- 1Natural Resources Institute, Texas A&M University, College Station, TX, United States
- 2Department of Natural Resources and Environmental Science, University of Nevada, Reno, NV, United States
- 3Nevada Department of Wildlife, Reno, NV, United States
- 4Montana Fish, Wildlife and Parks, Helena, MT, United States
Selection of forage and habitats is driven by nutritional needs of individuals. Some species may sacrifice nutritional quality of forage for the mother in favor of safety of offspring (risk-averse strategy), immediately following parturition. We studied diet quality and forage selection by bighorn sheep before and following parturition to determine how nutritional demands associated with rearing offspring influenced forage acquisition. We used desert bighorn sheep, Ovis canadensis nelsoni, to investigate that potential tradeoff. We captured and radio-collared female bighorn sheep from 2016 to 2018. We used vaginal implant transmitters (VIT)s in pregnant females to identify parturition and to capture and radio-collar neonates to monitor survival of young. We collected fecal samples throughout the breeding season and throughout the year to understand diet quality and composition throughout those temporal periods. We determined diet quality and composition for pre-parturient females, females provisioning offspring, females that lost offspring, and non-pregnant individuals using fecal nitrogen and DNA metabarcoding analyses. Additionally, we compared the diet quality and composition of offspring and adult females during the spring, as well as summer and winter months. Our results indicated differences in diet quality between individuals provisioning offspring and those whose offspring had died. Females that were provisioning dependent young had lower quality diets than those that lost their offspring. Diet composition among those groups was also markedly different; females that had lost an offspring had a more diverse diet than did females with dependent young. Diet quality differed among seasons, wherein offspring and adult females had higher quality diets during the spring months, with decreasing quality as the year progressed. Diet diversity was similar across seasons, although spring months tended to be most diverse. Our results support tradeoffs associated with risk-averse strategies made by adult females associated with parturition. Nutritional quality of forage was linked to provisioning status, indicating that females were trading diet quality for safety of offspring, but those females whose offspring had died selected high quality forages. Those results help explain habitat selection observed in mountain ungulates around parturition and provide further insight into the evolutionary processes and adaptive significance exhibited by those specialized artiodactyls.
Introduction
Nutritional ecology of large mammals has been of primary concern for scientists trying to understand the underlying drivers of population dynamics. Selection of forages to support reproduction is an important component of how individuals interact with their environment (Barboza et al., 2009; Parker et al., 2009). Nutritional interactions are present at all levels of an ecosystem and result in the population characteristics we observe in real time (Parker et al., 2009). For instance, individuals that obtain high-quality nutrition from their food, especially when growing, often are in better condition and obtain larger body size than those individuals that consume lower quality forage (Cook et al., 2004; Monteith et al., 2009; Parker et al., 2009; Tollefson et al., 2010; Jackson et al., 2021). Individuals on a higher nutritional plane are more likely to survive, produce more offspring during their lifetime and, thus, exhibit high reproductive fitness (Stewart et al., 2005; Parker et al., 2009; Stephenson et al., 2020). Furthermore, individuals that have access to better nutrition during portions of the year and have higher body condition are better able to survive prolonged periods of low-quality forage or harsh climatic conditions than those with lesser nutritional resources (Mautz, 1978; Stewart et al., 2005; Monteith et al., 2013; Monteith K. L. et al., 2014).
Selection of high-quality forage is dependent upon environmental constraints in the region, but selection of forages also varies among individuals in the population. Over long temporal scales, seasonal variation in availability and quality of forage become linked with life-history characteristics of animals inhabiting the region. For instance, timing of parturition among large mammals has evolved to coincide with the highest available forage quality to meet the metabolic needs of lactation (Bowyer, 1991; Long et al., 2016; Neumann et al., 2020). Furthermore, ungulates often select high-quality forages, usually early growth of forbs and grasses during spring and early summer but switch to browsing on shrubs during autumn and winter months, when vegetation quality is often lower and forage is limited (Boeker et al., 1972; Collins and Urness, 1983; Miller and Gaud, 1989; Beck and Peek, 2005a). Seasonal shifts in forage selection allow individuals to maintain better body condition, produce larger offspring, and higher recruitment rates, resulting in increased reproductive fitness (Parker et al., 2009).
Selection of forage often varies with sex (Bleich et al., 1997 2019; Barboza and Bowyer, 2000, 2001), reproductive status (Clutton-Brock et al., 1982; Cook et al., 2013), and population density (Stewart et al., 2011; Bowyer et al., 2014, 2020). Intraspecific competition leads to much of the variation we observe among individuals in selection of nutritional resources across the landscape. Polygynous ungulates exhibit strong sexual dimorphism and often exhibit a dramatic difference in body size between males and females. As a result, dietary requirements often differ between sexes and are reflected in differences in selection of forages and often linked to reproductive success (Barboza and Bowyer, 2000, 2001; Schroeder et al., 2010). Males are usually larger bodied than females, have larger rumens, fewer metabolic demands associated with reproduction, may thrive on lower quality forage than that required by reproductive females, and have no contribution to survival of offspring beyond mating (Barboza and Bowyer, 2000). Furthermore, males can use habitats with greater predation risk than females with dependent young (Bleich et al., 1997; Barboza and Bowyer, 2000). In addition to meeting nutritional requirements associated with gestation, following parturition females must protect and provision their offspring until weaning (Oftedal, 1985; Robbins, 1993; Barboza et al., 2009). High-quality diets acquired by parturient females, or those provisioning dependent young, result in larger offspring with higher survival and recruitment (Keech et al., 2000; Monteith et al., 2009; Heffelfinger et al., 2018). Lactating females often lose body condition, as a result of increased protein and energy demands, while non-reproductive females are able to regain somatic reserves during similar time periods (Holt et al., 1992; Barboza and Parker, 2008), likely resulting in selection of different forages by females of varying reproductive status.
While provisioning young, some species may trade nutrition of the mother for greater safety and higher survival of offspring, thereby exhibiting risk-averse behavior (Festa-Bianchet, 1988; Berger, 1991; Bleich et al., 1997; Long et al., 2009). Females making this tradeoff can only do so for a limited period because metabolic needs during lactation are higher than at other times of the year and must be met to ensure offspring obtain the appropriate amount of nutrients during growth (Barboza et al., 2009). Therefore, we expect the degree of those nutritional tradeoffs to decline as juveniles grow and females exhaust somatic reserves obtained prior to parturition. This tradeoff does not necessarily indicate that females select the highest quality diets available, but rather they select vegetation that is sufficient to overcome the constraints associated with gestation and lactation, while also enhancing safety of offspring. Additionally, if females can access high quality and abundant vegetation while simultaneously selecting habitat types that increase the safety and survival of offspring (Pierce et al., 2004; Heffelfinger et al., 2020), then a tradeoff would not be expected to occur.
Quantifying diet quality and composition, at multiple states of reproduction, is useful to understanding habitat selection and life-history strategies of wild ungulates. Furthermore, this information can positively influence the conservation of target species through habitat management. For instance, identifying how diet content and quality adjust across different life-history strategies can be used to improve the reproductive fitness of individuals by focusing habitat management on those forage species that best improve an individuals’ nutritional condition. Additionally, this information can be used to ensure that translocation sites have similar vegetative characteristics to support individuals throughout different reproductive periods, therefore, increasing the likelihood of success of the translocation through high survival and the recruitment of offspring.
We used a population of desert bighorn sheep (Ovis canadensis nelsoni) to identify changes in diet composition and quality throughout the year, especially around reproduction (prior to parturition, following parturition and while lactating) and for females following death of their offspring. Our objectives were to (1) identify diet composition and quality across seasons and between adult females and their offspring and (2) identify diet composition and quality across the parturition season based on timing of parturition and offspring provisioning status. We hypothesized that if females with offspring traded nutrition for safety of offspring, then those females without offspring would have higher quality diets than those with dependent young. Additionally, we hypothesized that females that were not provisioning offspring would have higher diet diversity compared to those with dependent young, because they likely are able to exploit a greater diversity of habitat types than mothers with dependent young.
Materials and methods
Study site
Our study was conducted on Lone Mountain (38○ 2’N, 117○ 31’W) and the Weepah Hills (37○ 56’N, 117○ 30’W), which are located about 25 km west of Tonopah, Nye Co., Nevada (Figure 1). The area ranges from 1,400 to 2,800 m in elevation and is occupied by a variety of plant taxa that are representative of Great Basin and Mojave Desert ecosystems. Low elevations were primarily comprised of Wyoming sagebrush (Artemisia tridentata spp. Wyomingensis), spiny hopsage (Grayia spinosa), Stansbury cliffrose (Purshia stansburiana), and shadscale saltbush (Atriplex confertifolia). At mid-to high-elevations, species such as Utah Juniper (Juniperus osteosperma), single-leaf pinyon (Pinus monophyla), Stansbury cliffrose, and Wyoming sagebrush were common. Low to mid elevations were defined by more open habitat types, while mid to high elevations were dominated by pinyon-juniper woodlands. Lone Mountain contained large, contiguous areas of precipitous terrain, while the Weepah Hills had patchy distributions of precipitous terrain. The ranges received an annual average of 11.8 cm (SD = 1.6 cm) of precipitation and 60.1 cm (SD = 48.2 cm) of snowfall throughout the duration of our study (January 2016 to December 2018). Temperatures during summer months ranged from 16.2°C (SD = 0.9°C) to 31.1°C (SD = 0.4°C) and winter months ranged from-4.6°C (SD = 0.4°C) to 5.6°C (SD = 1.4°C). High temperatures during summer months resulted in most water sources in the Weepah Hills drying up; as a result, most wildlife populations were restricted to Lone Mountain and the northern section of the Weepah Hills. Throughout the duration of our study, drought conditions varied based on Palmer Drought Severity Index scores (PDSI, Palmer, 1965). During spring months, PDSI scores indicated that 2017 was the wettest and 2018 was the driest year (2016: = 0.33, SD = 0.49; 2017: = 3.5, SD = 0.7; 2018: = −1.66, SD = 0.42). The area was home to several ungulate species, including (in order of abundance) desert bighorn sheep, mule deer (Odocoileus hemionus), pronghorn (Antilocapra americana), and feral horse (Equus ferus). There also was a diverse suite of predators, including mountain lion (Puma concolor), bobcat (Lynx rufus), coyote (Canis latrans), grey fox (Urocyon cinereoargenteus), and golden eagle (Aquila chrysaetos). The bighorn sheep population was considered to be increasing and potentially approaching carrying capacity based on aerial surveys conducted by the Nevada Department of Wildlife (Blum, 2021).
