- 1Department of Landscape Architecture, Planning and Management, Swedish University of Agricultural Sciences, Alnarp, Sweden
- 2School of Ecosystem and Forest Sciences, The University of Melbourne, Parkville, VIC, Australia
- 3Department of Biosystems and Technology, Swedish University of Agricultural Sciences, Alnarp, Sweden
- 4Department of Forestry and Wood Technology, Linnaeus University, Växjö, Sweden
Designing green infrastructure in cities requires vegetation that has multiple outcomes and functions, particularly using plants that have both attractive visual or aesthetic features and high biodiversity values. Plantings that have high visual appeal are more highly valued by people and increase their feeling of wellbeing. Increasing biodiversity in cities is one of the major challenges facing urban planning and design. However, balancing biodiversity and aesthetic outcomes in urban planting design is complex, and to date there are few methods that can be used to guide plant selection. To address this knowledge gap, we investigated the use of a colour theory framework for planting arrangements to see if we could design vegetation that is highly aesthetic and has high biodiversity. We did this by configuring planting combinations for living walls in Malmö, Sweden, using principles based on Johannes Itten’s colour theories. The plant combinations on each wall were graphically arranged using (1) colour analysis of each plant and (2) design of the plant species into two colour schemes: light-dark colour concept and a complementary colour concept. For each species used in the compositions we created a biodiversity classification, based on its pollination value, “nativeness” and conservation value as a cultivar; and a plant visual quality classification, based on the performance from living walls studies. The graphical colour composition and interlinked biodiversity value were then compared to designs created with randomly selected plant species. The results showed that it is possible to design a living wall based on colour theory without compromising with biodiversity outcomes, namely species richness, pollination and the nativeness of the species. The results also indicate the potential application of this design approach to deliver greater aesthetic appreciation and enjoyment from plantings. While more work is needed, this study has shown that a theoretical colour framework can be a useful tool in designing green infrastructure to improve delivery of both cultural and regulatory ecosystem services.
Introduction
Urban vegetation can be designed to deliver biodiversity outcomes and provide regulatory ecosystem services, particularly through green infrastructure. In addition, visual qualities derived from urban vegetation can provide aesthetic enjoyment and appreciation, delivering cultural ecosystem services, but are dependent on socio-behavioural pathways for positive public health effects (van den Bosch and Ode Sang, 2017).
Perceived visual quality and visual satisfaction in outdoor environments derives from a viewed landscape and can be evaluated from a formal aesthetic approach where the landscape quality or beauty is seen as an attribute in the eye of the beholder (Lothian, 1999). Perceived attributes, such as landform, water bodies and colours, are part of the formal aesthetic approach and are included in the objectivist paradigm (Daniel and Vining, 1983; Lothian, 1999). Colour as a property has a broad spectrum, and in landscape design compositions the attribute “colour” has its origins in design theory and design principles, sometimes using gestalt theory as an evaluation tool (Lang, 1994; Bell, 2012; Yilmaz et al., 2018).
Yilmaz et al. (2018) states that landscape professionals can influence visual qualities and viewer’s aesthetic satisfaction via careful use of design principles, in turn contributing to positively affect the visual quality (Ulrich, 1983; Arriaza et al., 2004; Polat and Akay, 2015) and evoke positive restorative effects (Hoyle et al., 2017). In the planning and design of green infrastructure the aesthetic property colour and it’s physical attribute, contrast, give variation to planting composition schemes (Bell, 2004; Dunnett, 2019). A conscious use of colour and colour contrast in the design of urban green installations can improve human experiences of pleasantness (Thorpert, 2019), provide positive visual preferences (Polat and Akay, 2015) and contribute to increased experiences of beauty and harmony (Eroğlu et al., 2012; Oleksiichenko et al., 2018; Huang and Lin, 2019). A common strategy to achieve variation and distinction through colour contrast in compositions is to use disparity in the brightness level, as well as the use of complementary contrast in design compositions (Robinson, 2004; Bell, 2012). For example, the concept of complementary contrast is an effective design parameter in landscape architecture and has proven to contribute to harmonious arrangements (Westland et al., 2007; Bell, 2012) and increase the correlation between visual preferences and complementary colour contrast in urban green spaces (Polat and Akay, 2015). In the context of colour and visual preferences, it is important to highlight that personal attitudes, biases and cultural background or variations in value systems all contribute to how we perceive colours (Patton, 2002). A study by Kendal et al. (2012) showed that diversity in visual preferences depends on features such as foliage colour and flower size as well as non-visual characteristics such as drought tolerance and “nativeness.”
Biodiversity, according to Wilcox (1984), is defined as the variation of life forms on earth, including variation in genes, species and biotopes, and functions from factors, the species richness and the distribution of the individuals among these species, the evenness. High species diversity positively affects ecosystems and human life (Hooper et al., 2005, 2012), while biodiversity loss is recognised as one of the most severe human induced global challenges (Rockström et al., 2009). Biodiversity loss causes ecosystems to capture less resources, reducing biomass production and causing instability in the ecosystem (Cardinale et al., 2012) and can mitigate invasive species (Naeem et al., 2000; Kennedy et al., 2002); changes which can be irreversible or extremely difficult and expensive to alter. Biodiversity in urban environments differs from that in rural environments; urbanisation tends to decrease biodiversity (Knapp et al., 2008), especially in city centres (McKinney, 2002). Areas with only moderate urbanisation can show an increase in biodiversity, primarily due to the introduction of non-native species being faster than the extinction of native species (Kowarik, 1995). This increase may also be due to factors like intrinsic high plant species richness in settlement areas, intermediate disturbance theory and introduction by species (McKinney, 2008), resulting in urbanisation leading to homogenisation of native plant species (Kühn and Klotz, 2006).
Urban areas are nowadays a hotspot for threatened species (Ives et al., 2016) and species conservation in these areas can be seen as a practical response to mitigate the loss of species globally (Kowarik, 2011). This is resulting in significant efforts in habitat creation to counteract biodiversity loss in rural and agricultural environments (Knapp et al., 2008). A systematic review by Berthon et al. (2021) found that most studies indicate a positive influence of native plant species on at least one measure of biodiversity, justifying their priority in urban green settings to support native animals. While conservation of native plant species is increasingly important, the conservation of culturally valuable species is also important in the context of heritage values. Especially the conservation of genetic variation within horticultural species, to find varieties that may be more useful in a future changed climate.
Biological diversity includes among other aspects to rehabilitate and restore degraded ecosystems and support the recovery of threatened species, for example through the conscious use of development and implementation of management strategies (Convention on biological diversity, 2021). It is also important to pay attention to the role of local and traditional knowledge in the conservation and sustainable use of biodiversity in situ where health is connected to biological diversity, a basic human right, and an important indicator of sustainable development, providing ecosystem services essential to human health and wellbeing (Convention on biological diversity, 2021).
The decline of insects, especially pollinators, is a major factor in the decline of global biodiversity (Goulson, 2019; Wagner et al., 2021). As a result, more attention is being given to the creation of urban habitats that can sustain more pollinators and increase biodiversity (Baldock et al., 2015). Green infrastructure technologies such as green roofs and walls are increasingly being used to support more urban biodiversity (Collins et al., 2017; Mayrand and Clergeau, 2018; Filazzola et al., 2019). Green walls have been shown to benefit birds (Chiquet et al., 2013) and insects (Madre et al., 2015; Ridzuan et al., 2021), although the number of studies regarding pollinators is limited (Chiquet, 2014; Dover, 2015; Ridzuan et al., 2021). Vegetation traits identified as having a positive impact on insect populations include flowering (Ridzuan et al., 2021) and foliage (Chiquet, 2014). Designing green infrastructure installations such as green- and living walls with greater species diversity and with species that support insects could be a way used to increase biodiversity in urban areas and is a major research question in this study.
Vertical greening is categorised as either facade greening, climbing plants growing on a building facade (Perini et al., 2013); or living walls, plants growing directly on a wall surface in either felt-based hydroponic systems or soil-cell modular systems (Riley, 2017). Living walls are becoming difficult to define, due to differences in components, materials and vegetation, as well as advances in design and technology (Perini et al., 2013; Manso and Castro-Gomes, 2015; Riley, 2017; Bustami et al., 2018). Regardless of these differences, living walls are increasingly being used in urban environments because of the many benefits they can provide. These include greater building thermal performance, particularly cooling (Wong et al., 2010; Chen et al., 2013), improvements in thermal comfort and carbon sequestration (Charoenkit and Yiemwattana, 2016), reductions in noise (Wong et al., 2010; Azkorra et al., 2015) and air pollution (Weerakkody et al., 2017), opportunities for localised food production (Nagle et al., 2017; Mårtensson et al., 2014) and improvements to urban hydrology, including grey water reuse (Fowdar et al., 2017). Much of the research into living walls has focussed on plant growth and performance (Riley, 2017), including species across a range of groups and climates (Mårtensson et al., 2014, 2016; Jørgensen et al., 2018; Dvorak et al., 2021) and the potential to support urban biodiversity (Collins et al., 2017; Filazzola et al., 2019). While there have been studies exploring the broader social benefits of living walls (Pérez-Urrestarazu et al., 2017; Bustami et al., 2018), the analysis of their aesthetic values remains a major gap in the literature (Radić et al., 2019).
