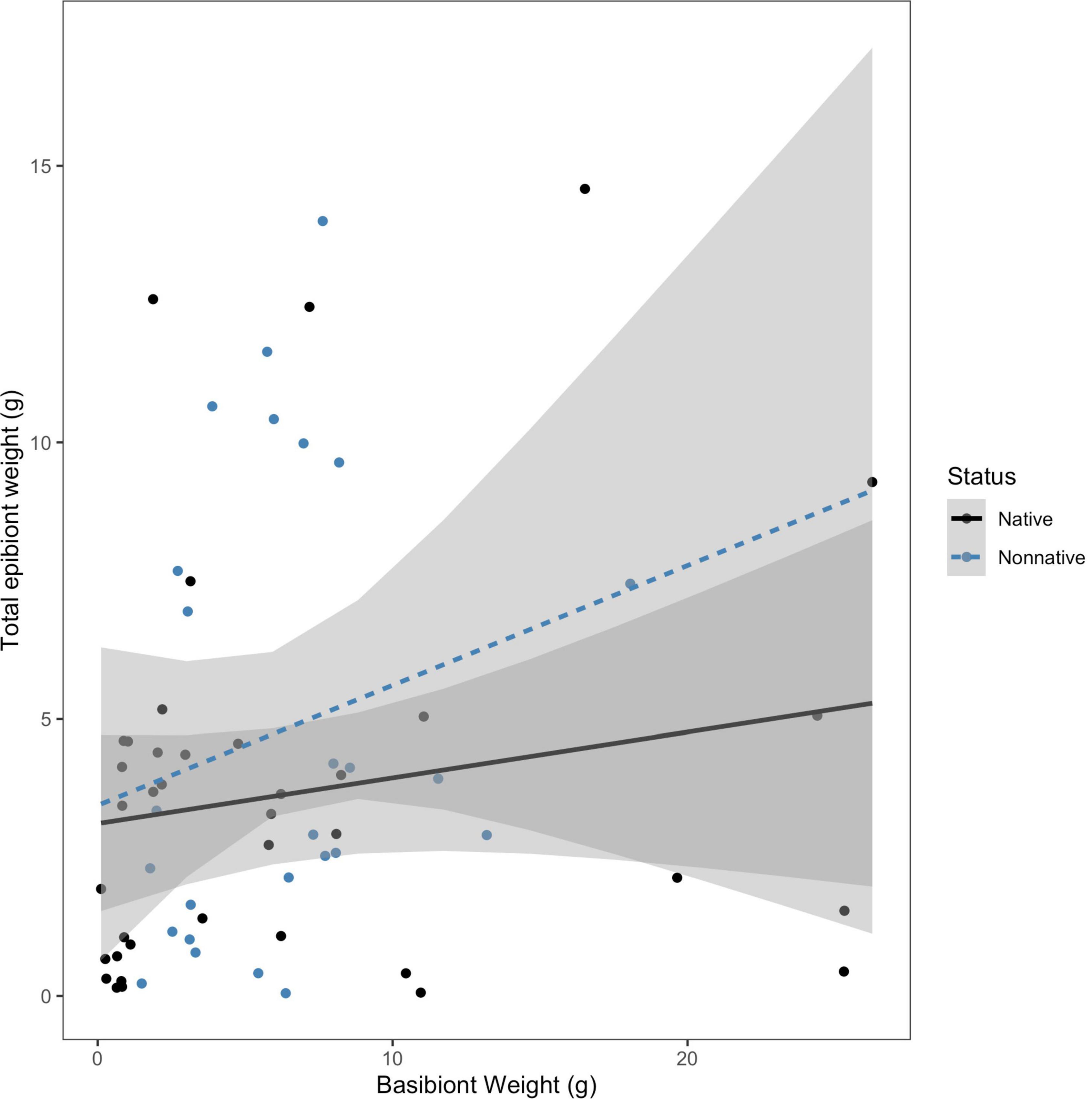
Figure 5. The effect of basibiont weight (g) on total epibiont weight (g), by basibiont status. There was no interaction between status of the basibiont and basibiont weight in determining the total epibiont weight on each basibiont (F = 0.4013; df = 1, 60; p = 0.529).
