- 1Chubu University Academy of Emerging Sciences, Kasugai, Japan
- 2Department of Human Cultures, Musashi University, Nerima, Japan
- 3Graduate School of Veterinary Medicine and Life Science, Nippon Veterinary and Life Science University, Musashino, Japan
- 4Graduate School of Asian and African Area Studies, Kyoto University, Kyoto, Japan
- 5Wildlife Research Center of Kyoto University, Kyoto, Japan
- 6Japan Monkey Centre, Inuyama, Japan
- 7Institute for Tropical Biology and Conservation, Universiti Malaysia Sabah, Kota Kinabalu, Malaysia
- 8Department of Biology, Faculty of Science, Chulalongkorn University, Bangkok, Thailand
- 9National Primate Research Center of Thailand, Chulalongkorn University, Saraburi, Thailand
Male coalition-like formation, recently found in stump-tailed macaques (Macaca arctoides), occurs when several top-ranking males collaboratively guard females to prevent mating with other rival males and actively share secured mating opportunities with their allies. We lack a comprehensive understanding of the proximate mechanisms underlying such male coalitions, e.g., the effect of genetic relatedness. Such cooperative partner choice among males is particularly interesting in animals, such as M. arctoides, that live in matrilineal (female philopatry/male dispersal) society. Theoretically, in such a social system, females, and not males, are often related to each other by kinship. Thus, the occurrence of cooperative behavior between related females are generally common, but it would be rare between unrelated/non-kin males in such matrilineal society. Herein, for the first time, we report detailed copulatory behaviors, including mating and reproductive success, in relation to male coalition-like formation in free-ranging M. arctoides following direct behavioral observation and genetic analysis. We found that coalition-forming male–male alliances often occur among both individuals that are highly related and those that are less related. We identified two groups with different mating strategies, i.e., single-male monopoly and coalition-male monopoly groups. In both groups, nearly 80% of copulations were monopolized by a single male or by coalition males. However, the single-male monopoly strategy allows opportunistic/sneaky copulations by other males with a relatively high probability. Thus, the degree of reproductive success did not reflect mating success. In contrast, the males employing a coalition strategy successfully shared their mating and reproductive success, particularly in the largest group. Compared with single-male monopolized groups, the coalition-male monopoly groups copulated with a considerably more number of females, suggesting that coalition males can effectively guard against opportunistic/sneaky copulation by rival males. We also found that coalition-forming male–male alliances often occur regardless of the degree of kinship/relatedness, indicating the complexity and flexibility inherent in the male social bond of M. arctoides.
Introduction
A fundamental problem in behavioral ecology is determining mechanisms that regulate differential reproduction among individuals, and intrasexual competition is considered one of the important factors closely associated with such mechanisms (Darwin, 1871). In theory, male–male competitions occur because males seek to maximize their reproductive success by mating with as many females as possible, and thus compete over access to mates in general (Emlen and Oring, 1977; Clutton-Brock, 1989). Male reproductive success has been studied in many animal taxa to understand differential reproduction (or reproductive skew) in males possibly caused by male–male competition (Clutton-Brock, 1988). Among primates that exhibit a stunning variation in both social structure and organization, male reproductive success has been historically difficult to measure, and consequently, male mating success is assumed to be a sufficiently reliable indicator to reflect male reproductive success, wherein success is often linked to dominance status (Robinson, 1982; Fedigan, 1983; Cowlishaw and Dunbar, 1991). With the development of genetic techniques, based on both observational and genetic data, this has been repeatedly tested in various primate species but has not yielded consistent results. Some studies show that mating and reproductive success are both closely related to male dominance status (e.g., Cowlishaw and Dunbar, 1991; de Ruiter and van Hooff, 1993; Wickings et al., 1993), although the skew in male reproductive success is often less pronounced than that predicted from observed mating success (e.g., Berard et al., 1993; Inoue et al., 1993; Constable et al., 2001; Young et al., 2013). Therefore, the variation in the relationship between mating and reproductive success is increasingly drawing attention.
In contrast, considering alternative male reproductive tactics to mitigate competition if males cannot achieve a high rank is equally important (Setchell et al., 2008). Coalition formation, defined as cooperation in an aggressive or competitive context, where one animal intervenes in an ongoing conflict between two parties to support one side (Harcourt and de Waal, 1992), is one of the notable alternative tactics in males to improve their dominance-based status, which improves their mating success (Noë and Sluijter, 1990). Coalition formation in males has been reported in various mammalian taxa, e.g., cetaceans (Diaz-Aguirre et al., 2018; Gerber et al., 2021) and carnivores (Packer et al., 1991; Caro and Collins, 2010), although it has most extensively been studied in primates (van Schaik et al., 2006). Nonetheless, at present, we lack a comprehensive understanding of the proximate mechanisms underlying such cooperative coalition behaviors, i.e., who helps whom (Rodrigues and Kokko, 2016).
In primates, male–male coalitions are generally observed in the context of conflict/aggression and are widely found in nearly all major phyletic lines, with the exception of Malagasy prosimians. The frequency of observed coalitions differs across species and even within families or genera (Bissonnette et al., 2014). Although there is a trend that kinship contributes to driving the propensity for male–male coalitions (Silk, 1992, 2009), it would hardly explain such nepotism in female-philopatric–male-dispersal species common in non-human primates compared with male-philopatric species of some taxa, i.e., colobuses, apes, atelids, and lemurids (Pusey and Packer, 1986; Furuichi et al., 2015). Therefore, male–male coalitions, particularly in female-philopatric species, are interesting in the context of partner choice because males (possibly non-related males) are compelled to cooperate for the limited resource that cannot be shared. Male–male competition for females is universal in biological theory. In addition to the choice of partner in male–male coalitions, evaluating the effects of such coalitions on male reproductive success would provide critical implications for the evolutionary background of male–male coalition. Till date, in non-human primates, empirical studies demonstrating the link between male reproductive success and the participation of males in coalitions have been rare (but see Schulke et al., 2010).
The genus Macaca is the most widely distributed group of non-human primates in Asia (with the exception of the North African Barbary macaque), and their societies are characterized by profound unity and great diversity (Fa and Lindburg, 2005; Thierry, 2007). Of the 12 macaque species, male–male coalitions have been detected in 10 species, although the frequency of male–male coalitions varies across species (Bissonnette et al., 2014). Notably, of the species groups, the Sinica-arctoides lineage, including Macaca assamensis, M. radiata, M. sinica, M. thibetana, and M. arctoides (Delson, 1980; Jiang et al., 2016), is prominent in exhibiting pronounced male affiliations and frequent male–male coalition formation compared with other Macaca species groups (Bissonnette et al., 2014; Ostner and Schülke, 2014).
Similar to other macaques, M. arctoides (stump-tailed macaque) lives in multi-male–multi-female social groups and displays female philopatry and male dispersal (Thierry, 2011). Previous studies focusing on their social interactions have reported their tolerant or relaxed social hierarchy (de Waal and Ren, 1988; de Waal and Luttrell, 1989; Butovskaya, 1993). These social/individual characteristics are mainly based on data from females in captivity living in a semi-free-ranging condition. A notable exception is a study by Richter et al. (2009) that investigated social interactions among males in a free-ranging population and suggested that M. arctoides males are less tolerant than predicted by previous captive studies, which have mostly focused on females. Conversely, although male–male coalition in M. arctoides is assumed (Bissonnette et al., 2014), a detailed description of male–male coalition has not been reported so far except in the authors’ previous study (Toyoda et al., 2020b).
Coalition-like formations, recently found in free-ranging M. arctoides, have been interpreted as a cooperative behavior in which several top-ranking males collaboratively engage in guarding females against mating with other rival males, by possibly using a specific copulation call (Toyoda et al., 2020a), and actively share secured mating opportunities with allies (Toyoda et al., 2020b). In fact, in some male-philopatric primate species, such as muriquis and chimpanzees, males are tolerant to group males and share copulations with receptive females (Nishida, 1983; Watts, 1998; Strier et al., 2002), although little is known about such sharing copulations among males in female-philopatric species, i.e., coalition-like formation with the context found in M. arctoides. The rarity of such coalition in M. arctoides and intraspecific variations in the occurrence of such coalition is intriguing; male–male coalition-like formation has been observed in some groups but not in other groups living in the same habitat (Toyoda et al., 2020b). Therefore, M. arctoides males are promising models that could be particularly useful for evaluating how male–male coalitions contribute to reproductive success by comparing male reproductive success in groups that form male–male coalitions with those that do not.
Copulatory behaviors are one of the commonly studied themes in M. arctoides, e.g., male-specific copulatory calls at the time of ejaculation (Toyoda et al., 2020a) and harassment in relation to copulation (Gouzoules, 1974; Niemeyer and Chamove, 1983) have been described. A rarely observed copulatory pattern known as serial copulation (Estep et al., 1984; Fooden, 1990; Brereton, 1994) could be particularly important for understanding coalition-like formation in M. arctoides because it is always observed in this context (Toyoda et al., 2020b). During serial copulation, males copulate several times in a short interval, with repeated copulations involving ejaculation, although this species is a single-mount ejaculator (i.e., thrusting continues through a single uninterrupted mount until ejaculation is achieved). Here, in an attempt to understand the mating system in relation to the underlying mechanism of male–male coalitions, we first reported the copulatory behaviors, including specific copulation types, of M. arctoides. Second, through behavioral observations, we quantified the mating success of each male in multiple groups by comparing male–male coalition with non-coalition formations. Third, we quantified reproductive success based on genetic paternity tests and compared its extent between male–male coalitions and non-coalition formations. Lastly, we examined the relatedness among males, including the pairs that formed coalitions, and discussed the attributes that influence cooperative partner choice in male M. arctoides.
Materials and Methods
Study Site and Animals
This study was conducted in a mixed landscape and forest fragments at the Khao Krapuk Khao Taomor Non-Hunting Area, Phetchaburi Province, Thailand (12°47′59″ N, 99°44′31″ E) from September 2015 to June 2017. The study area, covering ca. 4 km2 of the ranging area of all groups, was mountainous and included secondary and bamboo forests, roads and human premises, such as temples, pineapple orchards and houses of local residents. Data on rainfall and temperatures were obtained from a nearby weather station of the Kaeng Krachan National Park (approximately 12 km from the nearest periphery and approximately 62 km from the center of the national park area from the study site), and the mean annual temperature and rainfall were approximately 27°C and 1,070 mm, respectively (Wijitkosum, 2012).
Five groups of M. arctoides were habituated to observers; all adults, most of the sub-adults and some juveniles were identified individually by their facial characteristics, scars or other remarkable physical traits (Toyoda et al., 2020a,b). The five study groups of M. arctoides included 391 individuals, i.e., Ting-Group: 120 individuals, including 30 and 33 adult males and females, respectively; Nadam-Group: 75 individuals, including 20 and 30 adult males and females, respectively; Third-Group: 79 individuals, including 23 and 23 adult males and females, respectively; Fourth-Group: 74 individuals, including 16 and 27 adult males and females, respectively; Wngklm-Group: 43 individuals, including 8 and 11 adult males and females, respectively [see 39 in details].