Living walls are also a useful experimental prototype to examine individual plant performance and different planting designs. Most living wall systems can be adjusted, in terms of irrigation and nutrition, to provide optimum conditions for growing less common species, including native plants (Dvorak et al., 2021) and those with high biodiversity values (Mayrand and Clergeau, 2018). The vertical layout of living walls means that novel plant communities can also be configured under what are fairly uniform conditions, with competition restricted to a few neighbouring plants, with growing conditions that are easy to manipulate. The evaluation of species traits or attributes related to visual traits or to biodiversity and cultural values can be made through short growth experiments.
This study asks the question—can a living wall be designed to be aesthetically pleasing according to Johannes Ittens’s colour theory and at the same time support high biodiversity outcomes? The study examines and theoretically analyses if it is possible to design living walls that contain high biodiversity and at the same time obtain an appealing aesthetic expression from a colour theoretical point of view using Johannes Itten’s concepts light-dark- and complementary contrast as interpretation models (2002:36).
Materials and Methods
To collect data for interpretation of the graphically arranged design models we completed in situ plant growth trials, made plant colour observations in situ, created theoretical modelling of colour contrast interpretations models and calculated biodiversity outcomes of selected plant species. Three biodiversity factors were calculated from the selected plants and linked to the two redesigned conceptual colour contrast models, pollination value, “nativeness” and valuable cultivars. The individual plant visual performance data was derived from in situ living walls based on classification of classifying individual plants growth, vitality and survival.
We used a hypergeometric distribution to test if the biodiversity outcome in the two colour contrast interpretation models (light-dark and complementary) differed from a random choice of available plants. We also tested, through simulation, the two colour conceptual models to see if the used plant species visual performance were different from a random colour model. Finally, we combined calculated biodiversity classification value with the visual plant performance to gain a conceptual design framework for future planting design of living walls.
Plant Materials and Used Living Wall Systems
The data of plant species used in the study were sampled in situ from two experiments conducted in southern Sweden (Malmö), in November 2014, using three types of living wall systems. The first of these was a capillary and hydroponic mat system with pockets (wall type 1 in Figure 1); each pocket filled with 1500 grams of substrate composed of 90% (vol.%) pumice and 10% compost. One or two plants were planted in each pocket at a density of 31 plants per m2, the total planted area was 16.8 m2. The second was a hydroponic Rockwool mineral wool based panel system (wall type 2 in Figure 1). Nine plants were planted in each panel at a density of 25 plants per m2, the total planted area was 22.4 m2. The third living wall type is a container system (wall type 3 in Figure 1). The containers contain a substrate composed of 60% pumice, 20% compost and 20% sand and host 5 plants each. The containers are arranged in columns with a distance of 20 cm between the rows and the total area of the wall covered 8 m2 with a density of 25 plants per m2. The three wall systems are classified as a continuous living wall system CLWS according to Manso and Castro-Gomes (2015) and were irrigated and fertilised to optimum levels. All the walls were facing south and installed on buildings in the south of Sweden between 2012 and 2014 at two locations. Wall type 1 and 2 was located on a masonry wall in a closed area and wall type 3 in a residential housing area at public disposal. The criteria used in plant selection for the experiment was based on tolerance to drought and solar radiation, their origin (native to Sweden), edible traits, conservation status and lifeform (e.g. deciduous: geophyte: tufting: woody: sedge/rush: grass). The list of the 48 plant species planted in the three wall types is provided in Figure 2 and visible in Figure 1.
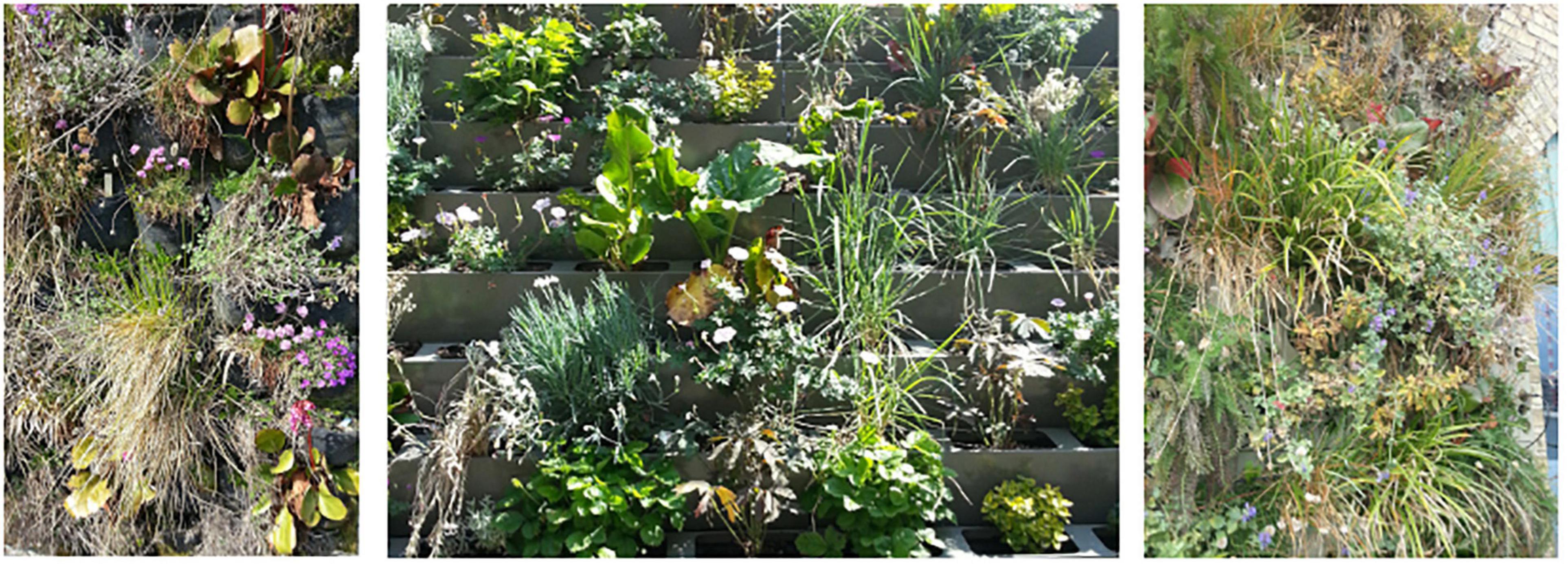
Figure 1. Capillary mat pocket living wall system (Left: wall type 1, “pockets”); container living wall system (Centre: wall type 3, “containers”) and mineral wool living wall system (Right: wall type 2, “panel system”) growing in southern Sweden used to determine colour and visual plant performance of plants. (Photograph Ann-Mari Fransson).
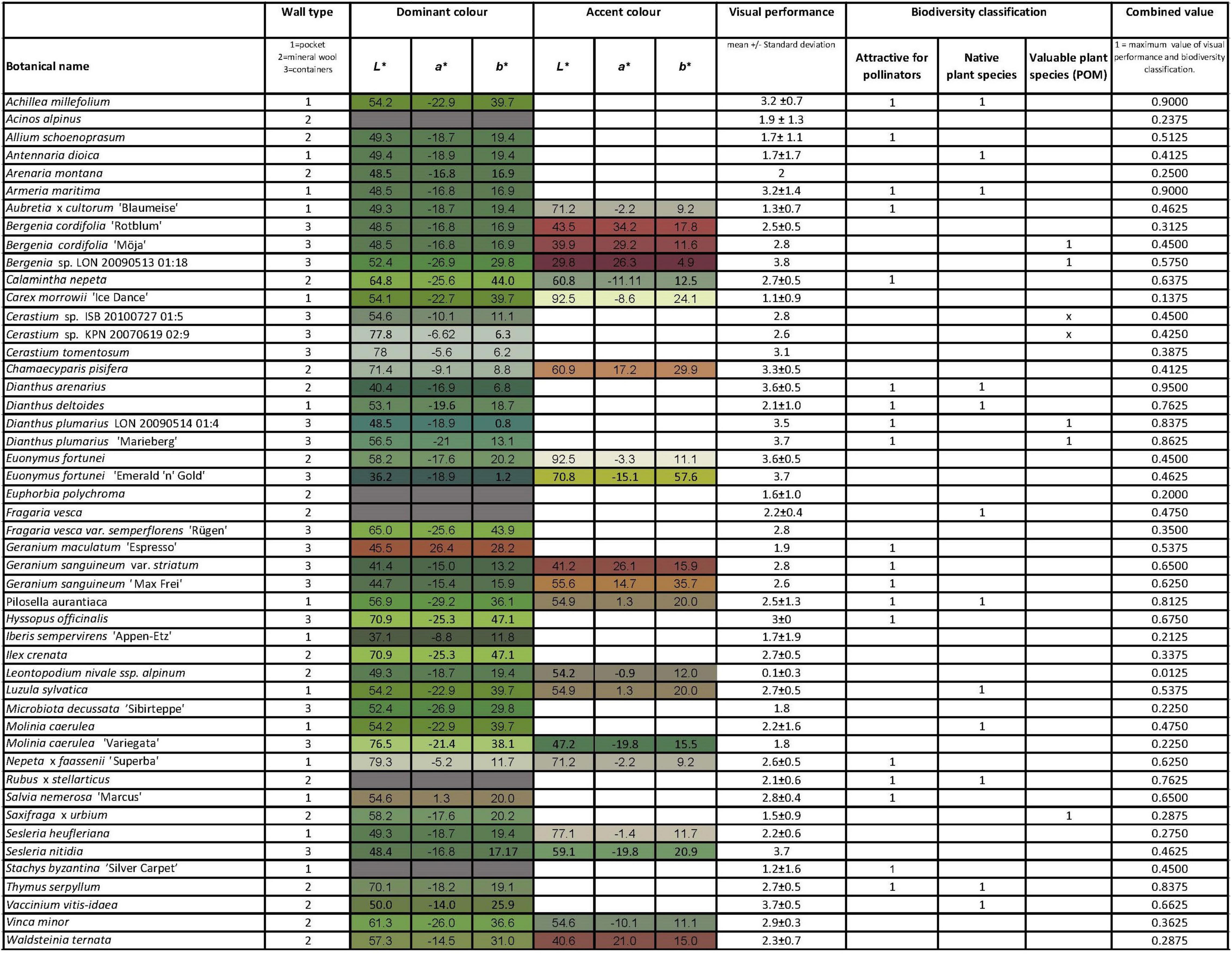
Figure 2. Compilation of the species studied, and the species mean visual performance, biodiversity classification and the combined value. Plant species marked with grey hue indicates that no colour measurements have been performed. Number 1 in the Biodiversity classification columns indicated that the plant species: is attractive for pollinators; belongs to native plant species or is cultivated culturally valuable plant species (POM).
