- 1School of Biological Sciences, University of Reading, Reading, United Kingdom
- 2Laboratory of Immunoregulation (iREG), Department of Microbiology, Immunology and Parasitology, Biological Sciences Centre, Federal University of Santa Catarina, Florianopolis, SC, Brazil
- 3Faculty of Environmental Science and Engineering, Southern Cross University, Lismore, NSW, Australia
- 4The Biological Intelligence (BI) Lab, Faculty of Science, Southern Cross University, Lismore, NSW, Australia
Over recent decades, our philosophical and scientific understanding of cognition has changed dramatically. We went from conceiving humans as the sole truly cognitive species on the planet to endowing several organisms with cognitive capacities, from considering brains as the exclusive seat of cognition to extending cognitive faculties to the entire physical body and beyond. That cognition could extend beyond the organism’s body is no doubt one of the most controversial of the recent hypotheses. Extended cognition (ExC) has been discussed not only to explain aspects of the human cognitive process, but also of other species such as spiders and more recently, plants. It has been suggested that ExC could offer insights for the grounding of environmentally extended cognitive traits in evolved ecological functions. Here, we reviewed the ecological literature for possible ExC examples that satisfy the mutual manipulability criterion, which can be used to establish experimentally the boundaries of cognitive systems. Our conclusion is that ExC might be far more common than previously thought, and present in organisms as diverse as plants, fungi, termites, spiders, mammals, and slime moulds. Experimental investigation is needed to clarify this idea which, if proven correct, could illuminate a new path into understanding the origins and evolution of cognition.
1. Introduction
Perception and communication of information as well as learning, remembering, problem-solving and decision-making, are faculties routinely considered cognitive. But what is cognition? Relatively easy to observe in most living organisms, the term is hard to define and lacks a widely accepted consensus on its meaning (Bayne et al., 2019). Here, we take an all-inclusive definition to describe cognition as the process by which living organisms perceive and process information, and value, store and use this information to increase their chances of survival (Neisser, 1976; Shettleworth, 2010; Bayne et al., 2019; Lyon et al., 2021). And what do we mean by this tricky word: information? This ambiguous term can be understood in different ways. A broadly accepted interpretation is that of ‘statistical information,’ formalised by Shannon (1948) and commonly employed in physics, which refers to decrease in uncertainty of a system. While this definition can also be useful in the life sciences, information sensu Shannon becomes highly problematic when we talk about biological systems, where information is context-dependent (e.g. the same object can convey different information depending on the context; see Witzany and Baluška, 2012; Lyon et al., 2021). Hence, we believe it is more appropriate to talk about semantic information, which refers to the ‘meaning’ attributed by a biological system to an object, thus changing the behaviour or functioning of this system (Harms, 2006). It is not the aim of this paper to debate the different concepts of information, but for a detailed discussion on the three broad ‘classes’ of information, see Harms (2006).
Cognition refers to a particular interaction of the components of a biological system that makes the whole system flexibly adaptable and responsive to the environmental conditions (Smart et al., 2017). As a basic requirement for life to occur, cognition co-emerged with (rather than after) life and is present in all living beings with different degrees of complexity (Lyon et al., 2021). Departing from a very simple form of cognition present in our last common ancestor, cognition evolved differently in each branch of the tree of life, depending on the evolutionary trajectory, the perceptual apparatus and body structure and the needs and problems of each lineage. As a result, different organisms from bacteria to plants to animals have different cognitive systems that serve the purpose of keeping them alive (Lyon et al., 2021). We have long considered cognition as a feature possessed by humans and other primates, and understood most other animals to lack higher cognitive faculties (or to perform higher cognitive faculties in less complex ways than humans). Many cognitive scientists might not agree that cognitive faculties can be found beyond a handful of animal species and non-animal organisms like plants or slime moulds, partly because it is assumed that a large brain and a complex neural system are necessary to support cognitive faculties. Nevertheless, more recent research has included studies on cognitive processes of invertebrates (Mathel and Dickel, 2017; Perry et al., 2017), and non-traditional kingdoms, such as plants (Trewavas, 2016; Souza et al., 2018; Castiello, 2021), fungi (Aleklett and Boddy, 2021), slime moulds (Boussard et al., 2019), and bacteria (Shapiro, 2007, 2020). These new studies have forced us to reassess what cognition is, what is it for and where it occurs in the living world.
The recent inclusion of non-traditional model systems in the field of cognitive science testifies to an ongoing shift in our philosophical and scientific understanding of cognition. For example, let us take the notion of cognition as entirely contingent on specific structures of cognitive systems, such as the brain, and the accompanying computational metaphor of the brain as an extremely efficient information-processing computer, a metaphor we inherited from the early cybernetics/information theorist movement (Shannon, 1948; Weiner, 1948). These ideas have deeply guided our understanding of cognition and the brain most prominently since the 1960s with the emergence of cognitive psychology (Neisser, 1967; Figure 1). However, despite being highly developed, our brain is not an efficient computer and simply does not have the capacity to process every piece of information we encounter. Instead, we use ‘heuristics,’ mental shortcuts that allow us to make this information overload manageable so that we can arrive at solutions for given situations (Simon, 1955; Golstein and Gigerenzer, 2002), a phenomenon we share with numerous other species (Marsh, 2002; Hobson et al., 2021) with and without brains (Latty and Beekman, 2015). Crucially, this means that cognition does not work simply as computation and, contrary to what is commonly assumed, its substrate does not need to be a brain, given that information-processing and decision-making can happen even in its absence. Cognition, thus, operates differently from what our conventional understanding would suggest. Moreover, recent research shows that even in neural organisms, not all information is stored in synaptic connections. For example, non-associative long-term memory was found to be stored in the RNA of the sea snail Aplysia californica. Naïve sea snails became sensitised to a stimulus they never encountered before when the RNA of sensitised sea snails was transferred to them via injection in their haemocoels (Bedecarrats et al., 2018). Interestingly, transmission of a learned behaviour was also found in the aneural organisms Physarum polycephalum through the fusion of their cytoplasms, in the plural (Vogel and Dussutour, 2016). The fact that brains or neurons are not always necessary for information-processing and learning strongly suggests that the basis of information-processing and storage can be non-neural, and cognitive processes do not, necessarily, require a brain and central nervous system (CNS). If brains and CNS are dispensable for cognition to exist (although, of course, crucial for proper cognitive functioning in the organisms that have them), where else is cognition?
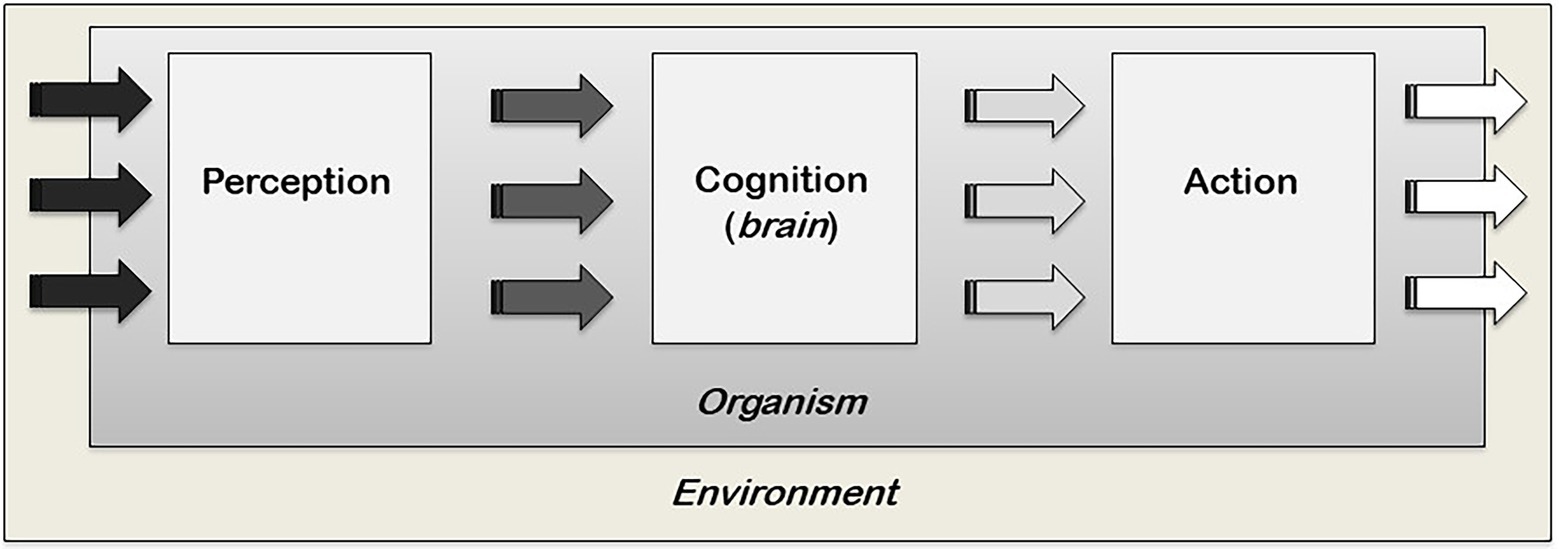
Figure 1. A cognitivist modular and linear view of cognition. Perception builds a discrete and abstract representation of the local environment which is, in turn, transformed by the cognitive processes of the brain into actions. Within this model, decision-making resides and is confined within the middle box of the brain. This model is also known as the ‘Classic Sandwich Model’ where the outer slices of perception and action are peripheral to the inner filling of cognition and separate from one another. They are also separate from cognition, which interfaces between perception and action (adapted from Burr, 2017).