Although the study macaques were free ranging, their ranging area was restricted between the north and south by large roads. Food provisioning by locals or visitors was occasionally observed along the road or around the temple ground. This population was geographically isolated from the other populations, i.e., Kaeng Krachan National Park, and no new immigrant males from other sites were observed during the study period (Toyoda et al., 2017; Toyoda and Malaivijitnond, 2018).
Behavioral Observation
Behavioral data on the five study groups were collected continuously by AT during the daily observation period from 09:00 to 17:00. The group that was first encountered each day was selected as the target group and followed for as long as possible. When the target group could not be followed further, e.g., when the group traveled along cliffs, we terminated the observation session and selected another group, which was subsequently chosen as the target group. We followed each target group alternately for 289 days, and the total observation times for the five aforementioned groups were 970.7 h throughout the study period. All copulations observed in the target group were recorded. Observation conditions were excellent, and the copulations of most group members were generally recorded when copulation occurred. Video cameras (JVC GZ-RX500 and Sony HDR-PJ675) were used to record the behavior in detail.
In most cases, copulations began with a genital inspection of the female by the male (Figure 1), and male-specific copulation calls were often observed during the copulations (Toyoda et al., 2020a). The copulations observed in the study groups were divided into two categories: single and serial copulation types (Figure 1). The single copulation type was defined as a behavior in which a male mounted a female and thrust with intromission (Estep et al., 1984). The serial copulation type was defined as a sequence of single copulations that occurred more than four times with an interval of <30 min between each single copulation; the rationale for this definition is that 95% of the inter-copulation intervals were ≤30 min (Toyoda et al., 2020b) and the mean number of sequential serial copulations in three different previous studies was four (Brereton, 1994). In addition, the serial copulation type was subdivided into two sub-categories: male coalition mode and non-coalition mode. If multiple males were involved in the context of serial copulation with shared mating opportunities, previously known as coalition-like formation (Toyoda et al., 2020b), we defined this behavior as male coalition mode. Alternatively, the behavior was defined as non-coalition mode if only one male engaged in serial copulation (Figure 2).
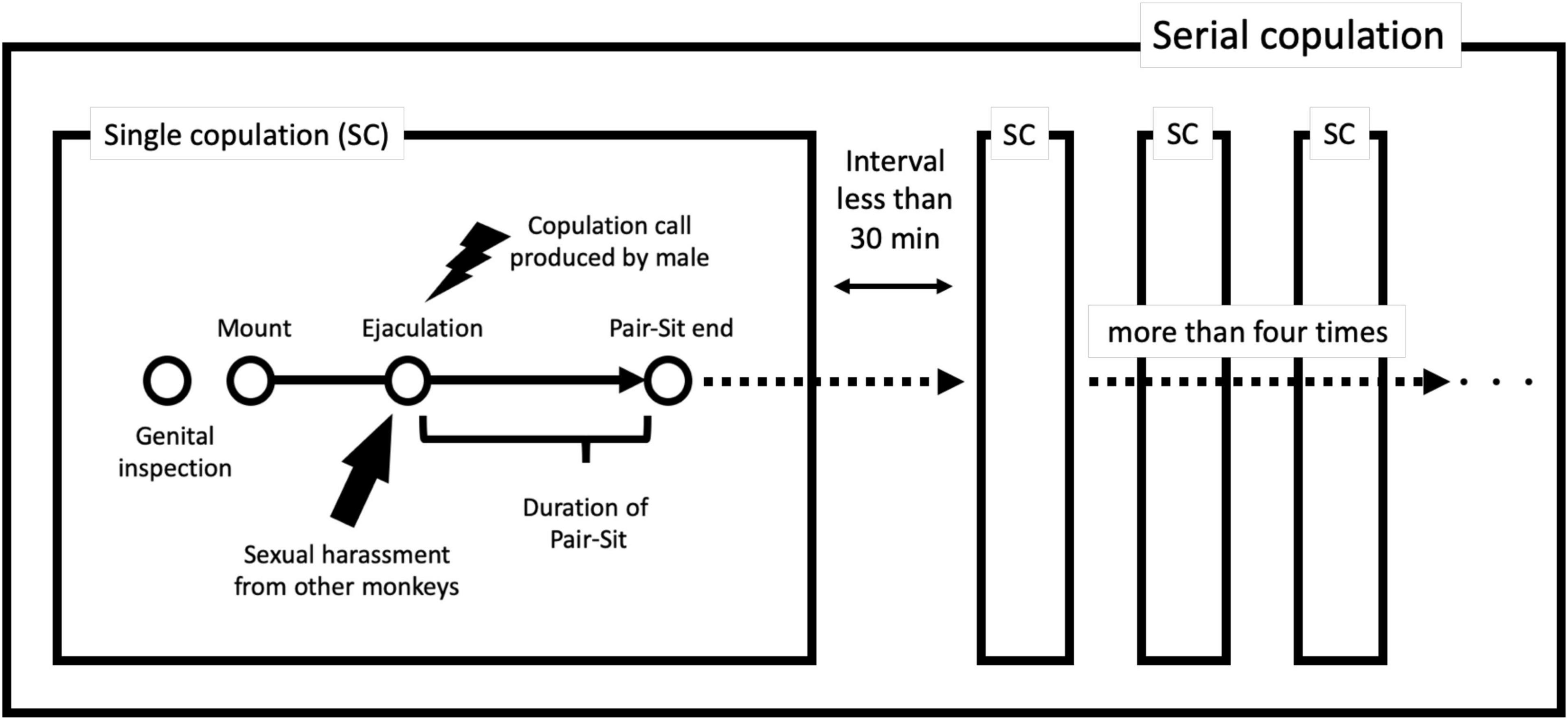
Figure 1. Schematic diagram of the copulating sequence of M. arctoides. In most cases, mating begins with a genital inspection of the female by the male. Because M. arctoides is a single-mount ejaculator, copulation generally leads to ejaculation through thrusting in a single mount. When more than four instances of single copulations occur in succession within a 30-min interval, this continued copulation is classified as serial copulation.
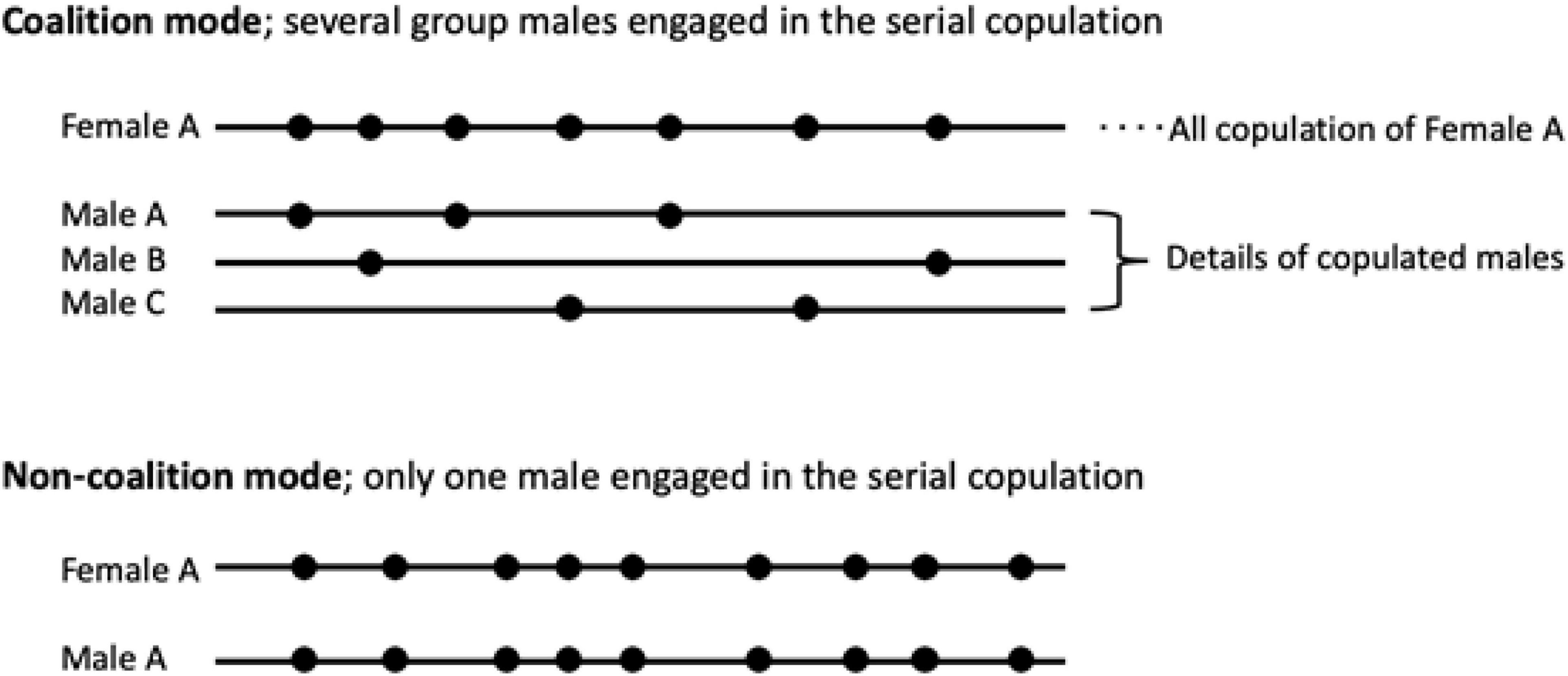
Figure 2. Conceptual diagram of the male coalition mode and non-coalition mode. In the context of serial copulation targeting one female, it is defined as coalition mode when multiple males are engaged and non-coalition mode when only one male is engaged.
DNA Sampling and Genotyping
As a DNA resource, buccal cells were non-invasively collected during the research period using a rope swab (Toyoda et al., 2021). A sterilized polyester rope was cut into 10-cm pieces, which were then soaked in a 20% sugar solution to bait individuals. Once used, rope swabs were immediately collected and quickly transferred to 3 mL lysis buffer containing 0.5% (w/v) sodium dodecyl sulfate, 100 mM ethylenediaminetetraacetic acid (pH 8.0), 100 mM Tris-HCl (pH 8.0) and 10 mM NaCl (Hayaishi and Kawamoto, 2006). Epithelial cells detached from the intestinal wall, collected from freshly dropped fecal samples, were also used as a DNA resource from group members when buccal cells were unavailable. A sterile cotton bud, which was soaked in 2 mL lysis buffer, was used to swab the surface of the feces at least three times (Hayaishi and Kawamoto, 2006).