Colour Determination and Analysis
Colour determinations were performed in situ, and the inherent foliage colours of the used plant species and varieties were documented from plants growing in the three living wall systems. The walls were fertilised to remove any possible interactions from nutrient deficiencies.
Out of the 48 plant species included in the three living wall systems, five plant species were excluded due to problems with visibility and accessibility. Colour analyses of the remaining 43 plant species were performed using colour charts from the RHS Colour Chart 5th edition. The colours were documented and systematically ordered in relation to each plant species intrinsic foliage colour and assessment of the plant species’ dominant colours. Some species were registered having a dominant and an accent foliage colour (Figure 2). Only the foliage colours were documented, any flowers were excluded from the study. To avoid glitter and reflective surfaces caused by direct sunlight, the colour assessments were conducted between 10 a.m. and 2 p.m. in overcast weather.
The perceived 43 colours according to the RHS Colour Chart 5th system were converted into the CIE (L*a*b*) space. The CIE (L*a*b*) system is directly modelled on human colour perception (Tkalčič and Tasič, 2003) and represents differences in colour that correspond to human vision (Kendal et al., 2013). The CIE (L*a*b*) colour space communicate colour perception and show relationship between the two colour dimensions a* (green at negative a* values to magenta at positive a* values) and b* (blue at negative b* values to yellow at positive b* values). CIE (L*a*b*) has a linear measure of lightness (L*) where L* = 0 represents darkest black and 100 brightest white. The CIE (L*a*b*) colour values of the perceived 43 colours is visible in Figure 2.
Graphical Redesign of Living Wall Using Colour Theory
In order to be able to theoretically test Johannes Itten’s concepts, light-dark- and complementary contrast, two graphical designs were prepared with species from the 2012 to 2014 living wall experiments in Malmö, Sweden. The number of selectable species and in turn available colour choices in the study, limited the number of alternative interpretation models. However, the two graphical redesigns were solely based on Johannes Itten’s design principles and we based the colour contrast compositions on what Itten (2002) considered a “well-balanced and colourful arrangement.” To ensure a balanced composition, each plant species CIE (L*a*b*) values have been used as a basis and inspiration for the development of the two graphic redesigns; light-dark, and complementary colour concepts. The two redesigns were designed without knowledge of each species biodiversity outcome. This approach enabled the designs to be as close to Johannes Itten’s colour contrast concepts as possible, without being influenced by factors other than colour theory.
The two graphical redesigns used 25 plant species per m2 of a living wall area. This was done to resemble the design structure of two of the studied living walls and the ratio of 25 plant species per m2, which satisfies a goal of moderate to high species richness in a Northern European context (Crawley, 1986). The graphical designs based on light-dark contrast and complementary contrast are presented via colour schemes (Figure 3), together with the plant species included in each design (Figure 4). To examine if either of these two graphical designs: light-dark colour scheme (A) and complementary colour scheme (B) were extreme, with respect to the variables studied, the colour schemes were compared with a randomly selected colour scheme (C) (see Figure 4).
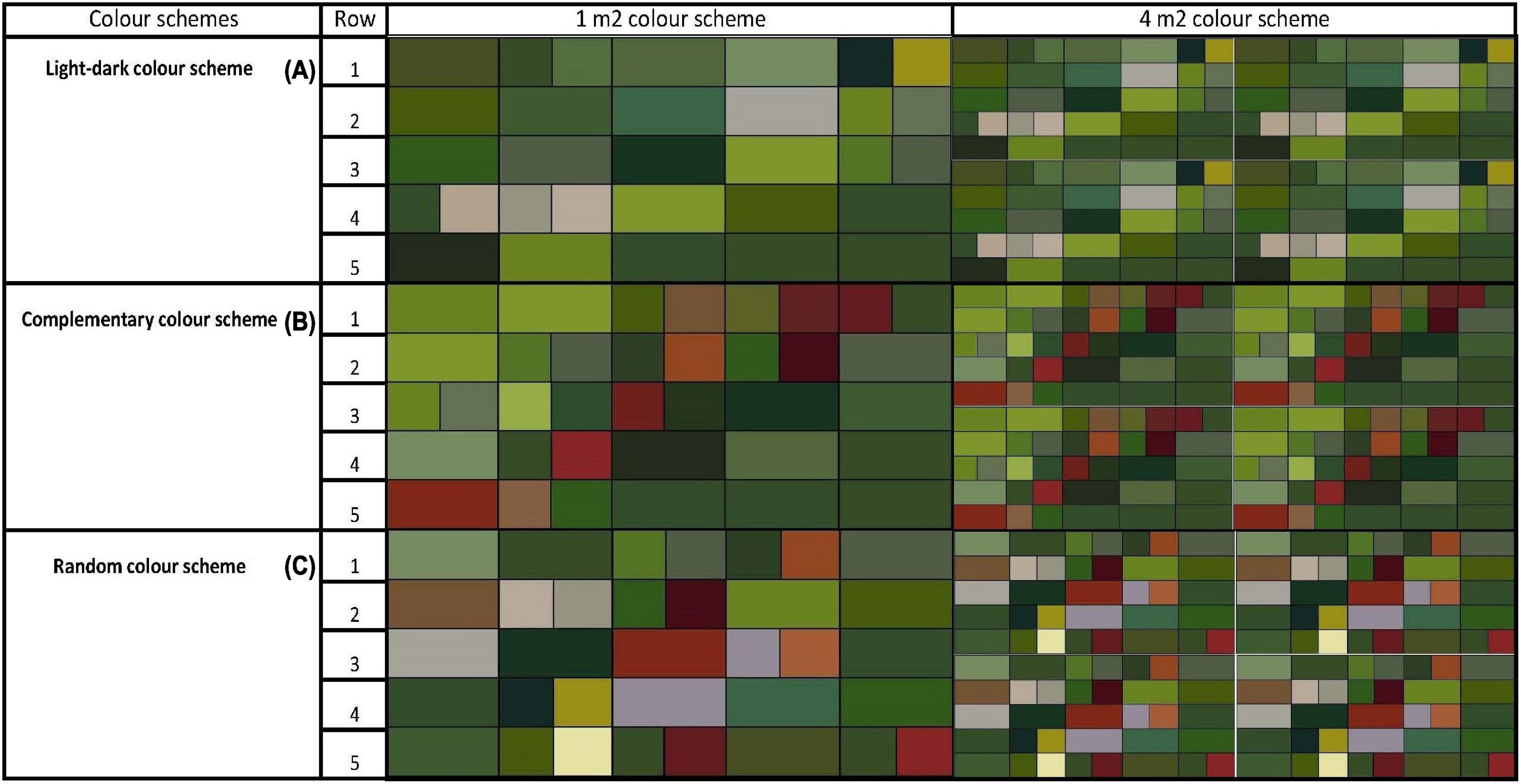
Figure 3. Graphically rearranged plant designs of living walls using two colour schemes, light-dark (A) and complementary (B) and one random scheme (C) illustrated in 1 m2 and 4 m2 view.