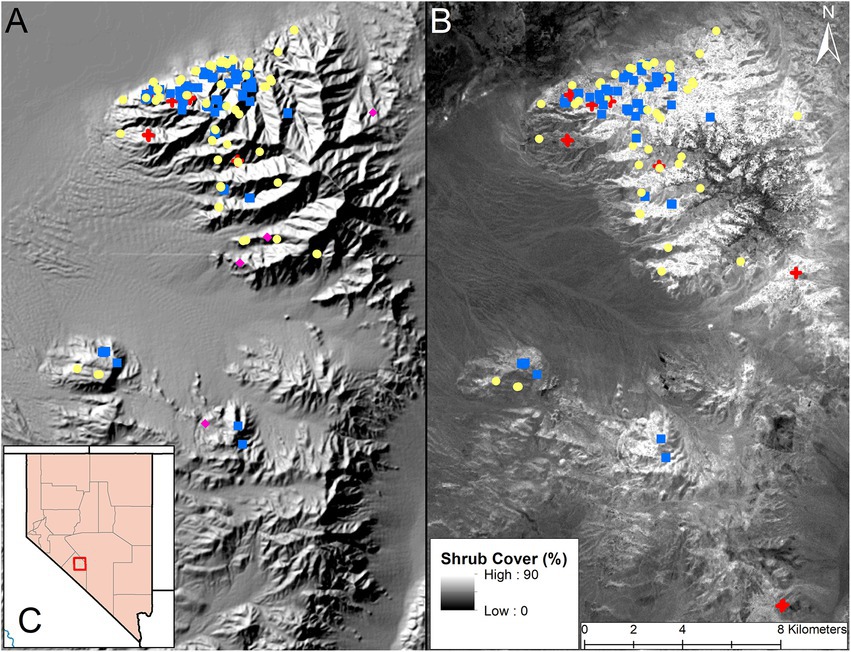
Figure 1. Map of the Lone Mountain and Weepah Hills study area outside of Tonopah, Nevada (2016–2018). Image (A) shows the location of fecal nitrogen samples used in our diet analyses, overlaid on a hill shade layer of the mountain range. Yellow circles represent pre-parturition samples, blue squares represent post parturition samples, red crosses represent post juvenile mortality samples, and pink diamonds represent not pregnant samples. Image (B) shows the location of diet composition samples used in our diet analyses, overlaid on a shrub cover layer (www.rangeland.app) of the mountain range. Shrub cover ranged from 0 to 90 percent throughout the region, lighter colors represent areas with high shrub cover and dark colors represent areas with low shrub cover. Yellow circles represent pre-parturition samples, blue squares represent post parturition samples, and red crosses represent post juvenile mortality samples. Image (C) shows the location of the Lone Mountain study site within a county outline map of Nevada.
Data collection and processing
We captured female desert bighorn sheep on Lone Mountain using a net-gun fired from a helicopter (Krausman et al., 1985) during the winters of 2016 to 2018 (2016: n = 16, 2017: n = 20, 2018: n = 27). We limited captures to no more than 2 individuals per social group to maintain independence of sampling units (Zar, 2010). Captured individuals were uniquely marked and fit with Iridium Global Positioning System (GPS) satellite radio-collars (VECTRONIC Aerospace). Radio-collars were scheduled to collect locations at a fix rate of 6 locations per day from January to September and 2 locations per day from October through December, to maximize battery life. We collected fecal samples from each captured individual. We measured subcutaneous fat thickness, estimated body-condition scores, and estimated ingesta-free body fat for all captured individuals using ultrasonography and palpation (Stephenson et al., 2020). We used ultrasonography to determine pregnancy status of all captured individuals (Stephenson et al., 1995) and inserted vaginal implant transmitters (VITs; VECTRONIC Aerospace) into the birth canal of pregnant females.
To evaluate how female bighorn sheep adjusted diets throughout the parturition season, while provisioning offspring, and to determine provisioning status of adult females, we captured neonates and tracked their survival. Upon notification of parturition events from GPS radio-collars on maternal females, we waited a minimum of 4 h following parturition before we attempted captures to allow neonates to bond with their mothers. We fitted neonates with expandable VHF radio-collars, and monitored their survival through 4 months of life, at which time juveniles were likely to be weaned from their mothers. We found no evidence of human-induced mortality on handled neonates (Blum, unpublished data). All handling of animals was approved by the Institutional Animal Care and Use Committee at the University of Nevada, Reno (Protocol #00651) and followed guidelines established by the American Society of Mammalogists for care and handling of wild mammals (Sikes and The Animal Care and Use Committee of the American Society of Mammalogists, 2016).
Diet quality collection and analyses
Timing of parturition varied in our study area among years (2016: = 4/21/2016, SD = 18.03; 2017: = 759SD = 4/11/2017, 2018: = 4/3/2018, SD = 14.31). Throughout the parturition seasons, we collected 1,223 fecal samples (2016: n = 169, 2017: n = 759, 2018: n = 295) from radio-collared females. We collected fecal pellets using individual GPS locations from the morning of (i.e., < 0900) collection. We attempted to collect multiple samples from each radio-collared individual prior to parturition, with at least 1 week separating sampling events. Following parturition, we collected fecal samples from each radio-collared individual about 7, 21, and 35 days post parturition; if a neonate died within 30 days post-parturition, we restarted the sampling schedule to 7, 21, and 35 days following the death of the neonate. Seasonal sampling intervals included juvenile (juvenile samples from individuals >1 week old), spring (March through May), summer (June through August), and winter (November through February; primarily from captures). Sampling intervals for parturition periods included pre-parturition (samples collected prior to parturition), post parturition (samples collected following parturition), post juvenile mortality (samples collected following the death of a juvenile), and not pregnant were samples collected from females that were not pregnant upon capture, but within the same monthly sampling intervals. We attempted to obtain visual observations on females to ensure samples were from radio-collared individuals. Given the mobility of individuals and challenges navigating the landscape, identifying pellet groups from radio-collared individuals was difficult. When visual confirmation was not possible, we collected >10 pellets from each fecal pile within 50 m of the GPS location, and we recorded radio-collared individuals within the immediate area (i.e., < 50 yards) at the time of sampling. We only collected samples that were identified as fresh (i.e., wet, odorous, and soft). Samples were stored in a -20° C freezer at the end of each day.
We analyzed 425 samples (2016: n = 31, 2017: n = 285, 2018: n = 109) for percent nitrogen in feces to estimate quality across seasons and around parturition. When selecting samples for analysis, we made sure that all sampled individuals were represented in each of the parturition periods. Because many fecal piles were collected at each GPS location for collared individuals, we randomly selected multiple samples (i.e., piles) of those collected fecal piles at each GPS location. Therefore, we analyzed multiple samples per individual per parturition period. Samples were oven-dried for >48 h at 60°C to remove moisture from samples. After drying, samples were ground in a Spex Sample Prep Mixer Mill 8,000 M Mill Grinder for 5 min to ensure homogenization and combustion. Samples were then weighed into tins (~2 mg) for elemental analysis in a Costech 4,010 Elemental Analyzer (Costech Analytical Technologies, Inc., Valencia, CA, United States).
We analyzed fecal nitrogen data using JAGS 4.3 software (Plummer, 2003) through program R (R Core Team, 2021) via the jagsUI package (Kellner, 2021). We performed a two-way analysis of variance (ANOVA) in a Bayesian hierarchical framework to estimate the relationship between fecal nitrogen, year, and the previously defined seasons (i.e., juvenile, spring, summer, and winter). The ANOVA was parameterized in a means parameterization framework, where the mean fecal nitrogen was estimated independently for each year and season combination, with a random intercept term for individual bighorn sheep. We assigned each estimated mean a vague, normally distributed prior with a mean of 0 and variance of 0.001, while the error term was given a uniform distribution with a mean of 0 and variance of 100. For the random intercept, we estimated a vague prior with a normal distribution with a mean of 0 and the variance was estimated from a gamma distribution with a mean of 0.001, and variance of 0.001. We approximated posterior distributions by sampling 20,000 Markov chain Monte Carlo (MCMC) iterations and discarded the first 500 iterations across 3 chains.
We performed an Analysis of Covariance (ANCOVA) in a Bayesian hierarchical framework to estimate the relationship between fecal nitrogen, ordinal date, and the parturition period of interest. Similar to the previously described ANOVA, the ANCOVA was parameterized so that each parameter estimate represented that parameter’s group mean (means parameterization). We modeled fecal nitrogen of individual fecal samples by estimating separate means for each parturition period and accounted for seasonal variation in fecal nitrogen content by adding an additive linear effect of ordinal day. We also incorporated a random intercept based on individual because we had multiple samples for each individual throughout our data. We defined vague prior distributions for all estimated parameters within our model. Parturition period and ordinal date were estimated via a normal distribution with a mean of 0 and variance of 0.001. The error term was estimated from a uniform distribution with a mean of 0 and variance of 100, while the random intercepts for individuals were defined by a gamma distribution with a mean of 0 and the variance was estimated from a gamma distribution with a mean of 0.001, and variance of 0.001. To observe the relationships between percent fecal nitrogen, parturition period, and ordinal date, we predicted fecal nitrogen values for ordinal date 115 (April 25) and plotted the predicted posterior distributions. We selected that date because it was ~2 weeks following the mean date of parturition (April 12).
Additionally, we estimated residuals and predicted values for this analysis. We observed no relationship between residual and predicted values within the dataset. We also estimated a Bayesian value of p and determined model convergence via visual investigation of MCMC chains and Gelman-Rubin diagnostic statistics <1.1. We calculated the Bayesian value of p through a sum of squares measure of discrepancy, where we compared the lack of fit of the observed dataset and that of a predicted dataset (Gelman et al., 1996). The discrepancy measures for each dataset were then tested to quantify the proportion of times that the predicted dataset was greater than that of the observed dataset (Kéry, 2010). Our model investigating parturition period had a Bayesian value of p of 0.51, indicating good fit.
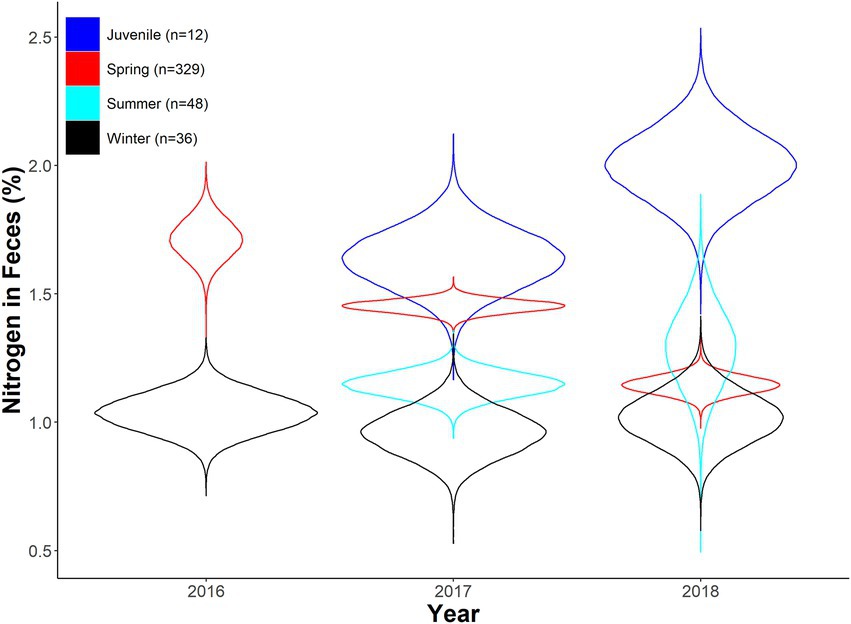
Figure 2. Expected fecal nitrogen percent as a function of year and season for desert bighorn sheep at Lone Mountain, Nevada (2016–2018). Violin plots represent the posterior distributions for predicted mean values derived from an ANOVA model of fecal nitrogen modeled as a function of year and season (with interaction term), which was fitted in a Bayesian framework (n = 10).
