- 1Vertebrates Zoology Research Group, University of Alicante, Alicante, Spain
- 2Terrestrial Vertebrates Group, Cavanilles Institute of Biodiversity and Evolutionary Biology, University of Valencia, Valencia, Spain
- 3Environment Science and Solutions SL, Valencia, Spain
- 4Bird Monitoring Unit, SEO/BirdLife, Madrid, Spain
Several studies have shown in different organisms how their movements can be fitted to different patterns to optimize search of food resources. According to abundance and availability of resources, different strategies will be optimal, such as Lévy and Brownian random search. We analyze the movement patterns of four species of migratory raptors with different degrees of ecological specialization in diet during the breeding and wintering periods to evaluate the differences according to species and season: the Egyptian Vulture, the Short-toed Snake Eagle, the Booted Eagle, and the Red Kite. From GPS locations, we obtained a set of segments and lengths that were analyzed to verify their fitting to the functions of Lévy and Brownian strategies. Egyptian Vulture’s trajectories fitted to both patterns during the breeding period, whereas during the wintering period most trajectories fitted a Brownian pattern. In the case of the Short-toed Eagle, fit was greater to a Lévy strategy throughout the year, while Booted Eagles and Red Kites exhibited a combination of search patterns. These differences could be accounted for different feeding strategies and environmental context over the annual cycle. In species with a specialized diet (i.e., Short-toed Eagle) the Lévy pattern would maximize the encounters with scarce and unpredictable resources, whereas for species with a broad trophic niche (i.e., Booted Eagle and Red Kite), movements could be adapted to exploit different resources according to their abundance. Scavengers like the Egyptian Vulture shift also between search strategies according to the distribution of carrion. Therefore, the analysis of food search patterns can be used as an indirect indicator to track changes in food availability across a broad range of environmental conditions. This is particularly important under the current context of global change which is largely expected to affect migratory species that spend their vital cycle in distant areas.
Introduction
Animals have different strategies to locate food resources, related to their movement capacity. This searching for food is an essential activity because it influences their survival and reproduction. Therefore, it is expected that search strategies should maximize the number of encounters with target resources, while minimizing movement costs (Zollner and Lima, 1999). Depending on environmental conditions, different strategies will be optimal. Recent studies have shown that birds travel farther distances in homogeneous environments as a result of distribution and abundance of food resources (Tucker et al., 2019). In these homogeneous environments animals are expected to use random search strategies (Mueller and Fagan, 2008; Sims et al., 2012).
In recent years, special attention has been drawn to two strategies of random movement: the Brownian pattern and the Lévy motion (e.g., Viswanathan et al., 2011; Sims et al., 2012; Reyna-Hurtado et al., 2018). The Brownian search would be the most appropriate in productive environments, with abundant and predictable resources (Viswanathan et al., 1996, 2011; Humphries et al., 2010), while if resources are scarce and unpredictably distributed, the most advantageous strategy would be a Lévy food search strategy (Viswanathan et al., 1996, 1999; Bartumeus et al., 2002, 2005; Humphries et al., 2010). The Brownian pattern consists of a set of random short movements whereas the Lévy search strategy is a fractal movement that combines an elevated number of small displacements connected by long distance steps, covering large distances (Viswanathan et al., 1996, 1999; Bartumeus et al., 2005). Although some studies suggest that Lévy movements would be less frequent than it was previously thought (Benhamou, 2007; Edwards et al., 2007; Pyke, 2015; Auger-Méthé et al., 2016), others provide evidence of this behavior in different animals, such as insects (Reynolds et al., 2007; Reynolds and Frye, 2007; Miramontes et al., 2014), primates and other mammals (Austin et al., 2004; Ramos-Fernández et al., 2004; Boyer et al., 2006; Focardi et al., 2009; Ramos et al., 2016; Reyna-Hurtado et al., 2018), predatory fish (Sims et al., 2008, 2012; Humphries et al., 2010), or birds (Viswanathan et al., 1996; Reynolds, 2012, 2014; López-López et al., 2013; Reynolds et al., 2015).
Due to the importance on how trophic niche determine food search strategies (e.g., Sims et al., 2012; Reyna-Hurtado et al., 2018), here we analyze the movement patterns of four migratory raptors with different degrees of ecological specialization in their diet: the Egyptian Vulture Neophron percnopterus (an scavenger), the Short-toed Snake Eagle Circaetus gallicus (with a specialized diet), the Booted Eagle Aquila pennata and the Red Kite Milvus milvus (both with a much varied diet that ranges from a generalist predator in the former and a facultative scavenger in the latter). All four are migratory species that use largely soaring flight for displacement, but with differences in their feeding behavior. Due to their migratory behavior, these species use areas in different geographical regions and are thus excellent indicators of environmental change. Egyptian Vultures, Booted Eagles, and Short-toed Snakes Eagles have their breeding areas in Western Europe and their wintering quarters in the Sahel (Cramp and Simmons, 1980), while the majority of Red Kite populations breed in Central Europe and move to the Iberian Peninsula to spend the winter there (Fabienne, 2009; De la Puente and De la Torre, 2015).
In the case of the Egyptian vulture, differences in the movement patterns between breeding and wintering periods were reported by López-López et al. (2013), alternating search strategies according to the environmental context. Following López-López et al. (2013) rationale, we will extend our analyses to four species and we predict that there will be differences among them depending on geographic region, period of year and trophic niche of each species. Therefore, we analyzed their movements to assess if they fit to Lévy or Brownian movement patterns using GPS tracking information. Specifically, our main objectives were: (1) to identify foraging movement patterns and test for differences among species according to trophic niche; and (2) to check if foraging strategies vary according to season and environmental context.