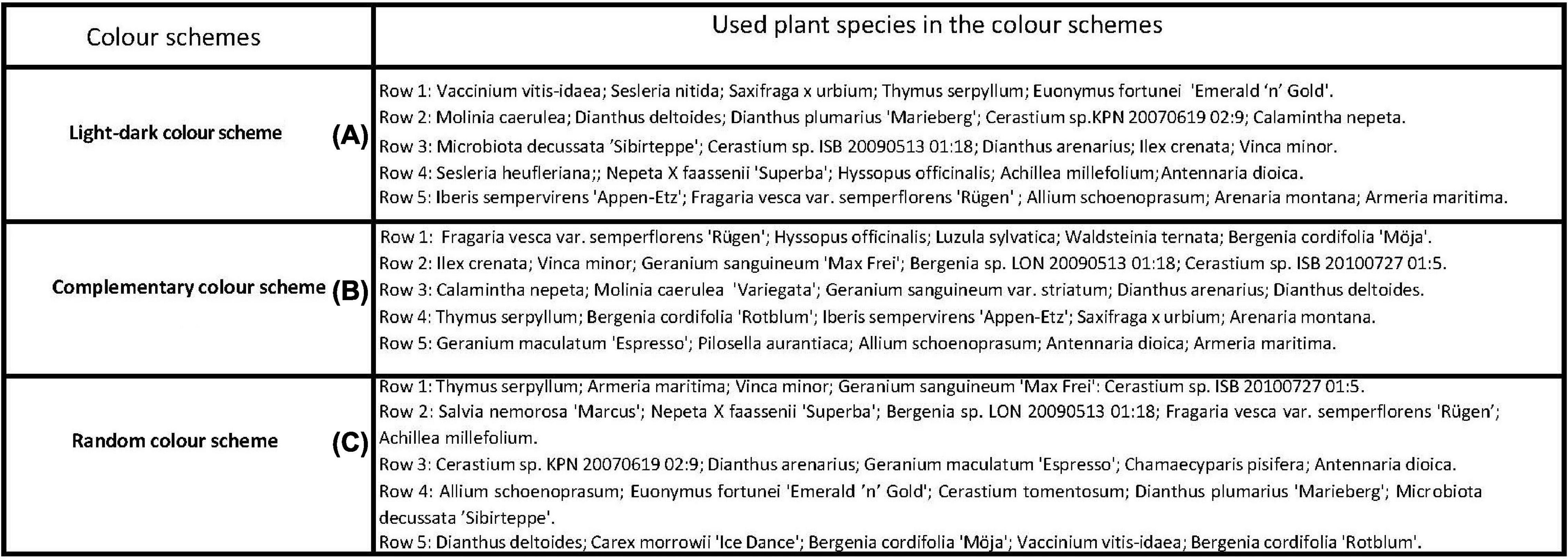
Figure 4. The species used to create a design based on light-dark design and complementary design and one random design for a theoretical living wall.
Visual Plant Performance Assessment of the Plants Used in the Study
We calculated the visual plant performance using a 5 point scale where 0: 100% dead (no observed living tissue) 1: > 50% dead (just alive), 2: 25–50% dead (severely affected), 3: < 25% dead (slightly affected), and 4: 0% dead (thriving), and was based on Mårtensson et al. (2014). To gain a value of visual plant performance, we assessed between 5 and 8 specimens of the individual species to gain a mean value between 0 and 4. The value represents the plants ability to grow and thrive in combination with the species on these living walls. The visual plant performance data of each plant species in the study is shown in Figure 2. To estimate if the mean values of the plants in the light-dark (A) and complementary (B) graphical designs differed from the mean values in a random design we did a simulation of the mean values. The probability distribution of the mean visual plant performance of a random choice of 25 plant species was simulated using 10,000 simulations of plant species compositions out of the 43 plant species included in the study.
Biodiversity Classification
Plant Selection for Biodiversity Classification
Three plant selection criteria were used to define a biodiversity classification in this study—attractiveness for pollinators, native plant species and valuable cultivars. Pollinators within this study are defined as pollinating insects, firstly bees, bumblebees and syrphids but also butterflies. Plants attractive for pollinators data was gained from three main sources: Garbuzov and Ratnieks (2014), Rollings and Goulson (2019), and Goulson (2021). Two further sources, Teräs (1976) and Fussell and Corbet (1992), were used to explore flower visits by bumblebees. Observations from Haaland (2015) were also included. Plant attractiveness for pollinators is not well researched at a species level and not available in the literature (Garbuzov and Ratnieks, 2014). Therefore, in this study we classified plant species regarding their attractiveness for pollinators at a genus level. Further, in this study plant species were included based on evidence to support their potential attraction for pollinators, rather than more quantitative evidence in the literature (i.e., how many pollinators visited a certain plant species in a certain time). This means also that plant species could be classified as being attractive even if the numbers of visiting insects was low, but insect visits were recorded. In this way, the following plant genera were classified as potentially being attractive for pollinators: Achillea, Allium, Armeria, Aubretia, Calamintha, Dianthus, Geranium, Hieracium, Hyssopus, Nepeta, Rubus, Salvia, Stachys, and Thymus (Figure 2).
A total of 12 species in this study are native to Sweden according to Mossberg (2010) (Figure 2). Seven plant cultivars with high conservation value were also included based on their “cultural heritage” value. This was based on data collected from the National genebank for the preservation of older varieties of ornamental species, otherwise known as “The program for diversity of cultivated species” or the acronym POM. These plant cultivars have cultural-historical values, as well as the diversity values, attributes that are included in Convention on biological diversity (2021).
Biodiversity Classification and Data Analysis
Each individual species is classified as 1 or 0 for three variables, the “attractiveness for pollinators,” whether it is “native plant species” and whether it is “cultivated culturally valuable plant species.” To combine these components, a biodiversity value was calculated using the formula
All components in this equation are either 0 or 1, and since none of the plant species included in the study are classified as “native plant species” and “culturally valuable plant species,” the biodiversity value is between 0 and 1. The values were weighted based on that it is twice as valuable if a plant is native than cultivated and three times as valuable if it is attractive for pollinators than cultivated giving a relationship between attractive for pollinators: native: cultivated culturally valuable of 3:2:1, where close to 1 expresses a high value for biodiversity value (see Figure 2).
To see if the biodiversity in terms of attractiveness for pollinators, number of native plant species and number of cultivated culturally valuable plant species in light-dark (A) and complementary (B) is in some way extreme, we compared the graphical designs to a random choice of 25 species out of the 43 species available. For this purpose, we use the fact that the number of elements with a given property in a random choice from a finite population is distributed according to a hypergeometric distribution. In this case, the population size is N = 43, the number of chosen elements is n = 25, and K is the number of elements in the populations with the given property. In this study, the parameters and the observed values in the hypergeometric distribution for the different situations are in Table 1. In the analyses, we compared the values obtained in the graphical designs with the probability distribution of the hypergeometric distribution, and were able to see if the values obtained in the graphical designs were extreme.
Biodiversity Classification and Visual Plant Performance Values
To have a same weighted value for the visual plant performance and biodiversity values, the value for visual plant performance was standardised to between 0 and 1 by dividing the values with the maximum value for the visual plant performance (4).
The “standardised visual plant performance” is now on the same scale as the biodiversity value and the combined value can be defined as
Where 0.5 in the formula expresses the fact that the weight for the two components is the same.
The combined value is a value between 0 and 1 where close to 1 expresses a high value for the visual plant performance and the biodiversity value (see Figure 2).
Results
Outcome of Colour Contrast Interpretation Models, Plant Biodiversity Classification, and Visual Plant Performance
The sections below display the design structure using the light-dark (A) and complementary (B) interpretation models as well as the outcome of visual plant performance in each design. The conceptual models are tested against a biodiversity classification and a combined value of biodiversity and visual plant performance.
Design Outcome and Structure of Light-Dark and Complementary Interpretation Models
A total of 39 plant species, from 43, were used in the preparation of light-dark (A) and complementary (B) graphical redesigns with a species richness of 25 plants/m2. In the design of the colour schemes A and B, Johannes Itten’s contrast of extension has been used as a base, where contrast of extension is correctly expressed, a contrast of proportion. Consideration and attention to the coloured areas in design A and B has been an important issue in the actual choice of plant species from Figure 2. To achieve a brightness effect in colour scheme A and B (Figure 3), a clear distinction and graduation of light and dark values are incorporated, and repetition and grouping are used to increase the brightness effect.
Light-dark colour scheme (A) is composed using structures of diagonal direction with contrasting and groupings of light and dark green/grey values in coloured boxes (Figure 3). A clear diagonal orientation in the composition creates perspective illusions, movement and depth into the arrangement (Itten, 2002). The rectangular boxes to the left and the right of the diagonals consist of medium green values, where the medium values in the composition increases the light-dark contrast effect. The complementary colour scheme (B) is arranged via two visually clear areas of light and dark green values in coloured boxes. The dark values are placed in the lower part of the composition and the light green values in the upper part. The dark values placed in the lower part of the composition stabilises the colour elements in the arrangement. In contrast, yellowish lighter green values are perceived as weightless colour elements and are placed in the upper part of the colour arrangement. The coloured rectangular boxes in colour scheme C are randomly placed with 25 randomly chosen plant species. Green, red and grey hues with a mix of light, medium and dark values are visible in a non-designed arrangement.
