- 1Department of Middle Grades and Secondary Education, Georgia Southern University, Savannah, GA, United States
- 2Nebraska Department of Environment and Energy, Lincoln, NE, United States
- 3Department of Statistics, University of Nebraska–Lincoln, Lincoln, NE, United States
- 4Department of Biological Sciences, Idaho State University, Pocatello, ID, United States
- 5School of Natural Resources, University of Nebraska–Lincoln, Lincoln, NE, United States
Terrestrial invertebrates are important subsidies to fish diets, though their seasonal dynamics and importance to tropical stream consumers are particularly understudied. In this year-round study of terrestrial invertebrate input to two Trinidadian headwater streams with different forest canopy densities, we sought to (a) measure the mass and composition of terrestrial inputs with fall-in traps to evaluate the influences of seasonality, canopy cover, and rainfall intensity, and; (b) compare terrestrial and benthic prey importance to Anablepsoides hartii (Hart’s Rivulus), the dominant invertivorous fish in these streams, by concurrently measuring benthic and drifting invertebrate standing stocks and the volume and composition of invertebrates in Rivulus guts throughout the year. The biomass of terrestrial invertebrate fall-in was 53% higher in the wet versus dry season; in particular, ant input was 320% higher. Ant biomass fall-in also increased with the density of canopy cover among sampling locations within both streams. Greater precipitation correlated with increased ant inputs to the more open-canopied stream and increased inputs of winged insects in the more closed canopy stream. Concurrently, the biomass of benthic invertebrates was reduced by more than half in the wet season in both streams. We detected no differences in the total volume of terrestrial prey in Rivulus diets between seasons, though ants were a greater proportion of their diet in the wet season. In contrast, benthic prey were nearly absent from Rivulus diets in the wet season in both streams. We conclude that terrestrial invertebrates are a substantial year-round prey subsidy for invertivores in tropical stream ecosystems like those we studied, which may contrast to most temperate streams where such terrestrial inputs are significantly reduced in the cold season. Interestingly, the strongest seasonal pattern in these tropical streams was observed in benthic invertebrate biomass which was greatly reduced and almost absent from Rivulus diets during the wet season. This pattern is essentially the inverse of the pattern observed in many temperate streams and highlights the need for additional studies in tropical ecosystems to better understand how spatial and temporal variation in terrestrial subsidies and benthic prey populations combine to influence consumer diets and the structure of tropical stream food webs.
Introduction
The movement of organisms, energy, and nutrients across habitat boundaries is often an important contributor to recipient ecosystem processes, populations, and communities (Polis et al., 1997; Reiners and Driese, 2001; Gounand et al., 2018). In the context of streams, terrestrially derived inputs like woody debris, dissolved organic matter and leaf litter and their implications for the structure and function of stream organisms and ecosystems have been well studied (Hynes, 1975; Wallace et al., 1997; Marcarelli et al., 2011). In addition, decades of studies have shown that terrestrial invertebrates can be an important, high quality prey subsidy to stream fishes (Hunt, 1975; Mason and MacDonald, 1982; Garman, 1991; Edwards and Huryn, 1995; Cloe and Garman, 1996; Wipfli, 1997; Nakano et al., 1999a; Kawaguchi and Nakano, 2001; Romero et al., 2005), and may allow these animals to achieve abundances beyond those that could be supported by local in situ production (Allen, 1951; Huryn, 1996; Wipfli, 1997; Nakano and Murakami, 2001; Kawaguchi et al., 2003; Bellmore et al., 2013; Behn and Baxter, 2019). Importantly, the majority of this research has identified strong seasonality in terrestrial invertebrate input to streams and their contribution to stream food webs; however, the bulk of the work has been geographically biased toward northern temperate ecosystems (see reviews by Baxter et al., 2005; Sullivan et al., 2012), and the effect that wet-dry season dynamics have on the magnitude and importance of terrestrial subsidies to tropical stream consumers is not well understood.
Dozens of temperate-zone studies have shown that terrestrial invertebrate input to streams is highly seasonal, typically peaking in the summer and approaching zero in the winter, and that these inputs fuel stream fishes, with attendant community and ecosystem-level consequences (see Baxter et al., 2005; Marcarelli et al., 2011 for reviews). As an illustration, in a well-studied stream of northern Japan, summer peaks in terrestrial invertebrate inputs coincided with a reduced availability of in situ prey biomass (benthic invertebrates), and fish feeding behavior and diet mirrored these changes, with 50–90% of fish diets consisting of terrestrial invertebrates during summer and 0% during the winter (Kawaguchi and Nakano, 2001), such that nearly half of the annual energy budget for the entire fish assemblage (126.5 kJ m–2 yr–1) was sustained by this subsidy (Nakano and Murakami, 2001). Moreover, experimental reduction of terrestrial invertebrate input to this stream in summer using a greenhouse cover reduced fish growth and population biomass (Nakano et al., 1999b; Kawaguchi et al., 2003; Baxter et al., 2007), but also induced a trophic cascade via a shift in fish diets from terrestrial to local aquatic prey (Nakano et al., 1999b; Baxter et al., 2004), which, in turn, decreased aquatic insect emergence and reduced riparian predators, such as spiders (Baxter et al., 2004). These examples serve to demonstrate that, in at least some temperate regions, seasonal inputs of terrestrial invertebrates subsidize populations of stream fishes with important consequences for community dynamics and ecosystem processes. Yet, despite observations pointing to cross-biome differences in phenology and relative contribution of this subsidy to fish diets (e.g., Rundio and Lindley, 2008, 2012; Zhang and Richardson, 2011; Behn and Baxter, 2019), there have been few studies of this phenomenon conducted outside temperate latitudes.
Tropical streams are understudied compared to their temperate counterparts and this is particularly true regarding the influence of terrestrial invertebrate subsidies (Wantzen et al., 2006; Dudgeon, 2011). Although the use of terrestrial invertebrates as a food resource for tropical stream fishes has been apparent for decades (e.g., Lowe-McConnell, 1975; Goulding, 1980; Winemiller et al., 2006), compared to work in temperate systems, there have been relatively few investigations of the seasonality of this subsidy or its importance to fishes (but see Angermeier and Karr, 1983; Moyle and Senayake, 1984; Chan et al., 2008). Primary productivity may be too low to support in situ fish production in many tropical streams, leading to a greater baseline reliance of tropical consumers on terrestrial resources (Lowe-McConnell, 1975; Goulding, 1980; Winemiller et al., 2006). Furthermore, seasonality in tropical ecosystems is defined more by uneven temporal distribution of rainfall, rather than temperature changes. Dry seasons in tropical forests are characterized by peaks in leaf fall (Wright and Cornejo, 1990) and reduced diversity and abundance of terrestrial insects (Erwin and Scott, 1980; Smythe, 1982; Broadhead, 1983; Kato et al., 1995; Novotny and Basset, 1998; Ades and Dudgeon, 1999). In contrast, the relative importance of terrestrial invertebrate subsidies might be expected to increase during the wet season due to reductions in benthic macroinvertebrate abundance caused by streambed scouring (Pringle et al., 1988; Resh et al., 1988; Boulton et al., 1992; Palmer et al., 1996) and increased terrestrial invertebrate abundance (Flecker and Feifarek, 1994; Ades and Dudgeon, 1999). In addition, terrestrial invertebrate delivery rates are likely to increase during precipitation due to dislodgement from overstory vegetation, as wind, rain, and flooding are known to influence their delivery to temperate streams (e.g., Mason and MacDonald, 1982; Edwards and Huryn, 1995; see Baxter et al., 2005 for review). Terrestrial invertebrate delivery to tropical streams is likely to vary with canopy density because the forest canopy houses a variety of invertebrate taxa that are vertically stratified (Bates, 1944; Wilson, 1959; Sutton et al., 1983; Longino and Nadkarni, 1990). Thus, there is also a need to investigate how variation in overstory vegetation interacts with precipitation to influence the delivery of terrestrial subsidies to tropical stream ecosystems.
Our objectives through this study were to evaluate how seasonality, riparian canopy structure, and precipitation influence the rates of terrestrial invertebrate input to two tropical headwater streams in Trinidad, West Indies, and in turn, to investigate the importance and variability of this prey subsidy to an important consumer of invertebrates in these streams, the generalist-feeding killifish Anablepsoides hartii (Hart’s Rivulus). With respect to the latter, we were especially interested in whether Rivulus tracked seasonal changes in the delivery and availability of terrestrial versus aquatic-derived benthic and drifting prey. We hypothesized that benthic aquatic insect biomass would decline during the wet season, and that precipitation would increase the flux of terrestrial subsidies through the physical dislodgement and delivery of invertebrates to streams (Supplementary Figure 1). We also predicted that differences in motility among terrestrial invertebrates, which affect their ability to seek shelter, would result in differential responses of winged versus non-winged invertebrate fall-in rates across precipitation and canopy cover conditions. Finally, we predicted that the differences in benthic invertebrate abundance and terrestrial invertebrate fall-in rates would be expressed in the absolute and relative composition of invertebrate prey in the drift and within the guts of Rivulus (Figure 1).
