- 1Centre for Ecology and Conservation, University of Exeter, Exeter, United Kingdom
- 2Konrad Lorenz Institute of Ethology, Department of Interdisciplinary Life Sciences, University of Veterinary Medicine, Vienna, Austria
- 3KRC Ecological Ltd., Bryansford, United Kingdom
Long-distance migration in birds is a complex syndrome that involves high energy costs and, in some species, substantial physiological re-organisation. Such flexible migratory phenotypes are commonly associated with bird species flying non-stop across vast ecological barriers, where there are few opportunities to stop and refuel en route. Prior to making migratory flights, some species have been found to atrophy organs that are not required (e.g., digestive organs) and grow those associated with powering flight (pectora muscles and heart), presumably to optimise costs. However, most studies of this flexibility have required sacrificing study animals and this has limited our capacity to measure individual variation and its potential consequences. Here we investigate the behavioural and, indirectly, physiological adaptation of an arctic breeding long-distance migrant the light-bellied brent goose Branta bernicla hrota, during spring staging in southwest Iceland. We use a sequential sampling approach to record behavioural observations and conduct stable isotope analysis of faecal samples from uniquely marked individuals to assess protein catabolism. Individuals showed a three-phase fuel deposition process, with initial slow intake rates followed by hyperphagia and then a period of inactivity immediately prior to migratory departure (despite multiple days with favourable wind conditions). The C:N ratio and δ15N values in faeces were significantly linked to fat deposition during the latter stages and suggests catabolism (reorganisation of proteins) occurring prior to departure. Our results suggest a strategic delay in migratory departure to enable reorganisation into a flying phenotype and that the extent of this varies among individuals.
Introduction
Long-distance migration is a remarkable behaviour involving the cyclical movement of animals often directed by seasonal variability of global resources (Piersma et al., 1999; Saino et al., 2010; Soriano-Redondo et al., 2020). Despite the high energetic costs of travelling such long distances, it is a strategy that enables species to expand their range, exploiting specific habitat niches best suited to their current life history stage (Hedenström, 2008). Although widespread in the animal kingdom, it is commonly associated with bird species and often characterised by extensive non-stop flight over vast ecological barriers (Saino et al., 2010; Hou and Welch, 2016). This can be physiologically demanding as sparse opportunities for groundings cause extended periods of fasting while still maintaining high metabolic activity (Lindström and Piersma, 1993; Piersma and Gill, 1998; Bauchinger and Biebach, 2001; Bauchinger et al., 2005; Scott et al., 2015; Remisiewicz et al., 2016).
To deal with the high energetic costs of migration, birds often rely on staging sites along their migration route. These act as stepping stones where individuals can rest and refuel before resuming the journey (Mehlman et al., 2005). Staging and refuelling birds undergo significant physiological changes, switching between wholly catabolic states during flight to a largely anabolic state during stationary periods (Landys-Ciannelli et al., 2003). In an effort to efficiently replenish energy stores and gain substantial amounts of adipose fat, birds will enter a state of hyperphagia upon arrival at the staging site (Lindström, 2003). However, fat accumulation is not the only physiological change occurring during a stopover (Karasov and Pinshow, 1998; Battley et al., 2000; Gannes, 2002). The avian digestive system is known to be flexible with associated muscles and organs undergoing hypertrophy during hyperphagic feeding and atrophy during flight induced fasting (McWilliams and Karasov, 2001; McWilliams et al., 2004). Likewise, organs involved in maintaining high performance sustained migratory flights such as pectoral muscle and heart, undergo hypertrophy in the days prior to departure from the staging grounds (Marsh, 1984; Gaunt et al., 1990; Evans et al., 1992; Piersma, 1998; Piersma et al., 1999; Lindström et al., 2000; Bauchinger and Biebach, 2005; Dietz et al., 2007). Due to the excessive metabolic cost needed to maintain the digestive system and the energetic cost associated with carrying its weight, Piersma (1998) suggests that long-distance migrants with no immediate necessity for these organs gain an advantage in reducing them before embarking on a migratory flight. For example, bar-tailed godwits (Limosa lapponica), increase stomach mass by 30% during the first half of spring staging only to decrease its mass by 20% before migratory departure (Piersma and Lindström, 1997; Piersma and Gill, 1998). Jehl (1997) provides further evidence for this migratory strategy of phenotypic flexibility, finding eared grebes (Podiceps nigricollis) pre-departure reduce their stomach, liver and intestine by up to 57%.
The energetic constraints for avian migrants vary according to environmental conditions encountered en route and their life history strategies, the latter being particularly acute for large-bodied High Arctic breeders. Given the scarce foraging opportunities available on arrival at the breeding site, they are often capital breeders, relying on endogenous resources to initiate and finance breeding (Drent et al., 2007; Drent and Daan, 1980; Jaatinen et al., 2016). While Arctic migrants can benefit from 24-h daylight and abundant food resources over summer, this is restricted to a narrow period where conditions are optimal to successfully breed and fledge offspring (Poussart et al., 2000; Drent et al., 2007; Jónsson et al., 2009). Reproductive success is shown to significantly decline with a later laying date, however, eggs laid earlier in the season were found to be formed using a higher proportion of endogenous resources (Lepage et al., 2000; Bêty et al., 2004; Schmutz et al., 2006). This highlights the importance for High Arctic migrants to gain adequate resources at staging sites along the flyway, enabling a timely arrival and rapid initiation of breeding (Hupp et al., 2006; Schmutz et al., 2006).
A number of studies have highlighted the extent of phenotypic flexibility during spring migration in birds [e.g., Red knot (Calidris canutus rogersi): Battley and Piersma, 1997, (canutus/islandica) Piersma et al., 1993; Great knot (Calidris tenuirostris): Battley et al., 2000, Eared Grebe: Jehl, 1997, Bar-tailed Godwit: Piersma and Gill, 1998, Dark-eyed junco (Junco hyemalis): Ramenofsky et al., 1999, Garden warbler (Sylvia borin): Biebach, 1998; Bauchinger et al., 2005, Greater snow goose (Anser caerulescens atlanticus): Gauthier et al., 1984]. However, our understanding of this phenomenon has often come from a small number of sacrificed individuals, as shown in the above-mentioned studies, or from controlled feeding and simulated migration experiments (e.g., Karasov et al., 2004; Pierce and McWilliams, 2004). Research specifically interested in flight physiology, measuring changes in protein and lipid catabolism are able to use non-invasive sampling techniques in situ (e.g., pigeons (Columba livia): Hatch et al., 2002, Western sandpiper (Calidris mauri): Guglielmo and Williams, 2003]. However, many of these studies remain cross sectional, only measuring an individual’s physiological state once. These methodologies inhibit the study of within-individual level variation and the potential link to downstream fitness consequences (Piersma and Gill, 1998). Our study presents a route to overcoming difficulties in longitudinal sampling by using behavioural observations and stable isotope analyses of faecal deposits as proxies for large scale reorganisation of internal tissues. We sequentially sampled from marked light-bellied brent geese (Branta bernicla hrota) during their spring migration staging in southwest Iceland. While stable isotope measures: C:N ratio, 13C/12C (δ13C) and 15N/14N (δ15N) have been extensively used to characterise the diets of animals, they can also be used to infer physiological reorganisation (Hobson and Clark, 1992; Inger and Bearhop, 2008). Birds experiencing nutritional stress regardless of diet are known to mobilise and deposit proteins between bodily tissues resulting in additional isotopic discriminations and the enrichment of heavier nitrogen isotope in faeces (Hobson and Clark, 1992). By considering stable isotope analyses alongside food intake rate there is the potential to infer rates of protein catabolism in staging migratory birds. This in turn allows us to assess the extent to which individual animals exhibit phenotypic flexibility, through the reorganisation of body proteins in preparation for a period of migratory flight and subsequent fasting. The east Canadian High Arctic population of Light-bellied brent geese are an excellent model system for such a study. They are herbivorous capital breeding migrants, staging in west Iceland prior to a long-distance flight into the breeding grounds and there are a large number of individually marked birds in the population making it easy to follow individuals through time (Inger et al., 2010).
We predict that phenotypic flexibility will be evident in the light-bellied brent geese during their one staging event on spring migration, manifesting as (1) elevated foraging and locomotor activity soon after arrival, (2) increased δ15N and decreased C:N ratio in feaces just prior to migratory departure, and (3) a period of reduced foraging and locomotor activity prior to departure irrespective of favourable wind conditions being present.
Materials and Methods
Study System
We conducted our study in Álftanes (southwest Iceland, 64° 06′N, 22° 00′W), a main spring staging site for light-bellied brent geese of the East Canadian High Arctic population (ECHA). We carried this study out during the brent goose spring migration staging (01/05–27/05/2017), a critical period of intense foraging, where birds accumulate energy stores for migratory flight and breeding (Inger et al., 2008, 2010). In late April, the population has a staggered migration from wintering grounds along the Irish coasts to staging sites in west Iceland (Inger et al., 2008). They typically resume migration en masse approximately one month later to their breeding grounds in the Canadian High Arctic (Gudmundsson et al., 1995; Clausen et al., 2003; Robinson et al., 2004). The ECHA population amounts to approximately 35000 individuals of which over 10% are uniquely marked with coloured Darvic leg rings displaying individual alphanumeric combinations (Cleasby et al., 2017). We conducted this study on marked individuals only and all observations in this study were made from inside of a vehicle, through an Opticron HR 80 GA ED telescope with an SDLv2 40936 zoom eyepiece (×54 zoom) and Meopta Meo Star B1 12 × 50 HD binoculars, causing minimal disturbance to geese. We observed birds at opportunistically selected field sites around Álftanes from 6.00 h to 22.00 h.
The age of geese (juvenile/adult) was visually determined in the field by observation of plumage colouring along the edges of wing coverts, clearly distinguishing juveniles from adults (Reed, 1993). We identified relationships between individuals [partners/family with parent(s) and offspring/single adults/single juveniles] by observing proximity and cohesion during birds’ movements, which was found to represent a reliable method of identifying first-order relatedness among individuals (Harrison et al., 2010). We recorded flock size and considered individuals at least 20 goose lengths apart as a different flock.
