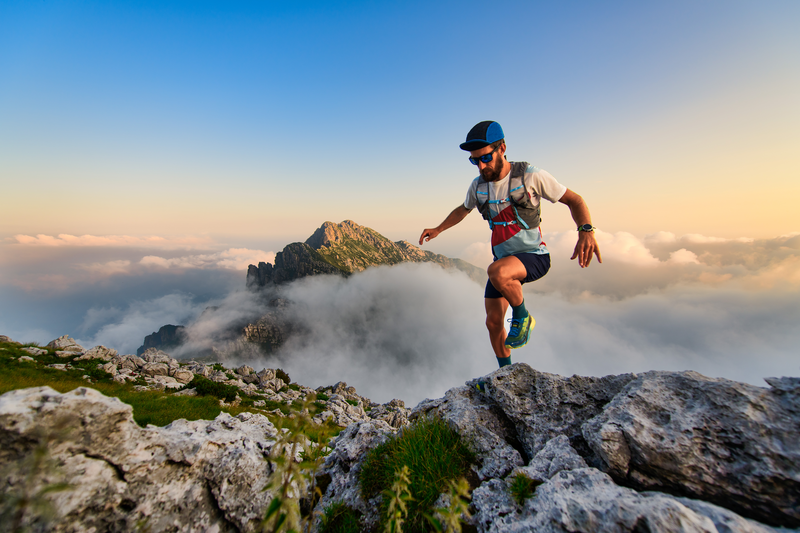
95% of researchers rate our articles as excellent or good
Learn more about the work of our research integrity team to safeguard the quality of each article we publish.
Find out more
ORIGINAL RESEARCH article
Front. Ecol. Evol. , 04 January 2023
Sec. Conservation and Restoration Ecology
Volume 10 - 2022 | https://doi.org/10.3389/fevo.2022.1103937
This article is part of the Research Topic Key Determinants of Biodiversity, Ecosystem Functioning and Restoration in Climate Change Sensitive Ecosystems View all 24 articles
Plants growing in forests at different succession stages in diverse habitats may adopt various life strategies from the perspective of plant functional traits. However, species composition differs with forest succession, and the effects of forest succession on traits have often been explored without considering the effects of species identity. We comprehensively investigated intraspecific variations in 12 traits of six overlapping species (two tree species and four understory shrub species) in three typical subtropical evergreen broad-leaved forests at different succession stages in eastern China. We found that intraspecific variations differed among traits. Fine root specific length presented large intraspecific variation, leaf area, specific leaf area and fine root tissue density showed medium intraspecific variations, and other traits displayed small intraspecific variations. Trees and understory shrubs in the early-stage forest exhibited higher leaf thickness, dry matter contents and tissue densities of leaves, roots, twigs, and stems and lower leaf area and specific leaf area. Those in the medium- and late-stage forests displayed contrasting trait characteristics. From the perspective of plant functional traits, plants in the early-stage forest formed a series of trait combinations for a resource conservative strategy with a low growth rate to adapt to fragile habitats with poor soil nutrients and changeable soil temperature and humidity, and those in the medium- and late-stage forests (especially the former) formed converse trait combinations for a resource acquisitive strategy with a high growth rate to adapt to low light availability and strongly competitive habitats. Our study reveals that plants in forests at different succession stages adopt various life strategies and provides data to the TRY and China plant trait databases.
Plant functional traits are measurable characteristics formed from long-term plant-environment interactions and are closely linked to the growth, reproduction, and survival processes of plants (Violle et al., 2007; Garnier et al., 2016; Kattge et al., 2020). A single trait and the combinations of multiple traits not only respond to plant life history strategy, biodiversity, vegetation, environment, and disturbance changes but also affect ecosystem processes and functions (Cornelissen et al., 2003; Vandewalle et al., 2010; Mensens et al., 2017; Wang et al., 2020). Therefore, the study of plant functional traits is at the forefront of modern ecology and global change ecology (Meng et al., 2007; Liu and Ma, 2015; Blondeel et al., 2020).
Forests are the largest vegetated areas in global terrestrial ecosystems and play important roles in global biodiversity maintenance and climate change mitigation (Melillo et al., 1993; Anderson-Teixeira et al., 2015). Plant functional traits, such as morphological traits and C: N: P ecological stoichiometry of leaves, roots, stems, and twigs (especially leaves) in forests worldwide have been intensively investigated at local, regional and global scales (McGroddy et al., 2004; Chave et al., 2009; Díaz et al., 2016; He et al., 2019; Guerin et al., 2021; Liu et al., 2022). Compared to interspecific trait variations (trait differences among species) of woody plants in forests, intraspecific trait variations (trait differences among independent individuals within a species) are yet to be fully investigated. However, intraspecific trait variations are non-negligible, and they may account for an average of 32% of the total trait variations based on a global database (Siefert et al., 2015). Environmental factors, such as climate, topography, and soil nutrition, greatly affect intraspecific trait variations (Hultine and Marshall, 2000; Thuiller et al., 2004; Quested et al., 2007; Wang et al., 2016a).
In addition, most plant functional trait studies conducted in forests have focused on dominant trees, and understory shrubs have rarely been described. As an important component of forest ecosystems, understory shrubs influence the energy and water cycles of forests and participate in forest renewal, development, and succession (Cao et al., 2020). In limited trait studies on understory shrubs, the latitudinal distribution pattern and driving factors of leaf traits (Luo et al., 2019), the correlation between morphological traits and nitrogen and phosphorus nutrients (Wang et al., 2017), relationships between twigs and leaf traits at different succession stages (Ma, 2014), and different phenotypes of understory shrubs (Wang et al., 2016b) have been reported. Light conditions and canopy coverage are the main environmental factors driving intraspecific variations in leaf traits (Burton et al., 2017). However, studies on intraspecific trait variation in understory shrubs in forests at different succession stages in different habitats are scarce (Kumordzi et al., 2019; Vanneste et al., 2019; Cao et al., 2020).