Diet composition processing and analyses
To identify diet composition, we randomly selected and analyzed 236 samples from capture and field collection (2016: n = 31, 2017: n = 131, 2018: n = 74) for DNA metabarcoding analysis,1 a method that has enhanced the accuracy of quantifying diets without direct observations of foraging (Sousa et al., 2019). To further increase the accuracy of the DNA results, we also submitted samples of known vegetation (n = 27 species). We used the exact sequence variants (ESVs) from the known plant species to refine the fecal diet data obtained from Jonah Ventures. We subset the dataset to include the ESVs that together made up >95% of the total read counts in the dataset, since those low read counts either may be the result of sequencing error, or contributed limited information to diet composition. In February 2021, given that many ESVs were still unidentified beyond family, or were linked to plant species not associated with the region, we used the Basic Local Alignment Search Tool (BLAST) to find regions of similarity between samples and match ESVs with known sequences in a public database. We used the Nucelotide BLAST tool available through the National Center for Biotechnology Information.2 We also used local and statewide plant databases to determine if a species may be present in the region. We investigated the plant species results with high query cover (>90%) and percent identity (>90%) to determine if they were present within the study region. If a search resulted in multiple possible species, genus, or family in the sample, we reported the highest taxonomic level. We used this information to adjust ESVs to the finest taxonomic category and no coarser than the family level.
We used the read count data of identified plants that were > 95% of the total read counts in the dataset to calculate a Shannon diversity index for the season and parturition categories that were previously defined (Shannon, 1948). Our sample size of fecal samples in the not pregnant category was inadequate (n = 4), and that group was not included in the parturition period analyses for diet diversity. We performed the same structure for Bayesian hierarchical models that were used for our fecal nitrogen analyses to compare diet diversity across the different seasons and between parturition groups. For the seasonal model, we modeled diversity of diet of individual fecal samples and estimated the relationship between year and season, with a random intercept for individual bighorn sheep. For the parturition model, we estimated parturition level effects on diet diversity by modeling the diversity of diet of individual fecal samples and estimated the relationship between diversity of diet and parturition period with an additive effect of ordinal date and a random intercept for individual bighorn sheep. We used the same prior distributions as the previously described models and estimated posterior distributions using 20,000 MCMC iterations and discarding the first 500 iterations across 3 chains. We estimated a Bayesian value of p of 0.51 for our parturition model of diet diversity, indicating strong fit. Furthermore, we estimated the predicted posterior distributions to visualize the relationship between diet diversity, parturition period, and ordinal date by plotting predictive values at ordinal date 115 (April 25).
We used read counts to determine if the diets across seasons and between the parturition groups were different through a permutational multivariate analysis of variance (PERMANOVA). This was accomplished using Bray-Curtis distances to quantify the dissimilarity between each group through the adonis function in the “vegan” package in program R (Oksanen et al., 2013). Across both PERMANOVA models, we tested for interactions between the continuous (i.e., year for seasonal model; ordinal date for parturition period model) and grouping variables (i.e., season and parturition period).
We followed similar methods to those presented in Nielsen and Matocq (2021) by calculating frequency of occurrence and relative read abundance of plant taxa in fecal samples to estimate consumption of plant taxa at the individual and group levels (i.e., [juvenile, spring, summer, and winter]; [pre-parturition, post parturition, and post juvenile mortality]). We considered a plant taxon to be present in an individual fecal sample if it made up ≥5% of the total composition (Deagle et al., 2019). Relative abundance was calculated for each plant species within individual fecal samples to identify the percent contribution each plant made to each sample. We then averaged the relative abundance values for females with and without dependent young. To determine which plants were significantly associated with each group, we used the “signassoc” function in the “indicspecies” package in program R (De Cáceres and Legendre, 2009) to analyze the presence and absence matrix and relative read abundance datasets. We investigated both frequency of occurrence and relative read abundance measurements in our dataset because diet composition datasets are prone to recovery bias (Deagle et al., 2019).
Results
We found minimal overlap in season*year interactions in our seasonal fecal nitrogen model, indicating strong differences in fecal nitrogen among seasons and years (Table 1). In spring 2017, diet quality of adult females overlapped that of juveniles, but did not overlap the following year. In 2018, we observed strong overlap in diet quality among spring, summer, and winter diet quality, whereas only summer and winter diets overlapped in 2017 (Table 1). Juveniles (i.e., young of the year) had the highest fecal nitrogen regardless of year, likely because they were gaining extra nutrients from nursing (Table 1; Figure 2). Adult females tended to have their highest quality diets during spring months across all years; spring diet quality notably declined from 2016 to 2018 (Table 1; Figure 2). Diet quality during summer months was consistently higher than winter months, yet summer and winter months overlapped 19 and 28% in 2017 and 2018 (Table 1; Figure 2). Diet quality during winter was consistently lowest (Table 1; Figure 2).
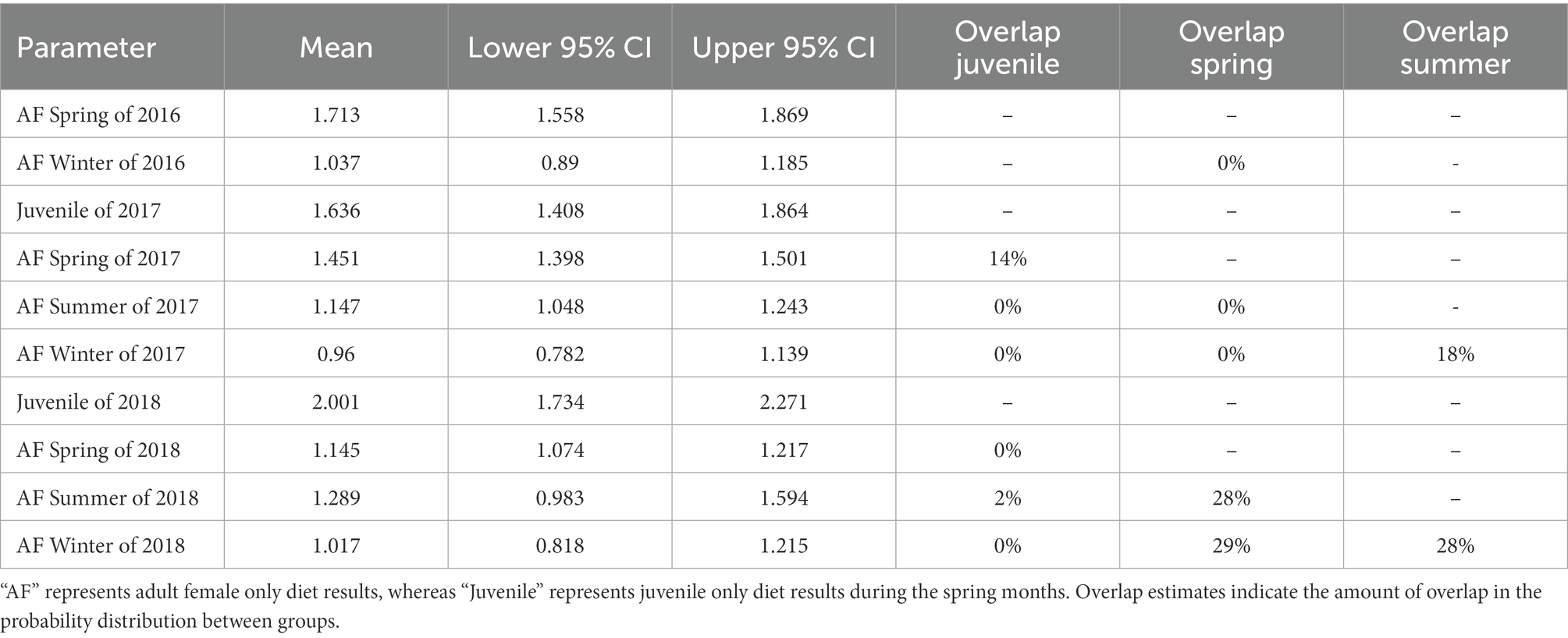
Table 1. Results from the ANOVA in a Bayesian hierarchical framework testing the effect of year and seasonal groups (i.e., Spring: March – May, Summer: June – August, Winter: November – February) on the percent of fecal nitrogen in the diets of desert bighorn sheep at Lone Mountain, Nevada (2016–2018).
We found overlap in all parameters for parturition period and a positive relationship of ordinal date on percent fecal nitrogen (Table 2). Predictions from estimates for parturition period at ordinal date 115 (April 25) indicated strong overlap in nitrogen content in fecal samples between post-parturient females and those that had not given birth (Figure 3). Pre-parturient females had more variation in percent fecal nitrogen than those that were provisioning offspring (Table 2; Figure 3), but pre-parturient females had lower fecal nitrogen. Predictions at ordinal date 115 indicated females that had lost a neonate and those that were not pregnant had the highest percent of nitrogen present in feces (Figure 3).
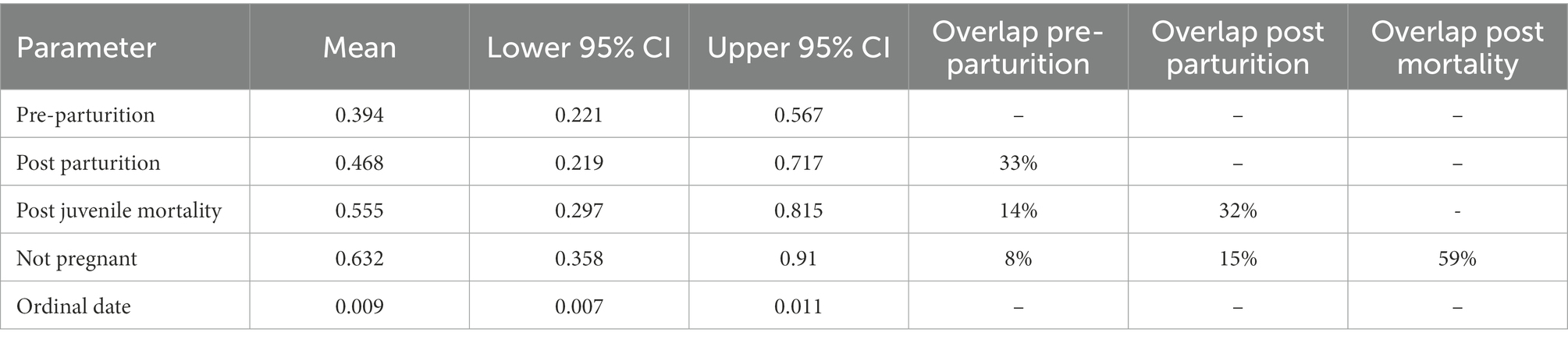
Table 2. Results from the ANCOVA in a Bayesian hierarchical framework testing the effect of Ordinal date and parturition groups (i.e., Pre-parturition, post parturition, post juvenile mortality, and not pregnant) on the percent of fecal nitrogen in the diets of desert bighorn sheep at Lone Mountain, Nevada (2016–2018).
