- 1Department of Biology, University of Miami, Coral Gables, FL, United States
- 2Department of Biology, University of Florence, Florence, Italy
- 3Department of Evolution, Ecology and Behavior, School of Integrative Biology, University of Illinois at Urbana-Champaign, Champaign, IL, United States
- 4Department of Biology, University of Rochester, Rochester, NY, United States
Brain plasticity is widespread in nature, as it enables adaptive responses to sensory demands associated with novel stimuli, environmental changes and social conditions. Social Hymenoptera are particularly well-suited to study neuroplasticity, because the division of labor amongst females and the different life histories of males and females are associated with specific sensory needs. Here, we take advantage of the social wasp Polistes dominula to explore if brain plasticity is influenced by caste and sex, and the exploitation by the strepsipteran parasite Xenos vesparum. Within sexes, male wasps had proportionally larger optic lobes, while females had larger antennal lobes, which is consistent with the sensory needs of sex-specific life histories. Within castes, reproductive females had larger mushroom body calyces, as predicted by their sensory needs for extensive within-colony interactions and winter aggregations, than workers who frequently forage for nest material and prey. Parasites had different effects on female and male hosts. Contrary to our predictions, female workers were castrated and behaviorally manipulated by female or male parasites, but only showed moderate differences in brain tissue allocation compared to non-parasitized workers. Parasitized males maintained their reproductive apparatus and sexual behavior. However, they had smaller brains and larger sensory brain regions than non-parasitized males. Our findings confirm that caste and sex mediate brain plasticity in P. dominula, and that parasitic manipulation drives differential allocation of brain regions depending on host sex.
Introduction
Brain plasticity enables adaptive responses to different sensory demands such as novel stimuli, changing environments and social conditions (Taborsky and Oliveira, 2012; O’Donnell et al., 2013; Anderson and Finlay, 2014; Kamhi et al., 2017; Jernigan et al., 2021). For instance, neuroplasticity has evolved across many lineages as a result of variable selective pressures acting on the cognitive demands of sensory and perceptual systems (Barton et al., 1995; Barton and Harvey, 2000; Catania, 2005). Since brain tissue is energetically expensive, plasticity in specific brain structures may be linked to the sensory and processing needs of adaptive behaviors (Isler and Van Schaik, 2006; Niven and Laughlin, 2008; Riveros and Gronenberg, 2010; Pyza, 2013; Montgomery et al., 2016; Dunbar and Shultz, 2017; Rozanski et al., 2021). Investment in neural tissue may be mediated by experience (Jones et al., 2009; Riveros and Gronenberg, 2010; Cabirol et al., 2018), diet (Murphy et al., 2014; DeCasien et al., 2017), environmental stimuli (Burns et al., 2009; Axelrod et al., 2018), tradeoffs with reproduction (Pitnick et al., 2006), and/or endocrine factors (Ball et al., 2002; Lendvai et al., 2013). Previous studies also provide compelling evidence for how neuroplasticity adaptively supports the division of labor in complex insect societies and matches sensory specialization (Groh and Meinertzhagen, 2010; Giraldo et al., 2013; Kamhi et al., 2017; Gordon et al., 2019; Arganda et al., 2020; Baudier et al., 2021).
Given that specialized behaviors are associated with a range of caste-specific sensory needs, corresponding investment in neural tissue is expected (Gronenberg et al., 1996; Ehmer et al., 2001; O′Donnell et al., 2007; Seid et al., 2011; Rehan et al., 2015; Arganda et al., 2020; Penick et al., 2021). Therefore, social insects provide the opportunity to compare differential investment in sensory brain regions between castes and sexes with different life histories (Gronenberg and Riveros, 2009; Beani et al., 2014), while controlling for genetic background. In insect brains, visual input travels from the eyes and is received and processed by the optic lobes, while olfactory input is received by the antennal lobes (Strausfeld, 1989; Anton and Homberg, 1999; Gronenberg and Hölldobler, 1999). From these lower-order sensory neuropils, projection neurons convey the computed information to the mushroom bodies (Akalal et al., 2006). In these higher-order brain centers, the chemical and visual information is further processed and integrated with internal information by intrinsic neurons and finally projected to premotor areas. The substructures of the mushroom body calyces, act as learning and memory centers that integrate sensory information and foraging experience. Specifically, olfactory information is processed in the lip, the visual information in the collar, and both sensory stimuli in the basal ring (Ehmer and Hoy, 2000; Akalal et al., 2006; Fahrbach, 2006). Finally, the central complex is implicated in spatial navigation (Pfeiffer and Homberg, 2014; Honkanen et al., 2019; Le Moël et al., 2019).
Here, we take advantage of the primitively eusocial paper wasp Polistes dominula to test how brain plasticity is associated with behavioral flexibility (Pardi, 1996; O’Donnell et al., 2014, 2018; O’Donnell and Bulova, 2017; Rozanski et al., 2021). In this temperate wasp species, the recognition of nestmates, caste, and sex relies on both chemical and visual cues (Dani et al., 2001; Cappa et al., 2016, 2020; Beani et al., 2019; Cini et al., 2019). Females are morphologically similar and organized in a flexible caste system, according to a dominance hierarchy (Pardi, 1948). The reproductive castes emerge in mid-summer and consist of males and gynes that will become foundresses the following spring. Gynes remain on the natal nest without performing any colony tasks and then mate, form winter aggregations, and enter diapause (Reeve, 1991). The following spring, foundresses initiate construction of nests and compete for the dominant position establish a dominance hierarchy. The dominant foundress monopolizes egg-laying, and the first offspring will become workers (Strassmann et al., 2004). Therefore, subordinate foundresses and workers are involved in nest building and defense, the rearing of larvae, and foraging (West-Eberhard, 1969). In the mid-summer, adult males and new gynes emerge. Males abandon the nest early after emergence and display lek-behavior at landmarks where they mate with gynes and die at the end of fall (Beani, 1996; Beani et al., 2014). Gynes store sperm for reproduction during the following spring (Cappa et al., 2013).
In addition, P. dominula is also parasitized by the strepsipteran insect Xenos vesparum, which provides a great opportunity to explore the effect of this parasite in allocation of brain tissue (Hughes and Libersat, 2018; Libersat et al., 2018). X. vesparum larvae enter worker wasp larvae in the early summer and develop inside their hosts (Manfredini et al., 2010). When the hosts emerge as adults, the parasites undergo pupation and behaviorally manipulate their hosts (Hughes et al., 2004b). After metamorphosis, adult female parasites remain as obligate endoparasites inside the host, while adult males emerge from the host, and mate with females. Parasite pupae decrease the size of corpora allata in female hosts and castrate them by irreversibly inhibiting ovary development (Strambi and Strambi, 1973; Strambi et al., 1982; Hughes et al., 2004b; Beani, 2006). Parasitized workers abandon the colony and aggregate on selected plants where parasite mating occurs (Hughes et al., 2004b; Beani et al., 2018). In contrast, male wasps are less-frequently parasitized, and instead maintain their reproductive apparatus and sexual behavior (Beani et al., 2011; Cappa et al., 2014). After mating, female parasites extend the lifespan of their worker host to overwinter like a gyne (Beani et al., 2021). Instead, female and male hosts parasitized by males die at the end of the summer (Beani et al., 2021).
While brain plasticity within and across social insects has been extensively studied (Godfrey and Gronenberg, 2019), no studies have explored plasticity within a species that has morphologically similar individuals, various colony tasks, and a parasite that potentially alters brain morphology. We predicted that the relative volume of selected brain regions reflects specific sensory needs for each caste and sex (reproductive females, female workers, and males) (Rozanski et al., 2021). We also predicted higher volume of visual regions in males to detect and identify potential mates or rival males in a lek, compared to females. On the contrary, we expected more olfactory processing by females compared to males due to social interactions in the colony. We also tested for the effect of parasitic manipulation in brain allometry. We predicted a stronger parasite effect in the brain of workers, because they are castrated and show strong behavioral manipulation, compared to parasitized males who reproduce and show no changes in behavior. Finally, little is known about the specific neuroendocrine effects of female and male parasites toward female and male hosts. Based on the strong behavioral alterations induced by the parasite on worker wasps (Strambi and Strambi, 1973; Beani et al., 2017), we expected a reduction of corpora allata regardless of parasite sex. Conversely, given the mild parasite impact on male hosts (Cappa et al., 2014; Beani et al., 2017), we predicted a small effect of X. vesparum on male corpora allata size.
Materials and Methods
Field Collection
We collected reproductive females (N = 10 foundresses and N = 9 gynes), non-parasitized workers (N = 10), workers parasitized by one X. vesparum female (N = 11) or by one X. vesparum male (N = 11), non-parasitized males (N = 10), and males parasitized by one or two X. vesparum males (N = 9). All samples were collected during the first days of July of 2016 and 2018, in the plain of Sesto Fiorentino (Tuscany, Italy). Males parasitized by X. vesparum females and parasitized gynes are lacking in our data set, due to the protandrous emergence of X. vesparum (Hughes et al., 2004a), and to the scarcity of male and gyne reproductive larvae during the infection period in the early summer. Wasps from each caste emerge synchronously and at specific times throughout the summer, which controls for age (Molina and O’Donnell, 2008) and seasonality effects that can influence brain development. Non-parasitized and parasitized hosts are easily distinguished by inspecting for parasite extrusions between the abdominal tergites, and parasites can be identified as female or male because of the shape of their pupal sac (Figure 1B). Finally, to verify which individuals were parasitized, their abdomens were preserved and dissected in 70% ethanol. We confirmed the absence of pupal parasites in female workers and males without any visible signs of parasitism. In parasitized workers and males, we also confirmed sex of the parasites and gonad development predicted for each category (Figure 1C). Finally, we preserved each head capsule individually in a glyoxal fixative for subsequent histological sectioning (Prefer, Anatech Ltd., Battle Creek, United States).
