- 1The Nature Conservancy Centre (TNC), New Delhi, India
- 2Ashoka Trust for Research in Ecology and the Environment, Bengaluru, India
- 3Department of Biology, University of Massachusetts, Boston, MA, United States
Placentation refers to the mode of ovule attachment on the wall of the ovary. In multiovulate ovaries, placentation influences interactions among developing seeds with varying degrees of kinships. Placentation is a taxonomically informative character in flowering plants, yet little has been written about the origin and evolutionary trends of various placentation types in flowering plants since Puri’s and Stebbins’ work, over six decades and almost four decades ago, respectively. More recently, some authors have written about the evolution of placentation in certain groups, but an overall perspective for angiosperms is lacking. For 421 families of angiosperms, we collected data on placentation types and ovule number, and analyzed the data in the phylogenetic context using recent comprehensive phylogeny of angiosperms to test the hypotheses on the evolution of various placentation types and their association with ovule number. The distribution of placentation types across flowering plants suggests that axile placentation, followed by parietal and basal placentation, occurs more frequently than laminar and free central placentation that are very rare. Our results are more consistent with evolutionary trends proposed by Puri than by Stebbins and suggest that marginal placentation is the ancestral and most primitive placentation type, while axile is the most advanced. Placentation types show strong association with ovule number. Finally, our results on ovule number and placentation types indicate that most angiosperms may fall into two categories: one with one or few ovule(s) and basal placentation, and another with many ovules and parietal and axile placentation. Kin selection within ovaries may play a role in explaining the observed patterns. Overall, our results provide new insights into the evolution of placentation, particularly into the drivers underlying the diversification of various placentation types.
Introduction
Placentation refers to the mode of ovule attachment on the ovary wall. The ovary in most flowering plants is a compound structure formed by the union of two or more carpels. The number of ovules found within the ovary, the number of carpels fusing to form the compound ovary, and the position of ovules within the ovary following the union of carpels have generated several types of placentation in flowering plants. Little has been written about the evolution of placentation since Puri’s (1952) classic paper nearly 65 years ago. The last comprehensive account was by Stebbins (1974), almost 40 years ago. Recently, evolution of placentation has been studied in a few angiosperm orders such as Malpighiales (Endress et al., 2013) and Vitales (Ickert-Bond et al., 2014), but an overall perspective across angiosperms, considering evolutionary relationships of taxa, is lacking.
Flowers in most of the early branching/early diverging lineages of angiosperms have one to many free carpels, each carpel with one or few ovules along the margins sealed by secretion (Figure 1Ba; Puri, 1952; Stebbins, 1974; Endress and Igersheim, 2000). Early in the evolution of angiosperms, the carpels fused to form a compound ovary (Figures 1Bb,h). The fusion of the carpels led to a bi- or plurilocular ovary, with the number of locules being defined by the number of carpels fusing with each other. In this bi- or plurilocular ovary, the ovules develop along the axis of the central column (Figure 1Bb), on fused margins of their own carpels. Both Puri (1952) and Stebbins (1974) traced the evolution of various types of placentation from this axile placentation (Figures 1Ab,Bb) and considered marginal placentation as the simplest and most primitive type (Figure 1). Puri (1952) suggested that evolution of placentation in flowering plants is complex, progressing in several directions along at least five divergent pathways (Figure 1A), whereas Stebbins (1974) argued that placentation evolution in angiosperms is uniform, proceeding in a single direction along three parallel pathways (Figure 1B). In the first pathway, there has been a reduction in the number of ovules to one in each chamber and subsequently to a unilocular ovary with a few or one ovule with basal placentation (Figures 1Ad,Bd). The unilocular ovary with basal placentation evolved further into an ovary with free central placentation, a trend accompanied by an increase in the number of ovules (Figure 1Be). In the second pathway, the initial stages were the same as in the first. There was a decrease in the number of ovules to one in each chamber, but the ovules were pendulous rather than basal (Figure 1Bf). This was followed by the evolution of a unilocular ovary with one pendulous ovule (Figure 1Bg). The third pathway also led to a unilocular ovary, with ovules borne along the ridges or all along the ovary wall. This is known as parietal placentation (Figures 1Ae,Be). The number of ovules, and presumably seeds as well, are generally large.
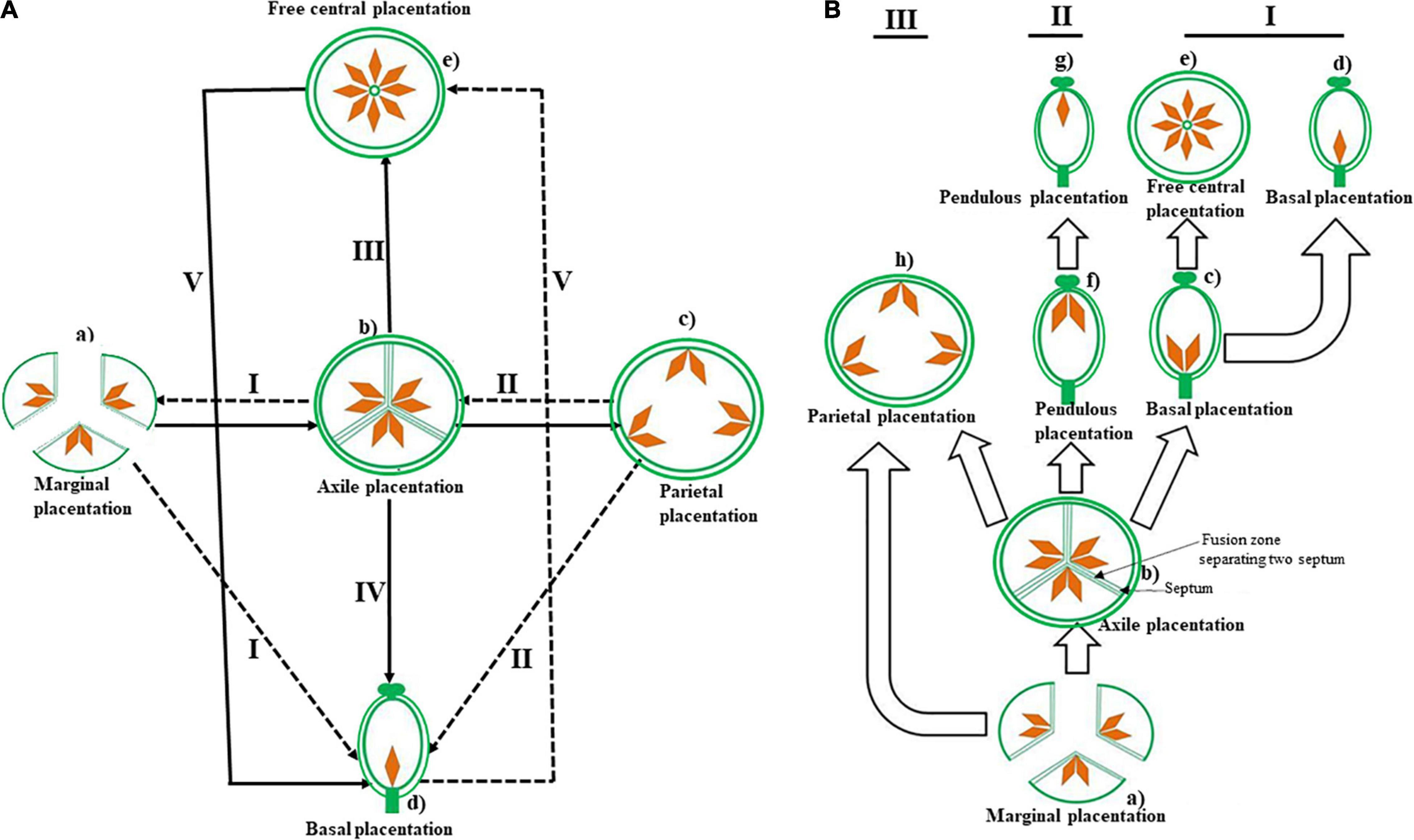
Figure 1. The hypothetical schematic diagram of the evolutionary trend and relationship of various placentation types in the angiosperm flower as proposed by Puri (1952) (A) and Stebbins (1974) (B). Transverse section of ovary with different placentation types is also shown in the figure: (A-a) marginal placentation, (b) axile placentation, (c) parietal placentation, (d) basal placentation, and (e) free central placentation; (B-a) apocarpous gynoecium, (b) axile placentation, (c,d) basal placentation, (e) free central placentation, (f,g) pendulous placentation, and (h) parietal placentation. The different pathways within author’s proposed scheme are given in roman numbers (I, II, III, IV, and V). Dotted arrows indicate possibilities of evolution, but these have yet to be proved.
Both Puri (1952) and Stebbins (1974) examined drivers of evolution in placentation in terms of morphology, anatomy, and resource utilization. In species with multiovulate flowers, developing seeds compete for finite resources. The position and the degree of isolation among ovules may have an impact on competitive outcomes. Recently, we argued that competition may also be influenced by kin selection that may increase cooperation among developing seeds and reduce deleterious effects of competition (Bawa, 2016; Bawa et al., 2019). Genetic relatedness of developing seeds within an ovary may be influenced by specialized pollinators capable of delivering many pollen grains from a single pollen parent to a flower. Here we suggest that the correlated evolution of placentation, ovule position, and ovule number can also be explained in the context of sibship and kin selection within an ovary.