Abdominal Profile Index
We opportunistically re-sighted marked birds and recorded fat deposition on foraging geese by visually determining abdominal profile indices (API; Owen, 1981; Boyd et al., 1998; Clausen et al., 2003; Madsen and Klaassen, 2006) on a seven-point scale (1–7; 1 being lowest and 7 the highest score; Inger et al., 2008) with accuracy of 0.5. The API score is linearly related to body mass and highly correlated with the size of abdominal fat reserves (Fox et al., 1999; Madsen and Klaassen, 2006). It is commonly used on geese and shorebirds as they are known to predominantly store energy in their abdominal cavity (Owen, 1981; Wiersma and Piersma, 1995; Boyd, 2000; Tinkler et al., 2007). We used APIs as an indication of body condition and weight gain. We have evidence from this population and other closely related taxa that it correlates with body mass and subsequent reproductive success (Ebbinge and Spaans, 1995; Tinkler et al., 2007; Inger et al., 2008; Harrison et al., 2011; Harrison et al., 2013).
Peck and Pace Rate
We measured foraging behaviour of individual geese through focal sampling of peck and pace rates. Identifiable geese were initially randomly selected then resampled each week of the staging period. We classified pecks as movement of the beak toward the ground, thereby touching the substrate, and paces as every step taken by an individual (Inger et al., 2006a). We used tally counters to record the number of pecks and paces over 1–2 min and obtained a rate by dividing the number of pecks/paces by the total length of observation. We did not include observations in which birds simultaneously did not peck nor pace in this study. Additionally, we recorded length and occurrence of sitting behaviour when geese sat on the ground during a focal observation.
Stable Isotopes
We used faecal sample collection of marked geese for stable isotope analyses. Given brent geese feed on saltmarsh (marine) and terrestrial grasses, which are isotopically distinct with δ15N values being lower in terrestrial grasses and δ13C values being considerably higher in marine grasses, we classified all field sites accordingly accounting for the resulting difference in carbon and nitrogen stable isotope ratios in faecal samples (Inger et al., 2006b). For collection of faecal samples, we split the field season into three time periods: early (May 1–10) reflecting arrival and the onset of hyperphagia, mid (May 11–19) reflecting a full hyperphagia and late (May 20–27) reflecting the cessation of hyperphagia and preparation for migration. We chose these time periods in response to the feeding rate of geese measured across the spring, i.e., initial increase of feeding rates and API (onset of hyperphagia), reaching a peak toward the end of the staging season (full hyperphagia) and a subsequent drop of feeding rates and API until departure (cessation of hyperphagia). Such a mass trajectory and feeding rate pattern has also been recorded in other Branta populations during spring staging, for example in dark-bellied brent geese (Branta bernicla bernicla) on their last spring staging site before embarking on a long-distance flight to their Arctic breeding grounds (Dokter et al., 2018). In the early period, we collected samples from a range of identifiable individuals and then resampled from these target individuals in each subsequent time period, to track individual variation throughout staging. We collected faecal samples from known individuals with one person observing the targetted goose until it defecated and a second collecting the sample by following directions of the observer through two-way radios. Faecal samples were stored at −20°C in conventional plastic-zip lock bags until analyses.
We subsampled faecal samples along a central horizontal plane of the cylindrical pellet. We freeze-dried samples, ground them into a homogenous powder, and weighed them to 0.7 mg (±0.1 mg) in tin capsules. Stable carbon and nitrogen isotope measurements were determined by a Thermoquest EA1110 elemental analyser interfaced with a Europa Scientific 2020 isotope ratio mass spectrometer (Elemtex Ltd., Cornwall, United Kingdom). Stable isotope ratios were expressed as δ notation, measured in parts per thousand (‰) relative to international standards Pee Dee Belemnite for carbon and air for nitrogen according to the following equation: δX = [(Rsample/Rstandard) – 1] × 1000 where X is 15N or 13C and R is the corresponding ratio of 15N/14N or 13C/12C. We report stable isotope ratios as δ13C values for carbon and δ15N values for nitrogen and data are corrected for sample mass and instrument drift. Measurement precision (standard deviation), based on within-run replicate measures of the laboratory standard, was better than 0.25‰ for δ15N and 0.21‰ for δ13C isotopic values.
Wind Conditions
Past studies have shown that long-distance migrants will time their departure to maximise wind support (Grönroos et al., 2012). Using the package “RNCEP” (Kemp et al., 2012) in R, we interpolated the 10m above ground U wind (east/west) and V wind (north/south) components (m/s) for a central point on the Álftanes peninsula (64° 06′N, 22° 00′W) using the NCEP/NCAR dataset (Kalnay et al., 1996), provided by the NOAA/OAR/ESRL PSD, Boulder, Colorado, United States1. Measurements were interpolated on the hour each day between 15 May (15 days prior to departure) and 5 June 2017 (5 days post departure) inclusive.
To model U and V wind as a function of wind support and crosswind we estimated the birds flight direction (heading) on migratory departure using the “geosphere” package (Hijmans et al., 2017). By studying the migratory tracks obtained from birds in this population, it is known that light bellied brent geese fly west north west toward Greenland, therefore a reference point on the southeast coast of Greenland was used to calculate heading (65° 42′N, 37° 42′W). Wind support and crosswind were calculated using methods described by Safi et al. (2013) (Figure 1). Positive values associated with wind support represented a tail wind and negative values a head wind. Crosswind was taken as an absolute value irrespective of direction (Safi et al., 2013).
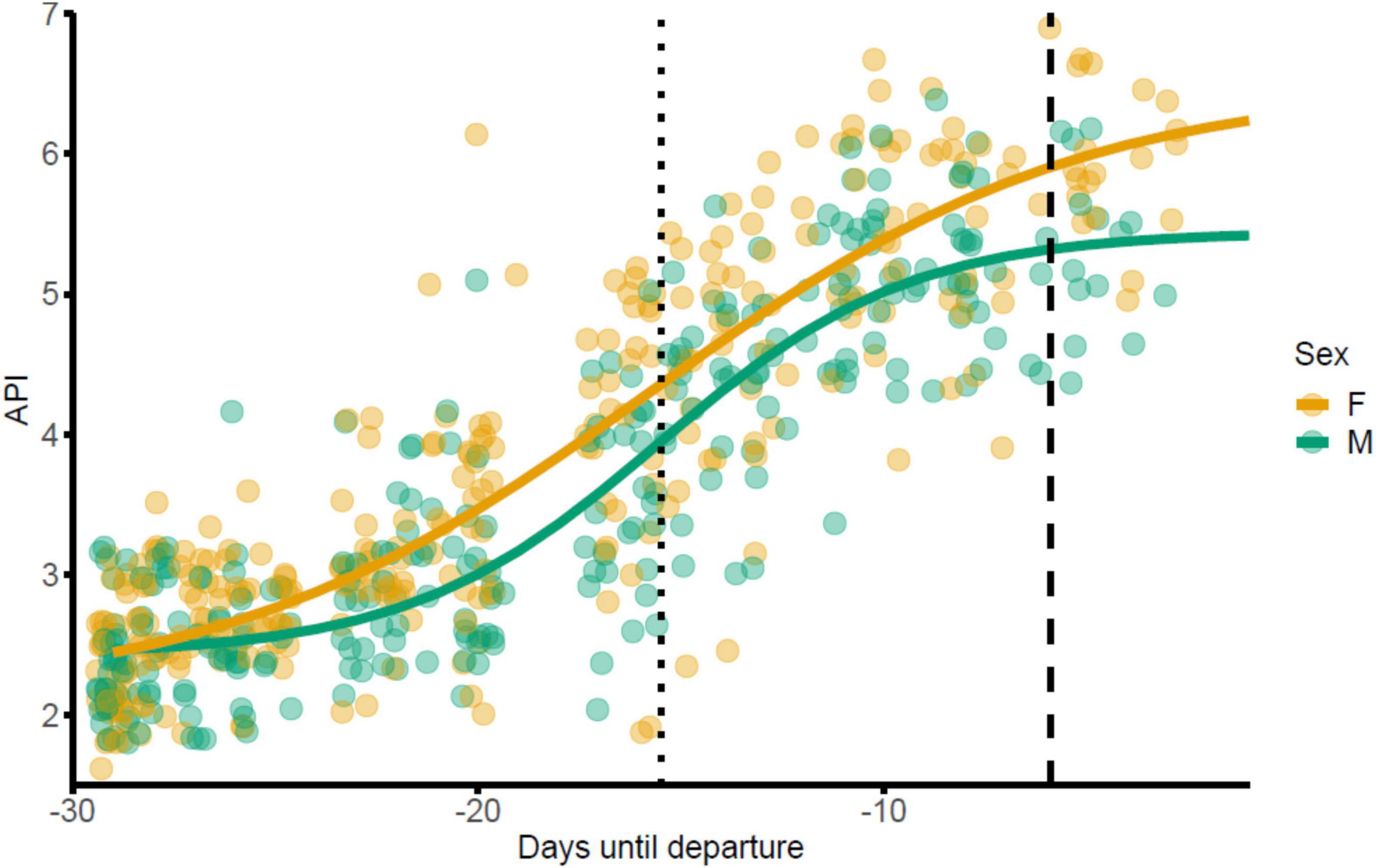
Figure 1. Increase in body condition of brent geese during spring staging in Álftanes, Iceland, as measured by the abdominal profile index (API). Migration to breeding grounds was resumed en masse by brent geese on 30/05/17. Females are represented in orange and males in green. The dotted line is the point of inflection, when the rate of weight gain begins to slow (x = 14–15/05/17). The dashed line represents the trough in the second derivative of the sigmoidal function, when the rate of weight gain begins to attenuate (x = 24/05/17).
Statistical Analyses
We conducted all data analyses using R software version 3.3.1. (R Core Team, 2016). We determined the sex of marked individuals as described in Bodey et al. (2020) by using a discriminant function based on morphological differences between wing length and head-bill of male and female individuals, recorded during catches when darvic rings are attached. All birds that did not fit male or female morphological criteria were classified as “unknown” and were excluded from statistical analyses.
Abdominal Profile Index
To determine the inflection point in observed API values in our dataset, we defined an nls function in the native stats package using API as a dependent variable and including date in May as an independent variable. For this function, we modelled date in May as sigmoid equation as the sigmoid curve best fit the distribution of API values and therefore allowed for an adequate estimation of the inflection point. We further used a linear plateau model to determine an approximate date during spring staging when weight gain of brent geese attenuated. We defined an nls function in the native stats package with date and API score as x and y variables along with three parameters aiming to define the best fit intercept, slope, and critical x value (Mangiafico, 2016). Once we determined the critical x value (=date when API started attenuating), we used linear models, fitted with the R package lme4 (Bates et al., 2014), to test if API and date in May were correlated before API score attenuation and after API attenuation. We used API as a dependent variable and individual sex and date in May as independent variables. We did not add ID of geese as a random effect to the models as each individual only occurred once in the dataset.