From the collected specimens, DNA was extracted following the procedure described in Kawamoto et al. (2013), and potential polymerase chain reaction (PCR) inhibitors were removed by adding 600 mg of hydrolyzed starch (Wako, Osaka, Japan) to 1.5 mL of lysis buffer per sample. Samples were then incubated at 36°C for 10 min and centrifuged at 1000 × g for 15 min. Finally, 750 μL of each supernatant was processed using a commercially available DNA clean-up system (Wizard SV Gel and PCR Clean-Up System; Promega, Madison, WI, United States). The DNA was eluted into 50 μL pure water.
Ten polymorphic loci (D8S1106, D19S582, D6S2793, D7S2004, D14S306, D18S537, D17S1290, D13S765, D11S2002, and D3S1768; refer to the details in the Supplementary Material), which exhibited sufficient variation and successful amplification, were amplified and used for genetic analysis (Toyoda and Malaivijitnond, 2018). These Loci were formulated in three combinations when we did multiplex PCR, avoiding overlap of fluorescent dyes and allele sizes; D8S1106, D19S582, and D6S2793 were in combination-A, D7S2004, D14S306, D18S537, and D17S1290 were in combination-B, and D13S765, D11S2002, and D3S1768 were in combination-C. The fragment analysis from sample collection to the PCR process conducted in Thailand was conducted using Macrogen, Inc. (Seoul, South Korea). The resulting fragment data were subsequently analyzed using GeneMapper software v. 4.1 (Applied Biosystems).
In total, 230 samples, including 224 buccal samples (95 adult males, 68 adult females and 50 infants) and 6 fecal samples (only infants) were successfully amplified and analyzed with observed heterozygosity, ranging from 0.431 to 0.794, as calculated by GenAlEx 6.3 (Peakall and Smouse, 2012). Each marker contained between 3 and 12 alleles (Toyoda and Malaivijitnond, 2018). To ensure genotype consistency, PCR was performed at least twice based on the allelic dropout rates calculated by PEDANT 1.0 (Johnson and Haydon, 2007). Noted, however, if the results of the first and second tests were the same, we used those results, though if they differed, we conducted a third test and used the results of the one that confirmed reproducibility. The deviation from Hardy–Weinberg equilibrium was assessed using a χ2-test with Bonferroni correction, and we confirmed that the tested loci did not deviate from the Hardy–Weinberg equilibrium (Supplementary Table 1).
Genetic Analysis
Parentage analysis was conducted using CERVUS 2.0 (Tristan Marshall, Edinburgh, United Kingdom) to evaluate the effects of copulation occupancy rate or coalition mode on reproductive success in males. The paternity of infants was tested using the males in each group and all males in the five study groups, and the results were compared based on allele mismatches and logarithm of the odds scores. Paternity was determined using maximum likelihood testing at confidence levels of >80% for relaxed confidence and >95% for rigorous confidence. Relatedness was estimated using the Queller and Goodnight estimator implemented in GenAlEx 6.3 to evaluate the structural kinship features in the population among males. The program estimated relatedness values by allele sharing of pairs and allele frequency among the population of this study site. Together with calculations conducted using GenAlEx, we also calculated maximum likelihood estimates of relatedness between each dyad of individuals (Wagner et al., 2006) using ML-RELATE (Kalinowski et al., 2006). Kin relationships between each dyad were also estimated using ML-RELATE by assignment to one of four kinship categories with the highest likelihood: parent–offspring (PO), full siblings (FS), half siblings (HS) and unrelated (U).
Statistical Analysis
We performed Fisher’s exact tests to compare ratios of the numbers of infants sired from each male group (male reproductive success) with the ratio derived from the number of counted copulations in each group male (male mating success). We analyzed the data for differences in the pair-wise relatedness of males (calculated by GenAlEx) among the study groups using a Kruskal–Wallis test. We also performed the Steel–Dwass test for multiple comparisons (Hsu, 1996) to compare the pair-wise relatedness of males among the study groups. Relatedness among males within groups was also compared using the asymptotic 2-sample permutation test. Apart from the analyses using the relatedness by GenAlEx, we statistically evaluated the categorical kinship, especially between the males observed coalition behaviors, by ML-RELATE; noted that the test was performed by repeating the test 1,000,000 times to obtain the p-value. First, we statistically examined whether a pair of the males judged as PO, FS, or HS, could be dismissed as unrelated (U). Second, we also examined whether a pair of the males judged as U, could be dismissed as HS. We used R 3.1.0 for statistical analyses (R-Core-Development-Team, 2021), and the significance level was set at 0.05.
Results
Copulatory Behaviors
Intra- and Inter-Group Copulations
During the 21 months of observation, 433 cases of copulation behaviors, i.e., 408 cases (94.2%) of intra-group copulation and 25 cases (5.7%) of inter-group copulation, were observed (Table 1). Inter-group copulations were rarely observed while encountering two groups, and it was generally observed that males approached females in another group. Of the 408 cases of intra-group copulations, 322 cases terminated in ejaculation (78.9%); notably, only 7 cases (8.1%) of interruption from other males were observed, although the reason for copulation without ejaculation was not apparent in most of these cases. Conversely, in 25 cases of inter-group copulations, only 7 cases (28.0%) were those of ejaculatory copulations, whereas 18 cases (72.0%) were terminated without ejaculation, i.e., the male stopped copulation spontaneously possibly because of the appearance of other group members, but no direct interference from other males was observed. Female partner choice was not clear based on direct observations (but see the discussion); when copulation began, females often attempted to flee, screamed or resisted. The males, however, often forced copulation. Of the 347 mating cases for which it was possible to record whether or not the females resisted, 134 (38.6%) copulations were recorded as forced.
Single and Serial Copulation Types
In total, 26 cases of serial copulation, including 213 single copulation cases observed (49.2% of all observed single copulation cases), were recorded in the 5 study groups, i.e., 9, 4, 5, 6, and 2 cases of serial copulation type, such as 64 (38.1% of all single observed copulation cases), 21 (48.8%), 65 (55.8%), 48 (49.0%), and 15 (38.5%) single copulation cases were observed in the Ting-Group, Nadam-Group, Third-Group, Fourth-Group and Wngklm-Group, respectively. Both copulation types were observed in intra-group copulations, but only single copulations were observed in inter-group copulations.
Coalition and Non-coalition Modes
Coalition mode forming male–male alliances (Figure 3) was only observed in three study groups (Ting-Group, Nadam-Group and Fourth-Group) during the serial copulation time. In the Ting-Group, males with four different combination patterns on coalition membership were observed, i.e., coalition01: TNG-M02, TNG-M03, TNG-M07, and TNG-M09; coalition02: TNG-M09, TNG-M17, and TNG-M18; coalition03: TNG-M07 and TNG-M08; and coalition04: TNG-M04, TNG-M08, and TNG-M30. In contrast, males with two fixed members in the Nadam-Group, i.e., NDM-M01 and NDM-M03, and three fixed members in the Fourth-Group, i.e., FTH-M01, FTH-M02, and FTH-M15, were observed. However, this coalition mode was never observed in the Third-Group and Wngklm-Group, and a single male performed all serial copulations observed in these two groups.
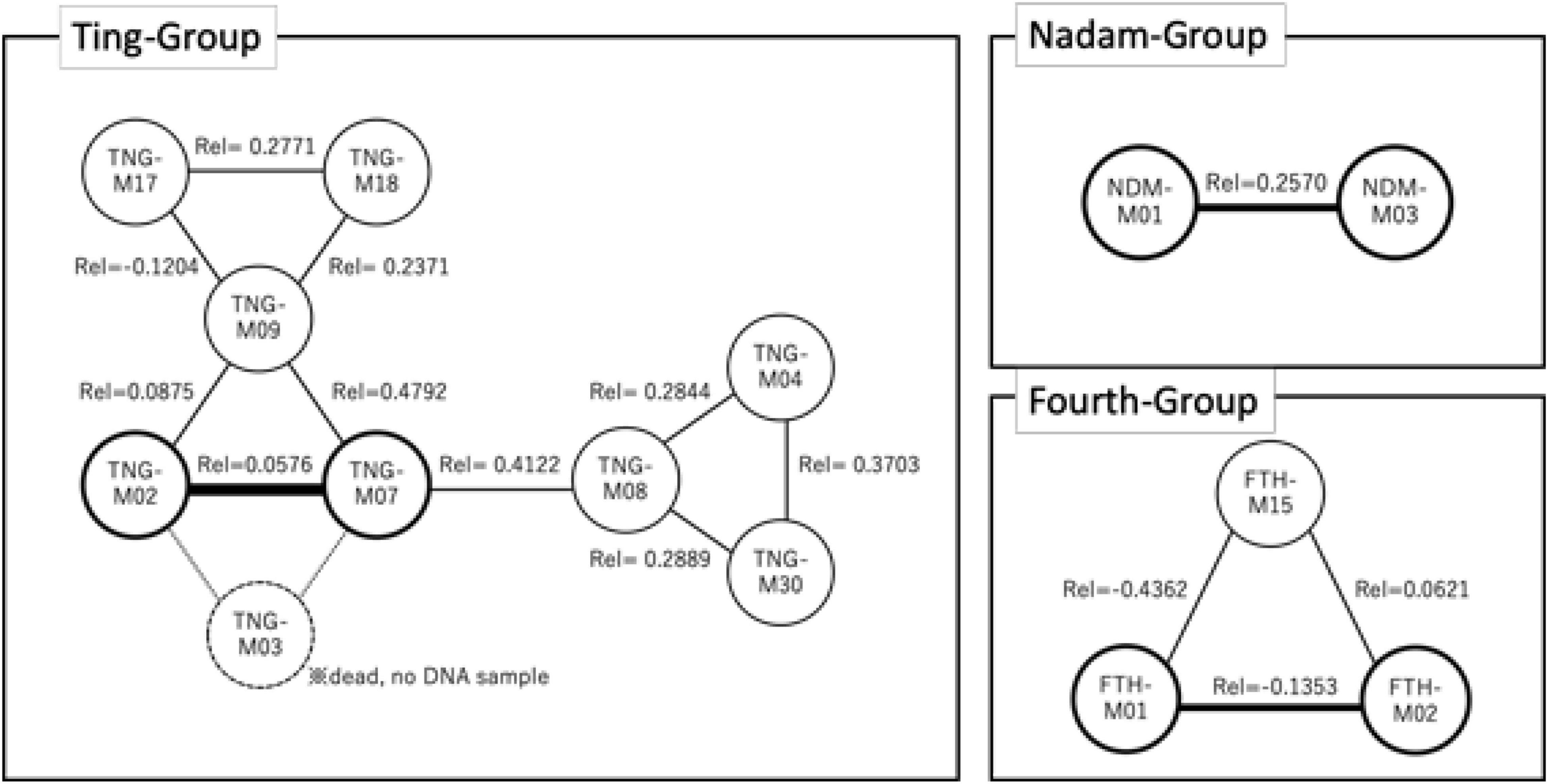
Figure 3. Details of the membership of the coalition mode in each male coalition monopoly group. The thickness of the line segment reflects the number of times copulation was shared. Rel indicates the pair-wise-relatedness value between individuals.