The evolutionary trends proposed by Puri (1952), and Stebbins (1974) considered the prevalent phylogeny of angiosperms at that time, which differed considerably from contemporary phylogenetic approaches based on comparative phylogenetic methods (PCMs) that have recently emerged as powerful tools to gain insights into morphological, physiological, and anatomical trait evolution across angiosperms (Willis et al., 2014; Chomicki et al., 2017; Bawa et al., 2019; Carta et al., 2020). Thus, we first map placentation types and ovule number/locule across the comprehensive angiosperm phylogeny and conduct comparative phylogenetic analyses to address the following questions: 1. What is the frequency of various types of placentation in angiosperms? 2. How consistent are the evolutionary trends proposed by Puri (1952; Figure 1A) and Stebbins (1974; Figure 1B), considering the evolutionary relationships of taxa across flowering plants? 3. What is the association between placentation types and other reproductive traits such as ovule position, ovule number/locule, degree of separation among developing seeds, and kinship relationships among seeds? Such associations, we argue, can provide new insights into the evolution of placentation in flowering plants.
Materials and Methods
Placentation and Associated Trait Data Collection
To understand the evolutionary trends and diversity of placentation types across angiosperms, we attempted to infer placentation evolution in a phylogenetic framework, thus we needed to code different placentation types as different character states. By referring to published literature on placentation (Puri, 1952, 1961; Rohweder, 1967; Takhtajan, 1991; Endress, 1994, 2011a,b,2015; Igersheim and Endress, 1998; Carlsward et al., 2011; Endress and Doyle, 2009; Supplementary Data 1), we recognize two placentation types in apocarpous gynoecia: (1) marginal (sub marginal by other authors, e.g., Takhtajan, 2009; Figures 1Aa,Ba), when ovules borne on the fused margin of the same carpel in unicarpellate gynoecia, and postgenital fusion of the involute margins at the point of contact, result in a syncarpous gynoecium; and (2) laminar (often referred to as laminar-diffuse placentae), describing ovules being scattered over the inner carpel surface rather than the central column; and five placentation types in syncarpous gynoecia: (1) axile (Figures 1Ab,Bb), the most common placentation type, where ovules are found at the curved inward margins of the carpels or central column of ovary where septa meet; (2) apical (Figures 1Bf,Bg), modification of axile placentation, where the gynoecium is unilocular and a single or few ovules is/are inserted at the apex of the gynoecium in longitudinal section; (3) basal (Figures 1Ad,Bd), modification of axile placentation, where the single ovule is inserted at the base of the ovary; (4) free central (Figures 1Ae,Be), also modified from axile placentation, except that the gynoecium is unilocular and septa are absent and often swollen placenta are formed at the base of the ovary (Primulaceae) or septa disintegrate during later development stages and the central column of the ovary remains (Caryophyllaceae) (Rohweder, 1967; Rohweder and König, 1971; Rohweder and Urmi-König, 1975); and (5) parietal (Figures 1Ac,Bh), when ovules and their associated placenta are borne on the periphery where adjoining carpels meet and septa do not extend to the center in the unilocular gynoecium. These seven recognized placentation types encompass the full range of placentation diversity in flowering plants.
The placentation data for each flowering plant family (the family delimitation is based on APG IV) (Chase et al., 2016) were aggregated from data collected for 771 taxa, of which 367 families had 722 entries at the genus level and the other 49 at the family level. These data were compiled from hundreds of published records and online databases (Supplementary Data 1). Of the 722 genus level entries spread across 367 families, 232 families had placentation data available for single genus, 98 families had data available for two to three genera, and only 37 families had available data from four, five or >5 genera. Of the 416 families, 340 families (82%) were represented by a single placentation type, 62 families (15%) were represented by two placentation types, and 14 families (3%) were represented by three to four placentation types. Considering the variability of placentation across flowering plants, and the computational challenge in estimating evolutionary parameters for an unordered discrete character with multiple polymorphic states of placentation, inclusion of families with more than one placentation type will create computational challenge. Therefore to overcome this computation challenge, in case of families with more than two types of placentation, we referred to the literature to find out which placentation types is ancestral or derived in that family. For example, in Xyridaceae the axile is the ancestral placentation whereas basal, free central, and parietal are derived types (Nardi et al., 2021). Thus, we used axile as the primary placentation type for Xyridaceae in our analysis. By following this procedure, we also included families with more than one placentation types in the analysis. In total, we compiled placentation and associated trait data for all 416 angiosperm families recognized by the APG IV classification (Chase et al., 2016; Supplementary Data 1).
Generation of Family Level Dated Phylogenetic Hypothesis
Taxonomic information on the 421 angiosperm families used in the present study was standardized against the Plant List Database1 and Angiosperm Phylogeny Website.2 Of the 421 families, 403 were recognized in the APG IV classification system (Chase et al., 2016). Remaining families were synonyms. Furthermore, a comprehensive family level dated phylogeny of flowering plants was reconstructed using the following procedure. First, we randomly retained a single species for each of the 421 angiosperm families in the Zanne et al. (2014a,b) species (31,749 species) level phylogeny of flowering plants using drop.tip function in PHYTOOLS (Revell, 2012). Then, we replaced the species names with the respective family names to develop family-level dated phylogenetic hypotheses for flowering plants.
To account for phylogenetic uncertainty because of limited phylogenetic resolution and branch length variation, we further generated 100 dated phylogenetic topologies from the 100-bootstrap set of species level time-scaled MLE trees deposited by Zanne et al. (2014b) in the Dryad database3 by following a procedure similar to that outlined above. All subsequent analyses were run and averaged across all 101 trees, which includes one maximum clade credibility tree and the 100-bootstrap set of time-scaled MLE trees.
Comparative Phylogenetic Analysis
First, to test whether the evolution of placentation and other associated traits (ovule number) in flowering plants was constrained by their phylogenetic relatedness, we performed the phylogenetic signal test using two alternative methods: Blomberg’s K (Blomberg et al., 2003) and Pagel’s lambda (λ) (Pagel, 1999). The λ value varies between 0 and 1, while K varies from 0 to ∞. The Pagel’s λ and Blomberg’s K are expected to be equal to 1 and >1, respectively, if there is a strong phylogenetic dependence of traits. However, λ > 0 or K < 1 correspond to some degree of trait lability. Alternatively, λ or K values of 0 imply that there is no phylogenetic dependence. We tested for significance in the phylogenetic signal assessed by Pagel’s λ and Blomberg’s K (null hypothesis of λ and K = 0) by 1,000 randomizations of species names in phylogeny under the ARD (variable transition rate) transition model (Supplementary Table 1). The significance of λ and K was assessed with a likelihood ratio test (Pagel, 1999). The likelihood ratio test compares the likelihood of λ, and K calculated from the true tree to the likelihood of 0. The function of these methods is available in the R package phytools (Revell, 2012).
We also estimated character retention rate, which refers to the degree of synapomorphy in a given character (here, placentation type) using the retention index (RI) measure in Mesquite v. 2.75 (Maddison and Maddison, 2011). RI values range between 0 and 1, the value of 1 indicates maximum synapomorphy, meaning that the character (here, placentation type) originated just once in the common ancestor of all the extant taxa that exhibit it, or the character is derived from that common ancestor. In contrast, an RI value of 0 indicates maximum homoplasy, such that every appearance of the character on the tree is a result of a new, independent event.
To examine the evolutionary trend of placentation in flowering plants, we mapped different placentation types onto the dated phylogenetic tree of flowering plants using four different types of ancestral state reconstruction methods: maximum parsimony in Mesquite v. 2.75 (Maddison and Maddison, 2011), maximum likelihood (ML) and reversible jump Markov chain Monte Carlo (RJMCMC) in BayesTraits v. 2.0 (Pagel and Meade, 20064), and stochastic character mapping (SIMMAP; Bollback, 2006) using the package “phytools” (Revell, 2012) implemented in R (R Development Core Team, 2013). Stochastic character mapping methods overcome the inherent limitations of parsimony-based ancestral reconstruction (Huelsenbeck et al., 2003; Bollback, 2006). In this method, character change is assumed to follow a “continuous-time Markov process” and implements stochastic substitution models for both molecular and non-molecular characters to simulate the character histories (Nielsen, 2002; Bollback, 2006). We assumed transition rates to be variable for placentation type (discrete character) [ARD, supported as best model (Supplementary Table 1)] in all analyses, and for minimum and maximum ovule number (continuous character), we assumed early burst (EB) model (Supplementary Table 1). We ran a ML analysis for 10,000 iterations, and RJMCMC analysis for 5,050,000 iterations with a burn-in of 50,000 iterations, and the chain was sampled every 100th iteration, creating a posterior distribution of 50,000 sample points assuming reversible-jump hyperprior. For SIMMAP analyses, we ran 1,000 simulations. The ancestral state reconstruction results are sensitive to root state and can potentially bias the results depending on root state. To account for this bias and to test whether changing the root state can potentially influence the ancestral state reconstruction results, we tested alternative hypotheses with ML and RJMCMC models by fixing the root state of placentation to the following states: marginal, laminar, basal, apical, free central, axile, and parietal. We then compared harmonic mean log-likelihood scores across the constrained models to determine which constrained model better explained the ancestral state of placentation in extant flowering plants, and the best model was chosen using Akaike information criterion (AIC) statistics. We further estimated transition numbers based on parsimony and SIMMAP analysis of ancestral state reconstruction. For parsimony analyses, transition numbers were estimated using the “minCharChange” function in paleotree (Bapst, 2012). For SIMMAP analyses, transition numbers were obtained from the “describe.simmap” function in the package “phytools” (Revell, 2012). We also counted the number of character changes between states and summed the time spent in each state along all tree edges.