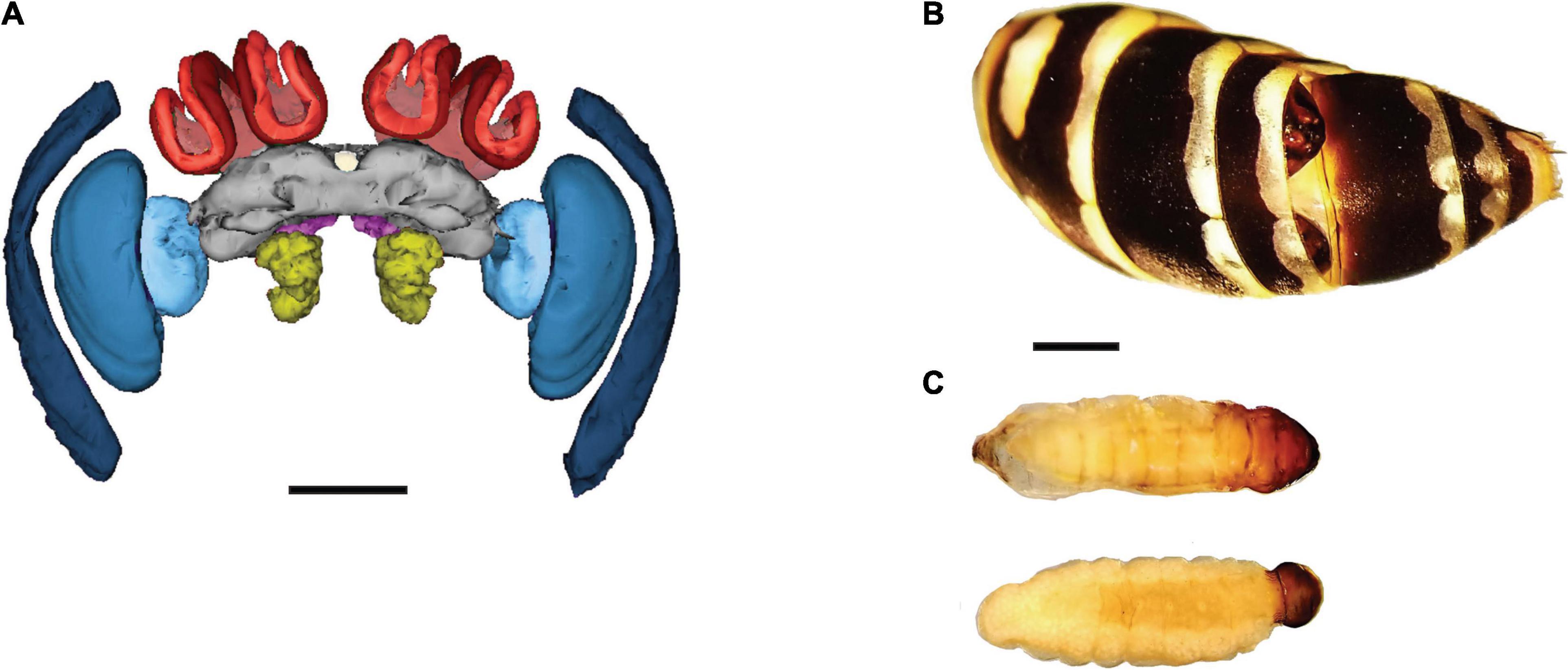
Figure 1. Study system: a female brain, the abdomen of a parasitized female, and Xenos vesparum parasites. (A) Frontal view of a 3-D reconstructed brain of a female Polistes dominula. The sensory brain regions are color coded: substructures of the optic lobes (blue), antennal lobes (yellow), lip (bright red), collar (dark red) and the central complex (white). All sensory regions are normalized by the central brain (gray). For reference, the subesophageal zone is shown in bright pink and the mushroom body peduncles in light red. Scale bar = 500 μm. (B) A host abdomen shows a male X. vesparum pupa (top) and the cephalothorax of a neotenic female X. vesparum pupa (bottom) extruding from the tergites. (C) Same larvae of X. vesparum after being dissected from the host’s abdomen: male parasite on top and female on the bottom. (B,C) Are scaled (scale bar = 2 mm).
Histology and Measurement of Brain Regions
We first dehydrated each head capsule with a series of increasing ethanol and acetone concentrations. We then used the established concentrations for the Embed 812 resin kit (Electron Microscopy Sciences, Hatfield, United States) to embed the head capsule while maintaining their brain dimensions, following the histology protocol for Polistes wasps (O’Donnell et al., 2015; Rozanski et al., 2021). The samples were moved repeatedly between an open-air rocking shaker (Thermo Fisher Scientific, Waltham, United States) and a vacuum to improve infiltration of the solvent.
Next, we placed each embedded head capsule in an individual plastic mold filled with the same concentration of resin in an oven at 60°C. After 72 h, the resin was polymerized. We sectioned each brain in consecutive coronal sections with a thickness of 17 μm and stained the tissue with toluidine blue, to visualize clearly defined boundaries for each brain region. We photographed the consecutive brain sections for each specimen using a Canon EOS 5D Mark III mounted on a Leica DM IL LED microscope at 4 x magnification, including a scale of 1,000 μm.
Using the AxioVision SE64 (Zeiss, NY, United States), we outlined the area for each individual brain region (Figure 1A). We traced the antennal lobes and the three substructures of the optic lobes: medulla, lobula and lamina. We also traced the two calyx substructures process olfactory and visual stimuli: lip and collar, respectively, and the central complex. The remaining structures in the protocerebrum were grouped as the central brain (Figure 1A), following the established method for this species (Rozanski et al., 2021) and ants (Sheehan et al., 2019). Outlining of brain regions was done blind to the category for each sample. We quantified each brain region for every other section per brain, as this method shows high accuracy (i.e., < 3.5% error for 34 μm thick sections) (Ehmer and Hoy, 2000). We then determined the volume for each region by multiplying the area by the distance between sections (34 μm). We generated the 3-D brain reconstruction by using the software RECONSTRUCT (Fiala, 2005). To control for the effect of head size, we measured head width. Finally, we determined the cross-sectional area of the corpora allata by measuring the diameter of one of the two glands, following the method previously used for this species (Strambi and Strambi, 1973).
Statistical Analyses
We explored if differential volume in specific brain regions among phenotypes was the result of changes in allometric scaling (Ott and Rogers, 2010; Eberhard and Wcislo, 2011; Seid et al., 2011; O’Donnell et al., 2013; Stöckl et al., 2016; Sheehan et al., 2019). In P. dominula, the optic lobe represents on average 42% of the brain and may have an effect on relative neuropil scaling (Rozanski et al., 2021). Therefore, we compared investment in each sensory brain region to the central brain, instead of by the whole brain (Ott and Rogers, 2010; Stöckl et al., 2016; Sheehan et al., 2019).
We used the allometric equation y = a*xβ for the scaling relationship between brain regions x and y. We then logarithmically transformed the estimates β (slope) and α (intercept of a regression) by using the linear equation log(y) = βlog(x) + log(a), where log(a) = α (Dubois, 1897; Huxley and Teissier, 1936). Standardized Major (SMA) regression analyses were calculated by using the SMATR v.3 package for R (WartonI, Wright et al., 2006, Warton et al., 2012).
First, we tested for a common slope among non-parasitized phenotypes as a baseline comparison, consisting of males, reproductive females and workers (H0 = βmales = βreproductives = βworkers). We implemented log-likelihood tests followed by post hoc pairwise comparisons provided in the SMATR package. Since allometric scaling did not differ significantly between foundresses and gynes, we pooled them under a new category called “reproductives.” Second, we tested for a common slope among non-parasitized workers, with one female parasite and with one male parasite, and between non-parasitized and parasitized males. The volume of brain regions did not differ between male wasp parasitized by one or two male X. vesparum, so we also pooled them. We compared allometric changes in the whole brain with head width, central brain with whole brain, and pooled sensory regions with changes in the central brain. Finally, we explored the allometric relationship between each sensory brain region and central brain, following our established method for this wasp species (Rozanski et al., 2021).
For categories that shared a common slope, we used log-likelihood tests to calculate the slope index (SI) for the brain region comparisons described above. The SI determined if a brain region is allometric (β≠ 1), meaning that sensory brain region (y)/central brain (x) would change with size. We also used a Wald Test to calculate the common shift (H0 = equal axis among phenotypes), for any shift along the x axis. Finally, we calculated how much larger a sensory region (y) is compared to the central brain (y), by using a grade shift index (GSI) to compare phenotypes (i.e., H0 = αmales = αreproductives = αworkers). The GSI reflected changes in intercept α (elevation) with no changes in the slope β. This method facilitates pairwise volumetric comparisons between phenotypes (i.e., eα males– α reproductives), by implementing a Wald test. For example, if GSI > 1, males had larger volume of a brain region compared to reproductives, and if GSI < 1 the relationship would be inverse. We specify the direction of change for each of the analyzed categories in “Results” section and Supplementary Tables 1, 2. Lastly, we also ran a Kruskal-Wallis test with subsequent pairwise comparisons to determine corpora allata growth across castes and to test the effect of both parasite and host sex.