2. Cognition within and beyond the body
The notion of cognition as an input–output mechanism tied to a CNS performing computations is typical of the cognitivist approach and has guided much of the cognitive research since the 1950s. However, another understanding of cognition, known as post-cognitivism, has been developed since the 1970s (e.g. Bateson, 1972; Dreyfus, 1972; Maturana and Varela, 1980) with the contribution of many different scholars with quite differentiated set of views. Among the range of views and research programmes within post-cognitivism, one particularly influential is the Santiago theory of cognition, initiated by Humberto Maturana and Francisco Varela (Varela et al., 2016). From this perspective, the processing of information is based on dynamic and reciprocal interactions of perception and action in the environment because living organisms are embedded in the environment and cannot be studied separated from it (Figure 2). Furthermore, cognition is an essential feature of life itself, and has evolved before the emergence of the first metacelluar organisms (Lyon et al., 2021). Cognition is what ensures the ability of organisms to manipulate and exploit the environment around them to secure their survival. As a process evolved billions of years before brains, the realisation of cognition requires, first and foremost, a living body, and not a CNS. Hence, cognition is an embodied and embedded process, present in all living organisms, occurring in the entire body (embodied), and entangled with the environment where the organisms are embedded in (Clark, 1997; Varela et al., 2016; Cazalis et al., 2017; Souza et al., 2018). For example, the way human cognition is altered and modulated by the gut through the gut-brain axis (Tillisch et al., 2013; Bagga et al., 2018), and by the skeletal muscles (e.g. Coles et al., 2019; Onishi et al., 2022), indicates that our own cognitive processes are not restricted to the CNS but span throughout our entire body, involving digestive and muscular systems. This does not lessen the importance of the CNS in the cognitive process, but rather suggests that human cognition is not restricted to it.
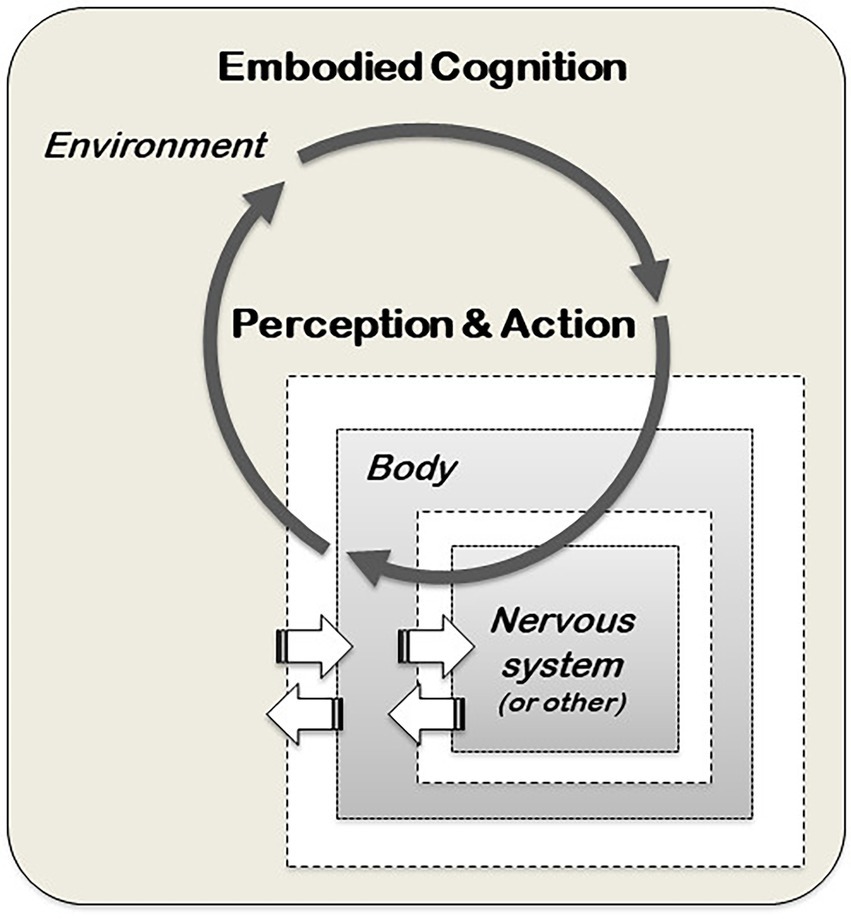
Figure 2. A post-cognitivist model of cognition. Within this model, the brain and nervous system (or other internal systems, such as the vascular system in plants) are embodied in the biological body of the organism, the body is situated in a local environment and behaviour is not a property of the organism, but an emergent property of the interacting dynamical system of nervous system-body-environment (adapted from Beer, 2000).
Clark and Chalmers (1998) proposed the idea of extended cognition in humans where cognition extends outside the boundaries of the physical body to objects in our immediate environment which we manipulate and transform into integral parts of our cognitive process. A functional coupling between the internal and external elements would underpin an extended cognition (Clark and Chalmers, 1998; Menary, 2010a). This coupling of the organism with environmental elements can be advantageous to the organism because the extension of the cognitive process beyond the boundary of the physical body can increase cognitive capacity. For example, it may allow the brain to offload the cognitive burden of processing some environmental information (Armitage et al., 2020), making certain cognitive tasks easier to perform.
An example of this cognitive burden offload is the use of pen and paper to solve a mathematical formula. By writing down on paper symbols and marks that aid the process, we externalise part of our short-term memory and information-processing ability. Without it, this cognitive task is significantly harder for most people. Today, our dependence on electronic gadgets for a myriad of tasks makes explicit more than ever how our cognition is extended to these objects (Armitage et al., 2020). More recently, it has also been proposed that even our gut microbiota, being able to modulate our cognitive abilities, composes our extended minds (Boem et al., 2021).
The extended cognition hypothesis has provided insight into the functioning of cognitive processes previously unknown in some organisms like spiders and slime moulds (Japyassú and Laland, 2017; Sims and Kiverstein, 2022). The possibility that organisms from different kingdoms (and two different phyla in the case of humans and spiders) extend their cognition suggests that the ability of extending cognition might be much more common and present in a wider range of organisms than previously thought.
In this article, we review the literature looking for organisms that could likely extend their cognition, focusing on niche-constructing organisms and how they manipulate their environment. Some studies did not directly test the extended cognition hypothesis but, we suspect, may at least partially satisfy the demarcating criterion adopted here (and described in the next section) and could provide insights into what extended cognition is for in those systems. We aim to provide an updated overview on where extended cognition occurs in the living world, proposing that this phenomenon could be far more common than expected.