We also tested whether abundance or occurrence frequency of different placentation types bias the estimates of transition rate shift between different placentation types and thus influence the results of different placentation types to act as either a net source or sink for transitions. For example, we observed a greater number of shifts from axile to other placentation types than vice versa (Figure 3A). We therefore repeated this test, correcting for the potential correlation between the number of transitions and the frequency of each placentation type in the dataset. The number of transitions to or from individual placentation types was multiplied by one minus the fraction of families with that placentation type and rescaled to the total number of transitions.
We used the phyloANOVA function in phytools (Revell, 2012) to run the simulation-based phylogenetic analysis of variance (Garland et al., 1993) to test for differences in ovule number/locule among angiosperm families with different placentation types. All analyses were run across 100 bootstrapped MLE trees, and results were averaged across all 100 trees.
Results
Distribution and Abundance Pattern of Various Placentation Types in Flowering Plants
The observed distribution of different placentation types in flowering plants indicates that placentation types restricted to both apocarpous (marginal) and syncarpous (basal/apical) gynoecium were already present in early flowering plants such as the early branching/early diverging lineages of angiosperms and magnoliids (Figure 2 and Supplementary Figure 1). However, the frequency of different placentation types indicates that placentation types restricted to unilocular gynoecium (marginal, basal, apical, and parietal) were more abundant than placentation types restricted to multilocular gynoecium (axile) in early flowering plants. We note that the rapid radiation of axile placentation (confined to multilocular gynoecium) mostly coincided with the divergence of monocots from early flowering plants (Figure 2 and Supplementary Figure 1). Moreover, the free central placentation most frequently occurred, and became abundant, in recently evolved angiosperms such as eudicots. Overall, axile, apical, basal, and parietal placentation were more abundant, whereas marginal, laminar, and free central placentation were less common or rare (Figure 2 and Supplementary Figure 1).
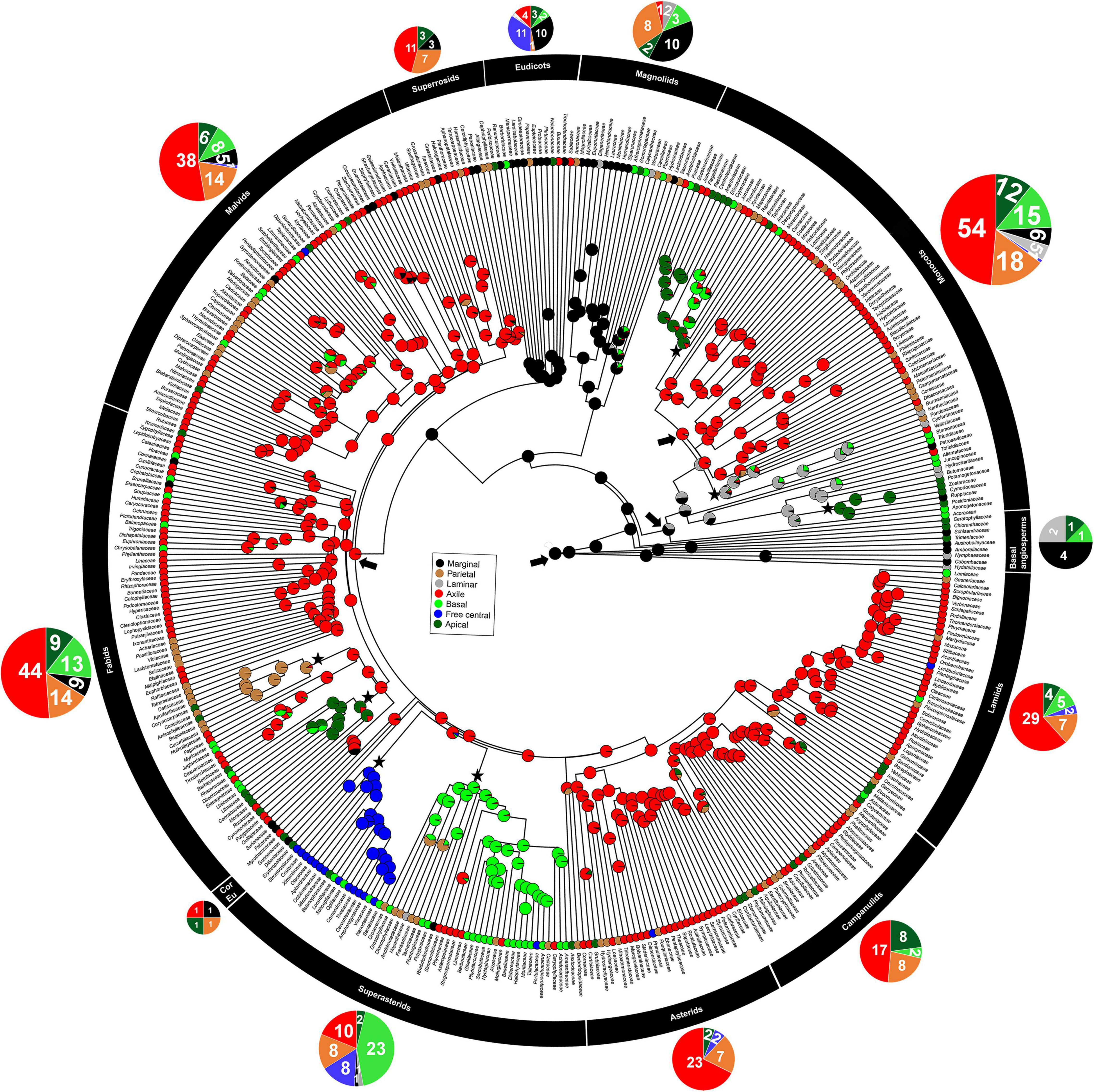
Figure 2. The phylogeny of extant angiosperms, showing plausible hypothesis of placentation evolution based on stochastic character mapping ancestral-state reconstructions of various placentation types for nodes of each family. Pie charts at nodes represent the posterior probabilities of each placentation type, averaged across 100 trees. Pie charts at the tips of the tree represent the proportion of placentation type within each family (see also Supplementary Data 1). Pie charts above the color strip represent the proportion of placentation classes (by family) within each 12 delineated major classes of angiosperms. The pie circle size is proportional to the number of extant families in the group. The groups indicated by black color strip represent major classes of angiosperm in their order of evolutionary origin according to the APG IV classification of flowering plant (Chase et al., 2016). The groups are, from top to bottom: basal angiosperms, magnoliids, monocots, eudicots, superrosids, malvids, fabids, core eudicots, superasterids, asterids, campanulids, and lamiids. The color legend indicates the specific placentation type. The arrows represent origin and major shift to different placentation types and orders where major shifts occurred are shown with asterisks.
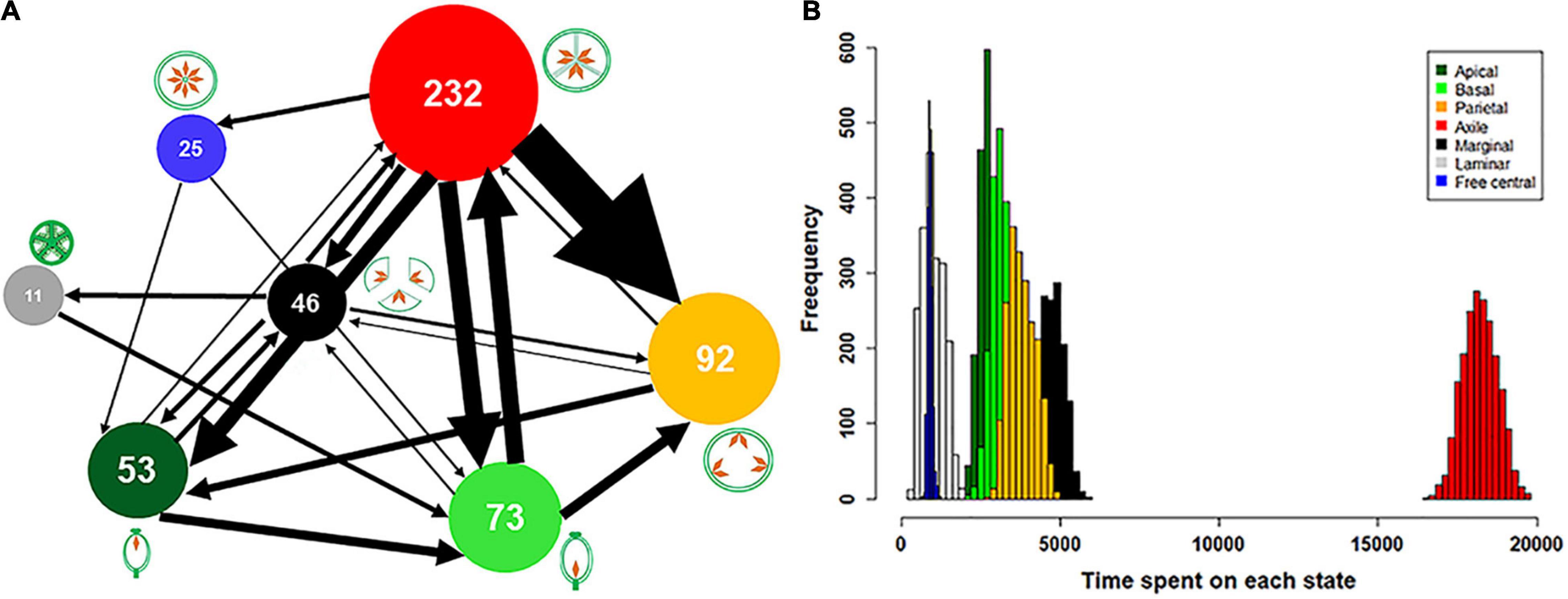
Figure 3. Schematic reorientation of number of evolutionary transitions and persistence time between each placentation class for extant families of angiosperms. (A) Evolutionary transitions between various placentation types (see also Supplementary Figure 3), and (B) Histogram of persistence time of the individual placentation type. The evolutionary transition and median persistence time for each placentation class was calculated for 1,000 separate character maps. Arrows indicate the direction and relative proportion (given by line thickness) of transitions between placentation types. The circle size is proportional to the number of extant families with the given type of placentation (also included inside the circle). Transition numbers and persistence time are based on stochastic mapping.