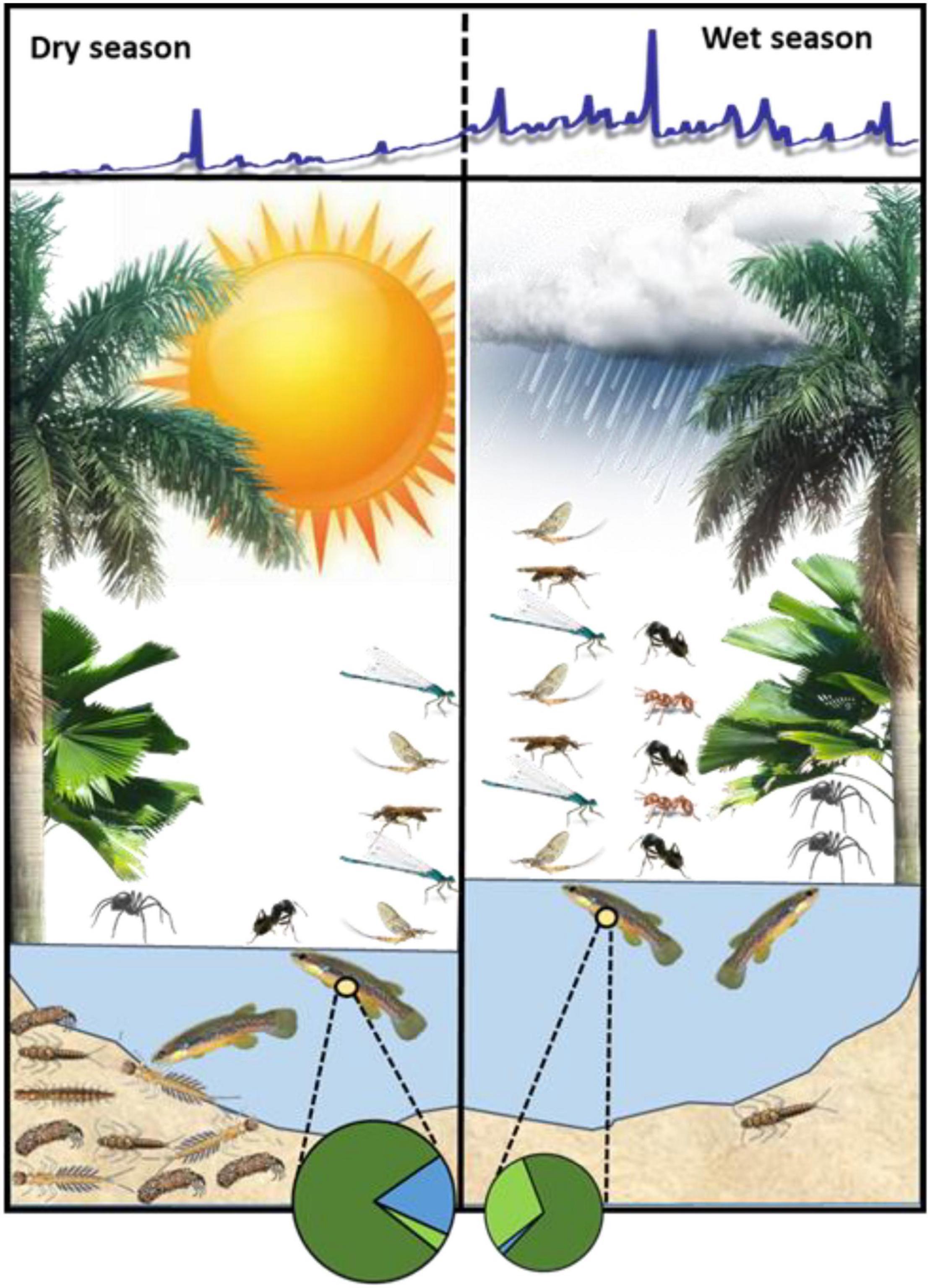
Figure 1. Seasonal differences in the supply of terrestrial and benthic food resources for Rivulus illustrate how benthic invertebrate production in the dry season provides a seasonal supplement to these fish. Terrestrial invertebrates include ants, winged adult insects, and non-ant, non-winged invertebrates (represented by spiders). Total falling input, and ant fall- in specifically, were significantly greater in the wet season than in the dry season, whereas winged adults and non-ant, non-winged invertebrates were similar between seasons. Benthic invertebrates were significantly depleted in the wet season due to frequent scouring flows. Pie diagrams represent the average proportional biovolume of invertebrate prey in Rivulus guts in each season. Invertebrate prey biovolume was almost twice as high in the dry season than in the wet season (reflected in pie diagram size). Detrital biovolume in Rivulus guts (not shown) dominated in both seasons and was about 25% higher in the dry season (Supplementary Table 3). Slices of each pie diagram represent the mean proportion of identifiable food items (terrestrial winged + non-ant, non-winged, dark green; ants, light green; aquatic, blue). The blue line above the figure is a hydrograph from a nearby stream (Upper La Laja, S. A. Thomas, unpublished data) that reflects the typical hydrology of this region during the dry and wet seasons.
Materials and Methods
Study Area
The study was conducted in two second order streams from March 2009 to August 2009 in the Northern Range of Trinidad (Figure 2 and Table 1): The Miguel (10°44′43′′N 61°13′11′′W) and the Tapana (10°44′38′′N 61°13′28′′W). Both drain into the Madamas River on the north side of Trinidad’s Northern Range. Vegetation in this region consists of both old growth (>100 years) and secondary (∼ 75 years) forest and our streams were selected to represent natural variation as well as legacy of land use through cultural practices.
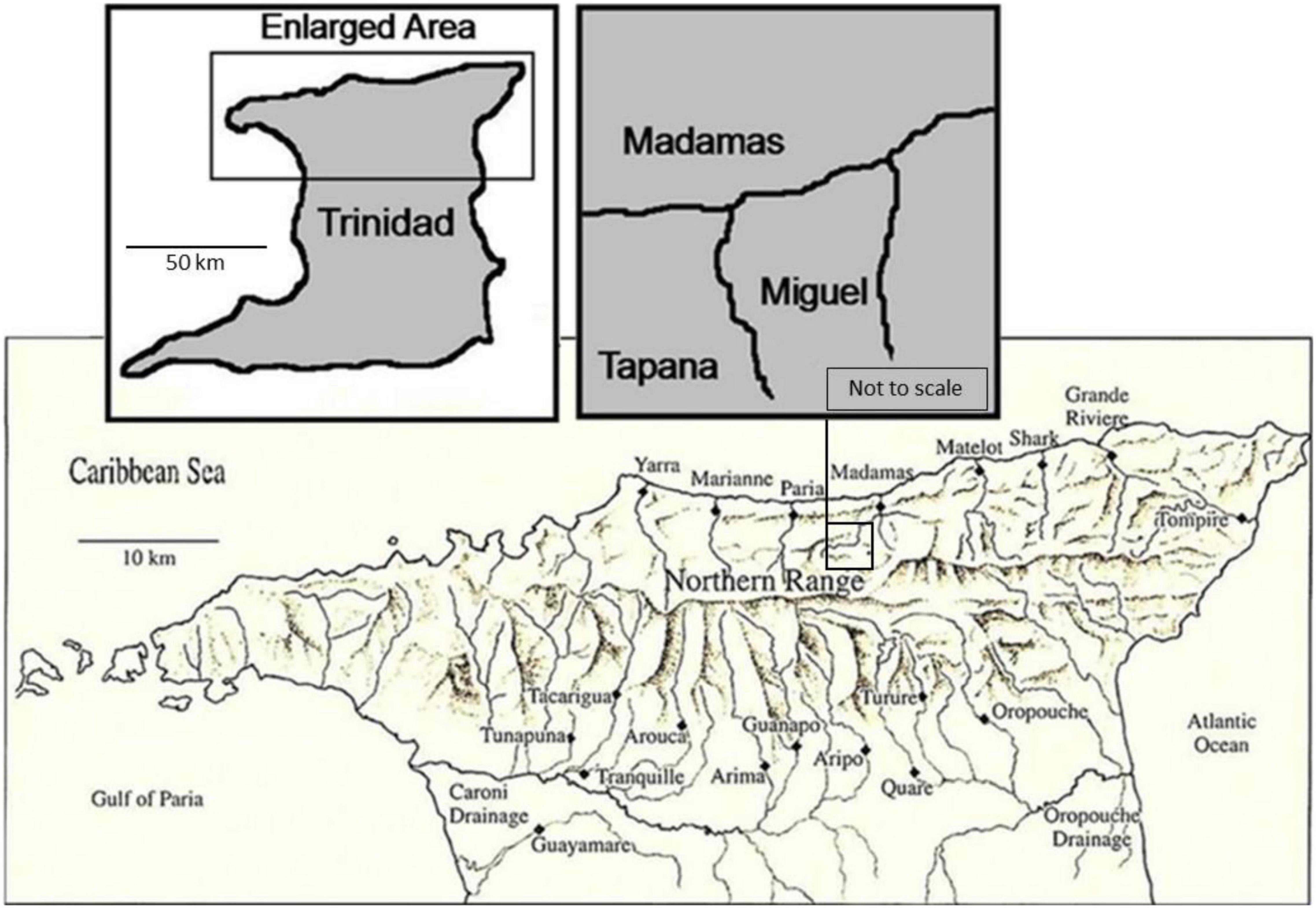
Figure 2. Map of sampled drainage in the Northern Range of Trinidad. Right insert includes details of Tapana and Miguel streams in the Madamas River Drainage.
The two study sites consisted of 150 m reaches, one at each stream. The Miguel site was characterized by secondary growth, including Heliconia, Wild Tobacco (Acnistus arborescens), Immortelle (Erythrina poeppigiana) and Banana (Musa sp.), and had been surrounded by an active plantation as recently as the 1950’s. The Tapana site was characterized by old growth forest with a dense canopy dominated by Wild Chataigne (Pachira insignis), Quasar Palm (Desmoncus major), Heliconia bihai, Hog Plum (Spondias mombin), Wild Cocoa (Licania biglandulosa), and Mountain Rose (Brownea latifolia).
The trees at both streams exhibit peak leaf fall at the end of the dry season (April) and typically bloom about a month after the onset of the wet season (June). Both sites exhibit a unimodal rainfall pattern, with elevated precipitation from June to December and less rainfall from January to May. The resident assemblages of aquatic invertebrates for both streams were dominated by insects of the orders Diptera, Ephemeroptera, Trichoptera, and Coleoptera, though Odonata, Plecoptera, and occasional representative of the Megaloptera were also observed (Owens, 2010). Rivulus were the dominant invertebrate consumer in each study stream.
