- 1Muping Coastal Environment Research Station, Yantai Institute of Coastal Zone Research, Chinese Academy of Sciences, Yantai, Shandong, China
- 2University of Chinese Academy of Sciences, Beijing, China
- 3Instituto Nacional de Investigación y Desarrollo Pesquero (INIDEP), Mar del Plata, Buenos Aires, Argentina
- 4Consejo Nacional de Investigaciones Científicas y Técnicas (CONICET), Buenos Aires, Argentina
- 5Shandong Key Laboratory of Marine Ecological Restoration, Shandong Marine Resource and Environment Research Institute, Yantai, Shandong, China
The genus Aurelia is one of the major contributors to jellyfish blooms in coastal waters, possibly due to its adaptive reproduction strategies. Different Aurelia lineages have adapted their reproduction modes to varying environmental conditions in their respective habitats. To understand the successful adaptation strategies, three strains of Aurelia coerulea and two strains of Aurelia solida polyps from different geographical areas were exposed to a range of temperatures and two food regimes, and the effects on reproduction rates were assessed. Asexual reproduction was significantly affected by the changes in these factors. The highest reproduction rate under sufficient food conditions was observed in the United States strain and the lowest was observed in the Israel strain, regardless of temperature, indicating the differences in the blooming potential. Six asexual reproduction modes were observed, of which lateral budding, lateral budding by means of stolons, and reproduction from parts of stolons were the main modes used by all Aurelia strains, except Aurelia solidaISR, for which reproduction by stolons was the main mode. The capability to switch reproductive strategies in response to environmental cues depending on the lineage predetermines the highly frequent blooming events of Aurelia.
1. Introduction
Conspicuous scyphozoans have received increasing attention in recent decades owing to their ability to form large blooms in their pelagic medusae stage, typically causing adverse effects on ecosystems and human activities (Condon et al., 2012; Graham et al., 2014). One of the most common blooming medusae is Aurelia, a genus comprising multiple cryptic species living in coastal and shelf-sea environments worldwide (Lawley et al., 2021). These cosmopolitan species have also been introduced in many regions worldwide (Dawson et al., 2005; Ki et al., 2008), further potentiating their expansion. Each local population is genetically differentiated and may have different reproductive traits as an adaptation to their habitats (Schroth et al., 2002). Moreover, each ecosystem is subject to environmental changes on various spatial and temporal scales. To cope with such variability, many organisms have evolved reproductive strategies that trade off their fitness in favor of risk reduction (Schnedler-Meyer et al., 2018).
Aurelia species are characterized by a metagenetic life cycle that comprises an alternation between sessile benthic polyps (hereafter referred to as polyps) and free-living pelagic medusae (Jarms, 2010). Polyps reproduce asexually, producing and releasing ephyrae through a process known as strobilation. In contrast to most scyphozoan species, where polyps propagate through one asexual mode (mono-mode species; Schiariti et al., 2014), Aurelia species present complex reproductive strategies that include various asexual modes that can even be simultaneously displayed to produce new polyps (multi-mode species; Schiariti et al., 2014). Although the final product of these propagation modes is always a new polyp, the use of a particular mode, or a combination of more than one, might have ecological implications related to the number of released medusae, and, consequently, the frequency and magnitude of jellyfish blooms. Although observations of the asexual reproduction of Aurelia species have accumulated in recent years (Lucas et al., 2012; Wang et al., 2012; Schiariti et al., 2014, 2015), the drivers of their diverse reproduction strategies and how they promote the maintenance of the polyp populations remain unclear.
Many researchers have identified the importance of temperature and food supply for the survival of Aurelia polyps (Ishii and Watanabe, 2003; Han and Uye, 2010; Wang et al., 2015; Wang and Li, 2015; Shi et al., 2016). In general, the asexual reproduction rates (Rr) and the number of new individuals increase with temperature (Han and Uye, 2010; Lucas et al., 2012); it is also believed that abundant nutrition increases the reproduction rates of Aurelia polyps (Ishii and Watanabe, 2003; Han and Uye, 2010; Kogovšek et al., 2010; Schiariti et al., 2014; Wang et al., 2015; Wang and Li, 2015). However, these studies were mostly focused on a single population of Aurelia polyps. Different Aurelia lineages might have unique adaptations concerning their reproductive capabilities and reproduction modes according to the environmental conditions of their respective habitats (Schiariti et al., 2014; Schnedler-Meyer et al., 2018), which may result in different blooming potentials. Therefore, understanding the asexual reproduction of Aurelia lineages in a broader population range in response to current environmental changes such as global warming and eutrophication, may effectively reveal the key drivers of jellyfish blooms, thereby providing vital fundamental to predict jellyfish-related disturbances in different regions.
In this study, we explored the effects of temperature and food availability on asexual reproduction rates and strategies of five strains of Aurelia polyps of different geographical origins. The polyps were exposed to three temperatures (10, 15, and 20°C) and two food regimes (starvation and feeding ad libitum), and the corresponding effects on Rr and strategies were measured. Experimental temperatures were selected based on the species culture conditions to ensure favorable temperature ranges for all studied strains.
2. Materials and methods
2.1. Origin of stem cultures and pre-experimental conditions
The specimens of three strains of A. coerulea and two strains of A. solida of different geographical origins were collected in their natural environment or from planulae-bearing medusae (Supplementary Table S1), and reared in laboratory conditions. The polyps were identified through a phylogenetic analysis of 16S rRNA gene sequences (Ender and Schierwater, 2003; Peng et al., 2021; Supplementary Table S1; Figure 1), using the maximum likelihood approach and General Time Reversible model with 1,000 bootstrap replicates (Nei and Kumar, 2000). Evolutionary analyses were conducted using the MEGA X software (Kumar et al., 2018). Reference 16S rRNA sequences of another Aurelia spp. were obtained from the NCBI database.