Materials and Methods
Data Collection
For this study, we used data corresponding to 39 individuals of four migratory raptor species tagged with GPS satellite transmitters. All birds were captured in different Spanish provinces, except for two Short-toed Eagles in Italy (Supplementary Table 1). Six Egyptian Vultures were captured between 2007 and 2009 (see García-Ripollés et al., 2010 for details), 14 Booted Eagles between 2011 and 2014 (see Mellone et al., 2013, 2015; Urios et al., 2017), seven Short-toed Eagles between 2008 and 2010 (see Pavón et al., 2010; Mellone et al., 2011), and 12 central European migratory Red Kites trapped in Spanish wintering grounds between 2013 and 2015. Egyptian Vultures and Booted Eagles were all adult individuals, whereas Red Kites were in general adults but three were first winter individuals (Supplementary Table 1). Short-toed Eagles were nestlings tagged in their nests when they were 55–60 days old. In all cases, GPS satellite transmitters were attached to the back of the animals using a tubular Teflon harness, which was tied with a cotton thread to ensure that the harness falls from the bird at the end of the tag’s life (Garcelon, 1985). Equipment’s weight was less than 3% of bird’s body mass, which is within the recommended limits (Kenward, 2001).
Egyptian Vultures’ and Short-toed Snake Eagles’ transmitters (45 g solar-powered GPS tags from Microwave Telemetry Inc.) were programmed to register locations every 2 h for both breeding and wintering period, Booted Eagles’ tags (22-g PTT-100 solar-powered Argos/GPS from Microwave Telemetry Inc., n = 9; 20–23-g SAKER GPS-GSM from Ecotone Telemetry, n = 4; 22-g Quantum 4000 Enhanced GPS data-logger from Telemetry Solutions, n = 1) to record locations every hour, and Red Kites’ tags every 1–2 h depending on the dates (22-g PTT-100 solar-powered Argos/GPS from Microwave Telemetry Inc., n = 3; 35-g GPS-GSM transmitter from North Star Science and Technology, n = 2) or only on an hourly basis (20–21-g SAKER GPS-GSM from Ecotone Telemetry, n = 7). Only locations recorded between 6:00 and 21:00 h (Greenwich Mean Time) were considered for this study, excluding nocturnal movements since all four species are diurnal. Relocations were filtered to exclude possible errors (i.e., 0–0 coordinates) as well as those corresponding to spring and autumn migrations. Thereby, only locations corresponding to the breeding/summering and wintering periods were selected. Following López-López et al. (2013), we defined a step as an interval in which any or both of the coordinates in two consecutive samples differed. The step length was calculated as the Euclidean distance between two consecutive positions, and due to the nominal accuracy of the GPS, distances below 25 m were eliminated. The last step of the day finishes at 21:00 h and the first step of the next day starts at 6:00 h. All steps used for the analysis were calculated with the same time lapse between fixes (1, 2, or 3 h depending on the data available for each season). With this time lapse, the splicing of the segments in the same direction is something exceptional during foraging, so each step can be considered independent of the others. Therefore, the distribution of move-step-length was obtained for each dataset (i.e., species-individual-year-period). In case of the juvenile Short-toed Snake Eagles, the period between the tagging and the onset of the first autumn migration was not taken into account for the analysis, since they were linked to the nest. Unlike Egyptian Vultures, Booted Eagles and Red Kites, which spent the summer months in Europe, Short-toed Snake Eagles did not breed and spent the spring-summer period in staging areas located in northern Africa (Mellone et al., 2011).
Model Fitting
We followed the methodology described in López-López et al. (2013) to fit the distribution of each dataset to Lévy and Brownian strategies. The Lévy flight was tested using the probability density function of a truncated Pareto distribution (TP), and the Brownian movement using the probability density function of a truncated exponential distribution (TEXP) and also a hyper-exponential distribution composed of two weighted exponential functions (a composite Brownian walk, CBW) (see Supplementary Material).
We used the Maximum Likelihood Estimation (MLE) to estimate the parameters of power-law distributions (White et al., 2008). We increased iteratively the values of xmin [xmax was defined as the maximum value observed in the move-step-lengths distribution dataset (Jansen et al., 2012)] taken from each dataset (species-individual-year-period) and we estimated the parameters of the equations of the three different models (Clauset et al., 2009). Therefore, we obtained as many sub-sets with different number of step lengths as different values of xmin, although we stopped the fitting algorithm when it reached the percentile 80% of the data to allow that a substantial amount of data was fitted to the tail of the candidate distribution (Humphries et al., 2010, 2012).
Maximum Likelihood Estimation equations used for fitting (Supplementary Material) were solved numerically maximizing the log-likelihood function of each model (Supplementary Figure 1). The corrected δ-Kolmogorov–Smirnov (K–S) test was used to test the goodness of fit (GOF) of the candidate distributions (Sokal and Rohlf, 1994), a method that tested the maximum distance between the observed and the estimated cumulative distribution function (equations used for these calculations are available in Supplementary Material).
For the selection of the best parameters for each model, we excluded combinations of parameters that did not fit in statistical terms using the K–S test (McGill, 2003; Edwards, 2008) and we selected the best fit among the different sub-sets of data within each dataset (species-individual-year-period) for each one of the candidate distributions (see López-López et al., 2013 for more details about the procedure).
Once the best fits for each tested model were obtained, in case there was more than one that gave significant results, we used model selection criteria to select the best model. We selected the two models that showed the best/lowest corrected GOF index and we obtained the Akaike weights (AICw; Burnham and Anderson, 2002; Johnson and Omland, 2004; see Supplementary Material) for each model and its competing model (calculated fitting the other model to the same sub-set of data). Then, we applied a truth-table (Humphries et al., 2012; Supplementary Figure 1) to evaluate the trade-off between goodness of fit and the number of steps used for fitting the model.
Statistical Analysis
We tested the differences between frequencies of foraging patterns within the two studied periods (breeding and wintering) for each species using a one-way contingency table with Yates’s chi-squared test. We also evaluated the differences in the frequencies of foraging patterns between breeding and wintering periods for each species using chi-squared tests in two-way contingency tables, and likewise we tested the differences among species within each period. The effect of individuals was controlled creating a null distribution of the test statistic (χ2sim) with Monte Carlo simulations (Gotelli and Ellison, 2004) permuting 10000 times the winning models between periods within each individual (Anderson and Ter Braak, 2003). P-value was calculated as the percentage of times that χ2obs was strictly greater than χ2sim (Gotelli and Ellison, 2004). Mann–Whitney test (data failed test for normality) was used to compare the descriptive variables between periods according to the species. The model fitting and selection were performed with MATLAB version 7.7.0 and the statistical analyses with IBM SPSS Statistics version 22.0.