Peck and Pace Rate
We used the glmmTMB R package (Magnusson et al., 2017) to design global generalised linear mixed models (GLMM) for peck and pace rates of individuals. We fitted both models with negative binomial errors and a log link function. The models had either peck or pace rate as the dependent variable and z transformed: Julian date, API, flock size (log transformed); whether the birds had a partner and/or juveniles, sex, and resource type (marine vs. terrestrial) as independent variables. We used z transformations for API, Julian date and flock size as their scales had a large amount of variation. Furthermore, we included the individual identification (leg ring combination) of each sampled goose as a random intercept term into the models as most geese were sampled multiple times throughout staging. We selected minimal adequate peck and pace rate models (MAMs) by using the dredge function of the R package MuMIn (Barton, 2009). The dredge function returns a list of all possible models with the given predictors, which we set to be ranked by second-order Akaike information criterion (AICc) values (Burnham and Anderson, 2004). For both (peck and pace) MAMs we chose the model with the lowest AICc value. To investigate changes in proportional occurrence of sitting behaviour in every week of staging, we used a repeated-measures ANOVA and Tukey’s post hoc test with Bonferroni correction.
Stable Isotopes
To determine the effect of independent variables: API, date, resource type (marine vs. terrestrial) and sex on the dependent variables: C:N ratio, 13C/12C (δ13C) or 15N/14N (δ15N) values obtained from faecal samples, we fitted linear mixed models using the lme4 R package (Bates et al., 2014). We added the individual goose ID as a random intercept term in the models. API was included as either the individuals recorded value (1–7) or categorised as below, above or at population average (group mean centring). We determined population average using the daily mean API values from re-sighting data collected throughout the staging period in Álftanes. We achieved MAMs by creating all possible models with the given predictors using the R dredge function and AICc comparisons. To determine the effects of date, habitat and sex on API (in the Stable isotope dataset), we fitted another linear mixed model with the given responses and individual goose ID as random effect. We used a Tukey’s post hoc test to determine differences in δ15N, δ13C and C:N for above/below API individuals across the weeks of spring staging.
Results
We recorded 1,185 re-sightings of 292 uniquely identifiable light-bellied brent geese between May 1–27, 2017 on 9 intertidal saltmarshes and 14 terrestrial field sites. Most birds arrived at study sites before the start of data collection (last days of April) but bird numbers continued to increase during the first week of our study. The bulk of the staging population departed en masse from the study area on May 30.
Abdominal Profile Index
The linear plateau model indicated that individuals in this study population reached a plateau in weight gain measured as an abdominal profile index (1–7), of approximately 6 between May 24 and 25 (n = 187 observations, 187 individuals, critical day value = 24.5, 95% CIs: 20.8–28.2, plateau y = 5.7, p < 0.001; Figure 1). Furthermore, a significant positive linear correlation between API and the number of days into staging was observed until May 24 (n = 172 observations, 172 individuals, r2 = 0.70; est. = 0.15, SE = 0.01, p < 0.001) but no such correlation was observed thereafter (n = 13 observations, 13 individuals, r2 = 0.41; est. = 0.05, SE = 0.22, p = 0.81). However, after May 24 female geese had higher API compared to males (n = 13 observations, 13 individuals, r2 = 0.41; est. = 22120.96, SE = 0.33, p = 0.02). A sigmoid function modelling API by day shows both males and females experience the inflection point in weight gain 15.3 days prior to departure (n = 187 observations, 187 individuals; r2 = 0.80, est. = 15.31, SE = 0.71, p < 0.001; Figure 1).
Peck and Pace Rate
The top ranking peck rate model, selected by lowest AICc value, included Julian date, the quadratic term of Julian date and resource type as independent variables (AICc = 1757.8, AICc null model = 1759.2; Table 1; model selection table in Supplementary Material 1). As might be expected given the trajectories, only the quadratic effect of Julian date had a statistically significant negative influence on peck rates during staging (n = 154 observations, 73 individuals, est. = −0.133, SE = 0.054, p = 0.013), meaning that peck rates of geese initially increased in the earlier part of the staging period and then decreased again at the end of staging (Figure 2).

Table 1. GLMM output with peck rate as dependent variable and food resource type, Julian date and quadratic effect of Julian date as independent variables.
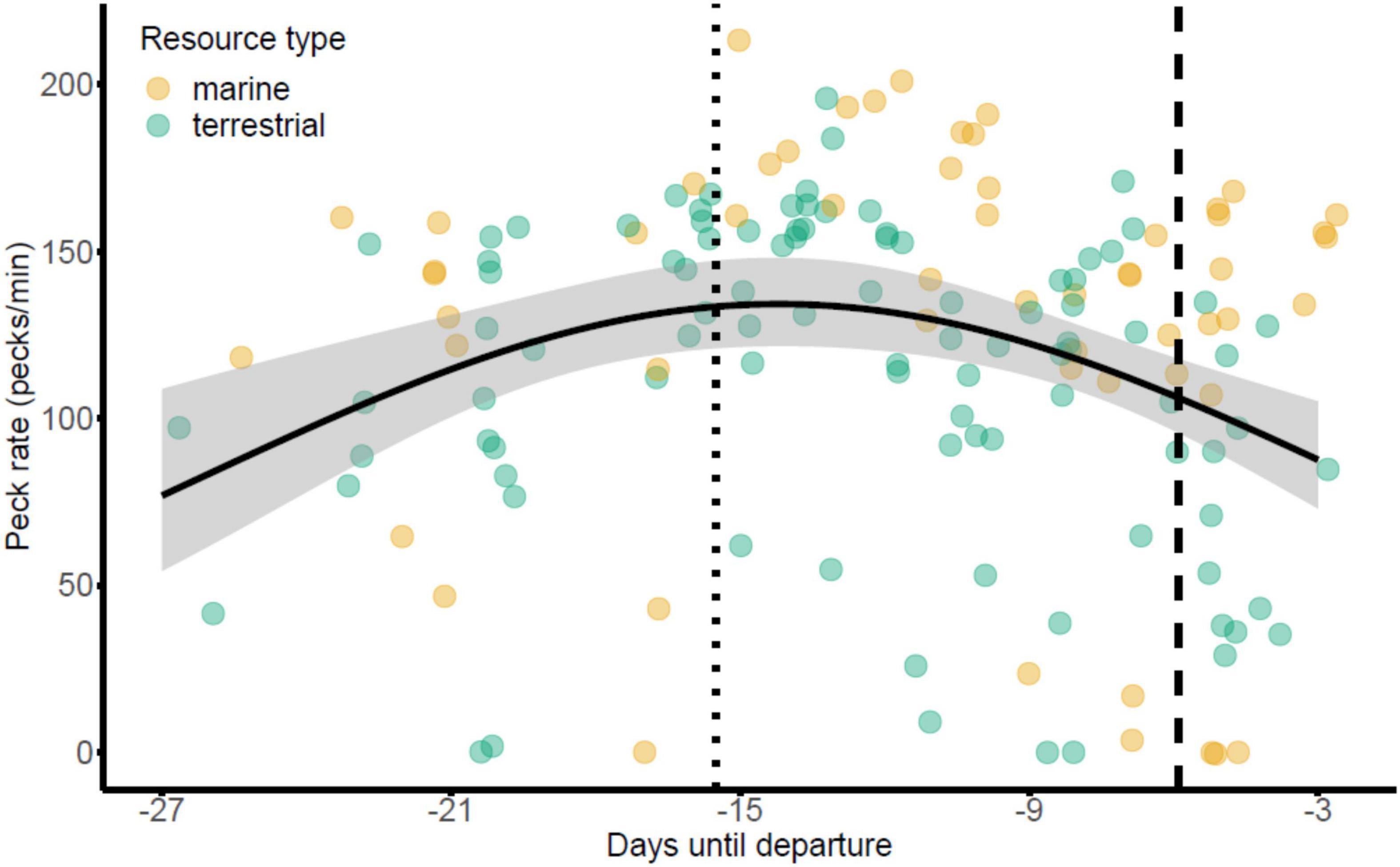
Figure 2. Relationship between peck rates and days until mass departure of light-bellied brent geese from their spring staging site in Álftanes, Iceland. The resource type being fed on during focal observations is coloured as either yellow for marine (saltmarsh) or green for terrestrial (grassland) resources. The black line depicts the quadratic effect of date on peck rates and grey shading represents the standard error. The dotted line is the point of inflection, when the rate of weight gain begins to slow (x = 14–15/05/17). The dashed line represents the trough in the second derivative of the sigmoidal function, when the rate of weight gain begins to attenuate (x = 24/05/17).
The best performing pace rate MAM only included Julian date as an independent variable but only differed marginally from the null model (AICc = 1,357.2, AICc null model = 1,357.5; see Supplementary Material 2 for AICc model selection table). Julian date did not influence pace rates statistically significantly (n = 154 observations, 73 individuals, est. = −0.089, SE = 0.058, p = 0.121). Yet, we observed that sitting behaviour differed statistically significantly between the four weeks of staging (F1,3 = 6.727, p < 0.001) and a Tukey post hoc test revealed that sitting behaviour was higher in the last week of staging compared to the previous three weeks (week 1 vs. week 4: p < 0.001; week 2 vs. week 4: p = 0.044; week 3 vs. week 4: p = 0.004).
Stable Isotopes
The best performing MAM for δ13C included habitat type as response variable whereas the best performing MAM for δ15N included habitat type as well as individual API. For C:N the best MAM included API and habitat type as well as their interaction as response variables. All three stable isotope measures showed a significant difference between samples collected on terrestrial or marine habitats, specifically δ13C and δ15N were both higher on marine sites (n = 130 observations, 59 individuals, δ13C: r2 = 0.35; est. = −1.42, SE = 0.17, p < 0.001; δ15N: r2 = 0.29; est. = −1.05, SE = 0.36, p = 0.004). We found δ15N was statistically significantly higher in birds with higher API (n = 130 observations, 59 individuals, δ15N: r2 = 0.29; est. = 0.33, SE = 0.11, p = 0.004, Figure 3C) and lower C: N ratios were found in faecal samples of individuals with a higher API (n = 130 observations, 59 individuals, r2 = 0.28, est. = −1.72, SE = 0.76, p = 0.02, Figure 3A). However, the results show no significant relationship between an individual’s API and habitat type they are feeding on (n = 130 observations, 59 = individuals; r2 = 0.83, est. = 0.24, SE = 0.14, p = 0.08). Using a Tukey post hoc test we also found that individuals with higher API than the population average had significantly different δ15N ratios in their faeces compared to those with lower than average API (p = 0.03, Figure 4C). Both individuals with above and below average API were feeding on similar resources with no significant difference in the δ13C values (p = 0.99, Figure 4B), there was also no difference in the C: N ratio in faeces (p = 0.16, Figure 4A).