Mating Success
In the Third-Group and Wngklm-Group, a single male mostly occupied the copulation opportunities (Table 1). The male in the Third-Group (TRD-M01) and Wngklm-Group (WKM-M01) successfully occupied 89.5 and 78.6% of the ejaculated copulations within groups, respectively, indicated as a “single-male monopoly group.” In contrast, several males in coalition modes occupied the copulation opportunities in the other three groups, indicated as a “male coalition monopoly group.” Males in coalition mode occupied 89.3, 79.1, and 87.2% of the ejaculated copulations in the Ting-, Nadam-, and Fourth-Groups, respectively.
Copulation distribution among females showed marked variation similar to that in the males in that copulation was not distributed evenly (Figure 4). Generally, the females were seldom to copulate with other males within the groups where mating opportunity was monopolized by a single male or male coalition (top of each graph). Likewise, such a tendency was found for copulations with other group males. Notably, males forming coalitions in male coalition monopoly groups could successfully copulate with a larger number of females within the groups, i.e., the Ting-Group (84.0% of the overall number of females in the group), Nadam-Group (70.0%) and Fourth-Group (75.0%), compared with the single male monopolizing copulation opportunities in each of the single-male monopoly groups, i.e., the Third-Group (41.6%) and Wngklm-Group (50.0%).
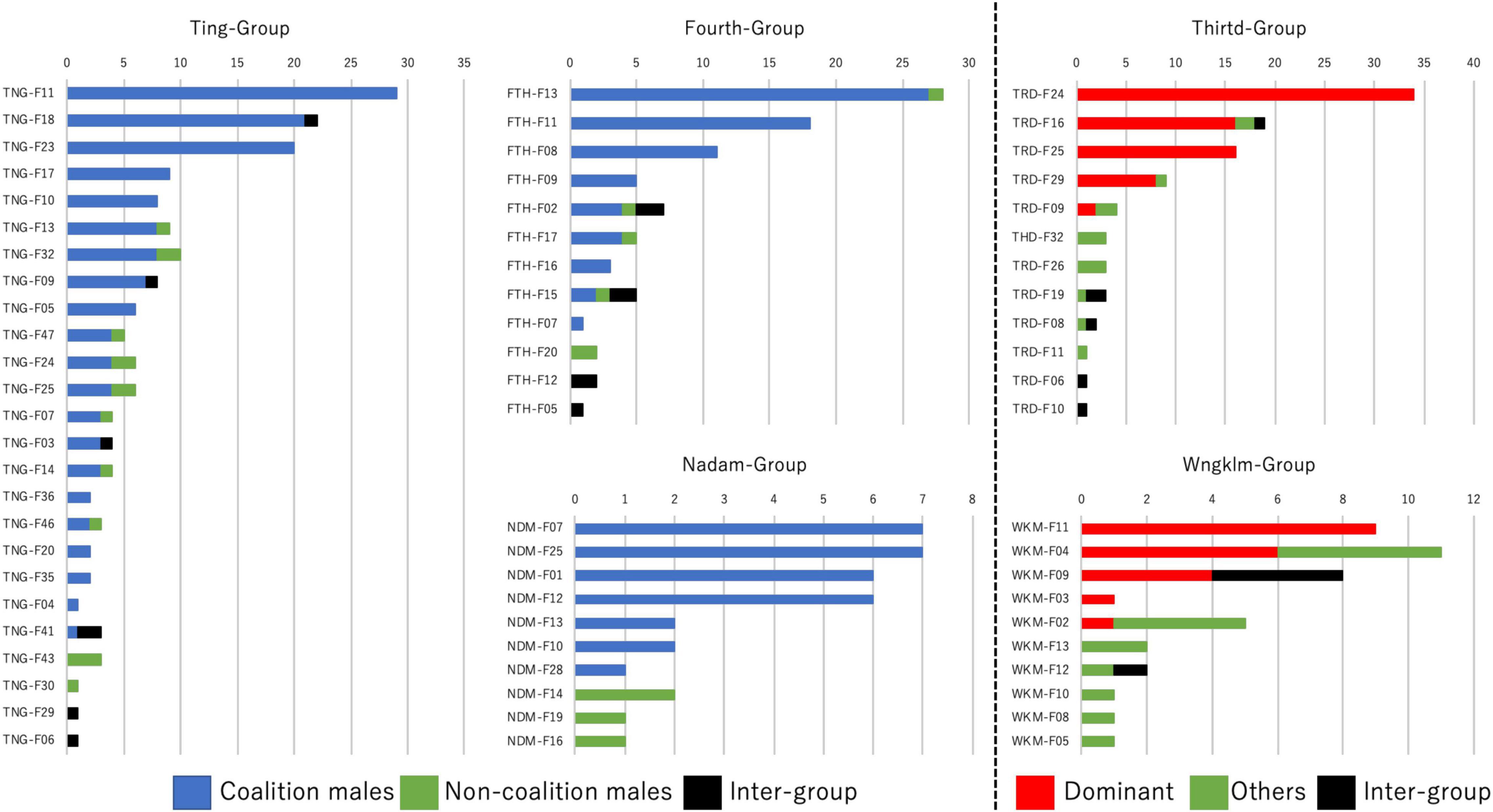
Figure 4. The distribution of female copulations in the study groups. In the Ting-Group, Fourth-Group, and Nadam-Group, copulations with males in coalition mode (blue) and non-coalition males (green) within groups and with males from different groups (black) are shown in different colors. In the Third-Group and Wngklm-Group, copulations with a single male monopolizing copulations (red) and other males (green) from within groups and from different groups (black) are shown in different colors.
Reproductive Success and Pair-Wise Relatedness in Males
Paternity Determination With a Comparison of Reproductive Success
DNA samples were collected from 48 mother–infant pairs within the possible 115 mother–infant pairs during the study period. The coverage rate of potential paternity candidacy was 91.7% (Toyoda and Malaivijitnond, 2018). We set a confidence level to guarantee the accurate judgment of the father candidate estimated using Cervus; however, we did not achieve this confidence level in six pairs. Because only two samples for mother–infant pairs were available in the Nadam-Group, we excluded this group from paternity determination. Consequently, we successfully determined the paternity of 36 mother–infant pairs with >80% confidence levels.
In the Third-Group, paternity was determined in 10 out of 13 tested infants. A single male (TRD-M01) who monopolized the copulations sired only four infants, although the male was the most successful in mating among all group males (Table 1). Additionally, the results of paternity determination showed that the degree of reproductive success did not reflect mating success in the Third-Group (Fisher’s exact test, P = 0.02). Of the 18 tested infants in the Ting-Group (male coalition monopoly group), paternity was determined for 16 infants. The six males in coalition mode sired six infants. We found that the degree of reproductive success reflected mating success in the Ting-Group (P = 0.27), suggesting that males involved in the coalition mode fairly shared their copulation opportunities.
It should be noted that the statistical evaluation of the difference between the mating and reproductive success in other groups (Wngklm-Group and Fourth-Group) was not performed because of the small sample size of infants for paternity determination. Nonetheless, although the sample size was not sufficient for statistical evaluation, in the single-male monopoly group, i.e., Wngklm-Group, the male (WKM-M01) that mostly occupied copulations, was surprisingly excluded as a paternal candidate for all tested infants; paternity was determined in five out of nine infants. The male (WKM-M03) that sired two infants was the most reproductive in the Wngklm-Group, although copulation by this male was not observed. In the Fourth-Group (male coalition monopoly group), the males who were involved in the coalition mode were excluded as paternal candidates for all tested infants for which paternity was determined; paternity was determined in five out of seven infants. Conversely, one infant was sired by each of the two males (FTH-M04 and FTH-M06), and they were the most reproductive in the Fourth-Group.
Although inter-group copulations were rarely observed, extra-group paternity was confirmed in the study groups, i.e., in 25.0–30.1% of all confirmed infants (Table 1). The reason for the variation in the percentages was that one male (WKM-M03) repeatedly migrated/immigrated between Third- and Wngklm-Group during the study period. More specifically, the extra-group paternity of the two infants in the Third-Group, two in the Ting-Group, two in the Wngklm-Group and three in the Fourth-Group was determined. Several infants with extra-group paternity tended to be related to a number of adult males in each group. More infants with extra-group paternity were detected in the groups with fewer males, i.e., the number of infants with extra-group paternity accounted for 12.5% in the Ting-Group (30 males), 20.0% in the Third-Group (23 males), 60% in the Fourth-Group (16 males) and 40–80% in the Wngklm-Group (8 males) from all confirmed infants in each group.
Male–Male Relatedness
In the results of GenAlEx analysis, there was a significant difference in the pair-wise relatedness of males within study groups across the five study groups (Kruskal–Wallis test: χ2 = 15.147, P = 0.004; Figure 5). The Steel–Dwass test for multiple comparisons showed that pair-wise relatedness of males within the Ting-Group was significantly higher than that of males within the Fourth-Group (t = 3.49, P = 0.0043). Both Ting-Group and Fourth-Group were male coalition monopoly groups, indicating that the overall structure of higher genetic relatedness among males was not always the driving force for male–male coalitions.