Results
The number of tracking seasons and locations for each individual is detailed in the Supplementary Table 2. The number of tracking season oscillated between 5 and 13 seasons for the Egyptian Vulture individuals, between 3 and 10 seasons for Booted Eagles, between 1 and 5 for Short-toed Snake Eagles, and between 1 and 7 for Red Kites. Overall, we recorded 44310 locations for Egyptian Vultures (average = 852 ± 548 locations/season), 44938 for Booted Eagles (average = 881 ± 567 locations/season), 20011 for Short-toed Snake Eagles (average = 1177 ± 585 locations/season), and 22952 (average = 740 ± 769 locations/season) for Red Kites.
We obtained a total of 74489 valid steps (Table 1). The number of steps per season differed significantly between periods for the Egyptian Vulture (Mann–Whitney test: Z = −5.580, p < 0.001) and Booted Eagle (Z = −2.115, p = 0.034), but not for the Short-toed Snake Eagle (Z = −1.608, p = 0.108) and the Red Kite (Z = −1.305, p = 0.192).
The frequency of foraging search patterns varied among species: for the Egyptian Vulture, 14.3% of trajectories fitted a Lévy pattern, 46.4% fitted a Brownian pattern and 39.3% did not fit any pattern during the breeding period (n = 28), whereas during the wintering period (n = 24) most trajectories fitted a Brownian pattern (91.7%) (Figure 1). These differences between both periods were significant (p = 0.002), in the same way that within the wintering period (p < 0.0001), but not within the breeding period (p = 0.096). In the case of Booted Eagles, both during the breeding (n = 30) and wintering (n = 21) periods there were a combination of patterns (Figure 1), without significant differences between them (p = 0.402) or within them (p = 0.365 and p = 0.961, respectively). In the case of Short-toed Snake Eagles, both during the summering (n = 6) and wintering (n = 11) periods the fit was much larger to a Lévy strategy: 66.7 and 63.6% of trajectories, respectively (Figure 1), without significant differences between both periods (p = 0.288); the differences were consistent within the wintering period (p = 0.041) but not for the summering (p = 0.378). For the Red Kite, the results were similar to those found for the Booted Eagle, with a combination of patterns for both periods [without significant differences between them (p = 0.245)]. There was larger fit to Lévy strategy [43.8% during the breeding (n = 16) and 66.7% during the wintering period (n = 15)] but also some fit to Brownian pattern (Figure 1). In this latter case, differences were significant within the wintering period (p = 0.007), but not during the breeding period (p = 0.541). Overall, considering the four species, significant differences for the search strategies were found among them for the wintering period (p < 0.0001), but not for the summer period (p = 0.063), when a combination of patterns was observed.
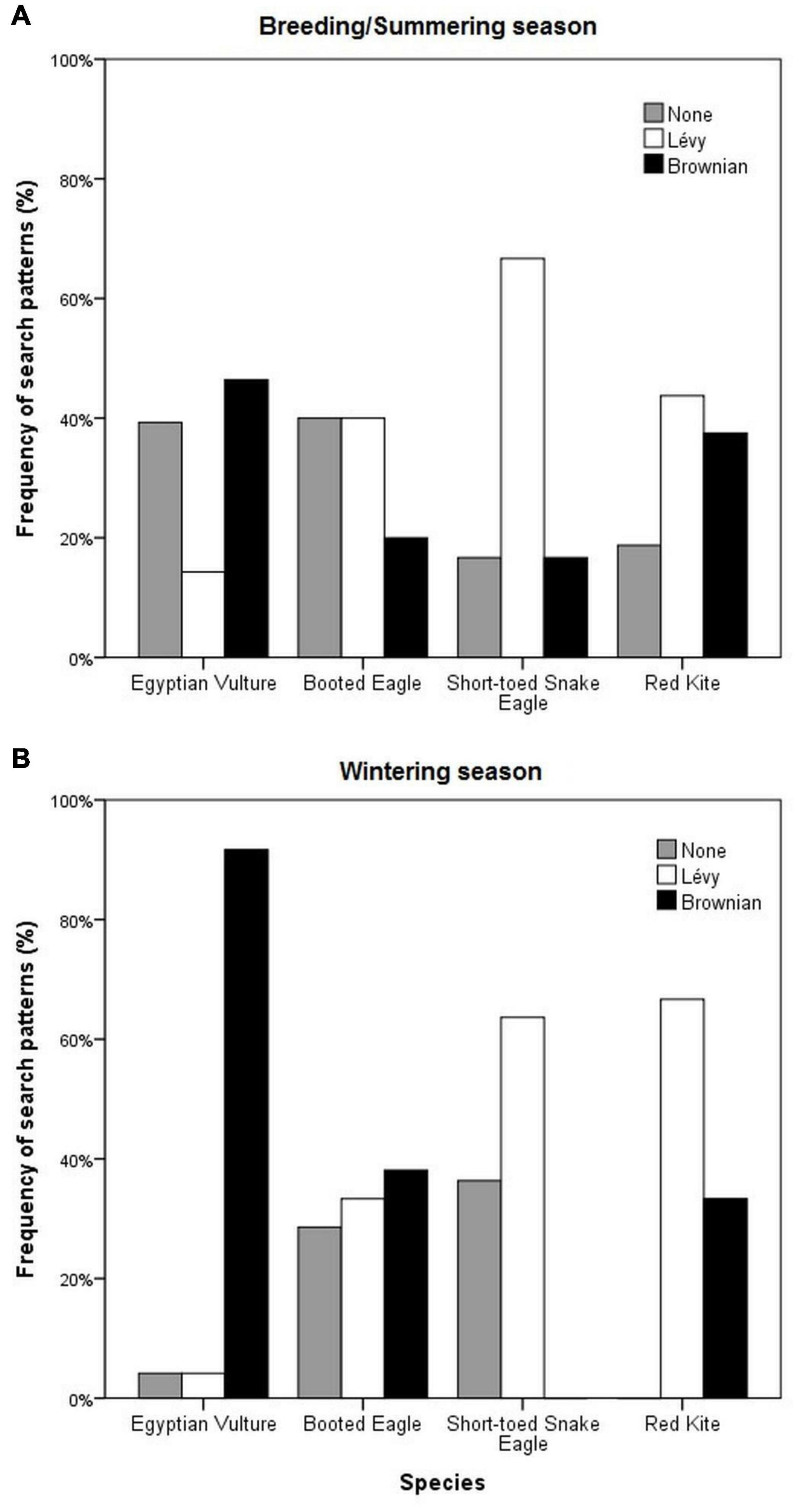
Figure 1. Frequency of Lévy and Brownian foraging search patterns of four raptor species (Egyptian Vulture, Booted Eagle, Short-toed Snake Eagle, and Red Kite) tracked by GPS satellite telemetry during the breeding/summering (A) and wintering season (B). Egyptian Vultures, Booted Eagles, and Short-toed Snakes Eagles had their wintering quarters in the African Sahel and the breeding/summering areas in the Iberian Peninsula (except the juvenile Short-toed Snake Eagles that spent the summer in northern Africa), whereas Red Kites spent the wintering season in the Iberian Peninsula and the breeding/summering season in Central Europe.
