- 1Co-Innovation Center for Sustainable Forestry in Southern China, College of Biology and the Environment, Nanjing Forestry University, Nanjing, China
- 2Jiangsu Academy of Forestry Sciences, Nanjing, China
- 3Yancheng Coastal Wetland Ecosystem National Research Station, Yancheng, China
Arbuscular mycorrhizal (AM) fungi play a crucial role in carbon (C), nitrogen (N), and phosphorous (P) biogeochemical cycling. Therefore, it is essential to determine the seasonal responses of the AM fungal community to N addition to understanding better the ecological processes against a background of intensified N deposition. Based on an ongoing field simulation experiment with five N addition levels (0, 5, 10, 15, and 30 gN·m−2·a−1) in a 5-year-old poplar plantation at Dongtai Forest Farm in Yancheng, Jiangsu province, eastern China, soil physicochemical properties, the root colonization rate, and the rhizosphere soil AM fungal community diversity and composition in four seasons (summer, autumn, winter, and spring) were investigated. Meanwhile, the relationships between the characteristics of the AM fungal community and soil environmental factors were analyzed. High-throughput sequencing showed that the dominant genera in the poplar plantation were Glomus (average relative abundance 87.52%), Diversispora (9.62%), and Acaulospora (1.85%). The addition of N significantly increased the root colonization rate in spring. The diversity of the AM fungal community (Chao and Shannon indexes) was primarily affected by seasonal change rather than N addition, and the diversity in summer was significantly lower than in the other three seasons. Redundancy analysis showed that soil temperature, available P, total P, and pH significantly affected the structure of the AM fungal community. It can be concluded N addition primarily influenced the root colonization rate, whereas seasonal change had a notable effect on the AM fungal community diversity. Although seasonal change and N addition greatly influenced the composition, seasonal change exerted a more substantial effect than N addition. These results will improve our understanding of the underground ecological processes in poplar plantation ecosystems.
1. Introduction
Global nitrogen (N) deposition caused by anthropogenic activities is increasing dramatically (Penuelas et al., 2013). The atmospheric N deposition rates have exceeded 20 kg N·ha−1·a−1 in the forest ecosystems of central and eastern China (Liu et al., 2011). Intensive deposition of reactive N can induce soil nutrient imbalances, which can alter plant productivity, abundance, and ecosystem function (Bobbink et al., 2010), as well as the soil microbial community diversity and composition in forest ecosystems (Tian et al., 2017; Wang et al., 2018).
Arbuscular mycorrhizal (AM) fungi can form a symbiosis with most terrestrial plants, thereby improving nutrient absorption and stress tolerance. In return, host plants provide photosynthetic products for the growth of AM fungi (Smith and Read, 2008). Consequently, AM fungal associations link the below- and above-ground communities in ecosystems and play crucial roles in carbon (C), N, and phosphorous (P) biogeochemical cycling. The high N availability and soil acidification caused by N deposition can influence AM fungal communities, thereby altering the related soil ecological processes (Lilleskov et al., 2019). Despite this recognition, the effect of N deposition on AM fungal communities remains ambiguous and is the subject of intense scientific debate because previous studies have reported negative (Chen et al., 2017; Williams et al., 2017; Treseder et al., 2018), positive results (Egerton-Warburton et al., 2007), or no effects (Mueller and Bohannan, 2015; Maitra et al., 2021). These discrepancies could have been caused by differences in host plant and ecosystem types, soil nutrient contents (N and P), and N addition amounts and durations (Han et al., 2020). Therefore, it is critical to conduct more studies on this topic better to understand AM fungi’s roles in ecosystem function.
AM fungi belong to the new Glomeromycota phylum; there is one class, four orders, 11 families, 25 genera, and about 300 species in this phylum (Redecker et al., 2013). Different AM fungi have contrasting growth strategies, hyphae production, and nutrient absorption abilities (Chagnon et al., 2013). For example, species within the Gigaporaceae family tend to allocate more biomass to external hyphae than intraradical structures, whereas species of Glomeraceae display the opposite tendency (Maherali and Klironomos, 2007). More external hyphae mean more photosynthetic products are needed to support the growth of AM fungi and their hyphal networks. Thus, the AM fungal communities tend to shift from Gigasporaceae to Glomeraceae upon anthropogenic N enrichment and experimental fertilization (Treseder et al., 2018). Furthermore, the soil N/P ratio or P availability also affects the response of AM fungi to N addition. The high production of external hyphae always represents competitive soil exploration and high P solubilization. Soil P limitation stimulated by N addition would increase the abundance of Gigasporaceae members (Egerton-Warburton et al., 2007). These results indicated that different groups of AM fungi might have contrasting responses to N addition in various environments.
Seasonal change can influence AM fungi by modifying climatic factors and the C supply derived from host plants (Dumbrell et al., 2011; Maitra et al., 2021). Seasonal variations of the AM fungal community have been studied in arable, wetland, grassland, and forest habitats. Generally, the density of spores, external hyphal length, and root colonization rates were higher in summer, or the growing period, than in winter and early spring (Mandyam and Jumpponen, 2008). Meanwhile, other studies suggested that AM fungi were not affected by season (Santos-Gonzalez et al., 2007; Maitra et al., 2021). Traditionally, the temporal dynamics of AM fungal community have been described based on the morphological analysis of their characteristic structures of AM fungi. However, some AM fungal structures cannot be precisely identified through their morphologies. Therefore, the resulting compositions will likely differ from field AM fungal communities (Davison et al., 2012). By contrast, molecular techniques can overcome the limitations of the traditional method. Therefore, DNA-based and morphological techniques can be used in ecological studies of the AM fungal community (Vieira et al., 2018).
As a critical tree species for afforestation, poplar has been widely planted in China, especially in the eastern coastal area (Fang, 2008). This region suffers severe N deposition (Liu et al., 2011). Based on a long-term experiment with five N addition levels (0, 5, 10, 15, and 30 gN·m−2·a−1) in a poplar plantation in Dongtai Forest Farm, Jiangsu Province, researchers found changes in soil organic carbon, soil fauna, and the microbial community structure (Bian et al., 2019; Yu et al., 2021). However, the response of AM fungi to N addition in the poplar plantation remains unknown. This study sampled poplar roots and rhizosphere soils in four seasons (summer, autumn, winter, and spring) after 6 years of continuous N additions. The effects of N addition and seasonal change on AM fungi were examined by traditional root colonization identification and high-throughput sequencing. We tested two hypotheses: (1) N addition and seasonal change could alter the AM fungal colonization rate and AM fungal community diversity and composition, and (2) N addition and seasonal change-induced variations of soil physicochemical properties contribute to the variation in the AM fungal community.
2. Materials and methods
2.1. Site description and soil sampling
The study site is located at Dongtai Forest Farm in Yancheng, Jiangsu province, eastern China (E120° 49′, N32° 52′). The region experiences a subtropical northern monsoon climate. The mean annual precipitation is 1,050 mm, and the mean annual temperature is 14.6°C. The growing season extends from May to October, whereas the non-growth season is from November to April. The soil is classified as fluvisol, according to the Food and Agriculture Organization (FAO) of the United Nations (Ge et al., 2018).