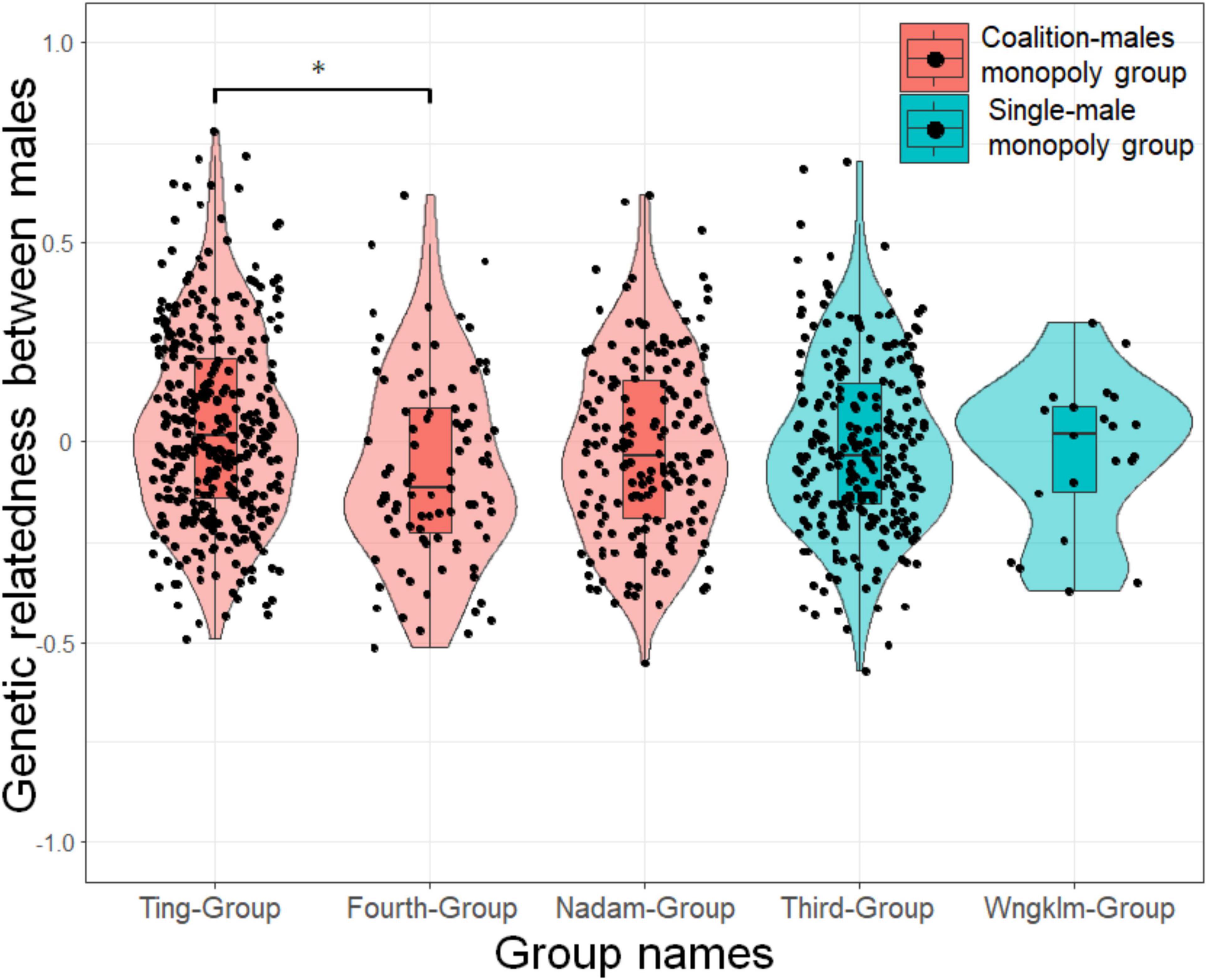
Figure 5. Violin plot showing the average relatedness between males in the five study groups. Different colors indicate the group types, i.e., male coalition monopoly or single-male monopoly groups.
Conversely, males tended to choose genetically closer males as coalition partners within the groups (Figure 6). In the Ting-Group, the relatedness among the male coalition was significantly higher than that among the other non-coalition males (asymptotic 2-sample permutation test, p < 0.05; Figure 6). Nonetheless, the coalitions were not always formed among the highly related males in the Ting-Group because relatedness fluctuated from 0.06 (TNG-M02 and TNG-M07) to 0.48 (TNG-M07 and TNG-M09) among the males forming the coalitions (Figure 3). In the other two groups, statistical comparisons of pair-wise relatedness were impossible because of the small number of males participating in the coalitions; however, the coalitions were not formed in the most closely related male combinations within the groups similar to that of the Ting-Group.
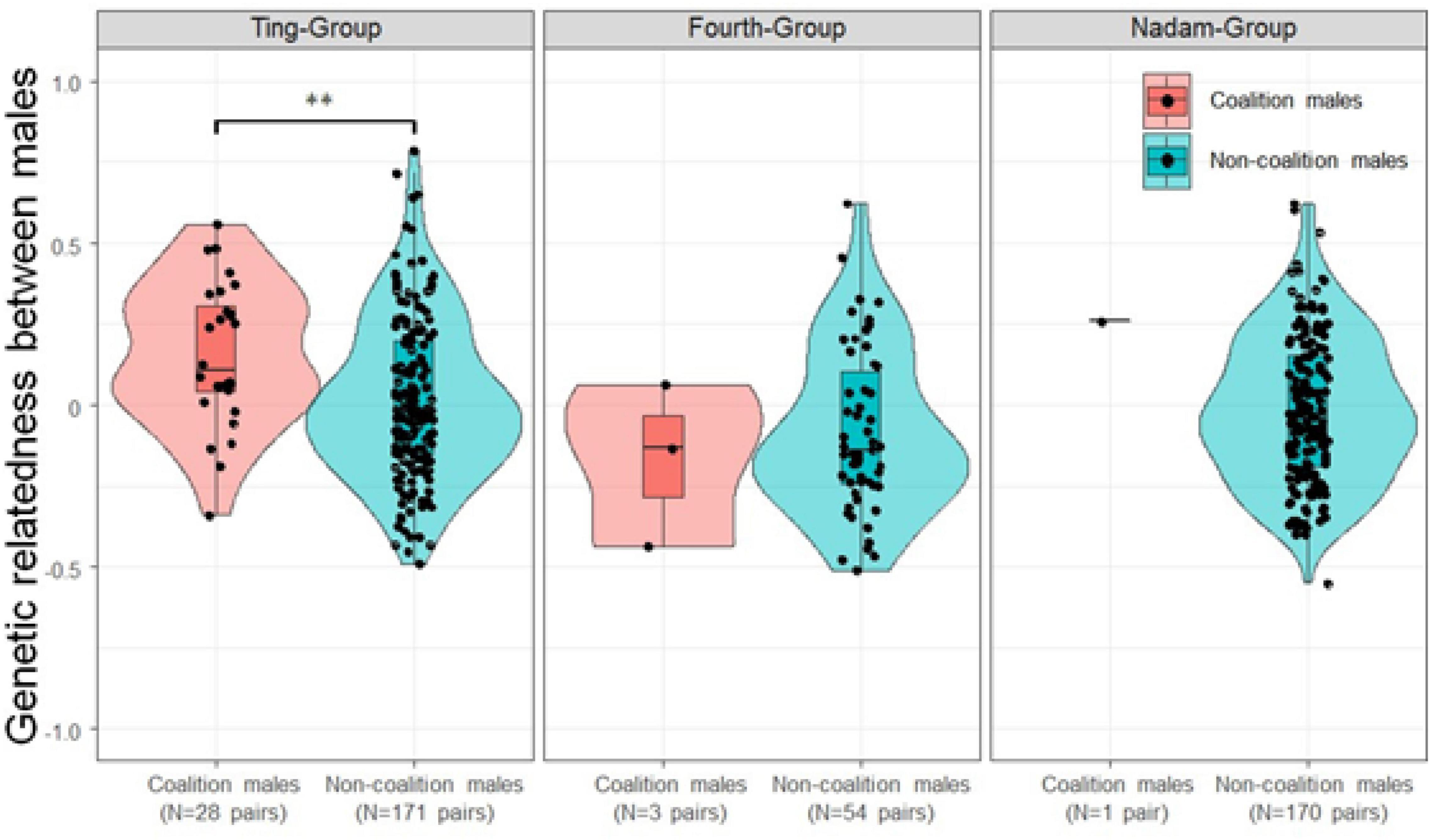
Figure 6. Violin plot showing the average relatedness between the males in coalition mode and other males in each male coalition monopoly groups. Note that conducting the asymptotic 2-sample permutation test was possible only in the Ting-Group and not in the Nadam-Group and Fourth-Group because of their small sample size.
Among the males forming the coalition, there were several male-male pairs categorized into PO, FS, and HS, especially in the Ting group (see the details in Supplementary Table 3). It was confirmed that a pair of the males judged as PO and FS could be dismissed as unrelated (U), though the pairs judged as HS could not be dismissed. In addition, all pairs of males judged as U could be dismissed as HS. Thus, The assigned kinship by ML-RELATE supported the results based on the relatedness. In the Ting-Group, among the 10 dyads of male pairs involved in the male coalition, 6 dyads were assigned as related, i.e., PO, FS, or HS. The other 4 dyads were assigned as unrelared. Note that only 3 of the 10 cases were statistically singinicant probably due to the small number of microsatellite markers used in this study. The male dyad of the Nadam-Group was assigned as HS, and the three male dyads of the Fourth-Group were assigned as unrelated (but not significant in all cases).
On the other hand, it was not certain that a particular combination of relationships can be affirmed as PO, FS, or HS. Therefore, to verify the accuracy of categorical judgment by ML-RELATE, based on the dataset of mothers and infants with clear consanguinity, we calculated the false negative rate for not judging the mother as PO and the false positive rate for judging extra-group females that should be non-consanguineous to the infant as PO, FS, and HS. The false negative rate was 26.9% and the false positive rate was 31.3%. These results were possibly because too few loci were analyzed for evaluating the pedigree relationship using ML-RELATE. Indeed, the data set used for analysis contained many mother–infant pairs; therefore, the proportions of the samples were biased. In the pedigree relationship estimated using ML-RELATE, combinations of males with the same age were determined to be POs; these were clearly incorrect estimations based on our field observations (Supplementary Table 3). Therefore, we could not deny that the males forming the coalition include “fathers and sons (PO)” or “brothers (FS).”
A common conclusion of the relatedness analysis of pairs formed by male coalitions using GenAlEx and ML-RELATE was that the coalition males of the Ting-Group contained potentially highly related male pairs, but that there was no evidence of relatedness between coalition males in Nadam-Group and Fourth-Group.
Discussion
Copulatory Behavior in Relation to Male–Male Coalition and Paternity
This study is the first to describe the detailed copulatory behaviors of M. arctoides in the wild. It, therefore, provides a complete depiction of their unique mating strategies, especially the coalition mode of forming male–male alliances with shared mating opportunities, which has never been reported in other female-philopatric primates. Echoing the observation of copulatory behavior in this study, Fooden (1990) noted the unique copulation, especially the so-called serial copulation, in M. arctoides to be strikingly different from other phylogenetically close macaque species, such as M. sylvanus and M. radiata. Additionally, as reported in captive M. arctoides (Estep et al., 1984; Brereton, 1994), in this study, we also found that only a few males monopolized the mating opportunities even in the free-ranging groups. Although the dominance rank was not systematically tested in this study, males who monopolized mating opportunities in our study were seemingly higher-ranking males in the groups as shown by previous studies on M. arctoides in captivity (Gouzoules, 1974; Niemeyer and Chamove, 1983; Estep et al., 1988). Furthermore, in this study, the distribution of copulations among females was not prominently skewed as observed in case of males; however, it was not evenly distributed, which is similar to previous findings of studies on M. arctoides in captivity (Estep et al., 1984; Brereton, 1994).
Although basic copulatory behaviors in free-ranging populations are comparable to those in captivity, our notable findings were the copulatory behaviors in relation to two different mating strategies by males, which has not been reported even in free-ranging populations (Richter et al., 2009). In this study, coalition mode was observed in three out of five study groups while engaging in serial copulations, as reported by Toyoda et al. (2020b). Based on the analysis, focusing on the number of males monopolizing the copulations within groups, we elucidated about two different group types with different mating strategies, i.e., single-male monopoly and male coalition monopoly. Nearly 80% of copulations were monopolized by a single male or several males (coalition mode). Because monopolizing access to fertile females is one of the universal strategies in non-human primates to maximize the reproductive success for males, e.g., chimpanzees (Nishida, 1990), mandrills (Setchell et al., 2005) and long-tailed macaques (Engelhardt et al., 2006), both mating strategies would be successful.