Discussion
The distribution and abundance of food resources can determine the different foraging search strategies (Humphries et al., 2010; Sims et al., 2012). Lévy’s flights would optimize the search for scarce and unpredictable resources, with the combination of short displacements and long distance relocation movements, and the Brownian pattern of more abundant and regularly distributed resources (Viswanathan et al., 1996; Shlesinger, 2009; Humphries et al., 2010; Reyna-Hurtado et al., 2018). So, considering these resources, the different diet could also influence the search patterns.
Our results showed that movement patterns vary in relation to the species and period considered (Figure 2), since there is a change in the environmental conditions. Our results for the Egyptian Vulture confirm those obtained by López-López et al. (2013), but now with a larger sample. Egyptian vulture’s movement patterns are influenced by its scavenging behavior, which is fundamentally based on carrion, although occasionally it can feed on small prey (Ferguson-Lees and Christie, 2001) and also by the different environmental context between its breeding and wintering areas (Anadón et al., 2010). Human dominated landscapes with the occurrence of predictable sources of food (i.e., vulture restaurants, legal and illegal dumping sites associated to intensive farming) prevail in the Iberian Peninsula. This could explain the combination of patterns observed during the breeding season, while in the Sahel, with a lower degree of human alteration of landscapes, the availability of food due to extensive livestock farming (Zwarts et al., 2009) eases finding food resources during wintering, hence being the Brownian pattern the preferred option (López-López et al., 2013).
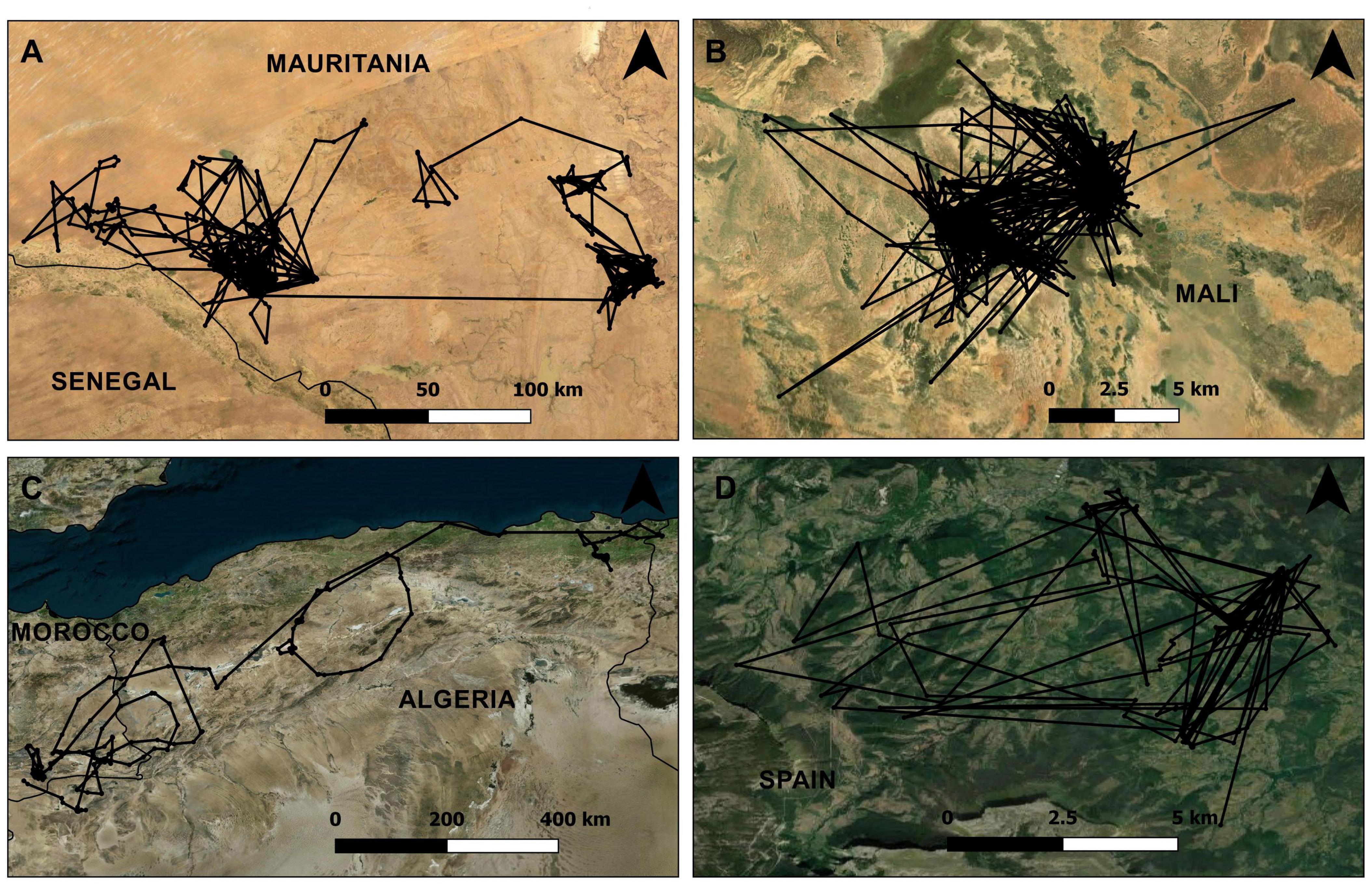
Figure 2. Lévy and Brownian trajectories. Examples of four tracks of raptors tracked by GPS satellite telemetry that fitted to the Brownian [(A) Egyptian Vulture; (B) Booted Eagle] and Lévy pattern [(C) Short-toed Snake Eagle and (D) Red Kite].