The farm was reclaimed from the coastal wetlands by constructing coastal levees. Poplar is one of the main tree species planted on the farm, and the area of the poplar plantation covers approximately 2000 hm2. The N addition experiment started in May 2012 and involved five N levels in 5-year-old poplar plantations (Populus deltoides L. ‘35’). We selected three sites (25 × 190 m), with the distance between any two sites being >1 km. Each site was divided into five plots (25 × 30 m) with a 10 m wide buffer zone. Five N addition treatments were randomly applied to the plots: N0 (control), N1 (5 gN∙m−2∙a−1), N2 (10 gN∙m−2∙a−1), N3 (15 gN∙m−2∙a−1), and N4 (30 gN∙m−2∙a−1). Nitrogen in the form of NH4NO3 has been applied annually during the growing season. Fertilizer with different N concentrations was sprayed into the corresponding plots below the canopy, and the control plots received the same amount of deionized water.
Root and soil samples were collected in July 2018, October 2018, January 2019, and April 2019 (representing summer, autumn, winter, and spring, respectively). Fine roots (< 2 mm) and rhizosphere soils of five poplars with the same growth rate were randomly sampled from each plot. Five samples from the same plot were mixed homogeneously into one sample, resulting in 60 sub-samples. The rhizosphere soils were sieved with a 2 mm mesh and subdivided into two parts. The first part was air-dried for soil physicochemical properties analyses. The other part was stored at −80°C for DNA extraction. The fine roots were washed and cut into 1 cm fragments to determine the proportion of the root colonized by AM fungi.
2.2. Root colonization rate and soil physicochemical properties determination
The root colonization rate was measured using the grid-crossing method (McGonigle et al., 1990). The average soil moisture and temperature of the sampling month were measured using an automatic sensor. A glass electrode determined soil pH in a 1:5 soil/water (w/v) suspension. Soil total nitrogen (TN) and total carbon (TC) were measured using an elemental analyzer (2,400 II, Perkin Elmer, Waltham, MA, United States). Soil-available phosphorus (AP) was extracted with 0.5 M NaHCO3, and total phosphorus (TP) was determined after digestion by alkali fusion. AP and TP were quantified using the Mo-Sb colorimetric method. Ammonium nitrogen (NH4+-N) and nitrate nitrogen (NO3−-N) were quantified using indophenol blue colorimetry and ultraviolet spectrophotometry, respectively (Lu, 2000).
2.3. DNA extraction, amplification, and sequencing
Total DNA was extracted from 0.5 g of frozen soil using a FastDNA kit (MP, Santa Ana, CA, United States). AM fungal 18S rDNA for Miseq pyrosequencing was amplified using a two-step PCR. In the first PCR, the primer pair AML1 and AML2 were used (Lee et al., 2008). The obtained products were used as templates for the second PCR using primers AMV4-5NF and AMDGR (Van Geel et al., 2014). PCR reactions were performed in a 20 μl reaction mixture containing 4 μl of 5× FastPfu buffer, 0.8 μl of each primer, 2 μl of dNTPs, 0.4 μl of DNA polymerase, 0.2 μl of bovine serum albumin (BSA), and 10 ng of DNA template. The PCR condition consisted of an initial denaturation at 95°C for 3 min, 30 cycles of denaturation (95°C for 30 s, 55°C for 30 s, and 72°C for 45 s), and a final extension at 72°C for 10 min. The PCR products were purified using an Axy Prep DNA Gel Extraction Kit (Axygen Biosciences, Union City, CA, United States) and then quantified using a Quanti Fluor™-ST (Promega, San Luis Obispo, CA, USA). The purified PCR products were sequenced on a Miseq PE250 pyrosequencer (Illumina, San Diego, CA, United States) at Majorbio Bio-Pharm Technology Co., Ltd. (Shanghai, China).
2.4. Bioinformatics and statistical analysis
Raw sequence data were quality-filtered and spliced using FASTP (version 0.19.61) and FLASH (version 1.2.112) software, respectively. Briefly, reads with a quality score < 20, ambiguous nucleotides, reads shorter than 200 bp (excluding barcode and primer sequences), or lacking a complete barcode were removed and excluded from further analysis. The remaining sequences were then clustered into operational taxonomic units (OTUs) at 97% similarity using UPARSE (version 7.13). The taxonomic identification of OTUs was performed by consulting the MaarjAM database (version 814). The Chao and Shannon indexes were calculated after normalizing the read number to 10,561 reads (the minimum sequence number of all samples) in Mothur (Schloss et al., 2009), and the AM fungal community composition was assessed at the genus and species levels. The Bray-Curtis distances of community compositions (based on the OTU compositions) under five N additions and four seasons were calculated using nonmetric multidimensional scaling (NMDS), and the significance of community differences was assessed by analysis of similarities (ANOSIM) in the vegan package in R 3.6 (Dixon, 2003). Additionally, the relationships between the AM fungal community composition and soil physicochemical properties were evaluated using redundancy analysis (RDA). Only the environmental factors with a variance inflation factor (VIF) of less than 10 were retained in the RDA analysis. The significance of the correlation between environmental factors and the community composition was determined using a Monte Carlo test in R (Dixon, 2003). To identify the main and interactive effects of seasonal changes and N addition treatments on the root colonization rate and the AM fungal community diversity and composition, multivariate analysis of variance (MANOVA) was performed in SPSS 18.0 for Windows (IBM Corp., Armonk, NY, United States). Lastly, associations between AM fungal community characteristics (including the root colonization rate, diversity, and the relative abundance of AM fungal groups) and environmental factors were determined using Spearman.
3. Results
3.1. Root AM fungal colonization rate
N addition significantly affected the root colonization rate; however, the effect of seasonal change and their interaction was not significant (Figure 1). Generally, with the increase in the level of N addition, the root colonization rate showed an increasing trend. In spring, the root colonization rate under N0 treatment was significantly lower than that under N addition (p < 0.05).
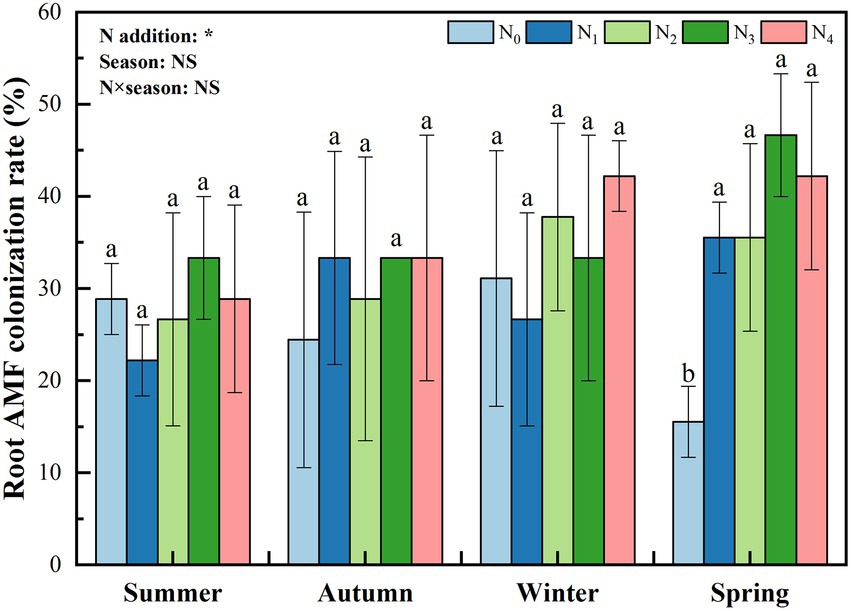
Figure 1. Seasonal change in poplar roots’ arbuscular mycorrhizal (AM) fungal colonization rate with different nitrogen additions. N0, N1, N2, N3, and N4 were 0, 5, 10, 15, and 30 gN·m−2·a−1, respectively. The values are the means of three replicates ± SD. Different lowercase letters within the same season indicate significant differences among nitrogen additions at the 0.05 level. Two-way ANOVA outputs are shown in the figure. NS, not significant; *, p < 0.05.