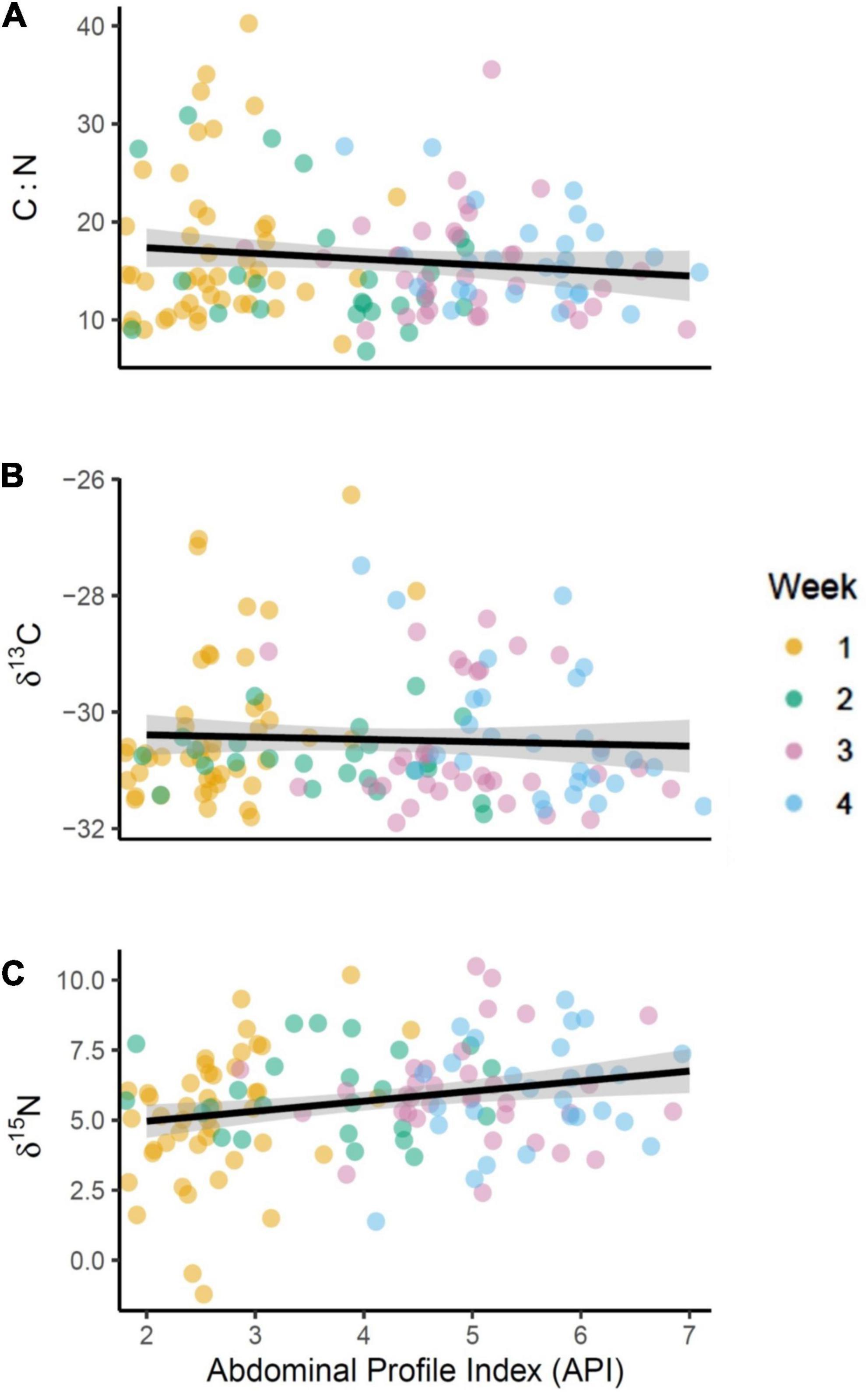
Figure 3. Variation in stable isotope measures (A) C:N ratio, (B) δ13C, and (C) δ15N associated with weight gain by brent geese during spring staging in Álftanes, Iceland (03/05/2017–27/05/2017). Weight gain measured according to abdominal profile index (API). Data displayed by the week of spring staging when the faecal sample was collected, orange (week 1), green (week 2), purple (week 3), and blue (week 4). Linear regression with standard error (grey shading). An increase in API had a significantly negative effect on C:N ratio (χ21 = 0.85, p = 0.039, r2 = 0.28) and no significant effect on δ13C (p = 0.36, r2 = 0.37). The δ15N values were significantly influenced by an interaction between staging week and API (χ21 = 8.10, p = 0.044, r2 = 0.26).
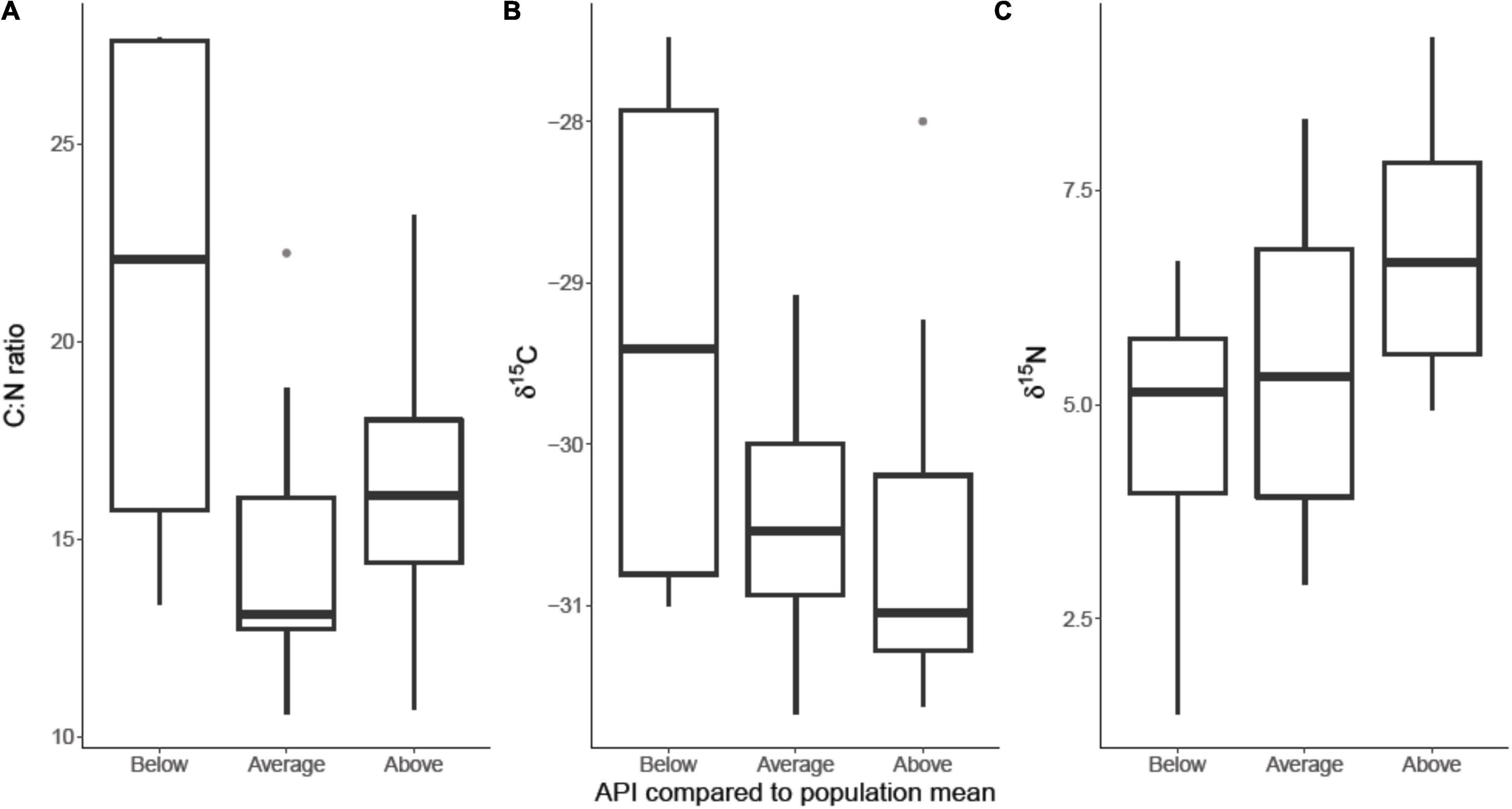
Figure 4. Variation in stable isotope measures (A) C:N ratio, (B) δ13C, and (C) δ15N associated with body condition of brent geese during the final week of spring staging in Álftanes, Iceland (20/05/2017–27/05/2017). Body condition measured as above, below or at population average for abdominal profile index (API). In week 4 individuals with an above-average API excreted significantly higher δ15N values than those with a below-average API (Tukey post hoc, p = 0.025).
Wind Conditions
Geese experienced an average wind support of +1.23 m/s between 15 May and 5 June, indicating a tail wind (Figure 5A) and a crosswind of 5.21 m/s (Figure 5B). On the evening before departure the geese experienced a strong head wind (−6.78 m/s) but this dropped at midnight to −1.11 m/s and became a light tail wind (0.17 m/s) at 06:00 30/05/21. There was an above-average crosswind (6.89–7.51 m/s) present between midnight and 11:00 on the day of departure. Favourable wind support (i.e., tail wind) and crosswind conditions suitable for migratory departure were frequently present between 24 May when API attenuates and 30 May when the geese depart en masse and in the days following (Figure 5).