Canopy Cover and Precipitation
The trees above each terrestrial invertebrate pan trap (description below) were identified to the lowest taxonomic level possible, and the density of the canopy above each pan trap was estimated using a convex densiometer (Lemmon, 1956). Four densiometer readings were taken above each pan trap: once facing upstream, once downstream, and once facing left and right. Readings were averaged and multiplied by 1.04 to determine the percent open canopy above each trap. Subtracting that number from 100 yielded the percent canopy density. Canopy density readings were repeated several times during both wet and dry seasons. Canopy density over pans at the Miguel stream ranged from 52 to 87% canopy cover, whereas the canopy density over pans hanging at the Tapana stream ranged from 88 to 97% canopy cover (Supplementary Figure 2). Based on these differences, we refer to the Miguel and Tapana sites as “open” and “closed,” respectively, while recognizing that the “open” site still has considerable canopy cover. Precipitation was quantified using a rain gauge (Tenite Metric) mounted ∼1 m above the ground service in a relatively open canopy area near the center of each study reach. Precipitation was recorded for all days over which pan traps collected samples (described below).
Invertebrate Sampling
Falling Input
Daily inputs of terrestrial invertebrates were estimated from samples collected from the pan traps at roughly the same time each day for 3 weeks each season. The time of day was noted each time a pan was emptied and all values were scaled accordingly to express fall in data as a daily rate (24 h). In each stream, 11 pan traps were deployed in spring (dry) and summer (wet) during the study period (one pan from Miguel was lost in the wet season). The pan traps had an area of 70.6 cm2 and were hung ∼0.5 m above the channel 10–15 m apart along the length of each study reach. Traps were hung using lines attached to each corner of the pan and extending to four trees in the riparian zone. Pan traps were filled with 2.5–5 cm of water and two to three drops of a phosphorus-free soap (Liqui-nox, SPI Supplies, West Chester, PA, United States). Though the surfactant used in the pan traps can potentially attract insects (Southwood, 1966; Edwards and Huryn, 1995), this method has been widely used to quantify the terrestrial invertebrate input into streams (e.g., Mason and MacDonald, 1982; Cloe and Garman, 1996; Wipfli, 1997; Nakano et al., 1999b,c; Kawaguchi and Nakano, 2001). Due to their design and location, these traps only measure terrestrial invertebrates entering from above and do not capture invertebrate subsidies delivered laterally across the stream bank. Pan contents and all other invertebrate samples were immediately preserved in 70% ethanol solution with Rose Bengal dye added to aid processing.
Benthic Invertebrates
Density and composition of benthic invertebrates were measured to assess changes in aquatic invertebrate prey resources for fish between the wet and dry seasons. A mini-Hess sampler (area = 0.032 m2, Wildco, Yulee, FL, United States) was used to collect benthic invertebrates from the stream bed 3 days prior to Rivulus gut content collection. Samples were collected using a random-stratified approach in which each stream reach was divided into pool and riffle habitats, with four samples collected randomly from each habitat type.
Drifting Invertebrates
Drifting invertebrates were collected twice during each of the wet and dry seasons at each site (n = 8) to assess seasonal changes in the composition and density of drifting invertebrates. Drifting invertebrates were collected from 18:00 to 21:00 to capture peak drifting rates (Tanaka, 1960; Waters, 1962; Elliot, 1969; Flecker and Feifarek, 1994; Allan, 1995). Stream drift was collected using 250-μm mesh drift nets (45 cm × 25 cm, 0.1 m2 opening, 1 m net length; Aquatic Research Instruments, Hope, ID, United States) that extended above the water surface to ensure that floating terrestrial invertebrates were captured. During the collection period, one net was placed at the bottom of each reach and was switched out each hour and emptied. The water volume sampled was estimated from depth and current velocity measurements taken at the left, center and right side of each net opening at mid-depth using a portable current meter (Marsh-McBirney Flo-Mate Model 2000 Portable Flowmeter, Hach Inc., Loveland, CO, United States). Discharge was measured at the downstream end of the study reach after each drift survey using the United States Geological Survey mid-section method (Orth, 1983). Flow and depth data were used to express the density of invertebrate drift as a concentration (mg m–3). Importantly, we chose to collect and report drifting invertebrates to provide snapshots in time of prey available to Rivulus, serving as an additional means by which to investigate seasonality of terrestrial and aquatic invertebrate availability. Although drift can vary immensely (Baxter et al., 2017), and these snapshots in time may not be entirely representative of the seasonal profile of drifting invertebrates, they were intended to provide for informative comparison with falling invertebrate input, which may include terrestrials that enter streams through other means than falling (e.g., lateral wash in). However, because Rivulus are often found in stream margins in areas of low velocity where they pick prey from the bottom, water column and its surface (Fraser et al., 1999), the seasonal availability of drifting terrestrial and aquatic invertebrates in the channel may not be as closely tied to Rivulus’ diets as it can be for fishes like salmonids of temperature streams that often focus on feeding from this source.
Invertebrate Identification
Invertebrates collected from pan traps and drift were sorted using a dissecting stereomicroscope (Motic Instruments K Series, Motic Inc., Richmond, BC, Canada) into the following groups: winged terrestrial insects, ants, non-winged terrestrials (i.e., larvae, spiders) and aquatic invertebrates. We chose these categories because of hypothesized differences between invertebrates with and without wings, and also because of the hypothesized importance of ants to Rivulus diets (Fraser et al., 1999). Terrestrial invertebrates were identified to order (Borror et al., 2005) and benthic invertebrates were identified to family (McCafferty, 1998; Merritt et al., 2008). Once sorted, invertebrates were dried at 58°C and weighed by category to the nearest 0.001 mg, and masses were converted to mg m–2 day–1 and mg m–3 for benthic and drift samples, respectively.
Fish Diet Survey and Analysis
Rivulus were sampled from the Miguel and Tapana streams during both seasons on the final evening of falling terrestrial input collection (2 days after final drift collection) to estimate seasonal variation in prey consumption. Rivulus is the sole fish and dominant consumer of invertebrates in each stream. The common name of this fish in Trinidad is the “jumping guabine” because it often leaves the water and “jumps” over land in order to colonize isolated aquatic habitats, and individuals can leap up to 14 cm above the water surface to reach or dislodge terrestrial prey from overhanging vegetation (Seghers, 1978; Fraser et al., 1999). Rivulus are known to feed on invertebrates and Poecilia (Gilliam et al., 1993), though Poecilia were not observed in the two streams under study nor were they found in any Rivulus gut analyses.
Rivulus are nocturnal feeders, and were caught after dark (21:00–23:00) by dip netting. During each season, 30 fish were sampled from each site, with 10 individuals collected from the bottom, middle, and upper 50 m sections of each stream reach, respectively. Only fish >40 mm in length were collected, thus our results do not reflect the diets of young fish. Collected fish were euthanized immediately by placing them on ice to stop digestion, then preserved in 10% formalin solution.
Our method for fish diet analysis was modified from Fraser et al. (1999). Fish length (from tip of snout to the tip of caudal fin) and wet weight were measured. Fish were then gutted, dried, and reweighed. Fish length ranged from 4 to 8.6 cm and dry weight from 0.11 to 1.45 g. To ensure the fish representing each stream by season group were similar in sex, length and weight, Rivulus were sorted into groups that each consisted of one fish from each of the Miguel dry season, Miguel wet season, Tapana dry season, and Tapana wet season that were similar in sex, length and weight, resulting in a total of 16 groups (N = 64) of fish to be analyzed. All materials were removed from the gut. Each prey item was identified to the lowest possible taxonomic level and labeled aquatic, terrestrial, unknown or detritus. Body length, width, and head width of each invertebrate was measured when applicable. Insect widths and lengths were converted to cylinders using the formula [π × (W/2)2 × L] to determine the volume represented by each invertebrate taxon. Body parts and detritus were uniformly layered 1 mm deep on a gridded dish and measured for length and width. The total volume of terrestrial or aquatic insects was determined by summing the volumes of all individuals and body parts. Invertebrates such as ants or chironomids have sclerotized head capsules or body parts which slowly decompose in fish guts. Therefore, these groups may be disproportionately represented in these analyses.
Statistical Analyses
The statistical analyses described below are organized by research questions. First we describe analyses determining seasonal variation in falling invertebrate biomass, benthic invertebrate biomass, and drifting invertebrate biomass, as well as in the volume of invertebrates comprising Rivulus guts. Then, we report results of analyses to determine the effects of canopy density and precipitation on falling invertebrate biomass. Biomass data were log-transformed for all of these tests. All statistical analyses were conducted using the SAS mixed, correlation and regression procedures (SAS 9.4, SAS Institute, Cary, NC, United States). Because p values describe a continuous measure of evidence and are influenced by small sample size (Gelman, 2013), we used a graded, weight of evidence approach to describe our certainty that results differed from what would be expected by chance alone (Wasserstein et al., 2019). Based upon this rationale, for all statistical tests we considered p values < 0.05 significant and strong evidence for an effect, and those between 0.05 and 0.1 as providing weak evidence for an effect that may have ecological meaning given the low sample size and statistical power of this study.
To determine the effects of canopy density and precipitation on falling invertebrate biomass, analysis of covariance was performed for each of the two streams with canopy density and precipitation as quantitative independent variables and pan as a categorical independent variable.
We used an analysis of variance (ANOVA) and mean comparisons to analyze effects of season, stream and their interaction on the biomass of total falling invertebrates into streams, as well as the three biomass subcategories that comprised it: winged terrestrial invertebrates, ants, and invertebrates that were neither winged nor ants that fell into the Miguel and Tapana streams. Season and stream were categorical variables. A total of 44 average values of daily input were included, 8 dates from the Miguel and 12 from the Tapana stream during the dry season, and 12 from each of the Miguel and Tapana streams during the wet season. As such, an average value for rain at each site each day was assigned to each of the 44 average daily values.
We also tested for categorical effects associated with stream and season on benthic invertebrate biomass in the Miguel and Tapana streams using ANOVA and mean comparisons. The ANOVA model accounted for season as a repeated measures factor, with habitat and stream as crossed factors and sampling location within each stream site nested in a habitat-site combination. Drifting invertebrate biomass were tested for categorical stream and season effects in these streams using a 2-factor ANOVA with interactions.
Lastly, we tested for categorical stream and season effects on the amounts and percentages of aquatic and terrestrial invertebrates in the guts of the 64 Rivulus from the Miguel and Tapana streams, again using a 2-factor ANOVA. The length of Rivulus was initially tested as a continuous variable in the models in case there were significant ontogenetic effects on their diet, but length was removed from the final analyses since we detected no evidence that its effect was significant among the fish we sampled.