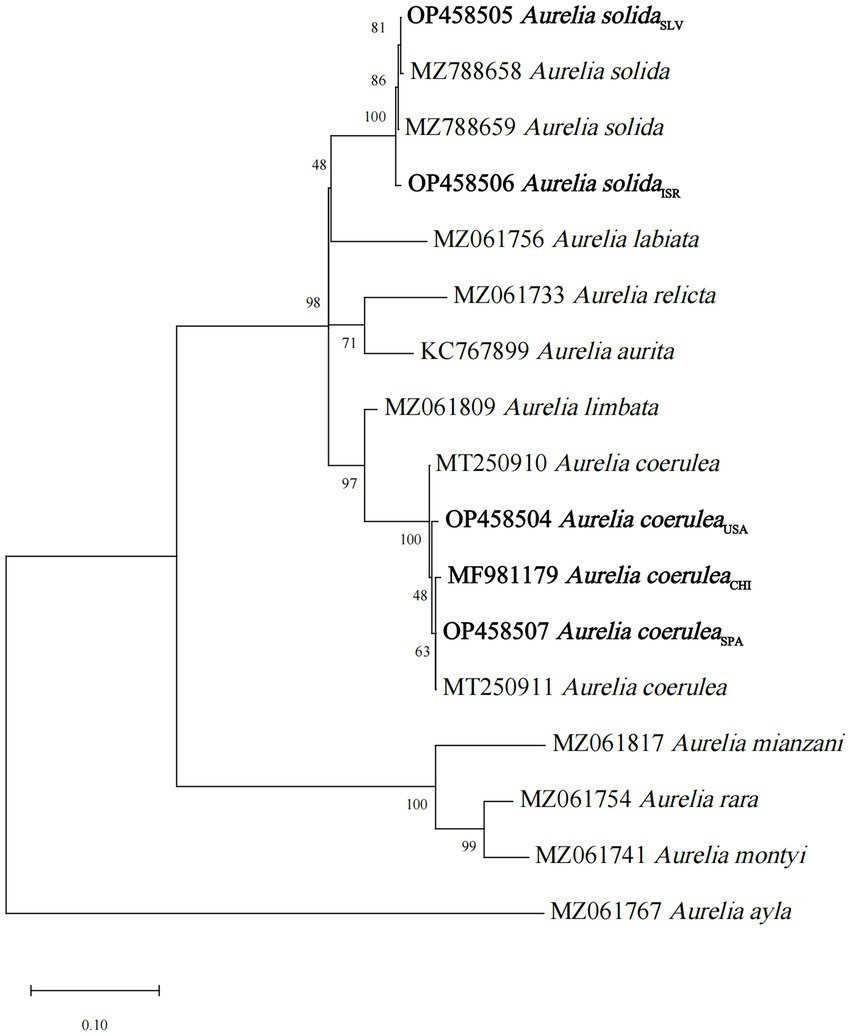
Figure 1. Maximum-likelihood tree for 16S rRNA gene sequences of the studied Aurelia scyphistomae strains.
Stem cultures were maintained inside incubators in dark conditions, and placed in plastic bottles (100 ml) filled with natural seawater (15°C, 32 PSU) filtered through a 0.45 μm membrane. They were fed newly hatched Artemia salina nauplii twice a week. The water was replaced 2–3 h after each feed, using a straw to avoid disturbing the surroundings or disrupting the attachment. Algae or shrimp that were attached to plastic bottles were removed using toothpicks and straws.
2.2. Experimental design and procedures
The combined effects of temperature and food supply were separately observed for each Aurelia strain. Individuals were exposed to 10, 15, and 20°C which were maintained by three independent cryogenic light incubators (GXZ, China), and to two feeding conditions, starvation and feeding ad libitum. Each experimental unit included one individual polyp with six replicates for a total of 36 initial polyps per strain. Fully developed polyps showing no external evidence of reproduction were detached from stem cultures and placed in experimental units (plastic dishes containing 50 ml of filtered seawater). Polyps were allowed to reattach and acclimate to the experimental temperatures for 1 week without food. They were maintained under dark conditions, except during observations (approximately 30 min) and water changes.
The corresponding experimental units were fed twice a week with the freshly hatched A. salina nauplii. Briefly, 2 h after each feed, water, debris, and uneaten food were discarded and replaced with filtered seawater of the corresponding temperature and salinity. In all the cases, the presence of food during the feeding period guaranteed the desired ad libitum conditions. Water was changed at the same time for the starvation groups.
Before feeding, the following variables were recorded under a stereoscopic microscope (OLYMPUS SZX10, Japan): (i) specific asexual reproduction modes; (ii) the total number of reproductive products to identify overall Rr; and (iii) the number of each type of reproduction product to identify specific Rr. Different asexual reproduction modes were identified following Adler and Jarms (2009) and Schiariti et al. (2014). All reproductive products unable to feed (motile or nonmotile, attached or detached from the mother polyp) were considered for the measurements of variables (ii) and (iii), respectively. Once tentacles had developed and feeding started, the products were considered new polyps and were picked out using forceps to avoid an increase in polyp density. The experiments were conducted for 6 weeks.
2.3. Data analysis
The null hypotheses were examined using the two-way ANOVA or Kruskal–Wallis tests depending on whether each data set met the assumptions; variance homogeneity and positive residual error were evaluated using the Levene test and Shapiro–Wilk test, respectively. In the case of significant differences, Tukey’s or Dunn’s post hoc tests were performed. In the cases of low to null reproduction or high mortality, the collected data were not tested against the studied factors as it was impossible to determine the interaction owing to missing data (no polyps or no reproductive products). In these cases, we used a t-test, one-way ANOVA, or non-parametric Mann–Whitney and Kruskal-Wallis tests, depending on whether each dataset met the assumptions. SPSS Statistics version 19 (IBM, Armonk, NY, United States) was used to perform all tests.
3. Results
We observed the following asexual reproduction modes (Figure 2A): typical lateral budding (LB), lateral budding by means of stolons (LBst), reproduction from the parts of stolons/stalks (ST), motile bud-like tissue particles (MP), podocysts (POD), and strobilation (STR), which were comprehensively described by Adler and Jarms (2009) and Schiariti et al. (2014). The number of polyp-to-polyp asexual reproduction modes displayed by each strain varied between four and five; all strains produced new polyps by means of LB, LBst, ST, and POD (Figure 2B). MP was formed by A. coeruleaUSA, A. coeruleaSPA and A. solidaISR. STR was only observed in A. coeruleaUSA. The survival rate of Aurelia polyps was 100% in all treatment combinations for all strains, indicating an overall tolerance to the experimental conditions.