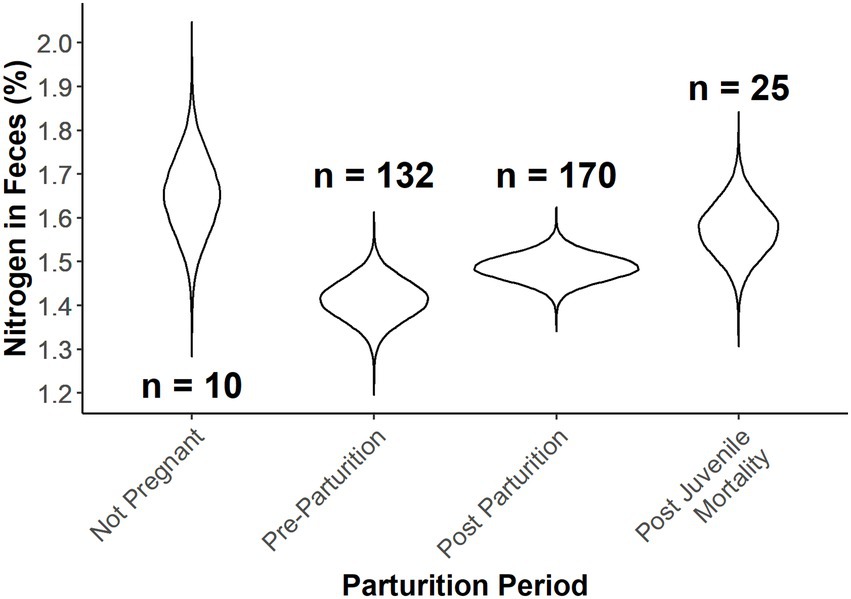
Figure 3. Expected fecal nitrogen percent as a function of Ordinal date and provisioning status of female desert bighorn sheep at Lone Mountain, Nevada (2016–2018). Violin plots represent the posterior distributions for the predicted mean values derived from an ANCOVA model of fecal nitrogen modeled as a function of provisioning status at Ordinal date 115, which was fitted in a Bayesian framework (n = 4).
We found strong overlap across season and year in diversity of diet in fecal samples. Diversity of diet varied across seasons as well as between juveniles and adults (Table 3; Figure 4). Furthermore, diet diversity decreased as the year progressed (Table 3; Figure 4). Juveniles tended to have a higher diversity of diet, however, there was strong overlap with adult samples across seasons (Table 3; Figure 4). Diet composition was distinct between seasons and age classes, although minimal variance was explained by the estimated parameters (Season: MS = 1.376, r2 = 0.071, value of p = 0.001; Year: MS = 0.622, r2 = 0.011, value of p = 0.02; Interaction: MS = 0.306, r2 = 0.016, value of p = 0.166).
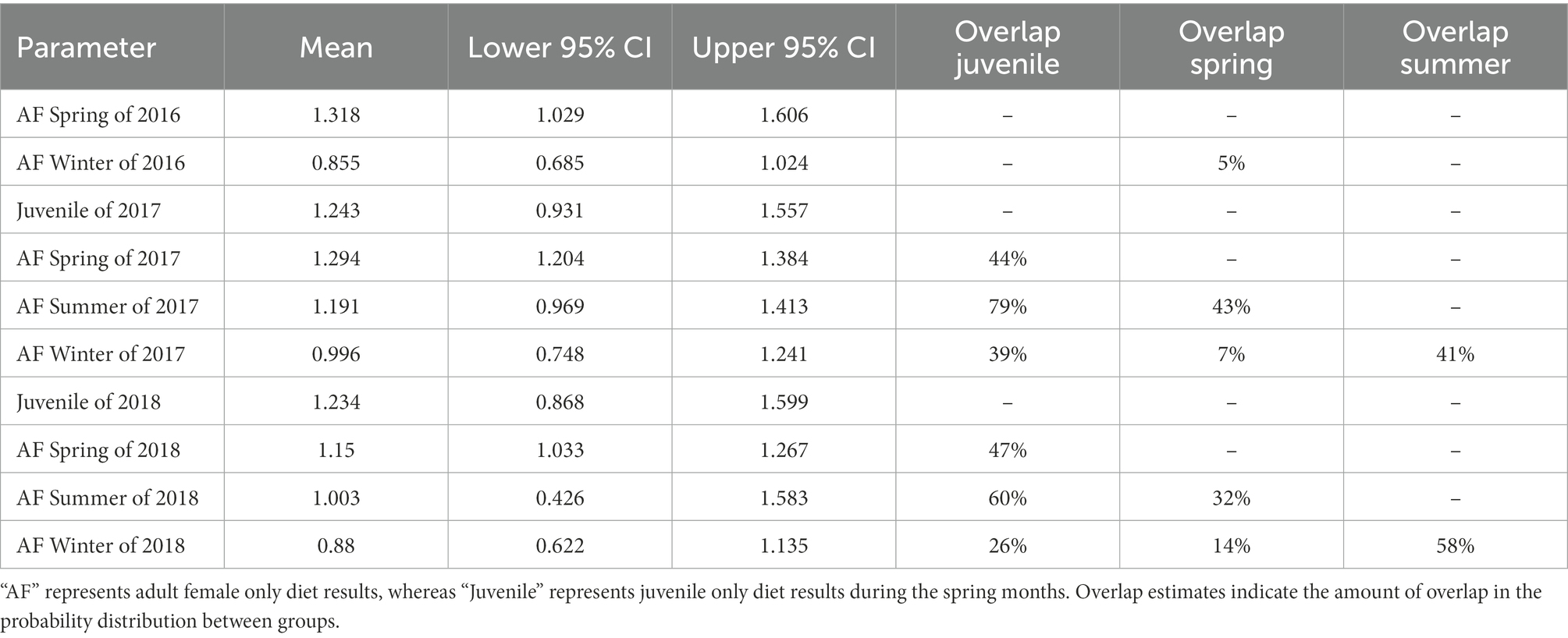
Table 3. Results from the ANOVA in a Bayesian hierarchical framework testing the effect of year and seasonal groups (i.e., Spring: March – May, Summer: June – August, Winter: November – February) on the diversity in the diets of desert bighorn sheep at Lone Mountain, Nevada (2016–2018).
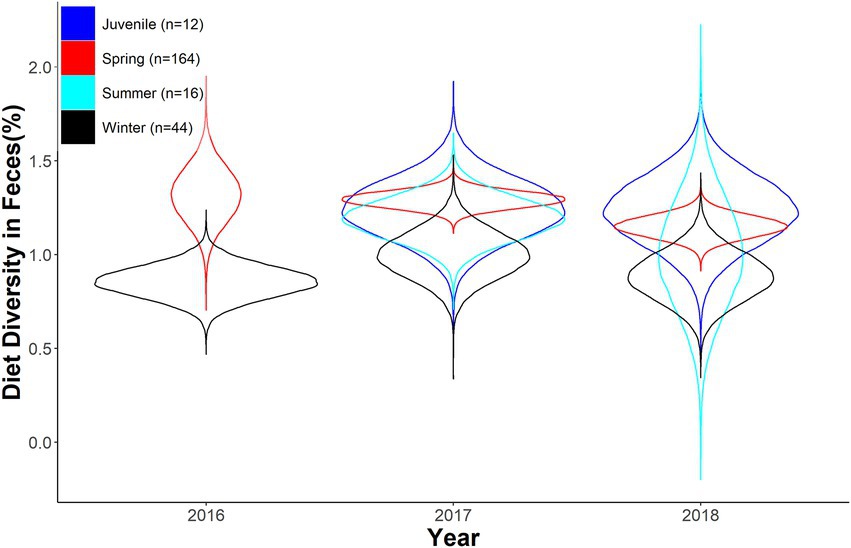
Figure 4. Expected Shannon diversity index as a function of year and season for desert bighorn sheep at Lone Mountain, Nevada (2016–2018). Violin plots represent the posterior distributions for predicted mean values derived from an ANOVA model of Shannon diversity index modeled as a function of year and season (with interaction term), which was fitted in a Bayesian framework (n = 10).
Diet diversity of females was also dependent on the parturition categories (Table 4; Figure 5). Females that lost offspring had the greatest diet diversity, although there was 36% overlap with females that were provisioning offspring (Table 4; Figure 5). Pre-parturient females had the lowest diet diversity, but overlapped diet diversity with females provisioning offspring by 14% (i.e., post parturition; Table 4; Figure 5). Diet composition was also distinct between parturition categories, although the variance explained by the estimated parameters was low (Parturition categories: MS = 0.968, r2 = 0.056, value of p = 0.001; Ordinal date: MS = 0.979, r2 = 0.028, value of p = 0.001).

Table 4. Results from the ANCOVA in a Bayesian hierarchical framework testing the effect of Ordinal date and parturition groups (i.e., Pre-parturition, post parturition, and post juvenile mortality) on the diversity in the diets of desert bighorn sheep at Lone Mountain, Nevada (2016–2018).
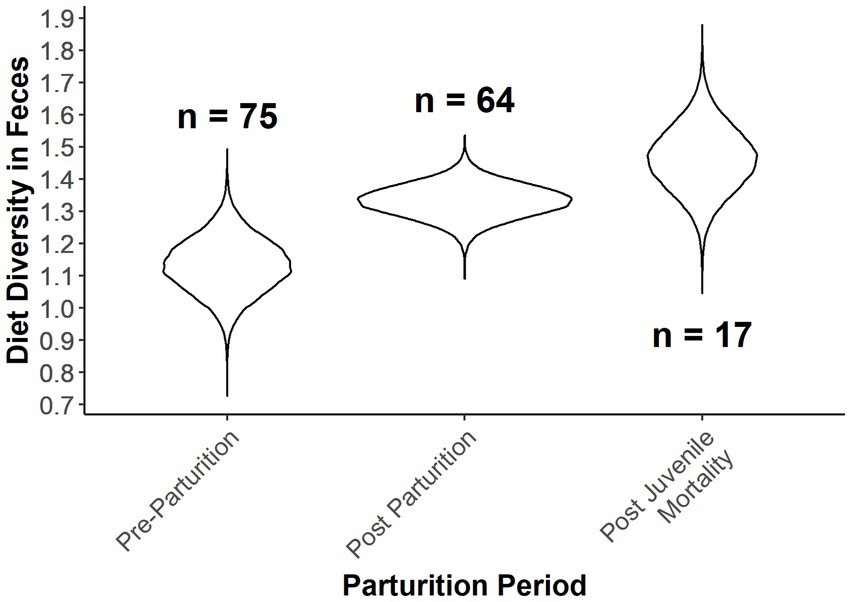
Figure 5. Expected Shannon diversity index as a function of Ordinal date and provisioning status of female desert bighorn sheep at Lone Mountain, Nevada (2016–2018). Violin plots represent the posterior distributions for the predicted mean values derived from an ANCOVA model of Shannon diversity index modeled as a function of provisioning status at Ordinal date 115, which was fitted in a Bayesian framework (n = 3).