Results
The results reported below are organized as follows. First, we report on the effect of seasonality, precipitation, and canopy density on falling invertebrate biomass. Next, we report seasonal effects on benthic and drifting invertebrate biomass. Finally, we report seasonal effects on invertebrates comprising the guts of Rivulus.
Falling Invertebrates
The total biomass of invertebrates falling into the two streams was 53% greater, on average, during the wet season compared to the dry season (p = 0.02, Figure 3 and Supplementary Table 1). This was principally driven by the input of ants to each stream, which was more than four times greater during the wet season than the dry (p < 0.001). We observed no seasonal differences in the fall-in rates for winged invertebrates (p = 0.70) or non-winged, non-ant invertebrates (p = 0.45). Additionally, we observed no differences in any of the biomass categories of falling input between the two streams (Figure 3) and no evidence of a stream by season interaction.
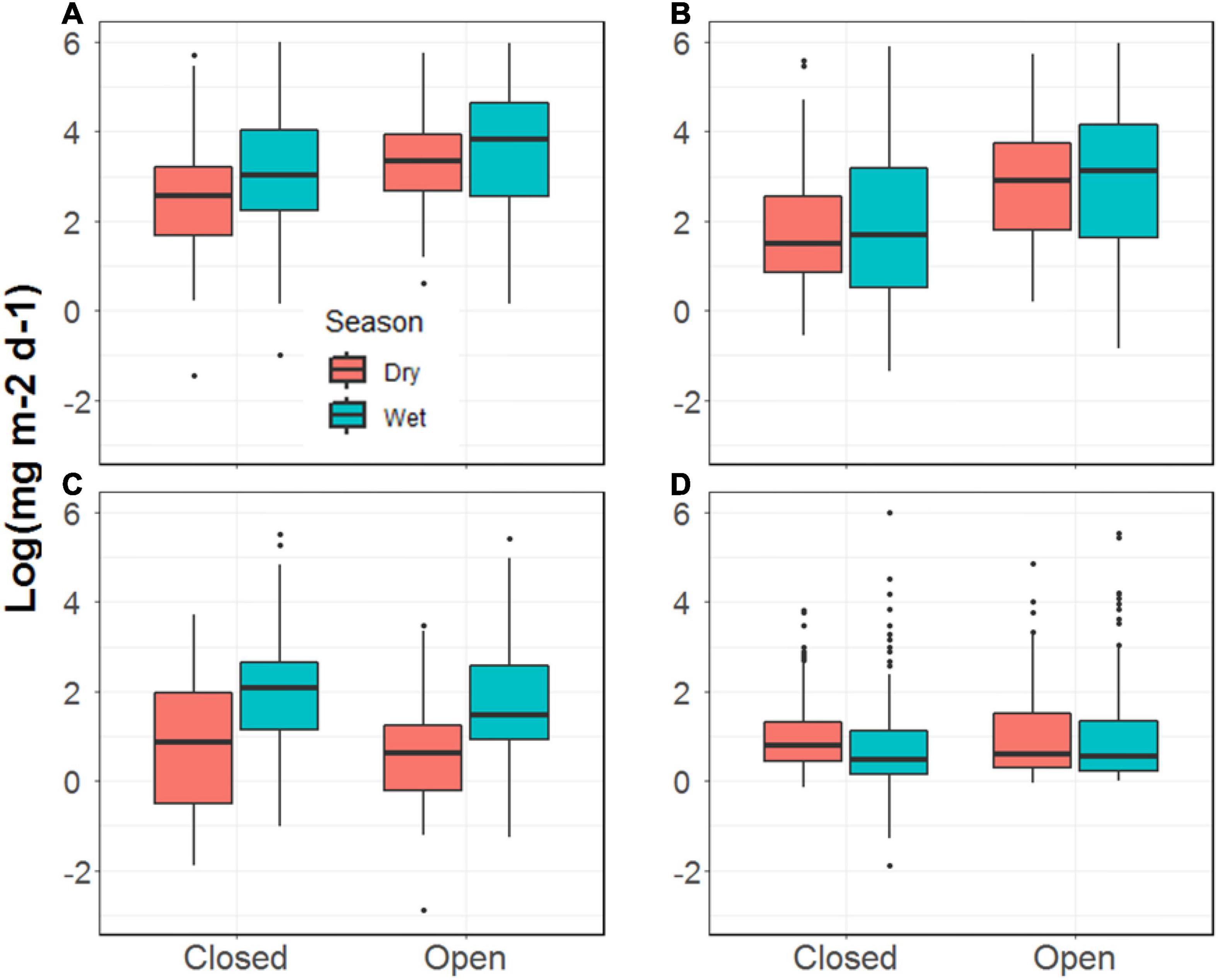
Figure 3. Invertebrate biomass falling into the Tapana (Closed) and Miguel (Open) streams, including (A) total mass; (B) flying mass; (C) ant mass; (D) other mass.
On the Miguel, the more open-canopied stream, we observed strong evidence for a positive relationship between precipitation and ant biomass falling into the stream (p = 0.002; Table 2), but not for total, winged, or other invertebrates. On the Tapana, the more closed-canopied stream, there was evidence for a positive relationship between precipitation and inputs of winged invertebrates (p = 0.03) and non-winged, non-ant invertebrates (p = 0.06), but not for total invertebrates or ants. Within each stream, we found strong evidence of a positive relationship between canopy density above a trap location and ant biomass falling into the stream (Miguel p = 0.02 and Tapana p < 0.001; Table 3). We found weak evidence in support of a positive relationship between canopy density above traps and total invertebrate biomass falling into traps in the Tapana (p = 0.10), but detected no relationship between canopy density above traps and biomass of winged-invertebrates falling into these locations across habitats in either stream.
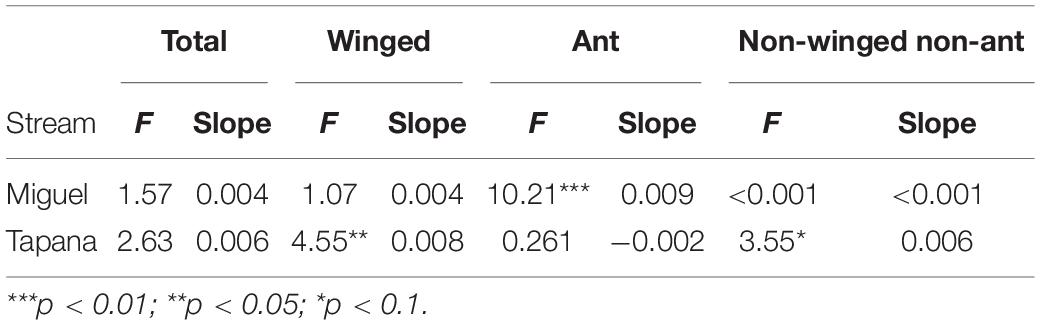
Table 2. Relationship between precipitation and invertebrate biomass falling into the Miguel and Tapana streams.
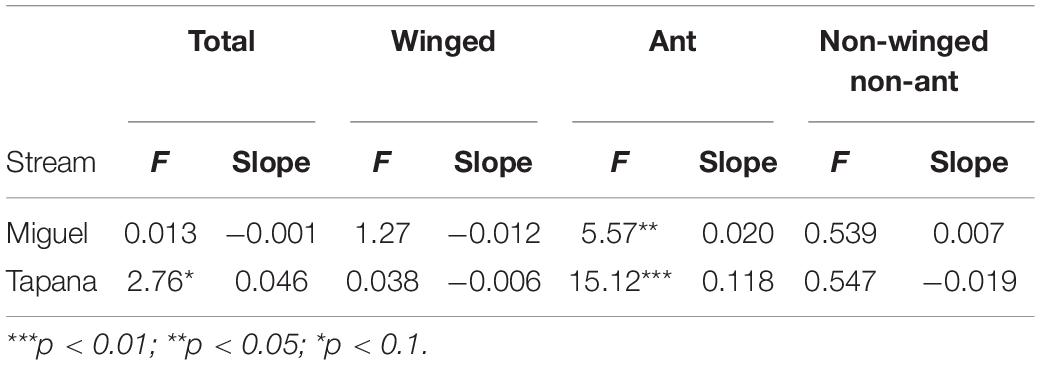
Table 3. Relationship between canopy density and invertebrate biomass falling into the Miguel and Tapana streams.
Benthic Invertebrates
The biomass of benthic invertebrates in the dry season (M = 382.22 mg m–2, SE = 92.45) was nearly triple that observed in the wet season (M = 129.65 mg m–2, SE = 31.32; p = 0.04; Figure 4) but did not differ between streams or habitat types (pools and riffles), nor did we detect any interactions among season, stream, or habitat.
Drifting Invertebrates
Terrestrial invertebrate biomass collected in the drift during the wet season was more than double that observed in the dry season (p = 0.01, Supplementary Table 2). The overall seasonal difference in drifting biomass was driven largely by winged invertebrates (p = 0.01) and ants (p = 0.01), which were much more common in the drift during the wet season. We detected no seasonal differences for total, aquatic, or non-winged, non-ant invertebrate biomass in the drift. The Miguel site had ∼2.5 times the biomass of ants in the drift (M = 0.07 mg m–3 hr–1, SE = 0.03) than did the Tapana (M = 0.03 mg m–3 hr–1, SE = 0.01; p = 0.04). We observed little difference between the two streams for any of the other categories of drifting invertebrates, nor was there evidence for an interaction between stream and season.
Invertebrates Comprising Rivulus Gut Contents
We found the total volume of invertebrates in Rivulus guts to be ∼60% greater during the dry versus the wet season (p = 0.07, Figure 5). We also found fifteen times the volume of aquatic invertebrates in Rivulus guts during the dry season than the wet (p < 0.001). There was also 45% more detritus in Rivulus guts during the dry season than during the wet season (p = 0.01, Figure 6 and Supplementary Table 3). In contrast, the volume of ants found in Rivulus guts was nearly 50% greater during the wet than the dry season (p = 0.03), though we observed no seasonal differences in total invertebrate or total terrestrial invertebrate volume in Rivulus guts. Nearly twice the volume of aquatic invertebrates was found in the guts of Rivulus from the more open-canopied Miguel (M = 7.87, SE = 2.95) than the Tapana (M = 4.37, SE = 3.26; p = 0.04) stream. No other differences were detected in Rivulus gut contents by stream, nor was there any evidence of stream × season interactions.