Environments (or habitats), species composition, and the structure of a forest community change with progressive succession. In early-stage forests, illumination is sufficient, but soil nutrients are poor, and soil temperature and humidity fluctuate. Such forests are committed to gap formation, understory initiation, and regeneration (Moridi et al., 2015; Zhang et al., 2016). In medium-stage forests, soil humidity and nutrients are greatly improved, but light availability is the lowest because of the highest canopy coverage and stand density. Such forests are in volume accumulation, lightning, and stem exclusion phases (Zhang et al., 1999; Wang et al., 2004; Yan et al., 2008; Moridi et al., 2015). Light conditions improve in late-stage forests because of slightly lower canopy coverage and stand density (Moridi et al., 2015; Zhang et al., 2016). Some species (turnover species) only appear in forests at one succession stage and disappear in the next stage, whereas, some species (overlapping species) grow in forests at many succession stages (Yang et al., 2014). Overlapping species in the early-stage forest may form a series of trait combinations for a resource conservative strategy to adapt to fragile habitats with poor soil nutrients and changeable soil temperature and humidity, and those in the medium- and late-stage forests may form converse trait combinations for a resource acquisitive strategy to adapt to low light availability and strongly competitive habitats (Li and Ma, 2002).
Originating from the uplift of the Qinghai–Tibet Plateau, a broad subtropical evergreen broad-leaved forest was found in China. This forest is a unique vegetation type on Earth and is essential to the global gene pool and regional environments (Song, 2013). In the present study, dominant overlapping species, including tree and understory shrub species, in three evergreen broad-leaved forests at different succession stages in eastern China were investigated as examples. The leaf, twig, stem, and root traits were comprehensively measured. Here, the life strategies of plants growing in forests at different succession stages in various habitats were determined from the perspective of plant functional traits. Specifically, the following predictions were made. (1) Overlapping species in the forests at three succession stages present significant differences in plant functional traits. (2) Plants (trees and understory shrubs) in the early-stage forest form a series of trait combinations with higher leaf thickness (LT), dry matter contents and tissue densities of leaves, roots, twigs, and stems and lower leaf area (LA) and specific leaf area (SLA) for a resource conservative strategy with a low growth rate. Plants in the medium- and late-stage forests form converse trait combinations for a resource acquisitive strategy with a high growth rate. This study provides basic data to global (Kattge et al., 2020) and China (He et al., 2019) plant trait databases and guides the sustainable management and ecological restoration of evergreen broad-leaved forests in eastern China.
Field measurements were conducted in three permanent monitoring plots of the Zhejiang Tiantong Forest Ecosystem National Observation and Research Station (121.783° E, 29.800° N) in the Tiantong National Forest Park, Zhejiang Province, Eastern China. This area is located in mid-subtropical China and controlled by monsoon climates. The mean annual temperature is 16.2°C, with temperatures of 4.2°C and 28.1°C in January and July, respectively. Considerably high and low temperatures are 38.7°C and −8.5°C, respectively. The growing degree days on the base of 10°C is 5,166.2°C. The mean annual precipitation and evaporation are 1,374.7 and 1,320.1 mm, respectively. The native vegetation is subtropical evergreen broad-leaved forest dominated by Castanopsis fargesii Franch. and Schima superba Gardn. et Champ. Existing forests in the Tiantong National Forest Park are classified as early-, medium-, and late-stage forests along a secondary succession series because of the intensity of human disturbances (Song and Wang, 1995). Three forest plots (each with an area of 2,500 m2, 50 m × 50 m) at three succession stages (i.e., early, medium and late stages) were established and investigated in 2007.
The early-stage forest (121.786° E, 29.801° N) is 20° south of the east and has a 25° slope. This forest is restored from a shrubland with a stand age of 35 y. The height and coverage of the tree layer are 8 m and 70%, respectively. Its dominant tree species is S. superba. Another common species in the tree layer is C. fargesii. Loropetalum chinense (R. Br.) Oliver and Symplocos sumuntia Buch. -Ham. ex D. Don are dominant understory shrub species. The medium-stage forest (121.788° E, 29.786° N) is 70° south of the east and has a 20° slope. The stand age is 90 y, and the height and coverage of the tree layer are 15 m and 95%, respectively. This forest is also dominated by S. superba, and C. fargesii is distributed in the subtree layer. The main understory shrub species are Eurya rubiginosa var. attenuata H. T. Chang, Camellia fraterna Hance, and S. sumuntia. The late-stage forest (121.787° E, 29.808° N) is 45° south of the east and has a 26° slope. This forest is in the climax stage with a stand age of 170 y. The height and coverage of the tree layer are 18 m and 90%, respectively. C. fargesii is the dominant tree species, and S. superba is widely distributed in the subtree layer. E. rubiginosa, C. fraterna, and L. chinense are common in the understory shrub layer.
Overlapping species, including two tree species (C. fargesii and S. superba) and four understory shrub species (C. fraterna, S. sumuntia, E. rubiginosa, and L. chinense), in the three forests were selected for trait measurements. Ten healthy dominant individuals per species in each plot were chosen. From each individual, a branch was collected from the sunlit tree canopy by using a long reach chain saw (tree species) or collected from the upper canopy by using a lopper (understory shrub species). Twenty mature leaves without visible damage were collected. An approximately 20 cm length current-year twig was sampled using a lopper, and a stem sample was determined using a SY-CO (trees) or a lopper (shrubs). The roots of trees were difficult to recognize, and C. fraterna was grown in rocks in the early-stage forest, so only fine roots (root diameter ≤ 2 mm) of three understory shrub species, that is, S. sumuntia, E. rubiginosa, and L. chinense, were sampled using a hoe.