In case of the Short-toed Eagle, we found a very different behavior that can be explained by its diet independently of the environmental context: both during summer, when the immature individuals range across northern Africa (Mellone et al., 2011), and in the wintering period in the Sahel, there was a predominant fit to the Lévy motion. This species has a more specialized diet, mostly based on snakes (Del Hoyo et al., 1994; Gil and Pleguezuelos, 2001), so facing such unpredictable resources in both environments (northern Africa and the Sahel), the Lévy pattern would maximize the encounters with them. It is necessary to take into account that the studied individuals were juvenile/immature individuals. Therefore, the Lévy search seems an optimal foraging strategy due to their lack of experience, which allows them to cover larger geographic areas in search for food with long distance steps in combination with short displacements to explore the terrain. Notwithstanding, some individuals (#80421, #80424) followed a Lévy strategy also during consecutive seasons in both periods (Supplementary Table 3) when they were in their 2nd–3rd calendar year, occupying the same area as the previous year, so inexperience would not be the only factor that would explain the preference for this pattern.
With regards to the Booted Eagle and the Red Kite, the two generalist species, results were different. Booted Eagles tracked here breed in the Iberian Peninsula and winter in the Sahel region (López-López et al., 2016; Vidal-Mateo and Urios, 2017). Interestingly, Booted eagles exhibit a combination of movement patterns in both periods. This species feeds mainly on small and medium-sized birds, but also on small mammals, and occasionally even insects (Cramp and Simmons, 1980; García-Dios, 2017), so that it could adapt its movements to exploit different food resources according to their abundance. Furthermore, many individuals shifted from one pattern to another in successive periods depending on environmental context (i.e., Iberian Peninsula or Sahel). Our results showed high individual variation, with some individuals following the same strategy during consecutive seasons within the same period (e.g., #917224211 followed the Lévy motion during the breeding period), while others shifted between the Brownian and Lévy pattern (e.g., #68328 or #118180; Supplementary Table 3). This variability has been observed in other animals, e.g., with marine birds (Humphries et al., 2012). The cognitive abilities and the capacity to recognize the suitable places used previously for feeding can be determining in this variation of the individual response (Hills, 2006; Gautestad, 2011; Lundy et al., 2012). Similar results were found for the Red Kite, which exhibited a combination of search patterns during breeding and wintering periods, although in different environments than the other studied species. In this case, Red Kites tracked here breed in Central Europe (Switzerland, Germany, and Poland) and winter in the Iberian Peninsula. This species is a facultative scavenger with a generalist diet, which feeds on small mammals, birds, reptiles or amphibians, and even carrion in dumps (Cramp and Simmons, 1980). Therefore, this ability to take advantage of different resources according to their availability (Blanco et al., 1990) could explain the observed variation in foraging search patterns. Unlike specialist predators such as the Short-toed Snake, Booted eagles and Red Kites have a generalist diet and this would allow them to occupy different habitats to find their food resources.
Animals can change their search strategies according to the abundance and distribution of resources (Humphries et al., 2010). Overall, our study provides empirical support for the predominance of Lévy walk foraging strategy in dietary specialists. However, the Brownian pattern occurs in combination with a Lévy foraging strategy in more generalist species, which exhibit a different functional response (typically a Type II or Type III sensu Holling, 1959) in relation to food density (Viswanathan et al., 1999) and with a preference for preys, that can change according to their abundance (Chesson, 1984). Other studies have reported this influence of feeding and food availability, such as Reyna-Hurtado et al. (2018), with primates according if they were frugivores or folivores. In this regard, individual variation can play an important role, with memory and cognitive abilities influencing resource search strategies, so not only environmental conditions and prey density would explain the shift from a Lévy to a Brownian search pattern (Hills, 2006; Wolf et al., 2009; Fronhofer et al., 2013). Indeed, these four species show a high level of wintering site fidelity with a good knowledge of the areas they occupy (Nachtigall, 2008; García-Ripollés et al., 2010; Pavón et al., 2010; López-López et al., 2014; Vidal-Mateo and Urios, 2017), and however, some individuals shifted the search pattern in successive years of the same annual period and others repeated the pattern (Supplementary Table 3). There were also some periods that did not fit to any of the studied patterns; these periods spanned months and therefore, although we filtered the data to exclude the migratory movements and the locations corresponding to resting times, other mixed or more complex behaviors may be included in the displacements and not only the search for resources (Edwards et al., 2007). In addition, animals can use more complicated strategies that do not have to be adjusted to the used models, which do not include all the factors that can affect the movement (Auger-Méthé et al., 2016).
In a scenario of global change, animals are forced to adapt their movements to the new conditions of the environment. As well as changes in movement patterns can be observed over the annual cycle depending on the environmental context, it is expected to observe these changes in movements according to possible alterations in the environment that affect the distribution of resources. Consequently, changes in the abundance and availability of resources could be reflected in movement patterns. In species with movements that fit predominantly to a Lévy pattern, the importance of preserving specific areas all over their range where they can find their food resources is essential. While, for those species that follow a Brownian search strategy, this measure would not be as effective. Finally, the analysis of food search patterns can be used as an indirect indicator to track changes in food availability across a broad range of environmental conditions all over the annual cycle. This is particularly important under the current context of global change which is largely expected to affect migratory species that spend their vital cycle in distant areas sometimes subject of different conservation scenarios.
Data Availability Statement
Publicly available datasets were analyzed in this study. This data can be found here: Movebank repository: (EB Terra Natura UA Spain and Migra Program projects): https://www.movebank.org.
Ethics Statement
Ethical review and approval was not required for the animal study because permissions for the capture and tagging of the birds were provided by the different regional and local governments. All applicable international, national, and institutional guidelines for the care and use of animals were followed.
Author Contributions
JV-M, JB-C, PL-L, and VU conceived the study. PL-L, CG-R, UM, JD, AB, and VU collected the data. JV-M and JB-C analyzed the data. JV-M and VU wrote the first draft of the manuscript. All authors edited the manuscript.