Results
Investment in Sensory Regions by Caste and Sex
All brain regions, except for the central complex showed a common slope, but had differences in the GSI, common shift and/or SI depending on the specific region (Figure 2 and Supplementary Table 1). Males and reproductive females had proportionally larger pooled sensory regions compared to workers (GSI = 1.056, P = 0.01 and GSI = 1.036, P = 0.006, respectively, Figure 2C). Males had proportionally smaller antennal lobes when compared to reproductive females (GSI = 0.87, P < 0.001), as an effect of both changes in elevation and a common shift (Figure 2D and Supplementary Table 1). Males also had smaller antennal lobe volume than workers (GSI = 0.926, P = 0.002, Figure 2D). Males had larger optic lobes than reproductive females (GSI = 1.064, P = 0.001) and workers (GSI = 1.103, P < 0.001, Figure 2E and Supplementary Table 1). Within females, reproductives had larger antennal lobes (GSI = 1.057, P = 0.002, Figure 2D) and calyces compared to workers (GSI = 1.042, P = 0.003, Figure 2F). Reproductives had increased optic lobe volume compared to workers (GSI = 1.037, P = 0.02, Figure 2E). Finally, workers showed an isometric increase in the central complex (P = 0.052), in contrast to a hypoallometric reduction of this navigational brain region in reproductive females and males (Figure 2I).
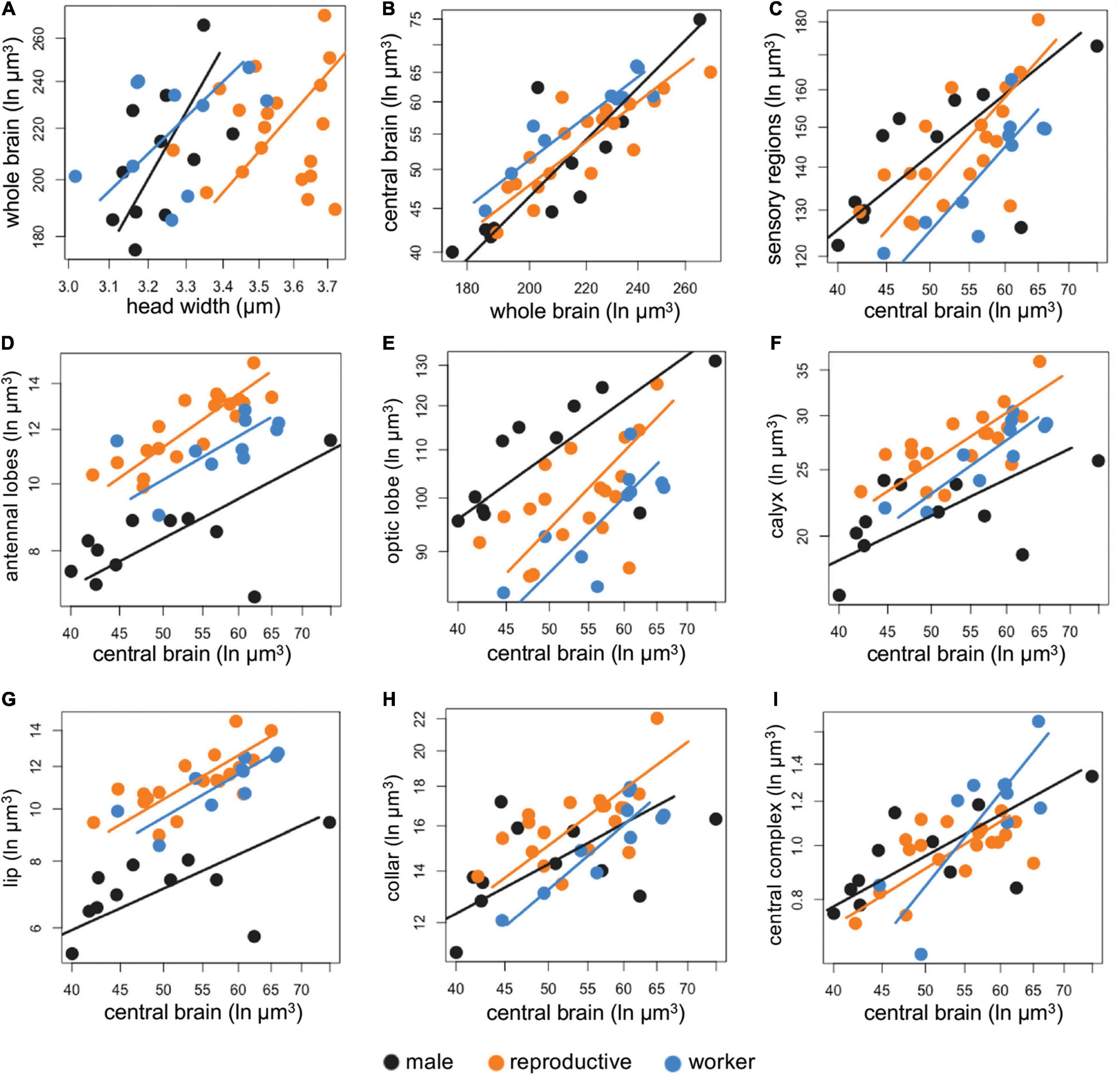
Figure 2. (A–I) Comparison of the investment in sensory regions by sex and caste. The volume of each brain region was log-transformed, and each dot represents one individual. The males are depicted in black, reproductives (gynes and foundresses) in dark orange and workers in blue. The corresponding colored lines represent the slope for each category, and each dot represents an individual. Given that most comparisons shared a common slope, see Supplementary Table 1 for full Standardized major axis (SMA) results.
Investment in Sensory Regions by Parasitized and Non-parasitized Wasps
Workers parasitized by one female or one male X. vesparum showed no differences in allocation of most sensory brain regions, compared to non-parasitized workers (Figures 3A, C, G). Indeed, non-parasitized workers shared a common slope with workers with a female or a male X. vesparum, and no volumetric differences in the antennal lobes (Figure 3D) or the optic lobes (Figure 3E and Supplementary Table 1). However, we did find a change in the slope index of the whole brain in workers parasitized by a female, compared to non-parasitized workers or those parasitized by a male (P < 0.001, Figure 3B and Supplementary Table 1). Workers parasitized by one female had an isometric pattern, resulting in larger calyces (P = 0.031, Figure 3F) and collars (P = 0.045, Figure 3H), than non-parasitized workers and those parasitized by one male. Lastly, workers with one male parasite had a hypoallometric reduction of the central complex in comparison to non-parasitized workers and those parasitized by a female (P = 0.027, Figure 3I).
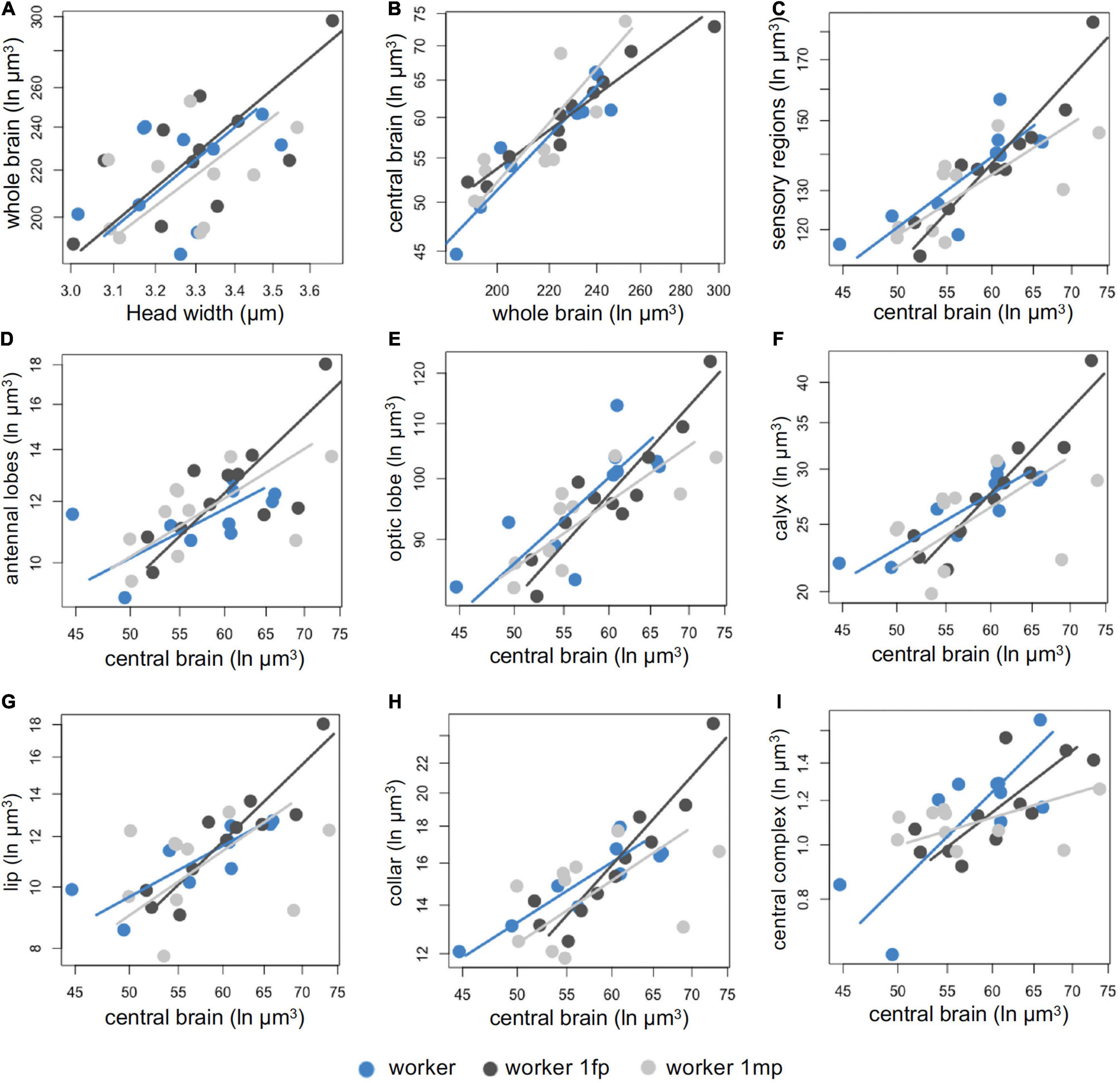
Figure 3. (A–I) Comparison of the investment in sensory regions by parasitized and non-parasitized workers. Categories are depicted as non-parasitized workers (blue), workers parasitized by one female X. vesparum (dark gray) and workers parasitized by one male X. vesparum (light gray). The corresponding color-coded line represents the slope for each category and each dot represents an individual. Some comparisons shared a common slope, see Supplementary Table 1 for full statistical results.