Phylogenetic Signal and Evolutionary Lability of Placentation
Our analysis revealed significant phylogenetic signal for placentation, indicating closely related flowering plants tend to evolve similar placentation type; in other words, placentation evolution is constrained by the phylogenetic relationships of taxa (Table 1). The conservative evolution of placentation is also evident in the observed distribution of placentation types across flowering plants: the occurrence of different placentation types is non-random across flowering plants, so that certain placentation types occur more frequently in certain lineages. For example, marginal placentation occurs disproportionately in early branching/early diverging lineages of angiosperms, magnoliids, and early lineage of eudicots, while free central and apical placentation are mostly restricted to superasterids (Figure 2 and Supplementary Figure 1).
The test for significant homoplasy or synapomorphy further indicated large degree of synapomorphy (i.e., conservative evolution) in placentation types across flowering plants. The observed RI value of 0.421 was significantly higher than null expectations indicating significant synapomorphy in placentation trait (Table 1). The high number of bi-directional transition rate shifts observed only in few placentation types also suggest a high degree of synapomorphy in this character (Figure 3A).
Ancestral State and Evolutionary Trend of Placentation
Placentation types restricted to both unilocular-apocarpous gynoecium (Hydatellaceae, Cabombaceae, Nymphaeaceae, Amborellaceae, Austrobaileyaceae, and Schisandraceae) and unilocular-syncarpous gynoecium (Aponogetonaceae, Acoraceae, Chloranthaceae, Ceratophyllaceae, and Trimeniaceae) are present in early branching/early diverging lineages of angiosperms (Figure 2 and Supplementary Figure 1). Nevertheless, based on all ancestral state estimates (Table 2 and Figure 2), marginal placentation (restricted to unilocular-apocarpous gynoecium) is the most probable ancestral placentation in flowering plants. Moreover, our ancestral state estimation result was not sensitive to placentation state (marginal) at the root. The comparison of MCMC and ML models with different types of placentation fixed as the root state, consistently supported the model with marginal placentation as the best fit model of ancestral state (Supplementary Table 2).
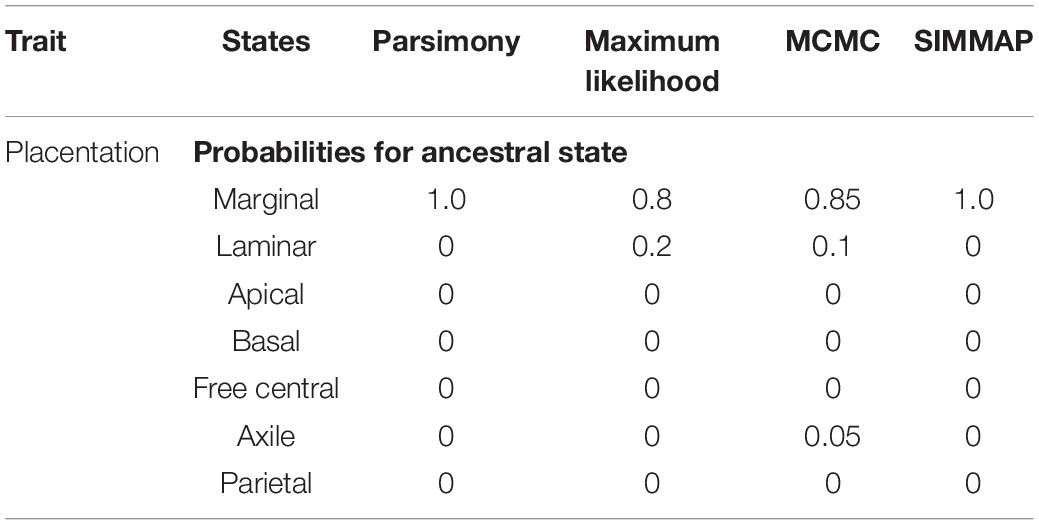
Table 2. Ancestral state estimates for placentation evolution in angiosperms using four different methods: parsimony, maximum likelihood (ML), reversible jump Markov chain Monte Carlo (RJMCMC), stochastic mapping (SIMMAP).
The laminar, apical, and free central placentation types were distributed less frequently across tips of phylogeny as compared to other placentation types, suggesting that these placentation types are prone to evolutionary loss, while the reverse is true for axile, parietal, marginal, and basal placentation (Figure 2). In the early diverging flowering plants, there were probably no major shifts from marginal to other placentation types (Figure 2). The first major shift from marginal to other placentation restricted to syncarpous gynoecia (axile, apical, and basal) was apparently associated with the origin of monocots, and the second major shift was associated with the origin of superrosids. Finally, there was a single shift each from axile to basal and axile to free central associated with superasterids (Figure 2 and Supplementary Figure 3). The parietal placentation was distributed more frequently at phylogenetic tips than at nodes, whereas the reverse was true for marginal and laminar placentation. In contrast, free central, basal, apical, and axile placentation were distributed across both phylogenetic nodes and tips (Figure 2).
The distribution pattern of placentation types at phylogenetic nodes and tips indicates their evolutionary history. The distribution of parietal placentation at tips rather than nodes suggests they are highly derived placentation types. The distribution of marginal and laminar placentation at nodes rather than tips suggests their primitive character, while the distribution of axile, basal, apical, and free central placentation across nodes and tips suggest that they are more advanced in flowering plants (Figure 2). In comparison to other placentation types, parietal placentation seems to have a high transition rate from axile placentation (Figure 3A and Supplementary Figure 2). Furthermore, axile, parietal, basal, and marginal placentation have been more persistent and prevalent in angiosperms than other placentation types (Figure 3B). These results further support the conservative evolution of these placentation types in flowering plants.
We observed both uni-directional and bi-directional evolution for different placentation types (Figures 1A, 3A). Thus, we noted bi-directional transition rate shifts between seven pairs of placentation types: from marginal to axile and vice-versa, marginal to parietal and vice-versa, from marginal to apical, basal, and vice-versa, axile to basal, apical, and vice-versa, and axile to parietal and vice-versa. We observed uni-directional transition rate shifts between nine pairs of placentation types: marginal to laminar, free central to basal and apical, axile to free central, parietal to apical, laminar to basal, apical to basal, and basal to parietal. However, we did not find transitions to laminar and free central placentation from other placentation types except from marginal and axile, respectively. Furthermore, with respect to transition rates, we observed a disproportionately high rate of transitions from axile to other placentation types followed by basal placentation. There were also disproportionately high uni-directional transition rates from apical to basal, basal to parietal, and parietal to apical placentation (Figure 3A and Supplementary Figure 2).
Our analysis of directional transition rate shifts revealed that axile placentation is acted as an “evolutionary hub” to which and from which the other placentation types evolved (Table 3 and Figure 3A). Moreover, considering the significant binomial test for the source-sink relationship, axile placentation followed by marginal was the only significant evolutionary “source” (i.e., the number of transitions from axile and marginal placentation to other types was significantly greater than the number of transitions to axile and marginal) as revealed from both parsimony and stochastic character mapping analyses (Table 3 and Figure 3A). Alternatively, our analysis consistently supported basal, apical, and parietal placentation as an evolutionary “sink” (i.e., the number of transitions from basal, apical, and parietal placentation to other types was significantly less than the number of transitions to basal, apical, and parietal), indicating that basal, apical, and parietal placentation are mostly evolved from other placentation types (Table 3 and Figure 2). Furthermore, correcting for transition bias due to the occurrence frequency of different placentation types did not change the overall pattern (Table 3).
The ancestral reconstruction analysis for ovule number revealed that both the common ancestor and early flowering plants had single or few ovules per locule (Supplementary Figure 3). As in placentation, the evolution of ovule number appears to be constrained by phylogenetic history, as indicated by high values of K and λ (Supplementary Figure 3).
Association of Placentation With Ovule Number and Other Traits
We found strong associations between placentation type and ovule number (Figure 4 and Supplementary Figure 1). Basal and apical placentation types were mostly associated with a single ovule or very rarely few or many ovules, whereas marginal placentation was associated with both single and high ovule number. Free central placentation was frequently associated with few ovules, rarely with many ovules. Laminar and axile placentation were associated with both low and high ovule number. Parietal placentation was most frequently associated with high ovule number and rarely with few ovules (Figure 4 and Supplementary Figure 1). Among placentation types, parietal placentation had the highest ovule number/locule, followed by laminar, axile and, marginal placentation, whereas basal, apical, and free central placentation had a low ovule number/locule (Figure 4 and Supplementary Figure 1). Early branching/early diverging lineages of angiosperms and magnoliids were most frequently associated with one or few ovules and with basal, apical, and marginal placentation, and rarely associated with high ovule number/locule with laminar and parietal placentation. In contrast, monocots, and the more recently evolved angiosperms such as eudicots (Asterids, Campanulids, Lamiids, etc.) were mostly associated with high ovule number and with axile and parietal placentation (Supplementary Figure 1).
