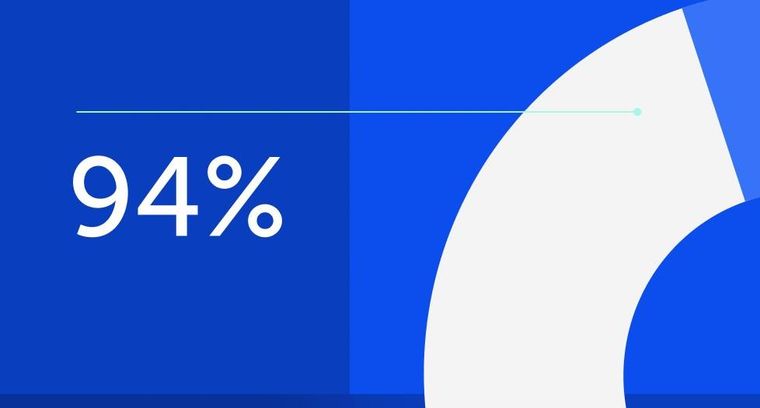
94% of researchers rate our articles as excellent or good
Learn more about the work of our research integrity team to safeguard the quality of each article we publish.
Find out more
ORIGINAL RESEARCH article
Front. Ecol. Evol., 08 March 2022
Sec. Paleoecology
Volume 10 - 2022 | https://doi.org/10.3389/fevo.2022.768464
This article is part of the Research TopicLessons from the Past: Linking the Paleofire Record and Fire Management in the Context of a Warming WorldView all 7 articles
The spatial component of past forest fires in temperate Europe has been little studied, despite the value of such data in quantifying human and natural factors driving fire activity and associated forest dynamics. Changes in fire regimes reported across a range of ecosystems call for a better understanding of variability in historic fires and may help define reference points that can be relied upon when discussing climate change effects. We provide the first dendrochronological reconstruction of historical fire sizes in Central Europe and analyze the minimum extent of fires during the last four centuries in a 9.2 km2 (920 ha) conifer-dominated section of Białowieża Forest, one of the largest continuous lowland forests of the subcontinent. We recorded 82 fires between 1666 and 1946, using 275 sample trees, while 92% of fires (76 out of 82) spread beyond the studied area. Fires varied considerably in size, from events recorded at only one site (1–200 ha) to fires recorded in more than half of the studied area, thus exceeding 500 ha in size. The fire cycle was 11 years over the whole study period, with three distinct periods revealed by the regime shift analysis. In the years 1670–1750, the fire cycle averaged 12 years. It shortened to 7 years between 1755–1840 and increased to 22 years over the 1845–1955 period. In comparison with present day data, the reconstructed fire density of 3.2 fires per 100 km2 (10 000 ha) and year exceeded lightning ignition density by one to two orders of magnitude, suggesting a significant contribution of human-related ignitions. Our results highlight the important role of fire disturbance in Białowieża Forest and provide critical baseline information to design biological conservation strategies for European forests.
Spatial patterns of fire disturbance is a critical feature of fire regimes, controlling their ecological and biochemical impacts at the stand, landscape, and regional scales (Niklasson and Granström, 2000; Beaty and Taylor, 2008). Climatic conditions, topography, and forest structure control the abundance, spatial distribution, and water content of forest fuels. Together with ignition patterns, these factors determine fire occurrence, spread, and severity (Agee, 1993; Taylor and Skinner, 1998; Beaty and Taylor, 2001). Human activities (land use in particular) significantly impact both fuels and ignitions, modifying the natural fire activity (Niklasson and Granström, 2000; Granström and Niklasson, 2008).
Recent changes in fire regimes documented across a range of forest ecosystems (Pausas and Keeley, 2009; Drobyshev et al., 2017) call for a better understanding of factors shaping fire activity within specific regions. In Europe, the number of tree ring studies on such detailed fire regime characteristics like frequency, seasonality, and severity of fires is increasing constantly (Lehtonen et al., 1996; Blanck et al., 2013; Drobyshev et al., 2014; Zin et al., 2015). However, spatially explicit reconstructions are few. They predominantly cover the northerly and southerly sections of European forests (Niklasson and Granström, 2000; Christopoulou et al., 2013; Storaunet et al., 2013; Rolstad et al., 2017; Kitenberga et al., 2019; Ryzhkova et al., 2020; cf. Szymczak et al., 2020). To our knowledge, such studies are missing in the case of temperate Europe.
Spatial tree ring reconstruction of fire occurrence combines information on the fire dates and the exact location of the dated wood samples. The presence of dendrochronologically datable material (dead and living fire-scarred wood) remains one of the main challenges in expanding the network of spatially explicit fire reconstructions. In temperate Europe, the scarcity of old-growth forests and limited availability of old trees and deadwood with fire scars reflects a legacy of long and intensive forest use (Pyne, 1997; Kaplan et al., 2009). In contrast to some of the North American forests, dominated by short-needled conifers and featuring stand-replacing fires that leave little evidence in the form of scarred trees (Kipfmueller and Kupfer, 2005; Kitzberger et al., 2007; Margolis and Balmat, 2009), mixed-severity fires likely dominated in European forests (Blanck et al., 2013; Zin et al., 2015). It is reasonable, therefore, to assume that generally low availability of scarred wood in this continent is not a result of naturally low levels of tree scarring, as one would suggest for some sections of the North American domain (Johnson, 1992; Agee, 1993).
The role of fire in affecting the dynamics of temperate European forests has received limited attention in the past (Walter, 1968; Faliński, 1986; Ellenberg, 1996). Insufficient empirical data on past fire disturbances, a negligible influence of lightning ignition upon modern fire activity (Ubysz and Szczygieł, 2006; Szczygieł et al., 2016; Brackhane et al., 2021), and consideration of European temperate zone as deciduous-dominated and less flammable domain (Ellenberg, 1996; Leuschner and Ellenberg, 2017b) have contributed to the view upon natural fires as very rare events in this region (Leuschner and Ellenberg, 2017a). In fact, a large part of the Central European temperate zone is dominated by flammable conifer forests (Brus et al., 2012; San-Miguel-Ayanz et al., 2021). However, long-term and efficient fire suppression (Granström and Niklasson, 2008; Castellnou et al., 2010; Wallenius, 2011) and high human ignition frequencies (Szczygieł et al., 2016; San-Miguel-Ayanz et al., 2021) resulted in the notion of fire being largely driven by humans. The role of fire as an important driver of natural forest dynamics in this part of Europe has, therefore, been generally disregarded.
Paleoecological data from Central Europe suggested fire as one of the factors affecting vegetation development during the Holocene, long before the rise of civilizations based on agriculture (Rösch, 2000). These reconstructions have promoted discussion on the openness of European forests (Vera, 2000; Svenning, 2002; Mitchell, 2005; Bobek et al., 2018; Feurdean et al., 2020) and the human impact on vegetation (Bradshaw and Sykes, 2014; Leuschner and Ellenberg, 2017a). The development gave momentum to the debate of various disturbances as drivers of ecosystem dynamics in the continent. Several paleoecological studies have documented fires in the European temperate forests in the past (Tinner et al., 2005; Novák et al., 2012; Adámek et al., 2015; Latałowa et al., 2015, 2016; Zimny et al., 2017; Feurdean et al., 2020). Although forming very solid evidence of biomass combustion in general, these charcoal-based records provided little insight into specific characteristics of fire regimes, such as fire seasonality, frequency, and fire size. Taphonomy, sediment mixing, diversity of deposition pathways, and landscape morphologies add to the complexity in the reconstruction of the fire regime with paleoecological data (Tolonen, 1986; Clark, 1988; Clark et al., 1998; Ohlson and Tryterud, 2000). Dendrochronological reconstructions may offer detailed data on fire regime characteristics, yet the number of existing studies and the knowledge on historical variability of fire regimes in temperate Europe remains limited (Niklasson et al., 2010; Zin et al., 2015; Spînu et al., 2020), especially in regard to fire sizes.
Białowieża Forest is one of the few existing lowland old-growth woodlands in Europe with primary character (Sabatini et al., 2018). It is regarded as a reference landscape for the region (Faliński, 1986; Ellenberg, 1996; Peterken, 1996; Jaroszewicz et al., 2019). The area enjoyed the status of a royal hunting ground since the 14th century. This helped to avoid its transformation into farmland and maintain its high conservation value (Hedemann, 1939; Schama, 1996; Samojlik, 2005, 2010; Samojlik et al., 2013). Białowieża Forest hosts an outstanding diversity of organisms, including its symbol, European bison Bison bonasus L., and other critically endangered species (Gutowski and Jaroszewicz, 2001; Krzyściak-Kosińska et al., 2012). Although partly affected by modern forest management throughout the 20th–21st centuries, Białowieża Forest encompasses many sections with a very high degree of naturalness, featuring veteran trees (often of large dimensions) and deadwood continuity (Jaroszewicz et al., 2019). These characteristics make this area a valuable site for dendroecological research, which is unique for the Central European region (Niklasson et al., 2010, and literature therein).
Fire-related dendrochronological studies in Białowieża Forest have focused on fire occurrence, stand-level return intervals, fire severity, and the role of fire in shaping tree demography (Niklasson et al., 2010; Zin et al., 2015; Spînu et al., 2020). Paleoecological reconstructions using charcoal data from peat bogs have documented the presence of fire over millennia (Dąbrowski, 1959; Mitchell and Cole, 1998; Latałowa et al., 2015, 2016; Zimny et al., 2017). The current study aims to expand the knowledge of fire activity in Białowieża Forest through reconstruction of the minimum sizes of the past fires in the conifer section of this area. We hypothesized that (H1) fires typically exceeded the size of single stands (c. 101 ha), (H2) fires were of highly variable spatial extent, and (H3) historical fires occurred at frequencies exceeding frequency of lightning-caused ignitions.