3. Demarcating criteria for extended cognition
One of the difficulties to investigate extended cognition is using a clear demarcating criterion for what can be considered cognitive (see Menary, 2010b). When Clark and Chalmers presented the ExC hypothesis, they proposed a demarcating criterion for extended cognitive systems called the parity principle (PP). Accordingly, if some element of the world functions as part of a process that, were it occurring in the brain (or body, see Sims and Kiverstein, 2022), we would not hesitate in dubbing it as part of the cognitive process, then it is part of the cognitive process while being used for that aim. However, the PP is problematic as a criterion and considered weak (Sutton, 2010; Smart, 2022). According to its critics, the PP fails to evidence differences between inner and outer (i.e. extended) components of the cognitive system by giving similar weights to the importance of what happens inside the body and outside it (Sutton, 2010; Smart, 2022). Besides, it is not a good criterion to limit which elements of the world can be considered part of a cognitive process, and it relies on a theoretical assumption of the mind based on functionalism, which overlooks how psychological states are physically sustained (Sutton, 2010; Kaplan, 2012). In other words, the substrate for cognition to happen would be irrelevant. Furthermore, the PP fails to prevent what is known as the ‘coupling-constitution fallacy’, when every object could be considered constitutive to the cognitive process just because it is coupled to the cognitive agent (Adams and Aizawa, 2001). Because something is coupled to a system showing causal dependence, it does not mean that it constitutes the system. This problem can further lead to what is known as the ‘cognitive bloat’: everything that is readily accessible to the cognitive agent would be considered as part of its cognitive system (Rupert, 2004; Clark, 2008; Allen-Hermanson, 2013). Consequently, Wikipedia or Google should be considered as parts of our cognitive process when we access these websites, meaning that our memory extends to all of these databases (Ludwig, 2015).
To solve problems related to the coupling-constitution fallacy and cognitive bloat, Kaplan (2012) proposed the mutual manipulability (MM) criterion of Craver (2007) to demarcate the boundaries of cognition. Kaplan noted how neurobiologists already demarcate cognitive processes within the body by mutually manipulating the components of the system to establish relations of constitutive relevance between them (Kaplan, 2012). Putting it very simply, to establish these relations, there are two conditions of MM that must be satisfied with interventions occurring at different levels of the phenomenon to be explained. When testing whether an element is part of a system in a particular way, an intervention in that system’s behaviour should cause a corresponding alteration in the element (this is known as top-down intervention). Similarly, an alteration in the element also must cause an alteration in the system’s functioning (bottom-up intervention). If both interventions are successful in establishing these causal relations, one can then say that the element is part of the mechanism, and both constitute the same system (Craver, 2007; Kaplan, 2012).
As an example, let us suppose that a scientist suspects that the cognitive process of an organism X involves the secretion and sensing of slime into the environment. According to the MM criterion, the scientist should submit X to a cognitive task that requires the use of slime. Depending on the challenge presented, the scientist observes that the organism X changes the chemical composition of the slime, which helps it to accomplish the task. To satisfy the first condition of the MM criterion, she or he administers a drug that interferes with the production of slime, observing that it affects the slime chemical composition and the organism’s response. Subsequently, in another set of experiments, the scientist applies a synthetic slime in the environment, confusing the organism X, which can no longer perform its task properly. The second condition of the MM criterion is satisfied, and the scientist concludes that the slime is part of the cognitive process of the organism X.
The MM criterion is a powerful tool to ensure that simple causal background conditions are not confused with extended cognition; in other words, the criterion prevents the ‘cognitive bloat’. For example, when we are performing a cognitive task Y, areas in the brain related to this task are known to be more activated, which increases its oxygen consumption. Someone could argue that oxygen, therefore, is part of our cognitive processing. However, this scenario does not satisfy both conditions of the MM criterion; supposing that it is possible to enhance the presence of oxygen in the same brain areas, hardly anyone would be inclined to think that it would make us spontaneously engage in cognitive task Y. Oxygen is a background condition as necessary as glucose or water to the normal functioning of the brain, but not an active part of the cognitive process (Kaplan, 2012; Parise et al., 2020).
The usefulness of MM to establish constitutive relevance has been questioned but also elaborated since it was proposed (Krickel, 2018; Craver et al., 2021; Smart, 2022). For example, critics to this method say that it is inadequate because it is spelled out in terms of interventions, which is an approach to establish causal relations, whereas constitutive relevance implies non-causal relations (Krickel, 2018). Consequently, when employing the MM, some additional care must be taken to consider, for example, the importance of time in the manipulations (Krickel, 2018) and the kind of interventions made (Craver et al., 2021). It will depend on the kind of experiment being performed and the system under study. Nevertheless, given the potential of MM to underpin empirical experiments on extended cognition, it was adopted by behavioural ecologists to study this phenomenon at least in spiders (Japyassú and Laland, 2017), and recently proposed for plants (Parise et al., 2020).
In an excellent review, Japyassú and Laland (2017) applied the MM criterion to analyse the behaviour of spiders and their webs, and concluded that spiders have their cognition extended to their webs. Both the spider and the web are part of the same cognitive system. The authors corroborate their hypothesis by presenting a series of experiments that satisfy the MM criterion. For example, spiders that weave orb-webs learn which sector of their web captures more prey. Hence, when they are hungry, they tend to direct their attention to the most profitable section of their webs by pulling the radial threads of that section. The tension in the threads makes them more accurate in perceiving the vibrations caused by even small prey, enhancing the chances of success in procuring food (Watanabe, 2000; Nakata, 2010; Mortimer et al., 2018). However, if the tension of another area of the web is experimentally increased by pulling its threads, the spider automatically directs its attention to that section, even though this is not the section the spider has learned to be most rewarding (Nakata, 2010, 2013). By satisfying both conditions of the MM criterion, we can conclude that the spider’s ability to process information and act on it is highly dependent on the web, and the web is part of the spider’s cognitive system. Other examples for the case of spider cognition extending to their webs can be found in Japyassú and Laland (2017).
Similarly, Parise et al. (2020) proposed that plants could also extend their cognitive abilities to the environment they manipulate. For example, plants could use the substances they exude through their roots, the soil microbiota, and the common mycorrhizal network as an extension of their cognitive system. Below, we provide some specific plant examples, and suggest that a model of plant extended cognition can be applied to the behaviour of other brainless, sessile, modular and niche-constructing organisms, like sponges and corals.
In summary, the MM is a promising criterion to explore extended cognition. However, depending on the species studied, the experimental conditions, and how the resulting data will be interpreted, the employment of the manipulations and the kind of interventions will vary. Krickel (2018) and Craver et al. (2021) are good references to help planning these experiments. For the purpose of this review, we will adopt a loose interpretation of the MM to make our point, also because none of the studies that will be discussed here were designed to test for extended cognition. We hope that our analysis below serves as an inspiration and a starting point for research looking for other cases of extended cognition in nature, where experiments will have to take into consideration the subtleties of the MM account.
4. Ariadne’s thread and the external memory
According to the legend, the Greek hero Theseus entered Mino’s labyrinth, where the monstruous Minotaur lived, with a ball of thread famously given to him by Ariadne in the hope that he could find his way back out of the deadly trap. Theseus unspooled the ball of thread as he entered the labyrinth; after defeating the beast, he was able to find his way out by following the thread.
Mechanisms to externalise memory such as the one employed by Theseus are common. We can find them in our daily life from the much less epic post-its glued over our computer or fridge to appointments noted in a diary or cell phone (Figure 3A). What these mechanisms have in common is the fact that they are ways of relieving us from the burden of remembering certain things, a mechanism that is relatively common across the domains of life. From the MM perspective, we could say that the thread is an extension of Theseus’ cognition. His mind is unable to remember all the turns taken inside the labyrinth; however, by actively unspooling the thread along the way, he successfully manipulates his environment (which satisfies the first condition of the MM criterion). In this case, we understand that a change in Theseus’ cognitive state, i.e. the one elicited by the challenge of entering the labyrinth, changes his interaction with the environment, i.e. unspooling the ball of thread. Since he is a human subject, we take his cognition for granted. However, if one wants to be sure of this top-down relation, one could experimentally drug Theseus or damage part of his brain, making him to forget Ariadne’s instructions, not unspooling the ball of thread, or even letting the thread go. He would never complete the navigational task in this case. To satisfy the second condition of the MM criterion (bottom-up intervention), we would need to manipulate the thread and observe Theseus’ behaviour. If someone maliciously cuts the thread or stretches it through another path, Theseus will never find his way back and die inside the labyrinth, to Ariadne’s grief. In other words, Theseus’ ability to navigate a maze is compromised if someone messes with the thread. Both conditions of the MM criterion are satisfied and we can say that, in that moment, Ariadne’s thread was part of Theseus’ cognitive system.