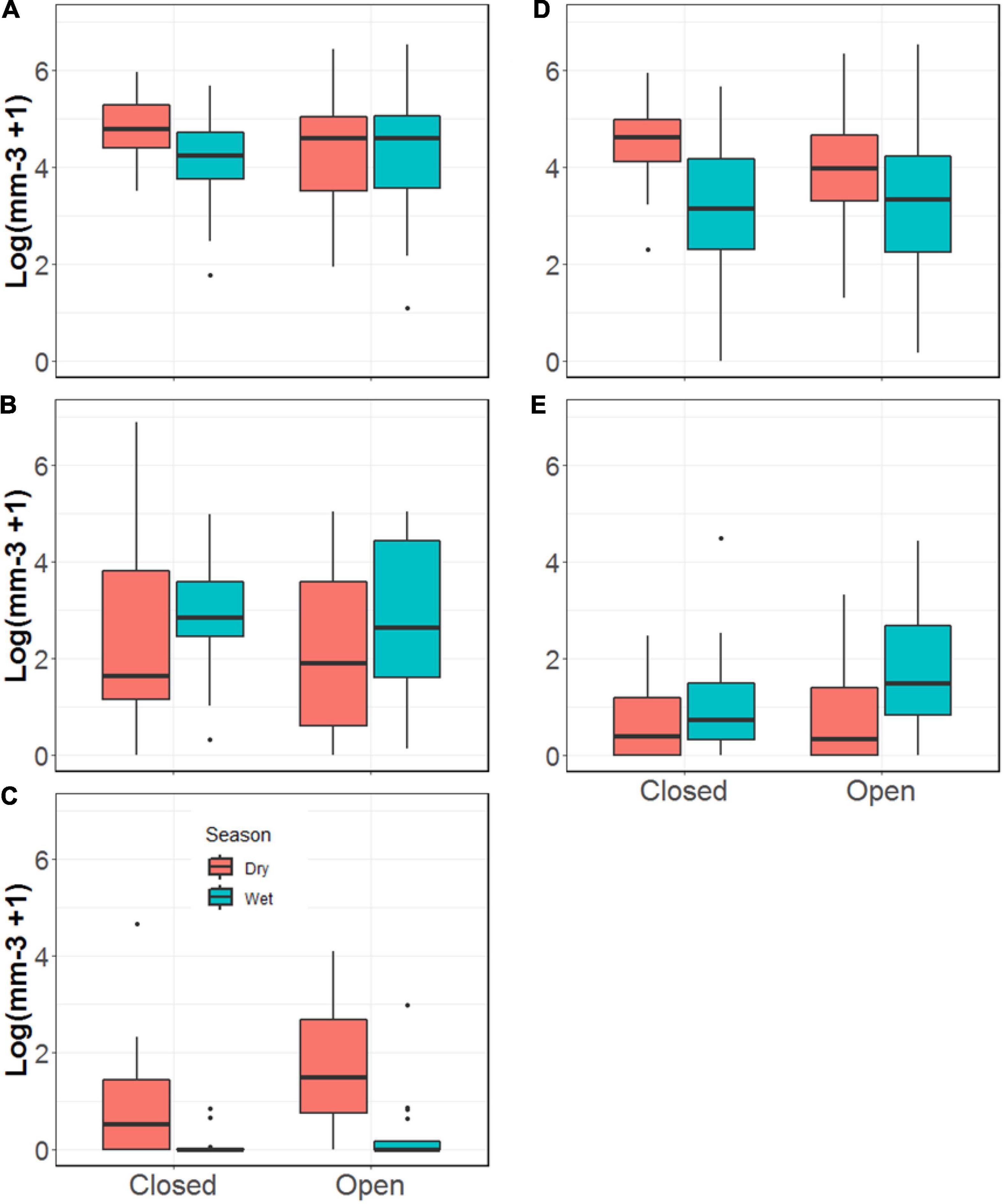
Figure 5. Volume of invertebrates and detritus found in the guts of Rivulus from the Tapana (Closed) and Miguel (Open) streams, including (A) total; (B) terrestrial; (C) aquatic; (D) detritus volume; and (E) ants.
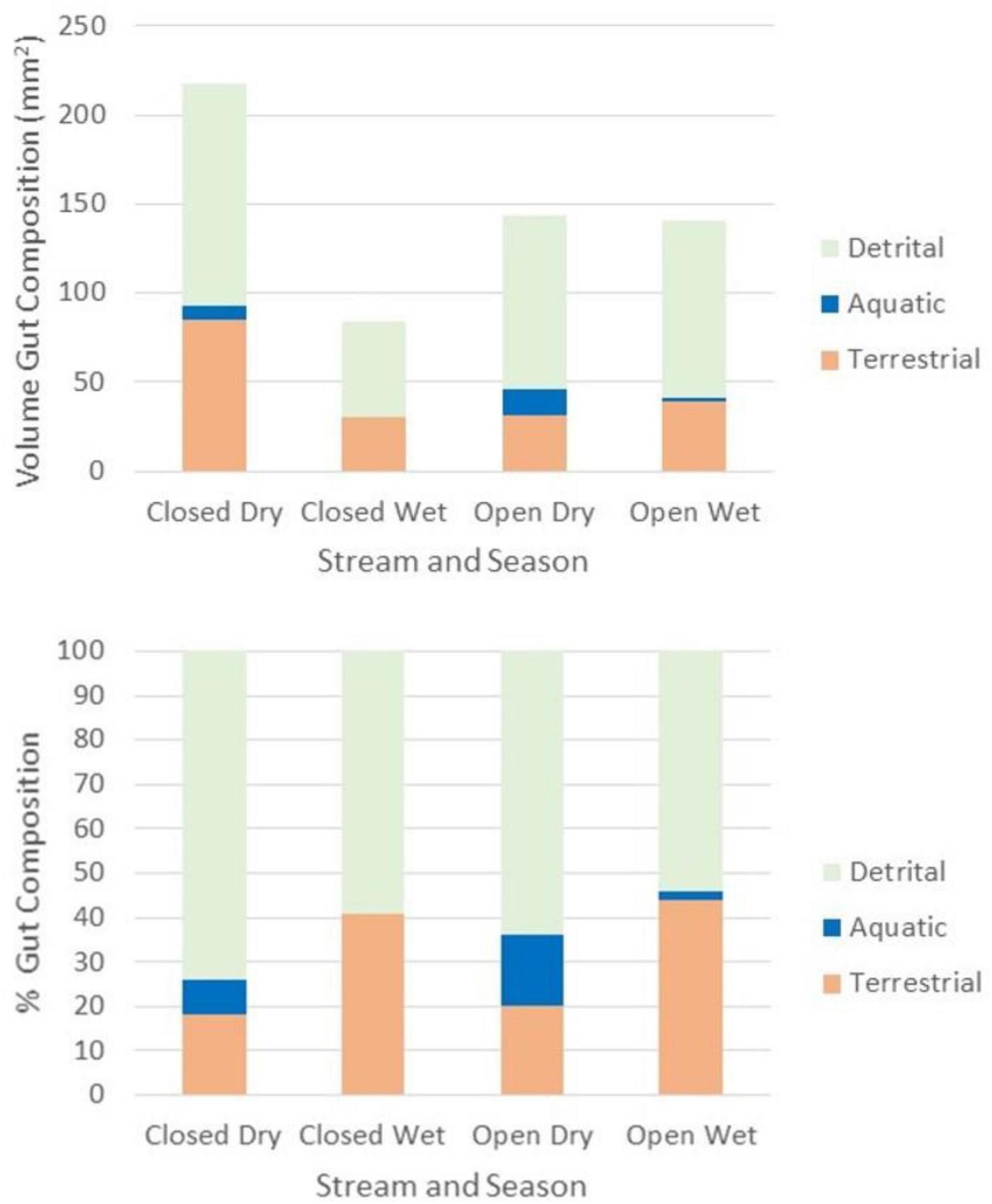
Figure 6. Volume (mm2) and percent composition of invertebrates comprising Rivulus guts by stream and season.
We also found the proportional representation of invertebrates comprising Rivulus guts to vary by season. Whereas the % volume of terrestrial invertebrates generally (p = 0.002), as well as ants specifically (p = 0.006), comprising Rivulus gut contents was greater in the wet season as compared to the dry, the % of aquatic invertebrates (p < 0.001) and detritus comprising Rivulus gut contents was greater in the dry season (p < 0.10). Additionally, we observed a greater % volume of aquatic invertebrates in Rivulus guts collected from the Miguel stream as compared to the Tapana (p = 0.07). The volume of the terrestrial and benthic invertebrates composing Rivulus guts by stream and season are represented in Figure 1.
Ants were by far the most abundant invertebrate present in Rivulus guts, though by volume they represented a small percentage of terrestrial invertebrates therein. Other terrestrials often present in Rivulus guts included coleopterans, dipterans, non-ant hymenopterans, and termites, as well as the occasional diplopod or spider. In terms of aquatic invertebrates represented in Rivulus guts, ephemeropterans were the most common, though chironomids, other dipterans, hydropsychid trichopterans, odonates, and hemipterans were also frequently present.
Discussion
Our findings indicate that terrestrial invertebrate inputs to tropical streams like those we studied can play an important role in sustaining fishes like the Rivulus populations in our investigation, and that precipitation-driven seasonality in the tropics, like its temperate counterpart, may have implications for the relative and absolute contributions of both terrestrial and aquatic invertebrates as prey for stream fishes. However, whereas seasonality in temperate regions has long been shown to drive dramatic dynamics in the input of terrestrial invertebrates to streams, resulting in changes in prey abundance, composition and use by fishes (Kawaguchi and Nakano, 2001; Nakano and Murakami, 2001; Romaniszyn et al., 2007; Rundio and Lindley, 2008), this study is among the first to quantify year-round variation in terrestrial invertebrate subsidies to tropical streams and its contribution to diets of fish relative to aquatic-derived invertebrate prey in such streams. Below, we discuss seasonal variation in the availability of terrestrial and aquatic invertebrates for Rivulus, including falling invertebrates (and mediating factors of canopy and precipitation), benthic, and drifting invertebrates, before considering how variation in the prey found in Rivulus’ guts reflects seasonal differences in availability. While bearing in mind the limited scope of our study we also evaluate the more general possibility, consistent with our findings, that tropical and temperate stream-riparian ecosystems may strongly differ in the manner in which seasonality influences terrestrial invertebrate subsidies and their impacts on stream fishes and food webs.