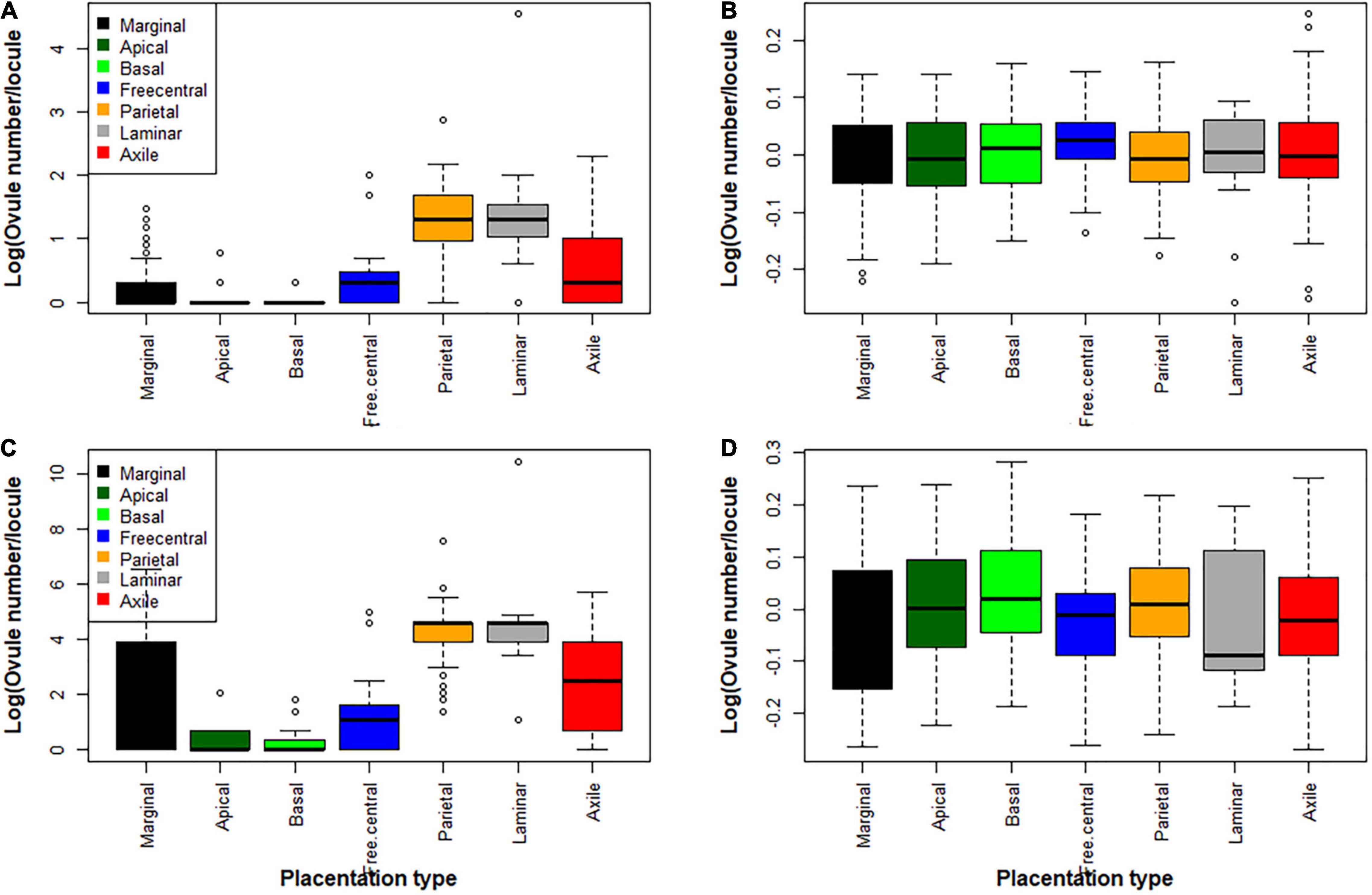
Figure 4. Variation in ovule number in relation to placentation types in flowering plants. (A,C) Phylogenetically not corrected (without accounting for phylogenetic relationship of taxa), and (B,D) phylogenetically corrected (after accounting for phylogenetic relationship of taxa). Box and whisker plots show the variation in ovule number among the different placentation types (see also Supplementary Figure 3), as calculated from both not corrected and phylogenetically corrected residuals. For each placentation category, the “box” represents the second quartile and the error bars (“whiskers”) are the first and third quartiles. The horizontal bar in each box represents the median.
We analyzed the association between ovule number/locule and placentation type while accounting for the phylogenetic relationship of taxa to determine whether the observed variation of ovule number with placentation type is constrained by evolutionary history (Figures 4B,D). The phylogenetic residuals of ovule number against placentation type indicate that accounting for phylogenetic relationships weakens associations (Figures 4B,D). However, associations remain significant (F = 49.48, P < 0.001). Thus, phyloANOVA results suggest that the strong association of ovule number with placentation type is not independent of phylogenetic relationship of taxa (λ = 0.544).
Discussion
Despite its recognition as a taxonomically informative character in the classification of flowering plants (Lindley, 1830), we have a limited understanding of the origin and evolutionary history of placentation diversity in flowering plants (Puri, 1952; Stebbins, 1974; Ickert-Bond et al., 2014). Many authors believe that different placentation types evolved conservatively and used placentation as a taxonomically informative character (Lindley, 1830; Troll, 1928; Gundersen, 1939; Endress and Matthews, 2012). On the other hand, based on the repeated evolution of various placentation types across distantly related lineages of flowering plants, others have argued that placentation evolution is a more labile trait, with limited utility as a taxonomic character (Endress, 1994; Ronse De Craene, 2010).
Our results demonstrate that the distribution of placentation types across the seed plants is non-random and evolves conservatively. Certain placentation types occur more frequently in certain lineages (e.g., marginal/laminar placentation occurs disproportionately in early branching/early diverging lineages of angiosperms, magnoliids and basal eudicots, while free central placentation is mostly restricted to Santalales and Ericales). Therefore, placentation is a systematically informative character to resolve generic and family level relationship in angiosperms (Endress et al., 2013; Ickert-Bond et al., 2014; Mori et al., 2015).
Evolutionary Trends in Placentation
Numerous attempts have been made to show that one type of placentation is more primitive than the other, and many authors have independently claimed that marginal, axile, or parietal placentation could be the most primitive and ancestral (Engler, 1909; Hagerup, 1938, 1939; Takhtajan, 1942, 1948, 1959, 1964; Joshi, 1947; Puri, 1952; Stebbins, 1974). However, considering the evolutionary history of lineages, and mapping various placentation types onto the recent comprehensive phylogenetic hypothesis of flowering plants, we note that the most likely ancestral placentation type in angiosperms is marginal, in line with the notion that an apocarpous gynoecium was present in the earliest flowering plants (Sauquet et al., 2017). The recently reported angiosperm fossils from the Jurassic named as Nanjinganthus (Fu et al., 2018; Taylor and Li, 2018) and basal angiosperm fossils from Cretaceous showing affinity with Nymphaeaceae such as Monetianthus mirus (Friis et al., 2009) and Pluricarpellatia (Mohr et al., 2008) and fossils of Archaefructus (Wang and Zheng, 2012) are all reported to have either marginal or laminar placentation, which further supports the primitive nature of placentation types restricted to apocarpous gynoecium in flowering plants. The marginal placentation subsequently evolved into other types. Indeed, Puri (1952) treated marginal placentation as the most primitive (Figure 1A). Bailey and his associates, based on the carpel structure of Ranunculales families, provided considerable evidence for holding marginal placentation as the simplest and most primitive (Bailey and Swamy, 1951; Eames, 1961). A recent study also concluded that marginal placentation is the most primitive and ancestral in flowering plants (Ickert-Bond et al., 2014). Xingxueanthus a plant fossil from the Jurassic is reported to have a free central placentation (Wang, 2018). However, the taxonomic position of Xingxueanthus is still debated, being either an early Angiosperm, a new class of Gymnosperm, or a poorly preserved conipherophyte cone. If future studies confirm the Xingxueanthus affinity with angiosperms, the occurrence of free central placentation in Jurassic angiosperms would contradict the notion that most primitive angiosperms had conduplicate carpels and marginal placentation, and free central placentation is always derived (Puri, 1952; Friis et al., 2009; Wang, 2018).