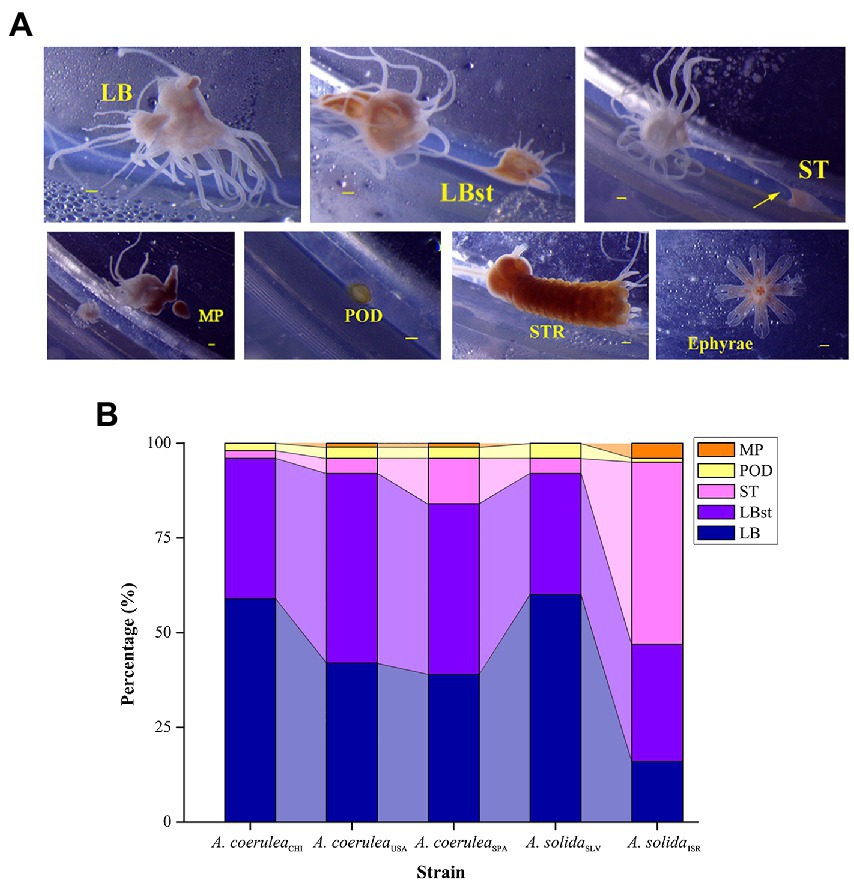
Figure 2. (A) Different asexual reproduction modes within Aurelia. LB: typical lateral budding; LBst: lateral budding by means of stolons; ST: reproduction from parts of stolons; MP: motile bud-like tissue particles; POD: podocysts; STR: strobilation. Scale bars = 100 μm. (B) Proportions of each reproduction mode within the total reproduction. LB: typical lateral budding; LBst: lateral budding by means of stolons; ST: reproduction from parts of stolons; MP: motile bud-like tissue particles; POD: podocysts.
3.1. Effects of temperature and food supply on polyp-to-polyp Rr and reproductive strategies
Rr significantly increased with food supply and temperature, except in A. coeruleaSPA, where this effect was not significant (Table 1; Figure 3). Rr generally increased with increasing temperature in polyps that were fed (Tukey’s post hoc test, p < 0.01; Figure 3) and the effects on starved polyps were not significant (Tukey’s post hoc test, p > 0.05; Figure 3). However, in A. coeruleaSPA, the effect of temperature was not significant regardless of the food supply (Tukey’s post hoc test, p > 0.05). However, Rr was higher when food was available for all strains and temperatures (Tukey’s post hoc test, p < 0.01), except for A. solidaISR at 10°C, where the effect of food was not significant (Tukey’s post hoc test, p > 0.05; Figure 3).
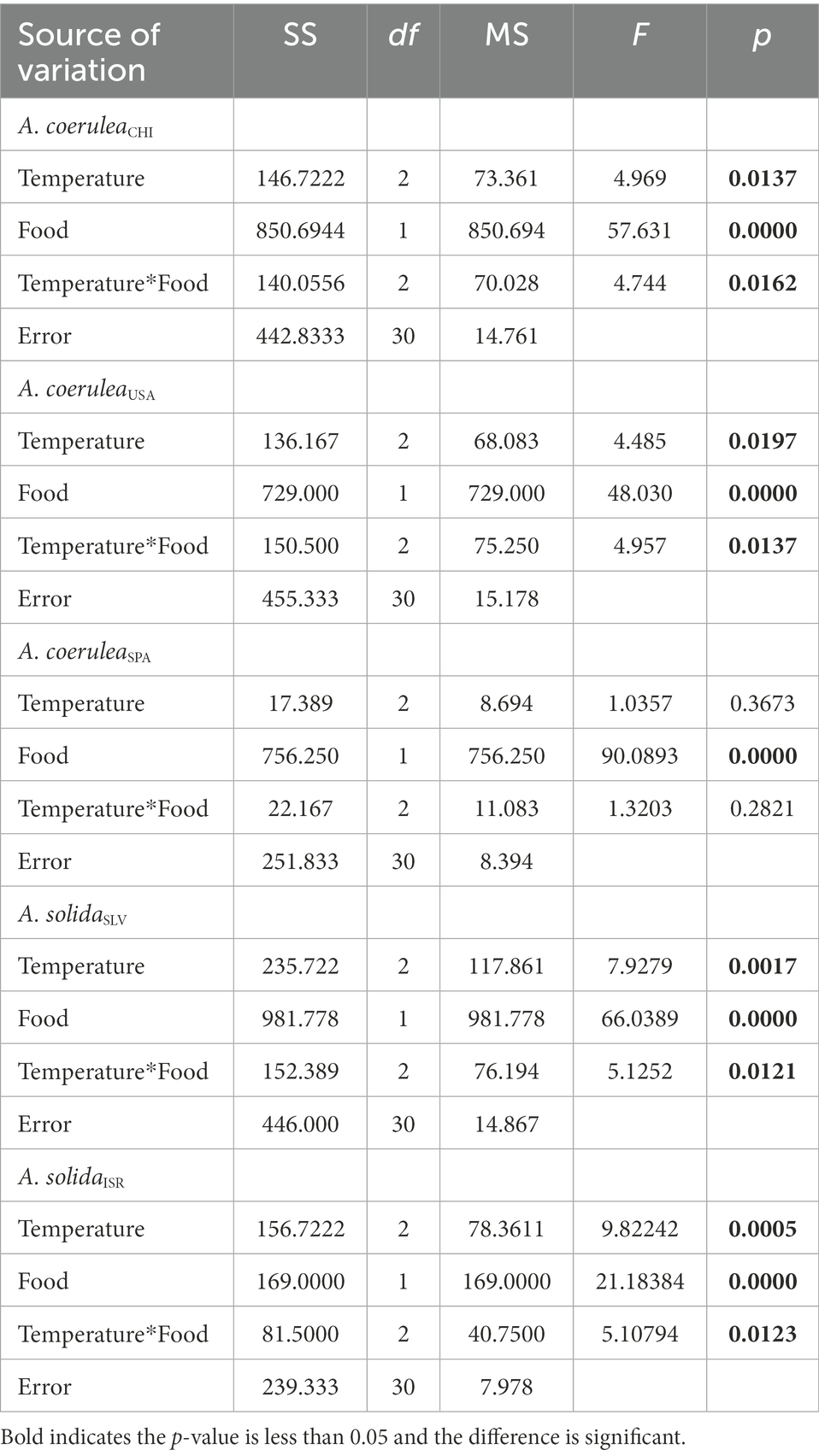
Table 1. Results of two-way ANOVA examining the effects of temperature and food supply on asexual reproduction rates of Aurelia scyphistomae species/strains.