Nonetheless, based on the genetic data assessing the paternity of infants in the study groups, benefits and drawbacks in two different mating strategies were revealed. If only one male can monopolize the females within the groups, such as the Third-Group and Wngklm-Group, it would be the best reproductive strategy. In reality, however, such a strategy allowed for opportunistic/sneaky copulations by other males with a relatively high probability. Thus, the degree of mating success did not reflect the reproductive success in the single-male monopoly group. Indeed, in the single-male monopolized groups, the peripheral males in whom copulations were never observed sired the same number of infants as the males that monopolized the observed copulations in the Third- and Wngklm-Groups (Table 1). Thus, opportunistic/sneaky copulations may serve as an effective strategy for those peripheral or non-group males who cannot constantly access females. Contrary to a study in captivity that showed that alpha males effectively achieved a virtual monopoly in siring an offspring (Hearn and Bauers, 1994), monitoring all group females by only one or a few males may be difficult in free-ranging populations. In fact, in an experimental study of captive M. arctoides, when higher-ranking males were removed from their view in captivity, lower-ranking males started copulating more frequently (Estep et al., 1988), supporting the fact that opportunistic/sneaky copulations commonly occur in natural habitats where subordinates have more opportunity to escape the view of dominant males for copulations.
Contrastingly, the males in coalition mode could successfully share their mating and reproductive success in the male coalition monopoly group, especially in the Ting-Group. Paternity determination by microsatellite analysis showed that reproductive success among the male coalition was almost equally distributed. In addition, compared with the single-male monopolized groups, males of the male coalition monopoly groups could successfully copulate with a larger number females in the group, suggesting that male copulation would effectively guard females against opportunistic/sneaky copulations by other rival males. Coalition formation is not only beneficial to cover a larger number of females as mating partners but also allows males to be more vigilant and capable of detecting other rival males approaching the females. Thus, advantageous factors, such as an effective mating guard, triggered male–male coalitions in M. arctoides, particularly in a larger group, as observed in the Ting-Group. Although it has been suggested that M. arctoides relies on non-visual cues to assess female reproductive status (Murray et al., 1985; Cerda-Molina et al., 2006), it has been noted to be a non-seasonal breeder, and the lack of visual signals, such as the swelling of the female’s perineum (Napier and Napier, 1967; Bertrand, 1969; Heldstab et al., 2021), supports the idea that keeping a constant watch on both females and rival males would be profitable by multiple males in a coalition compared with a single male in larger groups.
Nonetheless, this result should be interpreted with caution because the results of paternity determination showed that reproductive success did not reflect mating success even in the male coalition monopoly group, i.e., the Fourth-Group. In other words, despite the fact that the male coalition conducted most of the ejaculatory copulations, their reproductive success was lower than expected from the number of observed copulations, indicating that peripheral or non-group males have higher reproductive success than expected. Differing group size, particularly the number of males in groups, might have led to this inconsistency between the Ting- (30 adult males in total) and Fourth-Groups (16 males). A higher number of males in a group would allow males to organize multiple combinations of coalition formation, enabling more males to have copulation opportunities with females, as observed in the Ting-Group. Therefore, the gap between mating and reproductive success may be less prominent. In the case of the Fourth-Group, despite the observed male–male coalition, a single combination of coalition formation involving only three males was detected, which possibly induced the prominent skew of mating success in group males owing to the number of group males being lower than in the Ting-Group. Thus, in male coalition monopoly groups, the number of group males in relation to the number of males involved in the coalition formation and monopolizing copulation opportunities is key in explaining whether the group males can fairly share their mating and reproductive success.
Sneaky/Opportunistic Copulation in Relation to Partner Preference by Females
The gap between mating and reproductive success has been reported in other primates. A study on chimpanzees showed that while only alpha males monopolized females to achieve paternity, other high- and middle-ranking males achieved paternity through opportunistic mating (Constable et al., 2001), concluding that such mating may be an effective strategy for non-alpha males because they cannot monopolize females. In addition, several studies evaluating paternity in free-ranging M. fuscata showed a similar tendency that mating success does not necessarily result in high reproductive success, with this inconsistency being explained by female mate choice (Inoue et al., 1990; Soltis et al., 2001; Hayakawa, 2008). Indeed, mating success was positively correlated with male dominance in M. fuscata; however, paternity tests showed that higher-ranking males did not sire the infants within groups (Inoue et al., 1993). These results are consistent with the fact that M. fuscata females copulated with higher-ranking males with long tenures during the non-ovulation period. However, lower-ranking males with shorter tenures are selected as mating partners during the ovulation period (Inoue and Takenaka, 2008). Furthermore, a study on M. mulatta showed that dominance was not associated with reproductive success, with 4 of the 11 infants being sired by males from other social groups, and the four infants of unknown paternity were sired either by males who were not observed mating with the females or the lower-ranking males who were not fingerprinted (Berard et al., 1993). This result supports the fact that females prefer low-ranking or non-group males as “less familiar” or “novel” partners as suggested in M. fuscata (Takahata et al., 1999). Although female preference in M. arctoides has not been studied well, an experimental study revealed that female proceptive behavior increases in the absence of high-ranking males (Estep et al., 1988), indicating that similar to that in other Macaca spp., effects of female preference is an important factor in understanding the gap between mating and reproductive success in M. arctoides.
Sneaky/opportunistic copulations in relation to female preference may be explained well by the number of group males in M. arctoides; although, female preferences are commonly difficult to assess (Clutton-Brock and McAuliffe, 2009). In the two study groups with a small number of males (Fourth- and Wngklm-Group), infants were mostly not sired by the male(s) monopolizing the mating opportunity in the groups but were sired by other rival males within groups or other groups. In theory, it should be easier to monitor all group members and detect sneaking copulations if females do not actively move away from the center of the group to follow less familiar or novel male partners within groups or in other groups. Therefore, given the observed low mating success by sneaking males but with relatively high reproductive success, it would be assumed that reproductive partner choice (not mating partner choice) is strongly affected by female preferences in those two study groups with a small number of males. Thus, because there were not many options for reproductive partner choice within Fourth- and Wngklm-Groups, females might have actively sought less familiar or novel partners as reported in other macaque species (Takahata et al., 1999). Furthermore, the two study groups with a larger number of males, especially in the Ting-Group, showed a less prominent gap between mating and reproductive success with less sneaky/opportunistic reproductive success, supporting our opinion that the number of males influence the level of female preference in M. arctoides.
Male Relatedness and Coalition
Genetic analysis focusing on the relatedness among males within groups partly revealed the factor determining the formation of male coalitions. In the Ting-Group, the largest male coalition monopoly group, including several combinations of male–male coalitions, relatedness among the male coalition was significantly higher than that in non-coalition males. Although this result can not immediately imply a “brotherly” coalition among males due to the trade-off issues of coalition formation, it is a reasonable outcome, wherein even if those males are competitors for paternity, the degree of inclusive fitness increases to form a coalition with males who are highly related. This explanation would also be supported by the fact that there was no extreme reproductive skew among the male coalitions in the Ting-Group.
Male–male coalitions or cooperative alliances, often involving close kin, e.g., maternal brothers, have been reported in primates with male philopatry, e.g., chimpanzees, bonobos and spider monkeys (Nishida and Hiraiwa-Hasegawa, 1987; Valero et al., 2006), and males are often tolerant to group males and share copulations with receptive females (Nishida, 1983; Watts, 1998; Strier et al., 2002). Thus, the question to be resolved is under what conditions can the degree of relatedness increase among certain males in a matrilineal social system, such as that of M. arctoides, and how the male coalition perceives such higher relatedness. One possibility is the male “parallel dispersal,” in which several males migrate from the same group to another group together, as observed in the study groups. During the study period, 36 cases of migration were observed, and 15 cases occurred from the same original group to the same group within 2 weeks after the predecessors moved on (unpublished observation by Toyoda). It is entirely possible that the “familiar” males, born and grown in the same group (possibly with high relatedness), chose them as coalition partners after their transfer to new groups. Male–male coalitions observed in non-human primates, e.g., Alouatta seniculus (Pope, 1990) and Cebus capucinus (Wikberg et al., 2014), have been suggested to be the results of such parallel dispersal (Jack et al., 2009). Further evaluation of such effects of parallel dispersal on increased relatedness and preference to form male coalitions in the matrilineal social system of M. arctoides is necessary in a future study.
However, the combination of low relatedness male–male coalitions in the Ting-Group and similar coalitions between low relatedness males were also prominent in other male coalition monopoly groups. Among primate species with male dispersal, observation of coalitions with non-kin alliances is less frequent in general. Under certain conditions of competition for access to sexually receptive females, such male–male coalitions with non-kin alliances have been observed mostly in the context of aggressive interactions. For instance, males may develop stable alliances with each other to overpower younger higher-ranking rivals in Papio cynocephalus (Cheney et al., 1986), to takeover groups of females in Alouatta seniculus (Crockett and Sekulic, 1984) and to support other males in agonistic coalitions in M. radiata (Silk, 1992) and Saimiri sciureus (Mitchell, 1994). Schulke et al. (2010) also demonstrated a causal link between differentiated social bonds and fitness in M. assamensis males, especially in the absence of strong kin bias. In both kin and non-kin male–male coalitions, increasing male mating opportunities leading to reproductive success may explain the formation of male–male alliances with either lower or higher relatedness among members in M. arctoides, which is consistent with the findings of previous studies.
Outlook
We followed up with multiple groups of M. arctoides over a period of 21 months. We successfully collected genetic samples from nearly all potential sires and approximately half of the mother–child pairs despite studying the population under natural conditions. Consequently, we were able to outline their reproductive success in relation to the mating strategies of males. We also revealed a rare phenomenon by which different social conditions and kinship structures within groups promoted a male–male coalition. In a future study, determining the full set of infant paternity with a longer observation period would provide further understanding of reproductive success spanning the entire lifetime through factors such as the history of males, female mate choice and genetic kinship.
Lastly, non-human primates often are representative reference models for understanding evolutionary trajectories of human cooperative behaviors, including the male–male coalition, which is common in human society (Silk, 2003). Similar to human society, coalition mode forming male–male alliances often occurs among relatives and non-relatives, indicating the complexity and flexibility inherent in the male social bond of M. arctoides. M. arctoides, thus, may be a useful species to understand the evolutionary functions of friendship, which is a fundamental element of our social life.
Data Availability Statement
The original contributions presented in the study are included in the article/Supplementary Material, further inquiries can be directed to the corresponding author/s.