Funding
Tracking of Booted Eagles and Red Kites has been made within the “Migra” program (www.migraciondeaves.org/en/) developed by SEO/BirdLife and financed by Fundación Iberdrola España. The Basque Government and the Biodiversity Foundation financed the tagging of several Red Kites in Spain. Tracking of Italian individuals was funded by Gallipoli Cognato Piccole Dolomiti Lucane Regional Park (Italy). Servicio de Vida Silvestre (Generalitat Valenciana) and different local governments funded part of the tagging. The funders were not involved in the study design, collection, analysis, interpretation of data, the writing of this article or the decision to submit it for publication. JV-M was supported by a FPU grant of Spanish Ministry of Education (reference FPU014/04671).
Conflict of Interest
CG-R was employed by the company Environment Science and Solutions SL.
The remaining authors declare that the research was conducted in the absence of any commercial or financial relationships that could be construed as a potential conflict of interest.
The authors declare that this study received funding from Fundación Iberdrola España. The funder was not involved in the study design, collection, analysis, interpretation of data, the writing of this article or the decision to submit it for publication.
Publisher’s Note
All claims expressed in this article are solely those of the authors and do not necessarily represent those of their affiliated organizations, or those of the publisher, the editors and the reviewers. Any product that may be evaluated in this article, or claim that may be made by its manufacturer, is not guaranteed or endorsed by the publisher.
Acknowledgments
We are thankful to all volunteers that helped during all the fieldwork. This article is part of the Ph.D. thesis of JV-M at the University of Alicante.
Supplementary Material
The Supplementary Material for this article can be found online at: https://www.frontiersin.org/articles/10.3389/fevo.2022.666238/full#supplementary-material
References
Anadón, J. D., Sánchez-Zapata, J. A., Carrete, M., Donázar, J. A., and Hiraldo, F. (2010). Large scale human effects on an arid African raptor community. Anim. Conserv. 13, 495–504. doi: 10.1111/j.1469-1795.2010.00369.x
Anderson, M. J., and Ter Braak, C. J. F. (2003). Permutation tests for multi-factorial analysis of variance. J. Stat. Comput. Simulat. 73, 85–113. doi: 10.1080/00949650215733
Auger-Méthé, M., Derocher, A. E., DeMars, C. A., Plank, M. J., Codling, E. A., and Lewis, M. A. (2016). Evaluating random search strategies in three mammals from distinct feeding guilds. J. Anim. Ecol. 85, 1411–1421. doi: 10.1111/1365-2656.12562
Austin, D., Bowen, W. D., and McMillan, J. I. (2004). Intraspecific variation in movement patterns: modeling individual behaviour in a large marine predator. Oikos 105, 15–30. doi: 10.1111/j.0030-1299.1999.12730.x
Bartumeus, F., Catalan, J., Fulco, U. L., Lyra, M. L., and Viswanathan, G. M. (2002). Optimizing the encounter rate in biological interactions: lévy versus Brownian strategies. Phys. Rev. Lett. 88:097901. doi: 10.1103/PhysRevLett.88.097901
Bartumeus, F., Da Luz, M. G. E., Viswanathan, G. M., and Catalan, J. (2005). Animal search strategies: a quantitative random-walk analysis. Ecology 86, 3078–3087. doi: 10.1890/04-1806
Benhamou, S. (2007). How many animals really do the Lévy walk? Ecology 88, 1962–1969. doi: 10.1890/06-1769.1
Blanco, J. C., Hiraldo, F., and Heredia, B. (1990). Variations in the diet and foraging behaviour of a wintering red kite (Milvus milvus) population in response to changes in food availability. Ardeola 37, 267–278.
Boyer, D., Ramos-Fernandez, G., Miramontes, O., Mateos, J. L., Cocho, G., Sarralde, H., et al. (2006). Scale-free foraging by primates emerges from their interaction with a complex environment. Proc. R. Soc. B 273, 1743–1750. doi: 10.1098/rspb.2005.3462
Burnham, K. P., and Anderson, D. R. (2002). “Monte carlo insights and extended examples,” in Model Selection and Multimodel Inference, eds K. P. Burnham and D. R. Anderson. (New York, NY: Springer). doi: 10.1007/978-0-387-22456-5_5
Chesson, P. L. (1984). Variable predators and switching behavior. Theor. Populat. Biol. 26, 1–26. doi: 10.1016/0040-5809(84)90021-2
Clauset, A., Shalizi, C. R., and Newman, M. E. J. (2009). Power-law distributions in empirical data. SIAM Rev. 51, 661–703. doi: 10.1137/070710111
Cramp, S., and Simmons, K. E. L. (1980). The Birds of the Western Paleartic, Vol. 2. Oxford: Oxford University Press.
De la Puente, J., and De la Torre, V. (2015). Abstracts book of the II International Symposium on the Red Kite. Binaced (Huesca, Spain) 2015. Madrid: Grupo Ornitológico SEO-Monticola & Fondo de Amigos del Buitre.
Del Hoyo, J., Elliott, A., and Sargatal, J. (1994). Handbook of the Birds of the World, vol. 2: New World Vultures to Guineafowl. Barcelona: Lynx Edicions.
Edwards, A. M. (2008). Using likelihood to test for Lévy flight search patterns and for general power-law distributions in nature. J. Anim. Ecol. 77, 1212–1222. doi: 10.1111/j.1365-2656.2008.01428.x
Edwards, A. M., Phillips, R. A., Watkins, N. W., Freeman, M. P., Murphy, E. J., Afanasyev, V., et al. (2007). Revisiting Levy flight search patterns of wandering albatrosses, bumblebees and deer. Nature 449, 1044–1048. doi: 10.1038/nature06199
Fabienne, D. (2009). “Wintering ecology of the Red Kite in a population from northern Spain,” in Proceedings of the Red Kite international Symposium, (France: LPO).
Ferguson-Lees, J., and Christie, D. A. (2001). Raptors: Birds of Prey of the World. London: A & C Black Publications, Ltd, 1084.