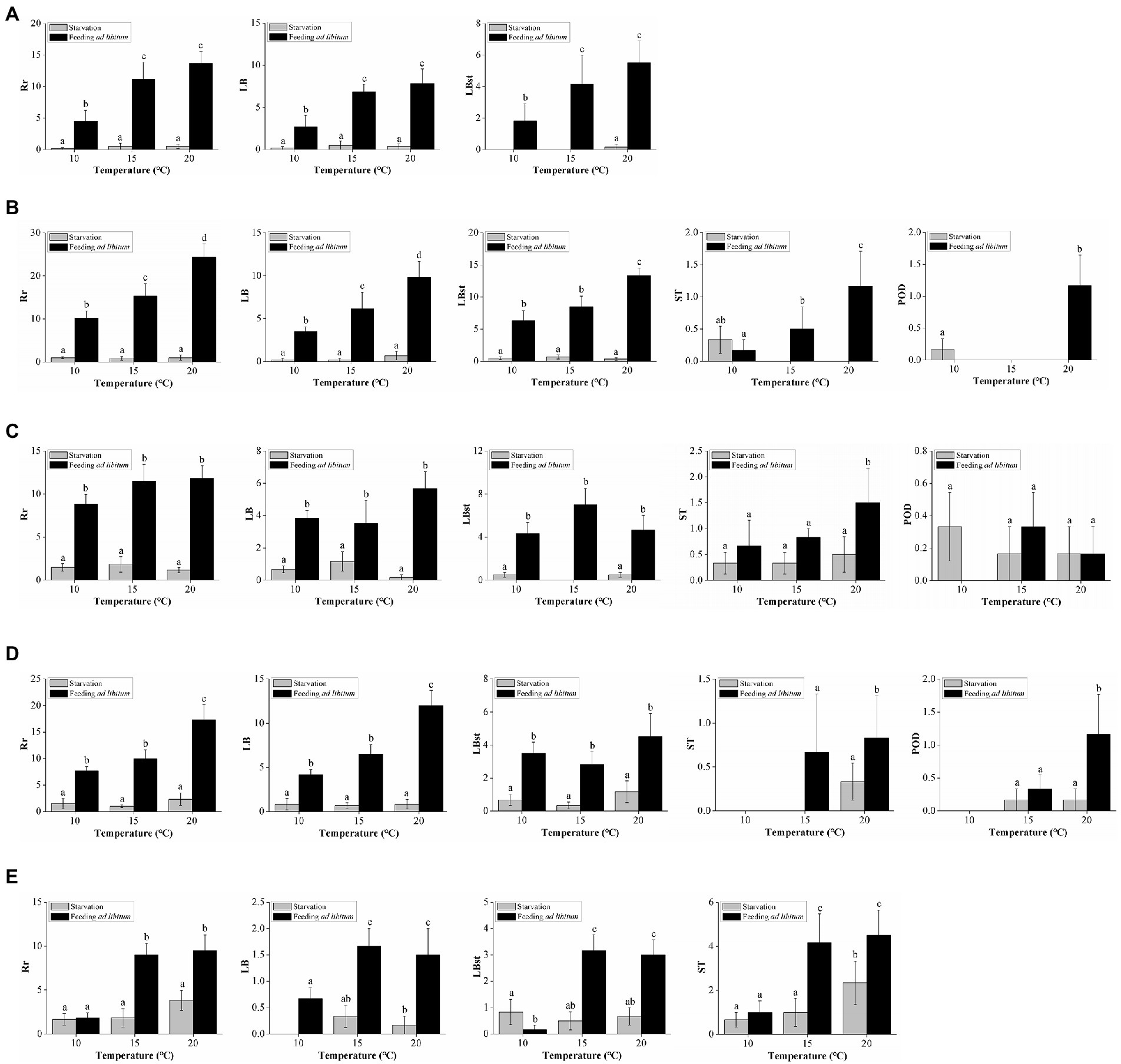
Figure 3. Effects of temperature and food supply on overall and specific reproduction rates of (A) Aurelia coeruleaCHI, (B) A. coeruleaUSA, (C) A. coeruleaSPA, (D) A. solidaSLV, (E) A. solidaISR. Rr: number of new polyps produced during the experiment; LB: number of polyps produced by typical lateral budding; LBst: number of polyps produced by lateral budding by means of stolons; ST: number of polyps produced from parts of stolons/stalks; POD: number of podocysts produced during the experiment. Data are mean numbers of each variable ± standard deviation. Different letters represent significant differences at α = 0.05.
Our experimental design did not allow for a statistical comparison of strains. However, it was possible to observe different trends in Rr A. coeruleaUSA reached the highest Rr, followed by similar values obtained by of A. coeruleaSPA and A. solidaSLV, and finally followed by A. coeruleaCHI and A. solidaISR. Despite these apparent differences in the total number of reproductive particles produced, the reproductive strategy displayed by each strain was similar for all, except A. solidaISR.