In contrast, parasitized and non-parasitized males showed differential allocation toward specific brain regions. They shared a common slope and differences in grade shifts for the following brain regions: whole brain, antennal brain, lip, and central complex (Supplementary Table 2). Parasitized males had a proportionally smaller whole brains than non-parasitized males (GSI = 1.15, P < 0.001, Supplementary Table 2 and Figure 4A). However, due to a common shift along the main slope axis, parasitized males had proportionally large antennal lobes (P = 0.01, Figure 4D), lip (P = 0.001, Figure 4G) and central complex (P < 0.001, Figure 4I) compared to non-parasitized males (Supplementary Table 1). In contrast, parasitized males showed a disproportionately reduced volume of the central brain (P = 0.02, Figure 4B), but disproportionately large volume of pooled sensory regions (P = 0.03 Figure 4C), optic lobes (P = 0.03, Figure 4D), calyces (P = 0.04, Figure 4F), and collar (P = 0.02, Figure 4H) compared to non-parasitized males (Figure 4 and Supplementary Table 2).
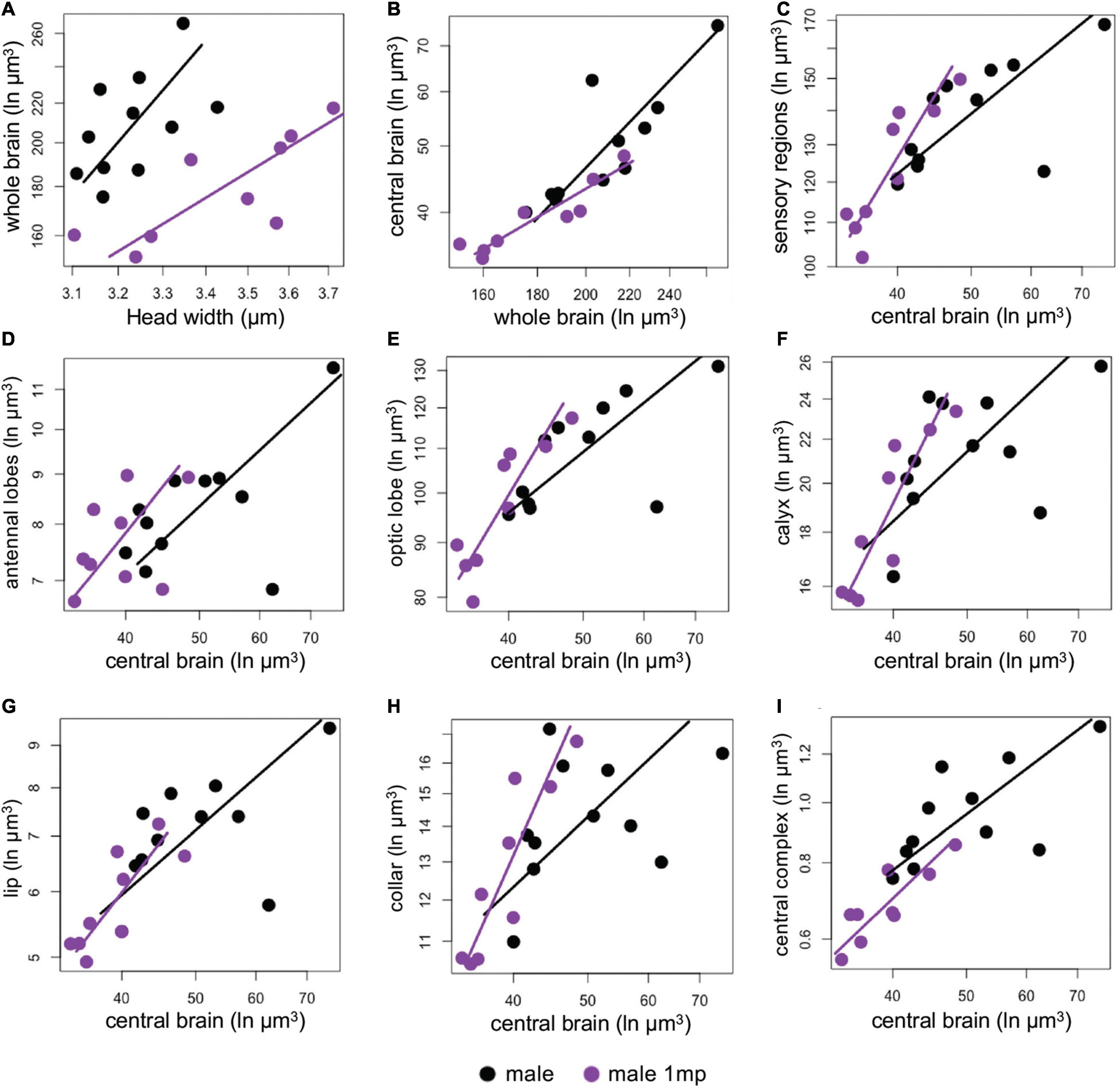
Figure 4. (A–I) Comparison of the investment in sensory regions by parasitized (purple) and non-parasitized males (black). SMA fits are log-transformed per categories with the lines based on intercepts and slopes (purple for parasitized and black for non-parasitized males). Most volumetric comparisons did not share a common slope, see Supplementary Table 2 for full SMA tests.
Corpora Allata Development According to Sex, Caste, and Parasitism
The corpora allata were significantly smaller in all males compared to females (χ2 = 46.86, df = 6, P < 0.001, Figure 5). Although not significant, foundresses showed the expected trend toward large corpora allata compared to gynes (Z = 2.02, P = 0.07) and workers (Z = 0.79, P = 0.06). Post hoc pairwise tests showed no significant differences in workers parasitized by one female (Z = 1.62, P = 0.1) and by one male (Z = 4.41, P = 0.5) compared to non-parasitized workers. Gland size also did not differ according to parasite sex (Z = −0.84, P = 0.39), or between parasitized and non-parasitized males (Z = −0.02, P = 0.98).
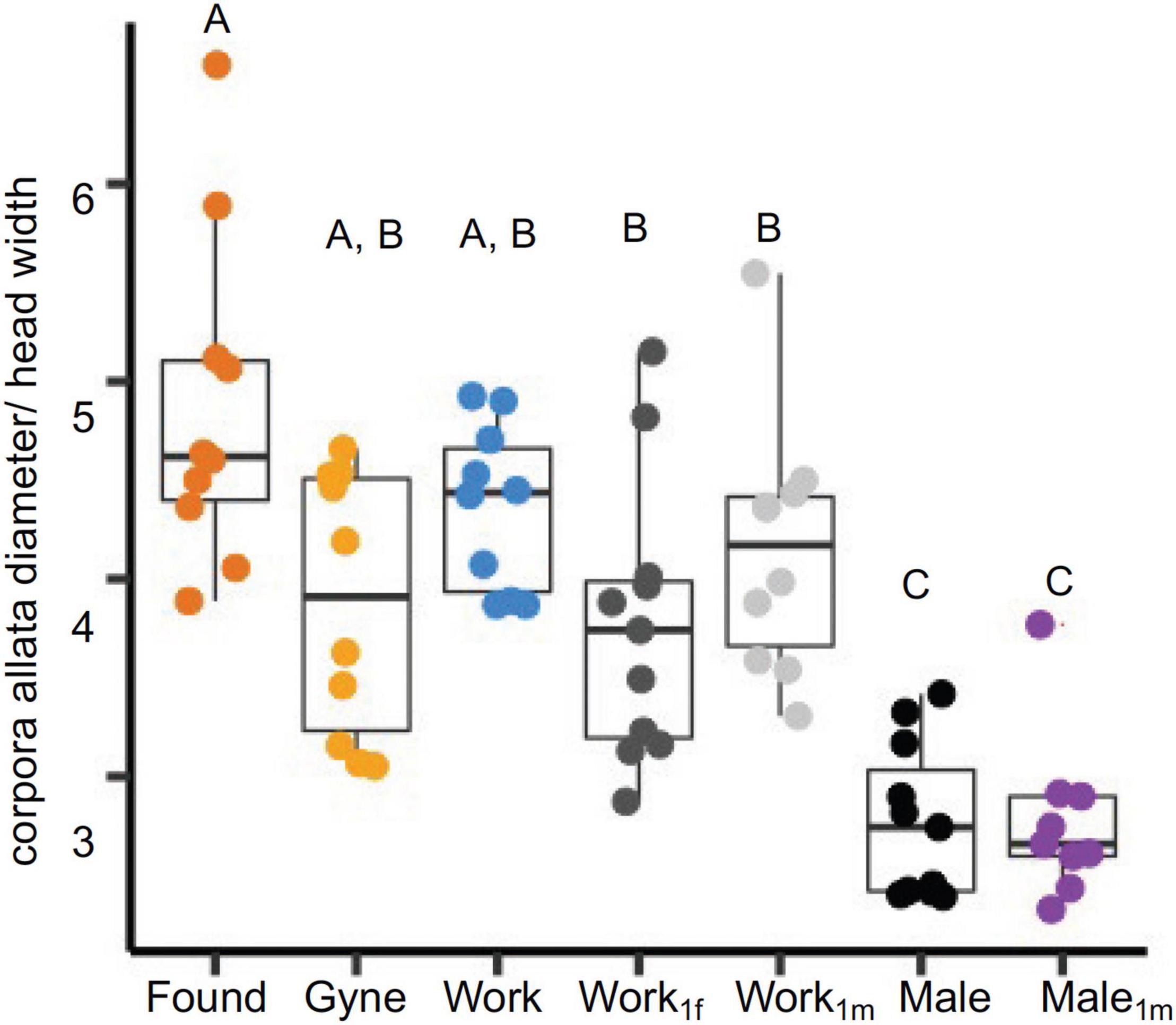
Figure 5. Corpora allata size in reproductive females, workers, males, parasitized workers, and parasitized males. The cross-sectional area of the corpora allata was calculated by measuring its diameter in microns and normalized with head size. Wasp categories are color coded: found (foundresses), gynes, work (workers), work1fm (workers with 1 female parasite), work1m (worker with 1 male parasite), males and male1m (male with 1 male parasite). Each boxplot shows the median, 25th and 75th percentiles. The whiskers show the 5th and 95th percentiles.