Diet composition was largely similar across seasons, with some variation in both frequency of occurrence and relative read abundance (Table 5). Shadscale saltbush, horsebrush (Tetradymia spp.), and granite prickly phlox (L. pungens) were the only plant taxa that showed significant differences across seasons in both frequency of occurrence (shadscale saltbush: value of p = 0.02, horsebrush: value of p = 0.04, granite prickly phlox: value of p = 0.02) and relative read abundance (shadscale saltbush: value of p = 0.02, horsebrush: value of p = 0.02, granite prickly phlox: value of p = 0.02) datasets (Table 5). Shadscale saltbush was only present in the spring and winter diets of adult females, whereas granite prickly phlox and horsebrush were only present in winter diets. Saxifragaceae (value of p = 0.04) was only present in the spring and summer diets of adults (Table 5). Relative read abundance indicated that Stansbury cliffrose (value of p = 0.02), increased in individual diets throughout the year (Table 5).
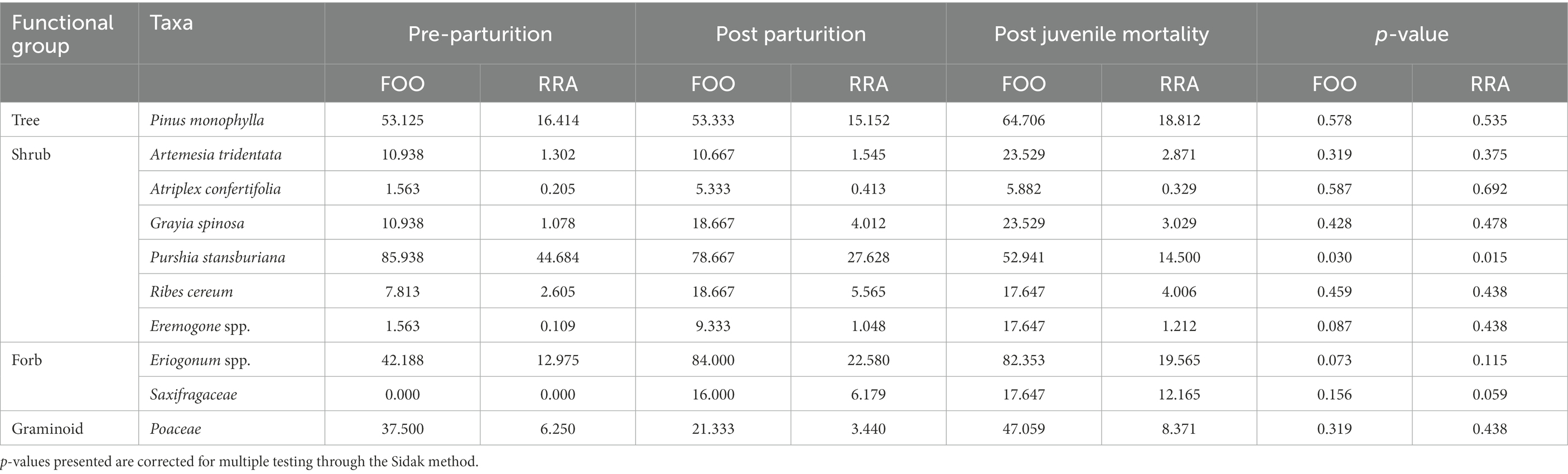
Table 5. Frequency of occurrence (FOO) and relative read abundance (RRA) of the top 12 unique plant taxa (based on total read counts) identified in the diets of desert bighorn sheep with differing parturition and provisioning statuses (i.e., Pre-parturition, post parturition, and post juvenile mortality), at Lone Mountain, Nevada (2016–2018).
Frequency of occurrence and relative read abundance results indicate that females in different parturition groups had very similar diets (Table 6). Only pre-parturient females had higher presence and concentrations of Stansbury cliffrose in their diet compared to post-parturient and females that lost a juvenile to mortality (Table 6). Additionally, females that lost a juvenile to mortality had lower presence and concentrations of Stansbury cliffrose in their diet than females that were provisioning young (Table 6). Saxifragaceae only appeared in diets of females that were provisioning young or had lost a juvenile (Table 6). The frequency of occurrence of buckwheat was higher in females prior to parturition and greatly reduced following parturition (Table 6).
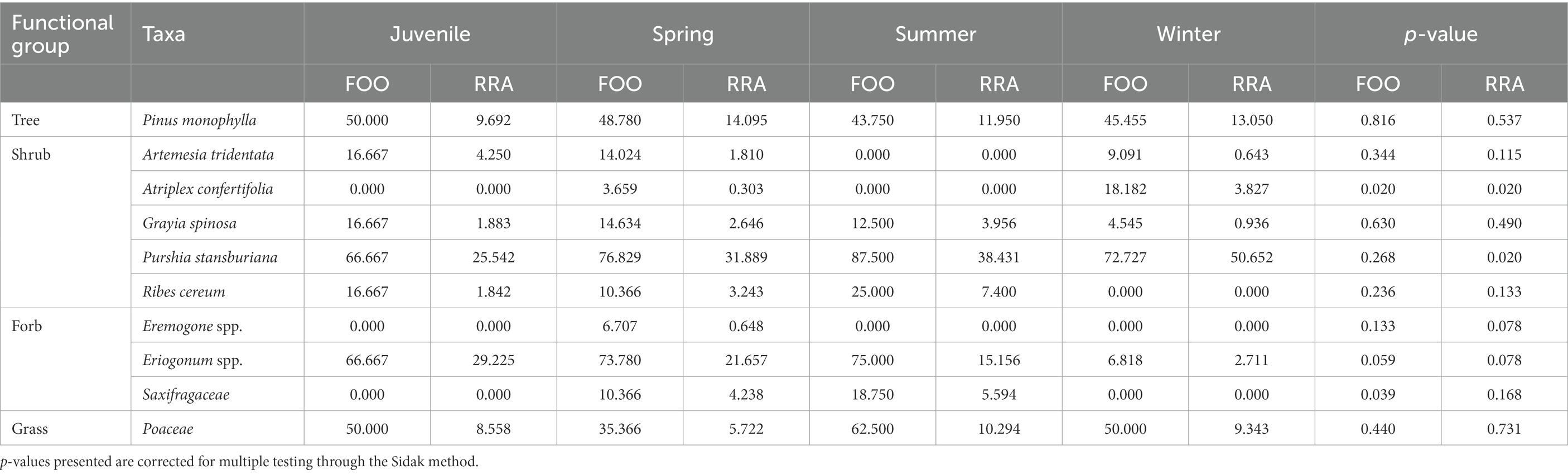
Table 6. Frequency of occurrence (FOO) and relative read abundance (RRA) of the top 12 unique plant taxa (based on total read counts) identified in the diets of desert bighorn sheep across seasons (i.e., Spring: March – May, Summer: June – August, Winter: November – February) and between juveniles and adults in spring months, at Lone Mountain, Nevada (2016–2018).
Discussion
We found that juveniles had higher diet quality and diversity than adult females during the spring months. Those results were not surprising, given that juveniles were consuming milk in addition to vegetation, which increases nitrogen content in feces (Jenkins et al., 2001; Taillon et al., 2013). During spring months, juveniles had a wider credible interval for diversity of diets than adult females, which is likely related to lower sample sizes of juveniles. Juveniles selected plant species that had a high prevalence in the spring diets of adult females, which is consistent with habitat selection being a learned behavior (Nielsen et al., 2013; Larue et al., 2018). Nevertheless, juveniles did not sample plant species to the degree that adult females did, likely because vegetation is not the primary source of nutrition during their first 2 months of life, allowing juveniles to be more selective. That relationship may possibly be a result of how quickly juveniles learn to sample plants rather than an innate understanding of the highest quality or more palatable species.
Diet quality, diversity, and composition were dependent upon season and year for adult females. Our results indicated that fecal nitrogen was higher during spring and summer than winter months, which consistently had the lowest fecal nitrogen. Diet quality also regressed each year of the study, which may be related to drought conditions, although 2017 was the wettest year (PDSI: = 3.5, SD = 0.7) of our study, while 2018 was the driest (PDSI: = −1.66, SD = 0.42). Furthermore, spring and summer months had higher diet diversity than winter months, although the diet diversity was similar between summer and winter in 2018. Spring and early summer months coincide with vegetation green-up (Snyder et al., 2016); therefore, it is not surprising that females had higher fecal nitrogen and diet diversity during this period. This result is further supported by our diet composition data, because forbs became more prominent in diets during the spring, and females consumed more species of forbs during this period. In the Great Basin, many plant species senesce as precipitation decreases and temperatures rise, resulting in a reduction in protein and digestible energy available for herbivores during summer and winter months (Beck and Peek, 2005b). Many annual and perennial forbs are not available to herbivores year-round, resulting in individuals adjusting selection to species that are available during the summer and winter months (Krausman et al., 1989; Miller and Gaud, 1989; Bleich et al., 1997). Parturition of bighorn sheep at Lone Mountain occurred during spring months, March through May (Blum, 2021), when forage availability and quality were highest. The third trimester of pregnancy and lactation are the most expensive periods for female ungulates in terms of requirements for protein and digestible energy (Barboza et al., 2009). Therefore, our results are consistent with the notion that timing of parturition is likely the result of selection for females to give birth during the most nutritionally beneficial time period in our study system. Tradeoffs associated with behaviors that are risk-averse or risk-prone relative to risk of predation are associated with montane ungulates around parturition periods (Festa-Bianchet, 1988; Berger, 1991; Bleich et al., 1997; Rachlow and Bowyer, 1998; Barten et al., 2001; Poole et al., 2007; Long et al., 2009). Previous research has shown females use habitat types that are linked to survival of juveniles, but potentially have reduced quality or availability of forage (Festa-Bianchet, 1988; Berger, 1991; Bleich et al., 1997; Rachlow and Bowyer, 1998; Blum, 2021). Nevertheless, females may not have to make those tradeoffs in some ecosystems, and it is more evolutionarily advantageous to select areas that provide high quality and abundant vegetation, in addition to safety for offspring (Pierce et al., 2004; Heffelfinger et al., 2020).