Discussion
In our study, there was no relationship between epibiont and basibiont status. However, while we found no preference for basibiont by status, there was a significantly more diverse assemblage of epibionts on native basibionts. This suggests that non-native basibiont species do not support as many epibiont species as native basibiont taxa.
Basibiont Status
The data we gathered may suggest that non-native basibiont organisms inhabiting an area may result in lower biodiversity of associated epibionts. There is evidence in the literature that non-native species may lead to community diversity decreases overall (Blackburn et al., 2004; Gaertner et al., 2009); though the extent of such declines was dependent on the invading species and the ecosystem in which the study was conducted (Gaertner et al., 2009). Arnold et al. (2016) found that fewer epibiotic species settled on the non-native alga Undaria pinnatifida, compared to native members of the same phylum (Laminaria ochroleuca, Saccharina latissima, and Saccorhiza polyschides). However, contrast these findings to Munari (2008), who found that in the Mediterranean, a non-native mussel basibiont Musculista senhousia ultimately led to higher biodiversity in areas that it invaded. These mussels supported over double the number of non-native epibionts, when compared to native basibionts. In these studies, as well as in the current study, the focus was primarily on the diversity of epibionts on each basibiont; however, an additional factor to consider in community structure is how foundational basibiont species may ameliorate stressful habits for mobile species (e.g., the non-native Mytilus galloprovincialis in South Africa, per Robinson et al., 2007).
Basibiont Taxon
This contradicting evidence from Munari (2008) may simply mean that basibiont taxon characteristics matter more than basibiont invasion status in determining which epibionts will settle. In our study, mussels were the most common native basibiont taxa; therefore, mussel surface topography may be an important factor that determines settlement potential. We also found no overall relationship between basibiont status coupled with either basibiont weight (a proxy for size) on either the total weight of the epibionts on each basibiont sample or the percent of basibionts that were non-native. Instead, surface microtopography and basibiont defenses may regulate the species that settle as epibionts (Wahl, 2009). Therefore, the specific species of basibiont present in the community may determine the quality, and perhaps quantity, of settling species.
Variation of multiple surface characteristics and morphology have been shown to impact which epibionts settle on a basibiont. Basibiont microtopographies and ability to chemically control epibiosis may also determine whether epibionts will settle on a basibiont (Marszalek et al., 1979; Stachowitch, 1980; Davis et al., 1989; Wahl et al., 1998; Lee and Qian, 2003; Dobretsov et al., 2006). Defenses against epibionts vary by species (Wahl, 2009). For example, mussels possess an antifouling periostracum (Bers et al., 2006), while algal species produce secondary metabolites that prevent colonization by epibionts (Nylund et al., 2005; Dobretsov et al., 2006). Ascidians also use mechanical and chemical defenses to minimize surface fouling (Wahl and Banaigs, 1991). Basibiont morphology also matters. Colonial animals are better competitors due to their indeterminate growth and asexual reproduction, while solitary animals survive due to their size and aggregation (e.g., mussel beds; Jackson, 1977). Drakard and Lanfranco (2016) found that macroalgal basibiont age (estimated by size) and surface area (estimated by coarseness) best predicted total abundance of epibionts, with the former measurement positively predicting species richness.
In our study, mussels supported the most epibiont phyla. Mussels, as ecosystem engineers, are a fundamental part of marine communities because they alter the substrate and facilitate interactions with many other species resulting in complex habitats (Jones et al., 1994; Gutiérrez et al., 2003; Robinson et al., 2007; Gutiérrez et al., 2019). Species richness increases in the presence of aggregating mussels (Seed, 1996; Chintiroglou et al., 2004; Borthagaray and Carranza, 2007). Mussels provide both substrate and food resources for interstitial and other associated species (Thiel and Ullrich, 2002). Çinar et al. (2008) found that Mytilus galloprovincialis assemblages supported non-native species, about 31% of the total individuals found in their study.