Discussion
We provide several lines of evidence supporting the focal hypothesis that brain plasticity facilitates differential sensory needs and life histories within the same species. First, reproductive females also had larger calyces compared to worker females, reflecting sensory needs associated with division of labor. Second, males and females showed a consistent and significant differential investment in volume of the optic and antennal lobes. This pattern implies a life history-based plasticity of otherwise genetically shared backgrounds in Polistes dominula. Furthermore, we provide novel evidence for the effect of the Xenos vesparum parasite in neural investment by female and male hosts. Contrary to our prediction, non-parasitized and parasitized workers show moderate volumetric differences in brain sensory regions, while parasitized males showed a more drastic effect in allocation of neural tissue compared to non-parasitized males. Overall, our results are consistent with differential investment in brain regions being advantageous across social wasp species (O′Donnell et al., 2011).
The observed differential investment in sensory brain regions reflect the distinct life cycles of P. dominula males and females, similarly to previous studies in bees and ants (Van Praagh et al., 1980; Arnold et al., 1985; Menzel et al., 1991; Gronenberg, 2008; Mysore et al., 2009). Together, these findings suggest that in social Hymenoptera, male sexual behavior is a fundamental driver of neural organization (Beani et al., 2014). Male P. dominula leave their nest within a few days after emergence and gather at distinct leks to increase their mating opportunities (Beani, 1996). When attempting to mate, males can visually distinguish between females and competing males, and between workers and gynes (Cappa et al., 2013; de Souza et al., 2017; da Silva et al., 2021). Therefore, larger optic lobes may facilitate detection and discrimination between potential mates or male intruders in their defended territories (Beani et al., 2014). Males have smaller antennal lobes, which is likely due to experiencing less complex olfactory stimuli, as they do not engage in frequent chemically-based social interactions in the colony. In contrast, reproductive females have proportionally larger antennal lobes, lips and collars, which is consistent with other studies that show sensory needs associated with division of labor, interactions among nestmates, learning and memory (Gronenberg et al., 1996; Ehmer and Hoy, 2000; O′Donnell et al., 2011; Mora-Kepfer, 2014; Jernigan et al., 2021; Mertes et al., 2021; Rozanski et al., 2021; Uy et al., 2021). Thus, the social environment of female wasps has a wider range of chemical and sensory processing cues compared to males (Beani et al., 2014).
Within females, reproductives had proportionally larger calyces than workers, which coincides with division of labor in these social wasps (O′Donnell et al., 2007). Foundresses consistently engage in social interactions both within the colony and as gynes during winter aggregations, utilizing visual and chemical cues toward recognition (Dani et al., 2001; Cini et al., 2019). In contrast, most workers spend less time interacting with foundresses and brood on the nest, and allocate more time performing tasks such as foraging for prey and building material (Gamboa et al., 1978). Our results are similar to studies in P. dominula (Ehmer et al., 2001), P. instabilis (Molina and O’Donnell, 2007), and Mischocyttarus mastigophorus (O′Donnell et al., 2007) that correlate large calyces with social dominance. Contrastingly, in ants, honey bees, and Polybia paper wasps, subordinate forager workers have large calyces (Withers et al., 1993; Gronenberg et al., 1996; O′Donnell et al., 2004). However, these social insects form large colonies, and show specialized division of labor and age polyethism. Specifically, workers transition from tasks inside the nest to more sensory-demanding tasks outside the nest such as navigation and learning landmarks, which supports the pattern of large calyces (Gronenberg and Riveros, 2009; O′Donnell et al., 2011; Cabirol et al., 2018). Finally, differences in nutrition may also influence allocation of brain tissue between workers and foragers. In P. metricus, lower nutrition is associated with higher foraging and brain gene expression in workers (Toth et al., 2009; Daugherty et al., 2011). Similarly, in the primitively-eusocial bee Augochlorella aurata, queens have larger mushroom bodies than workers, due to increased nutrition as larvae (Pahlke et al., 2019).
Contrary to our expectations, parasites have small effects on the brain architecture of workers and did not elicit a significant reduction in the corpora allata. Male parasites induced a reduction of the central complex, compared to non-parasitized workers or workers parasitized by one female. This difference in the central complex, which is mainly implicated in spatial navigation, is not consistent with the lack of differences in behavior between workers infected by the two sexes. Noticeably, workers parasitized by one X. vesparum female showed larger calyces than non-parasitized workers and those parasitized by one male. Interestingly, workers parasitized by one female enter diapause and resemble the behavioral and physiological phenotype of overwintering gynes, while workers parasitized by a male die at the end of summer like non-parasitized ones (Beani et al., 2021). Thus, it is likely that the female parasite should minimize cognitive impairment of its worker host.
In contrast, male parasites had a more drastic effect in the brain architecture of P. dominula males, which are parasitized less frequently than females. Parasitized males had significantly smaller whole brains and central brains than non-parasitized males. They also showed a significant increase in the volume of several sensory brain regions, including the antennal and optic lobes, and two substructures of the calyx: lip and collar. Remarkably, neuroendocrine manipulation does not seem to occur in parasitized males, as they develop their corpora allata, testes, seminal vesicles and accessory glands and attempt to mate (Cappa et al., 2014; Beani et al., 2017). The inability to castrate the male may likely result in brain manipulation instead. Given that the brain is an expensive tissue to produce (Niven and Laughlin, 2008; Keesey et al., 2020), the parasite may reallocate energy and resources to develop inside the host, resulting in the observed small brains.
Notably, only a few studies have tested for neuroanatomical changes induced by parasites that do not directly infect in the brain, but instead lodge inside insect body cavities. The parasitic fungus Ophiocordyceps manipulates the behavior of their ant host, but does not induce structural changes in the brain (Hughes et al., 2011). Instead, the fungal hyphae surround muscle fibers (Hughes et al., 2011; Fredericksen et al., 2017). The hairworm Paragordius tricuspidatus induces neurogenesis in the mushroom bodies of its cricket host, before manipulating it to jump into the water to continue the parasite’s lifecycle (Thomas et al., 2003). Infected crickets also showed differential expression of proteins in the head (Biron et al., 2006). Together with our findings, these results suggest that different parasites likely hijack distinct neural mechanisms to control the behavior of their insect hosts (Hughes and Libersat, 2018).
Overall, our results demonstrate that brain plasticity is associated with sensory needs in males and within female castes of P. dominula, but that parasitic manipulation can also drive differential investment of brain regions depending on both host and parasite sex. Intriguingly, workers infected with a female parasite show a strong manipulation effect of the parasite on caste determination, lipid storage, and prolonged lifespan in parasitized females that act as their main host (Beani et al., 2021), but more dampened effects on allocation of brain tissue. In turn, the reproductive apparatus and behavior of parasitized males are essentially unaffected, but they experience stronger volumetric changes in brain regions. Previous work has shown that X. vesparum drives gene expression changes of workers toward a gyne-like pattern; thus, the parasite is manipulating the transcriptomic plasticity of the caste system (Geffre et al., 2017). Parasitized females also show low levels of haemolymphatic protein and juvenile hormone compared to non-parasitized females, but with no difference between non- parasitized and parasitized males (Strambi and Strambi, 1973; Strambi et al., 1982). Our study shows that strepsipteran do not drive evident neuroanatomical changes in their females hosts, suggesting that this parasite may be relying on other manipulation mechanisms (Libersat et al., 2018).
Data Availability Statement
The raw data supporting the conclusions of this article will be made available by the authors, without undue reservation.
Author Contributions
LB and FC collected the wasp and parasite field samples. KG performed histological preparation of specimens and collected volumetric data. FU analyzed the data. KG, LB, FC, and FU contributed to the first draft of the manuscript. All authors provided input to concept and design of this project, data interpretation, along with reviewing and editing the final manuscript.
Funding
KG, MH, and FU were supported by a National Academies Keck Future Initiatives Grant (NAKFI). FC, DB, and LB were supported by University of Florence funds. MH was an associate at the Center for Advanced Study at the University of Illinois and a visiting fellow at the Wissenschaftskolleg zu Berlin. Samples were imported from Italy into the United States via USDA permit #128388.
Conflict of Interest
The authors declare that the research was conducted in the absence of any commercial or financial relationships that could be construed as a potential conflict of interest.
Publisher’s Note
All claims expressed in this article are solely those of the authors and do not necessarily represent those of their affiliated organizations, or those of the publisher, the editors and the reviewers. Any product that may be evaluated in this article, or claim that may be made by its manufacturer, is not guaranteed or endorsed by the publisher.
Acknowledgments
We thank Jamie Brunworth for assistance in quantifying brain regions. William Searcy, Al Uy and members of the Uy lab provided useful feedback in early versions of the manuscript.