Approximately 96% of the reproductive particles produced by A. coeruleaCHI corresponded to LB (59%) and LBst (37%). The effects of temperature and food supply on the specific asexual modes followed the same pattern as observed in the Rr analysis (Figure 3A). Numbers of produced ST (2%; n = 3) and POD (2%; n = 4) were too low to define any trends. No excystment was observed in the produced POD. No MP was produced by A. coeruleaCHI.
Approximately 92% of the reproductive particles produced by A. coeruleaUSA corresponded to LBst (50%) and LB (42%). The effects of temperature and food supply on these dominant asexual modes followed the same pattern as observed in the Rr analysis; values proportionally increased with increasing food availability at all temperatures, and with temperature, in the polyps that were fed (Figure 3B). ST, POD, and MP accounted for 8% of the total reproduction of this strain (Figure 2B). No effect of food supply was observed on ST at 10°C (Tukey’s post hoc test, p > 0.05; Figure 3B). No ST was produced by starved polyps at either 15°C or 20°C. The number of ST increased with temperature when food was available (Tukey’s post hoc test, p < 0.05; Figure 3B). Despite the low number of POD produced by this strain, a significant effect of temperature and food supply was observed (Tukey’s post hoc test, p < 0.05; Figure 3B). The number of MPs were too low to describe a trend (n = 2). No excystment was observed in POD.
Approximately 84% of the reproductive particles produced by A. coeruleaSPA corresponded to LBst (45%) and LB (39%). Numbers of particles in these modes increased with food availability, regardless of temperature (Figure 3C). ST, POD, and MP accounted for 16% of the total reproduction modes of this strain (Figure 2B). The effects of temperature and food supply on ST were not significant (Tukey’s post hoc test, p > 0.05), except for the fed polyps at 20°C. No excystment was observed in the produced POD, and MP were observed but at very low numbers to describe a trend (n = 3).
Approximately 92% of the reproductive particles produced by A. coeruleaSLV corresponded to LB (60%) and LBst (32%). The effects of temperature and food availability on LB followed the same pattern as in the Rr analysis, with values increasing with food availability at each temperature and increasing with temperature in the fed polyp groups only (Figure 3D). The effect of temperature on LBst production was not significant (two-way ANOVA, p > 0.05). The ST and POD accounted for 8% of the total reproductive particles of this strain (Figure 2B). ST was only observed at 15°C when food was available and at 20°C in both feeding regimes, however, the effects of temperature and food supply on this mode were not significant (Mann–Whitney test, p > 0.05; Figure 3D). No POD was produced at 10°C; a significant effect of temperature and food availability was observed, as a maximum value was reached at 20°C when food was available (Tukey’s post hoc test, p < 0.05; Figure 3D). No excystment was observed in POD. No MP were detected in this strain.
Despite some specific differences among these four Aurelia strains, all produced more than 84% of their reproductive particles by lateral budding (LB + LBst). However, A. solidaISR presented a different reproductive strategy, producing 48% of the reproductive particles in ST and 47% in both types of lateral buds (31% LBst and 16% LB). The effects of temperature and food availability on ST followed a similar pattern to that described for Rr, with values increasing with food supply at 15°C and 20°C, and with temperature in both feeding conditions (Figure 3E). The number of POD and MP accounted for was too low to describe a trend. No excystment was observed in POD.
3.2. Effects of temperature and food supply on strobilation
Strobilation was observed in A. coeruleaUSA only at 10°C in both feeding treatments, with 5/6 strobilae formed in the starvation mode and 4/6 in the feeding mode; the differences between the number of strobilations observed in each treatment and ephyrae produced by fed and starved polyps were not significant (Student’s t-test, p > 0.05). Polyps were only strobilated during the experimental period. Between 10 and 24 ephyrae were produced per strobilation when food was provided, and between 4 and 13 ephyrae were produced per strobilation when starved; the differences between the numbers of ephyrae per strobilation observed in each treatment were not significant (Student’s t-test, p > 0.05).
4. Discussion
The results of this study suggest that temperature and food supply seem to be the environmental factors that influence the Rr of Aurelia polyps, which determine the potential number of medusae released into the pelagic realm. The different responses of each strain may serve as the indicators of their potential to cause outbreaks. The highest Rr under sufficient food conditions regardless of temperature was observed in the United States strain, suggesting that jellyfish blooms are more likely to occur in this area than in any other tested areas; the Israel strain is the least likely to cause a jellyfish bloom, as it had the lowest Rr. Several laboratory experiments have investigated the effects of these factors on polyp reproduction by exposing polyps to different conditions, and most studies have revealed accelerated and increased production of new polyps in response to warm temperatures and high food availability (e.g., Purcell, 2007; Lucas et al., 2012; Schiariti et al., 2014), which are consistent with the results obtained in our study.
Food supply had the most significant effect on Rr, with starved polyps producing considerably few new polyps (or none). The importance of food supply in Aurelia polyp reproduction has been previously observed for different species and locations, indicating that higher food concentration promotes Rr (Lucas et al., 2012 and references therein). The contrasting feeding conditions used in this study did not allow for testing the gradual effects of food concentration but confirmed that a certain nutritional state is required for polyp reproduction. Nevertheless, mortality was null in all experiments, suggesting a high tolerance of these strains to unfavorable conditions. Although reproduction was minimal or null, 100% survival indicated that Aurelia polyps could tolerate starvation for 7 weeks within the studied temperature range. The ability to tolerate hunger has been observed in Aurelia polyps, even for considerably longer periods (up to 60 days; Spangenberg, 1967; Shick, 1975; Sun et al., 2017). However, the water that was filtered through a 0.45 μm mixed membrane might contain a certain concentration of picoplankton and bacteria that seeped through, providing nutrition for the livelihood of polyps (Kamiyama, 2011).
Without food limitations, the effect of temperature on Rr was clearly observed, with higher Rr being observed at warmer temperatures (Figure 3). This trend has been confirmed in several Aurelia species (most of them identified in the literature as A. aurita) both in the laboratory (Kakinuma, 1975 Keen, 1991; Han and Uye, 2010; Schiariti et al., 2014) and in field conditions (Gröndahl, 1988; Watanabe and Ishii, 2001; Willcox et al., 2008), with only few studies have reported the opposing trend, where fewer polyps were observed at warmer temperatures (Purcell, 2007; Winans and Purcell, 2010). These contrasting patterns have been attributed to the adaptations of Aurelia spp. to specific environments (Widmer et al., 2016). However, the lower budding rates at warmer temperatures reported by Purcell (2007), and Winans and Purcell (2010), corresponded to treatments where higher strobilation rates were observed. Therefore, although several species can simultaneously strobilate and produce new polyps, the available energy would have been utilized for ephyrae production at the expense of polyp production. In our study strobilation was only observed in A. coeruleaUSA at low rates, and therefore, the effect on the response of Rr could be neglected.