What Is the Role of Epibiosis in This Community?
Repeated invasion events, in which a non-native species facilitates additional invasions, may create a positive feedback loop (Simberloff and Von Holle, 1999). This “invasional meltdown” results in a snowball effect in which facilitation of one invader by another further exacerbates non-native species’ impacts on native species (Von Holle, 2011). Our study certainly did not demonstrate invasional meltdown as described in Simberloff (2006a). In fact, few studies directly demonstrate meltdown (Simberloff, 2006a). Gurevitch (2006) posits that invasional meltdown may be relatively uncommon and difficult to demonstrate. Very few papers show examples of marine meltdown (e.g., Grosholz, 2005; Geraldi et al., 2020); the majority of claimed meltdown events are based on terrestrial or freshwater data (e.g., Ricciardi, 2001; Jackson, 2015). Furthermore, Green et al. (2011) observe that the invasional meltdown hypothesis is controversial as few studies show positive feedback loops between invaders, in which amplified facilitative effects exist, and no studies at the time of their paper demonstrate facilitation of entry or spread of secondary invaders. Simberloff (2006a) agrees that specific evidence to show meltdown in the literature is rare.
Simberloff (2006a,b) and Gurevitch (2006) distinguish between facilitation and positive feedback. Facilitation occurs when one species aids another but does not necessarily receive a benefit in return. Positive feedbacks describe mutual facilitation, a population-level interaction where species facilitate one another. For example, an invader facilitates a species through predator deterrence while that species in turn ameliorates a harsh environment allowing the invading species to further establish. Gurevitch (2006) further claims that meltdown is an imprecise term that may refer to positive interactions, facilitative interactions, or actual meltdown, which includes feedback between invaders, amplifying their impacts, acting as an “autocatalytic” community-level process that accelerates replacement of native communities (Simberloff, 2006b; Green et al., 2011). Both Simberloff (2006b) and Gurevitch (2006) agree that studying positive feedbacks are likely to help in understanding invasion. Where, then, does epibiosis fall on the spectrum of facilitation, positive feedback, and meltdown? Epibiosis is not necessarily a mutualism since there are more benefits for epibiont species than basibiont species (Wahl, 1989). For example, basibionts provide additional substrate, and favorable hydrodynamic positions for filter feeding epibionts. In return, some epibionts provide potential protection against predation for basibionts, while many epibionts have been shown as harmful to basibionts (due to surface damage, competition, added weight leading to reallocation of resources away from growth, etc.; Wahl, 1989). If this is the case, then epibiosis may result in facilitation of invaders, but it is unclear if it is truly a mechanism for meltdown.
However, epibiosis may provide a vehicle for facilitation. Grosholz (2005) describes a model in which invaders arriving early in community establishment produce a change in the system, facilitating the establishment and spread of later invaders. In the case of epibiosis, a non-native basibiont, particularly if it is a strong competitor for space, may provide additional space for other settling species. While our study showed no preferential settlement on non-native or native basibionts, the availability of novel three-dimensional surfaces is expected to support additional species settlement. Improving habitat complexity is likely to increase potential for additional species, thereby increasing species richness (Crooks, 2002). Wonham et al. (2005) describe the facilitation of other species, including invaders, by the presence of the non-native Asian hornsnail (Batrillaria attrimentaria) on a mudflat. Generally, a fluctuating resource supply should increase invasions (Davis et al., 2000).