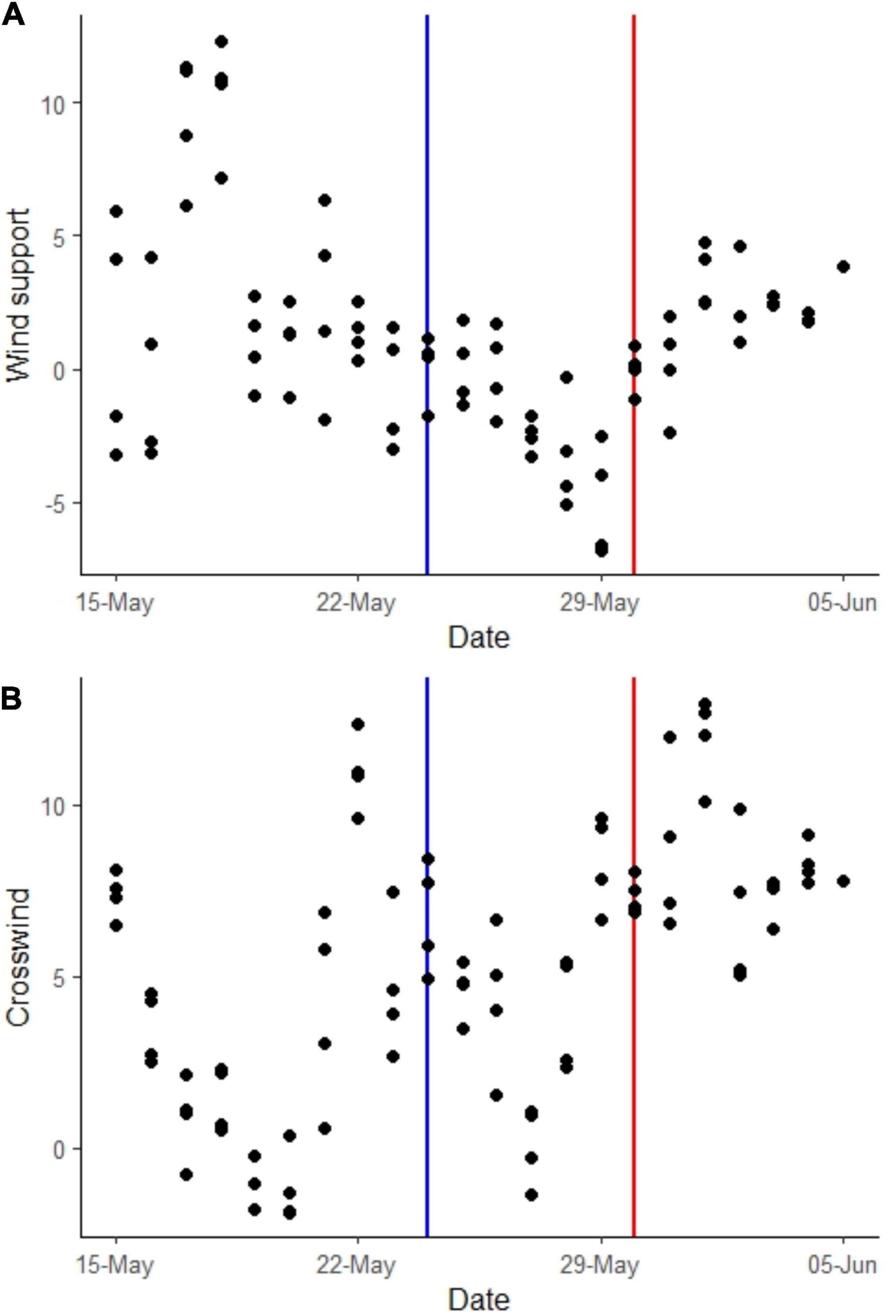
Figure 5. Wind conditions experienced by light-bellied brent geese (LBBG) staging on the Álftanes peninsula between 15/05/17 and 05/06/17. The blue line is the date when LBBG weight gain attenuates (24/05/17) and the red line is the date of their mass departure (30/05/17). Wind conditions were calculated as either (A) wind support, with positive values indicating a tailwind and negative values a head wind and (B) crosswind was taken as an absolute value irrespective of direction. Calculated with a reference heading direction on the southeast coast of Greenland (65° 42′N, 37° 42′W).
Discussion
Light-bellied brent geese demonstrate behavioural and physiological flexibility during their one-month spring staging period in southwest Iceland. The results show that weight gain in brent geese begins attenuating approximately seven days prior to departure from Iceland, with females being on average fatter than their male partners (Figure 1). Despite early arrival at breeding grounds being a key driver of hatching success, individuals chose not to capitalise on favourable wind conditions occurring during the last week of staging (Figure 5). This suggests that environmental cues are not the only factor driving migratory departure. The observed behavioural changes (Figure 2) and stable isotope results across the month of staging (Figures 3, 4) support the hypotheses that brent geese exhibit a period of hyperphagia followed by reduced foraging in response to migratory weight gain requirements. There is also evidence of protein reorganisation occurring prior to departure with a decrease in the C: N ratio (higher proportion of N in the faeces) and higher δ15N (indicating protein catabolism) toward the end of the staging period. This supports the prediction that physiological adjustments are occurring in preparation for the upcoming flight and capital breeding life history strategy. Of course, there could be other explanations for such a pattern, for example, if resources were abundant and departure date was hardwired due to constraints on the breeding grounds. However, 2017 was a typical year in terms of arrival dates, feeding rates and habitat choice. Moreover, fluctuating conditions on route and at arrival on the breeding grounds would tend to suggest that there should be some ability to optimise departure decisions.
Behaviour
Throughout the month of spring staging in Iceland, light-bellied brent geese significantly changed foraging rates and locomotion behaviour in relation to the time of staging. Initially geese arrived with low API, however despite this, we observed low foraging rates. This is often explained by the “gut limitation hypothesis,” whereby an individual experiences slow regeneration of digestive efficiency after a period of fasting (McWilliams and Karasov, 2005). The approximately 1,500 km non-stop migratory flight from Northern Ireland induces a period of fasting likely to cause atrophy of disused organs such as the digestive system. Regeneration of organs requires time and energy, hence an initial slow foraging period upon arrival in Iceland (McWilliams and Karasov, 2005). This has been observed in lesser snow geese, Chen caerulescens caerulesces where lower foraging rates were associated with individuals carrying atrophied digestive organs (Ankney and MacInnes, 1978). Furthermore, this hypothesis was also supported in passerine experiments, showing low food intake rates after a period of fasting even when food was offered ad libitum (Klaassen and Biebach, 1994; Hume and Biebach, 1996; Klaassen et al., 1997; Karasov and Pinshow, 2000; Karasov and McWilliams, 2005).
We found the time from arrival was the strongest predictor of variation in foraging rates during spring staging. After the initial slow start geese entered a state of hyperphagia, excessively eating in an effort to store enough energy to fuel the continuation of migration and initiation of breeding upon arrival in the High Arctic. As abdominal fat deposits increased so did the foraging rate but both foraging rates and fat gain decreased in the last period of staging, likely due to individuals approaching the target mass required to fuel migration and breeding. Additionally, it becomes more energetically advantageous to maintain a threshold weight as opposed to continue increasing bodyweight (Lindström, 2003), so in this last period before departure fatter individuals were observed sitting more. On average males had a lower threshold weight than females, potentially the result of mate guarding behaviour and a difference in energetic demand experienced by the sexes during long-distance migration and a capital breeding strategy. The reproductive success of female brent geese can change from 8 to 65% with an increase in their body mass at the breeding grounds (Inger et al., 2008). Light-bellied brent geese from the adjacent Svalbard/Greenland population are known to lay eggs within a week of arriving (Clausen et al., 2003), highlighting the importance of accumulating large fat deposits prior to departing the spring staging site.
The delay in mass departure despite attenuation of weight gain by both sexes suggests that there are other physiological limitations prolonging the staging period in Iceland. In response to fasting and in-flight starvation waterfowl and wader migrants have been shown to experience protein turnover within their organs (Bauchinger and McWilliams, 2010). Lesser snow geese, a similar-sized waterfowl were found to atrophy organs during a 5–6 day period of decreased foraging rates (Ankney and MacInnes, 1978). This is also commonly seen in migratory wader species such as red knots, Calidris canutus found to have a pre-departure turnover of protein, with the digestive organs shrinking and flight muscles (i.e., pectoral) increasing in size (Piersma et al., 1999). This phenotypic flexibility is a potential explanation for the behavioural changes observed in the brent geese during their pre-departure week of spring staging.
Physiology
The stable isotope component of this study in conjunction with the behavioural observations provides further evidence of phenotypic flexibility occurring in the light-bellied brent geese during spring staging in Iceland. We found significant changes in the C:N ratio and δ15N in the faeces of brent geese, in response to reaching peak abdominal fat. Both are indicators of a higher rate of protein turnover that would be expected in animals undergoing fasting and tissue-specific reorganisation, in this instance in preparation for the non-stop migratory flight to their breeding grounds in the Canadian High Arctic.
In comparison to other capital breeding migrants brent geese are physiologically constrained by a large energy requirement to body size ratio (Buchsbaum et al., 1986; Battley and Piersma, 2005). Their solely herbivorous diet has low nutrients and is high in structural components, with cellulose in plant cell walls making it hard to breakdown and digest (Buchsbaum et al., 1986). Therefore, they require a large gizzard and large small intestine to efficiently acquire nutrients and accumulate crucial energy deposits, constituting ∼95% lipids and ∼5% proteins, needed to fuel migration (Sedinger et al., 1989; Jenni and Jenni-Eiermann, 1998; Klasing, 1998; Battley and Piersma, 2005; Rozenfeld and Sheremetyev, 2016). Female geese in particular have a high energetic demand requiring significant fuel accumulation during the month of spring staging to rapidly initiate breeding on arrival and successfully breed. However, a large digestive system takes up crucial space within the abdomen and is metabolically costly during flight and capital breeding. Digestive organs constitute approximately 10% of a bird’s lean mass making it a significant burden on wing load (Pierce and McWilliams, 2004). The measured decrease in C:N ratio in brent goose faeces associated with heavier individuals as the staging month progressed is a likely result of a physiological trade-off occurring. As adipose fat is accumulated in the abdomen we can infer that the digestive organs are compromised to maximise available space (Battley and Piersma, 2005). Waterfowl are known to compensate for the low nutrient concentrations in their herbivorous diet by having a long small intestine improving nutrient absorption and also recycle excretory and endogenous nitrogen in the caecum, improving overall nitrogen balance (Sedinger, 1997; Barboza et al., 2008, p. 156). Given this, a compromised digestive system and resulting reduction in organ size will likely cause a reduction in assimilation efficiency causing the excretion of dietary nitrogen (Barton and Houston, 1993; Sedinger, 1997).