Białowieża Forest, recognized as one of the best-preserved temperate forests on the European Lowland, extends over approximately 1 500 km2 of the borderland between eastern Poland and western Belarus (52°30′–53°N, 23°30′–24°15′E). The area has a warm-summer humid continental climate (Kottek et al., 2006; cf. Jaroszewicz et al., 2019). During the 1955–2001 period, the mean annual precipitation was 633 mm and mean annual temperature was 6.8°C. In the same period, January was the coldest month with a mean temperature of –4.2°C and July was the warmest month with a mean temperature of 17.7°C (Pierzgalski et al., 2002).
The topography of the area is homogeneous and features an old-morainic plateau (135–202 m a.s.l.) which is built from glaciofluvial gravels, clays, and sands. The terrain is locally diversified by small river valleys, melt-out hollows with accumulated peat cover, and an elevated zone of glaciofluvial-kame origin that stretches throughout the central part of the forest and ends at the study area’s highest point – Kozia Góra located in Belarus. The main soil types of Białowieża Forest are oligotrophic podzol and rusty soils, eutrophic and mesotrophic brown and lessivé soils, and a range of gley and organic soil types, including peatbog and semi-bog soils (Kwiatkowski, 1994).
The area is characterized by a mosaic of different forest types. The common tree species are pedunculate oak (Quercus robur L.), small-leaved lime (Tilia cordata Mill.), Norway maple (Acer platanoides L.), hornbeam (Carpinus betulus L.), silver birch (Betula pendula Roth.), black alder (Alnus glutinosa (L.) Gaertn.), ash (Fraxinus excelsior L.), and downy birch (Betula pubescens Ehrh.). Among conifer species, Scots pine (Pinus sylvestris L.), and Norway spruce (Picea abies (L.) H. Karst.) dominate. Białowieża Forest is an area with very high habitat diversity, including mesic and mesotrophic habitats typical for deciduous and mixed deciduous forests, oligotrophic habitats of conifer communities, as well as bog alderwoods and streamside ash-alder forests as water-shaped forest types (Faliński, 1986; Jȩdrzejewska and Jȩdrzejewski, 1998; Sokołowski, 2004; Jaroszewicz et al., 2019).
Today, approximately 44% of the total forest area is covered by old-growth naturally regenerated stands (Jȩdrzejewska and Jȩdrzejewski, 1998; Jaroszewicz et al., 2019). Białowieża Forest encompasses forest stands that are both strictly protected (c. 53%) and managed (c. 47%). Various nature conservation, forest management, and land use regimes are in place, some of which include commercial timber production (Sokołowski, 2004; Krzyściak-Kosińska et al., 2012; Jaroszewicz et al., 2019). The Polish part of Białowieża Forest (approximately 635 km2) consists of the Białowieża National Park (c. 105 km2) and the adjacent managed forest (c. 500 km2), including several smaller nature reserves encompassing approximately 120 km2 in total. The whole Belarusian section of Białowieża Forest is approximately 875 km2, forming Belovezhskaya Pushcha National Park (Sokołowski, 2004; Jaroszewicz et al., 2019).
Twelve sites were used to study the spatial extent of fires in the conifer section of Białowieża Forest during the last four centuries (Table 1). Five of them (P1–P5) were the subject of the stand-scale fire history reconstructions (Zin et al., 2015; Zin, 2016). They were all mixed coniferous (P. sylvestris – P. abies) stands with tree density of approximately 700–800 stems⋅ha−1. Additional seven sites (S1–S7) were situated in neighboring conifer forest on flat terrain and lacked potential fire breaks (streams, bogs, swamps). Only one site (P3) was separated from the other by a small river valley (Figure 1).
Table 1. Characteristics of twelve study sites used to study the spatial extent of fires in the conifer section of Białowieża Forest, Belarus, during the 1666–1946 period (cf. Figure 1).
Figure 1. Location of the 9.2 km2 (920 ha) study area in a conifer-dominated landscape of Białowieża Forest, Belarus, encircled by black solid line (A,B). P1–P5: five study sites that were the subject of the stand-scale fire history reconstructions (Zin et al., 2015; Zin, 2016), located inside (P1–P2), and outside (P3–P5) the study area, denoted by black circles (A,B). S1–S7: seven additional study sites within the study area, depicted by white triangles (B). Map re-drawn after figure in Zin et al. (2015), modified (A).
Ground vegetation at all twelve study sites was composed of Vaccinium myrtillus L., V. vitis-idaea L., Trientalis europaea L., Dryopteris carthusiana (Vill.) H.P. Fuchs and Calamagrostis arundinacea (L.) Roth, and the ground layer by mosses: Pleurozium schreberi (Willd. ex Brid.) Mitt., Ptilium crista-castrensis (Hedw.) De Not., Hylocomium splendens (Hedw.) Schimp., and Dicranum undulatum Schrad. ex Brid. The surrounding stands were mostly conifer forests of analogous type, or humid conifer forests (with some share of Sphagnum spp. in the ground layer) and, occasionally, mixed forests with a higher share of deciduous tree species, like oak, birch, and hornbeam.
There is no previous knowledge from Białowieża Forest nor from temperate Europe that could indicate an appropriate size of the area to be sampled for the study of fire sizes. Earlier stand-scale fire history studies in the area covered 13 ha (Niklasson et al., 2010), 8.5 ha (Zin et al., 2015), and 43 ha (Spînu et al., 2020). It was not possible to delineate any fires in these areas with certainty, suggesting that fires had operated on a scale exceeding the size of those study areas.
Our earlier work in Białowieża Forest has shown a highly uneven distribution of datable wood material under the c. 1 km2 (100 ha) scale (Niklasson et al., 2010; Zin et al., 2015; Spînu et al., 2020). We, therefore, designed a grid of nine study sites aiming at a resolution of c. 1 km2 (100 ha). Collectively, the grid covered the area of 9.2 km2 (920 ha) and represented an area of a size one–two magnitudes higher than the previous stand-scale studies. In an effort to find some indication of the potential spread of very large fires (reaching c. 104 ha in size), we included in this study three additional satellite study sites (P3–P5) located outside the studied area of 9.2 km2 (920 ha) at the distance of c. 3 and 6.5 km. The resulting network of twelve study sites (Table 1) was expected to represent four magnitudes of the spatial fire occurrence: 101, 102, 103, and 104 ha (Figure 1).
In the study sites, we collected partial or full cross-sections from all available Scots pine stumps, logs and snags with fire scars with a chain saw, following the procedure of Arno and Sneck (1977) and McBride (1983). We also sampled all old living pines with fire scars (if such trees were present) and individuals with lowest DBH (diameter at breast height, 1.3 m above the ground) considering them as probable representatives of the youngest populations of that species (Zin et al., 2015). Living trees were sampled with an increment borer. During the field sampling, we did not note fire scars on any tree taxa other than Scots pine.
On each of the seven study sites (S1–S7), we sampled between four and 26 trees, on average 15 trees, with 104 trees in total. Along with the two previously sampled sites (P1 and P2), the total number of trees that were used for fire reconstructions in the studied area of 9.2 km2 (920 ha) reached 275. The comparison satellite sites (P3, P4, and P5) contained an additional 169 sample trees altogether (Table 1).
All wood samples were sanded to enable wood cell visibility under a dissecting microscope with 6–40 × magnification. The tree ring material was visually cross-dated by identifying local pointer years according to standard dendrochronological methodology (Stokes and Smiley, 1968; Yamaguchi, 1991). This allowed us to identify the exact years of fire occurrence. A fire was recorded in a given year and site, if we found at least one fully developed fire scar among all trees sampled in that site (Zin et al., 2015).
We assessed the extent of past fires by developing GIS-assisted reconstruction of the minimum fire areas by analyzing the spatial distribution of study sites, which were either affected (i.e., burned) or not affected (i.e., unburned) by fire in a given year (cf. Niklasson and Granström, 2000) among the population of recording sites. A recording site was a site with tree ring data covering the year in question.
As a burned site for a year, we regarded a study site with at least one sample tree with a fire scar in that year. This might potentially inflate our estimates of burned area as portions of the sites might well have remained unburned. However, this bias is believed to be less of a concern for fires recorded by multiple trees at the same site and by multiple sites. The situation with 1- and 2-year fire intervals recorded in the same tree in Białowieża Forest (cf. Zin et al., 2015) might be an evidence of a very quick fuel build-up. This suggests that fuel amounts in the study area do not limit fire occurrence, like in hemiboreal and boreal Scots pine forests, where the period of 5–10 years seems to be a minimum time span of the post-fire fuel succession which allows for the consecutive fire spread (Lehtonen et al., 1996; Niklasson and Drakenberg, 2001). Because scarring at such short intervals is weak (Dieterich and Swetnam, 1984; Swetnam and Baisan, 1996; Falk et al., 2011, and literature therein) not all trees might be scarred during such fires [see also Piha et al. (2013)].
As an unburned site, we considered a recording site without fire scars dated to the year in question. We drew fire borders halfway between burned and unburned study sites. In areas lacking recording sites, the border was drawn to circumscribe the burned site at the same distance as the shortest of the halfway distances to the existing unburned sites. To develop spatial reconstruction of the minimum fire areas we used QGIS software (v. 2.8.1-Wien; QGIS Development Team).