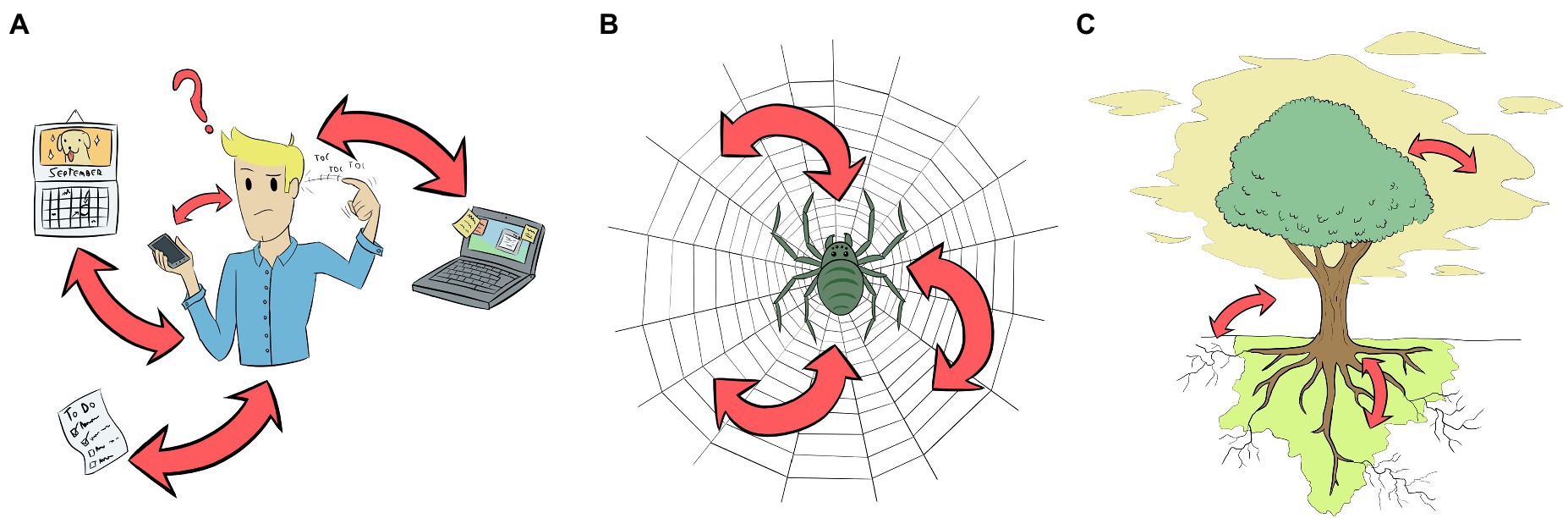
Figure 3. Representation of three different biological systems (two neural and one aneural) that may extend their cognitive process to the environment. Arrows represent the relationships between the organism and the objects that sustain the extended cognition (ExC). By experimentally manipulating these relations, ExC could be tested empirically. (A) Humans, the best-studied example, have a plethora of mechanisms for extending their cognitive process by modifying their environment, from ‘to-do lists’ to computers and cell phones. Gut microbiota could also be involved in human ExC (Boem et al., 2021), but is not represented here. (B) Spiders could extend their cognition to their webs; manipulations in the web lead to changes in spider cognitive states, and vice versa. (C) Plants are an example of aneural organism that could use ExC to support its cognitive process. In this case, through the manipulation of VOCs, root exudates and soil microbiota, and mycorrhizal symbionts. Similar processes may occur in other organisms without neurons or central nervous system like fungi, slime moulds and sea sponges.
Spiders also use threads to remember the path they have taken, especially when spinning their webs. This is a complex behaviour and the spider cannot remember all the steps all the time, especially in the earlier stage of web-spinning (Japyassú and Laland, 2017). Therefore, they rely on external cues perceived sequentially, in particular the position of the spiral thread that was previously glued to the radial threads (Eberhard and Hesselberg, 2012; Japyassú and Laland, 2017). Before moving from one radial thread to the other, the spider measures its distance from the spiral thread to know how to glue the new spiral thread. The experimental removal of a segment of the spiral thread creates conflicting cues to the spider, and usually, it ignores one cue in favour of another by skipping the measurement and simply glueing the new thread in a displaced spot. In the next round, it will use the newly glued thread as a guide, but an irregularity will remain in the web (Eberhard and Hesselberg, 2012). According to Japyassú and Laland (2017), much of the information to build the web is present in the web itself, and not within the spider’s CNS—just like the case of Theseus—and the information can be altered if we alter the shape of the web (Figure 3B). We think most researchers would also take for granted that spiders are cognitive organisms, which means that it is obvious that alterations in their cognitive states would change how the webs are spined and used. But as examples of top-down manipulations in the case of spiders, studies have demonstrated that interventions in the spider’s CNS regions related to web-building change dramatically the structure of their webs. These interventions include laser-induced brain damage (Witt, 1969), drug administration (Witt, 1971; Hesselberg and Vollrath, 2004; Albín et al., 2014), starvation (Watanabe, 2000), and even parasitoid wasps (Eberhard, 2000).
Interestingly, organisms with no brain also leave cues to help navigation. The slime mould Physarum polycephalum releases a slime composed of glycoproteins that function as an external memory of the places where it has already been (Reid et al., 2012). The slime is repulsive to the P. polycephalum; given the option, the slime mould chooses not to crawl over it. In an experiment with a U-shaped obstacle, the researchers observed that P. polycephalum uses the slime as a cue to navigate better and exit the maze more efficiently. When they manipulated the slime effectiveness by putting the P. polycephalum in a substrate entirely covered by slime, they masked its effect and the exit from the U-shaped obstacle was significantly impaired (Reid et al., 2012). The manipulation of an object interfered with the cognition of the slime mould, which could be considered a bottom-up intervention and suggests that P. polycephalum extends its cognition to the slime. To complete the MM criterion, researchers will have to think on experiments that manipulate the slime through interventions in the cognitive system of the slime mould. Such experiment would add robustness to claim of extended cognition of Sims and Kiverstein (2022) in slime moulds.
Plants are another group of brainless organisms that use external memory to solve their problems (Figure 3C). For example, Arabidopsis thaliana and Cucurbitaceae plants can actively manipulate the soil microbiota community in the rhizosphere. By altering the chemical composition of its root exudates, these plants inhibit the growth of some taxonomic groups of microbes and favour the development of others, leading to increased pathogen resistance and fitness (Huang et al., 2019; Zhong et al., 2022). This creates a microbiological community that is very specific to the exudates released, and this alteration can not only help to tackle pathogens, but also encode and store the memory of past events. For instance, Yuan et al. (2018) grew five generations of A. thaliana infected by Pseudomonas syringae in the same pot and substrate. When they planted a sixth, naïve plant in the same pot, and later infected it with P. syringae, the plant was significantly more resistant to this disease than the control, even though it never experienced the disease before (Yuan et al., 2018). This means that alterations in the plant (disease) change the microbial rhizosphere community, and alterations in the rhizosphere community change the behaviour of the plant. Besides, in another set of experiments, the authors applied artificial exudates that mimicked the chemical composition of A. thaliana exudates in naïve plants, which altered the microbiological community of the substrate, and the plants became more resistant to P. syringae than the control group (Yuan et al., 2018). This means that the manipulation of the soil or exudates by growing infected plants in the substrate or applying artificial exudates creates an immunological memory that primes the plants against the disease, which satisfies the second condition (bottom-up intervention) of MM. The phenomenon studied by Yuan et al. (2018) is called ‘soil memory,’ or ‘soil-borne legacy’, and has received interest because of its potential applications to agriculture. Although here we are talking about immunological memory, the fact that plants can manipulate the soil microbiota depending on their needs should be taken into account when studying cognitive traits of plants.