The order of the species in each colour scheme follows a structure with 5 × 5 rectangular boxes, in horizontal and vertical directions respectively. The species in each colour scheme (A, B, C) are arranged from left to right in the composition, with row 1 at the top on the left hand and row 5 starting at the bottom on the left hand of the colour schemes. In cases where a selected plant species were registered in the colour assessment having both a dominant and an accent colour, both the dominant and the accent colours are represented in the coloured rectangular boxes respectively. To show the outcome of the light-dark- and complementary compositions and the randomly selected model in a broader contextual situation, each 1 m2 colour scheme is visible via a 4 m2 colour scheme (see Figure 3).
Outcome of Visual Plant Performance in Light-Dark- and Complementary Interpretation Models
The two graphical design interpretation models visual plant performance were compared with 10 000 randomly chosen simulations (Figure 5), to examine if any of the colour schemes, A and B were extreme in relation to the factor visual plant performance. The mean visual plant performance for the 25 plant species in the graphical design light-dark (A) is 2.66 and for complementary (B) 2.51. The distribution of the mean value of a wall with 25 randomly chosen species out of the 43 was simulated using 10 000 simulations and the mean is 2.58 (Figure 5). The simulation show that the visual plant performance for light-dark (A) and complementary (B) are far from extreme and not significantly different from the random choices.
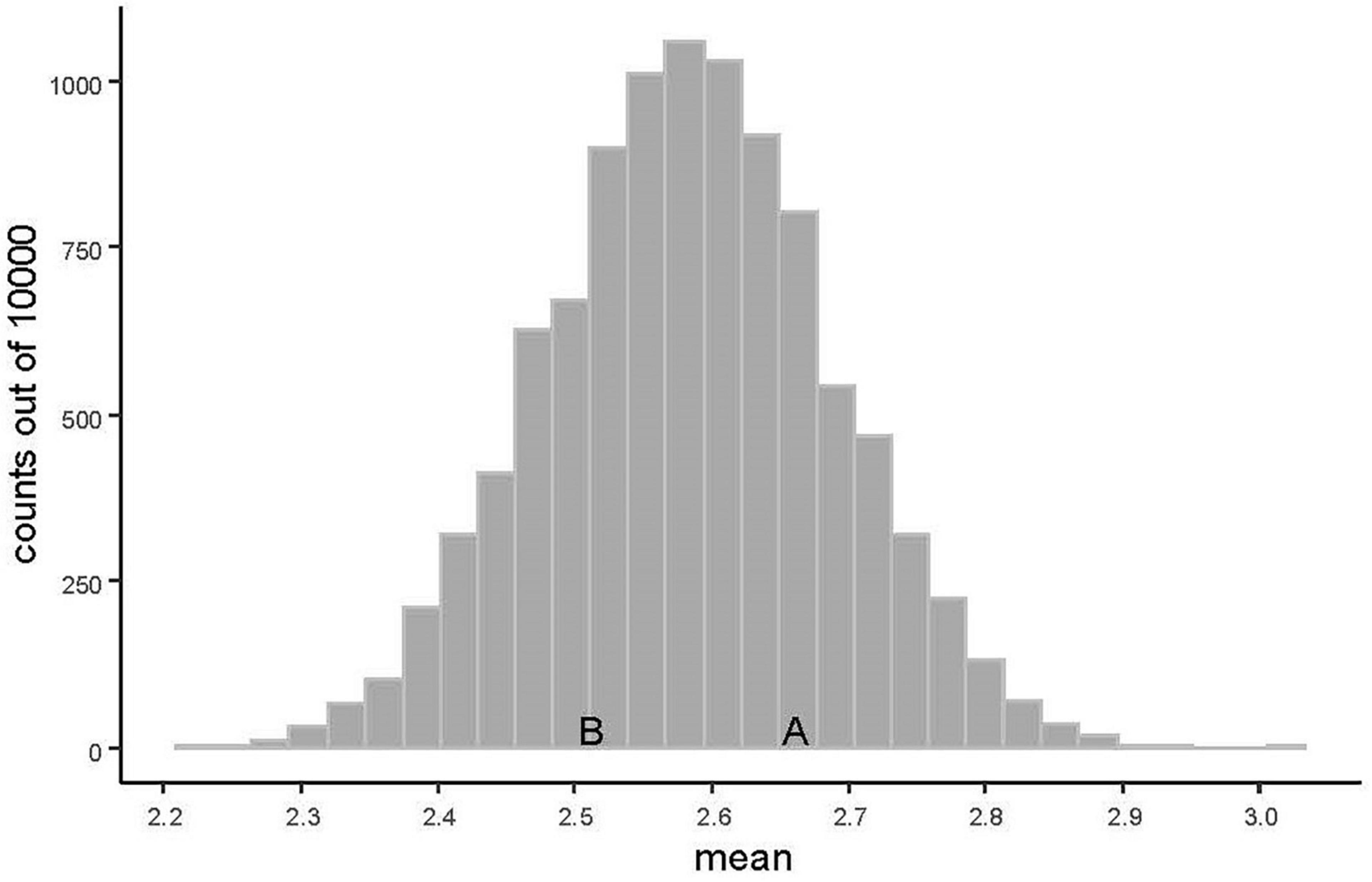
Figure 5. The distribution is based on 10,000 simulations of the mean value of 25 randomly chosen numbers from the values of visual plant performance. The letters correspond to the mean values for the graphical designs: light-dark (A) and complementary (B).
Outcome of Plant Biodiversity Classification of Tested Plants in the Study
For each of the 39 plant species we have created a biodiversity classification. Of the plant species, 17 were classified as being potentially attractive for pollinators (Figure 2). The light-dark (A) design included 10 plant species attractive for pollinators, while the complementary design (B) had 11 plant species (Figure 6, left). Compared with the hypergeometric distribution, neither of them were significantly different from a random choice of species. Of the plant species used in the colour schemes in Figure 2, 10 were classified as being native plant species. The light-dark design (A) included 8 native plant species and the complementary design (B) included 7 native plant species. Compared with the hypergeometric distribution, this is not significantly different from a random design (Figure 6, central). Of the plant species in Figure 2, 7 plant species were categorised as being valuable cultivars. The graphical designs light-dark (A) and complementary (B) both included 4 plant species valuable cultivars by POM. Compared with the hypergeometric distribution, this is what you could expect from a random choice, illustrated in Figure 6 (right).
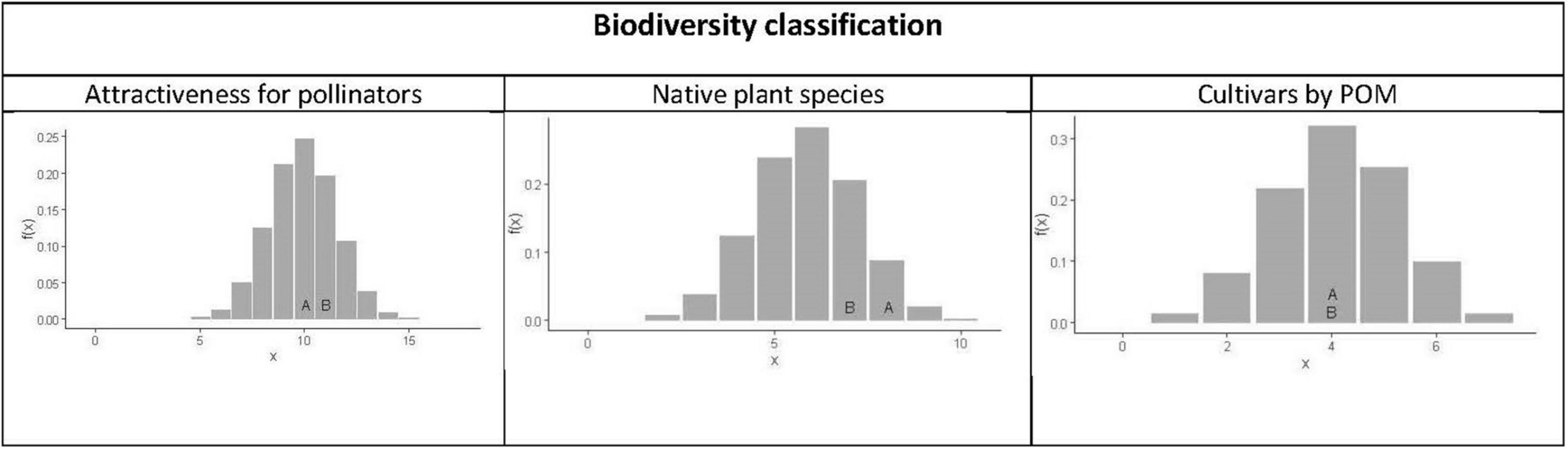
Figure 6. The probability function for the number of attractive plant species for pollinators (left), native plant species (central) and cultivars with conservation value of a random selection of the 43 species possible to use in the design. The letters A and B in the figures correspond to the number of species in the designs based on light-dark (A) and complementary (B) colour theory.
In conclusion, none of the graphical designs light-dark (A) and complementary (B) had an extreme number of plants attractive for pollinators, were native or culturally valuable, when compared to a random choice of plant species.