Fresh masses of leaf, twig, and stem samples were weighed using an electronic balance. Leaf thickness was measured using an electronic Vernier caliper. The length and volume of fine roots and LA were scanned using a WinFOLIA multipurpose leaf area meter (Regent Instruments, Canada). Leaf sample volume was calculated as the product of LA and LT, and twig and stem sample volumes were determined using the drainage method (Cornelissen et al., 2003). All samples were dried in an oven at 80°C for 72 h to determine their dry masses. The values of traits were calculated as shown in Table 1.
The coefficient of intraspecific variation (standard deviation divided by mean) was used to characterize the varying degrees of traits among individuals of a certain species. One-way ANOVA was conducted to determine the trait differences among plant growth forms (trees and understory shrubs) and species in the forests at three succession stages. The principal component analysis (PCA) was done to evaluate the effect of plant species on traits, and show the distributions of traits among plant species. Trait data were log-transformed prior to PCA analysis. Fisher’s least significant difference (homogeneity of variance) or Tamhane (heterogeneity of variance) methods were used to conduct post hoc tests after the homogeneity test of variances. All statistical analyses were performed using the SPSS version 20 and the CANOCO 5.
The 12 plant functional traits varied among plant species (Figure 1). Among the stem, twig, and leaf traits, C. fargesii exhibited the highest leaf dry matter content (LDMC) and the lowest stem dry matter content (SDMC) and stem tissue density (STD). S. superba showed the highest LA and leaf tissue density (LTD) and the lowest SLA, twig dry matter content (TDMC), and twig tissue density (TTD). S. sumuntia presented the lowest LDMC and LTD, E. rubiginosa had the highest LT. L. chinense displayed the highest SLA, TDMC, TTD, SDMC, and STD and the lowest LT and LA. C. fraterna had intermediate trait values. Among the root traits, S. sumuntia presented the lowest fine root dry matter content (RDMC) and fine root tissue density (RTD). E. rubiginosa had the highest RTD and the lowest fine root specific length (SRL). L. chinense displayed the highest SRL and RDMC.
Figure 1. Plant functional traits and their intraspecific variations in overlapping species in three evergreen broad-leaved forests. The circles and stars in the box plots indicate the abnormal values. The percentage of data in the box plots are the coefficients of trait intraspecific variation. 1–6 represent species in the ordinate: species number 1, Castanopsis fargesii; 2, Schima superba; 3, Camellia fraterna; 4, Symplocos sumuntia; 5, Eurya rubiginosa var. attenuata; 6, Loropetalum chinense. LT, leaf thickness; LA, leaf area; SLA, specific leaf area; LDMC, leaf dry matter content; LTD, leaf tissue density; TDMC, twig dry matter content; TTD, twig tissue density; SDMC, stem dry matter content; STD, stem tissue density; SRL, fine root specific length; RDMC, fine root dry matter content; RTD, fine root tissue density.
The intraspecific variations differed among plant functional traits (Figure 1). SRL exhibited the largest intraspecific variations. The intraspecific variations in the SRL of S. sumuntia and L. chinense were highly variable, indicated by large coefficients of intraspecific variation (56.1 and 51.7%, respectively). The coefficients of intraspecific variation of LA, SLA, and RTD generally ranged from 15 to 35%. Other traits displayed small intraspecific variations, indicated by small coefficients of intraspecific variation, especially for LDMC, SDMC, and STD, whose coefficients of intraspecific variation were generally less than 10%.
The LA, SDMC, and STD of trees were close in the forests at three succession stages (FLA = 2.904, FSDMC = 1.694, FSTD = 2.892, P > 0.05), whereas other traits showed significant differences (FLT = 20.858, FSLA = 20.012, FLDMC = 11.176, FLTD = 7.204, FTDMC = 4.497, FTTD = 8.709, P < 0.05; Figure 2). Trees in the early-stage forest presented the highest LT, LDMC, LTD, TTD, SDMC, and STD and the lowest LA and SLA; trees in the medium-stage forest exhibited the highest LA and SLA and the lowest LDMC, TDMC, TTD, SDMC, and STD; and those in the late-stage forest generally displayed intermediate trait values (Figure 2). Understory shrubs in the forests at three succession stages showed small discrepancies in LT, TDMC, and SDMC (FLT = 1.934, FTDMC = 0.169, FSDMC = 0.477, P > 0.05), but they exhibited significant differences in other traits (FLA = 2.082, FSLA = 6.294, FLDMC = 5.716, FLTD = 5.642, FTTD = 14.524, FSTD = 4.676, FSRL = 15.995, FRDMC = 9.023, FRTD = 6.521, P < 0.05; Figure 2). Shrubs in the early-stage forest presented the highest LT, TTD, RDMC, and RTD and the lowest LA, SLA, and SRL; shrubs in the medium-stage forest exhibited the highest SLA and SRL and the lowest LT, LDMC, LTD, TDMC, TTD, SDMC, STD, RDMC, and RTD; and those in the late-stage forest also displayed intermediate trait values (Figure 2).
Figure 2. Plant functional traits of plant growth forms in forests at different succession stages. Trait values with different letters among succession stages are significantly different (one-way ANOVA, P < 0.05). LT, leaf thickness; LA, leaf area; SLA, specific leaf area; LDMC, leaf dry matter content; LTD, leaf tissue density; TDMC, twig dry matter content; TTD, twig tissue density; SDMC, stem dry matter content; STD, stem tissue density; SRL, fine root specific length; RDMC, fine root dry matter content; RTD, fine root tissue density.