Supplementary Material
The Supplementary Material for this article can be found online at: https://www.frontiersin.org/articles/10.3389/fevo.2022.803437/full#supplementary-material
References
Akalal, D.-B. G., Wilson, C. F., Zong, L., Tanaka, N. K., Ito, K., and Davis, R. L. (2006). Roles for Drosophila mushroom body neurons in olfactory learning and memory. Learn. Mem. 13, 659–668. doi: 10.1101/lm.221206
Anderson, M. L., and Finlay, B. L. (2014). Allocating structure to function: the strong links between neuroplasticity and natural selection. Front. Hum. Neurosci. 7:918. doi: 10.3389/fnhum.2013.00918
Anton, S., and Homberg, U. (1999). Antennal Lobe Structure, Insect Olfaction. Berlin: Springer, 97–124.
Arganda, S., Hoadley, A. P., Razdan, E. S. I, Muratore, B., and Traniello, J. F. A. (2020). The neuroplasticity of division of labor: worker polymorphism, compound eye structure and brain organization in the leafcutter ant Atta cephalotes. J. Comp. Physiol. A 206, 651–662. doi: 10.1007/s00359-020-01423-9
Arnold, G., Masson, C., and Budharugsa, S. (1985). Comparative study of the antennal lobes and their afferent pathway in the worker bee and the drone (Apis mellifera). Cell Tissue Res. 242, 593–605.
Axelrod, C. J., Laberge, F., and Robinson, B. W. (2018). Intraspecific brain size variation between coexisting sunfish ecotypes. Proc. R. Soc. B 285:20181971. doi: 10.1098/rspb.2018.1971
Ball, G. F., Riters, L. V., and Balthazart, J. (2002). Neuroendocrinology of song behavior and avian brain plasticity: multiple sites of action of sex steroid hormones. Front. Neuroendocrinol. 23:137–178. doi: 10.1006/frne.2002.0230
Barton, R., Purvis, A., and Harvey, P. (1995). Evolutionary radiation of visual and olfactory brain systems in primates, bats and insectivores. Philos. Trans. R. Soc. Lond. Ser. B Biol. Sci. 348, 381–392. doi: 10.1098/rstb.1995.0076
Barton, R. A., and Harvey, P. H. (2000). Mosaic evolution of brain structure in mammals. Nature 405, 1055–1058. doi: 10.1038/35016580
Baudier, K. M., Bennett, M. M., Barrett, M., Cossio, F. J., Wu, R. D., O’Donnell, S., et al. (2021). Soldier neural architecture is temporarily modality-specialized but poorly predicted by repertoire size in the stingless bee Tetragonisca angustula. J. Comp. Neurol. 1–11.
Beani, L. (1996). “Lek-like courtship in paper-wasps: ‘a prolonged, delicate, and troublesome affair,” in Natural History and Evolution of Paper-Wasps, eds S. Turillazzi and M. J. West-Eberhard (Oxford: Oxford Univ. Press), 113–125.
Beani, L. (2006). Crazy wasps: when parasites manipulate the Polistes phenotype. Ann. Zool. Fennici 43, 564–574.
Beani, L., Bagneres, A.-G., Elia, M., Petrocelli, I., Cappa, F., and Lorenzi, M. (2019). Cuticular hydrocarbons as cues of sex and health condition in Polistes dominula wasps. Insectes Soc. 66, 543–553. doi: 10.1007/s00040-019-00721-z
Beani, L., Cappa, F., Manfredini, F., and Zaccaroni, M. (2018). Preference of Polistes dominula wasps for trumpet creepers when infected by Xenos vesparum: a novel example of co-evolved traits between host and parasite. PLoS One 13:e0205201. doi: 10.1371/journal.pone.0205201
Beani, L., Dallai, R., Cappa, F., Manfredini, F., Zaccaroni, M., Lorenzi, M. C., et al. (2021). A Strepsipteran parasite extends the lifespan of workers in a social wasp. Sci. Rep. 11:7235. doi: 10.1038/s41598-021-86182-6
Beani, L., Dallai, R., Mercati, D., Cappa, F., Giusti, F., and Manfredini, F. (2011). When a parasite breaks all the rules of a colony: morphology and fate of wasps infected by a Strepsipteran endoparasite. Anim. Behav. 82, 1305–1312. doi: 10.1016/j.anbehav.2011.09.012
Beani, L., Dessì-Fulgheri, F., Cappa, F., and Toth, A. (2014). The trap of sex in social insects: from the female to the male perspective. Neurosci. Biobehav. Rev. 46, 519–533. doi: 10.1016/j.neubiorev.2014.09.014
Beani, L., Marchini, D., Cappa, F., Petrocelli, I., Gottardo, M., Manfredini, F., et al. (2017). Subtle effect of Xenos vesparum (Xenidae, Strepsiptera) on the reproductive apparatus of its male host: parasite or parasitoid? J. Insect Physiol. 101, 22–30.
Biron, D., Ponton, F., Marché, L., Galeotti, N., Renault, L., Demey-Thomas, E., et al. (2006). Suicide’of crickets harbouring hairworms: a proteomics investigation. Insect Mol. Biol. 15, 731–742. doi: 10.1111/j.1365-2583.2006.00671.x
Burns, J. G., Saravanan, A., and Helen Rodd, F. (2009). Rearing environment affects the brain size of guppies: lab-reared guppies have smaller brains than wild-caught guppies. Ethology 115, 122–133. doi: 10.1111/j.1439-0310.2008.01585.x
Cabirol, A., Cope, A. J., Barron, A. B., and Devaud, J.-M. (2018). Relationship between brain plasticity, learning and foraging performance in honey bees. PLoS One 13:e0196749. doi: 10.1371/journal.pone.0196749
Cappa, F., Beani, L., and Cervo, R. (2016). The importance of being yellow: visual over chemical cues in gender recognition in a social wasp. Behav. Ecol. 27, 1182–1189. doi: 10.1093/beheco/arw025
Cappa, F., Bruschini, C., Cervo, R., Turillazzi, S., and Beani, L. (2013). Males do not like the working class: male sexual preference and recognition of functional castes in a primitively eusocial wasp. Anim. Behav. 86, 801–810. doi: 10.1016/j.anbehav.2013.07.020
Cappa, F., Cini, A., Signorotti, L., and Cervo, R. (2020). Rethinking recognition: social context in adult life rather than early experience shapes recognition in a social wasp. Philos. Trans. R. Soc. B 375:20190468. doi: 10.1098/rstb.2019.0468
Cappa, F., Manfredini, F., Dallai, R., Gottardo, M., and Beani, L. (2014). Parasitic castration by Xenos vesparum depends on host gender. Parasitology 141, 1080–1087. doi: 10.1017/S003118201400047X
Catania, K. C. (2005). Evolution of sensory specializations in insectivores. Anatom. Rec. Part A 287, 1038–1050. doi: 10.1002/ar.a.20265
Cini, A., Cappa, F., Pepiciello, I., Platania, L., Dapporto, L., and Cervo, R. (2019). Sight in a clique, scent in society: plasticity in the use of nestmate recognition cues along colony development in the social wasp Polistes dominula. Front. Ecol. Evol. 7:444.
da Silva, R. C., Van Meerbeeck, L., do Nascimento, F. S., Wenseleers, T., and Oi, C. A. (2021). Close-range cues used by males of Polistes dominula in sex discrimination. Sci. Nat. 108:15. doi: 10.1007/s00114-021-01730-4
Dani, F. R., Jones, G. R., Destri, S., Spencer, S. H., and Turillazzi, S. (2001). Deciphering the recognition signature within the cuticular chemical profile of paper wasps. Anim. Behav. 62, 165–171. doi: 10.1006/anbe.2001.1714
Daugherty, T., Toth, A., and Robinson, G. (2011). Nutrition and division of labor: effects on foraging and brain gene expression in the paper wasp Polistes metricus. Mol. Ecol. 20, 5337–5347. doi: 10.1111/j.1365-294X.2011.05344.x
de Souza, A. R., Lino-Neto, J., Tibbetts, E. A., Turillazzi, S., and Beani, L. (2017). The leks of Polistes dominula paper wasps: tiny abdominal spots play a critical role in male attacks toward potential rivals. Ethol. Ecol. Evol. 29, 410–419. doi: 10.1080/03949370.2016.1197970
DeCasien, A. R., Williams, S. A., and Higham, J. P. (2017). Primate brain size is predicted by diet but not sociality. Nat. Ecol. Evol. 1, 1–7. doi: 10.1038/s41559-017-0112
Dubois, E. (1897). Sur le rapport du poids de l’encéphale avec la grandeur du corps chez les mammifères. Bull. Mém. Soc. Anthropol. Paris 8, 337–376. doi: 10.3406/bmsap.1897.5705
Dunbar, R., and Shultz, S. (2017). Why are there so many explanations for primate brain evolution? Philos. Trans. R. Soc. B Biol. Sci. 372:20160244. doi: 10.1098/rstb.2016.0244
Eberhard, W. G., and Wcislo, W. T. (2011). Grade changes in brain–body allometry: morphological and behavioural correlates of brain size in miniature spiders, insects and other invertebrates. Adv. Insect Physiol. 40, 155–214. doi: 10.1016/b978-0-12-387668-3.00004-0
Ehmer, B., and Hoy, R. (2000). Mushroom bodies of vespid wasps. J. Comp. Neurol. 416, 93–100. doi: 10.1002/(sici)1096-9861(20000103)416:1<93::aid-cne7>3.0.co;2-f
Ehmer, B., Reeve, H. K., and Hoy, R. R. (2001). Comparison of brain volumes between single and multiple foundresses in the paper wasp Polistes dominulus. Brain Behav. Evol. 57, 161–168. doi: 10.1159/000047234
Fahrbach, S. E. (2006). Structure of the mushroom bodies of the insect brain. Annu. Rev. Entomol. 51, 209–232.