3.2. Arbuscular mycorrhizal fungal community diversity
A total of 1,264,310 high-quality sequences were identified from all 60 samples (ranging from 10,561 to 24,418 reads per sample; mean 21,068), clustered into 227 OTUs. Rarefaction curves suggested that the sequencing depth in this study was sufficient to reflect the community structure in the samples (Supplementary Figure 1). Seasonal changes significantly affected the Chao and Shannon indexes of the AM fungal community (p < 0.01, Table 1); however, the effect of N addition and their interaction was insignificant. Compared with summer, the Chao and Shannon indexes of AM fungal community were significantly higher in winter, spring, and autumn.
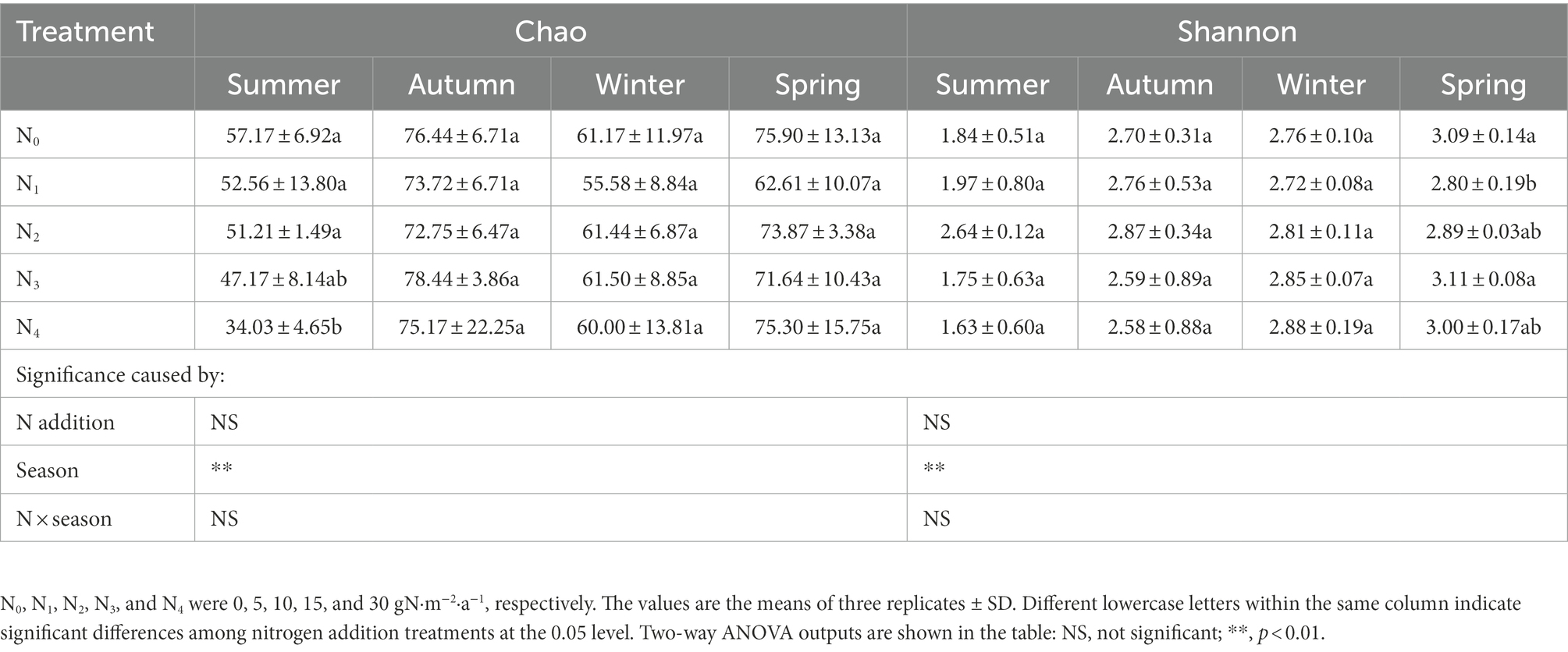
Table 1. Seasonal changes in the Chao and Shannon indexes of the AM fungal community in the poplar plantations with different nitrogen additions.
3.3. Arbuscular mycorrhizal fungal community composition
Analysis of Similarity (ANOSIM) revealed that the structures of the AM fungal community were significantly influenced by seasonal changes (R = 0.554, p = 0.001; Figure 2) and N treatments (R = 0.05, p = 0.031). ANOSIM analysis was also performed on AM fungal community structures among different N additions in the same season. We found that there were significant differences in AM fungal community structures between N additions in spring (R = 0.772, p = 0.001), winter (R = 0.704, p = 0.001), and summer (R = 0.387, p = 0.009), but no in autumn (R = 0.039, p = 0.358).
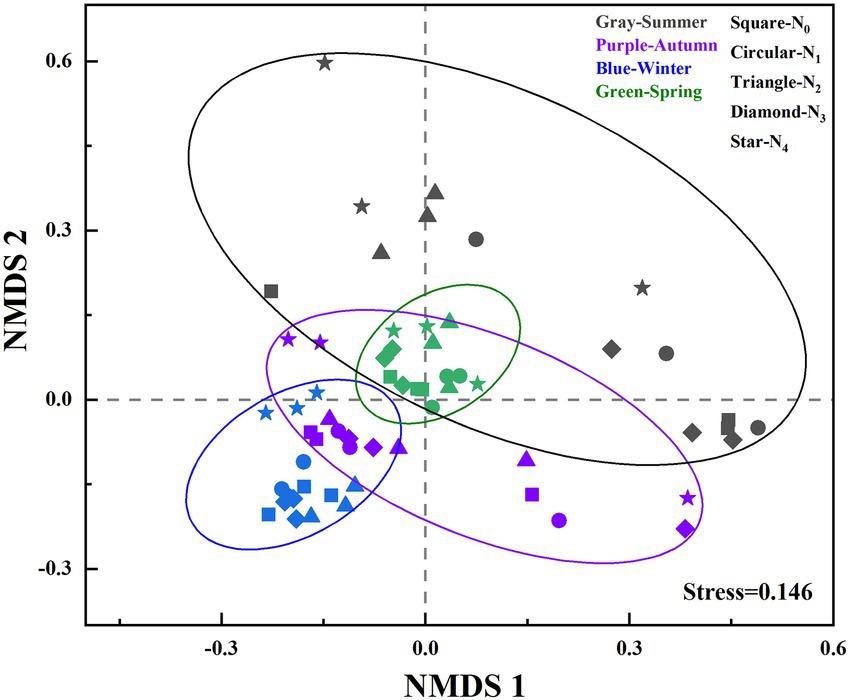
Figure 2. Nonmetric multidimensional scaling (NMDS) of seasonal changes in the soil AM fungal community compositions (based on Bray-Curtis distances and the presence of OTUs) in the poplar plantations under different nitrogen additions. N0, N1, N2, N3, and N4 were 0, 5, 10, 15, and 30 gN·m−2·a−1, respectively.