Ethics Statement
The animal study was reviewed and approved by the Department of National Parks, Wildlife and Plant Conservation of Thailand.
Author Contributions
AT, TM, and IM conceptualized the initial idea. AT performed the field observation. AT, YK, and KM conducted genetic analysis at the laboratory. SM arranged the sampling and laboratory analyses in Thailand. AT and IM performed and interpreted the statistical analyses and drafted the manuscript. All authors contributed to the final version of the manuscript.
Funding
This study was financed by JSPS KAKENHI (#16J0098 to AT and #19KK0191 to AT and IM), Young Science Explorer Grant of National Geographic Foundation for Science and Exploration – Asia (#Asia-22-15 to AT), Kyoto University Foundation (to AT), the Sasakawa Scientific Research Grant from The Japan Science Society (#2020-5028 to AT), and the Cooperation Research Programs of Wildlife Research Center and Primate Research Institute, Kyoto University (#2015-Jiyuu-8 to AT), and it was supported by the Japan Science and Technology Agency, Core Research for Evolutional Science and Technology 17941861 (#JPMJCR17A4).
Conflict of Interest
The authors declare that the research was conducted in the absence of any commercial or financial relationships that could be construed as a potential conflict of interest.
Publisher’s Note
All claims expressed in this article are solely those of the authors and do not necessarily represent those of their affiliated organizations, or those of the publisher, the editors and the reviewers. Any product that may be evaluated in this article, or claim that may be made by its manufacturer, is not guaranteed or endorsed by the publisher.
Acknowledgments
We thank Warayut Nilpaung and Chuchat Choklap, the superintendents of the Khao Krapuk Khao Taomor Non-Hunting Area; Phanlerd Inprasoet, Wanchai Inprasoet, and Napatchaya Techaatiwatkun for providing valuable support that ensured the success of our fieldwork; Yuzuru Hamada, Hiroki Koda, Ikuma Adachi, and Takeshi Nishimura as well as all our colleagues for their support. All data acquisitions and procedures during the fieldwork were approved by the National Research Council of Thailand (#0002/6910) and the Department of National Parks, Wildlife and Plant Conservation of Thailand. We also complied with the guidelines for field studies of the Primate Research Institute, Kyoto University.
Supplementary Material
The Supplementary Material for this article can be found online at: https://www.frontiersin.org/articles/10.3389/fevo.2022.802012/full#supplementary-material
References
Berard, J. D., Nürnberg, P., Epplen, J. T., and Schmidtke, J. (1993). Male rank, reproductive behavior, and reproductive success in free-ranging rhesus macaques. Primates 34, 481–489. doi: 10.1007/978-3-0348-8583-6_45
Bissonnette, A., Franz, M., Schülke, O., and Ostner, J. (2014). Socioecology, but not cognition, predicts male coalitions across primates. Behav. Ecol. 25, 794–801.
Brereton, A. R. (1994). Copulatory behavior in a free-ranging population of stumptail macaques (Macaca arctoides) in Mexico. Primates 35, 113–122. doi: 10.1007/bf02382048
Butovskaya, M. (1993). Kinship and different dominance styles in groups of three species of the genus Macaca (M. arctoides, M. mulatta, M. fascicularis). Folia Primatol. 60, 210–224. doi: 10.1159/000156694
Caro, T. M., and Collins, D. A. (2010). Male cheetah social organization and territoriality. Ethology 74, 52–64. doi: 10.1111/j.1439-0310.1987.tb00921.x
Cerda-Molina, A. L., Hernández-López, L., Rojas-Maya, S., Murcia-Mejía, C., and Mondragón-Ceballos, R. (2006). Male-induced sociosexual behavior by vaginal secretions in Macaca arctoides. Int. J. Primatol. 27, 791–807. doi: 10.1007/s10764-006-9045-0
Cheney, D., Seyfarth, R., and Smuts, B. (1986). Social relationships and social cognition in nonhuman primates. Science 234, 1361–1366. doi: 10.1126/science.3538419
Clutton-Brock, T. H. (1988). Reproductive Success: Studies of Individual Variation in Contrasting Breeding Systems. Chicago, IL: University of Chicago Press.
Clutton-Brock, T. H. (1989). Mammalian mating systems. Proc. R. Soc. Lond. B Biol. Sci. 236, 339–372.
Constable, J. L., Ashley, M. V., Goodall, J., and Pusey, A. E. (2001). Noninvasive paternity assignment in Gombe chimpanzees. Mol. Ecol. 10, 1279–1300. doi: 10.1046/j.1365-294x.2001.01262.x
Cowlishaw, G., and Dunbar, R. I. M. (1991). Dominance rank and mating success in male primates. Animal Behav. 41, 1045–1056.
Crockett, C. M., and Sekulic, R. (1984). “Infanticide in red howler monkeys (Alouatta seniculus),” in Infanticide: Comparative and Evolutionary Perspectives, eds G. Hausfater and S. B. Hrdy (New York, NY: Aldine), 173–191.
de Ruiter, J. R., and van Hooff, J. A. R. A. M. (1993). Male dominance rank and reproductive success in primate groups. Primates 34, 513–523.
de Waal, F. B. M., and Luttrell, L. M. (1989). Toward a comparative socioecology of the genus Macaca: different dominance styles in rhesus and stumptail monkeys. Am. J. Primatol. 19, 83–109. doi: 10.1002/ajp.1350190203
de Waal, F. B. M., and Ren, R. (1988). Comparison of the reconciliation behavior of stumptail and rhesus macaques. Ethology 78, 129–142.
Delson, E. (1980). “Fossil macaques, phyletic relationships and a scenario of development,” in The Macaques: Studies in Ecology, Behavior, and Evolution, ed. D. G. Lindburg (New York, NY: Van Nostrand Reinhold), 10–30.
Diaz-Aguirre, F., Parra, G. J., Passadore, C., and Möller, L. (2018). Kinship influences social bonds among male southern Australian bottlenose dolphins (Tursiops cf. australis). Behav. Ecol. Sociobiol. 72:190.
Emlen, S. T., and Oring, L. W. (1977). Ecology, sexual selection, and the evolution of mating systems. Science 197, 215–223. doi: 10.1126/science.327542
Engelhardt, A., Heistermann, M., Hodges, J. K., Nürnberg, P., and Niemitz, C. (2006). Determinants of male reproductive success in wild long-tailed macaques (Macaca fascicularis)—male monopolisation, female mate choice or post-copulatory mechanisms? Behav. Ecol. Sociobiol. 59, 740–752.
Estep, D. Q., Bruce, K. E., Johnston, M. E., and Gordon, T. P. (1984). Sexual behavior of group-housed stumptail macaques (Macaca arctoides): temporal, demographic and sociosexual relationships. Folia Primatol. 42, 115–126. doi: 10.1159/000156154
Estep, D. Q., Nieuwenhuijsen, K., Bruce, K. E. M., De Neef, K. J., Walters, P. A., Baker, S. C., et al. (1988). Inhibition of sexual behaviour among subordinate stumptail macaques, Macaca arctoides. Anim. Behav. 36, 854–864.
Fa, J. E., and Lindburg, D. G. (2005). Evolution and Ecology of Macaque Societies. Cambridge, MA: Cambridge University Press.
Fedigan, L. M. (1983). Dominance and reproductive success in primates. Am. J. Phys. Anthropol. 26, 91–129. doi: 10.1002/ajpa.1330260506
Fooden, J. (1990). The bear macaque, Macaca arctoides: a systematic review. J. Hum. Evol. 19, 607–686. doi: 10.1016/0047-2484(90)90002-s
Gerber, L., Wittwer, S., Allen, S. J., Holmes, K. G., King, S. L., Sherwin, W. B., et al. (2021). Cooperative partner choice in multi-level male dolphin alliances. Sci. Rep. 11:6901. doi: 10.1038/s41598-021-85583-x
Gouzoules, H. (1974). Harassment of sexual behavior in the stumptail macaque, Macaca arctoides. Folia Primatol. 22, 208–217. doi: 10.1159/000155625
Harcourt, A. H., and de Waal, F. B. M. (1992). Coalitions and Alliances in Humans and Other Animals. New York, NY: Oxford University Press.
Hayaishi, S., and Kawamoto, Y. (2006). Low genetic diversity and biased distribution of mitochondrial DNA haplotypes in the Japanese macaque (Macaca fuscata yakui) on Yakushima Island. Primates 47, 158–164. doi: 10.1007/s10329-005-0169-1
Hayakawa, S. (2008). Male-female mating tactics and paternity of wild Japanese Macaques (Macaca fuscata yakui). Am. J. Primatol. 70, 986–989. doi: 10.1002/ajp.20580
Hearn, J. P., and Bauers, K. A. (1994). Patterns of paternity in relation to male social rank in the stumptailed macaque, Macaca arctoides. Behaviour 129, 149–176. doi: 10.1163/156853994x00596
Heldstab, S. A., van Schaik, C. P., Muller, D. W. H., Rensch, E., Lackey, L. B., Zerbe, P., et al. (2021). Reproductive seasonality in primates: patterns, concepts and unsolved questions. Biol. Rev. Camb. Philos. Soc. 96, 66–88. doi: 10.1111/brv.12646
Inoue, E., and Takenaka, O. (2008). The effect of male tenure and female mate choice on paternity in free-ranging Japanese macaques. Am. J. Primatol. 70, 62–68. doi: 10.1002/ajp.20457
Inoue, M., Mitsunaga, F., Nozaki, M., Ohsawa, H., Takenaka, A., Sugiyama, Y., et al. (1993). Male dominance rank and reproductive success in an enclosed group of Japanese macaques: with special reference to post-conception mating. Primates 34, 503–511. doi: 10.1007/bf02382661
Inoue, M., Takenaka, A., Tanaka, S., Kominami, R., and Takenaka, O. (1990). Paternity discrimination in a Japanese macaque group by DNA fingerprinting. Primates 31, 563–570. doi: 10.1159/000156548
Jack, K., Schoof, V., and Isbell, L. (2009). What traits promote male parallel dispersal in primates? Behaviour 146, 701–726. doi: 10.24272/j.issn.2095-8137.2020.085
Jiang, J., Yu, J., Li, J., Li, P., Fan, Z., Niu, L., et al. (2016). Mitochondrial genome and nuclear markers provide new insight into the evolutionary history of Macaques. PLoS One 11:e0154665. doi: 10.1371/journal.pone.0154665
Johnson, P. C., and Haydon, D. T. (2007). Maximum-likelihood estimation of allelic dropout and false allele error rates from microsatellite genotypes in the absence of reference data. Genetics 175, 827–842. doi: 10.1534/genetics.106.064618
Kalinowski, S. T., Wagner, A. P., and Taper, M. L. (2006). ML-RELATE: a computer program for maximum likelihood estimation of relatedness and relationship. Mol. Ecol. Notes 6, 576–579. doi: 10.1111/j.1471-8286.2006.01256.x
Kawamoto, Y., Takemoto, H., Higuchi, S., Sakamaki, T., Hart, J. A., Hart, T. B., et al. (2013). Genetic structure of wild bonobo populations: diversity of mitochondrial DNA and geographical distribution. PLoS One 8:e59660. doi: 10.1371/journal.pone.0059660
Mitchell, C. L. (1994). Migration alliances and coalitions among adult male South American squirrel monkeys (Saimiri sciureus). Behaviour 130, 169–190. doi: 10.1163/156853994x00514
Murray, R. D., Bour, E. S., and Smith, E. O. (1985). Female menstrual cyclicity and sexual behavior in stumptail Macaques (Macaca arctoides). Int. J. Primatol. 6, 101–113. doi: 10.1007/bf02693698
Niemeyer, C. L., and Chamove, A. S. (1983). Motivation of harassment of matings in stumptailed macaques. Behaviour 87, 298–322. doi: 10.1163/156853983x00480
Nishida, T. (1983). Alpha status and agonistic alliance in wild chimpanzees (Pan troglodytes schweinfurthii). Primates 24, 318–336. doi: 10.1007/bf02381978
Nishida, T. (1990). The Chimpanzees of the Mahale Mountains: Sexual and Life History Strategies. Tokyo: University of Tokyo Press.