Fiala, J. C. (2005). Reconstruct: a free editor for serial section microscopy. J. Microsc. 218, 52–61. doi: 10.1111/j.1365-2818.2005.01466.x
Fredericksen, M. A., Zhang, Y., Hazen, M. L., Loreto, R. G., Mangold, C. A., Chen, D. Z., et al. (2017). Three-dimensional visualization and a deep-learning model reveal complex fungal parasite networks in behaviorally manipulated ants. Proc. Natl. Acad. Sci. U.S.A. 114, 12590–12595. doi: 10.1073/pnas.1711673114
Gamboa, G. J., Heacock, B. D., and Wiltjer, S. L. (1978). Division of labor and subordinate longevity in foundress associations of the paper wasp, Polistes metricus (Hymenoptera: Vespidae). J. Kans. Entomol. Soc. 343–352.
Geffre, A. C., Liu, R., Manfredini, F., Beani, L., Kathirithamby, J., Grozinger, C. M., et al. (2017). Transcriptomics of an extended phenotype: parasite manipulation of wasp social behaviour shifts expression of caste-related genes. Proc. R. Soc. B Biol. Sci. 284:20170029. doi: 10.1098/rspb.2017.0029
Giraldo, Y. M., Patel, E., Gronenberg, W., and Traniello, J. F. (2013). Division of labor and structural plasticity in an extrinsic serotonergic mushroom body neuron in the ant Pheidole dentata. Neurosci. Lett. 534, 107–111. doi: 10.1016/j.neulet.2012.11.057
Godfrey, R. K., and Gronenberg, W. (2019). Brain evolution in social insects: advocating for the comparative approach. J. Comp. Physiol. A 205, 13–32. doi: 10.1007/s00359-019-01315-7
Gordon, D. G., Zelaya, A., Arganda-Carreras, I., Arganda, S., and Traniello, J. F. (2019). Division of labor and brain evolution in insect societies: neurobiology of extreme specialization in the turtle ant Cephalotes varians. PLoS One 14:e0213618. doi: 10.1371/journal.pone.0213618
Groh, C., and Meinertzhagen, I. A. (2010). Brain plasticity in Diptera and Hymenoptera. Front. Biosci. 2:268–288. doi: 10.2741/s63
Gronenberg, W. (2008). Structure and function of ant (Hymenoptera: Formicidae) brains: strength in numbers. Myrmecol. News 11, 25–36.
Gronenberg, W., Heeren, S., and Hölldobler, B. (1996). Age-dependent and task-related morphological changes in the brain and the mushroom bodies of the ant Camponotus floridanus. J. Exp. Biol. 199, 2011–2019. doi: 10.1242/jeb.199.9.2011
Gronenberg, W., and Hölldobler, B. (1999). Morphologic representation of visual and antennal information in the ant brain. J. Comp. Neurol. 412, 229–240. doi: 10.1002/(sici)1096-9861(19990920)412:2<229::aid-cne4>3.0.co;2-e
Gronenberg, W., and Riveros, A. J. (2009). “Social brains and behavior: past and present,” in Organization of Insect Societies: From Genome to Sociocomplexity, eds J. Gadau and J. Fewell (Cambridge, MA: Harvard University Press), 377–401.
Honkanen, A., Adden, A., da Silva Freitas, J., and Heinze, S. (2019). The insect central complex and the neural basis of navigational strategies. J. Exp. Biol. 222(Pt Suppl 1):jeb188854. doi: 10.1242/jeb.188854
Hughes, D. P., Andersen, S. B., Hywel-Jones, N. L., Himaman, W., Billen, J., and Boomsma, J. J. (2011). Behavioral mechanisms and morphological symptoms of zombie ants dying from fungal infection. BMC Ecol. 11:13. doi: 10.1186/1472-6785-11-13
Hughes, D. P., Kathirithamby, J., Turillazzi, S., and Beani, L. (2004b). Social wasps desert the colony and aggregate outside if parasitized: parasite manipulation? Behav. Ecol. 15, 1037–1043. doi: 10.1093/beheco/arh111
Hughes, D. P., Kathirithamby, J., and Beani, L. (2004a). Prevalence of the parasite Strepsiptera in adult Polistes wasps: field collections and literature overview. Ethol. Ecol. Evol. 16, 363–375. doi: 10.1080/08927014.2004.9522627
Hughes, D. P., and Libersat, F. (2018). Neuroparasitology of parasite–insect associations. Annu. Rev. Entomol. 63, 471–487. doi: 10.1146/annurev-ento-020117-043234
Huxley, J. S., and Teissier, G. (1936). Terminology of relative growth. Nature 137, 780–781. doi: 10.1038/137780b0
Isler, K., and Van Schaik, C. P. (2006). Metabolic costs of brain size evolution. Biol. Lett. 2, 557–560. doi: 10.1098/rsbl.2006.0538
Jernigan, C. M., Zaba, N. C., and Sheehan, M. J. (2021). Age and social experience induced plasticity across brain regions of the paper wasp Polistes fuscatus. Biol. Lett. 17:20210073. doi: 10.1098/rsbl.2021.0073
Jones, T. A., Donlan, N. A., and O’Donnell, S. (2009). Growth and pruning of mushroom body Kenyon cell dendrites during worker behavioral development in the paper wasp, Polybia aequatorialis (Hymenoptera: Vespidae). Neurobiol. Learn. Mem. 92, 485–495. doi: 10.1016/j.nlm.2009.06.007
Kamhi, J. F., Sandridge-Gresko, A., Walker, C., Robson, S. K., and Traniello, J. F. (2017). Worker brain development and colony organization in ants: does division of labor influence neuroplasticity? Dev. Neurobiol. 77, 1072–1085. doi: 10.1002/dneu.22496
Keesey, I. W., Grabe, V., Knaden, M., and Hansson, B. S. (2020). Divergent sensory investment mirrors potential speciation via niche partitioning across Drosophila. eLife 9:e57008. doi: 10.7554/eLife.57008
Le Moël, F., Stone, T., Lihoreau, M., Wystrach, A., and Webb, B. (2019). The central complex as a potential substrate for vector based navigation. Front. Psychol. 10:690. doi: 10.3389/fpsyg.2019.00690
Lendvai, ÁZ., Bókony, V., Angelier, F., Chastel, O., and Sol, D. (2013). Do smart birds stress less? An interspecific relationship between brain size and corticosterone levels. Proc. R. Soc. B Biol. Sci. 280:20131734. doi: 10.1098/rspb.2013.1734
Libersat, F., Kaiser, M., and Emanuel, S. (2018). Mind control: how parasites manipulate cognitive functions in their insect hosts. Front. Psychol. 9:572. doi: 10.3389/fpsyg.2018.00572
Manfredini, F., Benati, D., and Beani, L. (2010). The strepsipteran endoparasite Xenos vesparum alters the immunocompetence of its host, the paper wasp Polistes dominulus. J. Insect Physiol. 56, 253–259. doi: 10.1016/j.jinsphys.2009.10.009
Menzel, J., Wunderer, H., and Stavenga, D. (1991). Functional morphology of the divided compound eye of the honeybee drone (Apis mellifera). Tissue Cell 23, 525–535. doi: 10.1016/0040-8166(91)90010-q
Mertes, M., Carcaud, J., and Sandoz, J.-C. (2021). Olfactory coding in the antennal lobe of the bumble bee Bombus terrestris. Sci. Rep. 11:10947. doi: 10.1038/s41598-021-90400-6
Molina, Y., and O’Donnell, S. (2007). Mushroom body volume is related to social aggression and ovary development in the paperwasp Polistes instabilis. Brain Behav. Evol. 70, 137–144. doi: 10.1159/000102975
Molina, Y., and O’Donnell, S. (2008). Age, sex, and dominance-related mushroom body plasticity in the paperwasp Mischocyttarus mastigophorus. Dev. Neurobiol. 68, 950–959. doi: 10.1002/dneu.20633
Montgomery, S. H., Mundy, N. I., and Barton, R. A. (2016). Brain evolution and development: adaptation, allometry and constraint. Proc. R. Soc. B Biol. Sci. 283:20160433. doi: 10.1098/rspb.2016.0433
Mora-Kepfer, F. (2014). Context-dependent acceptance of non-nestmates in a primitively eusocial insect. Behav. Ecol. Sociobiol. 68, 363–371. doi: 10.1007/s00265-013-1650-2
Murphy, T., Dias, G. P., and Thuret, S. (2014). Effects of diet on brain plasticity in animal and human studies: mind the gap. Neural Plast. 2014:563160. doi: 10.1155/2014/563160
Mysore, K., Subramanian, K., Sarasij, R., Suresh, A., Shyamala, B. V., VijayRaghavan, K., et al. (2009). Caste and sex specific olfactory glomerular organization and brain architecture in two sympatric ant species Camponotus sericeus and Camponotus compressus (Fabricius, 1798). Arthropod Struct. Dev. 38, 485–497. doi: 10.1016/j.asd.2009.06.001
Niven, J. E., and Laughlin, S. B. (2008). Energy limitation as a selective pressure on the evolution of sensory systems. J. Exp. Biol. 211, 1792–1804. doi: 10.1242/jeb.017574
O’Donnell, S., and Bulova, S. (2017). Development and evolution of brain allometry in wasps (Vespidae): size, ecology and sociality. Curr. Opin. Insect Sci. 22, 54–61. doi: 10.1016/j.cois.2017.05.014
O’Donnell, S., Bulova, S. J., Barrett, M., and Fiocca, K. (2018). Size constraints and sensory adaptations affect mosaic brain evolution in paper wasps (Vespidae: Epiponini). Biol. J. Linn. Soc. 123, 302–310. doi: 10.1093/biolinnean/blx150
O’Donnell, S., Bulova, S. J., DeLeon, S., Khodak, P., Miller, S., and Sulger, E. (2015). Distributed cognition and social brains: reductions in mushroom body investment accompanied the origins of sociality in wasps (Hymenoptera: Vespidae). Proc. R. Soc. B Biol. Sci. 282:20150791. doi: 10.1098/rspb.2015.0791
O′Donnell, S., Clifford, M., and Molina, Y. (2011). Comparative analysis of constraints and caste differences in brain investment among social paper wasps. Proc. Natl. Acad. Sci. U.S.A. 108, 7107–7112. doi: 10.1073/pnas.1017566108
O’Donnell, S., Clifford, M. R., Bulova, S. J., DeLeon, S., Papa, C., and Zahedi, N. (2014). A test of neuroecological predictions using paperwasp caste differences in brain structure (Hymenoptera: Vespidae). Behav. Ecol. Sociobiol. 68, 529–536. doi: 10.1007/s00265-013-1667-6
O’Donnell, S., Clifford, M. R., DeLeon, S., Papa, C., Zahedi, N., and Bulova, S. J. (2013). Brain size and visual environment predict species differences in paper wasp sensory processing brain regions (Hymenoptera: Vespidae, Polistinae). Brain Behav. Evol. 82, 177–184. doi: 10.1159/000354968
O′Donnell, S., Donlan, N., and Jones, T. (2007). Developmental and dominance-associated differences in mushroom body structure in the paper wasp Mischocyttarus mastigophorus. Dev. Neurobiol. 67, 39–46. doi: 10.1002/dneu.20324
O′Donnell, S., Donlan, N. A., and Jones, T. A. (2004). Mushroom body structural change is associated with division of labor in eusocial wasp workers (Polybia aequatorialis, Hymenoptera: Vespidae). Neurosci. Lett. 356, 159–162. doi: 10.1016/j.neulet.2003.11.053
Ott, S. R., and Rogers, S. M. (2010). Gregarious desert locusts have substantially larger brains with altered proportions compared with the solitarious phase. Proc. R. Soc. B Biol. Sci. 277, 3087–3096. doi: 10.1098/rspb.2010.0694
Pahlke, S., Jaumann, S., Seid, M. A., and Smith, A. R. (2019). Brain differences between social castes precede group formation in a primitively eusocial bee. Sci. Nat. 106:49. doi: 10.1007/s00114-019-1644-7
Pardi, L. (1996). “Polistes: analysis of a society,” in Natural History and Evolution of Paper-Wasps, eds S. Turillazzi and M. J. West-Eberhard (Oxford: Oxford University Press), 1–17.