The results also show a significant increase in δ15N of faecal samples as the month progresses and individuals accumulate abdominal fat stores. A higher δ15N is usually indicative of an animal feeding at a higher trophic level (Bearhop et al., 2000; Inger and Bearhop, 2008). However, brent geese are strictly herbivorous and we did not observe a change in their resource use. A study by Fuller et al. (2005) confirmed that δ15N values were not only influenced by dietary intake but also fluctuations in the body’s nitrogen homeostasis, suggesting that the increase in δ15N in the goose faeces might have an internal physiological explanation. Migratory birds are known to build, dispense and restore components of their body throughout their annual life history stages, strategically enhancing fuel storage and reducing flight costs (Fox and Kahlert, 2005). Empirical evidence from terminal studies on long-distance migratory water birds demonstrates pre-departure atrophy with up to 50% reduction in digestive tissues occurring at the staging site (Piersma et al., 1993; Jehl, 1997; Piersma and Lindström, 1997; Piersma and Gill, 1998).
The reorganisation of internal organs prior to migratory departure enables a bird to reallocate proteins away from the expensive disused digestive system into the synthesis of flight muscles (McLandress and Raveling, 1981; Jehl et al., 2015). When an animal’s body is in a stable feeding state, assimilation of dietary protein tends to result in light 14N being preferentially excreted, resulting in an increase in body tissue δ15N, relative to the diet (Bearhop et al., 2000; Inger and Bearhop, 2008; Krausman and Cain, 2013). However, the enrichment of 15N seen in the faeces of heavier brent geese is commonly found during periods of nutritional stress when higher rates of protein catabolism can often occur (Hobson et al., 1993). In this case, we have inferred that the increase in δ15N represents a controlled reallocation of proteins in preparation for migratory departure.
An alternative explanation for the increase in δ15N in the faeces is that the geese are entering a state of starvation in response to the low food intake, initiating the catabolism of proteins as a source of energy. However, this is unlikely as birds and mammals are known to prioritise lipid utilisation until fat stores drop below a critical limit (Jenni et al., 2000). For migratory bird species, this was shown to be as low as 5–10% of fat remaining and only then do they shift to catabolising protein to meet energy requirements (Cherel et al., 1988; Schwilch et al., 2002). Given that abdominal fat stores remain constant once a threshold weight is reached, it is unlikely that the protein catabolism measured in this study using stable isotope techniques is the result of foraging rates decreasing (i.e., starvation), rather shows phenotypic flexibility in the migratory light-bellied brent geese.
Despite female brent geese carrying a higher energetic burden upon reaching the breeding grounds, the sexes showed no difference between the δ15N values and C:N ratio in their faeces. This highlights the significant cost of carrying potentially redundant organs and the importance of pre-departure protein catabolism and synthesis in an effort to reduce wing load and improve flight efficiency.
Conclusion
In this study, we used a non-invasive sequential sampling method to track behavioural and physiological changes in light-bellied brent geese across the month of spring staging. Our results show phenotypic flexibility of this Arctic breeder, in response to the high energetic demands of long-distance migration and a capital breeding life history strategy. A decrease in foraging and locomotion rate in the last week of staging coincided with the attenuation of weight gain. During this last week, we also found fatter individuals had a lower C:N ratio and higher δ15N values in their excretion. All these factors support the prediction that it is beneficial for brent geese to undergo a reorganisation of internal protein, changing from a body prioritising resource acquisition for fat deposition, to one promoting efficient flight. It should be noted that this study was only based on data collected in one spring staging season meaning that it is impossible to make assumptions on the repeatability of the occurrence of the behavioural and stable isotope patterns observed in this study. However, we have no reason to suspect that it was an atypical season. Future directions to improve our understanding of phenotypic flexibility during avian migration should include the non-invasive measurement of gut size throughout the staging period (i.e., using ultrasonography techniques (Vézina et al., 2012). By combining such measurements with behavioural studies and stable isotope data a more complete picture of the phenotypic flexibility during migratory staging could be gained.
Data Availability Statement
The raw data supporting the conclusions of this article will be made available by the authors, without undue reservation.
Ethics Statement
The animal study was reviewed and approved by the CLES Penryn Ethics Committee.
Author Contributions
SB, TH, JS, and XH conceived the project. TH, JS, and SL carried out the fieldwork. TH, JS, and XH analysed the data. TH and JS lead the writing of the manuscript, with contributions from all authors. All authors contributed to the article and approved the submitted version.
Funding
SB was supported by an ERC consolidators grant (STATEMIG 310820).
Conflict of Interest
The authors declare that the research was conducted in the absence of any commercial or financial relationships that could be construed as a potential conflict of interest.
Publisher’s Note
All claims expressed in this article are solely those of the authors and do not necessarily represent those of their affiliated organizations, or those of the publisher, the editors and the reviewers. Any product that may be evaluated in this article, or claim that may be made by its manufacturer, is not guaranteed or endorsed by the publisher.
Acknowledgments
The authors thank Oli T and Rich Inger for their help in the field.
Supplementary Material
The Supplementary Material for this article can be found online at: https://www.frontiersin.org/articles/10.3389/fevo.2022.749534/full#supplementary-material
Footnotes
References
Ankney, C. D., and MacInnes, C. D. (1978). Nutrient reserves and reproductive performance of female Lesser Snow Geese. Auk 95, 459–471.
Barboza, P. S., Parker, K. L., and Hume, I. D. (2008). Integrative Wildlife Nutrition. Berlin: Springer.
Barton, N. W., and Houston, D. C. (1993). A comparison of digestive efficiency in birds of prey. Ibis 135, 363–371. doi: 10.1111/j.1474-919x.1993.tb02107.x
Bates, D., Mäechler, M., Bolker, B., and Walker, S. (2014). Fitting linear mixed –effects models using lme4. J. Stat. Softw. 67, 1–48. doi: 10.18637/jss.v067.i01
Battley, P. F., and Piersma, T. (1997). Body composition of Lesser Knots (Calidris canutus rogersi) preparing to take off on migration from northern New Zealand. Notornis 44, 137–150.
Battley, P. F., and Piersma, T. (2005). “Adaptive interplay between feeding ecology and features of the digestive tract in birds,” in Physiological and Ecological Adaptations to Feeding in Vertebrates, eds J. M. Starck and T. Wang (Enfield: Science Publishers), 201–228.
Battley, P. F., Piersma, T., Dietz, M. W., Tang, S., Dekinga, A., and Hulsman, K. (2000). Empirical evidence for differential organ reductions during trans-oceanic bird flight. Proc. R. Soc. Lond. B 267, 191–195. doi: 10.1098/rspb.2000.0986
Bauchinger, U., and Biebach, H. (2001). Differential catabolism of muscle protein in Garden Warblers (Sylvia borin): flight and leg muscle act as a protein source during long-distance migration. J. Comp. Physiol. B 171, 293–301. doi: 10.1007/s003600100176
Bauchinger, U., and Biebach, H. (2005). Phenotypic flexibility of skeletal muscles during long-distance migration of garden warblers: muscle changes are differentially related to body mass. Annu. N.Y. Acad. Sci. 1046, 271–281. doi: 10.1196/annals.1343.025
Bauchinger, U., and McWilliams, S. R. (2010). Extent of phenotypic flexibility during long-distance flight is determined by tissue-specific turnover rates: a new hypothesis. J. Avian Biol. 41, 603–608. doi: 10.1111/j.1600-048x.2010.05137.x
Bauchinger, U., Wohlmann, A., and Biebach, H. (2005). Flexible remodelling of organ size during spring migration of the garden warbler (Sylvia borin). Zoology 108, 97–106. doi: 10.1016/j.zool.2005.03.003
Bearhop, S., Teece, M. A., Waldron, S., and Furness, R. W. (2000). Influence of lipid and uric acid on δ13C and δ15N values of avian blood: implications for trophic studies. Auk 117, 504–507.
Bêty, J., Giroux, J. F., and Gauthier, G. (2004). Individual variation in timing of migration: causes and reproductive consequences in greater snow geese (Anser caerulescens atlanticus). Behav. Ecol. Sociobiol. 57, 1–8.
Biebach, H. (1998). Phenotypic organ flexibility in Garden Warblers Sylvia borin during long-distance migration. J. Avian Biol. 29, 529–535.
Bodey, T. W., Cleasby, I. R., Blount, J. D., McElwaine, G., Vigfusdottir, F., and Bearhop, S. (2020). Consistent measures of oxidative balance predict survival but not reproduction in a long-distance migrant. J. Anim. Ecol. 89, 1872–1882. doi: 10.1111/1365-2656.13237
Boyd, H. (2000). Abdominal profiles of barnacle geese Branta leucopsis at staging areas in Iceland in May. Wildfowl 51, 33–47.
Boyd, H., Fox, A. D., Kristiansen, J. N., Stroud, D. A., Walsh, A. J., and Warren, S. M. (1998). Changes in abdominal profiles of Greenland White-fronted Geese during spring staging in Iceland. Wildfowl 49, 57–71.
Buchsbaum, R., Wilson, J., and Valiela, I. (1986). Digestibility of plant constituents by Canada geese and Atlantic brant. Ecology 67, 386–393.
Burnham, K. P., and Anderson, D. R. (2004). Multimodel inference: understanding AIC and BIC in model selection. Sociol. Methods Res. 33, 261–304. doi: 10.1177/0049124104268644
Cherel, Y., Robin, J.-P., and Le Maho, Y. (1988). Physiology and biochemistry of long-term fasting in birds. Can. J. Zool. 66, 159–166. doi: 10.1016/j.envint.2020.105866
Clausen, P., Green, M., and Alerstam, T. (2003). Energy limitations for spring migration and breeding: the case of brent geese Branta bernicla tracked by satellite telemetry to Svalbard and Greenland. Oikos 103, 426–445.
Cleasby, I. R., Bodey, T. W., Vigfusdottir, F., McDonald, J. L., McElwaine, G., Mackie, K., et al. (2017). Climatic conditions produce contrasting influences on demographic traits in a long-distance Arctic migrant. J. Anim. Ecol. 86, 285–295. doi: 10.1111/1365-2656.12623
Dietz, M. W., Piersma, T., Hedenström, A., and Brugge, M. (2007). Intraspecific variation in avian pectoral muscle mass: constraints on maintaining manoeuvrability with increasing body mass. Funct. Ecol. 21, 317–326. doi: 10.1111/j.1365-2435.2006.01234.x
Dokter, A. M., Fokkema, W., Bekker, S. K., Bouten, W., Ebbinge, B. S., Müskens, G., et al. (2018). Body stores persist as fitness correlate in a long-distance migrant released from food constraints. Behav. Ecol. 29, 1157–1166. doi: 10.1093/beheco/ary080
Drent, R. H., and Daan, S. (1980). The prudent parent: energetic adjustments in avian breeding. Ardea 68, 225–252. doi: 10.5253/arde.v68.p225
Drent, R. H., Eichhorn, G., Flagstad, A., Van der Graaf, A. J., Litvin, K. E., and Stahl, J. (2007). Migratory connectivity in Arctic geese: spring stopovers are the weak links in meeting targets for breeding. J. Ornithol. 148, 501–514.