Both Puri (1952) and Stebbins (1974) traced the evolution of various types of placentation from axile placentation assuming it is primitive and other placentation types are derived from it. However, our comparative phylogenetic analysis of placentation types indicates that axile is the most widespread placentation type. Further, our results support the derived evolution of parietal placentation from axile placentation as reported by others (Lindsey, 1940; Wilkinson, 1944, 1949; Gauthier, 1950). However, independent evolution of parietal placentation from marginal placentation is also plausible. The disproportionately high uni-directional transition rate observed between axile and parietal placentation in our study supports the strong possibility that parietal placentation is derived from axile. Although parietal placentation appears to be derived from axile placentation in most cases (by retraction of the septa), the opposite should also be considered, such as intruding parietal placentae becoming axile. For example, in many families the septa do not fuse completely in the center of ovary and the term “incompletely septate with centre-angled (axile) placentation” is used (Leins and Erbar, 2010). Others have termed this condition parietal (in the upper part of the ovary) to axile (in the lower part) with intruded parietal placentae, i.e., in Gesneriaceae (Weber, 1971). This type of placentation condition (upper parietal, lower axile with intruded parietal placentae) is found to occur in taxa within 30 different families (Achariaceae, Passifloraceae, Lacistemataceae, etc.) (Soltis et al., 2011). Thus, it is believed that in taxa within many families axile is derived from parietal placentation. Parietal placentation may also be a non-homologous state, derived from apocarpous gynoecia (parasyncarpous ovaries) or from syncarpous axile ovaries (possibly in Annonaceae and Berberidopsidaceae). Further, our findings suggest that the origin and high radiation of axile placentation mostly coincides with the evolution of monocots. In dicots, axile placentation represents an independent evolution from apocarpous ancestors (Remizowa et al., 2010). The absence of axile placentation in early branching/early diverging lineages of angiosperms, but its prevalence associated with the spread and radiation of flowering plants, also supports the view that axile placentation is the most advanced (Figure 2 and Supplementary Figure 1).
The basal and apical placentation can also develop in different ways which are not necessarily homologous. Apical placentation is confusing and difficult to delimit from axile placentation or from laminar placentation, and it can be the result of a shift of ovules to the top of the ovary by the growth of a central column but can also be the result of a partial reduction in the number of ovules. Furthermore, basal placentation linked with an apocarpous ovary is not derived in the same way as basal placentation linked with a syncarpous ovary. Because of these limitations it is difficult to accurately infer the evolutionary trends of different placentation types in flowering plants. Further, the shift from axile, parietal, basal, and apical placentation to marginal can be explained by multiple derived origin of apocarpous gynoecium from either syncarpous gynoecia or basally connate carpels in many families of monocots (Doyle and Endress, 2000; Chen et al., 2004; Remizowa et al., 2010; Rudall et al., 2011; Sokoloff et al., 2013) and core eudicots (Endress et al., 1983). The general evolutionary trend of the angiosperms from apocarpy toward syncarpy is reversed at least in these groups (Endress et al., 1983; Doyle and Endress, 2000; Chen et al., 2004; Remizowa et al., 2010; Rudall et al., 2011). For example, in Coryphoid palms apocarpy has arisen independently four times from syncarpy (Rudall et al., 2011). In Alismatidae apocarpy has evolved either from basally connate carpels or from syncarpy (Chen et al., 2004). Therefore, it is possible that the shift from placentation types associated with syncarpous gynoecia (axile, parietal, basal, and apical) to marginal placentation is linked to changes from syncarpy to apocarpy (Endress et al., 1983; Sokoloff et al., 2013).
The most interesting aspect of our results is that phylogenetic analyses provide general validation of the evolutionary trends proposed by Puri (1952) and Stebbins (1974). Puri (1952) suggested that evolution of placentation in flowering plants is complex and progressed in several directions. In contrast, Stebbins (1974) argued that placentation evolution is uniform and has proceeded in a single direction. Our results indicate that placentation evolution in flowering plants is neither uniform nor unidirectional as suggested by Stebbins (1974). Rather, as suggested by Puri (1952), placentation evolution seems to have progressed along several lines. Although our estimated directional transition rate shift between different placentation types supported the trends proposed by both Puri (1952; Figure 1A) and Stebbins (1974; Figure 1B), our results are more in agreement with Puri (1952) than with Stebbins (1974).
Our results provide strong evidence that axile placentation acted as the evolutionary source from which various other placentation types restricted to syncarpous gynoecium have evolved. Puri (1952) also suggested that apical, basal, parietal, and free central placentation are mostly derived from axile placentation, and he speculated on the possible evolution of basal and apical placentation also from marginal placentation.
Placentation Association With Ovule Number and Other Traits
Our phyloANOVA results revealed that, despite strong evolutionary constraints on ovule number, there is a strong association between placentation type and ovule number/locule (Figure 4) across flowering plant species. In an elegant recent study, Ronse de Craene (2021) has also shown relationship between ovule number and placentation type in Caryophyllales. We observed that parietal placentation was always associated with high ovule number (multi-ovulate ovaries), followed by axile, laminar, and marginal placentation. In contrast, basal, apical, and free central placentation was associated with one or few ovules.
Unilocular ovaries with single ovule show basal or apical placentation. The increase in ovule number among angiosperms with parietal placentation is concomitant with an evolutionary pathway leading to paracarpous gynoecia (characterized by carpels fused into a unilocular ovary with parietal placentas) via eusyncarpous forms (characterized by carpels fused at the center of the gynoecium and usually forming a plurilocular ovary with axile placentas) (Endress, 1994). The evolutionary transition to high ovule number is evident among many closely related families with constant carpel number such as Scrophulariaceae to Orobanchaceae; Scrophulariaceae to Gesneriaceae; Ericaceae to Pyrolaceae/Monotropaceae; Cypripediaceae/Apostasiaceae to Orchidaceae. All these families have constant carpel number and transition occurs from axile/apical to parietal placentation with increase in ovule number per locule. Thus, the evolution of placentation is associated with increase or decrease of ovule number.
Kress (1981) suggested that the evolution of uniovulate and multiovulate ovaries in angiosperms may be understood in terms of competitive interactions among the ovules or developing seeds. Bawa (2016) postulated that plants may reduce competition among developing seeds either by reducing the number of ovules to one or by increasing the genetic relatedness of seeds within a multiovulate ovary via the evolution of specialized pollination mechanisms that deliver many pollen grains from a single pollen parent. Thus, various placentation types can be viewed as a means to reduce competition among ovules. Both Puri (1952) and Stebbins (1974) also invoked resource allocation and, implicitly, competition within the ovary as the selective force in the diversification of placentation types. Axile placentation prevalent in angiosperms isolates ovules in a multilocular ovary, thereby reducing deleterious effects of competition. Similarly, septa often isolate developing seeds in ovaries with marginal placentation. Indeed, septa can also reduce the spread of pathogens and parasites from one part of the ovary to another.
What then explains the evolution of parietal and free central placentation offering no possibility for isolation? Our analysis, as also noted by Puri (1952) and Stebbins (1974), shows that free central placentation is rare, usually has few ovules and only occasionally many ovules (as in some Primulaceae and Lentibulariaceae). Deleterious effects of competition in parietal placentation may be reduced by kin selection, as for example in orchid flowers in which all the ovules are sired by a single pollinium. Thus, all the developing seeds within a flower are full sibs, paving the way for kin selection and reducing the negative consequences of sibling competition.
Kin cooperation should depend on communication among developing ovules within the ovary. There is growing evidence that even unrelated plants can communicate to confer benefits to the group of individuals cooperating with each other (Karban, 2021). Communication is implicit in studies demonstrating recognition of kin in competition studies dealing with seedlings from full and half sib families (Dudley and File, 2007; Biedrzycki et al., 2010; File et al., 2012; Anten and Chen, 2021). Signaling pathways involving gibberellins that can modulate ovule number exist in ovaries (Gomez et al., 2018) and there is a whole body of literature on how even with a developing ovule, different combinations of paternal and maternal genomes may determine the outcome of competitive interactions (Haig, 2013). A negative effect of kin selection within the ovary could be a reduction in the genetic variance of progeny within an ovary due to the full sibship of hundreds or thousands of seeds. However, genetic variation within the progeny of a single plant occurs not only at the level of flowers but also among flowers, and in the case of large plants, probably among different branches.
Apart from fertilization of flowers by a single pollen donor, self-pollination can also increase sibship. Short development time between fertilization and seed maturation could also minimize competitive interactions by reducing the time available for competition. It should be interesting to determine if there is a disproportionate representation of species with parietal placentation characterized by either specialized pollination modes, selfing, or short development time between fertilization and seed maturation.
Interestingly, we observed that basal, parietal, and axile placentation are more persistent placentation types in angiosperms, thus providing evidence for bimodal distribution pattern of both placentation and ovule number across flowering plants. Ganeshaiah and Shaanker (1992) also showed that seed number per fruit in the British flora shows a bimodal distribution, grouping species with one or a few seeds per fruit and species with many seeds per fruit. Bawa et al. (2019) showed the same trend in monocotyledons. Our results on ovule number and placentation types further confirm the trends for angiosperms as a whole. Together, these findings provide growing evidence for flowering plants falling into two categories based on two alternative sets of associated traits, starting from ovule number to placentation to pollination mechanisms and possibly to sexual systems – all explained in the context of kin selection (Bawa, 2016).
The type of data and the analyses and the number of taxa used considerably influence the results and interpretation of our as well as other studies. In the present study, analyses were done at family level mostly using placentation data of single taxa per family. Therefore, incomplete sampling of placentation data may influence inferred results and interpretation of placentation evolution in angiosperms in the present study. It should also be noted that the results and interpretation of the present study also strongly depend on number of distinct placentation types recognized and the placentation classification scheme used. However, we believe our results are as robust, if not more, than the earlier studies (Puri, 1952; Ickert-Bond et al., 2014). The development of various placentation types is strongly influenced by reproductive traits such as ovule number, position, shape, gynoecium structural type, carpel fusion, postgenital fusion pattern, etc. Therefore, future work should focus on analyzing these traits along with placentation in comparative phylogenetic framework to gain deeper understanding of placentation evolution in flowering plants. Finally, the comparative phylogenetic analysis framework used here can also be used to study the evolution of other reproductive and morphological traits in seed plants and can provide useful insights about their origin and evolutionary trends across flowering plants.