The AM fungal community in the poplar plantations was assigned to 4 orders, 7 families, 8 genera, and 43 species. Figure 3 and Supplementary Figure 2 present the AM fungal community compositions on the genus and species level, respectively. The genera Glomus (average relative abundance, 87.52%), Diversispora (9.62%), Acaulospora (1.85%), unclassified Glomeromycetes (0.61%), Scutellospora (0.22%), Archaeospora (0.12%), unclassified Diversisporaceae (0.05%), and Paraglomus (0.005%) were detected in the samples. The genus Glomus showed the highest abundance, consisting of 28 species. The genus Diversispora could be further divided into three main species: Diversispora VTX00060, Diversispora VTX00040, and unclassified Diversispora (Supplementary Figure 2).
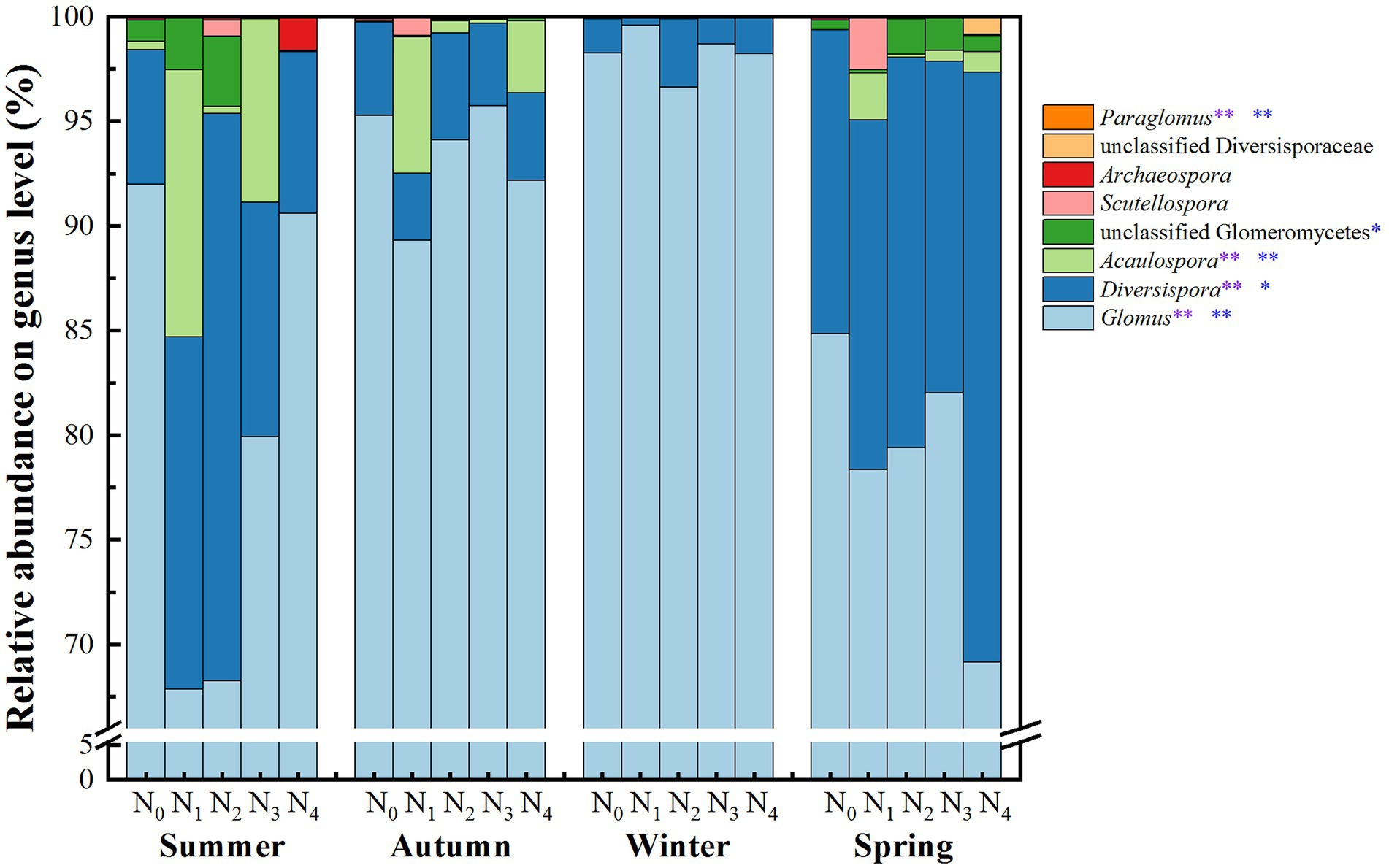
Figure 3. Average relative abundances of the AM fungal groups at the genus level in the poplar plantations under different nitrogen additions and seasons. N0, N1, N2, N3, and N4 were 0, 5, 10, 15, and 30 gN·m−2·a−1, respectively. The values are the means of three replicates. Two-way ANOVA outputs are shown in the figure: the purple * and ** indicate significant differences among different seasons at the 0.05 and 0.01 levels, respectively, and the blue * and ** indicate significant differences among nitrogen additions at the 0.05 and 0.01 levels, respectively.
The relative abundances of Glomus, Diversispora, Acaulospora, and Paraglomus were significantly affected by N addition treatments and seasonal changes. The abundances of Glomus in winter (98.3%) and autumn (93.3%) were higher than those in spring (78.8%) and summer (79.7%). In comparison, the abundances of Diversispora, Acaulospora, and Paraglomus in winter and autumn were lower than those in spring and summer (p < 0.05, Figure 3). Compared with N0 treatment, N addition significantly decreased the relative abundances of Glomus and had a trend to increase the relative abundances of Acaulospora and Diversispora. The relative abundances of Diversispora and Paraglomus under N2 treatment were higher than those under the other treatments, while the highest relative abundances of Glomus (92.6%) and Acaulospora (5.39%) were under N0 and N1 treatments, respectively.
3.4. Drivers of the root colonization rate and the AM fungal community diversity and composition
Soil pH, total P, C/N ratio, NO3−-N, and NH4+-N were significantly affected by seasonal change, N additions, and their interaction effects (Table 2). Soil total C, available P, moisture, and temperature were significantly influenced by seasonal change. Specifically, NO3−-N concentrations increased dramatically from N0 (6.37 mg·kg−1) to N4 (19.42 mg·kg−1) treatment (p < 0.01). Soil temperature decreased from summer (July 2018, 20.23°C) to winter (January 2019, 5.55°C) and then increased from winter to spring (April 2019, 14.64°C). For soil moisture, the order was winter > spring > summer >autumn.