Factors Influencing the Input of Falling Invertebrates
We found seasonality to have large implications for the delivery of terrestrial invertebrates to the tropical streams we studied. In these Trinidadian streams, the daily biomass of terrestrial invertebrates falling into the stream increased ∼50% from the dry season to the wet season, with falling ant input increasing more than 300%. The seasonal variation in falling invertebrate input is likely associated with reduced overall abundance commonly observed in tropical forests during the dry season (Erwin and Scott, 1980; Smythe, 1982; Broadhead, 1983; Kato et al., 1995; Novotny and Basset, 1998; Ades and Dudgeon, 1999), similar to reduced abundance of terrestrial invertebrates observed during the winter in temperate regions. Indeed, our findings are consistent with the few studies of resource subsidy seasonality in tropical streams. For example, Angermeier and Karr (1983) reported preliminary evidence from Panamanian streams that terrestrial arthropod inputs differed between wet and dry seasons, and Chan et al. (2008), whose study of Hong Kong streams is likely the most rigorous to date, found that dry-season biomass of terrestrial subsidies was approximately one-third of that occurring in the wet season. We observed slightly diminished effects of seasonality in our streams, with 50% greater biomass of falling inputs in the wet vs. dry seasons and 140% greater biomass of terrestrial invertebrates in the drift during the wet season. It is also worth noting that the seasonal variation in falling invertebrate biomass observed in this study was much less than seasonal differences reported for forested streams in temperate regions, where terrestrial invertebrate input to streams approaches zero during the winter season (Baxter et al., 2005).
Although we did not observe strong differences in falling invertebrate input between the more open vs. more closed-canopy streams, we did find that falling invertebrate input was mediated by the canopy density above collection traps and by precipitation events. For example, increasing canopy density at a sampling location was associated with higher total invertebrate input only in the more closed-canopy stream. On the other hand, we detected a positive relationship between canopy density and the input of ants at both streams. Chan et al. (2008) reported similar results from streams whose riparian vegetation differed much more starkly than our comparison. They found that fall-in rates of terrestrial arthropod biomass were three times higher in forested streams compared to a shrubland stream in Hong Kong. Such findings likely reflect the greater number of terrestrial invertebrates housed in dense canopies and their potential to fall into adjacent streams (Bates, 1944; Wilson, 1959; Sutton et al., 1983; Longino and Nadkarni, 1990).
We also found a positive relationship between precipitation events and invertebrate subsidies to these tropical streams, though this relationship was complex and differed between the two study streams. For example, precipitation events significantly increased ant inputs in the more open canopy site but had no detectable effect on delivery of other invertebrates to this stream. In contrast, rain events increased inputs of winged and non-winged inverts in our denser canopy site but had relatively little effect on the delivery rates of ants to this stream. This study was not designed to explore the specific mechanisms responsible for invertebrate dislodgement and delivery to streams, which may reflect complex relationships between vegetation composition, terrestrial arthropod assemblages, and even variation in factors such as wind (Baxter et al., 2005), but our results suggest such studies are warranted.
Seasonal Availability of Terrestrial and Aquatic Invertebrates
Like their terrestrial counterparts, we observed strong seasonality in benthic aquatic invertebrate availability in these tropical streams, though these fluctuations differed from those patterns commonly observed for many temperate streams. Whereas terrestrial invertebrate input to our study streams, and thus availability of these prey for Rivulus, peaked during the wet season, benthic invertebrate biomass all but disappeared in the wet season. Low and consistent flows combine with peaks in leaf fall and canopy openness to promote the growth in benthic invertebrate populations in the dry season, whereas frequent high flow events typical of the wet season reduce leaf and biofilm standing stocks and dislodge substrate and associated invertebrates (Resh et al., 1988; Flecker and Feifarek, 1994; Poff et al., 1997). Importantly, though we found terrestrial invertebrate subsidies to be higher in the wet season, they remained available year round (Figure 6) and as such, were an important diet item for Rivulus throughout the year. In contrast, aquatic invertebrate availability was extremely low during the wet season compared to the dry season. This pattern was even more pronounced in the Rivulus gut contents. Whereas terrestrial invertebrates were well represented in Rivulus diets year round, aquatic invertebrates were only a substantial fraction of Rivulus gut contents during the dry season and all but disappeared from their guts in the wet season. These observations regarding the importance of terrestrial invertebrates to Rivulus diets are consistent with food web studies using stable isotopes in similar Trinidadian streams, which concluded that Rivulus derives approximately 50% of their assimilated resources from terrestrial sources (Collins et al., 2016).
Terrestrial invertebrates fall into the stream where they mix in the water column with aquatic invertebrates that either intentionally or accidentally left the benthos to drift downstream. This invertebrate “drift” is an important dietary source for many fishes but any importance it may have for Rivulus diets remains unexplored. Despite stark seasonal differences in benthic aquatic invertebrate biomass, we did not detect seasonal differences in the biomass of aquatic prey in the drift. Given that benthic invertebrates comprising Rivulus guts disappeared in the wet season, it does not appear that drifting aquatic invertebrates during the wet season were an important food resource for Rivulus. In contrast to aquatic invertebrate availability in the wet season, terrestrial prey biomass observed in the drift increased in the wet season by 140%, but we did not observe a concurrent increase in total terrestrial invertebrates comprising Rivulus guts during the wet season. Although observations of Rivulus feeding from the drift have been made (B. Lamphere, personal communication, July 7, 2021; E. Zandona, personal communication, August 20, 2021), these findings suggest that drift feeding may not be an important feeding strategy for Rivulus in these streams. However, the 300% increase in drifting ants observed during the wet season does align with the 300% increase in ants observed in Rivulus gut in the wet season. Whether those ants were consumed from the drift, or were eaten after falling into the puddled waters on stream margins (which Rivulus are known to frequent), is not clear. In any case, measurements of drift are notoriously “noisy” (i.e., few measurements of a phenomenon that is highly variable in time; Baxter et al., 2017). Additionally, our observations of Rivulus at the two focal streams suggest they frequent pools and stream margins and rarely occupy the free flowing water column where the drift was collected. Thus, though they raise interesting questions and may aid in interpretation of our other findings, our estimates of drift may not have been reliably representative of prey availability to Rivulus.
The phenological timing of the availability and use of terrestrial prey subsidies relative to in situ resources by fish are thought to mediate the broader food web and ecosystem effects of such subsidies (e.g., Takimoto et al., 2009; Marcarelli et al., 2020; Takimoto and Sato, 2020; Yang, 2020) and our observations appear consistent with these expectations. Interestingly, the wet-season increases we observed in terrestrial invertebrate prey subsidies to these tropical streams occurred at precisely the time when aquatic benthic invertebrate availability to stream fish was at its lowest. Some temperate stream settings experience a similar phenomenon in terms of asynchrony in the timing of the delivery of terrestrial invertebrate subsidies relative to availability of in situ aquatic prey for fishes. For example, for a northern Japan stream, Nakano and Murakami (2001) showed that terrestrial invertebrate input was lowest during winter when aquatic invertebrate biomass was on the rise, whereas terrestrial prey inputs peaked in late summer/early fall when aquatic invertebrate prey availability for stream fish was on the decline. Yet, this pattern may not apply to all temperature streams. For instance, Rundio and Lindley (2008, 2012) observed more synchrony in aquatic and terrestrially derived prey resources, and suggested terrestrially derived invertebrates provided a more consistent, year-round subsidy to the salmonid fishes in California streams under a Mediterranean-type climate. The Trinidadian streams we studied may represent a kind of hybrid of these, in that our findings suggest that substantial terrestrial prey input occurs year-round, but that the peaks in the two prey sources are asynchronous such that the greatest terrestrial subsidy occurs when aquatic prey resources are at their lowest. The duration and dynamics of this prey subsidy point to the potential for it to sustain higher populations of Rivulus in these streams than would occur without it, a hypothesis that remains to be tested. If there were such demographic consequences (i.e., not just shifts in feeding behavior), then, in turn, this could lead to stronger top-down effects of fishes like Rivulus on in situ benthic invertebrates with attendant implications for stream organic matter dynamics (as has been demonstrated in some temperate stream ecosystems; e.g., Marcarelli et al., 2020), possibilities that deserve investigation. In any case, just as considerable variation in terrestrial invertebrate subsidies have been described among different temperate stream settings, the patterns we observed for these Trinidadian streams may or may not be generalizable to other tropical contexts, and there remains a need for study of these phenomena across more tropical settings.
Conclusion
We found that terrestrial invertebrate subsidies can be a significant year-round food resource for supporting fish populations in these Trinidadian streams, suggesting they may be an important ecological driver in other tropical stream food webs. Similar research in temperate zones has documented how seasonality controls the timing and magnitude of terrestrial subsidies delivered to streams and its importance to stream food webs relative to benthic invertebrate prey (Kawaguchi and Nakano, 2001; Nakano and Murakami, 2001; Romaniszyn et al., 2007). In temperate streams, consumption of terrestrial invertebrates by fish typically increases in the summer when falling input peaks and is relatively minor during other times of the year (Kawaguchi and Nakano, 2001). We observed a very different condition in Trinidad. In our focal streams, Rivulus responded more strongly to seasonal variation in benthic invertebrate abundance than to changes in falling input of terrestrial prey items. Falling terrestrial input was significantly greater in the wet season compared to dry season input, but these subsidies were relatively high year round. In contrast, benthic invertebrate populations varied dramatically between wet and dry seasons such that aquatic invertebrate consumption was negligible in the wet season. As a result, our tropical stream sites display a pattern that is the inverse of what has been observed in temperate streams. Whereas terrestrial invertebrate input subsidizes fish diets in temperate streams, benthic invertebrate production appears to “subsidize” a more continuous supply of terrestrial invertebrate prey in the tropical sites we studied. Though limited in scope, our findings suggest that the flow of prey from the riparian zone to the stream may be just as important, perhaps even more so, for the trophic ecology of animals in tropical streams as it is for temperate ecosystems, but that seasonal patterns in the relative importance of terrestrial vs. aquatic prey are likely to differ between streams in tropical versus temperate latitudes.