The traits of overlapping species in forests at three succession stages differed to varying degrees (Figures 3, 4 and Supplementary Table 1). In general, plants in the early-stage forest exhibited high LT, LDMC, LTD, TDMC, TTD, SDMC, STD, RDMC, and RTD and low SLA and SRL; plants in the medium-stage forest presented contrary trait characteristics compared with those in the early-stage forest; and those in the late-stage forest displayed intermediate trait values except high LA (Figure 4). Specifically, the LA, TDMC, TTD, SDMC, and STD of C. fargesii exhibited small discrepancies in the forests at three succession stages (FLA = 1.851, FTDMC = 2.945, FTTD = 0.649, FSDMC = 0.183, FSTD = 0.424, P > 0.05). However, C. fargesii in the early-stage forest exhibited significantly higher LT, LDMC, and LTD and significantly lower SLA (FLT = 7.101, FLDMC = 7.469, FLTD = 4.041, FSLA = 12.254, P < 0.05; Figure 4). All traits of S. superba showed significant differences in the forests at three succession stages (P < 0.05). S. superba in the early-stage forest showed significantly higher LT, LDMC, SDMC, and STD and significantly lower LA and SLA (FLT = 64.481, FLDMC = 10.140, FSDMC = 15.454, FSTD = 19.011, FLA = 11.506, FSLA = 41.995, P < 0.05); S. superba in the medium-stage forest showed significantly lower LTD, TDMC, and TTD (FLTD = 5.079, FTDMC = 16.451, FTTD = 17.975, P < 0.05; Figure 4). The LA, SDMC, and STD of C. fraterna did not differ in the forests at three succession stages (FLA = 3.021, FSDMC = 0.318, FSTD = 0.243, P > 0.05). Conversely, C. fraterna in the early-stage forest had significantly higher LT and TDMC (FLT = 11.128, FTDMC = 15.314, P < 0.05); C. fraterna in the medium-stage forest had significantly higher SLA and significantly lower LDMC (FSLA = 8.543, FLDMC = 13.261, P < 0.05); and C. fraterna in the late-stage forest had significantly higher LTD and TTD (FLTD = 27.678, FTTD = 9.051, P < 0.05; Figure 4). The LDMC, LTD, and TDMC of S. sumuntia presented small discrepancies in the forests at three succession stages (FLDMC = 2.181, FLTD = 1.721, FTDMC = 5.545, P > 0.05). However, S. sumuntia in the early-stage forest presented significantly higher LT, TTD, and RTD and significantly lower SLA (FLT = 2.999, FTTD = 3.754, FRTD = 6.109, FSLA = 6.277, P < 0.05); S. sumuntia in the medium-stage forest presented significantly higher SRL and significantly lower SDMC, STD, and RDMC (FSRL = 12.966, FSDMC = 10.480, FSTD = 5.873, FRDMC = 30.602, P < 0.05); and S. sumuntia in the late-stage forest presented significantly higher LA (FLA = 4.846, P < 0.05; Figure 4). The LT, SLA, LDMC, LTD, SRL, and RTD of E. rubiginosa exhibited no differences in the forests at three succession stages (FLT = 0.745, FSLA = 1.043, FLDMC = 0.566, FLTD = 2.468, FSRL = 1.329, FRTD = 0.453, P > 0.05). By comparison, E. rubiginosa in the medium-stage forest exhibited significantly higher TDMC and SDMC and significantly lower TTD and STD (FTDMC = 3.110, FSDMC = 4.452, FTTD = 49.393, FSTD = 22.889, P < 0.05). E. rubiginosa in the late-stage forest exhibited significantly higher LA and significantly lower RDMC (FLA = 3.057, FRDMC = 4.201, P < 0.05; Figure 4). The LA, LTD, TDMC, TTD, SDMC, and STD of L. chinense displayed small discrepancies in the forests at three succession stages (FLA = 1.863, FLTD = 1.427, FTDMC = 3.032, FTTD = 3.858, FSDMC = 0.534, FSTD = 1.092, P > 0.05). Conversely, L. chinense in the medium-stage forest exhibited significantly higher SRL and significantly lower LT, SLA, LDMC, RDMC, and RTD (FSRL = 9.020, FLT = 6.386, FSLA = 14.432, FLDMC = 7.311, FRDMC = 2.477, FRTD = 6.157, P < 0.05; Figure 4).
Figure 3. PCA showing the distribution of plant functional traits among species in forests at different succession stages. (A,D) Early-stage forest; (B,E) medium-stage forest; (C,F) late-stage forest. LT, leaf thickness; LA, leaf area; SLA, specific leaf area; LDMC, leaf dry matter content; LTD, leaf tissue density; TDMC, twig dry matter content; TTD, twig tissue density; SDMC, stem dry matter content; STD, stem tissue density; SRL, fine root specific length; RDMC, fine root dry matter content; RTD, fine root tissue density.
Figure 4. Plant functional traits of overlapping species in forests at different succession stages. Trait values with different letters among succession stages are significantly different (one-way ANOVA, P < 0.05). 1–6 represent species in the ordinate: species number 1, Castanopsis fargesii; 2, Schima superba; 3, Camellia fraterna; 4, Symplocos sumuntia; 5 Eurya rubiginosa var. attenuata; 6, Loropetalum chinense. LT, leaf thickness; LA, leaf area; SLA, specific leaf area; LDMC, leaf dry matter content; LTD, leaf tissue density; TDMC, twig dry matter content; TTD, twig tissue density; SDMC, stem dry matter content; STD, stem tissue density; SRL, fine root specific length; RDMC, fine root dry matter content; RTD, fine root tissue density.