Several factors may determine invasibility of a community. Sher and Hyatt (1999) propose that both invader and environmental traits are incorporated into models predicting invasibility. Stachowicz et al. (1999) studied invasibility of marine ecosystems by looking at recruitment in sessile, suspension-feeding invertebrate communities and found that less diverse communities harbored more non-native invaders. Stachowicz et al. (2002) determined that this relationship was present at multiple scales. They found that the factors that control space availability also contributed to invasion success. Levine (2000) describes species loss at small scales reducing invasion resistance (that is, making them more prone to invasion), however, at community-level scales, diverse communities may be more likely to be invaded due to additional factors such as propagule supply (Kolar and Lodge, 2001).
Future Steps and Comments on the Study
This study is intended as an initial observational snapshot of communities in geographically close sites in Maine; it is not sufficient nor intended to be representative of patterns or the full range of taxa along coastal communities in other regions. It is not appropriate to assume that the impacts of non-native basibionts would have a similar impact in all marine ecosystems, as other factors may be at play in determining community structure (i.e., propagule pressure and other biotic components, as well as abiotic components; Lodge, 1993). The impact of an invader may very well depend on the factors leading to formation of the community in which it has invaded (Parker et al., 1999). With an observational focus, our study used basibionts that varied in size, and likely age, which may have affected the epibionts that were present. Longer-lived and larger species may be colonized by species at a different time period than younger or smaller species, which may impact epibiont communities on each basibiont.
There are multiple opportunities to expand on our study to better understand the role of epibiosis and facilitation in marine communities. This study may further be broadened to examining the impacts of within-species size variation on epibiont composition and diversity. In addition, a phenological time-series study to determine population impact of native species both before and after invasion would be ideal (Simberloff, 2006a). We do not know how the presence of basibionts changes overall community diversity because we did not measure the diversity before and after invasion. Furthermore, by expanding this study geographically, we can look at larger scale impacts on biodiversity in areas dominated by native and non-native basibiont foundational taxa. Future research should also investigate how epibiont-basibiont relationships differ by location to determine whether trends observed in this study are universal or vary with location. According to Jackson (2015), specific interactions between associated non-native species should be studied further as it is “a critical area of ecology.” As epibiosis is a relationship between two different pairs of species, it is one of the relationships that requires additional attention in understanding the structure of marine communities, and this interaction provides an excellent model to understand facilitation in nearshore communities.
Conclusion
Our study has indicated that while there is no direct association between epibionts and basibionts by status, native basibionts support a more diverse group of organisms. Hobbs et al. (2006) asks if novel systems are on the increase and whether such ecosystems will predominate at the end of the present century. How does this change our understanding of “wild” or “natural” ecosystems? Is invasional meltdown and other specialized concepts necessary for understanding the changes ecosystems are facing, or are they simply a typical example of ecosystem dynamics? Studies on epibiosis, particularly between non-native epibionts and their host, have focused primarily on the impacts on the basibiont (e.g., Saier and Chapman, 2004; Auker, 2010; Dijkstra and Nolan, 2011), rather than facilitative, community-wide effects. This study sheds light on the connection between epibiosis, invasion, and facilitative effects, with the hope that more studies will investigate epibiosis as a facilitator of invasions.
Data Availability Statement
The original contributions presented in this study are included in the article/supplementary material, further inquiries can be directed to the corresponding author.
Author Contributions
LA conceived of the research and collected the samples. KL processed and analyzed the samples. LA and KL wrote and edited the manuscript. Both authors contributed to the article and approved the submitted version.
Funding
Misericordia University funded this research as part of a Student Research Grant awarded to KL in Spring 2020.
Conflict of Interest
The authors declare that the research was conducted in the absence of any commercial or financial relationships that could be construed as a potential conflict of interest.
Publisher’s Note
All claims expressed in this article are solely those of the authors and do not necessarily represent those of their affiliated organizations, or those of the publisher, the editors and the reviewers. Any product that may be evaluated in this article, or claim that may be made by its manufacturer, is not guaranteed or endorsed by the publisher.