Ebbinge, B. S., and Spaans, B. (1995). The importance of body reserves accumulated in spring staging areas in the temperate zone for breeding in dark-bellied brent geese Branta b. bernicla in the high Arctic. J. Avian Biol. 26, 105–113. doi: 10.2307/3677058
Evans, P. R., Davidson, N. C., Uttley, J. D., and Evans, R. D. (1992). Premigratory hypertrophy of flight muscles: an ultra- structural study. Ornis Scand. 23, 238–243.
Fox, A. D., and Kahlert, J. (2005). Changes in body mass and organ size during wing moult in non-breeding greylag geese Anser anser. J. Avian Biol. 36, 538–548.
Fox, A. D., Kahlert, J., Walsh, A. J., Stroud, D. A., Mitchell, C., Kristiansen, J. N., et al. (1999). Patterns of body mass change during moult in three different goose populations. Wildfowl 50, 45–56.
Fuller, B. T., Fuller, J. L., Sage, N. E., Harris, D. A., O’Connell, T. C., and Hedges, R. E. (2005). Nitrogen balance and δ15N: why you’re not what you eat during nutritional stress. Rapid. Commun. Mass Spectrom. 19, 2497–2506. doi: 10.1002/rcm.2090
Gannes, L. Z. (2002). Mass change pattern of blackcaps refuelling during spring migration: evidence for physiological limitations to food assimilation. Condor 104, 231–239. doi: 10.1650/0010-5422(2002)104[0231:mcpobr]2.0.co;2
Gaunt, A. S., Hikida, R. S., Jehl, J. R. Jr., and Fenbert, L. (1990). Rapid atrophy and hypertrophy of an avian flight muscle. Auk 107, 649–659. doi: 10.2307/4087994
Gauthier, G., Bedard, J., Huot, J., and Bedard, Y. (1984). Protein reserves during spring staging in Greater Snow Geese. Condor 86, 210–212.
Grönroos, J., Green, M., and Alerstam, T. (2012). To fly or not to fly depending on winds: shorebird migration in different seasonal wind regimes. Anim. Behav. 83, 1449–1457.
Gudmundsson, G. A., Benvenuti, S., Alerstam, T., Papi, F., Lilliendahl, K., and Åkesson, S. (1995). Examining the limits of flight and orientation performance: satellite tracking of brent geese migrating across the Greenland ice-cap. Proc. R. Soc. Lond. B 261, 73–79. doi: 10.1098/rspb.1995.0119
Guglielmo, C. G., and Williams, T. D. (2003). Phenotypic flexibility of body composition in relation to migratory state, age, and sex in the western sandpiper (Calidris mauri). Physiol. Biochem. Zool. 76, 84–98. doi: 10.1086/367942
Harrison, X. A., Blount, J. D., Inger, R., Norris, D. R., and Bearhop, S. (2011). Carry-over effects as drivers of fitness differences in animals. J. Anim. Ecol. 80, 4–18. doi: 10.1111/j.1365-2656.2010.01740.x
Harrison, X. A., Hodgson, D. J., Inger, R., Colhoun, K., Gudmundsson, G. A., McElwaine, G., et al. (2013). Environmental conditions during breeding modify the strength of mass-dependent carry-over effects in a migratory bird. PLoS One 8:e77783. doi: 10.1371/journal.pone.0077783
Harrison, X. A., Tregenza, T., Inger, R., Colhoun, K., Dawson, D. A., Gudmundsson, G. A., et al. (2010). Cultural inheritance drives site fidelity and migratory connectivity in a long-distance migrant. Mol. Ecol. 19, 5484–5496. doi: 10.1111/j.1365-294X.2010.04852.x
Hatch, K. A., Pinshow, B., and Speakman, J. R. (2002). The analysis of 13C/12C ratios in exhaled CO2: its advantages and potential application to field research to infer diet, changes in diet over time, and substrate metabolism in birds. Integr. Comp. Biol. 42, 21–33. doi: 10.1093/icb/42.1.21
Hedenström, A. (2008). Adaptations to migration in birds: behavioural strategies, morphology and scaling effects. Philos. Trans. R. Soc. B 363, 287–299. doi: 10.1098/rstb.2007.2140
Hijmans, R. J., Williams, E., Vennes, C., and Hijmans, M. R. J. (2017). Package ‘geosphere’. Spherical Trigon. 1:7.
Hobson, K. A., and Clark, R. G. (1992). Assessing avian diets using stable isotopes I: turnover of 13C in tissues. Condor 94, 181–188. doi: 10.2307/1368807
Hobson, K. A., Alisauskas, R. T., and Clark, R. G. (1993). Stable-nitrogen isotope enrichment in avian tissues due to fasting and nutritional stress: implications for isotopic analyses of diet. Condor 95, 388–394.
Hou, L., and Welch, K. C. (2016). Premigratory ruby-throated hummingbirds, Archilochus colubris, exhibit multiple strategies for fuelling migration. Anim. Behav. 121, 87–99. doi: 10.1016/j.anbehav.2016.08.019
Hume, I. D., and Biebach, H. (1996). Digestive tract function in the long-distance migratory garden warbler, Sylvia borin. J. Comp Physiol. B 166, 388–395.
Hupp, J. W., Schmutz, J. A., and Ely, C. R. (2006). The prelaying interval of emperor geese on the Yukon-Kuskokwim Delta, Alaska. Condor 108, 912–924.
Inger, R., and Bearhop, S. (2008). Applications of stable isotope analyses to avian ecology. Ibis 150, 447–461. doi: 10.1111/j.1474-919x.2008.00839.x
Inger, R., Bearhop, S., Robinson, J. A., and Ruxton, G. (2006a). Prey choice affects the trade-off balance between predation and starvation in an avian herbivore. Anim. Behav. 71, 1335–1341.
Inger, R., Ruxton, G. D., Newton, J., Colhoun, K., Robinson, J. A., Jackson, A. L., et al. (2006b). Temporal and intrapopulation variation in prey choice of wintering geese determined by stable isotope analysis. J. Anim. Ecol. 75, 1190–1200. doi: 10.1111/j.1365-2656.2006.01142.x
Inger, R., Gudmundsson, G. A., Ruxton, G. D., Newton, J., Colhoun, K., Auhage, S., et al. (2008). Habitat utilisation during staging affects body condition in a long distance migrant, Branta bernicla hrota: potential impacts on fitness? J. Avian Biol. 39, 704–708.
Inger, R., Harrison, X. A., Ruxton, G. D., Newton, J., Colhoun, K., Gudmundsson, G. A., et al. (2010). Carry-over effects reveal reproductive costs in a long-distance migrant. J. Anim. Ecol. 79, 974–982. doi: 10.1111/j.1365-2656.2010.01712.x
Jaatinen, K., Öst, M., and Hobson, K. A. (2016). State-dependent capital and income breeding: a novel approach to evaluating individual strategies with stable isotopes. Front. Zool. 13:24. doi: 10.1186/s12983-016-0157-x
Jehl, J. R. Jr. (1997). Cyclical changes in body composition in the annual cycle and migration of the Eared Grebe Podiceps nigricollis. J. Avian Biol. 28, 132–142.
Jehl, J. R. Jr., Henry, A. E., and Swanson, D. L. (2015). Ratios, adaptations, and the differential metabolic capability of avian flight muscles. J. Avian Biol. 46, 119–124. doi: 10.1111/jav.00506
Jenni, L., and Jenni-Eiermann, S. (1998). Fuel supply and metabolic constraints in migrating birds. J. Avian Biol. 126, 521–528.
Jenni, L., Jenni-Eiermann, S., Spina, F., and Schwabl, H. (2000). Regulation of protein breakdown and adrenocortical response to stress in birds during migratory flight. Am. J. Physiol. Regul. Integr. Comp. Physiol. 278, R1182–R1189. doi: 10.1152/ajpregu.2000.278.5.R1182
Jónsson, J. E., Gardarsson, A., Gill, J. A., Petersen, A., and Gunnarsson, T. G. (2009). Seasonal weather effects on the common eider, a subarctic capital breeder, in Iceland over 55 years. Clim. Res. 38, 237–248.
Kalnay, E., Kanamitsu, M., Kistler, R., Collins, W., Deaven, D., Gandin, L., et al. (1996). The NCEP/NCAR 40-year reanalysis project. Bull. Am. Meteor. Soc. 77, 437–472.
Karasov, W. H., and McWilliams, S. R. (2005). “Digestive constraints in mammalian and avian ecology,” in Physiological and Ecological Adaptations to Feeding in Vertebrates, eds J. M. Starck and T. Wang (Enfield: Science Publishers), 87–112.
Karasov, W. H., and Pinshow, B. (1998). Changes in lean mass and in organs of nutrient assimilation in a long-distance passerine migrant at a springtime stopover site. Physiol. Zool. 71, 435–438. doi: 10.1086/515428
Karasov, W. H., and Pinshow, B. (2000). Test for physiological limitation to nutrient assimilation in a long-distance passerine migrant at a springtime stopover site. Physiol. Biochem. Zool. 73, 335–343. doi: 10.1086/316746
Karasov, W. H., Pinshow, B., Starck, J. M., and Afik, D. (2004). Anatomical and histological changes in the alimentary tract of migrating blackcaps (Sylvia atricapilla): a comparison among fed, fasted, food-restricted, and refed birds. Physiol. Biochem. Zool. 77, 149–160. doi: 10.1086/381465
Kemp, M. U., Emiel van Loon, E., Shamoun-Baranes, J., and Bouten, W. (2012). RNCEP: global weather and climate data at your fingertips. Methods Ecol. Evol. 3, 65–70. doi: 10.1111/j.2041-210x.2011.00138.x
Klaassen, M., and Biebach, H. (1994). Energetics of fattening and starvation in the long-distance migratory garden warbler, Sylvia borin, during the migratory phase. J. Comp Physiol. B 164, 362–371. doi: 10.1007/bf00302551
Klaassen, M., Lindström, Å, and Zijlstra, R. (1997). Composition of fuel stores and digestive limitations to fuel deposition rate in the long-distance migratory thrush nightingale, Luscinia luscinia. Physiol. Zool. 70, 125–133. doi: 10.1086/639556
Krausman, P. R., and Cain, J. W. (2013). Wildlife Management and Conservation: Contemporary Principles and Practices. Baltimore: JHU Press.