Few studies on intraspecific trait variations have been conducted compared with studies on interspecific trait variation in the world’s forests, and most trait studies have focused on trees and their leaf traits, such as LA, LDMC, and SLA (Rosbakh et al., 2015; Siefert et al., 2015; Zhou, 2016; Heilmeier, 2019; Zhang et al., 2019). The intraspecific trait variation characteristics of understory shrubs and those of the twigs, stems, and roots of trees have rarely been reported (Burton et al., 2017; Zhong et al., 2018; Cao et al., 2020). The present study comprehensively investigated the intraspecific variation characteristics of 12 traits of leaves, twigs, stems, and roots of six common species (two tree species and four understory shrub species) in three typical subtropical evergreen broad-leaved forests in eastern China. This study could fill the gap in plant functional trait studies in subtropical China.
Since the application of succession in plant ecology research, studies on vegetation succession have been widely performed. Biodiversity and carbon storage were often used as indicators to evaluate vegetation succession processes in early studies (Ni et al., 2015; Capellesso et al., 2021). Plant functional traits and trait-based community ecology (a theory using trait-based approaches to determine community composition, structures and functions) offer an alternative approach to reveal the colonization, survival, growth, and death processes of plants and plant communities in different succession vegetation (Song et al., 2018). However, species compositions vary in different succession vegetations, and traits of different species were measured in previous studies. Rather than considering several dominant species in different succession vegetation (Chazdon, 2008; Muscarella et al., 2017), the present study focused on overlapping species and provided another pathway to understand the interaction of plant functional traits and vegetation succession.
Plant functional traits are codetermined by species identity (genetic factors) and environment (Scheiner and Lyman, 1991; Weiher and Keddy, 1995). Environments promote the coexistence of species with similar functional traits in local habitats, but intraspecific trait variations cause differences in the life history strategy of a certain species or different species (Ackerly and Cornwell, 2007; Auger and Shipley, 2013; Siefert et al., 2015; Kumordzi et al., 2019). However, the species composition of a plant community differs with the environment. The effects of environments on traits are often discussed without considering the effects of species identity, or some statistical analysis methods, such as two-way ANOVA, are used in species–environment interactive analysis in other studies (Zhong et al., 2018; Cao et al., 2020). In the present study, overlapping species in three typical subtropical evergreen broad-leaved forests with different habitats in eastern China are investigated as an example to explore the effects of the environment on traits. This investigation method is preferred because of the direct exclusion of the effects of species identity.
Root traits often exhibit the largest interspecific and intraspecific variations resulting from complex and diverse below-ground habitats (Comas and Eissenstat, 2004). Leaf traits display larger interspecific and intraspecific variations than twig and stem traits (Ackerly and Reich, 1999; Garnier et al., 2001; Zhong et al., 2018). In the present study, variations in the intraspecific traits of overlapping species in the forests at three succession stages with different habitats ranged from 2.8% to 56.1%, with an average of 13.5%. Some traits of roots and leaves considerably varied. SRL, RTD, LA, and SLA also differed, but RDMC, LT, LTD, and LDMC (especially the last leaf trait) slightly varied. Consistent with early studies (Garnier et al., 2001), our study showed that intraspecific variations in twig and stem traits slightly differed.
A single trait and the combinations of multiple traits can indicate the adaptation and response of plants to certain environments, expose the plant trade-off strategies of resource acquisition and utilization, and reveal the different life history strategies of plants in different environments (Wright et al., 2004; Díaz et al., 2016). Some species (resource acquisitive strategy adopters) present a high SLA, leaf nitrogen content, photosynthetic and respiratory rates, and short lifespans. Some other species (resource conservative strategy adopters) display low SLA, leaf nitrogen content, photosynthetic and respiratory rates, and long lifespan (Chen and Xu, 2014). Such plant and trait combinations favor niche overlap and competition reduction, rich biodiversity maintenance, and ecosystem stability enhancement (Liu and Ma, 2015). In the present study, the early-stage forest is a relatively fragile ecosystem with poor soil nutrients and fluctuating soil temperature and humidity. Trees and understory shrubs in such forests allocate more nutrients to resist adverse environments. Thus, they adopt a slow investment-return (resource conservation) strategy and form a series of trait combinations with higher LT, LDMC, LTD, TDMC, TTD, SDMC, STD, RDMC, and RTD and lower SLA and SRL for storing more nutrients and growing slower. However, in the medium- and late-stage forests, trees and understory shrubs are exposed to lower light availability and stronger competition. They adopt a quick investment-return (resource acquisitive) strategy and are associated with a series of trait combinations with higher LA, SLA, and SRL and lower LT, LDMC, LTD, TDMC, TTD, SDMC, STD, RDMC, and RTD for faster growth. Plants in medium-stage forests are more resource acquisitive for lower light availability and stronger competition than those in late-stage forests.
The remaining primary evergreen broad-leaved forests only account for 4% of its total distribution area in eastern China because of intensive human disturbances. Degraded secondary forests, shrub and grass communities, and bare land are distributed everywhere. However, excellent hydrothermal conditions promote secondary forest succession once human disturbances stop. Evergreen broad-leaved forests may recover gradually in this area. Therefore, research on the secondary succession mechanism and restoration ecology of evergreen broad-leaved forests should be strengthened (Song, 2013). Understanding the trend of changes in trait characteristics with vegetation succession and the life strategies of plants adapted to different succession vegetation is important not only for providing data to the global and China plant trait databases but also for establishing regional forest management and restoration in eastern China.
The original contributions presented in this study are included in the article/Supplementary material, further inquiries can be directed to the corresponding author.
LL and YW conceived and designed the research. LL and HX analyzed the data. XQ contributed to the field work. LL wrote the first draft with substantial input from YW. All authors contributed to the article and approved the submitted version.
This study was funded by the Open Research Fund of Zhejiang Tiantong Forest Ecosystem National Observation and Research Station (TTK201902) and the Zhejiang Provincial Natural Science Foundation of China (LQ20C030003 and LQ22C030001).