Acknowledgments
We are grateful to Dana Morse and Struan Coleman at the University of Maine’s Darling Marine Center, and South Bristol Fisherman’s Co-op for providing the sites we used to collect our samples. We would also like to thank Cathy Mazak’s Momentum Writing Community and the Misericordia University College of Arts and Sciences Summer Writing Retreat cohort for their collective encouragement while working on this manuscript. Finally, we are appreciative of the review and comments by Cosima Wiese, Greg Ruiz, and Annika Cornelius, whose feedback greatly improved this manuscript.
References
Arnold, M., Teagle, H., Brown, M. P., and Smale, D. A. (2016). The structure of biogenic habitat and epibiotic assemblages associated with the global invasive kelp Undaria pinnatifida in comparison to native macroalgae. Biol. Invasions 18, 661–676. doi: 10.1007/s10530-015-1037-6
Auker, L. (2010). The Effects of Didemnum vexillum Overgrowth on Mytilus edulis Biology and Ecology. Ph.D. dissertation. Durham: University of New Hampshire.
Bers, A. V., Prendergast, G. S., Zürn, C. M., Hansson, L., Head, R. M., and Thomason, J. C. (2006). A comparative study of the anti-settlement properties of mytilid shells. Biol. Lett. 2, 88–91. doi: 10.1098/rsbl.2005.0389
Blackburn, T. M., Cassey, P., Duncan, R. P., Evans, K. L., and Gaston, K. J. (2004). Avian extinction and mammalian introductions on oceanic islands. Science 305, 1955–1958. doi: 10.1126/science.1101617
Borthagaray, A. I., and Carranza, A. (2007). Mussels as ecosystem engineers: their contribution to species richness in a rocky littoral community. Acta Oecol. 31, 243–250. doi: 10.1016/j.actao.2006.10.008
Chintiroglou, C.-C., Damianidis, P., Antoniadou, C., Lantzouni, M., and Vafidis, D. (2004). Macrofauna biodiversity of mussel bed assemblages in Thermaikos Gulf (northern Aegean Sea). Helgoland Mar. Res. 58, 62–70. doi: 10.1007/s10152-003-0169-8
Çinar, M. E., Katagan, T., Koçak, F., Öztürk, B., Ergen, Z., Kocatas, A., et al. (2008). Faunal assemblages of the mussel Mytilus galloprovincialis in and around Alsancak Harbour (Izmir Bay, eastern Mediterranean) with special emphasis on alien species. J. Mar. Syst. 71, 1–17. doi: 10.1016/j.jmarsys.2007.05.004
Cohen, A. N., and Carlton, J. T. (1998). Accelerating invasion rate in a highly invaded estuary. Science 279, 555–558. doi: 10.1126/science.279.5350.555
Crooks, J. A. (2002). Characterizing ecosystem-level consequences of biological invasions: the role of ecosystem engineers. Oikos 97, 153–166. doi: 10.1034/j.1600-0706.2002.970201.x
Davis, A. R., Target, N. M., McConnell, O. J., and Young, C. M. (1989). “Epibiosis of marine algae and benthic invertebrates: natural products chemistry and other mechanisms inhibiting settlement and overgrowth,” in Bioorganic Marine Chemistry, Vol. 3, ed. P. J. Scheuer (Heidelberg: Springer Berlin), 85–114. doi: 10.1007/978-3-642-74560-7_4
Davis, M. A., Grime, J. P., and Thompson, K. (2000). Fluctuating resources in plant communities: a general theory of invasibility. J. Ecol. 88, 528–534. doi: 10.1016/j.watres.2005.05.036
Dijkstra, J. A., and Nolan, R. (2011). Potential of the invasive colonial ascidian, Didemnum vexillum, to limit escape responses of the sea scallop, Placopecten magellanicus. Aquat. Invasions 6, 451–456. doi: 10.3391/ai.2011.6.4.10
Dobretsov, S. V., Dahms, H.-V., Harder, T., and Qian, P.-Y. (2006). Allelochemical defense against epibiosis in the macroalgae Caulerpa racemosa var. turbinata. Mar. Ecol. Prog. Ser. 318, 165–175. doi: 10.3354/meps318165
Drakard, V. F., and Lanfranco, S. (2016). “Influence of macroalgal morphology on epiphyte community structure in the Maltese islands,” in Proceedings of the 41st CIESM Congress, Rapp. Comm. int. Mer Médit, Vol. 41, Kiel, 358.