We calculated fire cycle as the time required to burn an area equivalent to the study area (Reed et al., 1998). To obtain area estimates of burned areas, we calculated the proportion of study sites burned in each fire and reconstructed minimum fire areas as described previously. To this end, we divided the number of study sites which actually recorded a fire (i.e., were burned) in the year in question by the number of recording sites for that year. We then used the following formula:
where: FC is the fire cycle (years), TSA is the total studied area (ha), PS is the time period studied (years) and TBA is the total burned area over this time period (ha). To obtain 10 and 90% confidence limits for the calculated fire cycle values, we resampled with replacement the pool of fire years 1000 times (Ryzhkova et al., 2020).
To study temporal changes in the fire cycle, we applied a regime shift detection algorithm (Rodionov, 2004). It uses sequential t-tests, which diagnose regime change when the cumulative sum of normalized deviations from the mean of a new regime differs from the mean value of the current regime, calculated on a predefined moving time frame. The algorithm is fed with a threshold value, below which the capability of the algorithm to determine a regime change declines (Rodionov, 2004). To increase the ability of the algorithm to detect the short-term changes in fire activity, we set this threshold to 10 years. We also designated the significance level of t-tests to 0.05 and the Huber’s weight parameter, which controlled for weights assigned to the outliers, to 1.
During the 1666–1946 period, we recorded 82 individual fires that included nine cases of two spatially separated fires dated in the same year within the studied area of 9.2 km2 (920 ha). The earliest fire in our analyses (1666) marked the onset of the period when the number of recording sites (i.e., study sites with tree ring chronology) exceeded 50% of the total number of study sites. We documented nine fire years (1702, 1718, 1731, 1732, 1742, 1748, 1828, 1838, 1882) when burned sites were clearly separated by several unburned sites in between and we regarded these years as featuring two separate fires. For 73 fire years, our reconstruction suggested a single fire spreading over the area (Supplementary Figure 1).
The spatial extent of fires, expressed by the number of burned sites, varied from one site up to eight, with the majority (42 of the 82 analyzed) dated at one site (Figure 2). Only six of the fires, all one-site fires, were reasonably contained inside the grid, thus indicating a fire size of up to 200 ha. Figure 3 gives an example of such a fire in 1755. The remaining 76 fires had one or more open borders, their calculated sizes to be regarded as minimum areas burned. By using the GIS-assisted reconstruction, we obtained minimum fire area values that varied from 33 up to c. 800 ha (Figure 4). The three largest fires within the studied area occurred in 1795, 1809, and 1825 (Figure 5), with estimated minimum areas of 700–900 ha. Out of those, the 1795 fire was also documented at the P3 site and the 1809 fire at all of the satellite sites (P3–P5) outside the studied area. In total, eleven of the dated fire years were recorded at the adjacent P3 site c. 3 km from the SE border of the studied area. Twelve of the dated fire years were recorded at the P4 and P5 sites located 6.5 km to the W (Supplementary Figure 1).
Figure 2. Distribution of fire sizes approximated by the number of sites burned during a particular fire within the study area.
Figure 3. An example of a one-site fire in 1755, with the reconstructed area of 188.3 ha (black dashed line) within the 9.2 km2 (920 ha) study area (black solid line). White circles: burned trees, i.e., sample trees which recorded a fire (i.e., had a fire scar) in the year in question, black dots: unburned trees, i.e., sample trees with tree ring chronology covering the year in question, gray solid line: watercourse, dark gray dashed line: forest compartments.
Figure 5. The three largest fires, which were reconstructed within the 9.2 km2 (920 ha) study area (black solid line): in 1795 – reconstructed minimum area of 823.0 ha (A), 1809 – reconstructed minimum area of 844.3 ha (B), and 1825 – reconstructed minimum area of 823.0 ha (C). White circles: burned trees, i.e., sample trees which recorded a fire (i.e., had a fire scar) in the year in question, black dots: unburned trees, i.e., sample trees with tree ring chronology covering the year in question, gray solid line: watercourse, dark gray dashed line: forest compartments, black dashed line: reconstructed fire area.
The cumulative number of fires showed some distinct periods of abrupt increases and relaxations in fire activity (around 1718, 1775, 1803, and 1838) and exhibited a deacrease in fire occurrence which started in the mid-1800s (Figure 6).
The historical fire cycle varied in time. It was 11 years during the 1666–1946 period, the 90% confidence envelope being 8 to 50 years. During 1670–1750, the fire cycle was 12 years (with 90% confidence envelope of 10 to 16 years). During 1755–1840, it shortened to 7 years (with the confidence envelope of 6 to 8 years). During 1845–1955, fire activity declined with the fire cycle being 22 years (with 90% confidence envelope of 15 to 40 years) (Figure 7).
Figure 7. Dynamics of the fire cycle calculated on reconstructed minimum fire areas. The gray line denotes the reconstructed burned areas binned over 5 years and smoothed with loess function. Shifts in the fire cycles, as identified by the regime shift analysis (Rodionov, 2004), are represented by thick red lines.
Over the studied area and the whole period analyzed (1666–1946), the average fire return interval was 3.4 years. This corresponds to 3.2 ignitions per 100 km2 (10 000 ha) and year. The only fire broadly known from historical sources – that of 1811 – was confirmed in our analyzes, burning over half of the studied sites (Supplementary Figure 1).
We present the first spatial reconstruction of the forest fire regime in temperate Europe. It adds a new dimension to the understanding of the long-term forest fire dynamics in this subcontinent. Fires have undoubtedly been inferred over most of the Holocene in the region and in Białowieża Forest from the analyzes of charcoal remains (Dąbrowski, 1959; Mitchell and Cole, 1998; Rösch, 2000; Tinner et al., 2005; Novák et al., 2012; Adámek et al., 2015; Latałowa et al., 2015, 2016; Zimny et al., 2017), with several paleoecological studies particularly discussing their role in shaping Scots pine-dominated forests (Novák et al., 2012; Adámek et al., 2015). However, the knowledge on spatial characteristics of fires and the link up to the present day high-resolution fire monitoring has been lacking. Existing tree ring data from Białowieża Forest produced firm evidence of the key role of fire over the last ∼400 years both in conifer and deciduous communities (Niklasson et al., 2010; Zin et al., 2015; Spînu et al., 2020). It suggested that fires were possibly a landscape-scale phenomena, affecting a wide spectrum of habitats, from xeric to mesic ones, as has been documented for the temperate region of North America (Flatley et al., 2015). However, these data gave little information on fire sizes, spatial extent, and perhaps most importantly, the ignition densities.
Our reconstruction supported both (H1) and (H2): fire size typically exceeded stand scale (c. 101 ha) and fires varied strongly in size. Small fires (up to c. 200 ha) dominated the reconstructed period, with a few events attaining large sizes – a pattern that has earlier been revealed in other forest fire reconstructions (Niklasson and Granström, 2000; Heyerdahl et al., 2001; Storaunet et al., 2013; Rolstad et al., 2017). A number of features of the studied landscape apparently favoured fire spread: the area is dominated by generally flat or very gently undulating topography, with few depressions and swampy areas, and lacks lakes (Kwiatkowski, 1994). Such landscape evidently has the potential for large fires to develop, as evidenced by the fires in 1795, 1809, and 1825, recorded at most of the study sites (Figure 5), including sites well outside the studied area. The fire in 1809 seems to be an extreme case, since it was documented at all of the satellite sites (P3–P5), located at the distance of c. 3 km to the E (P3) and c. 6.5 km to the W (P4–P5) (Supplementary Figure 1).
Given that most of the conifer-dominated landscapes of Białowieża Forest have similar topographical features with few wetlands, rivers, and lakes (Kwiatkowski, 1994), we suspect that large fires have been common all over the Białowieża Forest area.
For establishing more firmly maximum fire sizes, we estimate that the sampling effort and aerial extent needs to be approximately 5–10 times larger than this study. Such sampling should preferably also include more mesic, deciduous-dominated parts of the landscape, where past fire occurrence has been already confirmed (Spînu et al., 2020).
The 1811 fire dated on half of the study sites was actually the only fire broadly known from historical sources (Ronke, 1830; Genko, 1902–1903; Faliński, 1986). We documented other fires, like those of 1803 or 1809, that seemed to be similarly widespread (Figure 5; Supplementary Figure 1). Clearly, there is a very strong discrepancy between the fire record derived from written sources and our tree ring reconstructed fires (cf. Niklasson et al., 2010). The reasons behind this remain unclear. One of the explanations could be possible gaps in the historical source material (Samojlik et al., 2016), even though the amount of archival data on the environmental history of Białowieża Forest is growing constantly (Samojlik, 2010; Samojlik et al., 2020). An alternative explanation is that fires were so common and not worth mentioning, unless humans were negatively affected in some way, as has been demonstrated in a recent study of anthropogenic fire regimes in North America (McClain et al., 2021).
Since so few fires were clearly contained within our studied area, we suggest that the fire cycle is a robust measure to characterize the past fire activity.
The dynamics of reconstructed fire activity corresponds broadly to changes in land use in Białowieża Forest.
During the 1670–1750 period, the fire cycle was 12 years (Figure 7), being one of the shortest cycles reported from conifer-dominated European forests (Storaunet et al., 2013; Rolstad et al., 2017; Kitenberga et al., 2019; Pinto et al., 2020; Ryzhkova et al., 2020). During the second half of the 1600s, a series of uprisings and wars were responsible for a decline in human population of the Białowieża Forest area and an increased demand for additional income to the royal treasury. Tar and potash production were introduced (Hedemann, 1939; Samojlik, 2010), activities that involved use of fire (Brincken, 1826; Samojlik, 2016) and likely promoted fire occurrence (Niklasson et al., 2010). Traditional forest beekeeping, cattle pasturing, and collecting of the resinous Scots pine wood for kindling (that was chopped off from the bottom of the scorched tree trunks), all activities associated with the use of fire (Brincken, 1826; Genko, 1902–1903; Samojlik et al., 2020), were common in the area at least since the 1500s (Hedemann, 1939; Samojlik et al., 2016, 2019).