We thank the Zhejiang Tiantong Forest Ecosystem National Observation and Research Station for assistance in all field work. YW acknowledges the support of Zhejiang Qianjiangyuan Forest Biodiversity National Observation and Research Station. We are grateful to Prof. Jian Ni for his suggestions for improving the manuscript.
The authors declare that the research was conducted in the absence of any commercial or financial relationships that could be construed as a potential conflict of interest.
All claims expressed in this article are solely those of the authors and do not necessarily represent those of their affiliated organizations, or those of the publisher, the editors and the reviewers. Any product that may be evaluated in this article, or claim that may be made by its manufacturer, is not guaranteed or endorsed by the publisher.
The Supplementary Material for this article can be found online at: https://www.frontiersin.org/articles/10.3389/fevo.2022.1103937/full#supplementary-material
Ackerly, D. D., and Cornwell, W. K. (2007). A trait-based approach to community assembly: Partitioning of species trait values into within- and among-community components. Ecol. Lett. 10, 135–145. doi: 10.1111/j.1461-0248.2006.01006.x
Ackerly, D. D., and Reich, P. B. (1999). Convergence and correlations among leaf size and function in seed plants: A comparative test using independent contrasts. Am. J. Bot. 86, 1272–1281. doi: 10.2307/2656775
Anderson-Teixeira, K. J., Davies, S. J., Bennett, A. C., Gonzalez-Akre, E. B., Muller-Landau, H. C., Wright, S. J., et al. (2015). CTFS-ForestGEO: A worldwide network monitoring forests in an era of global change. Glob. Change Biol. 21, 528–549. doi: 10.1111/gcb.12712
Auger, S., and Shipley, B. (2013). Inter-specific and intra-specific trait variation along short environmental gradients in an old-growth temperate forest. J. Veg. Sci. 24, 419–428. doi: 10.1111/j.1654-1103.2012.01473.x
Blondeel, H., Perring, M. P., Depauw, L., Lombaerde, E. D., Landuyt, D., Frenne, P. D., et al. (2020). Light and warming drive forest understorey community development in different environments. Glob. Change Biol. 26, 1681–1696. doi: 10.1111/gcb.14955
Burton, J. I., Perakis, S. S., McKenzie, S. C., Lawrence, C. E., and Puettmann, K. J. (2017). Intraspecific variability and reaction norms of forest understorey plant species traits. Funct. Ecol. 31, 1881–1893. doi: 10.1111/1365-2435.12898
Cao, J. Y., Liu, J. F., Yuan, Q., Xu, D. Y., Fan, H. D., Chen, H. Y., et al. (2020). Traits of shrubs in forests and bushes reveal different life strategies. Chin. J. Plant Ecol. 44, 715–729. doi: 10.17521/cjpe.2020.0024
Capellesso, E. S., Cequinel, A., Marques, R., Sausen, T. L., Bayer, C., and Marques, M. C. M. (2021). Co-benefits in biodiversity conservation and carbon stock during forest regeneration in a preserved tropical landscape. For. Ecol. Manag. 492:119222. doi: 10.1016/j.foreco.2021.119222
Chave, J., Coomes, D., Jansen, S., Lewis, S. L., Swenson, N. G., and Zanne, A. E. (2009). Towards a worldwide wood economics spectrum. Ecol. Lett. 12, 351–366. doi: 10.1111/j.1461-0248.2009.01285.x
Chazdon, R. L. (2008). “Chance and determinism in tropical forest succession,” in Tropical forest community ecology, eds W. Carson and S. A. Schnitzer (Hoboken, NJ: Wiley-Blackwell Publishing), 384–408.
Chen, Y. T., and Xu, Z. Z. (2014). Review on research of leaf economics spectrum. Chin. J. Plant Ecol. 38, 1135–1153. doi: 10.3724/SP.J.1258.2014.00108
Comas, L. H., and Eissenstat, D. M. (2004). Linking fine root traits to maximum potential growth rate among 11 mature temperate tree species. Funct. Ecol. 18, 388–397. doi: 10.1111/j.0269-8463.2004.00835.x
Cornelissen, J. H. C., Lavorel, S., Garnier, E., Díaz, S., Buchmann, N., Gurvich, D. E., et al. (2003). A handbook of protocols for standardised and easy measurement of plant functional traits worldwide. Aust. J. Bot. 51, 335–380. doi: 10.1071/BT02124
Díaz, S., Kattge, J., Cornelissen, J. H. C., Wright, I. J., Lavorel, S., Dray, S., et al. (2016). The global spectrum of plant form and function. Nature 529, 167–171. doi: 10.1038/nature16489
Garnier, E., Laurent, G., Bellmann, A., Debain, S., Berthelier, P., Ducout, B., et al. (2001). Consistency of species ranking based on functional leaf traits. New Phytol. 152, 69–83. doi: 10.1046/j.0028-646x.2001.00239.x
Garnier, E., Navas, M.-L., and Grigulis, K. (2016). Plant functional diversity: Organism traits, community structure, and Ecosystem properties. Oxford: Oxford University Press. doi: 10.1093/acprof:oso/9780198757368.001.0001
Guerin, G. R., Gallagher, R. V., Wright, I. J., Andres, S. C., Falster, D. S., Wenk, E., et al. (2021). Environmental associations of abundance-weighted functional traits in Australian plant communities. Basic Appl. Ecol. 58, 98–109. doi: 10.1016/j.baae.2021.11.008
He, N. P., Liu, C. C., Piao, S. L., Sack, L., Xu, L., Luo, Y. Q., et al. (2019). Ecosystem traits linking functional traits to macroecology. Trends Ecol. Evol. 34, 200–210. doi: 10.1016/j.tree.2018.11.004
Heilmeier, H. (2019). Functional traits explaining plant responses to past and future climate changes. Flora 254, 1–11. doi: 10.1016/j.flora.2019.04.004
Hultine, K. R., and Marshall, J. D. (2000). Altitude trends in conifer leaf morphology and stable carbon isotope composition. Oecologia 123, 32–40. doi: 10.1007/s004420050986
Kattge, J., Bönisch, G., Díaz, S., Lavorel, S., Prentice, I. C., and Wirth, C. (2020). TRY plant trait database-enhanced coverage and open access. Glob. Change Biol. 26, 119–188. doi: 10.1111/gcb.14904
Kumordzi, B. B., Aubin, I., Cardou, F., Shipley, B., Violle, C., and Johnstone, J. (2019). Geographic scale and disturbance influence intraspecific trait variability in leaves and roots of North American understorey plants. Funct. Ecol. 33, 1771–1784. doi: 10.1111/1365-2435.13402
Li, Q. K., and Ma, K. P. (2002). Advances in plant succession ecophysiology. Acta Phytoecol. Sin. 26, 9–19.