5. Exudates: The liquid border of cognition?
Root exudates are one of the most important means by which plants interact and manipulate their underground world. Beyond the probiotic and mnemonic functions mentioned above, it seems that they are also important for the plant to synchronise its flowering. Brassica rapa is a plant that preferentially blooms when daylight time reaches or passes a certain threshold of hours. However, it can also bloom under the non-inductive condition of short days, when daylight duration is below that threshold. Falik et al. (2014) observed that when grown under long-day conditions and blooming, this plant likely alters the composition of its root exudates (as suggested by the results of the experiment—the authors did not analyse exudate chemical composition) which possibly could be considered as satisfying the first condition of the MM. Subsequently, the researchers manipulated the exudates of B. rapa grown under short-day conditions by watering it with leachates of blooming plants exposed to long-days (a manipulation of the exudates that satisfies the second condition of the MM). The modification of exudates changed the information available to the plants, but this was aboveground-borne information previously processed and encoded by other plants. As a result, even under the non-inductive condition, the plants accelerated its bloom and increased fruit production (Falik et al., 2014).
Furthermore, many allelopathic plants—plants that exude toxic substances through their roots, in general gaining competitive advantage over other plants—are sensitive to the exudates they release. One important hypothesis to explain this self-toxicity proposes that this effect enables the plant to perceive and explore its three-dimensional environment around it. For example, Falik et al. (2005) demonstrated that the accumulation of root exudates between Pisum sativum roots and an obstacle in the soil promotes growth inhibition and even root withering. Consequently, the plant can stop its growth towards an obstacle even before its roots get close to it (Falik et al., 2005). When the researchers manipulated the root exudates by adding activated carbon or potassium permanganate, which inactivated the exudates, it supressed the root obstacle avoidance mechanism. As a result, the roots grew towards the obstacle as if it was not present (Falik et al., 2005).
Mahall and Callaway (1991, 1992) studied two species of desert plants: Larrea tridentata and Ambrosia dumosa. The first one is an allelopathic plant, while the latter is not. To understand the distribution of these plants in the field (L. tridentata, randomly scattered; A. dumosa, clumped), Mahall and Callaway studied their root interactions in a set of experiments. They discovered that L. tridentata uses allelopathic exudates to interact with roots of the same plant or of other plants. The accumulation of allelopathic exudates between two roots of L. tridentata leads to the inhibition of its growth, and this interaction structures the distribution of the roots in the soil and the distribution of the plants in the field. However, when the exudates were experimentally removed from the soil with activated carbon, this inhibitory effect ceased, and the plant grew its roots regardless of the presence of other roots.
These experiments (Mahall and Callaway, 1991, 1992; Falik et al., 2005) suggest that exudate accumulation provides the plant information about the three-dimensional environment around belowground organs. This process is almost entirely external to the plant, but leads to an optimised placement of roots, likely with effects on plant distribution in the environment (Mahall and Callaway, 1991, 1992) and fitness. Besides, the experiments suggest that activated carbon is a useful tool to test the hypothesis of root exudates as part of the plant’s cognitive process. It is a promising, relatively simple and cheap technique to investigate extended cognition in plants. The experimental removal of root exudates followed by the observation of plant behaviour could lead to insights on the contribution exudates make to the plant cognitive process.
There is another way by which plants could use exudates to understand their three-dimensional environment. When a seed germinates, it is important for the young plant to be aware of the soil volume around it, for it is a limiting resource to be exploited. Therefore, it is imperative the germinating seed acquires non-nutritional information about the immediate substrate to match its growth to the available soil volume. Restrains in growth are due to forecasted limitations, occurring irrespective of nutrient or water availability (Wheeldon et al., 2021). To explain this phenomenon, Wheeldon et al. (2021) carried out a series of experiments on the growth and development of wheat (Triticum aestivum cv. Mulika) and found that this plant actively assesses the substrate volume in a two-step process. Wheeldon and colleagues proposed that the plant first releases a soluble, rapidly diffusible substance that spreads through the substrate (‘substrate volume-sensing signal’, SVS). Its concentration will depend on the substrate volume and modulate the shoot growth to match the substrate volume. However, the roots also secrete another, less mobile substance (‘root density-sensing signal’, RDS) that will signal to the roots its density, inhibiting its growth. These substances do not diffuse across the entire substrate but remain restricted to the roots’ influence zone and modulate rooting patterns, preventing the roots of growing too much and, perhaps, competing with each other.
This idea is very intriguing despite the unknown nature of the SVS and RDS. The authors do not discuss the possibility of the SVS and RDS signals being of other kind like sound, which is another possible mechanism for the plants to sense their three-dimensional environment (Gagliano, 2013, 2015). Regardless of their nature (chemical or acoustic), SVS and RDS are strong candidates to compose the extended cognitive system of wheat. In that case, one condition of the MM criterion is secured: the plant actively produces these chemicals or sounds and uses them to sense the environment. It is worthy to explore how manipulating SVS and RDS influences plant growth. If this second condition of the MM is satisfied, then we would have another example of extended cognition in plants.
The hypothesis of cognitive processes extending to exudates could be applied to fungi as well, for they also exude components in the soil that could help in their navigation and foraging behaviour in their three-dimensional environment. For example, Aleklett et al. (2021) observed that hyphae of the fungus Gymnopus confluens matched the speed of its growth with the width of the channel it is growing in. The wider the channel, the faster it grows. The authors also observed fungi’s exudates released in the environment, which could be regulating the reproductive activity of the fungi themselves, and also helping to process information about the environment around the hyphae. In this case, fungal exudates could play an analogous role as plant exudates in regulating reproduction (Falik et al., 2014) and root navigation (Mahall and Callaway, 1992; Falik et al., 2005). It is tempting to hypothesise that this is a form of extended cognition in fungi, but this possibility awaits rigorous experimental testing.
6. Self-generated chemotactic gradients
The modification of the organism’s surroundings to aid navigation in complex environments has been demonstrated to be employed even by single migrating cells. Chemotactic gradients are known for driving the steering of cells when they displace themselves. This phenomenon is present in cells migrating during embryonic development, the displacement of protists in the environment, immune responses and the spread of tumorous cells during metastasis (Donà et al., 2013; Evans and Wood, 2014; Tweedy et al., 2016, 2020). However, there is evidence that the gradients that guide these cells are not imposed externally, but generated by the cells themselves when they metabolise attractant substances in the medium (Donà et al., 2013; Tweedy et al., 2016). As the cells modify the environment around them, they guide their own migration. This allows single cells to explore the environment around by sensing it from a distance, sampling it and using this information to decide the path to take. With mathematical modelling simulations confirmed by experiments with the protist Dictyostelium discoideum and mouse pancreatic cancer cells navigating complex mazes, Tweedy et al. (2020) demonstrated that the breakdown of, respectively, cAMP and lysophosphatidic acid from the environment creates self-generated gradients that guide the navigation of these cells in complex labyrinths. Remarkably, the cells use this gradient to anticipate dead ends from a distance, before entering them, and choosing the path that leads to the source of attractant. The authors observed that the capacity to generate such gradients and the structure of the environment are important to this navigation. When they manipulated the capacity of D. discoideum to break down cAMP, enhancing it by mutation, or preventing attractant breakdown by delivering to the wild type D. discoideum the non-degradable attractant Sp-cAMPS instead of cAMP, they observed an outperform in the maze (compared to the control) in the mutated D. discoideum and almost no progress in the migration of the group exposed to Sp-cAMPS (Tweedy et al., 2020). In the same study, the authors tested different labyrinth shapes, some of them designed to confound the cell’s self-generated gradient. In these cases, both D. discoideum and cancer cells choose the dead end instead of the ‘right’ path, indicating that the manipulation of the self-generated gradient influences the cell’s ability to migrate in complex environments.
The study of Tweedy et al. (2020) seems to have satisfied both conditions of the MM criterion by manipulating the cells’ capacity to perform a cognitive task and the environment modified by the cells, with clear effect in their performance. Through intervention in the cell, they manipulated the chemotactic gradients, and through interventions in the chemotactic gradients, they manipulated cell cognitive behaviour. These results suggest that even single cells, be they part of a higher organism or not, are able to manipulate the environment to increase their ability of problem-solving—which leads to the appealing idea that even cells extend their cognitive process.