Penick, C. A., Ghaninia, M., Haight, K. L., Opachaloemphan, C., Yan, H., Reinberg, D., et al. (2021). Reversible plasticity in brain size, behaviour and physiology characterizes caste transitions in a socially flexible ant (Harpegnathos saltator). Proc. R. Soc. B Biol. Sci. 288:20210141. doi: 10.1098/rspb.2021.0141
Pfeiffer, K., and Homberg, U. (2014). Organization and functional roles of the central complex in the insect brain. Annu. Rev. Entomol. 59, 165–184. doi: 10.1146/annurev-ento-011613-162031
Pitnick, S., Jones, K. E., and Wilkinson, G. S. (2006). Mating system and brain size in bats. Proc. R. Soc. B Biol. Sci. 273, 719–724. doi: 10.1098/rspb.2005.3367
Pyza, E. M. (2013). Plasticity in invertebrate sensory systems. Front. Physiol. 4:226. doi: 10.3389/fphys.2013.00226
Rehan, S. M., Bulova, S. J., and O’Donnell, S. (2015). Cumulative effects of foraging behavior and social dominance on brain development in a facultatively social bee (Ceratina australensis). Brain Behav. Evol. 85, 117–124. doi: 10.1159/000381414
Riveros, A. J., and Gronenberg, W. (2010). Sensory allometry, foraging task specialization and resource exploitation in honeybees. Behav. Ecol. Sociobiol. 64, 955–966. doi: 10.1007/s00265-010-0911-6
Rozanski, A. N., Cini, A., Lopreto, T. E., Gandia, K. M., Hauber, M. E., Cervo, R., et al. (2021). Differential investment in visual and olfactory brain regions is linked to the sensory needs of a wasp social parasite and its host. J. Comp. Neurol. 1–12. doi: 10.1002/cne.25242
Seid, M. A., Castillo, A., and Wcislo, W. T. (2011). The allometry of brain miniaturization in ants. Brain Behav. Evol. 77, 5–13. doi: 10.1159/000322530
Sheehan, Z. B., Kamhi, J. F., Seid, M. A., and Narendra, A. (2019). Differential investment in brain regions for a diurnal and nocturnal lifestyle in Australian Myrmecia ants. J. Comp. Neurol. 527, 1261–1277. doi: 10.1002/cne.24617
Stöckl, A., Heinze, S., Charalabidis, A., El Jundi, B., Warrant, E., and Kelber, A. (2016). Differential investment in visual and olfactory brain areas reflects behavioural choices in hawk moths. Sci. Rep. 6:26041. doi: 10.1038/srep26041
Strambi, A., and Strambi, C. (1973). Influence du developpement du parasite Xenos Vesparum rossi, (Insecte, Strepsiptere) sur le systeme neuroendocrinien des femelles de Polistes (Hymenoptere, Vespide) au de leur vie imaginale. Arch. Anatomie Microsc. Morphol. Exp. 62, 39–54.
Strambi, C., Strambi, A., and Augier, R. (1982). Protein level in the haemolymph of the wasp Polistes gallicus L. at the beginning of imaginal life and during overwintering. Action of the strepsiterian parasite Xenos vesparum rossi. Experientia 38, 1189–1191.
Strassmann, J. E., Fortunato, A., Cervo, R., Turillazzi, S., Damon, J. M., and Queller, D. C. (2004). The cost of queen loss in the social wasp Polistes dominulus (Hymenoptera : Vespidae). J. Kan. Entomol. Soc. 77, 343–355.
Strausfeld, N. J. (1989). “Beneath the compound eye: neuroanatomical analysis and physiological correlates in the study of insect vision,” in Facets of Vision, eds D. G. Stavenga and R. C. Hardie (Berlin: Springer), 317–359. doi: 10.1007/978-3-642-74082-4_16
Taborsky, B., and Oliveira, R. F. (2012). Social competence: an evolutionary approach. Trends Ecol. Evol. 27, 679–688. doi: 10.1016/j.tree.2012.09.003
Thomas, F., Ulitsky, P., Augier, R., Dusticier, N., Samuel, D., Strambi, C., et al. (2003). Biochemical and histological changes in the brain of the cricket Nemobius sylvestris infected by the manipulative parasite Paragordius tricuspidatus (Nematomorpha). Int. J. Parasitol. 33, 435–443. doi: 10.1016/s0020-7519(03)00014-6
Toth, A., Bilof, K., Henshaw, M., Hunt, J., and Robinson, G. (2009). Lipid stores, ovary development, and brain gene expression in Polistes metricus females. Insectes Soc. 56, 77–84. doi: 10.1007/s00040-008-1041-2
Uy, F. M. K., Jernigan, C. M., Zaba, N. C., Mehrotra, E., Miller, S. E., and Sheehan, M. J. (2021). Dynamic neurogenomic responses to social interactions and dominance outcomes in female paper wasps. PLoS Genet. 17:e1009474. doi: 10.1371/journal.pgen.1009474
Van Praagh, J., Ribi, W., Wehrhahn, C., and Wittmann, D. (1980). Drone bees fixate the queen with the dorsal frontal part of their compound eyes. J. Comp. Physiol. A 136, 263–266. doi: 10.1007/bf00657542
Warton, D. I. I, Wright, J., Falster, D. S., and Westoby, M. (2006). Bivariate line-fitting methods for allometry. Biol. Rev. 81, 259–291. doi: 10.1017/S1464793106007007
Warton, D. I., Duursma, R. A., Falster, D. S., and Taskinen, S. (2012). smatr 3-an R package for estimation and inference about allometric lines. Methods Ecol. Evol. 3, 257–259. doi: 10.1111/j.2041-210x.2011.00153.x
West-Eberhard, M. (1969). The social biology of polistine wasps. Miscellaneous Publ. Museum Zoolol. Univ. Michigan 140, 1–101.
Keywords: brain plasticity, parasite, parasitic manipulation of host, Polistes dominula, sensory brain regions, social wasp, strepsiptera, Xenos vesparum
Citation: Gandia KM, Cappa F, Baracchi D, Hauber ME, Beani L and Uy FMK (2022) Caste, Sex, and Parasitism Influence Brain Plasticity in a Social Wasp. Front. Ecol. Evol. 10:803437. doi: 10.3389/fevo.2022.803437
Received: 28 October 2021; Accepted: 03 January 2022;
Published: 28 January 2022.
Edited by:
Sara Arganda Carreras, Rey Juan Carlos University, SpainReviewed by:
Fabio Santos Nascimento, University of São Paulo Ribeirão Preto, BrazilAdam R. Smith, George Washington University, United States
Copyright © 2022 Gandia, Cappa, Baracchi, Hauber, Beani and Uy. This is an open-access article distributed under the terms of the Creative Commons Attribution License (CC BY). The use, distribution or reproduction in other forums is permitted, provided the original author(s) and the copyright owner(s) are credited and that the original publication in this journal is cited, in accordance with accepted academic practice. No use, distribution or reproduction is permitted which does not comply with these terms.
*Correspondence: Floria M. K. Uy, floria.uy@rochester.edu; Laura Beani, laura.beani@unifi.it
†These authors share senior authorship