Liu, L. B., Hu, J., Chen, X. Y., Xu, X., Yang, Y., and Ni, J. (2022). Adaptation strategy of karst forests: Evidence from the community-weighted mean of plant functional traits. Ecol. Evol. 12:e8680. doi: 10.1002/ece3.8680
Liu, X. J., and Ma, K. P. (2015). Plant functional traits–concepts, applications and future directions. Sci. Sin. Vitae 45, 325–339. doi: 10.1360/N052014-00244
Luo, Y. K., Hu, H. F., Zhao, M. Y., Li, H., Liu, S. S., and Fang, J. Y. (2019). Latitudinal pattern and the driving factors of leaf functional traits in 185 shrub species across eastern China. J. Plant Ecol. 12, 67–77. doi: 10.1093/jpe/rtx065
Ma, X. L. (2014). A study on twig and leaf traits of the shrub species in secondary forest succession process. Ph.D. thesis. Lanzhou: Northwest Normal University.
McGroddy, M. E., Daufresne, T., and Hedin, L. O. (2004). Scaling C, N, P stoichiometry in forests worldwide, implications of terrestrial redfield-type ratios. Ecology 85, 2390–2401. doi: 10.1890/03-0351
Melillo, J. M., McGuire, A. D., Kicklighter, D. W., Moore, I. B., Vorosmarty, C. J., and Schloss, A. L. (1993). Global climate change and terrestrial net primary production. Nature 363, 234–240. doi: 10.1038/363234a0
Meng, T. T., Ni, J., and Wang, G. H. (2007). Plant functional traits, environments and ecosystem functioning. Chin. J. Plant Ecol. 31, 150–165. doi: 10.17521/cjpe.2007.0019
Mensens, C., Laender, F. D., Janssen, C. R., Sabbe, K., and Troch, M. D. (2017). Different response-effect trait relationships underlie contrasting responses to two chemical stressors. J. Ecol. 105, 1598–1609. doi: 10.1111/1365-2745.12777
Moridi, M., Sefidi, K., and Etemad, V. (2015). Stand characteristics of mixed oriental beech (Fagus orientalis Lipsky) stands in the stem exclusion phase, northern Iran. Eur. J. For. Res. 134, 693–703. doi: 10.1007/s10342-015-0883-1
Muscarella, R., Lohbeck, M., Martínez-Ramos, M., Poorter, L., Rodríguez-Velázquez, J. E., van Breugel, M., et al. (2017). Demographic drives of functional composition dynamics. Ecology 98, 2743–2750. doi: 10.1002/ecy.1990
Ni, J., Luo, D. H., Xia, J., Zhang, Z. H., and Hu, G. (2015). Vegetation in karst terrain of southwestern China allocates more biomass to roots. Solid Earth 6, 799–810. doi: 10.5194/se-6-799-2015
Quested, H., Eriksson, O., Fortunel, C., and Garnier, E. (2007). Plant traits relate to whole-community litter quality and decomposition following land use change. Funct. Ecol. 21, 1016–1026. doi: 10.1111/j.1365-2435.2007.01324.x
Rosbakh, S., Romermann, C., and Poschlod, P. (2015). Specific leaf area correlates with temperature: New evidence of trait variation at the population, species and community levels. Alp. Bot. 125, 79–86. doi: 10.1007/s00035-015-0150-6
Scheiner, S. M., and Lyman, R. F. (1991). The genetics of phenotypic plasticity. II. Response to selection. J. Evol. Biol. 4, 23–50. doi: 10.1046/j.1420-9101.1991.4010023.x
Siefert, A., Violle, C., Chalmandrier, L., Albert, C. H., Taudiere, A., Fajardo, A., et al. (2015). A global meta-analysis of the relative extent of intraspecific trait variation in plant communities. Ecol. Lett. 18, 1406–1419. doi: 10.1111/ele.12508
Song, G. M., Han, T. T., Hong, L., Zhang, L. L., Li, X. B., and Ren, H. (2018). Advances in the studies of plant functional traits during succession. Ecol. Sci. 37, 207–213.
Song, Y. C. (2013). China evergreen broad-leaved forest: Classification, ecology, conservation. Beijing: Science Press.
Song, Y. C., and Wang, X. R. (1995). Vegetation and flora of Tiantong national forest park Zhejiang province. Shanghai: Shanghai Science and Technical Literature Press.