Outcome of Plant Species With a High Combined Value of Biodiversity and Visual Plant Performance
The top three species that had the highest combined biodiversity value and value for visual performance were: Dianthus arenarius, Achillea millefolium, and Armeria maritima. All three species are included in the graphical design light-dark (A) and two species in the complementary design (B). The combined values of the biodiversity value and visual plant performance is between 0 and 1, where 1 expresses the best possible combination of the visual plant performance and biodiversity value (attractiveness for pollinators, native plant species and valuable cultivars). The genus Dianthus with the species: Dianthus arenarius; Dianthus plumarius “Marieberg” and Dianthus deltoides had the highest sum between 0.95 and 0.76 (Figure 2). The calculations show that the values for the genus Geranium also were generally high, with a sum between 0.65 and 0.54.
Discussion
Colour Concepts as a Method for Achieving Aesthetics and Biodiversity Outcomes on Living Walls
The results from this study show that it is possible to design species rich living walls by implementing colour contrast concepts without compromising biodiversity. Both these outcomes, high colour contrast and biodiversity, were preferred by visitors to a botanical garden when they were asked to design meadows (Lindemann-Matthies and Bose, 2007). Flowers in particular have been shown to increase the attractive preference and the restorative effect of digitally designed urban green space (Wang et al., 2019), while flower colour diversity has been shown to influence both people’s aesthetic responses and pollinator interactions in direct sown meadow plantings (Hoyle et al., 2018). Colourful designs with a high diversity were created and selected as the most attractive when people could choose. Including both colour and diversity in designs of urban green space is of high importance to aesthetic preference and restorative potential in landscape design.
While neither of the graphical designs (i.e., light-dark and complementary) in this study had an extreme plant biodiversity classification compared to a random selection of plant species, the method provides consideration of this as a tool to improving plant selection in living walls. Even small patches of urban vegetation can support high species richness and diversity in cities, even for uncommon species (Vega and Küffer, 2021). Providing greater connections between these patches is crucial to achieve more urban habitat for biodiversity (Vega and Küffer, 2021) and living walls could be a way of providing these connections, especially as they have the potential to become “vertical corridors for wildlife” (Mayrand and Clergeau, 2018). These results were based on the colour determinations of plants growing on living walls facing south in the northern hemisphere (north-facing in the southern hemisphere). Plants colour will be related to the light intensity due to increased cuticle thickness and chlorophyll content on locations with high solar radiation as well as nutrient limitations. These specific designs are therefore restricted to living walls facing similar directions. Similarly, the visual plant performance will be directly related to the type of living wall system in use. However, the method used here—to design living walls guided by colour theory and using plants that are attractive for pollinators, have a level of “nativeness” and/or are valuable cultivars is more general and may be applied to any living wall system.
An intensification of cultural ecosystem services in green infrastructure installations could also lead to an increase in identity and senses of place (Eliasson et al., 2018). And while more aesthetically pleasing green infrastructure installations could foster more social cohesion and community, they are also not without some significant challenges. The use of colour concepts as a method in the design process can be particularly challenging for practitioners. We currently do not fully know the best way to design an outdoor composition focusing on the visual effects of colour contrasts. More research is needed on the perceived effects of a colour contrast composition giving contrasts to the perceived three-dimensional situation. In addition, studies of the overall colour performance of green installations in different contextual situations is required. It is also essential that practitioners involved in the planning- and design process have knowledge of the impact and potential effects of colour contrasts on human health and wellbeing.
Human Experiences Relative to Theoretical Colour Concepts on Living Walls
Previous studies show that the method for aesthetic outcome using colour contrasts as a design principle has the ability to affect human values, such as aesthetic enjoyment and appreciation. It has furthermore the capacity to contribute to increase visual quality and experiences of beauty, which in turn can be beneficial and positively associated with human health. This means that the conceptual design proposals developed in this study have a strong potential to affect humans positively, be highly valued by people and benefit human wellbeing. The design concepts use strategies already evaluated and proven to achieve harmonious arrangements and have the ability to influence visual qualities and viewer’s aesthetic satisfaction (e.g. Westland et al., 2007; Yilmaz et al., 2018). The conscious use of colour contrasts in plant compositions also has the potential to contribute to wellbeing outcomes, and increase experiences of pleasantness, beauty and harmony (Eroğlu et al., 2012; Oleksiichenko et al., 2018; Huang and Lin, 2019; Thorpert, 2019; Zhuang et al., 2021). In the light of the above reasoning, it is motivating to notice that green infrastructure installations, designed in a careful and conscious way, have the ability to be supportive for humans and contribute to the viewed quality in our daily life. However, it is important to point out that this study has been based on a two-dimensional way of thinking, which may suit living wall compositions, but will need complementary approaches in three dimensional planting designs.
Methodological Considerations
This study should be seen in the light of being explorative where the method allows the process to be creative in order to gain insightful information on the studied subject. We have investigated the possibilities to find a way to balance biodiversity and aesthetic outcomes from a theoretical point of view, and to bridge the knowledge gap in the design and planning process. The results give some indications that the method used could be applicable and a tool in the design of living walls and similar green infrastructure installations. However, the method has some limitations and should be seen as an attempt to create new ways to meet the requirements of designing with biodiverse vegetation that has multiple outcomes and functions as a goal.
In this study, we measured the colours from plants living in three types of living wall systems, where the systems were located facing south, respectively. How central each living wall system is for the intensification of vegetation colours has not been included in this study, but is something that can be prioritised in future studies. Also studies of the location of living walls in different latitude directions and its impact on plant colours is of importance to be able to create well-balanced aesthetic decisions in the future. A limitation in this study has been that only one time of determination has been included. A deeper understanding about seasonal changes and lightning conditions impact on plant colour performance could help ensure a balanced choice of plant mixtures and contribute to a holistic approach in relation to human health and wellbeing.
The methodology used to classify plant species with respect to their attractiveness for pollinators has some challenges. As noted, there is little empirical evidence of plant attraction for pollinators at a species level, in accordance to this we in this study included species based on their genus from the available literature. However, we are aware that attractiveness of plant species for pollinators can vary considerably within a genus, such as Geranium in Rollings and Goulson (2019). It has also to be noted that this way of classification leads to the fact that certain plant species as for example Dianthus deltoides were here classified as being attractive for pollinators, while the species investigated in the empirical study was a different one, here Dianthus barbatus (Rollings and Goulson, 2019). Nevertheless, it is assumed that the methodology chosen is justifiable in the context of this study. A further differentiation of plant species regarding their attractiveness would have been desirable but was dismissed because of the lack of data for all plant species.
This study has clear demarcation in the selection of choosable plant species and hence a limitation in the possibilities to develop design solutions. It is likely that additional plant species available in the design process could lead to an increased biodiversity value. It is also likely that an increase in the number of choosable plant species might provide major opportunities to create colourful design solutions and sustainable colour contrast effects. This in turn would lead to higher visual attractiveness and be more valued by people and increase their feeling of wellbeing.
The methodological approach used in this study, takes into consideration that both social and ecological plant traits is a useful way to make the plant choices in urban plantings more based on scientifically knowledge. However, the method needs to be validated, especially the weighting among the different values needs more research and inclusion of more parameters is recommended.
Conclusion and Future Studies
Each plant species selected for use in the two colour schemes were consistent with Itten’s concepts of “aesthetic attractiveness.” Based on knowledge from this study, we suggest that the use of proven theoretical colour models in the planning and design of species rich living walls and comparable green infrastructure installations have the possibility to give visually attractive urban installations, which in turn can be beneficial and positively associated with human health. The study furthermore shows that it is conceivable to design living walls containing multiple outcomes and functions. The study shows that the biodiversity value in the graphical colour designs used in the study is not significantly different from a random choice. Meaning that it is possible to add benefits of colour theory to a design of living walls without compromising biodiversity outcomes.
In conclusion, living walls have the potential to be more thoroughly designed and have the possibility to be more highly aesthetically pleasing and highly valued by people and increase their feeling of wellbeing, and at the same time deliver a high level of biodiversity values and species per m2.
The study also shows that green infrastructure installations designed in a well-balanced way have the potential to contribute toward both cultural- and regulatory ecosystem services. However, more work is needed to get a comprehensive representation of the visual feature, colour contrast and its potential as a design parameter in green infrastructure installations.
A deeper understanding about plant colours in various environmental settings during different light- and seasonal conditions could help ensure a balanced choice of colourful plants attractive for both humans and pollinators. This could also help identify optimal placement of coloured plants in relation to the surrounding contextual situation as well as in relation to colour theory. A follow-up from this study could be human subjective studies to assess preferences for different colour contrast models, also comparing studies of perceived plant colour, plants attractive for pollinators, indigenous and culturally valuable plant species and human experiences might enable an understanding of differences and similarities between perceived colour contrasts and biodiversity values. Longitudinal studies could also give indications about perceived colour contrast and biodiversity values in relation to human health and wellbeing and contribute to a more comprehensive understanding of the integration between biodiversity and colour theory in the design of living walls.
Data Availability Statement
The original contributions presented in the study are included in the article/supplementary material, further inquiries can be directed to the corresponding author/s.
Author Contributions
All authors listed have made a substantial, direct, and intellectual contribution to the work, and approved it for publication.