We found mixed support for our hypothesis that individuals without dependent young would have more diverse diets than those provisioning offspring. Females that lost offspring had a 64% likelihood of having a more diverse diet than those females that were provisioning offspring, lending some support to our hypothesis. Contrary to our hypothesis, however, pre-parturient females had an 86% likelihood of having a less diverse diet than those females that were provisioning offspring. Those results may be a function of selection for the highest quality plants, or because of fewer species of plants on the landscape when most females were not provisioning young, given that forbs and annual grasses became more prevalent as the spring months progressed (Nicholson et al., 2006). Our results may indicate that females selected some forages at a higher rate, therefore reducing diet diversity, which may also be linked to availability. Females may also be selecting forage to maximize overall intake by selecting for species that offer larger bite masses, such as Stansbury cliffrose, which may further reduce diet diversity. The differences in diet diversity between females that had lost offspring and those provisioning offspring likely indicated a tradeoff because vegetation availability was similar in those time periods, but females that lost offspring had more diverse diets than females provisioning young. An increase in diet diversity may allow females to maximize quality of their diet and more efficiently replace somatic reserves lost during gestation and lactation. However, this relationship may not occur if individuals selected strongly for vegetation with the highest nutrient content, which could result in a decrease in diversity. Further research has shown that females adjust habitat selection when provisioning offspring to areas that are more beneficial to survival of young rather than nutritional availability (Bleich et al., 1997; Blum, 2021). We suspect that females that were not constrained to steeper, rugged habitats had more diverse diets because they were not limited by the presence of dependent young. While the loss of a neonate has implications for fitness, females can increase the quality of future offspring by increasing their nutritional condition rapidly after the loss of their offspring (Monteith et al., 2013; Morano et al., 2013; Monteith K. L. et al., 2014). This behavior may lead to higher survival rates of offspring the following year and increase the likelihood of recruiting young.
Collecting fecal samples from specific individuals proved challenging in our study area. Given that we rarely were able to match a fecal pile with a specific radio-collared individual, we collected as many piles in proximity to morning GPS locations of specific females as possible. We analyzed multiple pellets from each group of samples to get a better idea of diet quality and composition within the group, as well as to increase the probability of analyzing the sample from the target individual. If our samples were not representative of specific parturition periods, we would expect no clear trends in diet quality or composition across females prior to parturition, while provisioning offspring, and following a loss of offspring, which was not the case. Additionally, female bighorn sheep typically form groups comprised of other individuals exhibiting similar provisioning status and that, in turn, influenced habitat selection (Blum, 2021). Individuals within a group should also be exposed to the same vegetation types when foraging. Thus, our results likely reflect the diet selection of females provisioning and not provisioning offspring in our study area.
Previous research indicates that lactation status influences the absorption rates for nitrogen in ungulates (Monteith K. B. et al., 2014). Those results indicate that given similar diets, lactating females will absorb greater amounts of nitrogen than non-lactating females and skew the nitrogen percent in feces (Monteith K. B. et al., 2014). Therefore, it is possible that our post parturition levels of fecal nitrogen are negatively skewed and that post parturient females have similar diet quality as females without dependent offspring. However, non-lactating females, especially those that were not pregnant, were commonly found in areas where male bighorn sheep occurred within the study area, which were segregated from parturient females (Blum, personal observation). Bleich et al. (1997) also showed that males used areas with higher quality vegetation during these periods, therefore, it is likely that non-lactating females were accessing higher quality forage than those females with dependent offspring. This was also supported by some of our diet composition results, which indicated that females that lost a neonate had higher frequency of occurrence and relative read abundance of some forbs and grasses in their diet. Therefore, we are confident that our results that indicate an apparent diet quality tradeoff are representative of the diet quality differences we observed between provisioning status.
We were unable to measure metabolizable energy content of fecal samples in our study; although, previous research has indicated that metabolizable and digestible energy, not digestible protein, was the limiting factor for ungulates during lactation (Holt et al., 1992; Parker et al., 1999). Energy and protein requirements increase substantially during lactation and are essential to the growth of offspring (Barboza et al., 2009; Parker et al., 2009). Although we cannot speak to the variation of metabolizable energy in the diet of bighorn sheep across seasons and parturition stages, similar relationships exist because protein and energy measurements are often correlated in ungulate diets throughout the year (Holt et al., 1992; Parker et al., 1999, 2009; Rad et al., 2015). The apparent tradeoffs of nutritional quality for safety of offspring are likely linked to metabolizable energy because females select areas with lower forage availability while provisioning offspring (Blum, 2021).
Our results indicated a high presence of single-leaf pinyon in bighorn sheep diets on Lone Mountain. We suspect that result is a combination of high concentrations of pollen across the landscape during spring months and low digestibility of some vegetation species (Ando et al., 2018). Given that different functional groups and plant taxa differ in digestibility, some species likely show higher presence in feces (Scasta et al., 2019). This bias is not unique to diet analyses using DNA but is often reported in microhistological analyses of diet composition (Anthony and Smith, 1974; Dearden et al., 1975). We did observe bighorn sheep foraging on single-leaf pinyon occasionally, but those events were rare and leads us to suspect that the high concentrations in bighorn diets is likely a result of pollen falling onto consumed vegetation (Minckley et al., 2008) and combined with low digestibility of pinyon pine. While Blum (2021) showed that female bighorn sheep avoided areas with a lot of trees, pinyon pine was present throughout the study area, albeit in low densities, therefore it is likely that pollen was widely distributed throughout the study area. DNA metabarcoding methods are an evolving technology that need further research and development to understand how estimated proportional diet composition represents actual dry matter intake. Additionally, primer biases may result in different amplification rates across forage species and result in courser taxa identification (Browett et al., 2021; Stapleton et al., 2022). To reduce the potential biases of DNA metabarcoding analyses, we used a combination of frequency of occurrence and relative read abundance. While relative read abundance measurements may be subject to higher rates of recovery bias, they tend to provide more accurate portrayals of population-level diets (Deagle et al., 2019). Additionally, frequency of occurrence measurements are generally less affected by recovery biases and are highly consistent in comparison to measurements of relative read abundance (Deagle et al., 2019). Using those measurements in conjunction provides a more accurate depiction of diet composition at the population-level and is encouraged to consider all sources of bias (Deagle et al., 2019).
To our knowledge, there are no other studies that have investigated the variation in diet quality and composition in free-ranging bighorn sheep associated with times around parturition, although many studies have addressed variation in diet quality and content across seasons or between adults and juveniles (Shank, 1982; Festa-Bianchet, 1988; Rominger et al., 1988; Krausman et al., 1989; Miller and Gaud, 1989; Irwin et al., 1993; Bleich et al., 1997; Denryter et al., 2020). Availability of forage species across most of those studies was highly variable and did not match the vegetation community available on Lone Mountain. Bighorn sheep rely on grass and shrubs as forage throughout much of their range (Bleich et al., 1997), which was also demonstrated in our study throughout the year (Table 5). Furthermore, most studies showed an increase in forbs in diets during spring and summer months, when they are more available and usually higher quality than at other times of the year (Krausman et al., 1989; Miller and Gaud, 1989; Wagner and Peek, 2006). Nevertheless, vegetation abundance and quality in other desert regions may not fall within these time periods (McKee et al., 2015). We also observed higher concentrations of forbs in diets of bighorn sheep during the spring and summer months, while availability of those forages was substantially reduced during the winter. Shrub concentration in the diets of bighorn sheep on Lone Mountain increased in winter months, similar to results in other research (Krausman et al., 1989; Irwin et al., 1993; Bleich et al., 1997). Other studies have shown strong similarities to diets of adult females and juveniles (Miller and Gaud, 1989; McKinney and Smith, 2007). We also showed a strong similarity between adult female diets and those of their offspring; our results, however, indicated higher degrees of selection for specific species by nursing juveniles, while diet richness of adults was greater.
Understanding diet characteristics are essential to the conservation of wild ungulates. Managing for ecosystems containing vegetation that meets the dietary needs of target species increases the health of populations. Biologists can use this information to guide habitat management and increase the nutritional availability across the landscape. For instance, our findings indicate that biologists should focus reseeding efforts on Stansbury cliffrose, buckwheat, species in the Saxifragaceae family, and native grasses when attempting to increase vegetation for desert bighorn sheep in this region. These species were among the most common in the diets of bighorn sheep following parturition and increasing their availability on the landscape will benefit females provisioning young. Understanding diet characteristics are also useful for identifying translocation sites for bighorn sheep. To increase the likelihood of a translocated population succeeding, biologists should select mountain ranges with similar vegetation types and availability as the source population. Furthermore, ensuring that those target mountain ranges have vegetation types that have the necessary nutrients to meet the metabolic needs of females is essential to the success of a population.
Our study provides further insight into the dietary relationships between female and juvenile bighorn sheep and their environments. A primary focus of nutritional ecology is the link between diet and the environment and gaining an understanding of how diet selection influences life history strategies and demography. We demonstrated tradeoffs between forage quality for females of different provisioning status, which lends further support to tradeoffs associated with risk-averse and risk-prone use of habitats, and forages that are commonly associated with montane ungulates. Selection for high quality forage, which often coincides with areas of greater risk of predation, when females are not provisioning young allows females to increase their somatic reserves or increase the nutrients directed towards subsequent reproduction. This relationship provides further insight into the habitat selection strategies of many montane ungulates around parturition, and how those strategies influence reproductive fitness of individuals.
Data availability statement
The datasets generated for this study are available on request to the corresponding author.
Ethics statement
The animal study was reviewed and approved by University of Nevada, Reno IACUC Committee (Protocol #00651).
Author contributions
MB, KMS, and MC conceived and designed the study with input from BW and VB. MB analyzed the data with input from KTS and KMS. MC, JB, and BW organized captures and aided in data collection. MC, KMS, and MB obtained funding with help from BW and VB. BS provided equipment and guidance for analysis of fecal samples for C and N. All authors contributed to writing and editing of the manuscript.
Funding
This work would not be possible without funding from the Nevada Department of Wildlife, The Fraternity of Desert Bighorn, Dallas Safari Club, Nevada Bighorns Unlimited, Wild Sheep Foundation, Wildlife Heritage Trust, Nevada Wildlife Record Book, the Desert Bighorn Council, and Nevada Muleys.
Acknowledgments
We would like to thank all who assisted with data collection, including B. Regan, J. Blum, T. Allen, J. Magee, J. Merrell, J. Jensen, B. Wagler, N. Nourn, D. Adams, J. Gundlach, P. Rebholz, J. Thalmann, R. Martinez, M. Osterhout, M. Disney, C. Irons, J. Miller, A. Mineau, N. Brascia, K. Lindberg, S. Watson, C. Crosby, C. McKee, N. Jackson, C. Schroeder, L. Heffelfinger, and P. Wolff. We would like to thank T. Stephenson for his assistance with measuring body condition and handling of individuals during captures. We thank M. Gritts for his help with R coding at various stages of the analyses. We would like to thank K. Denryter for a friendly review. We would like to thank all the volunteers and biologists who attended each adult capture. This is Professional Paper 140 from the Eastern Sierra Center for Applied Population Ecology.