Nishida, T., and Hiraiwa-Hasegawa, M. (1987). “Chimpanzees and bonobos: cooperative relationships among males,” in Primate Societies, eds B. B. Smuts, D. L. Cheney, R. M. Seyfarth, R. W. Wrangham, and T. T. Struhsaker (Chicago, IL: University of Chicago Press), 165–177.
Noë, R., and Sluijter, A. A. (1990). Reproductive tactics of male savanna baboons. Behaviour 113, 117–169.
Ostner, J., and Schülke, O. (2014). The evolution of social bonds in primate males. Behaviour 151, 871–906. doi: 10.1163/1568539x-00003191
Packer, C., Gilbert, D. A., Pusey, A. E., and O’Brieni, S. J. (1991). A molecular genetic analysis of kinship and cooperation in African lions. Nature 351, 562–565. doi: 10.1038/351562a0
Peakall, R., and Smouse, P. E. (2012). GenAlEx 6.5: genetic analysis in Excel. Population genetic software for teaching and research—an update. Bioinformatics 28, 2537–2539. doi: 10.1093/bioinformatics/bts460
Pope, T. R. (1990). The reproductive consequences of male cooperation in the red howler monkey: paternity exclusion in multi-male and single-male troops using genetic markers. Behav. Ecol. Sociobiol. 27, 439–446. doi: 10.1007/bf00164071
Pusey, A. E., and Packer, C. (1986). “Dispersal and philopatry,” in Primate Societies, eds B. B. Smuts, D. L. Cheney, R. M. Seyfarth, and R. W. Wrangham (Chicago, IL: University of Chicago Press), 250–266.
R-Core-Development-Team (2021). R: A Language and Environment for Statistical Computing. Vienna: Foundation for Statistical Computing.
Richter, C., Mevis, L., Malaivijitnond, S., Schulke, O., and Ostner, J. (2009). Social relationships in free-ranging male Macaca arctoides. Int. J. Primatol. 30, 625–642. doi: 10.1007/s10764-009-9364-z
Robinson, J. G. (1982). Intrasexual competition and mate choice in primates. Am. J. Primatol. 3, 131–144. doi: 10.1002/ajp.1350030520
Rodrigues, A. M., and Kokko, H. (2016). Models of social evolution: can we do better to predict ‘who helps whom to achieve what’? Philos. Trans. R. Soc. Lond. B Biol. Sci. 371:20150088. doi: 10.1098/rstb.2015.0088
Schulke, O., Bhagavatula, J., Vigilant, L., and Ostner, J. (2010). Social bonds enhance reproductive success in male macaques. Curr. Biol. 20, 2207–2210. doi: 10.1016/j.cub.2010.10.058
Setchell, J. M., Charpentier, M., and Wickings, E. J. (2005). Mate guarding and paternity in mandrills: factors influencing alpha male monopoly. Anim. Behav. 70, 1105–1120.
Setchell, J. M., Oliveira, R. F., Taborsky, M., and Brockmann, H. J. (2008). “Alternative reproductive tactics in primates,” in Alternative Reproductive Tactics: An Integrative Approach, eds R. Oliveira, M. Taborsky, and H. Brockmann (Cambridge, MA: Cambridge University Press), 373–398. doi: 10.1017/cbo9780511542602.016
Silk, J. B. (1992). “Patterns of intervention in agonistic contests among male bonnet macaques,” in Coalitions and Alliances in Humans and Other Animals, eds A. H. Harcourt and F. B. M. de Waal (Oxford: Oxford University Press), 215–232.
Silk, J. B. (2003). “Cooperation without counting: the puzzle of friendship,” in Dahlem Workshop Report: Genetic and Cultural Evolution of Cooperation, ed. P. Hammerstein (Cambridge, MA: MIT Press), 37–54.
Silk, J. B. (2009). Nepotistic cooperation in non-human primate groups. Philos. Trans. R. Soc. Lond. B Biol. Sci. 364, 3243–3254. doi: 10.1098/rstb.2009.0118
Soltis, J., Thomsen, R., and Takenaka, O. (2001). The interaction of male and female reproductive strategies and paternity in wild Japanese macaques, Macaca fuscata. Anim. Behav. 62, 485–494.
Strier, K., Dib, L., and Figueira, J. (2002). Social dynamics of male muriquis (Brachyteles arachnoides hypoxanthus). Behaviour 139, 315–342.
Takahata, Y., Huffman, M. A., Suzuki, S., Koyama, N., and Yamagiwa, J. (1999). Why dominants do not consistently attain high mating and reproductive success: a review of longitudinal Japanese macaque studies. Primates 40, 143–158. doi: 10.1007/BF02557707
Thierry, B. (2007). Unity in diversity: lessons from macaque societies. Evol. Anthropol. 16, 224–238.
Thierry, B. (2011). “The macaques: a double-layered social organization,” in Primates in Perspective, eds C. J. Campbell, A. Fuentes, K. C. Mackinnon, S. K. Bearder, and R. M. Stumpf (Oxford: Oxford University Press), 229–241.
Toyoda, A., and Malaivijitnond, S. (2018). The first record of dizygotic twins in semi-wild stump-tailed macaques (Macaca arctoides) tested using microsatellite markers and the occurrence of supernumerary nipples. Mamm. Study 43, 207–212.
Toyoda, A., Maruhashi, T., Malaivijitnond, S., and Koda, H. (2017). Speech-like orofacial oscillations in stump-tailed macaque (Macaca arctoides) facial and vocal signals. Am. J. Phys. Anthropol. 164, 435–439. doi: 10.1002/ajpa.23276
Toyoda, A., Maruhashi, T., Malaivijitnond, S., and Koda, H. (2020a). Dominance status and copulatory vocalizations among male stump-tailed macaques in Thailand. Primates 61, 685–694.
Toyoda, A., Maruhashi, T., Malaivijitnond, S., Koda, H., and Ihara, Y. (2020b). Mate sharing in male stump-tailed macaques as a possible case of coalition-like behavior to modify the group-wise fitness distribution. BioRxiv [Preprint]. doi: 10.1101/2020.01.30.927772
Toyoda, A., Matsudaira, K., Maruhashi, T., Malaivijitnond, S., and Kawamoto, Y. (2021). Highly versatile, non-Invasive method for collecting buccal DNA from free-ranging non-human primates. J. Trop. Biol. Conserv. 18, 251–267.
Valero, A., Schaffner, C. M., Vick, L. G., Aureli, F., and Ramos-Fernandez, G. (2006). Intragroup lethal aggression in wild spider monkeys. Am. J. Primatol. 68, 732–737. doi: 10.1002/ajp.20263
van Schaik, C. P., Pandit, S. A., and Vogel, E. R. (2006). “Toward a general model for male-male coalitions in primate groups,” in Cooperation in Primates and Humans, eds P. M. Kappeler and C. P. van Schaik (Berlin: Springer), 151–171.
Wagner, A. P., Creel, S., and Kalinowski, S. T. (2006). Estimating relatedness and relationships using microsatellite loci with null alleles. Heredity 97, 336–345. doi: 10.1038/sj.hdy.6800865
Watts, D. P. (1998). Coalitionary mate guarding by male chimpanzees at Ngogo, Kibale National Park, Uganda. Behav. Ecol. Sociobiol. 44, 43–55.
Wickings, E. J., Bossi, T., and Dixson, A. F. (1993). Reproductive success in the mandrill, Mandrillus sphinx: correlations of male dominance and mating success with paternity, as determined by DNA fingerprinting. J. Zool. 231, 563–574.
Wijitkosum, S. (2012). Impacts of land use changes on soil erosion in Pa Deng sub-district, adjacent area of Kaeng Krachan National Park, Thailand. Soil Water Res. 7, 10–17.
Wikberg, E. C., Jack, K. M., Campos, F. A., Fedigan, L. M., Sato, A., Bergstrom, M. L., et al. (2014). The effect of male parallel dispersal on the kin composition of groups in white-faced capuchins. Anim. Behav. 96, 9–17. doi: 10.1016/j.anbehav.2014.07.016
Keywords: Macaca arctoides, mating skew, reproductive skew, tolerance, relatedness, primates, non-invasive genetics
Citation: Toyoda A, Maruhashi T, Kawamoto Y, Matsudaira K, Matsuda I and Malaivijitnond S (2022) Mating and Reproductive Success in Free-Ranging Stump-Tailed Macaques: Effectiveness of Male–Male Coalition Formation as a Reproductive Strategy. Front. Ecol. Evol. 10:802012. doi: 10.3389/fevo.2022.802012
Received: 26 October 2021; Accepted: 11 February 2022;
Published: 07 March 2022.
Edited by:
Patricia Izar, University of São Paulo, BrazilReviewed by:
Pavel Munclinger, Charles University, CzechiaAnindita Bhadra, Indian Institute of Science Education and Research Kolkata, India
Copyright © 2022 Toyoda, Maruhashi, Kawamoto, Matsudaira, Matsuda and Malaivijitnond. This is an open-access article distributed under the terms of the Creative Commons Attribution License (CC BY). The use, distribution or reproduction in other forums is permitted, provided the original author(s) and the copyright owner(s) are credited and that the original publication in this journal is cited, in accordance with accepted academic practice. No use, distribution or reproduction is permitted which does not comply with these terms.
*Correspondence: Aru Toyoda, aru.toyoda@gmail.com
†ORCID: Aru Toyoda, orcid.org/0000-0003-1784-8553; Kazunari Matsudaira, orcid.org/0000-0003-2893-9928; Ikki Matsuda, orcid.org/0000-0002-0861-7801; Suchinda Malaivijitnond, orcid.org/0000-0003-0897-2632