Data Availability Statement
The original contributions presented in the study are included in the article/Supplementary Material, further inquiries can be directed to the corresponding author.
Author Contributions
KSB and KNS conceived the idea and designed the study. KNS collected the data and conducted the analyses. Both authors discussed the methods and results and wrote the manuscript.
Funding
This work was supported by National Science Foundation, USA-OPUS grant (NSF-OPUS 1354043) to KSB, and the Bawa Family Fund Fellowship to KNS.
Conflict of Interest
The authors declare that the research was conducted in the absence of any commercial or financial relationships that could be construed as a potential conflict of interest.
Publisher’s Note
All claims expressed in this article are solely those of the authors and do not necessarily represent those of their affiliated organizations, or those of the publisher, the editors and the reviewers. Any product that may be evaluated in this article, or claim that may be made by its manufacturer, is not guaranteed or endorsed by the publisher.
Acknowledgments
We thank Ashoka Trust for Research in Ecology and Environment (ATREE) for providing computation facility to undertake comparative phylogenetic analysis for the study.
Supplementary Material
The Supplementary Material for this article can be found online at: https://www.frontiersin.org/articles/10.3389/fevo.2022.784077/full#supplementary-material
Footnotes
- ^ http://www.theplantlist.org/
- ^ http://www.mobot.org/MOBOT/research/APweb/
- ^ http://datadryad.org/resource/doi:10.5061/dryad.63q27.2/3.1
- ^ http://www.evolution.reading.ac.uk/BayesTraitsV4.0.0/BayesTraitsV4.0.0.html
References
Anten, N. P., and Chen, B. J. (2021). Detect thy family: mechanisms, ecology and agricultural aspects of kin recognition in plants. Plant Cell Environ. 44, 1059–1071. doi: 10.1111/pce.14011
Bailey, I. W., and Swamy, B. G. L. (1951). The conduplicate carpel of dicotyledons and its initial trends of specialization. Am. J. Bot. 38, 373–379. doi: 10.2307/2437826
Bapst, D. W. (2012). paleotree: an R package for paleontological and phylogenetic analyses of evolution. Methods Ecol. Evol. 3, 803–807. doi: 10.1111/j.2041-210X.2012.00223.x
Bawa, K. S. (2016). Kin selection and the evolution of plant reproductive traits. Proc. R. Soc. B. Sci. 283:20160789. doi: 10.1098/rspb.2016.0789
Bawa, K. S., Ingty, T., Revell, L. J., and Shivaprakash, K. N. (2019). Correlated evolution of flower size and seed number in flowering plants (monocotyledons). Ann. Bot. 123, 181–190. doi: 10.1093/aob/mcy154
Biedrzycki, M. L., Jilany, T. A., Dudley, S. A., and Bais, H. P. (2010). Root exudates mediate kin recognition in plants. Commun. Integr. Biol. 3, 28–35. doi: 10.4161/cib.3.1.10118
Blomberg, S. P., Garland Jr, T., and Ives, A. R. (2003). Testing for phylogenetic signal in comparative data: behavioral traits are more labile. Evolution 57, 717–745. doi: 10.1111/j.0014-3820.2003.tb00285.x
Bollback, J. P. (2006). SIMMAP: stochastic character mapping of discrete traits on phylogenies. BMC Bioinformatics 7:88. doi: 10.1186/1471-2105-7-88
Carlsward, B. S., Judd, W. S., Soltis, D. E., Manchester, S. R., and Soltis, P. S. (2011). Putative morphological synapomorphies of Saxifragales and their major subclades. J. Bot. Res. Inst. Tex. 5, 179–196.
Carta, A., Bedini, G., and Peruzzi, L. (2020). A deep dive into the ancestral chromosome number and genome size of flowering plants. New Phytol. 228, 1097–1106. doi: 10.1111/nph.16668
Chase, M. W., Christenhusz, M. J. M., Fay, M. F., Byng, J. W., Judd, W. S., Soltis, D. E., et al. (2016). An update of the Angiosperm Phylogeny Group classification for the orders and families of flowering plants: APG IV. Bot. J. Linn. Soc. 181, 1–20. doi: 10.1111/boj.12385
Chen, J. M., Chen, D., Gituru, W. R., Wang, Q. F., and Guo, Y. H. (2004). Evolution of apocarpy in Alismatidae using phylogenetic evidence from chloroplast rbcL gene sequence data. Bot. Bull. Acad. Sin. 45, 33–40.
Chomicki, G., Coiro, M., and Renner, S. S. (2017). Evolution and ecology of plant architecture: integrating insights from the fossil record, extant morphology, developmental genetics, and phylogenies. Ann. Bot. 120, 855–891. doi: 10.1093/aob/mcx113
Doyle, J. A., and Endress, P. K. (2000). Morphological phylogenetic analysis of basal angiosperms: comparison and combination with molecular data. Int. J. Plant Sci. 161, S121–S153. doi: 10.1086/317578
Dudley, S. A., and File, A. L. (2007). Kin recognition in an annual plant. Biol. Lett. 3, 435–438. doi: 10.1098/rsbl.2007.0232
Endress, P. K. (1994). Diversity and Evolutionary Biology of Tropical Flowers. Cambridge: Cambridge University Press.
Endress, P. K. (2011a). Angiosperm ovules: diversity, development, evolution. Ann. Bot. 107, 1465–1489. doi: 10.1093/aob/mcr120
Endress, P. K. (2011b). “Changing views of flower evolution and new questions,” in Flowers on the Tree of Life, eds L. Wantorp and L. P. Ronse De Craene (Cambridge: Cambridge University Press), 120–141. doi: 10.1017/cbo9781139013321.005
Endress, P. K. (2015). Patterns of angiosperm development before carpel sealing across living angiosperms: diversity, and morphological and systematic aspects. Bot. J. Linn. Soc. 178, 556–591. doi: 10.1111/boj.12294
Endress, P. K., Davis, C. C., and Matthews, M. L. (2013). Advances in the floral structural characterization of the major subclades of Malpighiales, one of the largest orders of flowering plants. Ann. Bot. 111, 969–985. doi: 10.1093/aob/mct056
Endress, P. K., and Doyle, J. A. (2009). Reconstructing the ancestral flower and its initial specializations. Am. J. Bot. 96, 22–66. doi: 10.3732/ajb.0800047
Endress, P. K., and Igersheim, A. (2000). The reproductive structures of the basal angiosperm Amborella trichopoda (Amborellaceae). Int. J. Plant Sci. 161, S237–S248. doi: 10.1086/317571
Endress, P. K., Jenny, M., and Fallen, M. E. (1983). Convergent elaboration of apocarpous gynoecia in higher advanced dicotyledons (Sapindales, Malvales, Gentianales). Nord. J. Bot. 3, 293–300.
Endress, P. K., and Matthews, M. L. (2012). Progress and problems in the assessment of flower morphology in higher-level systematics. Plant Syst. Evol. 298, 257–276. doi: 10.1007/s00606-011-0576-2
Engler, A. (1909). Syllabus Der Pflanzenfamilien, 6th Edn. (Stuttgart: Gebrüder Borntraeger Verlag), 254.
File, A. L., Klironomos, J., Maherali, H., and Dudley, S. A. (2012). Plant kin recognition enhances abundance of symbiotic microbial partner. PLoS One 7:e45648. doi: 10.1371/journal.pone.0045648
Friis, E. M., Pedersen, K. R., von Balthazar, M., Grimm, G. W., and Crane, P. R. (2009). Monetianthus mirus gen. et sp. nov., a nymphaealean flower from the early cretaceous of portugal. Int. J. Plant Sci. 170, 1086–1101. doi: 10.1086/605120
Fu, Q., Diez, J. B., Pole, M., Garcıa-Avila, M., Liu, Z. J., Chu, H., et al. (2018). An unexpected noncarpellate epigynous flower from the Jurassic of China. eLife 7:e38827. doi: 10.7554/eLife.38827
Ganeshaiah, K. N., and Shaanker, R. U. (1992). Frequency distribution of seed number per fruit in plants: A consequence of the self-organizing process? Curr. Sci. 1925, 359–365.
Garland, T., Dickerman, A. W., Janis, C. M., and Jones, J. A. (1993). Phylogenetic analysis of covariance by computer simulation. Syst. Biol. 42, 265–292. doi: 10.1093/sysbio/42.3.265
Gauthier, R. (1950). The Nature of the Inferior Ovary in the Genus Begonia. Quebec: Université de Montréal.
Gomez, M. D., Barro-Trastoy, D., Escoms, E., Saura-Sánchez, M., Sánchez, I., Briones-Moreno, A. et al. (2018). Gibberellins negatively modulate ovule number in plants. Development 145:dev163865. doi: 10.1242/dev.163865
Gundersen, A. (1939). Flower buds and phylogeny of the dicotyledons. Bull. Torrey Bot. Club 66, 287–295. doi: 10.2307/2480851
Hacerup, O. (1938). On the origin of some angiosperms through Gnetales and the Coniferae. III. The gynaeceum of Salix cinerea. Biol. Medd. Dan. Vid. Selsk. 14, 1–34.
Hagerup, O. (1939). On the origin of some angiosperms through the Gnetales and the Coniferales. IV. The gynaeceum of Personatae. Kongel. Danske Videnskabernes Selskab Biol. Medd. 15, 1–39.