Conflict of interest
The authors declare that the research was conducted in the absence of any commercial or financial relationships that could be construed as a potential conflict of interest.
Publisher’s note
All claims expressed in this article are solely those of the authors and do not necessarily represent those of their affiliated organizations, or those of the publisher, the editors and the reviewers. Any product that may be evaluated in this article, or claim that may be made by its manufacturer, is not guaranteed or endorsed by the publisher.
Footnotes
References
Ando, H. C., Fujii, M., Kawanabe, Y., Ao, T. I., and Takenaka, A. (2018). Evaluation of plant contamination in metabarcoding diet analysis of a herbivore. Sci. Rep. 8:15563. doi: 10.1038/s41598-018-32845-w
Anthony, R. G., and Smith, N. S. (1974). Comparison of rumen and fecal analysis to describe deer diets. J. Wildl. Manag. 38, 535–540. doi: 10.2307/3800886
Barboza, P. S., and Bowyer, R. T. (2000). Sexual segregation in dimorphic deer: a new gastrocentric hypothesis. J. Mammal. 81, 473–489. doi: 10.1644/1545-1542(2000)081<0473:SSIDDA>2.0.CO;2
Barboza, P. S., and Bowyer, R. T. (2001). Seasonality of sexual segregation in dimorphic deer: extending the gastrocentric model. Alces 37, 275–292.
Barboza, P. S., and Parker, K. L. (2008). Allocating protein to reproduction in arctic reindeer and caribou. Physiol. Biochem. Zool. 81, 835–855. doi: 10.1086/590414
Barboza, P. S., Parker, K. L., and Hume, I. D. (2009). Integrative Wildlife Nutrition. Berlin, Heidelberg, Germany: Springer-Verlag.
Barten, N. L., Bowyer, R. T., and Jenkins, K. J. (2001). Habitat use by female caribou: tradeoffs associated with parturition. J. Wildl. Manag. 65, 77–92. doi: 10.2307/3803279
Beck, J. L., and Peek, J. M. (2005a). Diet composition, forage selection, and potential for forage competition among elk, deer, and livestock on aspen-sagebrush summer range. Rangeland Ecol. Manag. 58, 135–147. doi: 10.2111/03-13.1
Beck, J. L., and Peek, J. M. (2005b). Great Basin summer range forage quality: do plant nutrients meet elk requirements? Western North Am. Nat. 65, 516–527.
Berger, J. (1991). Pregnancy incentives, predation constraints and habitat shifts: experimental and field evidence for wild bighorn sheep. Anim. Behav. 41, 61–77. doi: 10.1016/S0003-3472(05)80503-2
Bleich, V. C., Blum, M. E., Shoemaker, K. T., Sustaita, D., and Holl, S. A. (2019). Habitat selection by bighorn sheep in a mesic ecosystem: the San Rafael Mountains, California, USA. Calif. Fish Game 105, 205–224.
Bleich, V. C., Bowyer, R. T., and Wehausen, J. D. (1997). Sexual segregation in mountain sheep: resources or predation? Wildl. Monogr. 134, 3–50.
Blum, M. E. (2021). Habitat selection of female desert bighorn sheep: tradeoffs associated with reproduction. Ph. D. Thesis, Reno: University of Nevada.
Boeker, E. L., Scott, V. E., Reynolds, H. G., and Donaldson, B. A. (1972). Seasonal food habits of mule deer in southwestern New Mexico. J. Wildl. Manag. 36, 56–63. doi: 10.2307/3799188
Bowyer, R. T. (1991). Timing of parturition and lactation in southern mule deer. J. Mammal. 72, 138–145. doi: 10.2307/1381988
Bowyer, R. T., Bleich, V. C., Stewart, K. M., Whiting, J. C., and Monteith, K. L. (2014). Density dependence in ungulates: a review of causes, and concepts with some clarifications. Calif. Fish Game 100, 550–572.
Bowyer, R. T., Stewart, K. M., Bleich, V. C., Whiting, J. C., Monteith, K. L., Blum, M. E., et al. (2020). Metrics of harvest for ungulate populations: misconceptions, lurking variables, and prudent management. Alces 56, 15–38.
Browett, S. S., Curran, T. G., O’Meara, D. B., Harrington, A. P., Guimarães Sales, N., Antwis, R. E., et al. (2021). Primer biases in the molecular assessment of diet in multiple insectivorous mammals. Mamm. Biol. 101, 293–304. doi: 10.1007/s42991-021-00115-4
Clutton-Brock, T. H., Lason, G. R., Albon, S. D., and Guinness, F. E. (1982). Effects of lactation on feeding behaviour and habitat use in wild red deer hinds. J. Zool. 198, 227–236. doi: 10.1111/j.1469-7998.1982.tb02072.x
Collins, W. B., and Urness, P. J. (1983). Feeding behavior and habitat selection of mule deer and elk on northern Utah summer range. J. Wildl. Manag. 47, 646–663. doi: 10.2307/3808601
Cook, R. C., Cook, J. G., Vales, D. J., Johsnon, B. K., Mccorquodale, S. M., Shipley, L. A., et al. (2013). Regional and seasonal patterns of nutritional condition and reproduction in elk. Wildl. Monogr. 184, 1–45. doi: 10.1002/wmon.1008
Cook, J. G., Johnson, B. K., Cook, R. C., Riggs, R. A., Delcurto, T., Bryant, L. D., et al. (2004). Effects of summer-autumn nutrition and parturition date on reproduction and survival of elk. Wildl. Monogr. 155, 1–61. doi: 10.2193/0084-0173(2004)155[1:EOSNAP]2.0.CO;2
De Cáceres, M., and Legendre, P. (2009). Associations between species and groups of sites: indices and statistical inference. Ecology 90, 3566–3574. doi: 10.1890/08-1823.1
Deagle, B. E., Thomas, A. C., McInnes, J. C., Clarke, L. J., Vesterinen, E. J., Clare, E. L., et al. (2019). Counting with DNA in metabarcoding studies: how should we convert sequence reads to dietary data? Mol. Ecol. 28, 391–406. doi: 10.1111/mec.14734
Dearden, B. L., Pegau, R. E., and Hansen, R. M. (1975). Precision of microhistological estimates of ruminant food habits. J. Wildl. Manag. 39, 402–407. doi: 10.2307/3799920
Denryter, K., Cook, R. C., Cook, J. G., Parker, K. L., and Gillingham, M. P. (2020). State-dependent foraging by caribou with different nutritional requirements. J. Mammal. 101, 544–557. doi: 10.1093/jmammal/gyaa003
Festa-Bianchet, M. (1988). Seasonal range selection in bighorn sheep: conflicts between forage quality, forage quantity, and predator avoidance. Oecologia 75, 580–586. doi: 10.1007/BF00776423
Gelman, A., Meng, X.-L., and Stern, H. S. (1996). Posterior predictive assessment of model fitness via realized discrepancies. Statis. Sin. 6, 733–807.
Heffelfinger, L. J., Stewart, K. M., Bush, A. P., Sedinger, J. S., Darby, N. W., and Bleich, V. C. (2018). Timing of precipitation in an arid environment: effects on population performance of a large herbivore. Ecol. Evol. 8, 3354–3366. doi: 10.1002/ece3.3718
Heffelfinger, L. J., Stewart, K. M., Shoemaker, K. T., Darby, N. W., and Bleich, V. C. (2020). Balancing current and future reproductive investment: variation in resource selection during stages of reproduction in a long-lived herbivore. Front. Ecol. Evol. 8:e00163. doi: 10.3389/fevo.2020.00163
Holt, B. S., Miller, W. H., and Wakeling, B. F. (1992). Composition and quality of mountain sheep diets in the Superstition Mountains, Arizona. Des. Bighorn Coun. Trans. 36, 36–40.
Irwin, L. L., Cook, J. G., McWhirter, D. E., Smith, S. G., and Arnett, E. B. (1993). Assessing winter dietary quality in bighorn sheep via fecal nitrogen. J. Wildl. Manag. 57, 413–421. doi: 10.2307/3809442
Jackson, N. J., Stewart, K. M., Wisdom, M. J., Clark, D. A., and Rowland, M. M. (2021). Demographic performance of a large herbivore: effects of winter nutrition and weather. Ecosphere 12:e03328. doi: 10.1002/ecs2.3328
Jenkins, S. G., Partridge, S. T., Stephenson, T. R., Farley, S. D., and Robbins, C. T. (2001). Nitrogen and carbon isotope fractionation between mothers, neonates and nursing offspring. Oecologia 129, 336–341. doi: 10.1007/s004420100755
Keech, M. A., Bowyer, R. T., Ver Hoef, J. M., Boertje, R. D., Dale, B. W., and Stephenson, T. R. (2000). Life-history consequences of maternal condition in Alaskan moose. J. Wildl. Manag. 64, 450–462. doi: 10.2307/3803243
Kellner, K. (2021). jagsPUI: a wrapper around ‘rjags’ to streamline ‘JAGS’ analyses. R package version 1, 1. Available at: https://CRAN.R-project.org/package=jagsUI
Kéry, M. (2010). Introduction to WinBUGS for Ecologists: Bayesian Approach to Regression, ANOVA, Mixed Models and Related Analyses Burlington, MA, USA: Academic Press.
Krausman, P. R., Hervert, J. J., and Ordway, L. L. (1985). Capturing deer and mountain sheep with a net-gun. Wildl. Soc. Bull. 13, 71–73.
Krausman, P. R., Leupold, B. D., Seegmiller, R. F., and Torres, S. G. (1989). Relationships between desert bighorn sheep and habitat in western Arizona. Wildl. Monogr. 102, 3–66.
Larue, B., Côté, S. D., St-Laurent, M.-H., Dussault, C., and Leblond, M. (2018). Natal habitat preference induction in large mammals-like mother, like child? Ecol. Evol. 8, 12629–12640. doi: 10.1002/ece3.4685
Long, R. A., Bowyer, R. T., Porter, W. P., Mathewson, P., Monteith, K. L., Findholt, S. L., et al. (2016). Linking habitat selection to fitness-related traits in herbivores: the role of the energy landscape. Oecologia 181, 709–720. doi: 10.1007/s00442-016-3604-7
Long, R. A., Kie, J. G., Bowyer, R. T., and Hurley, M. A. (2009). Resource selection and movements by female mule deer Odocoileus hemionus: effects of reproductive stage. Wildl. Biol. 15, 288–298. doi: 10.2981/09-003
Mautz, W. W. (1978). Sledding on a bushy hillside: the fat cycle in deer. Wildl. Soc. Bull. 6, 88–90.