The period of 1755–1840 marked an even shorter fire cycle of 7 years (Figure 7). Along with the fire-related activities observed in the previous period, charcoal burning was introduced during the second half of the 1700s (Hedemann, 1939; Samojlik, 2010), which may have further increased fire occurrence (Niklasson et al., 2010). This period of high fire activity is corroborated with other data. Stand-scale fire return intervals of 5 years were documented in Białowieża Forest during the 1700s (Niklasson et al., 2010; Zin et al., 2015; Spînu et al., 2020) and large influxes of charcoal and pollen of fire-associated plants were recorded in sediments during the 17th and 18th centuries (Latałowa et al., 2015, 2016; Zimny et al., 2017).
During the 1845–1955 period, the fire cycle increased to 22 years (Figure 7). This period encompassed the 1946 fire, the last fire on the studied sites (Figure 6). We propose that changes in the land management following incorporation of the area into the Russian Empire in 1795 were responsible for changes in the fire dynamics. Traditional forest beekeeping, tar, potash and charcoal production were progressively eliminated starting in the late 1700s (Brincken, 1826; Samojlik et al., 2020). In the case of forest beekeeping, the process of removal was gradual, supported by the government-ordered transition to apiaries and finalized after 1888, when Białowieża Forest became part of the Tsar’s private properties (Samojlik et al., 2019). Acquisition of the resinous wood chips from pine trees was prohibited in the 1840s and several measures were undertaken to eliminate that practice. However, they were unsuccessful and this activity lasted until the 20th century (Samojlik et al., 2019). Fire prevention in Białowieża Forest started already in the late 18th century (Hedemann, 1939), but it became rigorous during the 1800s (Genko, 1902–1903), as evidenced by the archival documents from the 19th century which mention several regulations concerning fire control (Samojlik et al., 2020, p. 92–93). Stand-scale fire histories in the area show decline in fire activity during the 1800s (Niklasson et al., 2010; Zin et al., 2015; Spînu et al., 2020). Paleoecological records confirm a substantial decrease in fire disturbance in the 19th century (Latałowa et al., 2015, 2016; Zimny et al., 2017). Like in many areas worldwide (Granström and Niklasson, 2008; Wallenius, 2011; Rolstad et al., 2017; Ryzhkova et al., 2020), active fire suppression in Białowieża Forest was strongly linked to the increasing interest in modern forest management, focusing on timber production (Genko, 1902–1903; Wiȩcko, 1984; cf. McGrath et al., 2015; Samojlik et al., 2020).
The decline in fire activity in Białowieża Forest occurred approximately 50 to 150 years later than in Northern Europe, where fires disappeared in the second half of the 1700s and in the early 1800s (Niklasson and Granström, 2000; Niklasson and Drakenberg, 2001; Wallenius, 2011; Drobyshev et al., 2014; Pinto et al., 2020). However, in the eastern section of the northern boreal forests fire decline occurred only in the late 19th century (Ryzhkova et al., 2020). Some areas escaped effective fire suppression policies, even until the middle of the 20th century (Kitenberga et al., 2019). At present, fire suppression is efficient and the fire cycle of Białowieża Forest equals c. 26 000 years, with an average fire size of c. 0.5 ha (Szczygieł et al., 2016). Data for the broader region reflect a similar picture with current fire cycles of several thousand years (Szczygieł et al., 2009; Drobyshev et al., 2021b; San-Miguel-Ayanz et al., 2021).
Paleoecological records support the notion of fire as a human-mediated phenomenon over most of temperate Europe. Charcoal influx in sediments from forests in the region has been shown to correlate with anthropogenic indicators, such as pollen of cultivated plants or spores of coprophilous fungi (Bradshaw and Lindbladh, 2005; Tinner et al., 2005; Pȩdziszewska and Latałowa, 2016; Czerwiński et al., 2021). Well-preserved old-growth woodlands with primary character such as Białowieża Forest also show signs of long-term human impact (Samojlik et al., 2013; Latałowa et al., 2015, 2016; Leuschner and Ellenberg, 2017a; Zimny et al., 2017; Jaroszewicz et al., 2019).
Climate and human land use might have jointly affected past fire activity in Białowieża Forest (cf. Bradshaw and Lindbladh, 2005; Novák et al., 2012; Pȩdziszewska and Latałowa, 2016). However, land use policies and increasingly efficient fire suppression were likely more important than the impact of climate variability on the fire regime in the most recent period. The warmer climate in Białowieża Forest that the region recorded since the second half of the 1800s (Jȩdrzejewska et al., 1997; Pierzgalski et al., 2002; Büntgen et al., 2011; Boczoń et al., 2018) was instead associated with a decline in fire activity, as revealed by our results. Similarly, increased early season fire hazard over most of Belarus and Poland, as indicated by the dynamics of the monthly drought code over the 20th century (Drobyshev et al., 2021b), has not been reflected in fire activity until now.
Disentangling the climatic and anthropogenic impacts on ecosystem dynamics is complex and probably not always possible (Bradshaw and Lindbladh, 2005; Pȩdziszewska and Latałowa, 2016). We propose that to explore causes and mechanisms behind the recorded changes in fire activity in Białowieża Forest in even greater detail, a larger sampling area should be considered and further, parallel research in historical and paleoecological archives should be continued (cf. Latałowa et al., 2015; Czerwiński et al., 2021).
Historical fires in Białowieża Forest occurred at frequencies exceeding frequency of lightning-caused ignitions (H3), since the number of fires we recorded exceeded the present day natural background level of ignition by a factor of 10–100, when compared with data on lightning ignition frequencies from Poland, Germany, and Latvia (Sannikov and Goldammer, 1996; Ubysz and Szczygieł, 2006; Szczygieł et al., 2016; Brackhane et al., 2021). The sizes of the largest fires, given that they originate from one or few ignitions, have implications on calculations of the ignition density on the landscape and hence, on the interpretation of the possible fire causes – whether they are caused by lightning or by people (Niklasson and Granström, 2000). Therefore, we think that our results must be interpreted with caution, since most fire areas were only partly reconstructed, and especially for the larger ones, the values we obtained may have been greatly underestimated. Obviously, our reconstruction lacks direct information on fire cause, which could potentially be corroborated with more extensive analyzes of independent historical data (court protocols, eye-witness accounts, diaries, etc.). However, such high ignition density level on the landscape points toward a strong anthropogenic influence on the fire regime for the studied area and period (Niklasson and Granström, 2000).
The multi-site approach with a high degree of sample replication at each study site also yielded a spatial dimension of the occurrence of short-interval fires (1–2 years) recorded both at the scale of a given tree stand, i.e., study site, and at the scale of an individual sample tree, rarely recorded in P. sylvestris trees (Ivanova et al., 2010; Zin et al., 2015). We documented several cases in which two multi-site fires were recorded in consecutive years at the same study site, although not in the same tree – as in the years: 1757 and 1758, 1769 and 1770, 1789 and 1790 (Supplementary Figure 1). However, only a 1-year interval recorded in the very same tree is definite evidence of fire passing the same point in space, as was actually documented by one of our sample trees (Figure 8). Nevertheless, the intermixed positions of scars in two consecutive years suggest that fire actually spread over the same stand in the succeeding year (Supplementary Figure 1), but likely with highly varying severity. We believe that a 1-year scarring interval is simply very rare because of fuel dynamics under frequent fire disturbance, mainly low fuel quantity (Schimmel and Granström, 1997), and fuel type. Ground fuels in the area we studied were likely quite different at the time of frequent fires (Zin et al., 2015) than the current picture (see section “Materials and Methods”). They were probably strongly dominated by grasses (and partly also by heather Calluna vulgaris (L.) Hull), which after fire regenerate faster than the common fuels of Scots pine forests today – ericaceous Vaccinium species and feathermosses (Skre et al., 1998; Marozas et al., 2007; Parro et al., 2009). The strong grass dominance in the fuel bed may also relate to the open, park-like stand structure resulting from repeated low- and mixed-severity fire disturbances (Brown and Wu, 2005; Zin, 2016). However, while fuel build-up in Białowieża Forest seems to be enough to sustain 1-year intervals for fires on a larger scale of a tree stand (Niklasson et al., 2010; Zin et al., 2015; Spînu et al., 2020), at the scale of an individual tree the fuel accumulation seems to be usually not sufficient to produce enough heat for a renewed scarring. The reason for this is likely a variation in fire behavior and heterogenous fire severity within the same fire event, which impact several features of the post-fire tree stand (e.g., Keyser et al., 2008; Iniguez et al., 2009; Parro et al., 2009; Adámek et al., 2016), including the following fuel succession (Skre et al., 1998; Adámek et al., 2016).
Figure 8. One-year fire interval recorded by a Scots pine tree (fire scars in 1774 and 1775, denoted by white arrows). It is the single case of a 1-year point-scale fire interval observed among 1137 analyzed P. sylvestris tree ring samples (representing 798 trees) from Białowieża Forest, Poland and Belarus (Zin, 2016). Photo: Ewa Zin.