7. Volatile organic compounds: The ‘mind’ is in the air
The most well-documented form of plant–plant communication is through volatile organic compounds (VOCs). These are substances released in the air that can be sensed by other plants and modulate their behaviour. Notoriously, they can be used by plants to communicate herbivory attack, and induce the transcription of defence-related genes in other plants, stimulate the production of other VOCs, induce the production of extrafloral nectar—which attracts predators of herbivores—activate direct defences and the release of hormones, trigger calcium depolarisation and also activate induced systemic resistance (reviewed in Heil and Karban, 2010).
A particular bouquet of VOCs is released depending on the stimulus a plant receives, and the different chemical compositions encode different messages (Heil and Karban, 2010). This mechanism probably evolved as a fast communication mechanism within individual plants. Without VOCs, communication between branch apexes may take several minutes or hours because this process would rely on electrical or chemical signals transported through the vascular bundles (Heil and Silva Bueno, 2007). Volatiles are ‘shortcuts’ for the information traffic in a plant crown. In that sense, we could say they have an importance for fast integration in the crown comparable to certain pathways for information in the animal brain, like the dopaminergic pathways or the corpus callosum connecting both brain hemispheres, which allow rapid convey of information to distant parts of the brain. The dopaminergic pathways, like the mesolimbic and mesocortical pathways, connect relatively distant areas of the brain, for instance, the ventral tegmental area to the ventral striatum and the prefrontal cortex, respectively. Disruptions on these connections lead to neurological problems related to motivation, reward, memory, learning and movement (Engert and Pruessner, 2008; Yamagushi et al., 2011). Similarly, human corpus callosum ensures that both brain hemispheres are connected and in interaction with one another for proper functioning of the brain as a whole. If this structure is severed, cognitive impairments arise. For example, the pathway that connects speech ability to the senses of the left side of the body is interrupted, and the subject can no longer describe verbally which object the left hand is holding, or what was seen in the left side of the visual field (Gazzaniga, 1967). This demonstrates that long-distance pathways for exchange of information are important for proper cognitive functioning, both in animals and plants.
Therefore, VOCs constitute part of the cognitive process of plants, as they evolved primarily as a system of internal communication. Plants alter its VOCs depending on what they are experiencing, normally when stimuli are related to predation (Heil and Silva Bueno, 2007; Heil and Karban, 2010), which could be considered as the first condition of the MM criterion. Conversely, if we manipulate the VOCs, we also manipulate the plant’s cognitive state. For example, when leaves of Lima bean plants (Phaseolus lunatus) undergo herbivory, they release in the air a bouquet of VOCs that communicate to other parts of the plant (and sometimes other plants nearby) the information of the attack, enabling the priming of the plant’s defences (Heil and Silva Bueno, 2007). In that case, the plants more promptly secrete extrafloral nectar, and in higher amounts, which attract the predators of their herbivores, causing the plants to be less damaged by herbivory. The VOCs are actively produced by plants to convey information throughout the crown and are related to what the plant is experiencing (Karban, 2015). Not surprisingly, the manipulation of these VOCs, such as their removal from the atmosphere (Heil and Silva Bueno, 2007) or the application of synthetic VOCs to undamaged plants (Heil and Kost, 2006), changes the plant strategy to fight herbivores, respectively, preventing its response or causing it to activate its defences following misleading information. This can be interpreted as the second condition of the MM being satisfied.
Sometimes, due to intraspecific variations in different populations, the same stimulus creates different VOC bouquets. In a study conducted with two populations of Artemisia tridentata, emitter plants received the same damage in the leaves, but the chemical composition of their VOCs was population-specific. Plants exposed to VOCs from the same population responded more effectively to the stimulus than plants that received VOCs from a different population. As a result, the latter plants underwent higher herbivory, as assessed 3 months later (Karban, 2015). By altering the VOCs in a plant headspace—which ‘meaning’ was presumably the same—the researchers altered the plant’s capacity to fight predators.
In summary, VOCs are part of the extended cognitive process of plants, ensuring rapid integration of information in the aboveground organs as an external resource that fills with relevant infochemicals the air around the plant’s crown.
8. Organism(s)-environment feedbacks
Many niche-constructing organisms are known for building complex structures out of their collective work. The behaviour of ants and termites is an important example of swarm intelligence, when organisms follow a small set of simple rules or have limited information-processing, but their interaction leads to the emergence of complex collective behaviours (Krause et al., 2010).
For example, it was previously thought that termites build their nests based on simple sets of pheromones released by their peers on soil pellets, in a process of indirect communication called stigmergy (Grassé, 1959). In this process, a worker would release in the environment a pheromone that would be perceived by another worker, which would also release more pheromones, and so on. In a positive feedback loop cycle, places with stronger pheromones would present more labour activity, and this would regulate overall nest behaviour (Calovi et al., 2019). Nevertheless, the pheromone aspect of stigmergy has been challenged since other mechanisms have been discovered, while no pheromone involved in nest construction has been identified to date (Camazine et al., 2003; Calovi et al., 2019). The instructions for nest-building seem to be elsewhere.
The construction of termite nests was demonstrated to be a complex, self-organised process, built upon a set of simple rules that each termite follows individually, which ultimately leads to the architectural complexity of the nest (Camazine et al., 2003; Facchini et al., 2020). Nevertheless, when building nests, termites are known for relying more on environmental cues than their own memory for making decisions (Fouquet et al., 2014). This characteristic, together with the fact that they are prime examples of niche-constructing organisms, makes termites promising candidates to extend their cognition.
Accordingly, Fouquet et al. (2014) demonstrated that termites rely more strongly on the surface structure of a site than on chemical marking to make decisions on where to dig and where to deposit soil pellets. In a set of experiments, they exposed the termites to structurally different environments and observed that the structure orients the deposition of pellets, which feedbacks-forwards to the building of the nest. For example, when they were exploring the territory, they do not wander randomly, but form trails. Because of their walking, the trails are more depressed than the environment around, which stimulates the termites to deposit pellets on the edges of the trails. Consequently, the trails become some sort of template for the building of the new nest and a kind of ‘collective memory’ for the new, emerging structure (Fouquet et al., 2014).
Calovi et al. (2019) hypothesised that the soil displacement activity of Macrotermes michaelseni termites would be determined by the inclination of the surface where they are working. To test it, they manipulated the surface by building a support with different, controlled inclinations. They covered it with nest sediments, placed termites on it and observed their activity. Their results demonstrate that termites are particularly sensitive to the surface curvature, which was correlated with soil displacement and building activity (in accordance with findings of Fouquet et al., 2014). The soil geography is the main cue that drives the termites’ constructing behaviour, and not necessarily the cues released in the environment. It is clear that the information-processing to build a nest does not occur solely inside the termites’ brains (Calovi et al., 2019). Based on their findings, the authors proposed that both the termites and the nest would be part of a same cognitive entity, where the nest serves as long-term memory repository and the guiding force to the construction of the nest itself. The termites are part of this cognitive process by ‘reading’ the forms of their own labour and continuing this work. In short, there is a cognitive process that involves each individual termite and their own, physical nest (Calovi et al., 2019).
The studies above suggest the possibility that termites may offload the cognitive burden of knowing how to build a complex nest not only to their own partners but also to the nest itself, which is a non-living component of their cognitive process1. The manipulation of the environment structure affects the building and structure of the nest because what guides the nest-building is the environment itself. This is different from humans adjusting the project of a house to the terrain where they are building because in this case the cognitive process of projecting the house is extrinsic to the environment, and not driven by it. Building a suspended house in an inclined terrain, for example, or dividing it in two floors, may be clever solutions, but not the only possible ones to build on this terrain. If the only way of building the house was tied to the interaction of the workers with the environmental characteristics of the building place, the idea of an extended cognitive process could be raised. Finally, other social and nest-building insects like ants, wasps and bees could be good candidates for examining extended cognition in these invertebrate systems in experiments specifically designed for testing this possibility.