Floerl, O., Pool, T. K., and Inglis, G. J. (2004). Positive interactions between nonindigenous species facilitate transport by human vectors. Ecol. Appl. 14, 1724–1736. doi: 10.1890/03-5399
Gaertner, M., Den Breeyen, A., Hui, C., and Richardson, D. M. (2009). Impacts of alien plant invasions on species richness in Mediterranean-type ecosystems: a meta-analysis. Prog. Phys. Geogr. 33, 319–338. doi: 10.1177/0309133309341607
Geraldi, N. R., Anton, A., Santana-Garcon, J., Bennett, S., Marbà, N., Lovelock, C. E., et al. (2020). Ecological effects of non-native species in marine ecosystems relate to co-occurring anthropogenic pressures. Glob. Chang. Biol. 26, 1248–1258. doi: 10.1111/gcb.14930
Green, P. T., O’Dowd, D. J., Abbott, K. L., Jeffery, M., Retallick, K., and MacNally, R. (2011). Invasional meltdown: invader-invader mutualism facilitates a secondary invasion. Ecology 92, 1758–1768. doi: 10.1890/11-0050.1
Grosholz, E. D. (2005). Recent biological invasion may hasten meltdown by accelerating historical introductions. Proc. Natl. Acad. Sci. U.S.A. 102, 1088–1091. doi: 10.1073/pnas.0308547102
Gurevitch, J. (2006). Commentary on Simberloff (2006): meltdowns, snowballs, and positive feedback. Ecol. Lett. 9, 919–921. doi: 10.1111/j.1461-0248.2006.00951.x
Gutiérrez, J., Bagur, M., and Palomo, M. (2019). Algal epibionts as coengineers in mussel beds: effects on abiotic conditions and mobile interstitial invertebrates. Diversity 11:17. doi: 10.3390/d11020017
Gutiérrez, J. L., Jones, C. G., Strayer, D. L., and Iribarne, O. O. (2003). Mollusks as ecosystem engineers: the role of shell production in aquatic habitats. Oikos 101, 79–90. doi: 10.1034/j.1600-0706.2003.12322.x
Hobbs, R. J., Arico, S., Aronson, J., Baron, J. S., Bridgewater, P., Cramer, V. A., et al. (2006). Novel ecosystems: theoretical and management aspects of the new ecological world order. Glob. Ecol. Biogeogr. 15, 1–7. doi: 10.1111/j.1466-822X.2006.00212.x
Jackson, J. B. C. (1977). Competition on marine hard substrata: the adaptive significance of solitary and colonial substrates. Am. Nat. 111, 743–767. doi: 10.1086/283203
Jackson, M. C. (2015). Interactions among multiple invasive animals. Ecology 96, 2035–2041. doi: 10.1890/15-0171.1
Jones, C. G., Lawton, J. H., and Shachak, M. (1994). Organisms as ecosystem engineers. Oikos 69, 373–386. doi: 10.1353/pbm.2003.0003
Kolar, C. S., and Lodge, D. M. (2001). Progress in invasion biology: predicting invaders. Trends Ecol. Evol. 16, 199–204. doi: 10.1016/s0169-5347(01)02101-2
Lee, O. O., and Qian, P.-Y. (2003). Chemical control of bacterial epibiosis and larval settlement of Hydroides elegans in the red sponge Mycale adherens. Biofouling 19, 171–180. doi: 10.1080/0892701021000055000
Levin, P. S., Coyer, J. A., Petrik, R., and Good, T. P. (2002). Community-wide effects of nonindigenous species on temperate rocky reefs. Ecology 83, 3182–3193. doi: 10.1890/0012-9658(2002)083[3182:CWEONS]2.0.CO;2
Levine, J. M. (2000). Species diversity and biological invasions: relating local process to community pattern. Science 288, 852–854. doi: 10.1126/science.288.5467.852