Focardi, S., Montanaro, P., and Pecchioli, E. (2009). Adaptive lévy walks in foraging fallow deer. PLoS One 4:e6587. doi: 10.1371/journal.pone.0006587
Fronhofer, E. A., Hovestadt, T., and Poethke, H. J. (2013). From random walks to informed movement. Oikos 122, 857–866. doi: 10.1111/j.1600-0706.2012.21021.x
Garcelon, D. K. (1985). Mounting Backpack Telemetry Packages on Bald Eagles. Arcata, CA: Institute for Wildlife Studies.
García-Ripollés, C., López-López, P., and Urios, V. (2010). First description of migration and wintering of adult Egyptian vultures Neophron percnopterus tracked by GPS satellite telemetry. Bird Study 57, 261–265. doi: 10.1080/00063650903505762
Gautestad, A. O. (2011). Memory matters: influence from a cognitive map on animal space use. J. Theor. Biol. 287, 26–36. doi: 10.1016/j.jtbi.2011.07.010
Gil, J. M., and Pleguezuelos, M. (2001). Prey and prey-size selection by the short-toed eagle (Circaetus gallicus) during the breeding season in Granada (south easternSpain). J. Zool. 255, 131–137. doi: 10.1017/S0952836901001182
Gotelli, N. J., and Ellison, A. M. (2004). A Primer of Ecological Statistics. Sunderland, MA: Sinauer Associates, Inc, 492.
Hills, T. T. (2006). Animal foraging and the evolution of goal-directed cognition. Cognit. Sci. 30, 3–41. doi: 10.1207/s15516709cog0000_50
Holling, C. S. (1959). Some characteristics of simple types of predation and parasitism. Can. Entomol. 91, 385–398. doi: 10.4039/Ent91385-7
Humphries, N. E., Queiroz, N., Dyer, J. R. M., Pade, N. G., Musyl, M. K., Schaefer, K. M., et al. (2010). Environmental context explains Lévy and Brownian movement patterns of marine predators. Nature 465, 1066–1069. doi: 10.1038/nature09116
Humphries, N. E., Weimerskirch, H., Queiroz, N., Southall, E. J., and Sims, D. W. (2012). Foraging success of biological Lévy flights recorded in situ. Proc. Natl. Acad. Sci. U. S. A. 10, 7169–7174. doi: 10.1073/pnas.1121201109
Jansen, V. A. A., Mashanova, A., and Petrovskii, S. (2012). Comment on “Lévy walks evolve through interaction between movement and environmental complexity. Science 335:918. doi: 10.1126/science.1215747
Johnson, J. B., and Omland, K. S. (2004). Model selection in ecology and evolution. Trends Ecol. Evol. 19. doi: 10.1016/j.tree.2003.10.013
López-López, P., Benavent-Corai, J., García-Ripollés, C., and Urios, V. (2013). Scavengers on the move: behavioural changes in foraging search patterns during the annual cycle. PLoS One 8:e54352. doi: 10.1371/journal.pone.0054352
López-López, P., De la Puente, J., Mellone, U., Bermejo, A., and Urios, V. (2016). Spatial ecology and habitat use of adult Booted eagles (Aquila pennata) during the breeding season: implications for conservation. J. Ornithol. 157, 981–993. doi: 10.1007/s10336-016-1357-z
López-López, P., García-Ripollés, C., and Urios, V. (2014). Individual repeatability in timing and spatial flexibility of migration routes of trans-Saharan migratory raptors. Curr. Zool. 60, 642–652. doi: 10.1093/czoolo/60.5.642
Lundy, M. G., Harrison, A., Buckley, D. J., Boston, E. S., Scott, D. D., Teeling, E. C., et al. (2012). Prey field switching based on preferential behaviour can induce Lévy flights. J. R. Soc. Interface 10:20120489. doi: 10.1098/rsif.2012.0489
McGill, B. (2003). Strong and weak tests of macroecological theory. Oikos 102, 679–685. doi: 10.1034/j.1600-0706.2003.12617.x
Mellone, U., De la Puente, J., López-López, P., Limiñana, R., Bermejo, A., and Urios, V. (2013). Migration routes and wintering areas of booted eagles Aquila pennata breeding in Spain. Bird Study 60, 409–413. doi: 10.1080/00063657.2013.781113
Mellone, U., De la Puente, J., López-López, P., Limiñana, R., Bermejo, A., and Urios, V. (2015). Seasonal differences in migration patterns of a soaring bird in relation to environmental conditions: a multi-scale approach. Behav. Ecol. Sociobiol. 69, 75–82. doi: 10.1007/s00265-014-1818-4
Mellone, U., Yáñez, B., Limiñana, R., Muñoz, R., Pavón, D., González, J. M., et al. (2011). Summer staging areas of non-breeding Short-toed Snake Eagles Circaetus gallicus. Bird Study 58, 516–521. doi: 10.1080/00063657.2011.598914
Miramontes, O., DeSouza, O., Paiva, L. R., Marins, A., and Orozco, S. (2014). Lévy flights and self-similar exploratory behaviour of termite workers: beyond model fitting. PLoS One 9:e111183. doi: 10.1371/journal.pone.0111183
Mueller, T., and Fagan, W. F. (2008). Search and navigation in dynamic environments – from individual behaviors to population distributions. Oikos 117, 654–664. doi: 10.1111/j.0030-1299.2008.16291.x
Nachtigall, W. (2008). Der Rotmilan (Milvus milvus, L. 1758) in Sachsen und Sudbrandenburg – Untersuchungen zu Verbreitung und Okologie. Doctoral.Dissertation. Halle: Univ. Halle-Wittenberg.