Data Availability Statement
The original contributions presented in the study are included in the article/Supplementary Material, further inquiries can be directed to the corresponding author.
Ethics Statement
The animal study was reviewed and approved by the UC Riverside Institutional Animal Care and Use Committee (IACUC).
Author Contributions
DO and ST conceived the project. DO collected the samples and prepared the data. KE, TH, and DO analyzed the data. DO, TH, CVB, and ST wrote the manuscript. All authors read and approved the final version of the manuscript.
Funding
Our research and preparation of this manuscript has been supported by the National Science Foundation (DEB-0623632 to David N. Reznick and DEB-1754224 to CVB).
Conflict of Interest
The authors declare that the research was conducted in the absence of any commercial or financial relationships that could be construed as a potential conflict of interest.
Publisher’s Note
All claims expressed in this article are solely those of the authors and do not necessarily represent those of their affiliated organizations, or those of the publisher, the editors and the reviewers. Any product that may be evaluated in this article, or claim that may be made by its manufacturer, is not guaranteed or endorsed by the publisher.
Acknowledgments
We appreciate David N. Reznick and Alex Flecker for guidance and input on research design, Carl Fitzjames for his local area expertise and assistance with logistics and sampling, Brad Lamphere, Eugenia Zandona, and Andres Lopez Sepulcre for their input on Rivulus feeding strategies, and Cinnamon Dowd for shared modeling expertise.
Supplementary Material
The Supplementary Material for this article can be found online at: https://www.frontiersin.org/articles/10.3389/fevo.2022.788625/full#supplementary-material
References
Ades, G. A. W., and Dudgeon, D. (1999). Insect seasonality in Hong Kong:a monsoonal environment in the northern tropics. Mem. Hong Kong Nat. Hist. Soc. 22, 81–97.
Allan, J. D. (1995). Stream Ecology - Structure and Function of Running Waters. London: Chapman and Hall.
Allen, K. R. (1951). The Horokiwi Stream: a study of a trout population. Bull. New Zeal. Depart. Fish. 10, 1–231. doi: 10.1111/j.1095-8649.2009.02227.x
Angermeier, P. L., and Karr, J. R. (1983). Fish communities along environmental gradients in a system of tropical streams. Environ. Biol. Fish. 9, 117–135.
Bates, M. (1944). Observations on the distribution of mosquitoes in a tropical forest. Ecology 25, 159–170. doi: 10.2307/1930689
Baxter, C. V., Fausch, K. D., Murakami, M., and Chapman, P. L. (2004). Fish invasion restructures stream and forest food webs by interrupting reciprocal prey subsidies. Ecology 85, 2656–2663. doi: 10.1890/04-138
Baxter, C. V., Fausch, K. D., Murakami, M., and Chapman, P. L. (2007). Invading rainbow trout usurp a terrestrial prey subsidy from native charr and reduce their growth and abundance. Oecologia 153, 461–470. doi: 10.1007/s00442-007-0743-x
Baxter, C. V., Fausch, K. D., and Saunders, W. C. (2005). Tangled webs: reciprocal flows of invertebrate prey link streams and riparian zones. Freshw. Biol. 50, 201–220. doi: 10.1111/j.1365-2427.2004.01328.x
Baxter, C. V., Kennedy, T. A., Miller, S. W., Muehlbauer, J. D., and Smock, L. A. (2017). “Macroinvertebrate drift, adult insect emergence and oviposition,” in Methods in Stream Ecology: Volume 1: Ecosystem Structure, eds F. R. Hauer and G. A. Lamberti (Cambridge, MA: Academic Press), 435–456. doi: 10.1016/b978-0-12-416558-8.00021-4
Behn, K. E., and Baxter, C. V. (2019). The trophic ecology of a desert river fish assemblage: influence of season and hydrologic variability. Ecosphere 10:e02583.
Bellmore, J. R., Baxter, C. V., Martens, K., and Connolly, P. J. (2013). The floodplain food web mosaic: a study of its importance to salmon and steelhead with implications for their recovery. Ecol. Appl. 23, 189–207. doi: 10.1890/12-0806.1
Borror, D. J., Triplehorn, C. A., and Johnson, N. F. (2005). Borror and DeLong’s Introduction to the Study of Insects. Baton Rouge, LA: Thompson Brooks.
Boulton, A. J., Peterson, C. G., Grimm, N. B., and Fisher, S. G. (1992). Stability of an aquatic macroinvertebrate community in a multiyear hydrologie disturbance regime. Ecology 73, 2192–2207. doi: 10.2307/1941467
Broadhead, E. (1983). “The assessment of faunal diversity and guild size in tropical forests with particular reference to Psocoptera,” in Tropical Rain Forest: Ecology and Management, eds S. L. Sutton, T. C. Whitmore, and A. C. Chadwick (Oxford: Blackwell), 107–120.
Chan, E. K., Zhang, Y., and Dudgeon, D. (2008). Arthropod ‘rain’into tropical streams: the importance of intact riparian forest and influences on fish diets. Mar. Freshw. Res. 59, 653–660. doi: 10.1071/mf07191
Cloe, W. W., and Garman, G. C. (1996). The energetic importance of terrestrial arthropod input to three warm-water streams. Freshw. Biol. 36, 105–114.
Collins, S. M., Kohler, T. J., Thomas, S. A., Fetzer, W. W., and Flecker, A. S. (2016). The importance of terrestrial subsidies in stream food webs varies along a stream size gradient. Oikos 125, 674–685. doi: 10.1111/oik.02713
Edwards, E. D., and Huryn, A. D. (1995). Annual contribution of terrestrial invertebrates to a New Zealand trout stream. N. Zeal. J. Mar. Freshw. Res. 29, 467–477. doi: 10.1080/00288330.1995.9516680
Elliot, J. M. (1969). Life history and biology of Sericostoma personatum Spence (Trichoptera). Oikos 20, 110–118.
Erwin, T. L., and Scott, J. C. (1980). Seasonal and size patterns, trophic structure, and richness of Coleoptera in the tropical arboreal ecosystem: the fauna of the tree Luehea seemannii Triana and Planch in the Canal Zone of Panama. Coleopterists’ Bull. 34, 305–322.
Flecker, A. S., and Feifarek, B. (1994). Disturbance and the temporal variability of invertebrate assemblages in two Andean streams. Freshw. Biol. 31, 131–142. doi: 10.1016/j.scitotenv.2020.144262
Fraser, D. F., Gilliam, J. F., MacGowan, M. P., Arcaro, C. M., and Guillozet, P. H. (1999). Habitat quality in a hostile river corridor. Ecology 80, 597–607.
Garman, G. C. (1991). Use of terrestrial arthropod prey by a stream-dwelling cyprinid fish. Environ. Biol. Fish. 30, 325–331. doi: 10.1007/bf02028848
Gelman, A. (2013). Commentary: P values and statistical practice. Epidemiology 24, 69–72. doi: 10.1097/ede.0b013e31827886f7
Gilliam, J. F., Fraser, D. F., and Alkins-Koo, M. (1993). Structure of a tropical stream fish community: a role for biotic interactions. Ecology 74, 1856–1870. doi: 10.1002/ecy.1616
Gounand, I., Harvey, E., Little, C. J., and Altermatt, F. (2018). Meta-ecosystems 2.0: rooting the theory into the field. Trends Ecol. Evol. 33, 36–46. doi: 10.1016/j.tree.2017.10.006
Hunt, R. L. (1975). “Use of terrestrial invertebrates as food by salmonids,” in Coupling of Land and Water Systems, ed. A. D. Hasler (New York, NY: Springer-Verlag), 137–152.
Huryn, A. D. (1996). An appraisal of the Allen paradox in a New Zealand trout stream. Limnol. Oceanogr. 41, 243–252. doi: 10.4319/lo.1996.41.2.0243
Hynes, H. B. N. (1975). The stream and its valley. Int. Vereinigung Theor. Angew. Limnol. Verhandlungen 19, 1–15.
Kato, M., Inoue, T., Hamid, A. A., Nagamitsu, T., Merdek, M. B., Nona, A. R., et al. (1995). Seasonality and vertical structure of light-attracted insect communities in a dipterocarp forest in Sarawak. Popul. Ecol. 37, 59–79. doi: 10.1007/bf02515762
Kawaguchi, Y., and Nakano, S. (2001). Contribution of terrestrial invertebrates to the annual resource budget for salmonids in forest and grassland reaches of a headwater stream. Freshw. Biol. 46, 303–316. doi: 10.1046/j.1365-2427.2001.00667.x
Kawaguchi, Y., Taniguchi, Y., and Nakano, S. (2003). Terrestrial invertebrate inputs determine the local abundance of stream fishes in a forested stream. Ecology 84, 701–708. doi: 10.1890/0012-9658(2003)084[0701:tiidtl]2.0.co;2
Lemmon, P. E. (1956). A spherical densiometer for estimating forest overstory density. For. Sci. 2, 314–320.
Longino, J. T., and Nadkarni, N. (1990). A comparison of ground ants and canopy leaf litter ants (Hymenoptera: Formicidae in a neotropical montane forest). Psyche 97, 81–93. doi: 10.1155/1990/36505
Marcarelli, A. M., Baxter, C. V., Benjamin, J. R., Miyake, Y., Murakami, M., Fausch, K. D., et al. (2020). Magnitude and direction of stream–forest community interactions change with timescale. Ecology 101:e03064. doi: 10.1002/ecy.3064
Marcarelli, A. M., Baxter, C. V., Mineau, M. M., and Hall, R. O. Jr. (2011). Quantity and quality: unifying food web and ecosystem perspectives on the role of resource subsidies in freshwaters. Ecology 92, 1215–1225. doi: 10.1890/10-2240.1
Mason, C. F., and MacDonald, S. M. (1982). The input of terrestrial invertebrates from tree canopies to a stream. Freshw. Biol. 12, 305–311. doi: 10.1111/j.1365-2427.1982.tb00624.x
McCafferty, W. P. (1998). Aquatic Entomology: The Fishermen’s and Ecologists’ Illustrated Guide to Insects and Their Relatives. Sudbury, MA: Jones and Bartlett Publishers.