The positive effects of temperature and food supply (as a proxy for nutritional state) on asexual Rr of polyps are common not only in Aurelia but also in different species of Scyphozoa. When food is sufficient, Aurelia uses excess energy to expand the polyp population rather than support individual livelihood (Lucas, 2001). However, when the stimulus for strobilation is present, polyps might start producing ephyrae at the expense of polyp Rr. Temperature changes (mostly a decrease in temperature) can trigger strobilation in several Aurelia species/strains (Kroiher et al., 2000; Fuchs et al., 2014; Sukhoputova and Kraus, 2017). In our study, all polyps were kept at 15°C during the acclimation period before the experiment, and one-third of them then suffered a 5°C temperature decrease when kept at 10°C. The strobilation observed in A. coeruleaUSA was likely triggered by this stimulus. However, the formation of ephyrae is not only induced by temperature changes but also by particular combinations of environmental factors specific to each strain (Berrill, 1949; Russell, 1970). The presence of only one of these factors alone is not sufficient to induce strobilation.
Our results confirmed previous experimental observations, indicating that Aurelia polyps can display most of the asexual reproduction modes described for Scyphozoa (Adler and Jarms, 2009). We also found that Aurelia strains produced new polyps mostly via non-motile buds (LB + LBst) and ST. Within optimal temperature and food availability ranges, Aurelia polyps propagate almost exclusively through these modes, colonizing substrates very rapidly (Han and Uye, 2010; Schiariti et al., 2014, 2015). However, room for expansion soon reaches a limit, and available substrate becomes a limiting factor that controls population size, reproductive output, and survival of polyps, similar to many other sessile marine invertebrates (Müller and Leitz, 2002; Purcell, 2007; Schiariti et al., 2015).
Utilization of these modes (mostly the budding types) allows rapid colonization of available substrates, reaching an average of 4 (A. solidaISR)-8 (A. coeruleaUSA) polyps per day. As described by Schiariti et al. (2014), the formation of a new polyp takes between 3 and 5 days from the first signs of the bud to its detachment. A new bud develops its tentacles and starts feeding before detachment, becoming a double-headed polyp with elevated feeding rates, allowing increased energy storage for reproductive and somatic growth. The same pattern was described by Han and Uye (2010) for A. aurita s.l. These rapid reproduction modes are utilized by all Aurelia species described so far when there is room for the attachment of new polyps (A. coerulea, A. solida, A. aurita, A. limbata, and A. labiata; Coyne, 1973; Keen and Gong, 1989; Han and Uye, 2010; Schiariti et al., 2014, 2015). However, Aurelia starts developing MP after a certain threshold of density is achieved (carrying capacity; Melica et al., 2014); therefore, the permanent low-density population during our experiment would explain the low rates of MP production observed for all strains. Thus, the multi-mode reproduction strategy allows Aurelia to rapidly colonize the available substrate rapidly in favorable conditions, encyst and withstand adverse conditions, and develop motile buds or detach to facilitate dispersion and avoid the negative effects of inter-or intraspecific competition for space and food.
In summary, temperature and nutrition play vital roles in regulating asexual reproduction of Aurelia strains. Starvation appeared to be a common limiting factor for reproduction at all experimental temperature values. However, temperature also affected Rr, with a higher number of produced polyps at higher temperatures. Therefore, the combined effects of temperature and food supply may synergistically affect polyp reproduction and density, both positively and negatively. Furthermore, the multi-mode reproduction strategy allows Aurelia to adapt to the changes in the environment. The capability to switch reproductive strategies in response to environmental cues gives Aurelia high adaptability (Schiariti et al., 2014, 2015). These features, in addition to the high tolerance of Aurelia polyps and medusae to changing environmental conditions (Lucas, 2001; Lucas et al., 2012), describe the high frequency of blooming events of the cosmopolitan Aurelia lineages.
Data availability statement
The original contributions presented in the study are included in the article/Supplementary material, further inquiries can be directed to the corresponding author.
Ethics statement
The authors declare that sampling of specimens was performed in the respect of ethical rules and Chinese laws. No approval of research ethics committees was required to accomplish the goals of this study because experimental work was conducted with an unregulated invertebrate species.
Author contributions
ZD, AS, and JZ contributed to the experimental designs of the manuscript. SX and FW conducted the experiment. FW, AS, SX, YM, TS, LW, and ZD contributed to the analysis of the data and writing of the manuscript. All authors contributed to the article and approved the submitted version.
Funding
This work was supported by grants from the National Natural Science Foundation of China (No. 41876138), Strategic Priority Research Program of the Chinese Academy of Sciences (No. XDA23050301), the National Science and Technology Fundamental Resources Investigation Program of China (2022FY100603), the Key Project of NSFC-Shandong Joint Fund (U2106208), Instrument Developing Project of the Chinese Academy of Sciences (No. YJKYYQ20180047), the Taishan Scholars Program, and FONCyT PICT 2018–03872.
Acknowledgments
We wish to thank the Marine Biology Station Piran, National Institute of Biology (Slovenia), Institut de Ciencies del Mar, CSIC, Barcelona (Spain), and the University of São Paulo (Brazil) for providing Aurelia polyp strains.
Conflict of interest
The authors declare that the research was conducted in the absence of any commercial or financial relationships that could be construed as a potential conflict of interest.
Publisher’s note
All claims expressed in this article are solely those of the authors and do not necessarily represent those of their affiliated organizations, or those of the publisher, the editors and the reviewers. Any product that may be evaluated in this article, or claim that may be made by its manufacturer, is not guaranteed or endorsed by the publisher.