Landys-Ciannelli, M. M., Piersma, T., and Jukema, J. (2003). Strategic size changes of internal organs and muscle tissue in the Bar-tailed Godwit during fat storage on a spring stopover site. Funct. Ecol. 17, 151–159. doi: 10.1046/j.1365-2435.2003.00715.x
Lepage, D., Gauthier, G., and Menu, S. (2000). Reproductive consequences of egg-laying decisions in snow geese. J. Anim. Ecol. 69, 414–427. doi: 10.1046/j.1365-2656.2000.00404.x
Lindström, A., Kvist, A., Piersma, T., Dekinga, A., and Dietz, M. W. (2000). Avian pectoral muscle size rapidly tracks body mass changes during flight, fasting, and fuelling. J. Exp. Biol. 203, 913–919. doi: 10.1242/jeb.203.5.913
Lindström, A. ° (2003). “Fuel deposition rates in migrating birds: causes, constraints and consequences,” in Avian Migration, eds P. Berthold, E. Gwinner, and E. Sonnenschein (Berlin: Springer), 307–320. doi: 10.1007/978-3-662-05957-9_21
Lindström, A. °, and Piersma, T. (1993). Mass changes in migrating birds: the evidence for fat and protein storage re-examined. Ibis 135, 70–78. doi: 10.1111/j.1474-919x.1993.tb02811.x
Madsen, J., and Klaassen, M. (2006). Assessing body condition and energy budget components by scoring abdominal profiles in free-ranging pink-footed geese Anser brachyrhynchus. J Avian Biol. 37, 283–287.
Magnusson, A., Skaug, H., Nielsen, A., Berg, C., Kristensen, K., Maechler, M., et al. (2017). Package ‘glmmTMB’. R Package Version 0.2.0. Available online at: https://cran.r-project.org/web/packages/glmmTMB/glmmTMB.pdf
Marsh, R. L. (1984). Adaptations of the grey catbird Dumetella carolinensis to long-distance migration: flight muscle hypertrophy associated with elevated body mass. Physiol. Zool. 57, 105–117.
McLandress, R. M., and Raveling, D. G. (1981). Changes in diet and body composition of Canada geese before spring migration. Auk 98, 65–79.
McWilliams, S. R., Guglielmo, C., Pierce, B., and Klaassen, M. (2004). Flying, fasting and feeding in birds during migration: a nutritional and physiological ecology perspective. J. Avian Biol. 35, 377–393.
McWilliams, S. R., and Karasov, W. H. (2001). Phenotypic flexibility in digestive system structure and function in migratory birds and its ecological significance. Comp. Biochem. Physiol. A Mol. Integr. Physiol. 128, 577–591. doi: 10.1016/s1095-6433(00)00336-6
McWilliams, S. R., and Karasov, W. H. (2005). “Migration takes guts: digestive physiology of migratory birds and its ecological significance,” in Birds of Two Worlds, eds P. Marra and R. Greenberg (Washington, DC: Smithsonian Inst. Press), 67–78.
Mehlman, D. W., Mabey, S. E., Ewert, D. N., Duncan, C., Abel, B., Cimprich, D., et al. (2005). Conserving stopover sites for forest-dwelling migratory landbirds. Auk 122, 1281–1290. doi: 10.1642/0004-8038(2005)122[1281:cssffm]2.0.co;2
Owen, M. (1981). Abdominal profile: a condition index for wild geese in the field. J. Wildl. Manage. 45, 227–230. doi: 10.2307/3807891
Pierce, B. J., and McWilliams, S. R. (2004). Diet quality and food limitation affect the dynamics of body composition and digestive organs in a migratory songbird (Zonotrichia albicollis). Physiol. Biochem. Zool. 77, 471–483. doi: 10.1086/383503
Piersma, T. (1998). Phenotypic flexibility during migration: optimization of organ size contingent on the risks and rewards of fuelling and flight? J. Avian Biol. 29, 511–520. doi: 10.2307/3677170
Piersma, T., and Gill, R. E. (1998). Guts don’t fly: small digestive organs in obese bar-tailed godwit. Auk 115, 196–203. doi: 10.2307/4089124
Piersma, T., Gudmundsson, G. A., and Lilliendahl, K. (1999). Rapid changes in the size of different functional organ and muscle groups during refuelling in a long-distance migrating shorebird. Physiol. Biochem. Zool. 72, 405–415. doi: 10.1086/316680
Piersma, T., Koolhaas, A., and Dekinga, A. (1993). Interactions between stomach structure and diet choice in shorebirds. Auk 110, 552–564. doi: 10.2307/4088419
Piersma, T., and Lindström, A. ° (1997). Rapid reversible changes in organ size as a component of adaptive behaviour. Trends Ecol. Evolut. 12, 134–138. doi: 10.1016/s0169-5347(97)01003-3
Poussart, C., Larochelle, J., and Gauthier, G. (2000). The thermal regime of eggs during laying and incubation in greater snow geese. Condor 102, 292–300. doi: 10.1093/condor/102.2.292
R Core Team (2016). R: A Language and Environment for Statistical Computing (v. 3.3.1.). Vienna: R Foundation for Statistical Computing.
Ramenofsky, M., Savard, R., and Greenwood, M. R. C. (1999). Seasonal and diel transitions in physiology and behavior in the migratory dark-eyed junco. Comp. Biochem. Physiol. A Mol. Integr. Physiol. Comp. Biochem. Phys. A 122, 385–397. doi: 10.1016/s1095-6433(99)00013-6
Reed, A. (1993). Duration of family bonds of Brent Geese Branta bernicla on the Pacific coast of North America. Wildfowl 44, 33–38. doi: 10.1016/0006-3207(82)90052-0
Remisiewicz, M., Tree, A. J., Underhill, L. G., and Burman, M. S. (2016). Age-specific variation in relationship between moult and pre-migratory fuelling in Wood Sandpipers Tringa glareola in southern Africa. Ibis 159, 91–102. doi: 10.1111/ibi.12436
Robinson, J. A., Colhoun, K., Gudmundsson, G. A., Boertmann, D., Merne, O. J., O’Brian, M., et al. (2004). Light-bellied Brent Goose Branta bernicla hrota (East Canadian High Arctic population) in Canada, Ireland, Iceland, France, Greenland, Scotland, Wales, England, the Channel Islands and Spain: 1960/61 - 1999/2000. Waterbird Review Series. Slimbridge: The Wildfowl & Wetlands Trust/Joint Nature Conservation Committee.
Rozenfeld, S. B., and Sheremetyev, I. S. (2016). Arctic Geese (Anser) and Brants (Branta) of Eurasia: an analysis of factors that control population dynamics and geographical ranges. Biol. Bull. Rev. 6, 436–455. doi: 10.1134/s2079086416050078
Safi, K., Kranstauber, B., Weinzierl, R., Griffin, L., Rees, E. C., Cabot, D., et al. (2013). Flying with the wind: scale dependency of speed and direction measurements in modelling wind support in avian flight. Mov. Ecol. 1, 1–13. doi: 10.1186/2051-3933-1-4
Saino, N., Rubolini, D., Von Hardenberg, J., Ambrosini, R., Provenzale, A., Romano, M., et al. (2010). Spring migration decisions in relation to weather are predicted by wing morphology among trans-Mediterranean migratory birds. Funct. Ecol. 24, 658–669. doi: 10.1111/j.1365-2435.2009.01659.x
Schmutz, J., Hobson, K., and Morse, J. (2006). An isotopic assessment of protein from diet and endogenous stores: effects on egg production and incubation behaviour of geese. Ardea 94, 385–397.
Schwilch, R., Grattarola, A., Spina, F., and Jenni, L. (2002). Protein loss during long-distance migratory flight in passerine birds: adaptation and constraint. J. Exp. Biol. 205, 687–695.
Scott, G. R., Hawkes, L. A., Frappell, P. B., Butler, P. J., Bishop, C. M., and Milsom, W. K. (2015). How bar-headed geese fly over the Himalayas. Physiology 30, 107–115. doi: 10.1152/physiol.00050.2014
Sedinger, J. S. (1997). Adaptations to and consequences of an herbivorous diet in grouse and waterfowl. Condor 99, 314–326. doi: 10.2307/1369937
Sedinger, J. S., White, R. G., Mann, F. E., Burris, F. A., and Kedrowski, R. A. (1989). Apparent metabolizability of alfalfa components by yearling Pacific Black Brant. J. Wildl. Manag. 53, 726–734.
Soriano-Redondo, A., Gutiérrez, J. S., Hodgson, D., and Bearhop, S. (2020). Migrant birds and mammals live faster than residents. Nat. commun. 11, 1–8. doi: 10.1038/s41467-020-19256-0
Tinkler, E., Montgomery, W. I., and Elwood, R. W. (2007). Parent–offspring associations in wintering brent geese: parental investment or mutual assistance? J. Zool. 272, 398–405. doi: 10.1111/j.1469-7998.2007.00281.x
Vézina, F., Williams, T. D., Piersma, T., and Guy Morrison, R. I. (2012). Phenotypic compromises in a long-distance migrant during the transition from migration to reproduction in the High Arctic. Func. Ecol. 26, 500–512. doi: 10.1111/j.1365-2435.2011.01955.x
Keywords: migratory birds, spring staging, phenotypic flexibility, atrophy, behaviour
Citation: Handby T, Slezacek J, Lupi S, Colhoun K, Harrison XA and Bearhop S (2022) Changes in Behaviour and Proxies of Physiology Suggest Individual Variation in the Building of Migratory Phenotypes in Preparation for Long-Distance Flights. Front. Ecol. Evol. 10:749534. doi: 10.3389/fevo.2022.749534
Received: 29 July 2021; Accepted: 18 January 2022;
Published: 16 February 2022.
Edited by:
Matthew Jason Noakes, Nicolaus Copernicus University in Toruń, PolandReviewed by:
Jose A. Masero, University of Extremadura, SpainScott McWilliams, University of Rhode Island, United States
Tori Mezebish, University of Rhode Island, United States, in collaboration with reviewer SM
Copyright © 2022 Handby, Slezacek, Lupi, Colhoun, Harrison and Bearhop. This is an open-access article distributed under the terms of the Creative Commons Attribution License (CC BY). The use, distribution or reproduction in other forums is permitted, provided the original author(s) and the copyright owner(s) are credited and that the original publication in this journal is cited, in accordance with accepted academic practice. No use, distribution or reproduction is permitted which does not comply with these terms.
*Correspondence: Xavier A. Harrison, x.harrison@exeter.ac.uk; Stuart Bearhop, s.bearhop@exeter.ac.uk
†These authors have contributed equally to this work and share first authorship