Lodge, D. M. (1993). Biological invasions: lessons for ecology. Trends Ecol. Evol. 8, 133–136. doi: 10.1016/0169-5347(93)90025-K
Marszalek, D. S., Gerchakov, S. M., and Udey, L. R. (1979). Influence of substrate composition on marine microfouling. Appl. Environ. Microbiol. 38, 987–995. doi: 10.1128/aem.38.5.987-995.1979
Molnar, J. L., Gamboa, R. L., Revenga, C., and Spalding, M. D. (2008). Assessing the global threat of invasive species to marine biodiversity. Front. Ecol. Environ. 6:485–492. doi: 10.1890/070064
Munari, C. (2008). Effects of the exotic invader Musculista senhousia on benthic communities of two Mediterranean lagoons. Hydrobiologia 611, 29–43. doi: 10.1007/s10750-008-9459-0
Nylund, G. M., Cervin, G., Hermansson, M., and Pavia, H. (2005). Chemical inhibition of bacterial colonization by the red algae Bonnemaisonia hamifera. Mar. Ecol. Prog. Ser. 302, 27–36. doi: 10.3354/meps302027
Parker, I. M., Simberloff, D., Lonsdale, W. M., Goodell, K., Wonham, M., Kareiva, P. M., et al. (1999). Impact: toward a framework for understanding the ecological effects of invaders. Biol. Invasions 1, 3–19. doi: 10.1023/A:1010034312781
Prenter, J., MacNeil, C., Dick, J. T. A., and Dunn, A. M. (2004). Roles of parasites in animal invasions. Trends Ecol. Evol. 19, 385–390. doi: 10.1016/j.tree.2004.05.002
Ricciardi, A. (2001). Facilitative interactions among aquatic invaders: is an “invasional meltdown” occurring in the Great Lakes? Can. J. Fish.Aquat. Sci. 58, 2513–2525. doi: 10.1139/f01-178
Ricciardi, A., and Bourget, E. (1998). Weight-to-weight conversion factors for marine benthic macroinvertebrates. Mar. Ecol. Prog. Ser. 163, 245–251. doi: 10.3354/meps163245
Robinson, T. B., Branch, G., Griffiths, C. L., Govender, A., and Hockey, P. A. R. (2007). Changes in South African rocky intertidal invertebrate community structure associated with the invasion of the mussel Mytilus galloprovincialis. Mar. Ecol. Prog. Ser. 340, 163–171. doi: 10.3354/meps340163
Saier, B., and Chapman, A. S. (2004). Crusts of the alien bryozoan Membranipora membranacea can negatively impact spore output from native kelps (Laminaria longicruris). Bot. Mar. 47, 265–271. doi: 10.1515/BOT.2004.031
Seed, R. (1996). Patterns of biodiversity in the macro-invertebrate fauna associated with mussel patches on rocky shores. J. Mar. Biol. Assoc. U. K. 76, 203–210. doi: 10.1017/S0025315400029131
Sher, A. A., and Hyatt, L. A. (1999). The disturbed resource-flux invasion matrix: a new framework for patterns of plant invasion. Biol. Invasions 1, 107–114. doi: 10.1023/A:1010050420466
Simberloff, D. (2006a). Invasional meltdown 6 years later: important phenomenon, unfortunate metaphor, or both? Ecol. Lett. 9, 912–919. doi: 10.1111/j.1461-0248.2006.00939.x
Simberloff, D. (2006b). Rejoinder to Simberloff (2006): don’t calculate effect sizes; study ecological effects. Ecol. Lett. 9, 921–922. doi: 10.1111/j.1461-0248.2006.00952.x
Simberloff, D., and Von Holle, B. (1999). Positive interactions of nonindigenous species: invasional meltdown? Biol. Invasions 1, 21–32.