Even though data is lacking, nothing precludes the idea that some fires in Białowieża Forest did consume more fuel, went deeper into the humus layer and/or were so severe that a subsequent build-up of fuel was slower (Schimmel and Granström, 1996, 1997). In the cumulative fire curve for our studied area (Figure 6), there are at least three cases in which fires were followed by much longer fire-free periods than usual. Perhaps these gaps in the fire record were a product of high-severity fires which occasionally occurred in Białowieża Forest (Zin et al., 2015). In case of the 1718 fire (in the site P1), we had a clear evidence of its high severity, as was proven by sudden growth depressions recorded in two surviving pines, and the subsequent cohort regeneration (Zin et al., 2015). Similar feedback mechanisms between fire severity and post-fire stand dynamics have been described, for example, in North American ponderosa pine (Pinus ponderosa Douglas ex Lawson and C. Lawson) ecosystems (Iniguez et al., 2009). However, further analysis of past fire behavior and subsequent fire intervals is needed to convincingly show the effect of high-severity fires in Białowieża Forest.
Fires have been present in Białowieża Forest throughout the Holocene (Dąbrowski, 1959; Latałowa et al., 2016) with the proportion of human ignitions likely to be increasing over time. However, it is still unknown whether human-influenced fire regimes differ fundamentally from natural, lightning-driven ones in terms of the resulting ecosystem structure and species composition. In a natural fire regime, fires may grow very large when unsuppressed, partially outweighing the effect of relatively few ignitions (Niklasson and Granström, 2000; Rolstad et al., 2017). Both natural- and human-driven fire activity has been effectively suppressed in the modern times and the lack of fire puts at risk the regeneration of fire-adapted canopy dominants, such as pine and oak (Spînu et al., 2020; Drobyshev et al., 2021a). This is especially true for Scots pine (Niklasson et al., 2010; Zin et al., 2015), which natural regeneration is proven to decline in Białowieża Forest over the last decades (Kuijper et al., 2010; Drozdowski et al., 2012; Paluch, 2015; Brzeziecki et al., 2020) – a pattern now regarded a region-wide phenomenon (Matuszkiewicz, 2007; Heinken, 2008). Thus, our results contribute directly to the ongoing discussion on management and conservation of Central European pinewoods (Walentowski et al., 2007; Heinken, 2008; Adámek et al., 2016). Moreover, a number of fire- and light-demanding species in Białowieża Forest have declined due to the long period of fire suppression and have been shown to respond positively to recent fires (Gutowski et al., 2020). Finally, vegetation (and fuel) changes currently experienced by the area due to the effective fire control (Szczygieł et al., 2016) may result in strong and unpredictable feedbacks upon future fire regimes (Pińol et al., 2005; Stephens et al., 2013; Steel et al., 2015). To sum up, future conservation and management strategies for Białowieża Forest and for the region need to seriously re-consider the fundamental role of fire in temperate forest ecosystems.
Although limited in the area covered, the study provided valuable quantitative information on the past forest fire activity, that has not been available for temperate European forests. In addition, the spatial study design brought the first quantification of the ignition density, pointing toward a strong human impact on ignitions. Fires in Białowieża Forest varied considerably in size, with the largest events probably exceeding 1 000 ha. Future studies could elucidate the role of wet forest types and depressions as fire breaks, as well as further document the size distribution of past fires (including both very large and very small events), which would further strengthen the discussion on possible fire causes. This type of study would also be important for the deeper understanding of the role of climate in driving the past fire regime in this region and potential future fire activity under climate change (cf. e.g., Drobyshev et al., 2014). Our results highlight the importance of fire disturbance in Białowieża Forest, show the potential for further spatial reconstructions for studies of past fire regimes in temperate Europe, and provide critical baseline information to design biological conservation and management strategies in European forests.
The raw data supporting the conclusions of this article will be made available by the authors, without undue reservation.
EZ and MN developed study ideas and design, collected the data, and wrote the first draft. ID developed parts of statistical setup. EZ legally organized and realized field work. EZ, ŁK, ID, and MN analyzed the data. All authors contributed equally to the writing of the text and have given their approval for publication.
This study was supported by the Polish Ministry of Science and Higher Education within Forest Research Institute’s (IBL) statutory activities (Grant No. 240601 to EZ). Additional support to EZ was provided by the Southern Swedish Forest Research Centre, SLU, Alnarp and by Stiftelsen Stina Werners Fond, Göholm (project: SSWF 14-2/52-4 Chdre). MN was supported by a grant from the Hasselblad Foundation (project: “Grazing and fire – key processes for biodiversity restoration in the temperate zone”). ID acknowledges support from the Swedish Research Council for Sustainable Development (FORMAS), project NordicFires (#2014-01866), funding from the Natural Sciences and Engineering Research Council of Canada (NSERC, project #RGPIN-2018-06637), Stiftelsen Stina Werners Fond (project #2021-106), and Nordic Forest Research (SNS, project #SNS-134).
The authors declare that the research was conducted in the absence of any commercial or financial relationships that could be construed as a potential conflict of interest.
All claims expressed in this article are solely those of the authors and do not necessarily represent those of their affiliated organizations, or those of the publisher, the editors and the reviewers. Any product that may be evaluated in this article, or claim that may be made by its manufacturer, is not guaranteed or endorsed by the publisher.
We are grateful to Emily K. Heyerdahl and three referees for constructive comments on the earlier versions of the manuscript, the administration of the Belovezhskaya Pushcha National Park for permission to collect tree ring samples, Vasili M. Arnolbik for assistance in all practical and formal matters related to sampling, Adam Kudlewski for help in fieldwork and sample preparation, Sergei Korotkov, Izabela Sondej, and Dariusz Graszka-Petrykowski for assistance in field sampling, and James Richards for help with linguistic corrections of the first draft.
The Supplementary Material for this article can be found online at: https://www.frontiersin.org/articles/10.3389/fevo.2022.768464/full#supplementary-material
Adámek, M., Bobek, P., Hadincová, V., Wild, J., and Kopecký, M. (2015). Forest fires within a temperate landscape: a decadal and millennial perspective from a sandstone region in Central Europe. For. Ecol. Manag. 336, 81–90. doi: 10.1016/j.foreco.2014.10.014
Adámek, M., Hadincová, V., and Wild, J. (2016). Long-term effect of wildfires on temperate Pinus sylvestris forests: vegetation dynamics and ecosystem resilience. For. Ecol. Manag. 380, 285–295. doi: 10.1016/j.foreco.2016.08.051
Arno, S. F., and Sneck, K. M. (1977). A Method for Determining Fire History in Coniferous Forests of the Mountain West USA. USDA Forest Service General Technical Report, Intermountain Forest and Range Experiment Station (INT-42). Ogden, UT: U.S. Department of Agriculture, 28.
Beaty, R. M., and Taylor, A. H. (2001). Spatial and temporal variation of fire regimes in a mixed conifer forest landscape, southern Cascades, California, USA. J. Biogeogr. 28, 955–966. doi: 10.1046/j.1365-2699.2001.00591.x
Beaty, R. M., and Taylor, A. H. (2008). Geographic Variation in Mixed-Conifer Forest Fire Regimes in California. USDA Forest Service General Technical Report PSW-GTR-189. Albany, CA: U.S. Department of Agriculture, 123–130.
Blanck, Y., Rolstad, J., and Storaunet, K. O. (2013). Low- to moderate-severity historical fires promoted high tree growth in a boreal Scots pine forest of Norway. Scand. J. For. Res. 28, 126–135. doi: 10.1080/02827581.2012.706635
Bobek, P., Svitavská Svobodová, H., Werchan, B., Soukupová, M., and Kuneš, P. (2018). Human-induced changes in fire regime and subsequent alteration of the sandstone landscape of Northern Bohemia (Czech Republic). Holocene 28, 427–443. doi: 10.1177/0959683617729443
Boczoń, A., Kowalska, A., Ksepko, M., and Sokołowski, K. (2018). Climate warming and drought in the Bialowieza Forest from 1950–2015 and their impact on the dieback of Norway Spruce stands. Water 10:1502. doi: 10.3390/w10111502
Brackhane, S., Reif, A., Zin, E., and Schmitt, C. B. (2021). Are natural disturbances represented in strictly protected areas in Germany? Glob. Ecol. Conserv. 26:e01436. doi: 10.1016/j.gecco.2020.e01436
Bradshaw, R. H. W., and Lindbladh, M. (2005). Regional spread and stand-scale establishment of Fagus sylvatica and Picea abies in Scandinavia. Ecology 86, 1679–1686. doi: 10.1890/03-0785
Bradshaw, R. H., and Sykes, M. T. (2014). Ecosystem Dynamics: From the Past to the Future. Chichester: Wiley-Blackwell.
Brincken, J. (1826). Mémoire Descriptif sur la Forȩt Impériale de Białowieża, en Lithuanie. Varsovie: Glücksberg.
Brown, P. M., and Wu, R. (2005). Climate and disturbance forcing of episodic tree recruitment in a southwestern ponderosa pine landscape. Ecology 86, 3030–3038. doi: 10.1890/05-0034
Brus, D. J., Hengeveld, G. M., Walvoort, D. J. J., Goedhart, P. W., Heidema, A. H., Nabuurs, G. J., et al. (2012). Statistical mapping of tree species over Europe. Eur. J. For. Res. 131, 145–157. doi: 10.1007/s10342-011-0513-5
Brzeziecki, B., Woods, K., Bolibok, L., Zaja̧czkowski, J., Drozdowski, S., Bielak, K., et al. (2020). Over 80 years without major disturbance, late-successional Białowieża woodlands exhibit complex dynamism, with coherent compositional shifts towards true old-growth conditions. J. Ecol. 108, 1138–1154. doi: 10.1111/1365-2745.13367
Büntgen, U., Tegel, W., Nicolussi, K., McCormick, M., Frank, D., Trouet, V., et al. (2011). 2500 years of European climate variability and human susceptibility. Science 331, 578–582. doi: 10.1126/science.1197175
Castellnou, M., Kraus, D., and Miralles, M. (2010). “Prescribed burning and suppression fire techniques: from fuel to landscape management,” in Best Practices of Fire Use – Prescribed Burning and Suppression Fire Programmes in Selected Case-Study Regions in Europe, eds C. Montiel and D. Kraus (Joensuu: European Forest Institute), 3–16.