9. Gut microbiota as part of cognition
Boem et al. (2021) proposed that the gut microbiota can act as a cognition element by modulating bidirectional neural pathways, neuroendocrine signalling, as well as its impact on the immune system. Boem and colleagues did not mention the MM criterion for demarcating extended cognition; but other researchers partially employed the MM criterion by altering the gut microbiota element and observing brain connectivity changes. For instance, Tillisch et al. (2013) demonstrated that chronic intake of fermented milk with living probiotic bacteria modulates brain activity related to emotional attention tasks in humans. In a more recent study, Bagga et al. (2018) used a 4-week treatment of a multi-strain oral probiotic supplement to evaluate changes in human brain activity using functional magnetic resonance imaging. After treatment, changes in decision-making and memory processes were observed, especially related to unpleasant stimuli when compared to neutral ones. It suggests that the impact of gut microbiota on human brain activity is even bigger in the context of emotions. Unfortunately, both experimental designs only satisfy one condition of the MM criterion (i.e. altering the ‘environment’ and observing its impacts on the cognitive system). If we are to say that gut microbiota is part of the human extended cognition process, it is critical that both conditions of the MM criterion are met. If the cognitive state of the subjects is proven to alter the gut microbiota just like gut microbiota alters the cognitive state of the subjects, we will have empirical evidence for another way by which humans and other mammals extend their cognition.
Moving from human to rodents, studies with mice have yielded more interesting results. Bottom-up manipulations have been made, for example, by transferring the gut microbiota of human patients with depression to rats pre-treated with antibiotics, causing them to develop depressive symptoms and anxiety-like behaviours (Kelly et al., 2016). Other manipulations of mice gut microbiota with probiotics or transfer of microbiota caused enhanced exploratory behaviour and reduced anxiety (Bercik et al., 2011; Bravo et al., 2011), while the absence of microbiota colonisation was demonstrated to change neurogenesis, the density of network connections in the brain, and alter behaviour in germ-free mice (Scott et al., 2020).
Finally, a study particularly interesting to our case was carried out by Chevalier et al. (2020). The researchers stressed mice using an unpredictable chronic mild stress (UCMS) protocol, which is a mouse model for depression. They observed that mice who had been through UCMS had altered gut microbiota characterised by losses in Lactobacillaceae, decreased endocannabinoid (eCB) signalling and as a consequence, presented reduced adult neurogenesis and depressive-like behaviours. This is an interesting example of top-down intervention that causes an alteration in gut microbiota. Then, Chevalier et al. (2020) orally transferred the faecal microbiota of UCMS and unstressed control mice to germ-free and pathogen-free naïve mice (treated with antibiotics). The researchers observed that recipients colonised with UCMS microbiota developed the same depressive-like symptoms and neurological disorders, an effect not observed in the mice that received control microbiota. This can be considered a bottom-up manipulation where an alteration in the gut microbiota (the putative element of the extended cognitive system) resulted in a corresponding alteration in the explanandum phenomenon (mouse cognition). Finally, Chevalier et al. (2020) did a further bottom-up manipulation when they supplemented UCMS-recipient mice with probiotic diet enriched with Lactobacillus plantarum. Consequently, this treatment reversed the depressive-like behaviour of mice and partially restored hippocampal neurogenesis. The authors concluded that dysbiosis disrupted fatty acid metabolism, reducing the availability of eCB signalling precursors in the mice gut, which caused the behavioural and neurological effects observed. This is a beautiful example of the MM criterion being employed—perhaps, inadvertently—to establish constitutive relevance of mice gut microbiota for mice (extended) cognition.
10. Conclusion
Here, we reviewed studies on a broad range of organisms that could likely extend their cognitions. This review is by no means exhaustive; rather, it provides a starting point for considering how common extended cognition might be in nature. We propose that extended cognition may be present in organisms as diverse as humans, mice, spiders, plants, fungi, termites, protists and even cancer cells, and may occur in many different manners. When we observe organisms manipulating their environment and behaving in ways that seem far more complex than it would be expected, we can suspect that there might be an extended cognitive process taking place. Consequently, extended cognition might be much more common than we currently think, especially if we consider that basal organisms also possess this ability. The need for designing studies that attend to the mutual manipulability criterion as a form of clearly demarcating the boundaries of cognitive systems and creating a common base for comparisons is essential. Experimentally testing for extended cognition in as many organisms as possible, could help shedding some light on the evolution of cognition, a process that appeared in the planet billions of years ago, well before the evolution of brains. Cognition emerges through the interaction between the cognitive agent and the environment (Clark, 1997; Lyon, 2006; Varela et al., 2016). In some cases, this interaction could have led to a tighter coupling between organisms and environment that facilitated the organisms’ cognitive process, thus increasing their adaptability. As an evolutionary consequence, some external elements became an integral part of the organisms’ cognitive system, a possibility that blurs even more the boundaries of cognitive processes. Studies on extended cognition may prove to be necessary for a better understanding of what cognition is, how it works and how it evolved.
Data availability statement
The original contributions presented in the study are included in the article/supplementary material, further inquiries can be directed to the corresponding author.
Author contributions
AP and MG conceived the manuscript. AP, GG, SW, and MG contributed to writing and discussions. All authors contributed to the article and approved the submitted version.
Funding
This work was partly funded by the Templeton World Charity Foundation (Grant TWCF-2020-20541).
Acknowledgments
The authors thank Madeleine Beekman, Pamela Lyon, Paco Calvo, and Gustavo Maia Souza for early discussions on non-neural cognition, and the reviewers for their comments. The authors also thank Raffaele Parise for the design of Figure 3.
Conflict of interest
The authors declare that the research was conducted in the absence of any commercial or financial relationships that could be construed as a potential conflict of interest.
Publisher’s note
All claims expressed in this article are solely those of the authors and do not necessarily represent those of their affiliated organizations, or those of the publisher, the editors and the reviewers. Any product that may be evaluated in this article, or claim that may be made by its manufacturer, is not guaranteed or endorsed by the publisher.
Footnotes
1. ^We acknowledge that the MM is a debated criterion for the identification of extended cognitive systems. For more information on this debate see Krickel (2018), Smart (2022), and references therein.
References
Adams, F., and Aizawa, K. (2001). The bounds of cognition. Philos. Psychol. 14, 43–64. doi: 10.1080/09515080120033571
Albín, A., Lacava, M., and Viera, C. (2014). Effects of gonyleptidine on the orb-web spider Araneus lathyrinus (Holmberg, 1875). Arachnology 16, 154–156. doi: 10.13156/arac.2012.16.5.154
Aleklett, K., and Boddy, L. (2021). Fungal behaviour: a new frontier in behavioural ecology. Trends Ecol. Evol. 36, 787–796. doi: 10.1016/j.tree.2021.05.006
Aleklett, K., Ohlsson, P., Bengtsson, M., and Hammer, E. C. (2021). Fungal foraging behaviour and hyphal space exploration in micro-structured soil chips. ISME J. 15, 1782–1793. doi: 10.1038/s41396-020-00886-7
Allen-Hermanson, S. (2013). Superdupersizing the mind: extended cognition and the persistence of cognitive bloat. Philos. Stud. 164, 791–806. doi: 10.1007/s11098-012-9914-7
Armitage, K. L., Bulley, A., and Redshaw, J. (2020). Developmental origins of cognitive offloading. Proc. R. Soc. B 287:20192927. doi: 10.1098/rspb.2019.2927
Bagga, D., Reichert, J. L., Koschutnig, K., Aigner, C. S., Holzer, P., Koskinen, K., et al. (2018). Probiotics drive gut microbiome triggering emotional brain signatures. Gut Microbes 9, 1–11. doi: 10.1080/19490976.2018.1460015
Bayne, T., Brainard, D., Byrne, R. W., Chittka, L., Clayton, N., Heyes, C., et al. (2019). What is cognition? Curr. Biol. 29, R608–R615. doi: 10.1016/j.cub.2019.05.044
Bedecarrats, A., Chen, S., Pearce, K., Cai, D., and Glanzman, D. (2018). RNA from trained Aplysia can induce an epigenetic engram for long-term sensitization in untrained Aplysia. eNeuro. 5:e0038. doi: 10.1523/ENEURO.0038-18.2018
Beer, R. D. (2000). Dynamical approaches to cognitive science. Trends Cogn. Sci. 4, 91–99. doi: 10.1016/S1364-6613(99)01440-0
Bercik, P., Denou, E., Collins, J., Jackson, W., Lu, J., Jury, J., et al. (2011). The intestinal microbiota affect central levels of brain-derived neurotropic factor and behavior in mice. Gastroenterology 141, 599–609.e3. doi: 10.1053/j.gastro.2011.04.052
Boem, F., Ferretti, G., and Caiani, S. Z. (2021). Out of our skull, in our skin: the microbiota-gut-brain axis and the extended cognition thesis. Biol. Philos. 36:14. doi: 10.1007/s10539-021-09790-6
Boussard, A., Delescluse, J., Pérez-Escudero, A., and Dussutour, A. (2019). Memory inception and preservation in slime moulds: the quest for a common mechanism. Philos. Trans. R. Soc. Lond. Ser. B Biol. Sci. 374:20180368. doi: 10.1098/rstb.2018.0368
Bravo, J. A., Forsythe, P., Chew, M. V., Escaravage, E., Savignac, H. M., Dinan, T. G., et al. (2011). Ingestion of lactobacillus strain regulates emotional behavior and central GABA receptor expression in a mouse via the vagus nerve. Proc. Natl. Acad. Sci. U. S. A. 108, 16050–16055. doi: 10.1073/pnas.1102999108
Burr, C. (2017). “Embodied decisions and the predictive brain” in Philosophy and Predictive Processing. eds. T. Metzinger and W. Wiese, vol. 7 (Frankfurt am Main, Germany: MIND Group), 1–23.