Merritt, R. W., Cummins, K. W., and Berg, M. B. (2008). An Introduction to the Aquatic Insects of North America, 4th Edn. Dubuque: Kendall-Hunt.
Moyle, P. B., and Senayake, F. R. (1984). Resource partitioning among the fishes of rainforest streams in Sri Lanka. J. Zool. 202, 195–223. doi: 10.1111/j.1469-7998.1984.tb05951.x
Nakano, S., Kawaguchi, Y., Taniguchi, Y., Miyasaka, H., Shibata, Y., Urabe, H., et al. (1999a). Selective foraging on terrestrial invertebrates by rainbow trout in a forested headwater stream in northern Japan. Ecol. Res. 14, 351–360. doi: 10.1046/j.1440-1703.1999.00315.x
Nakano, S., Miyasaka, H., and Kuhara, N. (1999b). Terrestrial–aquatic linkages: riparian arthropod inputs alter trophic cascades in a stream food web. Ecology 80, 2435–2441. doi: 10.1890/0012-9658(1999)080[2435:talrai]2.0.co;2
Nakano, S., Fausch, K., and Kitano, S. (1999c). Flexible niche partitioning under varying resource conditions via a foraging mode shift: a proposed mechanism for coexistence in stream-dwelling charrs. J. Anim. Ecol. 68, 1–15.
Nakano, S., and Murakami, M. (2001). Reciprocal subsidies: dynamic interdependence between terrestrial and aquatic food webs. Proc. Natl. Acad. Sci. 98, 166–170. doi: 10.1073/pnas.98.1.166
Novotny, V., and Basset, Y. (1998). Seasonality of sap-sucking insects (Auchenorrhyncha, Hemiptera) feeding on Ficus (Moraceae) in a lowland rain forest in New Guinea. Oecologia 115, 514–522. doi: 10.1007/s004420050549
Orth, D. J. (1983). “Aquatic habitat measurements,” in Fisheries Techniques, eds L. A. Nielsen and D. L. Johnson (Bethesda: American Fisheries Society), 71–84.
Owens, D. C. (2010). Seasonal Variation in Terrestrial Insect Subsidies to Tropical Streams and Implications for the Diet of Rivulus Hartii. [Unpublished master’s thesis]. Lincoln, NE: University of Nebraska.
Palmer, M. A., Arensburger, P., Martin, A. P., and Denman, D. W. (1996). Disturbance and patch-specific responses: the interactive effects of woody debris and floods on lotic invertebrates. Oecologia 105, 247–257. doi: 10.1007/BF00328554
Poff, N. L., Allan, J. D., Bain, M. B., Karr, J. R., Prestegaard, K. L., Richter, B. D., et al. (1997). The natural flow regime. BioScience 47, 769–784.
Polis, G. A., Anderson, W. B., and Holt, R. D. (1997). Toward an integration of landscape and food web ecology: The dynamics of spatially subsidized food webs. Annu. Rev. Ecol. Syst. 28, 289–316. doi: 10.1146/annurev.ecolsys.28.1.289
Pringle, C. M., Naiman, R., Bretschko, G., Karr, J. R., Oswood, M. W., Webster, J. R., et al. (1988). Patch dynamics in lotic systems: the stream as a mosiac. J. N. Am. Benthol. Soc. 7, 503–524.
Reiners, W. A., and Driese, K. L. (2001). The Propagation of Ecological Influences through Heterogeneous Environmental Space: events or conditions located within an environmental domain can lead to consequences elsewhere; the propagation of these influences through environmental space are the linkages that integrate ecological relationships over time and space. BioScience 51, 939–950.
Resh, V. H., Brown, A. V., Covich, A. P., Gurtz, M. E., Li, H. W., Minshall, G. W., et al. (1988). The role of disturbance in stream ecology. J. N. Am. Benthol. Soc. 7, 433–455.
Romaniszyn, E. R., Hutchens, J. J., and Wallace, J. B. (2007). Aquatic and terrestrial invertebrate drift in southern Appalachian Mountain streams: implications for trout food resources. Freshw. Biol. 52, 1–11.
Romero, N., Gresswell, R. E., and Li, J. L. (2005). Changing patterns in coastal cutthroat trout (Oncorhynchus clarki clarki) diet and prey in a gradient of deciduous canopies. Can. J. Fish. Aquat. Sci. 62, 1797–1807. doi: 10.1139/f05-099
Rundio, D. E., and Lindley, S. T. (2008). Seasonal patterns of terrestrial and aquatic prey abundance and use by Oncorhynchus mykiss in a California coastal basin with a Mediterranean climate. Trans. Am. Fish. Soc. 137, 467–480.
Rundio, D. E., and Lindley, S. T. (2012). Reciprocal fluxes of stream and riparian invertebrates in a coastal California basin with Mediterranean climate. Ecol. Res. 27, 539–550. doi: 10.1007/s11284-011-0920-6
Seghers, B. H. (1978). Feeding behavior and terrestrial locomotion in the cyprinodontid fish, Rivulus hartii (Boulenger). Int. Vereinigung Theor. Angerw. Limnol. 20, 2055–2059.
Smythe, N. (1982). “The seasonal abundance of night-flying insects in a Neotropical forest,” in The Ecology of a Tropical Forest: Seasonal Rhythms and Long Term Changes, eds E. G. Leigh Jr. A. S. Rand and D. M. Windsor (Washington, DC: Smithsonian Institution Press).
Sullivan, M. L., Zhang, Y., and Bonner, T. H. (2012). Terrestrial subsidies in the diets of stream fishes of the USA: comparisons among taxa and morphology. Mar. Freshw. Res. 63, 409–414. doi: 10.1071/mf11232
Sutton, S. L., Ash, C. P. J., and Grundy, A. (1983). The vertical distribution of flying insects in lowland rainforests of Panama, Papua New Guinea and Brunei. J. Linn. Soc. Lond. 78, 287–297. doi: 10.1111/j.1096-3642.1983.tb00868.x
Takimoto, G., Iwata, T., and Murakami, M. (2009). Timescale hierarchy determines the indirect effects of fluctuating subsidy inputs on in situ resources. Am. Nat. 173, 200–211. doi: 10.1086/595759
Takimoto, G., and Sato, T. (2020). Timing and duration of phenological resources: toward a mechanistic understanding of their impacts on community structure and ecosystem processes in stream food chains. Ecol. Res. 35, 463–473. doi: 10.1111/1440-1703.12098
Tanaka, H. (1960). On the daily change of the drifting of benthic animals in stream, especially on the types of daily changes observed in taxonomic groups of insects. Bull. Freshw. Fish Res. Lab. Tokyo 9, 13–24.
Wallace, J. B., Eggert, S. L., Meyer, J. L., and Webster, J. R. (1997). Multiple trophic levels of a forest stream linked to terrestrial litter inputs. Science 277, 102–104.
Wantzen, K. M., Ramírez, A., and Winemiller, K. O. (2006). New vistas in Neotropical stream ecology—Preface. J. N. Am. Benthol. Soc. 25, 61–65. doi: 10.1899/0887-3593(2006)25[61:nvinse]2.0.co;2
Wasserstein, R. L., Schirm, A. L., and Lazar, N. A. (2019). Moving to a world beyond “p< 0.05”. Am. Stat. 73, 1–19.
Waters, T. F. (1962). Diurnal periodicity in the drift of stream invertebrates. Ecology 43, 316–320. doi: 10.2307/1931988
Wilson, E. O. (1959). Some ecological characteristics of ants in New Guinea rain forest. Ecology 40, 437–447.
Winemiller, K. O., Montoya, J. V., Roelke, D. L., Layman, C. A., and Cotner, J. B. (2006). Seasonally varying impact of detritivorous fishes on the benthic ecology of a tropical floodplain river. J. N. Am. Benthol. Soc. 25, 250–262. doi: 10.1899/0887-3593(2006)25[250:sviodf]2.0.co;2
Wipfli, M. S. (1997). Terrestrial invertebrates as salmonid prey and nitrogen sources in streams: contrasting old-growth and young-growth riparian forests in southeastern Alaska, U.S.A. Can. J. Fish. Aquat. Sci. 54, 1259–1269.
Wright, S. J., and Cornejo, F. H. (1990). Seasonal drought and leaf fall in a tropical forest. Ecology 71, 1165–1175. doi: 10.2307/1937384
Yang, L. H. (2020). Toward a more temporally explicit framework for community ecology. Ecol. Res. 35, 445–462. doi: 10.1111/1440-1703.12099
Keywords: terrestrial subsidies, tropical streams, invertebrates, fish, drift, seasonality, canopy, precipitation
Citation: Owens DC, Heatherly TN, Eskridge KM, Baxter CV and Thomas SA (2022) Seasonal Variation in Terrestrial Invertebrate Subsidies to Tropical Streams and Implications for the Feeding Ecology of Hart’s Rivulus (Anablepsoides hartii). Front. Ecol. Evol. 10:788625. doi: 10.3389/fevo.2022.788625
Received: 03 October 2021; Accepted: 10 January 2022;
Published: 01 February 2022.
Edited by:
Jeff Wesner, University of South Dakota, United StatesCopyright © 2022 Owens, Heatherly, Eskridge, Baxter and Thomas. This is an open-access article distributed under the terms of the Creative Commons Attribution License (CC BY). The use, distribution or reproduction in other forums is permitted, provided the original author(s) and the copyright owner(s) are credited and that the original publication in this journal is cited, in accordance with accepted academic practice. No use, distribution or reproduction is permitted which does not comply with these terms.
*Correspondence: David C. Owens, ZGNvd2Vuc0BnZW9yZ2lhc291dGhlcm4uZWR1