Stachowicz, J. J., Fried, H., Osman, R. W., and Whitlatch, R. B. (2002). Biodiversity, invasion resistance, and marine ecosystem function: reconciling pattern and process. Ecology 83, 2575–2590.
Stachowicz, J. J., Whitlatch, R. B., and Osman, R. W. (1999). Species diversity and invasion resistance in a marine ecosystem. Science 286, 1577–1579. doi: 10.1126/science.286.5444.1577
Stachowitch, M. (1980). The epibiotic and endolithic species associated with gastropod shells inhabited by the hermit crabs Paguristes oculatus and Pagurus cuanensis. Mar. Ecol. 1, 73–101. doi: 10.1111/j.1439-0485.1980.tb00223.x
Thiel, M., and Ullrich, N. (2002). Hard rock versus soft bottom: the fauna associated with intertidal mussel beds on hard bottoms along the coast of Chile, and considerations on the functional role of mussel beds. Helgoland Mar. Res. 56, 21–30. doi: 10.1007/s10152-001-0098-3
Von Holle, B. (2011). “Invasional meltdown,” in Encyclopedia of Biological Invasions, Encyclopedias of the Natural World, Vol. 3, eds D. Simberloff and M. Rejmanek (Berkeley, CA: University of California Press), 360–364. doi: 10.1525/9780520948433-082
Wahl, M. (1989). Marine epibiosis. I. Fouling and antifouling: some basic aspects. Mar. Ecol. Progr. Ser. 58, 175–189. doi: 10.3354/meps058175
Wahl, M. (2009). “Epibiosis: ecology, effects and defenses,” in Marine Hard Bottom Communities, Ecological Studies 206, ed. M. Wahl (Heidelberg: Springer-Verlag), 61–72. doi: 10.1007/s00442-010-1830-y
Wahl, M., and Banaigs, B. (1991). Marine epibiosis. III. Possible antifouling defense adaptations in Polysyncraton lacazei (Giard) (Didemnidae, Ascidiacea). J. Exp. Mar. Biol. Ecol. 145, 49–63. doi: 10.1016/0022-0981(91)90005-H
Wahl, M., Kröger, K., and Lenz, M. (1998). Non-toxic protection against epibiosis. Biofouling 12, 205–226. doi: 10.1080/08927019809378355
Weiss, H. M. (1995). Marine Animals of Southern New England and New York. Hartford, CT: Connecticut Department of Environmental Protection.
Keywords: invasive species, community ecology, epibiosis, biodiversity, basibiont, facilitation
Citation: Lazzeri K and Auker LA (2022) The Role of Invasion Status and Taxon of Basibionts in Marine Community Structure. Front. Ecol. Evol. 10:806328. doi: 10.3389/fevo.2022.806328
Received: 31 October 2021; Accepted: 06 June 2022;
Published: 22 June 2022.
Edited by:
Roksana Majewska, North-West University, South AfricaReviewed by:
Gregory Ruiz, Smithsonian Environmental Research Center (SI), United StatesAnnika Cornelius, Alfred Wegener Institute Helmholtz Centre for Polar and Marine Research (AWI), Germany
Copyright © 2022 Lazzeri and Auker. This is an open-access article distributed under the terms of the Creative Commons Attribution License (CC BY). The use, distribution or reproduction in other forums is permitted, provided the original author(s) and the copyright owner(s) are credited and that the original publication in this journal is cited, in accordance with accepted academic practice. No use, distribution or reproduction is permitted which does not comply with these terms.
*Correspondence: Linda A. Auker, lauker@misericordia.edu