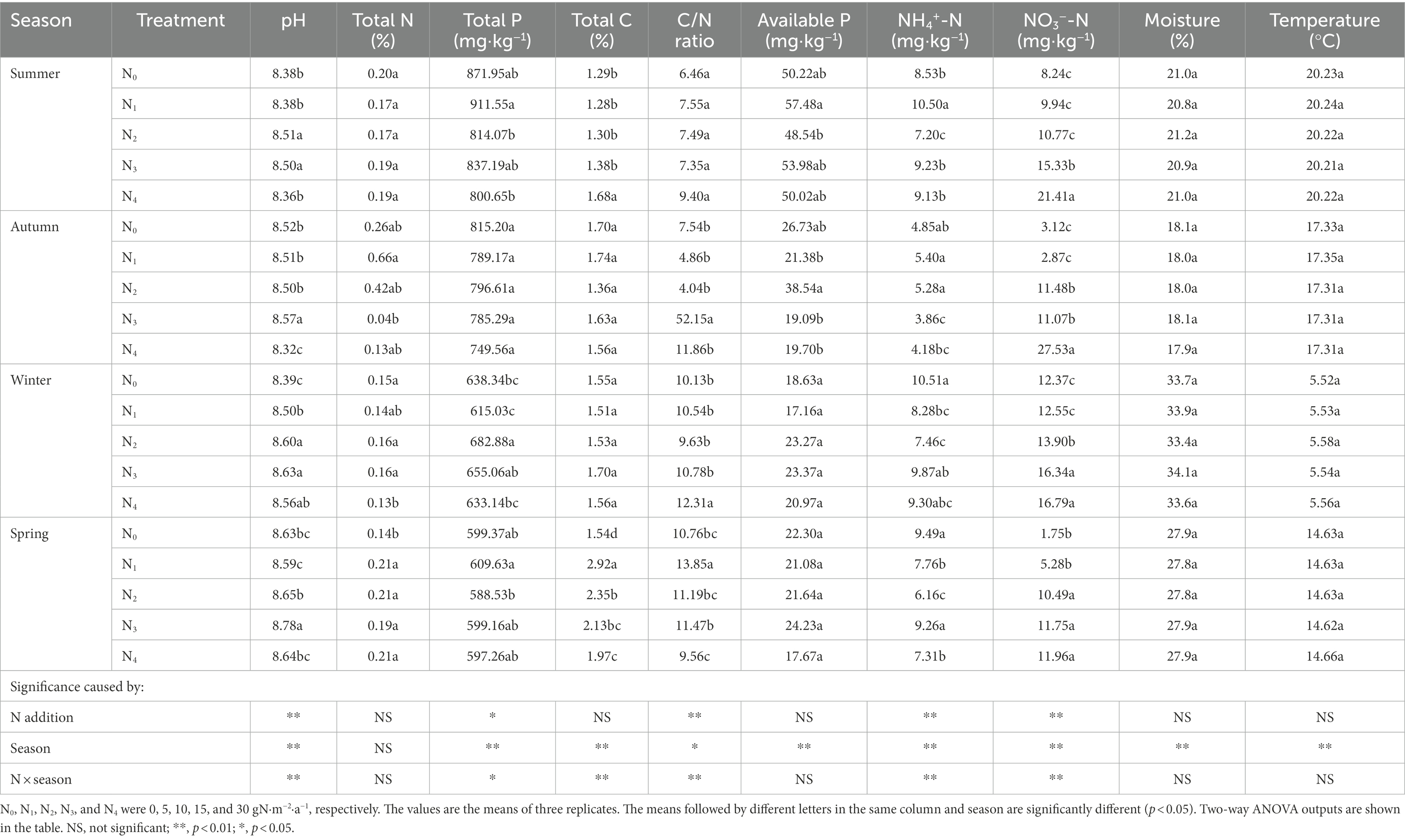
Table 2. Seasonal changes in soil physicochemical properties in the poplar plantations with different nitrogen additions.
The Chao index was largely associated with NH4+-N, available P, pH, NO3−-N, total P, and total C. The Shannon index was significantly associated with total P, pH, available P, temperature, and total C (p < 0.05, Table 3). NH4+-N (R = −0.477, p < 0.01) and total P (R = −0.494, p < 0.01) showed the highest correlation with the Chao index and the Shannon index, respectively. The root AM fungal colonization rate was significantly related to the C/N ratio, total C, pH, available P, and NO3−-N (p < 0.05, Table 3).
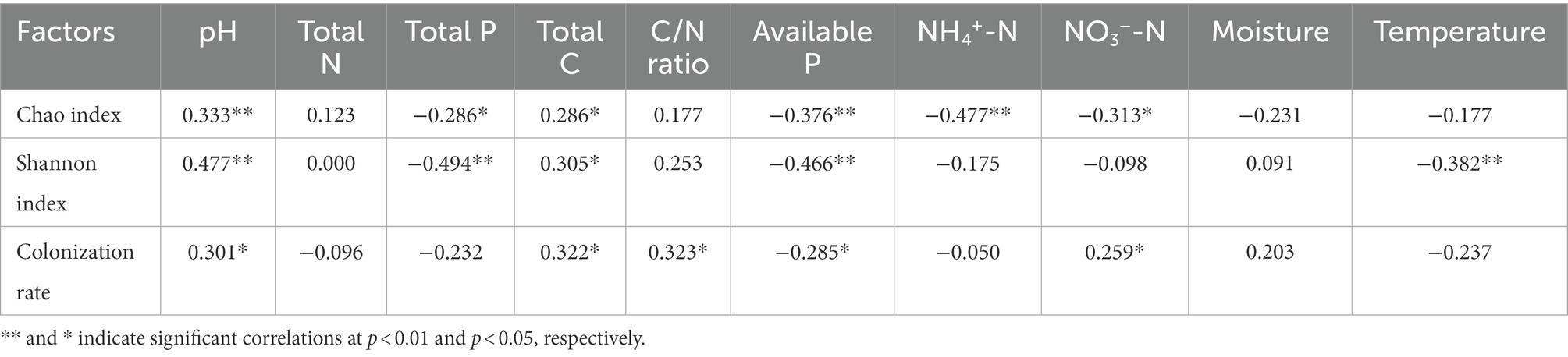
Table 3. Spearman correlation coefficients between the diversity of the AM fungal community and environmental factors (n = 60).
Nine environmental factors (pH, total C, total P, total N, available P, C/N ratio, NO3−-N, NH4+-N, and soil temperature) were chosen for the RDA analysis, which explained 35.45% (32.89% for the first two axes) of the total variances of the AM fungal community (p = 0.001, Figure 4). The Monte Carlo test indicated that the structure of the AM fungal community correlated with soil temperature, available P, total P, and pH (p < 0.05, Table 4). The correlation analysis between the relative abundance of the AM fungal genera and environmental factors showed that the relative abundances of Glomus, Diversispora, and Acaulospora correlated significantly with total N, NO3−-N, and temperature (Supplementary Table 1). The relative abundance of Scutellospora correlated positively with available P, total N, and temperature, while Archaeospora correlated significantly with total N, moisture, and NH4+-N (p < 0.05, Supplementary Table 1).
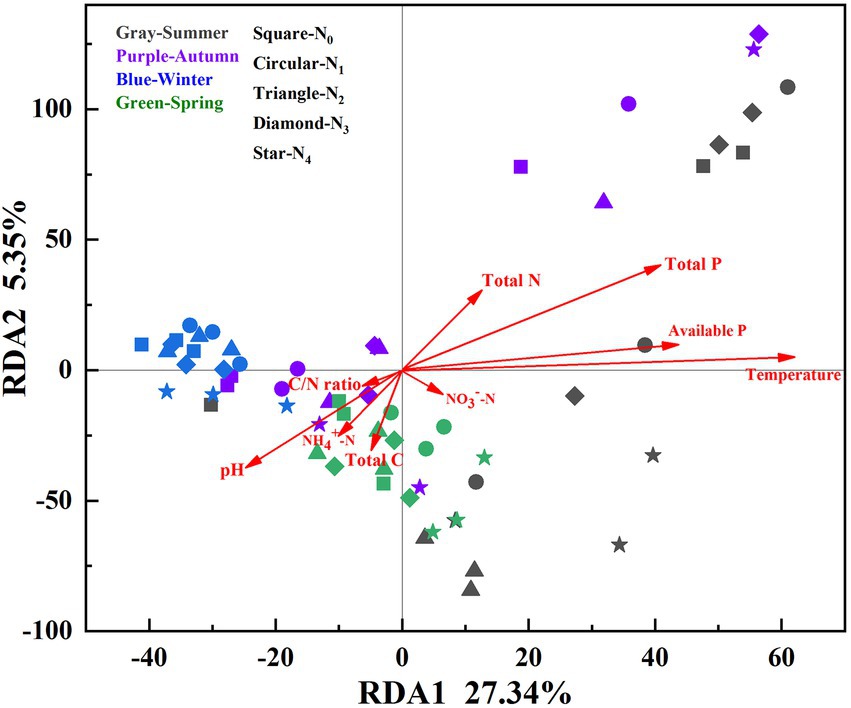
Figure 4. RDA for soil AM fungal community compositions (based on the species level) and environmental factors in the poplar plantations under different nitrogen additions in four seasons. N0, N1, N2, N3, and N4 were 0, 5, 10, 15, and 30 gN·m−2·a−1, respectively.