Christopoulou, A., Fulé, P. Z., Andriopoulos, P., Sarris, D., and Arianoutsou, M. (2013). Dendrochronology-based fire history of Pinus nigra forests in Mount Taygetos, Southern Greece. For. Ecol. Manag. 293, 132–139. doi: 10.1016/j.foreco.2012.12.048
Clark, J. S. (1988). Particle motion and the theory of charcoal analysis: source area, transport, deposition, and sampling. Quat. Res. 30, 67–80. doi: 10.1016/0033-5894(88)90088-9
Clark, J. S., Lynch, J., Stocks, B. J., and Goldammer, J. G. (1998). Relationships between charcoal particles in air and sediments in west-central Siberia. Holocene 8, 19–29. doi: 10.1191/095968398672501165
Czerwiński, S., Guzowski, P., Lamentowicz, M., Gałka, M., Karpińska-Kołaczek, M., Poniat, R., et al. (2021). Environmental implications of past socioeconomic events in Greater Poland during the last 1200 years. Synthesis of paleoecological and historical data. Quat. Sci. Rev. 259:106902. doi: 10.1016/j.quascirev.2021.106902
Dąbrowski, M. J. (1959). Późnoglacjalna i holoceńska historia lasów Puszczy Białowieskiej. Czȩść I. Białowieski Park Narodowy. Acta Soc. Bot. Poloniae 28, 197–248.
Dieterich, J. H., and Swetnam, T. W. (1984). Dendrochronology of a fire-scarred ponderosa pine. For. Sci. 30, 238–247.
Drobyshev, I., Bergeron, Y., Girardin, M. P., Gauthier, S., Ols, C., and Ojal, J. (2017). Strong gradients in forest sensitivity to climate change revealed by dynamics of forest fire cycles in the post Little Ice Age era. J. Geophys. Res. Biogeosci. 122, 2605–2616. doi: 10.1002/2017JG003826
Drobyshev, I., Granström, A., Linderholm, H. W., Hellberg, E., Bergeron, Y., and Niklasson, M. (2014). Multi-century reconstruction of fire activity in Northern European boreal forest suggests differences in regional fire regimes and their sensitivity to climate. J. Ecol. 102, 738–748. doi: 10.1111/1365-2745.12235
Drobyshev, I., Niklasson, M., Ryzhkova, N., Götmark, F., Pinto, G., and Lindbladh, M. (2021a). Did forest fires maintain mixed oak forests in southern Scandinavia? A dendrochronological speculation. For. Ecol. Manag. 482:118853. doi: 10.1016/j.foreco.2020.118853
Drobyshev, I., Ryzhkova, N., Eden, J., Kitenberga, M., Pinto, G., Lindberg, H., et al. (2021b). Trends and patterns in annually burned forest areas and fire weather across the European boreal zone in the 20th and early 21st centuries. Agric. For. Meteorol. 306:108467. doi: 10.1016/j.agrformet.2021.108467
Drozdowski, S., Brzeziecki, B., Żybura, H., Żybura, B., Gawron, L., Buraczyk, W., et al. (2012). Wieloletnia dynamika starodrzewów w zagospodarowanej czȩści Puszczy Białowieskiej: gatunki ekspansywne i ustȩpuja̧ce. Sylwan 156, 663–671. doi: 10.26202/sylwan.2012016
Faliński, J. B. (1986).Vegetation Dynamics in Temperate Lowland Primeval Forest. Ecological Studies in Białowieża Forest. Dordrecht: Dr W. Junk Publishers.
Falk, D. A., Heyerdahl, E. K., Brown, P. M., Farris, C., Fulé, P. Z., McKenzie, D., et al. (2011). Multi-scale controls of historical forest-fire regimes: new insights from fire-scar networks. Front. Ecol. Environ. 9, 446–454. doi: 10.1890/100052
Feurdean, A., Vannière, B., Finsinger, W., Warren, D., Connor, S. C., Forrest, M., et al. (2020). Fire hazard modulation by long-term dynamics in land cover and dominant forest type in eastern and central Europe. Biogeosciences 17, 1213–1230. doi: 10.5194/bg-17-1213-2020
Flatley, W. T., Lafon, C. W., Grissino-Mayer, H. D., and LaForest, L. B. (2015). Changing fire regimes and old-growth forest succession along a topographic gradient in the Great Smoky Mountains. For. Ecol. Manag. 350, 96–106. doi: 10.1016/j.foreco.2015.04.024
Genko, N. (1902-1903). Kharakteristika Belovezhskoi pushchi i istoricheskiya o nei dannyya. Lesnoi Zhurnal 22, 1012–1056, 22, 1269–1302, 23, 22–56.
Granström, A., and Niklasson, M. (2008). Potentials and limitations for human control over historic fire regimes in the boreal forest. Philos. Trans. R. Soc. B Biol. Sci. 363, 2353–2358. doi: 10.1098/rstb.2007.2205
Gutowski, J. M., and Jaroszewicz, B. (2001). Catalogue of the Fauna of Białowieża Primeval Forest. Warszawa: Instytut Badawczy Leśnictwa.
Gutowski, J. M., Sućko, K., Borowski, J., Kubisz, D., Mazur, M. A., Melke, A., et al. (2020). Post-fire beetle succession in a biodiversity hotspot: Białowieża Primeval Forest. For. Ecol. Manag. 461:117893. doi: 10.1016/j.foreco.2020.117893
Hedemann, O. (1939). L’histoire de la forêt de Białowieża (jusqu’a 1798). Warszawa: Institut de Recherches des Forêts Domaniales.
Heinken, T. (2008). Die natürlichen Kiefernstandorte Deutschlands und ihre Gefährdung. Beiträge Nordwestdeutsch. For. Versuchsanstalt 2, 19–41.
Heyerdahl, E. K., Brubaker, L. B., and Agee, J. K. (2001). Spatial controls of historical fire regimes: a multiscale example from the Interior West, USA. Ecology 82, 660–678. doi: 10.1890/0012-9658(2001)082[0660:scohfr]2.0.co;2
Iniguez, J. M., Swetnam, T. W., and Baisan, C. H. (2009). Spatially and temporally variable fire regime on Rincon Peak, Arizona, USA. Fire Ecol. 5, 3–21. doi: 10.4996/fireecology.0501003
Ivanova, G. A., Ivanov, V. A., Kukavskaya, E. A., and Soja, A. J. (2010). The frequency of forest fires in Scots pine stands of Tuva, Russia. Environ. Res. Lett. 5:015002. doi: 10.1088/1748-9326/5/1/015002
Jaroszewicz, B., Cholewińska, O., Gutowski, J. M., Samojlik, T., Zimny, M., and Latałowa, M. (2019). Białowieża Forest—a relic of the high naturalness of European forests. Forests 10:849. doi: 10.3390/f10100849
Jȩdrzejewska, B., and Jȩdrzejewski, W. (1998). Predation in Vertebrate Communities. The Białowieża Primeval Forest as a Case Study. Berlin: Springer.
Jȩdrzejewska, B., Jȩdrzejewski, W., Bunevich, A. N., Miłkowski, L., and Krasiński, Z. A. (1997). Factors shaping population densities and increase rates of ungulates in Białowieża Primeval Forest (Poland and Belarus) in the 19th and 20th centuries. Acta Theriol. 42, 399–451.
Johnson, E. A. (1992). Fire and Vegetation Dynamics: Studies From the North American Boreal Forest. Cambridge: Cambridge University Press.
Kaplan, J. O., Krumhardt, K. M., and Zimmermann, N. (2009). The prehistoric and preindustrial deforestation of Europe. Quat. Sci. Rev. 28, 3016–3034. doi: 10.1016/j.quascirev.2009.09.028
Keyser, T. L., Lentile, L. B., Smith, F. W., and Shepperd, W. D. (2008). Changes in forest structure after a large, mixed-severity wildfire in ponderosa pine forests of the Black Hills, South Dakota, USA. For. Sci. 54, 328–338.
Kipfmueller, K. F., and Kupfer, J. A. (2005). Complexity of successional pathways in subalpine forests of the Selway-Bitterroot Wilderness Area. Ann. Assoc. Am. Geogr. 95, 495–510. doi: 10.1111/j.1467-8306.2005.00471.x
Kitenberga, M., Drobyshev, I., Elferts, D., Matisons, R., Adamovics, A., Katrevics, J., et al. (2019). A mixture of human and climatic effects shapes the 250-year long fire history of a semi-natural pine dominated landscape of Northern Latvia. For. Ecol. Manag. 441, 192–201. doi: 10.1016/j.foreco.2019.03.020
Kitzberger, T., Brown, P. M., Heyerdahl, E. K., Swetnam, T. W., and Veblen, T. T. (2007). Contingent Pacific-Atlantic ocean influence on multicentury wildfire synchrony over western North America. Proc. Natl. Acad. Sci. U.S.A. 104, 543–548. doi: 10.1073/pnas.0606078104
Kottek, M., Grieser, J., Beck, C., Rudolf, B., and Rubel, F. (2006). World Map of the Köppen-Geiger climate classification updated. Meteorol. Zeitschr. 15, 259–263. doi: 10.1127/0941-2948/2006/0130
Krzyściak-Kosińska, R., Arnolbik, V., and Antczak, A. (eds) (2012). Białowieża Forest. “Belovezhskaya Pushcha/Białowieża Forest” World Heritage Site (33 BIS). Proposed Modification of the Criteria and Boundaries, Change of the Name of the Property. Nomination Dossier to the UNESCO for the Inscription on the World Heritage List. Available online at: https://whc.unesco.org/en/list/33/documents/ (accessed August 16, 2021).