Pavón, D., Limiñana, R., Urios, V., Izquierdo, A., Yáñez, B., Ferrer, M., et al. (2010). Autumn migration of juvenile short-toed eagles Circaetus gallicus from southeastern Spain. Ardea 98, 113–117. doi: 10.5253/078.098.0114
Pyke, G. H. (2015). Understanding movements of organisms: it’s time to abandon the Levy foraging hypothesis. Methods Ecol. Evolut. 6, 1–16. doi: 10.1111/2041-210X.12298
Ramos, A., Petit, O., Longour, P., Pasquaretta, C., and Sueur, C. (2016). Space use and movement patterns in a semi-free-ranging herd of european bison (Bison bonasus). PLoS One 11:e0147404. doi: 10.1371/journal.pone.0147404
Ramos-Fernández, G., Mateos, J. L., Miramontes, O., Cocho, G., Larralde, H., and Ayala-Orozco, B. (2004). Levy walk patterns in the foraging movements of spider monkeys (Ateles geoffroyi). Behav. Ecol. Sociobiol. 55, 223–230. doi: 10.1007/s00265-003-0700-6
Reyna-Hurtado, R., Teichroeb, J. A., Bonnell, T. R., Hernández-Sarabia, R. U., Vickers, S. M., Serio-Silva, J. C., et al. (2018). Primates adjust movement strategies due to changing food availability. Behav. Ecol. 29, 368–376. doi: 10.1093/beheco/arx176
Reynolds, A. M. (2012). Olfactory search behaviour in the wandering albatross is predicted to give rise to Lévy flight movement patterns. Anim. Behav. 83, 1225–1229. doi: 10.1016/j.anbehav.2012.02.014
Reynolds, A. M. (2014). Lévy flight movement patterns in marine predators may derive from turbulence cues. Proc. R. Soc. A 470:20140408. doi: 10.1098/rspa.2014.0408
Reynolds, A. M., Cecere, J. G., Paiva, V. H., Ramos, J. A., and Focardi, S. (2015). Pelagic seabird flight patterns are consistent with a reliance on olfactory maps for oceanic navigation. Proc. R. Soc. B 282:20150468. doi: 10.1098/rspb.2015.0468
Reynolds, A. M., and Frye, M. A. (2007). Free-flight odor tracking in drosophila is consistent with an optimal intermittent scale-free search. PLoS One 2:e354. doi: 10.1371/journal.pone.0000354
Reynolds, A. M., Smith, A. D., Menzel, R., Greggers, U., Reynolds, D. R., and Riley, J. R. (2007). Displaced honey bees perform optimal scale-free search flights. Ecology 88, 1955–1961. doi: 10.1890/06-1916.1
Shlesinger, M. F. (2009). Random searching. J. Phys. A 42:434001. doi: 10.1088/1751-8113/42/43/434001
Sims, D. W., Humphries, N. E., Bradford, R. W., and Bruce, B. D. (2012). Levy flight and Brownian search patterns of a free-ranging predator reflect different prey field characteristics. J. Anim. Ecol. 81, 432–442. doi: 10.1111/j.1365-2656.2011.01914.x
Sims, D. W., Southall, E. J., Humphries, N. E., Hays, G. C., Bradshaw, C. J. A., Pitchford, J. W., et al. (2008). Scaling laws of marine predator search behaviour. Nature 451, 1098–1102. doi: 10.1038/nature06518
Tucker, M. A., Alexandrou, O., Bierregaard, Jr. R.O, Bildstein, K. L., Böhning-Gaese, K., Bracis, C., et al. (2019). Large birds travel farther in homogeneous environments. Glob. Ecol. Biogeogr. 28, 576–587. doi: 10.1111/geb.12875
Urios, V., Bermejo, A., Vidal-Mateo, J., and De la Puente, J. (2017). Migración y ecología espacial de la población española de águila calzada. Monografía n.° 2 del programa Migra. Madrid: SEO/BirdLife. doi: 10.31170/0055
Vidal-Mateo, J., and Urios, V. (2017). “Ecología Espacial en el Periodo de Invernada,” in Migración y ecología de la población española de águila calzada. Monografía n.°2 del programa Migra, eds V. Urios, A. Bermejo, J. Vidal-Mateo, and J. De la Puente (Madrid: SEO/BirdLife), 63–70.
Viswanathan, G. M., Afanasyev, V., Buldyrev, S. V., Murphy, E. J., Prince, P. A., and Stanley, H. E. (1996). Levy flight search patterns of wandering albatrosses. Nature 381, 413–415. doi: 10.1038/381413a0
Viswanathan, G. M., Buldyrev, S. V., Havlin, S., da Luz, M. G., Raposo, E. P., and Stanley, H. E. (1999). Optimizing the success of random searches. Nature 401, 911–914. doi: 10.1038/44831
Viswanathan, G. M., Da Luz, M. G. E., Raposo, E. P., and Stanley, H. E. (2011). The Physics of Foraging: an Introduction to Biological Encounters and Random Searches. Cambridge: Cambridge University Press. doi: 10.1017/CBO9780511902680
White, E. P., Enquist, B. J., and Green, J. L. (2008). On estimating the exponent of power-law frequency distributions. Ecology 89, 905–912. doi: 10.1890/07-1288.1
Wolf, M., Friar, J. L., Merrill, E., and Turchin, P. (2009). The attraction of the known: the importance of spatial familiarity in habitat selection in wapiti Cervus elaphus. Ecography 32, 401–410. doi: 10.1111/j.1600-0587.2008.05626.x
Zollner, P. A., and Lima, S. L. (1999). Search strategies for landscape-level interpatch movements. Ecology 80, 1019–1030. doi: 10.1890/0012-9658(1999)080[1019:SSFLLI]2.0.CO;2
Keywords: Lévy, Brownian, GPS satellite telemetry, raptors, animal movement, diet
Citation: Vidal-Mateo J, Benavent-Corai J, López-López P, García-Ripollés C, Mellone U, De la Puente J, Bermejo A and Urios V (2022) Search Foraging Strategies of Migratory Raptors Under Different Environmental Conditions. Front. Ecol. Evol. 10:666238. doi: 10.3389/fevo.2022.666238
Received: 09 February 2021; Accepted: 02 March 2022;
Published: 28 March 2022.
Edited by:
Miguel Ferrer, Spanish National Research Council (CSIC), SpainReviewed by:
Darryl Jones, Griffith University, AustraliaVirginia Morandini, Oregon State University, United States
Copyright © 2022 Vidal-Mateo, Benavent-Corai, López-López, García-Ripollés, Mellone, De la Puente, Bermejo and Urios. This is an open-access article distributed under the terms of the Creative Commons Attribution License (CC BY). The use, distribution or reproduction in other forums is permitted, provided the original author(s) and the copyright owner(s) are credited and that the original publication in this journal is cited, in accordance with accepted academic practice. No use, distribution or reproduction is permitted which does not comply with these terms.
*Correspondence: Javier Vidal-Mateo, javier.vidalm90@gmail.com