Haig, D. (2013). Kin conflict in seed development: an interdependent but fractious collective. Annu. Rev. Cell Dev. Biol. 29, 189–211. doi: 10.1146/annurev-cellbio-101512-122324
Huelsenbeck, J. P., Nielsen, R., and Bollback, J. P. (2003). Stochastic mapping of morphological characters. Syst. Biol. 52, 131–158. doi: 10.1080/10635150390192780
Ickert-Bond, S. M., Gerrath, J., and Wen, J. (2014). Gynoecial structure of Vitales and implications for the evolution of placentation in the rosids. Int. J. Plant Sci. 175, 998–1032. doi: 10.1086/678086
Igersheim, A., and Endress, P. K. (1998). Gynoecium diversity and systematics of the paleoherbs. Bot. J. Linn. Soc. 127, 289–370. doi: 10.1111/j.1095-8339.1998.tb02102.x
Joshi, A. C. (1947). “The morphology of the gynaeceum,” in Proceedings of the 34th Indian Science Congress Session, Delhi, 1–18.
Karban, R. (2021). Plant communication. Annu. Rev. Ecol. Evol. Syst. 52, 1–24. doi: 10.1146/annurev-ecolsys-010421-020045
Kress, W. J. (1981). Sibling competition and evolution of pollen unit, ovule number, and pollen vector in angiosperms. Syst. Bot. 6, 101–112. doi: 10.2307/2418541
Leins, P., and Erbar, C. (2010). Flower and Fruit: Morphology, Ontogeny, Phylogeny. Function and Ecology. Stuttgart: Schweizerbart Science Publishers.
Lindley, J. (1830). An Introduction to the Natural System of Botany: Or, A Systematic View of the Organisation, Natural Affinities, and Geographical Distribution, of the Whole Vegetable Kingdom: Together with the uses of the Most Important Species in Medicine, the Arts, and Rural or Domestic Economy. London: Longman, Rees, Orme, Brown, and Green.
Lindsey, A. A. (1940). Floral anatomy in the Gentianaceae. Am. J. Bot. 27, 640–652. doi: 10.2307/2436985
Maddison, W., and Maddison, D. (2011). Mesquite: A Modular System for Evolutionary analysis. Mesquite. v. 2.75. Available online at: http://www.mesquiteproject.org/ (accessed June 23, 2020).
Mohr, B. A., Bernardes-de-Oliveira, M. E., and Taylor, D. W. (2008). Pluricarpellatia, a nymphaealean angiosperm from the lower cretaceous of northern Gondwana (Crato Formation. Brazil). Taxon 57, 1147–1158. doi: 10.1002/tax.574009
Mori, S. A., Smith, N. P., Huang, Y. Y., Prance, G. T., Kelly, L. M., and Matos, C. C. (2015). Toward a phylogenetic-based generic classification of neotropical lecythidaceae—II. status of allantoma, cariniana, couratari, couroupita, grias and gustavia. Phytotaxa 203, 122–137. doi: 10.11646/phytotaxa.203.2.2
Nardi, K. D., Campbell, L. M., and Oriani, A. (2021). Inferences on gynoecium evolution in Xyris (Xyridaceae, Poales) based on floral anatomy and development. Bot. J. Linn. Soc. 397, 396–419. doi: 10.1093/botlinnean/boab031
Nielsen, R. (2002). Mapping mutations on phylogenies. Syst. Biol. 51, 729–739. doi: 10.1080/10635150290102393
Pagel, M. (1999). Inferring the historical patterns of biological evolution. Nature 401, 877–884. doi: 10.1038/44766
Pagel, M., and Meade, A. (2006). Bayesian analysis of correlated evolution of discrete characters by reversible-jump Markov chain Monte Carlo. Am. Nat. 167, 808–825. doi: 10.1086/503444
Puri, V. (1961). The classical concept of angiosperm carpel: a reassessment. J. Indian Bot. Soc. 40, 511–524.
R Development Core Team (2013). R: A Language and Environment for Statistical Computing. Vienna: R Foundation for Statistical Computing.
Remizowa, M. V., Sokoloff, D. D., and Rudall, P. J. (2010). Evolutionary history of the monocot flower1. Ann. Mo. Bot. Gard. 97, 617–645. doi: 10.3417/2009142
Revell, L. J. (2012). phytools: an R package for phylogenetic comparative biology (and other things). Methods Ecol. Evol. 3, 217–223. doi: 10.1111/j.2041-210X.2011.00169.x
Rohweder, O. (1967). Centrospermen-Studien. 3. blütenentwicklung und blütenbau bei silenoideen (Caryophyllaceae). Bot. Jahrb. Syst. 86, 130–185.
Rohweder, O., and König, K. (1971). Centrospermen-Studien. 5. Bau der Blüten, Früchte und Samen von Pteranthus dichotomus Forssk. (Caryophyllaceae). Bot. Jahrb. Syst. 90, 447–468.
Rohweder, O., and Urmi-König, K. (1975). Centrospermen-Studien: beiträge zur morphologie, anatomie und systematischen stellung von Gymnocarpos Forssk. und Paronychia argentea Lam. (Caryophyllaceae). Bot. Jahrb. Syst. 96, 375–409.
Ronse De Craene, L. P. (2010). Floral Diagrams: an Aid to Understanding Flower Morphology and Evolution. Cambridge: Cambridge University Press.
Ronse de Craene, L. P. (2021). Gynoecium structure and development in core Caryophyllales: a matter of proportions. Bot. J. Linn. Soc. 195, 437–466. doi: 10.1093/botlinnean/boaa048
Rudall, P. J., Ryder, A., and Baker, W. J. (2011). Comparative gynoecium structure and multiple origins of apocarpy in coryphoid palms (Arecaceae). Int. J. Plant Sci. 172, 674–690. doi: 10.1086/659459
Sauquet, H., von Balthazar, M., Magallón, S., Doyle, J. A., Endress, P. K., Bailes, E. J., et al. (2017). The ancestral flower of angiosperms and its early diversification. Nat. Commun. 8:16047. doi: 10.1038/ncomms16047
Sokoloff, D. D., Remizowa, M. V., and Rudall, P. J. (2013). “Is Syncarpy an Ancestral Condition in Monocots and Core Eudicots,” in Early Events in Monocot Evolution, eds P. Wilkin and S. J. Mayo (Cambridge: Cambridge University Press), 60–81. doi: 10.1017/cbo9781139002950.004
Soltis, D. E., Smith, S. A., Cellinese, N., Wurdack, K. J., Tank, D. C., Brockington, S. F., et al. (2011). Angiosperm phylogeny: 17 genes, 640 taxa. Am. J. Bot. 98, 704–730. doi: 10.3732/ajb.1000404
Stebbins, G. L. (1974). Flowering Plants: Evolution Above the Species Level. London: Arnold, xviii, 399.
Takhtajan, A. (1942). The structural types of gynoecium and placentation. Bull. Armen. Branch Acad. Sci. USSR 3, 91–112.
Takhtajan, A. L. (1991). Evolutionary Trends in Flowering Plants. New York, NY: Columbia University Press.
Taylor, D. W., and Li, H. (2018). Paleobotany: Did flowering plants exist in the Jurassic period? eLife 7:e43421. doi: 10.7554/eLife.43421
Wang, X., and Zheng, X. T. (2012). Reconsiderations on two characters of early angiosperm Archaefructus. Palaeoworld 21, 193–201. doi: 10.1016/j.palwor.2012.10.002
Wilkinson, A. M. (1944). Floral anatomy of some species of Cornus. Bull. Torrey Bot. Club 71, 276–301. doi: 10.2307/2481708
Wilkinson, A. M. (1949). Floral anatomy and morphology of Triosteum and of the Caprifoliaceae in general. Am. J. Bot. 86, 481–489. doi: 10.2307/2438083
Willis, C. G., Baskin, C. C., Baskin, J. M., Auld, J. R., Venable, D. L., Cavender-Bares, J., et al. (2014). The evolution of seed dormancy: environmental cues, evolutionary hubs, and diversification of the seed plants. New Phytol. 203, 300–309. doi: 10.1111/nph.12782
Zanne, A. E., Tank, D. C., Cornwell, W. K., Eastman, J. M., Smith, S. A., FitzJohn, R. G., et al. (2014a). Three keys to the radiation of angiosperms into freezing environments. Nature 506, 89–92. doi: 10.1038/nature12872
Keywords: placentation, evolutionary trend, angiosperms, ancestral state, phylogenetic signal, synapomorphy, kin selection
Citation: Shivaprakash KN and Bawa KS (2022) The Evolution of Placentation in Flowering Plants: A Possible Role for Kin Selection. Front. Ecol. Evol. 10:784077. doi: 10.3389/fevo.2022.784077
Received: 27 September 2021; Accepted: 11 February 2022;
Published: 08 March 2022.
Edited by:
Marie-Stéphanie Samain, Instituto de Ecología (INECOL), MexicoReviewed by:
Louis Philippe Ronse De Craene, Royal Botanic Garden Edinburgh, United KingdomXin Wang, Nanjing Institute of Geology and Paleontology (CAS), China
Lorenzo Peruzzi, University of Pisa, Italy
Copyright © 2022 Shivaprakash and Bawa. This is an open-access article distributed under the terms of the Creative Commons Attribution License (CC BY). The use, distribution or reproduction in other forums is permitted, provided the original author(s) and the copyright owner(s) are credited and that the original publication in this journal is cited, in accordance with accepted academic practice. No use, distribution or reproduction is permitted which does not comply with these terms.
*Correspondence: Kamaljit S. Bawa, a2FtYWwuYmF3YUB1bWIuZWR1