Kuijper, D. P. J., Jȩdrzejewska, B., Brzeziecki, B., Churski, M., Jȩdrzejewski, W., and Żybura, H. (2010). Fluctuating ungulate density shapes tree recruitment in natural stands of the Białowieża Primeval Forest, Poland. J. Veg. Sci. 21, 1082–1098. doi: 10.1111/j.1654-1103.2010.01217.x
Latałowa, M., Zimny, M., Jȩdrzejewska, B., and Samojlik, T. (2015). “Białowieża Primeval Forest: a 2000-year interplay of environmental and cultural forces in Europe’s best preserved temperate woodland,” in Europe’s Changing Woods and Forests: From Wildwood to Managed Landscapes, eds K. J. Kirby and C. Watkins (Oxfordshire: CAB International), 243–264.
Latałowa, M., Zimny, M., Pȩdziszewska, A., and Kupryjanowicz, M. (2016). Postglacjalna historia Puszczy Białowieskiej – roślinność, klimat i działalność człowieka. Parki Narodowe i Rezerwaty Przyrody 35, 3–49.
Lehtonen, H., Huttunen, P., and Zetterberg, P. (1996). Influence of man on forest fire frequency in North Karelia, Finland, as evidenced by fire scars on Scots pines. Ann. Bot. Fennici 33, 257–263.
Leuschner, C., and Ellenberg, H. (2017a). “The Central European vegetation as the result of millennia of human activity,” in Ecology of Central European Forests: Vegetation Ecology of Central Europe, Vol. I, (Cham: Springer International Publishing), 31–116.
Leuschner, C., and Ellenberg, H. (2017b). Ecology of Central European Forests: Vegetation Ecology of Central Europe, Vol. I. Cham: Springer International Publishing.
Margolis, E. Q., and Balmat, J. (2009). Fire history and fire–climate relationships along a fire regime gradient in the Santa Fe Municipal Watershed, NM, USA. For. Ecol. Manag. 258, 2416–2430. doi: 10.1016/j.foreco.2009.08.019
Marozas, V., Racinskas, J., and Bartkevicius, E. (2007). Dynamics of ground vegetation after surface fires in hemiboreal Pinus sylvestris forests. For. Ecol. Manag. 250, 47–55. doi: 10.1016/j.foreco.2007.03.008
Matuszkiewicz, J. M. (2007). “Geobotanical identification of the development tendencies in forest associations in the regions of Poland – a synthetic survey,” in Geobotanical Identification of the Development Tendencies in Forest Associations in the Regions of Poland, ed. J. M. Matuszkiewicz (Warszawa: Polish Academy of Sciences).
McBride, J. R. (1983). Analysis of tree rings and fire scars to establish fire history. Tree Ring Bull. 43, 51–67.
McClain, W. E., Ruffner, C. M., Ebinger, J. E., and Spyreas, G. (2021). Patterns of anthropogenic fire within the Midwestern Tallgrass Prairie 1673–1905: evidence from written accounts. Nat. Areas J. 41, 283–300. doi: 10.3375/20-5
McGrath, M. J., Luyssaert, S., Meyfroidt, P., Kaplan, J. O., Bürgi, M., Chen, Y., et al. (2015). Reconstructing European forest management from 1600 to 2010. Biogeosciences 12, 4291–4316. doi: 10.5194/bg-12-4291-2015
Mirek, Z., Piȩkoś-Mirkowa, H., Zaja̧c, A., and Zaja̧c, M. (2002). Flowering Plants and Pteridophytes of Poland. A Checklist. Kraków: W. Szafer Institute of Botany.
Mitchell, F. J. G. (2005). How open were European primeval forests? Hypothesis testing using palaeoecological data. J. Ecol. 93, 168–177. doi: 10.1111/j.1365-2745.2004.00964.x
Mitchell, F. J. G., and Cole, E. (1998). Reconstruction of long-term successional dynamics of temperate woodland in Białowieża Forest, Poland. J. Ecol. 86, 1042–1059. doi: 10.1046/j.1365-2745.1998.00323.x
Niklasson, M., and Drakenberg, B. (2001). A 600-year tree-ring fire history from Norra Kvills National Park, southern Sweden: implications for conservation strategies in the hemiboreal zone. Biol. Conserv. 101, 63–71. doi: 10.1016/S0006-3207(01)00050-7
Niklasson, M., and Granström, A. (2000). Numbers and sizes of fires: long-term spatially explicit fire history in a Swedish boreal landscape. Ecology 81, 1484–1499. doi: 10.2307/177301
Niklasson, M., Zin, E., Zielonka, T., Feijen, M., Korczyk, A. F., Churski, M., et al. (2010). A 350-year tree-ring fire record from Białowieża Primeval Forest, Poland: implications for Central European lowland fire history. J. Ecol. 98, 1319–1329. doi: 10.1111/j.1365-2745.2010.01710.x
Novák, J., Sádlo, J., and Svobodová-Svitavská, H. (2012). Unusual vegetation stability in a lowland pine forest area (Doksy region, Czech Republic). Holocene 22, 947–955. doi: 10.1177/0959683611434219
Ochyra, R., Żarnowiec, J., and Bednarek-Ochyra, H. (2003). Census Catalogue of Polish Mosses. Kraków: Institute of Botany.
Ohlson, M., and Tryterud, E. (2000). Interpretation of the charcoal record in forest soils: forest fires and their production and deposition of macroscopic charcoal. Holocene 10, 519–525. doi: 10.1191/095968300667442551
Paluch, R. (2015). Wieloletnie zmiany składu gatunkowego drzewostanów naturalnych w Puszczy Białowieskiej. Sylwan 159, 278–288. doi: 10.26202/sylwan.2014083
Parro, K., Köster, K., Jõgiste, K., and Vodde, F. (2009). Vegetation dynamics in a fire damaged forest area: the response of major ground vegetation species. Baltic For. 15, 206–215.
Pausas, J. G., and Keeley, J. E. (2009). A burning story: the role of fire in the history of life. Bioscience 59, 593–601. doi: 10.1525/bio.2009.59.7.10
Pȩdziszewska, A., and Latałowa, M. (2016). Stand-scale reconstruction of late Holocene forest succession on the Gdańsk Upland (N. Poland) based on integrated palynological and macrofossil data from paired sites. Veg. Hist. Archaeobot. 25, 239–254. doi: 10.1007/s00334-015-0546-7
Peterken, G. F. (1996). Natural Woodland: Ecology and Conservation in Northern Temperate Regions. Cambridge: Cambridge University Press.
Pierzgalski, E., Boczoń, A., and Tyszka, J. (2002). Variability of precipitation and ground water level in the Białowieża National Park. Kosmos 51, 415–425.
Piha, A., Kuuluvainen, T., Lindberg, H., and Vanha-Majamaa, I. (2013). Can scar-based fire history reconstructions be biased? An experimental study in boreal Scots pine. Can. J. For. Res. 43, 669–675. doi: 10.1139/cjfr-2012-0471
Pińol, J., Beven, K., and Viegas, D. X. (2005). Modelling the effect of fire-exclusion and prescribed fire on wildfire size in Mediterranean ecosystems. Ecol. Model. 183, 397–409. doi: 10.1016/j.ecolmodel.2004.09.001
Pinto, G. A. S. J., Niklasson, M., Ryzhkova, N., and Drobyshev, I. (2020). A 500-year history of forest fires in Sala area, central Sweden, shows the earliest known onset of fire suppression in Scandinavia. Reg. Environ. Change 20:130. doi: 10.1007/s10113-020-01718-2
Pyne, S. J. (1997). Vestal Fire. An Environmental History, Told through Fire, of Europe and Europe’s Encounter with the World. Seattle: University of Washington Press.
Reed, W. J., Larsen, C. P. S., Johnson, E. A., and MacDonald, G. M. (1998). Estimation of temporal variations in historical fire frequency from time-since-fire map data. For. Science 44, 465–475.
Rodionov, S. N. (2004). A sequential algorithm for testing climate regime shifts. Geophys. Res. Lett. 31:L09204. doi: 10.1029/2004GL019448
Rolstad, J., Blanck, Y., and Storaunet, K. O. (2017). Fire history in a western Fennoscandian boreal forest as influenced by human land use and climate. Ecol. Monogr. 87, 219–245. doi: 10.1002/ecm.1244
Ronke, E. (1830). Do W. Jarockiego professora Kr. War. Uniwer. Niektóre uwagi wzglȩdem Puszczy Białowieżskiey. Powszechny Dziennik Krajowy 95, 418.
Rösch, M. (2000). Long-term human impact as registered in an upland pollen profile from the southern Black Forest, south-western Germany. Veg. Hist. Archaeobot. 9, 205–218. doi: 10.1007/Bf01294635
Ryzhkova, N., Pinto, G., Kryshen, A., Bergeron, Y., Ols, C., and Drobyshev, I. (2020). Multi-century reconstruction suggests complex interactions of climate and human controls of forest fire activity in a Karelian boreal landscape, North-West Russia. For. Ecol. Manag. 459:117770. doi: 10.1016/j.foreco.2019.117770
Sabatini, F. M., Burrascano, S., Keeton, W. S., Levers, C., Lindner, M., Pötzschner, F., et al. (2018). Where are Europe’s last primary forests? Divers. Distribut. 24, 1426–1439. doi: 10.1111/ddi.12778
Samojlik, T. (2005). Conservation and Hunting. Białowieża Forest in the Time of Kings. Białowieża: Mammal Research Institute.