McKee, C. J., Stewart, K. M., Sedinger, J. S., Bush, A. P., Darby, N. W., Hughson, D. L., et al. (2015). Spatial distributions and resource selection by mule deer in an arid environment: responses to provision of water. J. Arid Environ. 122, 76–84. doi: 10.1016/j.jaridenv.2015.06.008
McKinney, T., and Smith, T. W. (2007). Diets of adults and lambs of desert bighorn sheep during years of varying rainfall in Central Arizona. Southwest. Nat. 52, 520–527. doi: 10.1894/0038-4909(2007)52[520:DOAALO]2.0.CO;2
Miller, G. D., and Gaud, W. S. (1989). Composition and variability of desert bighorn sheep diets. J. Wildl. Manag. 53, 597–606. doi: 10.2307/3809182
Minckley, T. A., Bartlein, P. J., Whitlock, C., Shuman, B. N., Williams, J. W., and Davis, O. K. (2008). Associations among modern pollen, vegetation, and climate in western North America. Quat. Sci. Rev. 27, 1962–1991. doi: 10.1016/j.quascirev.2008.07.006
Monteith, K. L., Bleich, V. C., Stephenson, T. R., Pierce, B. M., Conner, M. M., Kie, J. G., et al. (2014). Life-history characteristics of mule deer: effects of nutrition in a variable environment. Wildl. Monogr. 186, 1–62. doi: 10.1002/wmon.1011
Monteith, K. B., Monteith, K. L., Bowyer, R. T., Leslie, D. M., and Jenks, J. A. (2014). Reproductive effects on fecal nitrogen as an index of diet quality: an experimental assessment. J. Mammal. 95, 301–310. doi: 10.1644/12-MAMM-A-306.1
Monteith, K. L., Schmitz, L. E., Jenks, J. A., Delger, J. A., and Bowyer, R. T. (2009). Growth of male white-tailed deer: consequences of maternal effects. J. Mammal. 90, 651–660. doi: 10.1644/08-MAMM-A-191R1.1
Monteith, K. L., Stephenson, T. R., Bleich, V. C., Conner, M. M., Pierce, B. M., and Bowyer, R. T. (2013). Risk-sensitive allocation in seasonal dynamics of fat and protein reserves in a long-lived mammal. J. Anim. Ecol. 82, 377–388. doi: 10.1111/1365-2656.12016
Morano, S., Stewart, K. M., Sedinger, J. S., Nicolai, C. A., and Vavra, M. (2013). Life-history strategies of north American elk: trade-offs associated with reproduction and survival. J. Mammal. 94, 162–172. doi: 10.1644/12-MAMM-A-074.1
Neumann, W., Singh, N. J., Stenbacka, F., Malmsten, J., Wallin, K., Ball, J. P., et al. (2020). Divergence in parturition timing and vegetation onset in a large herbivore-differences along a latitudinal gradient. Biol. Lett. 16:20200044. doi: 10.1098/rsbl.2020.0044
Nicholson, M. C., Bowyer, R. T., and Kie, J. G. (2006). Forage selection by mule deer: does nich vreadth increase with population density? J. Zool. 269, 39–49. doi: 10.1111/j.1469-7998.2006.00051.x
Nielsen, D. P., and Matocq, M. D. (2021). Differences in dietary composition and preference maintained despite gene flow across a woodrat hybrid zone. Ecol. Evol. 11, 4909–4919. doi: 10.1002/ece3.7399
Nielsen, S. E., Shafer, A. B. A., Boyce, M. S., and Stenhouse, G. B. (2013). Does learning or instinct shape habitat selection? PLoS One 8:e53721. doi: 10.1371/journal.pone.0053721
Oftedal, O. T. (1985). “Pregnancy and lactation” in Bioenergetics of Wild Herbivores. eds. R. J. Hudson and R. G. White (Boca Raton: CRC Press, Inc)
Oksanen, J., Blanchet, F. G., Kindt, R., Legendre, P., Minchin, P. R., Ohara, R. B., et al. (2013). Package ‘vegan’. Commun. Ecol. Pack. version 2, 1–295.
Palmer, W. C. (1965). Meteorological drought. Research paper number 45. Washington D.C.: US Department of Commerce.
Parker, K. L., Barboza, P. S., and Gillingham, M. P. (2009). Nutrition integrates environmental responses of ungulates. Funct. Ecol. 23, 57–69. doi: 10.1111/j.1365-2435.2009.01528.x
Parker, K. L., Gillingham, M. P., Hanley, T. A., and Robbins, C. T. (1999). Energy and protein balance of free-ranging black-tailed deer in a natural forest environment. Wildl. Monogr. 143, 3–48.
Pierce, B. M., Bowyer, R. T., and Bleich, V. C. (2004). Habitat selection by mule deer: forage benefits or risk of predation? J. Wildl. Manag. 68, 533–541. doi: 10.2193/0022-541X(2004)068[0533:HSBMDF]2.0CO;2
Plummer, M. (2003). JAGS: a program for analysis of Bayesian graphical models using Gibbs sampling. in Proceedings of the 3rd International Workshop on Distributed Statistical Computing, 20–23, March 2003, Technische Universität Wien, Vienna, Austria.
Poole, K. G., Serrouya, R., and Stuart-Smith, K. (2007). Moose calving strategies in interior montane ecosystems. J. Mammal. 88, 139–150. doi: 10.1644/06-MAMM-A-127R1.1
R Core Team. (2021). R: A language and environment for statistical computing. Vienna, Austria: R Foundation for Statistical Computing.
Rachlow, J. L., and Bowyer, R. T. (1998). Habitat selection by Dall’s sheep (Ovis dalli): maternal trade-offs. J. Zool. 245, 457–465. doi: 10.1017/S0952836998008097
Rad, E. B., Mesdaghi, M., Ahmad, N., and Abdullah, M. (2015). Nutritional quality and quantity of available forages relative to demand: a case study of the goitered gazelles of the Golestan National Park. Iran. Rangelands 37, 68–80. doi: 10.1016/j.rala.2015.01.004
Rominger, E. M., Dale, A. R., and Bailey, J. A. (1988). Shrubs in the summer diet of Rocky Mountain bighorn sheep. J. Wildl. Manag. 52, 47–50. doi: 10.2307/3801056
Scasta, J. D., Jorns, T., Derner, J. D., Lake, S., Augustine, D. J., Windh, J. L., et al. (2019). Validation of DNA metabarcoding of fecal samples using cattle fed known rations. Anim. Feed Sci. Technol. 255:114219. doi: 10.1016/j.anifeedsci.2019.114219
Schroeder, C. A., Bowyer, R. T., Bleich, V. C., and Stephenson, T. R. (2010). Sexual segregation in Sierra Nevada bighorn sheep, Ovis canadensis sierrae: ramifications for conservation. Arct. Antarct. Alp. Res. 42, 476–489. doi: 10.1657/1938-4246-42.4.476
Shank, C. C. (1982). Age-sex differences in the diets of wintering Rocky Mountain bighorn sheep. Ecology 63, 627–633. doi: 10.2307/1936781
Sikes, R. S., The Animal Care and Use Committee of the American Society of Mammalogists (2016). Guidelines of the American Society of Mammalogists for the use of wild mammals in research and education. J. Mammal. 97, 663–688. doi: 10.1093/jmammal/gyw078
Snyder, K. A., Wehan, B. L., Filippa, G., Huntington, J. L., Stringham, T. K., and Snyder, D. K. (2016). Extracting plant phenology metrics in a Great Basin watershed: methods and considerations for quantifying phenophases in a cold desert. Sensors 16:1948. doi: 10.3390/s16111948
Sousa, L. L., Silva, S. M., and Xavier, R. (2019). DNA metabarcoding in diet studies: unveiling ecological aspects in aquatic and terrestrial ecosystems. Environ. DNA 1, 199–214. doi: 10.1002/edn3.27
Stapleton, T. E., Weinstein, S. B., Greenhalgh, R., and Dearing, M. D. (2022). Successes and limitations of quantitative diet metabarcoding in a small, herbivorous mammal. Mol. Ecol. Resour. 22, 2573–2586. doi: 10.1111/1755-0998.13643
Stephenson, T. R., German, D. W., Cassirer, E. F., Walsh, D. P., Blum, M. E., Cox, M., et al. (2020). Linking population performance to nutritional condition in an alpine ungulate. J. Mammal. 101, 1244–1256. doi: 10.1093/jmammal/gyaa091
Stephenson, T. R., Testa, T. J., Adams, G. P., Sasser, S. R., Schwartz, C. C., and Hundertmark, K. J. (1995). Diagnosis of pregnancy and twinning in moose by ultrasonography and serum assay. Alces 31, 167–172.
Stewart, K. M., Bowyer, R. T., Dick, B. L., Johnson, B. K., and Kie, J. G. (2005). Density-dependent effects on physical condition and reproduction in north American elk: an experimental test. Oecologia 143, 85–93. doi: 10.1007/s00442-004-1785-y
Stewart, K. M., Bowyer, R. T., Dick, B. L., and Kie, J. G. (2011). Effects of density dependence on diet composition of north American elk Cervus elaphus and mule deer Odocoileus hemionus: an experimental manipulation. Wildl. Biol. 17, 417–430. doi: 10.2981/10-122
Taillon, J., Barboza, P. S., and Côté, S. D. (2013). Nitrogen allocation to offspring and milk production in a capital breeder. Ecology 94, 1815–1827. doi: 10.1890/12-1424.1
Tollefson, T. N., Shipley, L. A., Myers, W. L., Keisler, D. H., and Dasgupta, N. (2010). Influence of summer and autumn nutrition on body condition and reproduction in lactating mule deer. J. Wildl. Manag. 74, 974–986. doi: 10.2193/2008-529
Wagner, G. D., and Peek, J. M. (2006). Bighorn sheep diet selection and forage quality in Central Idaho. Northwest Sci. 80, 246–258.
Keywords: desert bighorn sheep, diet, Nevada, nutrition, Ovis canadensis nelsoni, parturition, reproduction, tradeoffs
Citation: Blum ME, Stewart KM, Cox M, Shoemaker KT, Bennett JR, Sullivan BW, Wakeling BF and Bleich VC (2023) Variation in diet of desert bighorn sheep (Ovis canadensis nelsoni): Tradeoffs associated with parturition. Front. Ecol. Evol. 10:1071771. doi: 10.3389/fevo.2022.1071771
Edited by:
Vicente Urios, University of Alicante, SpainReviewed by:
Kathleen Longshore, United States Department of the Interior, United StatesJuan F. Beltrán, Sevilla University, Spain
Copyright © 2023 Blum, Stewart, Cox, Shoemaker, Bennett, Sullivan, Wakeling and Bleich. This is an open-access article distributed under the terms of the Creative Commons Attribution License (CC BY). The use, distribution or reproduction in other forums is permitted, provided the original author(s) and the copyright owner(s) are credited and that the original publication in this journal is cited, in accordance with accepted academic practice. No use, distribution or reproduction is permitted which does not comply with these terms.
*Correspondence: Marcus E. Blum, ✉ marcus.blum@ag.tamu.edu