Table 4. Relationships between the composition of the arbuscular mycorrhizal (AM) fungal community and environmental factors revealed by Monte Carlo tests (n = 60).
4. Discussion
N addition positively affected the root AM fungal colonization rate in the poplar plantations. Specifically, root colonization rates under N additions were significantly higher than under N0 treatments in spring (Figure 1). The exchange of P and C is the fundamental function of the plant-AM fungal symbiosis; therefore, the soil P availability can strongly influence the response of AM fungi to N fertilization (Williams et al., 2017). In P-deficient soils, N fertilization-induced P limitation would stimulate plant C allocation to AM fungi to acquire sufficient P. However, in P-rich soil, plant dependency on AM fungi might be reduced, resulting in a decrease in C allocation to AM fungi (Johnson et al., 2013; Han et al., 2020). The soils under the poplar plantation in our study belong to the former category; thus, N addition increased the root AM fungal colonization rate. The correlation analysis also showed that the colonization rate correlated negatively with soil available P (p < 0.05, Table 3).
Seasonal variation played a notable role in the diversity of the AM fungal community. AM fungi must establish symbiosis with living plant roots to complete their life history; therefore, some studies found strong relationships between the phenology of plants and their mycorrhizal responsiveness. AM fungal characteristics were higher in the growing period than in the dormant season (Mandyam and Jumpponen, 2008). However, in the present study, the Chao and Shannon indexes were significantly higher in autumn, spring, and winter than in summer (Table 1). They tended to be negatively correlated with soil temperature (Table 3). Higher diversity in the colder seasons probably resulted from more even competition for limited C availability among AM fungal species (Dumbrell et al., 2011). In addition, poplar can form associations with AM fungi and ectomycorrhizal (EM) fungi simultaneously (Neville et al., 2002), and increased colonization by EM fungi in summer might influence AM fungal growth.
Although N addition and season influenced the AM fungal community compositions, seasonal change exerted a more substantial effect than N addition (Figure 2). Dumbrell et al. (2011) observed distinct AM fungal compositions in the cooler and warmer seasons. Similarly, the AM fungal assemblages in summer and winter were completely separate in the NMDS plots (Figure 2). The RDA results showed that the AM fungal community composition correlated significantly with temperature (Table 4). The mechanisms of the effect of temperature on the AM fungal communities can be classified as either indirect, by altering soil nutrient availability and host plants’ C allocation, or direct, by affecting AM fungal growth (Cotton, 2018). The RDA results also showed that the AM fungal community composition was greatly influenced by available P, total P, and pH (Table 4). N addition and season directly affected soil pH, P, and N availability (Table 2). Consequently, the effects of N addition and season on the AM fungal community composition were partially mediated through the soil (Zheng et al., 2014; Ji et al., 2021). Moreover, previous studies reported an indirect plant-mediated effect of N addition on AM fungal community composition in grassland ecosystems (Liu et al., 2012). In the poplar plantation ecosystem, nine environmental factors chosen for the RDA analysis explained 35.45% of the total variances of AM fungal community. Therefore, other environmental factors that affected the AMF community structure were not considered, e.g., the composition and diversity of the plant community. In future research, their impacts on the AM fungal community could be further investigated.
Generally, the AM fungal communities in poplar plantations were assigned to 4 orders, 7 families, 8 genera, and 43 species using high-throughput sequencing. The dominant genera were Glomus (average relative abundance 87.52%), Diversispora (9.62%), and Acaulospora (1.85%) (Figure 3). Using molecular techniques, former studies examined the structure of AM fungal communities in some forest ecosystems. In a subtropical forest, the dominant AM fungal groups across three seasons were Glomeraceae (78.82%), Archaesporaceae (11.06%), Claroideoglomeraceae (3.49%), Gigasporaceae (3.33%), Acaulosporaceae (1.61%), and Diversisporaceae (1.60%) (Maitra et al., 2021). In a rainforest, the AMF community was dominated by Glomus (96.72%), Acaulospora (2.42%), and Scutellospora (0.63%) (Pereira et al., 2022). Similarly, the genera Glomus, Diversispora, Scutellospora, and Paraglomus accounted for more than 95% of the sequences in a natural mixed broadleaf-conifer forest (Ji et al., 2021). Therefore, the dominant groups of the AM fungal community in different forest ecosystems vary. The study of the structure of the AM fungal community in the ecosystem of a poplar plantation can provide references for the exploration of their function.
Furthermore, in this study, N addition and season influenced the relative abundances of the dominant AM fungal groups (Figure 3). Compared with N0 treatment, N addition significantly decreased the relative abundances of Glomus, and had a trend toward increasing the relative abundances of Diversispora and Acaulospora, as demonstrated in previous investigations (Liu et al., 2015; Chen et al., 2017). The AM fungal community structure shifts were related to the competitive dynamics among AM fungi, changes in environmental conditions, or both (Dumbrell et al., 2011). Fertilization has been shown to have significant effects on the AM fungal population. For example, suppose the host plant reduces the allocation of C to the mycorrhiza under N addition. In that case, the intensified competition among AM fungi might result in a dominance of the AMF taxa, which can adapt to environments rich in N and limited in C (Johnson et al., 2015).
Moreover, because different AM fungi have contrasting soil exploration and P solubilization abilities, soil P availability affected the dependence of host plants on mycorrhizae (Treseder et al., 2018). The abundances of Glomus in winter and autumn were higher than in spring and summer, while the abundances of Diversispora and Acaulospora showed an opposite trend. The genus Glomus possessed a high sporulation rate and colonization ability via spore dispersal or mycelium fragments (Daniell et al., 2001); therefore, they were more likely to survive in relatively severe winter and autumn conditions.
5. Conclusion
In this study, we analyzed the seasonal changes of the root AM fungal colonization rate after six-year N addition in poplar using traditional morphological identification. We also detected the composition and diversity of AM fungal community in the rhizosphere soil using high-throughput sequencing. Our results showed that N addition primarily influenced the root colonization rate. In contrast, seasonal change had a notable effect on the Chao and Shannon indexes of the AM fungal community. Meanwhile, both the seasonal change and N addition impacted the composition of the AM fungal community. Soil temperature, available P, total P, and pH were the main drivers for the seasonal dynamics of AM fungal community in this poplar plantation.