Thuiller, W., Lavorel, S., Midgley, G., Lavergne, S., and Rebelo, T. (2004). Relating plant traits and species distributions along bioclimatic gradients for 88 Leucadendron taxa. Ecology 85, 1688–1699. doi: 10.1890/03-0148
Vandewalle, M., Bello, F., Berg, M. P., Bolger, T., Dolédec, S., Dubs, F., et al. (2010). Functional traits as indicators of biodiversity response to land use changes across ecosystems and organisms. Biodivers. Conserv. 19, 2921–2947. doi: 10.1007/s10531-010-9798-9
Vanneste, T., Valdés, A., Verheyen, K., Perring, M. P., Bernhardt-Römermann, M., Andrieu, E., et al. (2019). Functional trait variation of forest understorey plant communities across Europe. Basic Appl. Ecol. 34:02356900. doi: 10.1016/j.baae.2018.09.004
Violle, C., Navas, M. L., Vile, D., Kazakou, E., Fortunel, C., Hummel, I., et al. (2007). Let the concept of trait be functional! Oikos 116, 882–892. doi: 10.1111/j.0030-1299.2007.15559.x
Wang, C. Y., Xiao, H. G., Liu, J., and Zhou, J. W. (2016b). Differences in leaf functional traits between red and green leaves of two evergreen shrubs Photinia × fraseri and Osmanthus fragrans. J. For. Res. 28, 473–479. doi: 10.1007/s11676-016-0346-7
Wang, M., Wang, P. C., Guo, J. C., Xu, J. S., Chai, Y. F., and Yue, M. (2017). Relationships among leaf, stem and root traits of the dominant shrubs from four vegetation zones in Shanxi province, China. Isr. J. Ecol. Evol. 63, 25–32. doi: 10.1163/22244662-06301005
Wang, R. L., Yu, G. R., He, N. P., Wang, Q. F., Zhao, N., and Xu, Z. W. (2016a). Altitudinal variation in the covariation of stomatal traits with leaf functional traits in Changbai Mountain. Acta Ecol. Sin. 36, 2175–2184. doi: 10.5846/stxb201411042162
Wang, X. H., Huang, J. J., and Yan, E. R. (2004). Leaf litter decomposition of common trees in Tiantong. Acta Phytoecol. Sin. 28, 457–467. doi: 10.17521/cjpe.2004.0063
Wang, Y. Q., Cadotte, M. W., Chen, J. H., Mi, X. C., Ren, H. B., Liu, X. J., et al. (2020). Neighborhood interactions on seedling survival were greatly altered following an extreme winter storm. For. Ecol. Manag. 461:117940. doi: 10.1016/j.foreco.2020.117940
Weiher, E., and Keddy, P. A. (1995). Assembly rules, null models, and trait dispersion: New question front old patterns. Oikos 74, 159–164. doi: 10.2307/3545686
Wright, I. J., Reich, P. B., Westoby, M., Ackerly, D. D., Baruch, Z., Bongers, F., et al. (2004). The worldwide leaf economics spectrum. Nature 428, 821–827. doi: 10.1038/nature02403
Yan, E. R., Wang, X. H., and Zhou, W. (2008). Characteristics of litterfall in relation to soil nutrients in mature and degraded evergreen broad-leaved forests of Tiantong, east China. Chin. J. Plant Ecol. 32, 1–12.
Yang, X. D., Yan, E. R., Chang, S. X., Wang, X. H., Zhao, Y. T., and Shi, Q. R. (2014). Twig-leaf size relationship in woody plants vary intraspecifically along a soil moisture gradient. Acta Oecol. 60, 17–25. doi: 10.1016/j.actao.2014.07.004
Zhang, Q. F., Song, Y. C., and You, W. H. (1999). Relationship between plant community secondary succession and soil fertility in Tiantong, Zhejiang province. Acta Ecol. Sin. 19, 174–178.
Zhang, Q. Q., Zhou, L. L., Zhao, Y. T., Xu, M. S., and Yan, E. R. (2016). Litterfall dynamics of plants in a successional series of evergreen broad-leaved forests in Tiantong region, Zhejiang province. Chin. J. Ecol. 35, 290–299.
Zhang, R. Y., Li, Y. P., Ni, Y. L., Gui, X. J., Lian, J. Y., and Ye, W. H. (2019). Intraspecific variation of leaf functional traits along the vertical layer in a subtropical evergreen broad-leaved forest of Dinghushan. Biodivers. Sci. 27, 1279–1290. doi: 10.17520/biods.2019267
Zhong, Q. L., Liu, L. B., Xu, X., Yang, Y., Guo, Y. M., Xu, H. Y., et al. (2018). Variations of plant functional traits and adaptive strategy of woody species in a karst forest of central Guizhou province, southwestern China. Chin. J. Plant Ecol. 42, 562–572. doi: 10.17521/cjpe.2017.0270
Keywords: intraspecific trait variation, forest succession, overlapping species, life history strategy, subtropical forests
Citation: Liu L, Xia H, Quan X and Wang Y (2023) Plant trait-based life strategies of overlapping species vary in different succession stages of subtropical forests, Eastern China. Front. Ecol. Evol. 10:1103937. doi: 10.3389/fevo.2022.1103937
Received: 21 November 2022; Accepted: 13 December 2022;
Published: 04 January 2023.
Edited by:
Xiang Liu, Lanzhou University, ChinaReviewed by:
Zhonghua Zhang, Nanning Normal University, ChinaCopyright © 2023 Liu, Xia, Quan and Wang. This is an open-access article distributed under the terms of the Creative Commons Attribution License (CC BY). The use, distribution or reproduction in other forums is permitted, provided the original author(s) and the copyright owner(s) are credited and that the original publication in this journal is cited, in accordance with accepted academic practice. No use, distribution or reproduction is permitted which does not comply with these terms.
*Correspondence: Yunquan Wang, ✉ eXF3YW5nQHZpcC4xMjYuY29t
Disclaimer: All claims expressed in this article are solely those of the authors and do not necessarily represent those of their affiliated organizations, or those of the publisher, the editors and the reviewers. Any product that may be evaluated in this article or claim that may be made by its manufacturer is not guaranteed or endorsed by the publisher.
Research integrity at Frontiers
Learn more about the work of our research integrity team to safeguard the quality of each article we publish.