Conflict of Interest
The authors declare that the research was conducted in the absence of any commercial or financial relationships that could be construed as a potential conflict of interest.
Publisher’s Note
All claims expressed in this article are solely those of the authors and do not necessarily represent those of their affiliated organizations, or those of the publisher, the editors and the reviewers. Any product that may be evaluated in this article, or claim that may be made by its manufacturer, is not guaranteed or endorsed by the publisher.
References
Arriaza, M., Cañas-Ortega, J. F., Cañas-Madueñøo, J. A., and Ruiz-Aviles, P. (2004). Assessing the visual quality of rural landscapes. Landsc. Urban Plan. 69, 115–125. doi: 10.1016/j.landurbplan.2003.10.029
Azkorra, Z., Pérez, G., Coma, J., Cabeza, L. F., Burés, S., Álvaro, J., et al. (2015). Evaluation of green walls as a passive acoustic insulation system for buildings. Appl. Acoust. 89, 46–56. doi: 10.1016/j.apacoust.2014.09.010
Baldock, K. C. R., Goddard, M. A., Hicks, D. M., Kunin, W. E., Mitschunas, N., Osgathorpe, L. M., et al. (2015). Where is the UK’s pollinator biodiversity? The importance of urban areas for flower-visiting insects. Proc. Biol. Soc. 282:20142849. doi: 10.1098/rspb.2014.2849
Berthon, K., Thomas, F., and Bekessy, S. (2021). The role of ‘nativeness’ in urban greening to support animal biodiversity. Landsc. Urban Plann. 205:103959. doi: 10.1016/j.landurbplan.2020.103959
Bustami, R. A., Belusko, M., Ward, J., and Beecham, S. (2018). Vertical greenery systems: a systematic review of research trends. Build. Environ. 146, 226–237. doi: 10.1016/j.buildenv.2018.09.045
Cardinale, B. J., Duffy, E., Gonzalez, A., Hooper, D. U., Perrings, C., Venail, P., et al. (2012). Biodiversity loss and its impact on humanity. Nature 486, 59–67. doi: 10.1038/nature11148
Charoenkit, S., and Yiemwattana, S. (2016). Living walls and their contribution to improved thermal comfort and carbon emission reduction: a review. Build. Environ. 105, 82–94. doi: 10.1016/j.buildenv.2016.05.031
Chen, Q., Li, B., and Liu, X. (2013). An experimental evaluation of the living wall system in hot and humid climate. Energy Build. 61, 298–307. doi: 10.1016/j.enbuild.2013.02.030
Chiquet, C. (2014). The animal biodiversity of green walls in the urban environment. PhD.thesis. Stoke-on-Trent: Staffordshire University.
Chiquet, C., Dover, J. W., and Mitchell, P. (2013). Birds and the urban environment: the value of green walls. Urban Ecosyst. 16, 453–462. doi: 10.1007/s11252-012-0277-9
Collins, R., Schaafsma, M., and Hudson, M. D. (2017). The value of green walls to urban biodiversity. Land Use Policy. 64, 114–123. doi: 10.1016/j.landusepol.2017.02.025
Convention on biological diversity. (2021). Geneva Meetings 2022 nations convene in geneva to set stage for un biodiversity conference (COP-15). Available online at: https://www.cbd.int/ (accessed October 25, 2021).
Daniel, T. C., and Vining, J. (1983). “Measuring the quality of the natural environment – a psychophysical approach,” in Behaviour and the Natural Environment, eds I. Altman and J. F. Wohlwill (New York, NY: Plenum Press), 39–84. doi: 10.1111/joor.13296
Dover, J. (2015). Green Infrastructure. Incorporating Plants and Enhancing Biodiversity in Buildings and Urban Environments. Milton Park: Routledge.
Dvorak, B., Yang, S., Menotti, T., Pace, Z., Mehta, S., and Ali, A. K. (2021). Native plant establishment on a custom modular living wall system in a humid subtropical climate. Urban For. Urban Green. 63, 127–234.
Eliasson, I., Knez, I., and Fredholm, S. (2018). Heritage planning in practice and the role of cultural ecosystem services. Herit. Soc. 11, 44–69. doi: 10.1080/2159032X.2019.1576428
Eroğlu, E., Müderrisoğlu, H., and Kesim, G. A. (2012). The effect of seasonal change of plants compositions on visual perception. J. Environ. Eng. Landsc. Manag. 20, 196–205.
Filazzola, A., Shrestha, N., and MacIvor, J. S. (2019). The contribution of constructed green infrastructure to urban biodiversity: a synthesis and meta-analysis. J. Appl. Ecol. 56, 2131–2143. doi: 10.1111/1365-2664.13475
Fowdar, H. S., Hatt, B. E., Breen, P., Cook, P. L., and Deletic, A. (2017). Designing living walls for greywater treatment. Water Res. 110, 218–232. doi: 10.1016/j.watres.2016.12.018
Fussell, M., and Corbet, S. A. (1992). Flower usage by bumble-bees: a basis for forage plant management. J. Appl. Ecol. 29, 451–465. doi: 10.2307/2404513
Garbuzov, M., and Ratnieks, F. L. W. (2014). Listmania: the strengths and weaknesses of lists of garden plants to help pollinators. BioScience 64, 1019–1026. doi: 10.1093/biosci/biu150
Goulson, D. (2019). The insect apocalypse, and why it matters. Curr. Biol. 29, 942–995. doi: 10.1016/j.cub.2019.06.069
Goulson, D. (2021). Gardening for bumblebees – a practical guide to creating a paradise for pollinators.
Haaland, C. (2015). Abundances and movement of the Scarce Copper butterfly (Lycaena virgaureae) on future building sites at a settlement fringe in southern Sweden. J. Insect Conserv. 19, 255–264. doi: 10.1007/s10841-014-9708-7
Hooper, D. U., Adair, C., Cardinale, B., Byrnes, J., Hungate, B., Matulich, K., et al. (2012). A global synthesis reveals biodiversity loss as a major driver of ecosystem change. Nature 486, 105–108. doi: 10.1038/nature11118
Hooper, D. U., Chapin, F. S. III, Ewel, J. J., Hector, A., Inchausti, P., Lavorel, S., et al. (2005). Effects of biodiversity on ecosystem functioning: a consensus of current knowledge. Ecol. Monogr. 75, 3–35. doi: 10.1890/04-0922
Hoyle, H., Hitchmough, J., and Jorgensen, A. (2017). All about the ‘wow factor’? The relationships between aesthetics, restorative effect and perceived biodiversity in designed urban planting. Landsc. Urban plann. 164, 109–123. doi: 10.1016/j.landurbplan.2017.03.011
Hoyle, H., Norton, B., Dunnett, N., Richards, J. P., Russell, J. M., and Warren, P. (2018). Plant species or flower colour diversity? Identifying the drivers of public and invertebrate response to designed annual meadows. Landsc. Urban Plann. 180, 103–113. doi: 10.1016/j.landurbplan.2018.08.017
Huang, A., and Lin, Y. (2019). The effect of landscape colour, complexity and preference on viewing behaviour. Landsc. Res. 22, 1–14.
Itten, J. (2002). The Art of Color: The Subjective Experience and Objective Rationale of Color. New York, NY: Wiley & Sons.
Ives, C. D., Lentini, P. E., Threlfall, C. G., Ikin, K., Shanahan, D. F., Garrard, G. E., et al. (2016). Cities are hotspots for threatened species. Glob. Ecol. Biogeogr. 25, 117–126. doi: 10.1111/geb.12404
Jørgensen, L., Thorup-Kristensen, K., and Dresbøll, D. B. (2018). Against the wall—Root growth and competition in four perennial winter hardy plant species grown in living walls. Urban For. Urban Green. 29, 293–302. doi: 10.1016/j.ufug.2017.12.012
Kendal, Dave, Cindy, E., Hauser Georgia, E., Garrard, et al. (2013). Quantifying plant colour and colour difference as perceived by humans using digital images. PLoS One 8:1–11. doi: 10.1371/journal.pone.0072296
Kendal Dave Kathryn, J. H., Williams Nicholas, S. G., and Williams (2012). Plant traits link people’s plant preferences to the composition of their gardens. Landsc. Urban Plann. 105, 34–42. doi: 10.1016/j.landurbplan.2011.11.023
Kennedy, T. A., Naeem, S., Howe, K. M., Knops, J. M. H., Tilman, D., and Reich, P. (2002). Biodiversity as a barrier to ecological invasion. Nature 417, 636–638. doi: 10.1038/nature00776
Knapp, S., Kühn, I., Mosbrugger, V., and Klotz, S. (2008). Do protected areas in urban and rural landscapes differ in species diversity? Biodivers. Conserv. 17, 1595–1612. doi: 10.1007/s10531-008-9369-5
Kowarik, I. (1995). “Time lags in biological invasions with regard to the success and failure of alien species,” in Plant Invasions - General Aspects and Special Problems, eds P. Pyšek, K. Prach, M. Rejmánek, and M. Wade (Cambridge, MA: SPB Academic Publis), 15–38.