Supplementary material
The Supplementary material for this article can be found online at: https://www.frontiersin.org/articles/10.3389/fevo.2022.1071518/full#supplementary-material
References
Adler, L., and Jarms, G. (2009). New insights into reproductive traits of scyphozoans: special methods of propagation in Sanderia malayensis GOETHE, 1886 (Pelagiidae, Semaeostomeae) enable establishing a new classification of asexual reproduction in the class Scyphozoa. Mar. Biol. 156, 1411–1420. doi: 10.1007/s00227-009-1181-6
Berrill, N. J. (1949). Developmental analysis of scyphomedusae. Biol. Rev. 24, 393–409. doi: 10.1111/j.1469-185X.1949.tb00581.x
Condon, R. H., Graham, W. M., Duarte, C. M., Pitt, K. A., Lucas, C. H., Haddock, S. H. D., et al. (2012). Questioning the rise of gelatinous zooplankton in the world’s oceans. Bioscience 62, 160–169. doi: 10.1525/bio.2012.62.2.9
Coyne, J. A. (1973). An investigation of the dynamics of population growth and control in scyphistomae of the scyphozoan Aurelia aurita. Chesap. Sci. 14, 55–58. doi: 10.2307/1350704
Dawson, M. N., Gupta, A. S., and England, M. H. (2005). Coupled biophysical global ocean model and molecular genetic analyses identify multiple introductions of cryptogenic species. PNAS 102, 11968–11973. doi: 10.1073/pnas.0503811102
Ender, A., and Schierwater, B. (2003). Placozoa are not derived cnidarians: evidence from molecular morphology. Molec. Biol. Evol. 20, 130–134. doi: 10.1093/molbev/msg018
Fuchs, B., Wang, W., Graspeuntner, S., Li, Y., Insua, S., Herbst, E. M., et al. (2014). Regulation of polyp-to-jellyfish transition in Aurelia aurita. Curr. Biol. 24, 263–273. doi: 10.1016/j.cub.2013.12.003
Graham, W. M., Gelcich, S., Robinson, K. L., Duarte, C. M., Brotz, L., Purcell, J. E., et al. (2014). Linking human well-being and jellyfish: ecosystem services, impacts, and societal responses. Front. Ecol. Environ. 12, 515–523. doi: 10.1890/130298
Gröndahl, F. (1988). Interactions between polyps of Aurelia aurita and planktonic larvae of scyphozoans: an experimental study. Mar. Ecol. Prog. Ser. 45, 87–93. doi: 10.3354/meps045087
Han, C. H., and Uye, S. I. (2010). Combined effects of food supply and temperature on asexual reproduction and somatic growth of polyps of the common jellyfish Aurelia aurita sl. Plankton Benthos Res. 5, 98–105. doi: 10.3800/pbr.5.98
Ishii, H., and Watanabe, T. (2003). Experimental study of growth and asexual reproduction in Aurelia aurita polyps. Sess. Org. 20, 69–73. doi: 10.4282/sosj.20.69
Jarms, G. (2010). The early life history of Scyphozoa with emphasis on Coronatae. A review with a list of described life cycles. Verh. Naturwiss. Ver. Hamburg. 45, 17–31.
Kakinuma, Y. (1975). An experimental study of the life cycle and organ differentiation of Aurelia aurita Lamarck. Bull. Biol. Stn Asamushi. 15, 101–114. doi: 10.1093/gbe/evt048
Kamiyama, T. (2011). Planktonic ciliates as a food source for the scyphozoan Aurelia aurita (s.l.): feeding activity and assimilation of the polyp stage. J. Exp. Mar. Biol. Ecol. 407, 207–215. doi: 10.1016/j.jembe.2011.06.016
Keen, S. L. (1991). Clonal Dynamics and Life history Evolution in the Jellyfish Aurelia aurita. dissertation. Los Angeles, CA: University of California.
Keen, S. L., and Gong, A. J. (1989). Genotype and feeding frequency affect clone formation in a marine cnidarian (Aurelia aurita Lamarck 1816). Funct. Ecol. 3, 735–745. doi: 10.2307/2389506
Ki, J. S., Hwang, D. S., Kyoungsoon, S., Duk, Y. W., Donghyun, L., Kang, Y. S., et al. (2008). Recent moon jelly (Aurelia sp. 1) blooms in Korean coastal waters suggest global expansion: examples inferred from mitochondrial COI and nuclear ITS-5.8 S rDNA sequences. ICES J. Mar. Sci. 65, 443–452. doi: 10.1093/icesjms/fsn018
Kogovšek, T., Bogunovi, B., and Malej, A. (2010). Recurrence of bloom−forming scyphomedusae: wavelet analysis of a 200−year time series. Hydrobiologia 645, 81–96. doi: 10.1007/s10750-010-0217-8
Kroiher, M., Siefker, B., and Berking, S. (2000). Induction of segmentation in polyps of Aurelia aurita (Scyphozoa, Cnidaria) into medusae and formation of mirror-image medusa analgen. Int. J. Dev. Biol. 44, 485–490. doi: 10.1387/IJDB.11032183
Kumar, S., Stecher, G., Li, M., Knyaz, C., and Tamura, K. (2018). MEGA X: molecular evolutionary genetics analysis across computing platforms. Mol. Biol. Evol. 35, 1547–1549. doi: 10.1093/molbev/msy096
Lawley, J. W., Gamero-Mora, E., Maronna, M. M., Chiaverano, L. M., Stampar, S. N., Hopcroft, R. R., et al. (2021). The importance of molecular characters when morphological variability hinders diagnosability: systematics of the moon jellyfish genus Aurelia (Cnidaria: Scyphozoa). PeerJ 9:e11954. doi: 10.7717/peerj.11954
Lucas, C. H. (2001). “Reproduction and life history strategies of the common jellyfish, Aurelia aurita, in relation to its ambient environment,” in Jellyfish Blooms: Ecological and Societal Importance. eds. J. E. Purcell, W. M. Graham, and H. J. Dumont (Dordrecht: Springer).
Lucas, C. H., Graham, W. M., and Widmer, C. (2012). “Jellyfish life histories: role of polyps in forming and maintaining scyphomedusa populations,” in Advances in Marine Biology. ed. M. Lesser (London: Academic Press), 133–196.