Data availability statement
The datasets presented in this study can be found in online repositories. The names of the repository/repositories and accession number(s) can be found below: NCBI; SAMN31857539- SAMN31857598.
Author contributions
SP, ZG, and LM: designed the project and provided the funding. SP and MB: collected the samples and conducted the laboratory analysis. SP, WX, and ZG: performed the bioinformatics and statistical analysis. SP: wrote the original draft of the paper. LM: revised the manuscript and contributed to the conception. All authors have read and approved the final manuscript.
Funding
This work was supported by the Special Fund Project for Technology Innovation on Carbon Peak Carbon-neutral in 2021, Jiangsu Province (BE2022305), the Key Research Development Program of Jiangsu Province (BE2022792), the National Natural Science Foundation of China (41601254, 41877039, and 32271712), the Strategic Priority Research Program of the Chinese Academy of Sciences (XDB31000000), and the Jiangsu Forestry Science and Technology Innovation and Promotion Program (LYKJ [2021] 25).
Acknowledgments
We are very grateful to the editors, reviewers and experts who made suggestions for the manuscript.
Conflict of interest
The authors declare that the research was conducted in the absence of any commercial or financial relationships that could be construed as a potential conflict of interest.
Publisher’s note
All claims expressed in this article are solely those of the authors and do not necessarily represent those of their affiliated organizations, or those of the publisher, the editors and the reviewers. Any product that may be evaluated in this article, or claim that may be made by its manufacturer, is not guaranteed or endorsed by the publisher.
Supplementary material
The Supplementary material for this article can be found online at: https://www.frontiersin.org/articles/10.3389/fevo.2022.1101698/full#supplementary-material
Footnotes
References
Bian, H. X., Geng, Q. H., Xiao, H. R., Shen, C. Q., Li, Q., Cheng, X. L., et al. (2019). Fine root biomass mediates soil fauna community in response to nitrogen addition in ppoplar plantations (Populus deltoids) on the east coast of China. Forests 10:122. doi: 10.3390/f10020122
Bobbink, R., Hicks, K., Galloway, J., Spranger, T., Alkemade, R., Ashmore, M., et al. (2010). Global assessment of nitrogen deposition effects on terrestrial plant diversity: a synthesis. Ecol. Appl. 20, 30–59. doi: 10.1890/08-1140.1
Chagnon, P. L., Bradley, R. L., Maherali, H., and Klironomos, J. N. (2013). A trait-based framework to understand life history of mycorrhizal fungi. Trends Plant Sci. 18, 484–491. doi: 10.1016/j.tplants.2013.05.001
Chen, Y. L., Xu, Z. W., Xu, T. L., Veresoglou, S. D., Yang, G. W., and Chen, B. D. (2017). Nitrogen deposition and precipitation induced phylogenetic clustering of arbuscular mycorrhizal fungal communities. Soil Biol. Biochem. 115, 233–242. doi: 10.1016/j.soilbio.2017.08.024
Cotton, T. E. A. (2018). Arbuscular mycorrhizal fungal communities and global change: an uncertain future. FEMS Microbiol. Ecol. 94:fiy179. doi: 10.1093/femsec/fiy179
Daniell, T. J., Husband, R., Fitter, A. H., and Young, J. P. W. (2001). Molecular diversity of arbuscular mycorrhizal fungi colonising arable crops. FEMS Microbiol. Ecol. 36, 203–209. doi: 10.1016/S0168-6496(01)00134-9
Davison, J., Őpik, M., Zobel, M., Vasar, M., Metsis, M., and Moora, M. (2012). Communities of arbuscular mycorrhizal fungi detected in forest soil are spatially heterogeneous but do not vary throughout the growing season. PLoS One 7:e41938. doi: 10.1371/journal.pone.0041938
Dixon, P. (2003). Vegan, a package of R functions for community ecology. J. Veg. Sci. 14, 927–930. doi: 10.1111/j.1654-1103.2003.tb02228.x
Dumbrell, A. J., Ashton, P. D., Aziz, N., Feng, G., Nelson, M., Dytham, C., et al. (2011). Distinct seasonal assemblages of arbuscular mycorrhizal fungi revealed by massively parallel pyrosequencing. New Phytol. 190, 794–804. doi: 10.1111/j.1469-8137.2010.03636.x
Egerton-Warburton, L. M., Johnson, N. C., and Allen, E. B. (2007). Mycorrhizal community dynamics following nitrogen fertilization: a cross-site test in five grasslands. Ecol. Monogr. 77, 527–544. doi: 10.1890/06-1772.1
Fang, S. Z. (2008). Silviculture of poplar plantation in China: a review. Chin. J. Appl. Ecol. 19, 2308–2316. (in Chinese). doi: 10.13287/j.1001-9332.2008.0396
Ge, Z. W., Fang, S. Y., Chen, H. Y. H., Zhu, R. W., Peng, S. L., and Ruan, H. H. (2018). Soil aggregation and organic carbon dynamics in poplar plantations. Forests 9:508. doi: 10.3390/f9090508
Han, Y. F., Feng, J. G., Han, M. G., and Zhu, B. (2020). Responses of arbuscular mycorrhizal fungi to nitrogen addition: a meta-analysis. Glob. Chang. Biol. 26, 7229–7241. doi: 10.1111/gcb.15369
Ji, L., Yang, Y. C., Yang, N., Khan, A., and Yang, L. X. (2021). Seasonal variation of diversity and co-occurrence patterns of arbuscular mycorrhizal fungal communities in mixed broadleaf-conifer forests. Appl. Soil Ecol. 158:103782. doi: 10.1016/j.apsoil.2020.103782
Johnson, N. C., Angelard, C., Sanders, I. R., and Kiers, E. T. (2013). Predicting community and ecosystem outcomes of mycorrhizal responses to global change. Ecol. Lett. 16, 140–153. doi: 10.1111/ele.12085
Johnson, N. C., Wilson, G. W. T., Wilson, J. A., Miller, R. M., and Bowker, M. A. (2015). Mycorrhizal phenotypes and the law of the minimum. New Phytol. 205, 1473–1484. doi: 10.1111/nph.13172
Lee, J., Lee, S., and Young, J. P. W. (2008). Improved PCR primers for the detection and identification of arbuscular mycorrhizal fungi. FEMS Microbiol. Ecol. 65, 339–349. doi: 10.1111/j.1574-6941.2008.00531.x
Lilleskov, E. A., Kuyper, T. W., Bidartondo, M. I., and Hobbie, E. A. (2019). Atmospheric nitrogen deposition impacts on the structure and function of forest mycorrhizal communities: a review. Environ. Pollut. 246, 148–162. doi: 10.1016/j.envpol.2018.11.074
Liu, X. J., Duan, L., Mo, J. M., Du, E. Z., Shen, J. L., Lu, X. K., et al. (2011). Nitrogen deposition and its ecological impact in China: An overview. Environ. Pollut. 159, 2251–2264. doi: 10.1016/j.envpol.2010.08.002
Liu, Y. J., Johnson, N. C., Mao, L., Shi, G. X., Jiang, S. J., Ma, X. J., et al. (2015). Phylogenetic structure of arbuscular mycorrhizal community shifts in response to increasing soil fertility. Soil Biol. Biochem. 89, 196–205. doi: 10.1016/j.soilbio.2015.07.007
Liu, Y. J., Shi, G. X., Mao, L., Cheng, G., Jiang, S. J., Ma, X. J., et al. (2012). Direct and indirect influences of 8 yr of nitrogen and phosphorus fertilization on Glomeromycota in an alpine meadow ecosystem. New Phytol. 194, 523–535. doi: 10.1111/j.1469-8137.2012.04050.x
Lu, R. K. (2000). Chemical Analyzing Method on Soil Agriculture. Beijing: China Agriculture Science and Technology Press.