Kowarik, I. (2011). Novel urban ecosystems, biodiversity, and conservation. Environ. Pollut. 159, 1974–1983. doi: 10.1016/j.envpol.2011.02.022
Kühn, I., and Klotz, S. (2006). Urbanization and homogenization – comparing the floras of urban and rural areas in germany.biological conservation. 127, 292–300. doi: 10.1016/j.biocon.2005.06.033
Lindemann-Matthies, P., and Bose, E. (2007). Species richness, structural diversity and species composition in meadows created by visitors of a botanical garden in Switzerland. Landsc. Urban Plann. 79, 298–307. doi: 10.1016/j.landurbplan.2006.03.007
Lothian, A. (1999). Landscape and the philosophy of aesthetics: is landscape quality inherent in the landscape or in the eye of the beholder? Landscape and Urban Planning. 44, 177–198. doi: 10.1016/s0169-2046(99)00019-5
Madre, F., Clergeau, P. H., Machon, N., and Vergnes, A. (2015). Building biodiversity: Vegetated façades as habitats for spider and beetle assemblages. Glob. Ecol. Conserv. 3, 222–233. doi: 10.1016/j.gecco.2014.11.016
Manso, M., and Castro-Gomes, J. (2015). Green wall systems: a review of their characteristics. Renew. Sustain. Energy Rev. 41, 863–871. doi: 10.1016/j.rser.2014.07.203
Mårtensson, L., Wuolo, A., Fransson, A. M., and Emilsson, T. (2014). Plant performance in living wall systems in the Scandinavian climate. Ecol. Eng. 71, 610–614. doi: 10.1016/j.ecoleng.2014.07.027
Mårtensson, L. M., Fransson, A. M., and Emilsson, T. (2016). Exploring the use of edible and evergreen perennials in living wall systems in the Scandinavian climate. Urban For. Urban Green. 15, 84–88. doi: 10.1016/j.ufug.2015.12.001
Mayrand, F., and Clergeau, P. (2018). Green roofs and green walls for biodiversity conservation: a contribution to urban connectivity? Sustainability. 10:985. doi: 10.3390/su10040985
McKinney, M. L. (2002). Urbanization, biodiversity, and conservation. Bioscience 52, 883–890. doi: 10.1641/0006-3568(2002)052[0883:ubac]2.0.co;2
McKinney, M. L. (2008). Effects of urbanization on species richness: a review of plants and animals. Urban Ecosyst. 11, 161–176. doi: 10.1016/j.envint.2015.09.013
Naeem, S., Knops, J. M. H., Tilman, D., Howe, K. M., Kennedy, T., and Gale, S. (2000). Plant diversity increases resistance to invasion in the absence of covarying extrinsic factors. Oikos 91, 97–108. doi: 10.1034/j.1600-0706.2000.910108.x
Nagle, L., Echols, S., and Tamminga, K. (2017). Food production on a living wall: Pilot study. J. Green Build. 12, 23–38. doi: 10.3992/1943-4618.12.3.23
Oleksiichenko, N., Gatalska, N. V., and Mavko, M. (2018). The colour-forming components of park landscape and the factors that influence the human perception of the landscape colouring the colour-forming components of park landscape colouring. Theor. Empir. Res. Urban Manag. 13, 38–52.
Pérez-Urrestarazu, L., Blasco-Romero, A., and Fernández-Cañero, R. (2017). Media and social impact valuation of a living wall: the case study of the sagrado corazon hospital in seville (Spain). Urban For. Urban Green. 24, 141–148. doi: 10.1016/j.ufug.2017.04.002
Perini, K., Ottelé, M., Haas, E. M., and Raiteri, R. (2013). Vertical greening systems, a process tree for green façades and living walls. Urban Ecosystems. 16, 265–277. doi: 10.1007/s11252-012-0262-3
Polat, A. T., and Akay, A. (2015). Relationships between the visual preferences of urban recreation are users and various landscape design elements. Urban For. Urban Green. 14, 573–582. doi: 10.1016/j.ufug.2015.05.009
Radić, M., Brković Dodig, M., and Auer, T. (2019). Green facades and living walls—a review establishing the classification of construction types and mapping the benefits. Sustainability 11:4579. doi: 10.3390/su11174579
Ridzuan, N. H., Farouk, S. A., Razak, S. A., Avicor, S. W., Taib, N., and Hamzah, S. N. (2021). Insect biodiversity of urban green spaces in Penang Island, Malaysia. Int. J. Trop. Insect Sci 42, 275–284. doi: 10.1007/s42690-021-00543-2
Riley, B. (2017). The state of the art of living walls: Lessons learned. Build. Environ. 114, 219–232. doi: 10.1016/j.buildenv.2016.12.016
Rockström, J., Steffen, W., Noone, K., Persson, Å, Chapin, F. S. III, Lambin, E., et al. (2009). Planetary boundaries:exploring the safe operating space for humanity. Ecol. Soc. 14:32.
Rollings, R., and Goulson, D. (2019). Quantifying the attractiveness of garden flowers for pollinators. J. Insect Conserv. 23, 803–817. doi: 10.1007/s10841-019-00177-3
Teräs, I. (1976). Flower visits of bumblebees, bombus latr. (hymenoptera, apidae), during one summer. Ann. Zool. Fennici 13, 200–232.
Thorpert, P. (2019). Green Is Not Just Green. Human colour perception in urban green contexts. Ph.D.thesis. Alnarp: Department of Landscape Architecture, Planning and Management. Swedish University of Agricultural Sciences.
Tkalčič, M., and Tasič, J. F. (2003). Colour spaces: Perceptual, Historical and Applicational Background. Ljubljana: EUROCON, 304–308.
Ulrich, R. S. (1983). Aesthetic and affective response to natural environment. Hum. Behav. Environ. Adv. Theory Res 6, 85–125. doi: 10.3389/fnhum.2021.676032
van den Bosch, M., and Ode Sang, Å (2017). Urban natural environments as nature-based solutions for improved public health – a systematic review of reviews. Environ. Res 158, 373–384. doi: 10.1016/j.envres.2017.05.040
Vega, K. A., and Küffer, C. (2021). Promoting wildflower biodiversity in dense and green cities: The important role of small vegetation patches. Urban For. Urban Green. 62, 127–165.
Wagner, D. L., Grames, E. M., Forister, M. L., Berenbaum, M. R., and Stopak, D. (2021). Insect decline in the anthropocene: death by a thousand cuts. Proc Natl Acad Sci U.S.A. 118:e2023989118. doi: 10.1073/pnas.2023989118
Wang, R., Zhao, J., Meitner, M. J., Hu, Y., and Xu, X. (2019). Characteristics of urban green spaces in relation to aesthetic preference and stress recovery. Urban For. Urban Green. 41, 6–13. doi: 10.1016/j.ufug.2019.03.005
Weerakkody, U., Dover, J. W., Mitchell, P., and Reiling, K. (2017). Particulate matter pollution capture by leaves of seventeen living wall species with special reference to rail-traffic at a metropolitan station. Urban for. Urban Green. 27, 173–186. doi: 10.1016/j.ufug.2017.07.005
Westland, S., Laycock, K., Cheung, V., Henry, P., and Mahyar, F. (2007). Colour Harmony. Colour Design Creativity 1, 1–15.
Wilcox, B. A. (1984). “In situ conservation of genetic resources: determinants of minimum area requirements,” in National Parks, Conservation and Development, Proceedings of the World Congress on National Parks, eds J. A. McNeely and K. R. Miller (Washington, DC: Smithsonian Institution Press), 18–30. doi: 10.1371/journal.pone.0255418
Wong, N. H., Tan, A. Y. K., Tan, P. Y., and Wong, N. C. (2010). Acoustics evaluation of vertical greenery systems for building walls. Build. Environ. 45, 411–420. doi: 10.1016/j.buildenv.2009.06.017
Yilmaz, S., Özgüner, H., and Mumcu, S. (2018). An aesthetic approach to planting design in urban parks and greenspaces. Landsc. Res. 43, 965–983.
Keywords: green infrastructure, landscape aesthetics, living wall, green wall, ecosystem services pollinators, environmental appraisal
Citation: Thorpert P, Rayner J, Haaland C, Englund J-E and Fransson A-M (2022) Exploring the Integration Between Colour Theory and Biodiversity Values in the Design of Living Walls. Front. Ecol. Evol. 10:804118. doi: 10.3389/fevo.2022.804118
Received: 28 October 2021; Accepted: 23 March 2022;
Published: 19 April 2022.
Edited by:
Jennifer N. W. Lim, University of Wolverhampton, United KingdomReviewed by:
Olyssa Starry, Portland State University, United StatesDave Kendal, University of Tasmania, Australia
Copyright © 2022 Thorpert, Rayner, Haaland, Englund and Fransson. This is an open-access article distributed under the terms of the Creative Commons Attribution License (CC BY). The use, distribution or reproduction in other forums is permitted, provided the original author(s) and the copyright owner(s) are credited and that the original publication in this journal is cited, in accordance with accepted academic practice. No use, distribution or reproduction is permitted which does not comply with these terms.
*Correspondence: Petra Thorpert, UGV0cmEuVGhvcnBlcnRAc2x1LnNl