Melica, V., Invernizzi, S., and Caristi, G. (2014). Logistic density-dependent growth of an Aurelia aurita polyps population. Ecol. Model. 291, 1–5. doi: 10.1016/j.ecolmodel.2014.07.009
Müller, W. A., and Leitz, T. (2002). Metamorphosis in the cnidaria. Can. J. Zool. 80, 1755–1771. doi: 10.1139/z02-130
Nei, M., and Kumar, S. (2000). Molecular Evolution and Phylogenetics. New York, NY: Oxford University Press.
Peng, S. J., Liu, Q. Q., Wang, L., Sun, T. T., Shiganova, T., and Dong, Z. J. (2021). Molecular identification and population differentiation of Aurelia spp. ephyrae in sea cucumber aquaculture ponds of northern China. J. Ocean Limnol. 39, 989–996. doi: 10.1007/s00343-020-0022-9
Purcell, J. E. (2007). Environmental effects on asexual reproduction rates of the scyphozoan Aurelia labiate. Mar. Ecol. Prog. Ser. 348, 183–196. doi: 10.3354/meps07056
Russell, F. S. (1970). The Medusae of the British Isies. Volume 1I. Pelagic Scyphozoa, With a Supplement to the First Volume on Hydromedusae Cambridge. London: Cambridge University Press.
Schiariti, A., Melica, V., Kogovšek, T., and Malej, A. (2015). Density-dependent effects control the reproductive strategy and population growth of Aurelia aurita s.l. scyphistomae. Mar. Biol. 162, 1665–1672. doi: 10.1007/s00227-015-2704-y
Schiariti, A., Morandini, A. C., Jarms, G., Paes, R. V. G., Franke, S., and Mianzan, H. (2014). Asexual reproduction strategies and blooming potential in Scyphozoa. Mar. Ecol. Prog. Ser. 510, 241–253. doi: 10.3354/meps10798
Schnedler-Meyer, N. A., Pigolotti, S., and Mariani, P. (2018). Evolution of complex asexual reproductive strategies in jellyfish. Am. Nat. 192, 72–80. doi: 10.1086/697538
Schroth, W., Jarms, G., Streit, B., and Schierwater, B. (2002). Speciation and phylogeography in the cosmopolitan marine moon jelly. BMC Ecol, Evol. 2, 1–10. doi: 10.1186/1471-2148-2-1
Shi, Y., Mi, T. Z., Wang, J. Y., Chen, L. H., Wang, G. S., Zhen, Y., et al. (2016). The effect of temperature and food supply on the asexual reproduction of moon jelly (Aurelia sp. 1) polyps. Acta Ecol. Sin. 36, 786–794. doi: 10.5846/stxb201404170751
Shick, J. M. (1975). Uptake and utilization of dissolved glycine by Aurelia aurita scyphistomae: temperature effects on the uptake process; nutritional role of dissolved amino acids. Biol. Bull. 148, 117–140. doi: 10.2307/1540654
Spangenberg, D. B. (1967). Iodine induction of metamorphosis in Aurelia. J. Exp. Zool. 165, 441–449. doi: 10.1002/jez.1401650312
Sukhoputova, A. V., and Kraus, Y. A. (2017). Environmental factors inducing the transformation of polyp into medusae in Aurelia aurita (Scyphozoa). Russ. J. Dev. Bio. 48, 106–116. doi: 10.1134/s1062360417020072
Sun, M., Chai, Y., Dong, J., Fu, Z. L., Liu, Z. G., Lin, J. Q., et al. (2017). Effects of environmental factors on polyp survival and reproduction of Aurelia sp. 1. Acta Ecol. Sin. 37, 1309–1317. doi: 10.5846/stxb201606301323
Wang, N., and Li, C. L. (2015). The effect of temperature and food supply on the growth and ontogeny of Aurelia sp. 1 ephyrae. Hydrobiologia 754, 157–167. doi: 10.1007/s10750-014-1981-7
Wang, Y. T., Sun, S., and Li, C. L. (2012). Effects of temperature and food on asexual reproduction of the scyphozoan, Aurelia sp. 1 (in Chinese with English abstract). Oceanologia et Limnologia Sinica 43, 900–904. doi: 10.11693/hyhz201205004004
Wang, Y. T., Zheng, S., Sun, S., and Zhang, F. (2015). Effect of temperature and food type on asexual reproduction in Aurelia sp. 1 polyps. Hydrobiologia 754, 169–178. doi: 10.1007/S10750-014-2020-4
Watanabe, T., and Ishii, H. (2001). “In situ estimation of ephyrae liberated from polyps of Aurelia aurita using settling plates in Tokyo Bay, Japan,” in Jellyfish Blooms: Ecological and Societal Importance. eds. J. E. Purcell, W. M. Graham, and H. J. Dumont (Dordrecht: Springer), 247–258.
Widmer, C. L., Fox, C. J., and Brierley, A. S. (2016). Effects of temperature and salinity on four species of northeastern Atlantic scyphistomae (Cnidaria: scyphozoa). Mar. Ecol. Prog. Ser. 559, 73–88. doi: 10.3354/meps11879
Willcox, S., Moltschaniwskyj, N. A., and Crawford, C. M. (2008). Population dynamics of natural colonies of Aurelia sp. scyphistomae in Tasmania, Australia. Mar. Biol. 154, 661–670. doi: 10.1007/s00227-008-0959-2
Keywords: Aurelia sp., jellyfish blooms, asexual reproduction, adaptation, polyps
Citation: Wang F, Schiariti A, Xu S, Ma Y, Sun T, Wang L, Zhao J and Dong Z (2023) Asexual reproduction strategies in the moon jellyfish Aurelia (Cnidaria: Scyphozoa). Front. Ecol. Evol. 10:1071518. doi: 10.3389/fevo.2022.1071518
Edited by:
David Tarkhnishvili, Ilia State University, GeorgiaReviewed by:
Jigang Xia, Chongqing Normal University, ChinaLei Wang, South China Normal University, China
Copyright © 2023 Wang, Schiariti, Xu, Ma, Sun, Wang, Zhao and Dong. This is an open-access article distributed under the terms of the Creative Commons Attribution License (CC BY). The use, distribution or reproduction in other forums is permitted, provided the original author(s) and the copyright owner(s) are credited and that the original publication in this journal is cited, in accordance with accepted academic practice. No use, distribution or reproduction is permitted which does not comply with these terms.
*Correspondence: Zhijun Dong, ✉ empkb25nQHlpYy5hYy5jbg==
†These authors have contributed equally to this work and share first authorship