Samojlik, T. (2010). Traditional utilisation of Białowieża Primeval Forest (Poland) in the 15th to 18th centuries. Landsc. Archaeol. Ecol. 8, 150–164.
Samojlik, T. (2016). Rozkwit i upadek produkcji potażu w Puszczy Białowieskiej w XVII–XIX w. Rocz. Pol. Tow. Dendrol. 64, 9–16.
Samojlik, T., Fedotova, A., and Kuijper, D. P. J. (2016). Transition from traditional to modern forest management shaped the spatial extent of cattle pasturing in Białowieża Primeval Forest in the nineteenth and twentieth centuries. Ambio 45, 904–918. doi: 10.1007/s13280-016-0795-4
Samojlik, T., Fedotova, A., Daszkiewicz, P., and Rotherham, I. D. (2020). Białowieża Primeval Forest: Nature and Culture in the Nineteenth Century. Cham: Springer Nature Switzerland AG.
Samojlik, T., Fedotova, A., Niechoda, T., and Rotherham, I. D. (2019). Culturally modified trees or wasted timber: different approaches to marked trees in Poland’s Białowieża Forest. PLoS One 14:e0211025. doi: 10.1371/journal.pone.0211025
Samojlik, T., Rotherham, I. D., and Jȩdrzejewska, B. (2013). Quantifying historic human impacts on forest environments: a case study in Białowieża Forest, Poland. Environ. Hist. 18, 576–602. doi: 10.1093/envhis/emt039
San-Miguel-Ayanz, J., Durrant, T., Boca, R., Maianti, T., Libertá, G., Artés-Vivancos, T., et al. (2021). Forest Fires in Europe, Middle East and North Africa 2020. Luxembourg: Publicatios Office of the European Union.
Sannikov, S. N., and Goldammer, J. G. (1996). “Fire ecology of pine forests of Northern Eurasia,” in Fire in Ecosystems of Boreal Eurasia, eds V. V. Furyaev and J. G. Goldammer (Dordrecht: Kluwer Academic Publishers), 151–167.
Schimmel, J., and Granström, A. (1996). Fire severity and vegetation response in the boreal Swedish forest. Ecology 77, 1436–1450. doi: 10.2307/2265541
Schimmel, J., and Granström, A. (1997). Fuel succession and fire behavior in the Swedish boreal forest. Can. J. For. Res. 27, 1207–1216. doi: 10.1139/x97-072
Skre, O., Wielgolaski, F. E., and Moe, B. (1998). Biomass and chemical composition of common forest plants in response to fire in western Norway. J. Veg. Sci. 9, 501–510. doi: 10.2307/3237265
Sokołowski, A. W. (2004). Lasy Puszczy Białowieskiej. Warszawa: Centrum Informacyjne Lasów Państwowych.
Spînu, A. P., Niklasson, M., and Zin, E. (2020). Mesophication in temperate Europe: a dendrochronological reconstruction of tree succession and fires in a mixed deciduous stand in Białowieża Forest. Ecol. Evol. 10, 1029–1041. doi: 10.1002/ece3.5966
Steel, Z. L., Safford, H. D., and Viers, J. H. (2015). The fire frequency-severity relationship and the legacy of fire suppression in California forests. Ecosphere 6:8. doi: 10.1890/ES14-00224.1
Stephens, S. L., Agee, J. K., Fulé, P. Z., North, M. P., Romme, W. H., Swetnam, T. W., et al. (2013). Managing forests and fire in changing climates. Science 342, 41–42. doi: 10.1126/science.1240294
Stokes, M. A., and Smiley, T. L. (1968). An Introduction to Tree-Ring Dating. Chicago, IL: University of Chicago Press.
Storaunet, K. O., Rolstad, J., Toeneiet, M., and Blanck, Y. (2013). Strong anthropogenic signals in historic forest fire regime: a detailed spatiotemporal case study from south-central Norway. Can. J. For. Res. 43, 836–845. doi: 10.1139/cjfr-2012-0462
Svenning, J. C. (2002). A review of natural vegetation openness in north-western Europe. Biol. Conserv. 104, 133–148. doi: 10.1016/S0006-3207(01)00162-8
Swetnam, T. W., and Baisan, C. H. (1996). “Fire effects in southwestern forests. USDA forest service Gen. Tech. Rep. RM-GTR-286,” in Proceedings of the Second La Mesa Fire Symposium, March 29–31, 1994, Los Alamos.
Szczygieł, R., Kwiatkowski, M., and Kołakowski, B. (2016). Forest fire risk at Białowieża Primeval Forest. Bezpiecz. Techn. Pożarnic. (Safety & Fire Technology) 43, 143–160. doi: 10.12845/bitp.43.3.2016.13
Szczygieł, R., Ubysz, B., and Zawiła-Niedźwiecki, T. (2009). “Spatial and temporal trends in distribution of forest fires in Central and Eastern Europe,” in Wildland Fires and Air Pollution, eds A. Bytnerowicz, M. Arbaugh, A. Riebau, and C. Andersen (Amsterdam: Elsevier), 233–245.
Szymczak, S., Bräuning, A., Häusser, M., Garel, E., Huneau, F., and Santoni, S. (2020). A dendroecological fire history for central Corsica/France. Tree Ring Res. 76, 40–53. doi: 10.3959/trr2019-2
Taylor, A. H., and Skinner, C. N. (1998). Fire history and landscape dynamics in a late-successional reserve, Klamath Mountains, California, USA. For. Ecol. Manag. 111, 285–301. doi: 10.1016/s0378-1127(98)00342-9
Tinner, W., Conedera, M., Ammann, B., and Lotter, A. F. (2005). Fire ecology north and south of the Alps since the last ice age. Holocene 15, 1214–1226. doi: 10.1191/0959683605hl892rp
Tolonen, K. (1986). “Charred particle analysis,” in Handbook of Holocene Palaeoecology and Palaeohydrology, ed. B. E. Berglund (Chichester: John Wiley & Sons), 485–496.
Ubysz, B., and Szczygieł, R. (2006). A study on the natural and social causes of forest fires in Poland. For. Ecol. Manag. 234:S13.
Walentowski, H., Kölling, C., and Ewald, J. (2007). Die Waldkiefer – bereit für den Klimawandel? LWF Wissen 57, 37–46.
Wallenius, T. (2011). Major decline in fires in coniferous forests – reconstructing the phenomenon and seeking for the cause. Silva Fennica 45, 139–155. doi: 10.14214/sf.36
Walter, H. (1968). Die Vegetation der Erde in Öko-Physiologischer Betrachtung. Band II: Die Gemäßigten und Arktischen Zonen. Jena: VEB Gustav Fischer Verlag.
Yamaguchi, D. K. (1991). A simple method for cross-dating increment cores from living trees. Can. J. For. Res. 21, 414–416. doi: 10.1139/X91-053
Zimny, M., Latałowa, M., and Pȩdziszewska, A. (2017). “The late-holocene history of forests in the strict reserve of Białowieża National Park,” in The Forests of the Strict Reserve of Białowieża National Park, ed. A. Keczyński (Białowieża: Białowieski Park Narodowy), 29–59.
Zin, E. (2016). Fire History and Tree Population Dynamics in Białowieża Forest, Poland and Belarus. Ph.D. Thesis. Uppsala: Swedish University of Agricultural Sciences.
Keywords: dendrochronological reconstruction, disturbance regime, fire history, land use history, lowland forests, Pinus sylvestris
Citation: Zin E, Kuberski Ł, Drobyshev I and Niklasson M (2022) First Spatial Reconstruction of Past Fires in Temperate Europe Suggests Large Variability of Fire Sizes and an Important Role of Human-Related Ignitions. Front. Ecol. Evol. 10:768464. doi: 10.3389/fevo.2022.768464
Received: 31 August 2021; Accepted: 21 January 2022;
Published: 08 March 2022.
Edited by:
Michał Słowiński, Institute of Geography and Spatial Organization (PAN), PolandReviewed by:
Cesare Ravazzi, National Research Council (CNR), ItalyCopyright © 2022 Zin, Kuberski, Drobyshev and Niklasson. This is an open-access article distributed under the terms of the Creative Commons Attribution License (CC BY). The use, distribution or reproduction in other forums is permitted, provided the original author(s) and the copyright owner(s) are credited and that the original publication in this journal is cited, in accordance with accepted academic practice. No use, distribution or reproduction is permitted which does not comply with these terms.
*Correspondence: Ewa Zin, RS5aaW5AaWJsZXMud2F3LnBs
†ORCID: Ewa Zin, orcid.org/0000-0001-6081-440X; Łukasz Kuberski, orcid.org/0000-0001-9815-9746; Igor Drobyshev, orcid.org/0000-0002-5980-4316; Mats Niklasson, orcid.org/0000-0002-2476-1694
Disclaimer: All claims expressed in this article are solely those of the authors and do not necessarily represent those of their affiliated organizations, or those of the publisher, the editors and the reviewers. Any product that may be evaluated in this article or claim that may be made by its manufacturer is not guaranteed or endorsed by the publisher.
Research integrity at Frontiers
Learn more about the work of our research integrity team to safeguard the quality of each article we publish.