Maherali, H., and Klironomos, J. N. (2007). Influence of phylogeny on fungal community assembly and ecosystem functioning. Science 316, 1746–1748. doi: 10.1126/science.1143082
Maitra, P., Zheng, Y., Wang, Y. L., Mandal, D., Lu, P. P., Gao, C., et al. (2021). Phosphorus fertilization rather than nitrogen fertilization, growing season and plant successional stage structures arbuscular mycorrhizal fungal community in a subtropical forest. Biol. Fertil. Soils 57, 685–697. doi: 10.1007/s00374-021-01554-4
Mandyam, K., and Jumpponen, A. (2008). Seasonal and temporal dynamics of arbuscular mycorrhizal and dark septate endophytic fungi in a tallgrass prairie ecosystem are minimally affected by nitrogen enrichment. Mycorrhiza 18, 145–155. doi: 10.1007/s00572-008-0165-6
McGonigle, T. P., Miller, M. H., Evans, D. G., Fairchild, G. L., and Swan, J. A. (1990). A new method which gives an objective measure of colonization of roots by vesicular-arbuscular mycorrhizal fungi. New Phytol. 115, 495–501. doi: 10.1111/j.1469-8137.1990.tb00476.x
Mueller, R. C., and Bohannan, B. J. (2015). Shifts in the phylogenetic structure of arbuscular mycorrhizal fungi in response to experimental nitrogen and carbon dioxide additions. Oecologia 179, 175–185. doi: 10.1007/s00442-015-3337-z
Neville, J., Tessier, J. L., Morrison, I., Scarratt, J., Canning, B., and Klironomos, J. N. (2002). Soil depth distribution of ecto- and arbuscular mycorrhizal fungi associated with Populus tremuloides within a 3-year-old boreal forest clear-cut. Appl. Soil Ecol. 19, 209–216. doi: 10.1016/S0929-1393(01)00193-7
Penuelas, J., Poulter, B., Sardans, J., Ciais, P., van der Velde, M., Bopp, L., et al. (2013). Human-induced nitrogen-phosphorus imbalances alter natural and managed ecosystems across the globe. Nat. Commun. 4:2934. doi: 10.1038/ncomms3934
Pereira, C. M. R., López-García, A., Maia, L. C., Frøslev, T. G., Kjøller, R., and Rosendahl, S. (2022). Arbuscular mycorrhizal fungal communities of pristine rainforests and adjacent sugarcane fields recruit from different species pools. Soil Biol. Biochem. 167:108585. doi: 10.1016/j.soilbio.2022.108585
Redecker, D., Schussler, A., Stockinger, H., Sturmer, S. L., Morton, J. B., and Walker, C. (2013). An evidence-based consensus for the classification of arbuscular mycorrhizal fungi (Glomeromycota). Mycorrhiza 23, 515–531. doi: 10.1007/s00572-013-0486-y
Santos-Gonzalez, J. C., Finlay, R. D., and Tehler, A. (2007). Seasonal dynamics of arbuscular mycorrhizal fungal communities in roots in a seminatural grassland. Appl. Environ. Microbiol. 73, 5613–5623. doi: 10.1128/AEM.00262-07
Schloss, P. D., Westcott, S. L., Ryabin, T., Hall, J. R., Hartmann, M., Hollister, E. B., et al. (2009). Introducing mothur: open-source, platform-independent, community-supported software for describing and comparing microbial communities. Appl. Environ. Microbiol. 75, 7537–7541. doi: 10.1128/AEM.01541-09
Smith, S. E.and Read, D. J. (2008). Mycorrhizal symbiosis (Third edition). New York: Academic Press.
Tian, D., Jiang, L., Ma, S., Fang, W. J., Schmid, B., Xu, L. C., et al. (2017). Effects of nitrogen deposition on soil microbial communities in temperate and subtropical forests in China. Sci. Total Environ. 607-608, 1367–1375. doi: 10.1016/j.scitotenv.2017.06.057
Treseder, K. K., Allen, E. B., Egerton-Warburton, L. M., Hart, M. M., Klironomos, J. N., Maherali, H., et al. (2018). Arbuscular mycorrhizal fungi as mediators of ecosystem responses to nitrogen deposition: a trait-based predictive framework. J. Ecol. 106, 480–489. doi: 10.1111/1365-2745.12919
Van Geel, M., Busschaert, P., Honnay, O., and Lievens, B. (2014). Evaluation of six primer pairs targeting the nuclear rRNA operon for characterization of arbuscular mycorrhizal fungal (AMF) communities using 454 pyrosequencing. J. Microbiol. Methods 106, 93–100. doi: 10.1016/j.mimet.2014.08.006
Vieira, C. K., Marascalchi, M. N., Rodrigues, A. V., de Armas, R. D., and Sturmer, S. L. (2018). Morphological and molecular diversity of arbuscular mycorrhizal fungi in revegetated iron-mining site has the same magnitude of adjacent pristine ecosystems. J. Environ. Sci. 67, 330–343. doi: 10.1016/j.jes.2017.08.019
Wang, C., Lu, X. K., Mori, T., Mao, Q. G., Zhou, K. J., Zhou, G. Y., et al. (2018). Responses of soil microbial community to continuous experimental nitrogen additions for 13 years in a nitrogen-rich tropical forest. Soil Biol. Biochem. 121, 103–112. doi: 10.1016/j.soilbio.2018.03.009
Williams, A., Manoharan, L., Rosenstock, N. P., Olsson, P. A., and Hedlund, K. (2017). Long-term agricultural fertilization alters arbuscular mycorrhizal fungal community composition and barley (Hordeum vulgare) mycorrhizal carbon and phosphorus exchange. New Phytol. 213, 874–885. doi: 10.1111/nph.14196
Yu, X. Y., Zhu, Y. J., Wang, B., Liu, D., Bai, H., Jin, L., et al. (2021). Effects of nitrogen addition on rhizospheric soil microbial communities of poplar plantations at different ages. For. Ecol. Manag. 494:119328. doi: 10.1016/j.foreco.2021.119328
Keywords: nitrogen deposition, arbuscular mycorrhizal fungi, composition and diversity, season, plantation, eastern China
Citation: Peng S, Ban M, Xing W, Ge Z and Mao L (2022) Effects of nitrogen addition and seasonal change on arbuscular mycorrhizal fungi community diversity in a poplar plantation. Front. Ecol. Evol. 10:1101698. doi: 10.3389/fevo.2022.1101698
Edited by:
Taimoor Hassan Farooq, Central South University of Forestry and Technology, ChinaReviewed by:
Babar Iqbal, Jiangsu University, ChinaMuhammad Haroon U. Rashid, University of Agriculture, Pakistan
Copyright © 2022 Peng, Ban, Xing, Ge and Mao. This is an open-access article distributed under the terms of the Creative Commons Attribution License (CC BY). The use, distribution or reproduction in other forums is permitted, provided the original author(s) and the copyright owner(s) are credited and that the original publication in this journal is cited, in accordance with accepted academic practice. No use, distribution or reproduction is permitted which does not comply with these terms.
*Correspondence: Lingfeng Mao, ✉ maolingfeng2008@163.com