Calovi, D. S., Bardunias, P., Carey, N., Turner, J. S., Nagpal, R., and Werfel, J. (2019). Surface curvature guides early construction activity in mound-building termites. Philos. Trans. R. Soc. Lond. Ser. B Biol. Sci. 374:20180374. doi: 10.1098/rstb.2018.0374
Camazine, S., Deneubourg, J.-L., Franks, N. R., Sneyd, J., Theraulaz, G., and Bonabeau, E. (2003). Self-Organization in Biological Systems. Princeton, USA: Princeton University Press
Castiello, U. (2021). (re)claiming plants in comparative psychology. J. Comp. Psychol. 135, 127–141. doi: 10.1037/com0000239
Cazalis, R., Carletti, T., and Cottam, R. (2017). The living organism: strengthening the basis. Biosystems 158, 10–16. doi: 10.1016/j.biosystems.2017.04.007
Chevalier, G., Siopi, E., Guenin-Macé, L., Pascal, M., Laval, T., Rifflet, A., et al. (2020). Effect of gut microbiota on depressive-like behaviors in mice is mediated by the endocannabinoid system. Nat. Commun. 11:6363. doi: 10.1038/s41467-020-19931-2
Clark, A. (1997). Being There: Putting Brain, Body and World Together Again. Cambridge, MA: MIT Press
Clark, A., and Chalmers, D. (1998). The extended mind. Analysis 58, 7–19. doi: 10.1093/analys/58.1.7
Coles, N. A., Larsen, J. T., and Lench, H. C. (2019). A meta-analysis of the facial feedback literature: effects of facial feedback on emotional experience are small and variable. Psychol. Bull. 145, 610–651. doi: 10.1037/bul0000194
Craver, C. F., Glennan, S., and Povich, M. (2021). Constitutive relevance & mutual manipulability revisited. Synthese 199, 8807–8828. doi: 10.1007/s11229-021-03183-8
Donà, E., Barry, J. D., Valentin, G., Quirin, C., Khmelinskii, A., Kunze, A., et al. (2013). Directional tissue migration through a self-generated chemokine gradient. Nature 503, 285–289. doi: 10.1038/nature12635
Eberhard, W. G. (2000). Spider manipulation by a wasp larva. Nature 406, 255–256. doi: 10.1038/35018636
Eberhard, W. G., and Hesselberg, T. (2012). Cues that spiders (Araneae: Araneidae, Tetragnathidae) use to build orbs: lapses in attention to one set of cues because of dissonance with others? Ethology 118, 610–620. doi: 10.1111/j.1439-0310.2012.02048.x
Engert, V., and Pruessner, J. C. (2008). Dopaminergic and noradrenergic contributions to functionality in ADHD: the role of methylphenidate. Curr. Neuropharmacol. 6, 322–328. doi: 10.2174/157015908787386069
Evans, I. R., and Wood, W. (2014). Drosophila blood cell chemotaxis. Curr. Opin. Cell Biol. 30, 1–8. doi: 10.1016/j.ceb.2014.04.002
Facchini, G., Lazarescu, A., Perna, A., and Douady, S. (2020). A growth model driven by curvature reproduces geometric features of arboreal termite nests. J. R. Soc. Interface 17:20200093. doi: 10.1098/rsif.2020.0093
Falik, O., Hoffmann, I., and Novoplansky, A. (2014). Say it with flowers: flowering acceleration by root communication. Plant Signal. Behav. 9:e28258. doi: 10.4161/psb.28258
Falik, O., Reides, P., Gersani, M., and Novoplansky, A. (2005). Root navigation by self inhibition. Plant Cell Environ. 28, 562–569. doi: 10.1111/j.1365-3040.2005.01304.x
Fouquet, D., Costa-Leonardo, A. M., Fournier, R., Blanco, S., and Jost, C. (2014). Coordination of construction behavior in the termite Procornitermes araujoi: structure is a stronger stimulus than volatile marking. Insect. Soc. 61, 253–264. doi: 10.1007/s00040-014-0350-x
Gagliano, M. (2013). Green symphonies: a call for studies on acoustic communication in plants. Behav. Ecol. 24, 789–796. doi: 10.1093/beheco/ars206
Gagliano, M. (2015). In a green frame of mind: perspectives on the behavioural ecology and cognitive nature of plants. AoB Plants 7:plu075. doi: 10.1093/aobpla/plu075
Gazzaniga, M. S. (1967). The split brain in man. Sci. Am. 217, 24–29. doi: 10.1038/scientificamerican0867-24
Golstein, D. G., and Gigerenzer, G. (2002). Models of ecological rationality: the recognition heuristic. Psychol. Rev. 109, 75–90. doi: 10.1037/0033-295X.109.1.75
Grassé, P.-P. (1959). La théorie de la stigmergie: essai d’interprétation du comportement des termites constructeurs. Insect. Soc. 6, 41–80. doi: 10.1007/BF02223791
Harms, W. F. (2006). What is information? Three concepts. Biol. Theory 1, 230–242. doi: 10.1162/biot.2006.1.3.230
Heil, M., and Karban, R. (2010). Explaining evolution of plant communication by airborne signals. Trends Ecol. Evol. 25, 137–144. doi: 10.1016/j.tree.2009.09.010
Heil, M., and Kost, C. (2006). Priming of indirect defences. Ecol. Lett. 9, 813–817. doi: 10.1111/j.1461-0248.2006.00932.x
Heil, M., and Silva Bueno, J. C. (2007). Within-plant signaling by volatiles leads to induction and priming of an indirect plant defense in nature. Proc. Natl. Acad. Sci. U. S. A. 104, 5467–5472. doi: 10.1073/pnas.0610266104
Hesselberg, T., and Vollrath, F. (2004). The effects of neurotoxins on web geometry and web-building behaviour in Araneus diadematus cl. Physiol. Behav. 82, 519–529. doi: 10.1016/j.physbeh.2004.04.058
Hobson, E. A., Mønster, D., and DeDeo, S. (2021). Aggression heuristics underlie animal dominance hierarchies and provide evidence of group-level social information. Proc. Natl. Acad. Sci. U. S. A. 118:e2022912118. doi: 10.1073/pnas.2022912118
Huang, A. C., Jiang, T., Liu, Y.-X., Bai, Y.-C., Reed, J., Qu, B., et al. (2019). A specialized metabolic network selectively modulates Arabidopsis root microbiota. Science 364:eaau6389. doi: 10.1126/science.aau6389
Japyassú, H. F., and Laland, K. N. (2017). Extended spider cognition. Anim. Cogn. 20, 375–395. doi: 10.1007/s10071-017-1069-7
Kaplan, D. M. (2012). How to demarcate the boundaries of cognition. Biol. Philos. 27, 545–570. doi: 10.1007/s10539-012-9308-4
Kelly, J. R., Borre, Y., O’Brian, C., Patterson, E., El Aidy, S., Deane, J., et al. (2016). Transferring the blues: depression-associated gut microbiota induces neurobehavioural changes in the rat. J. Psychiatr. Res. 82, 109–118. doi: 10.1016/j.jpsychires.2016.07.019
Krause, J., Ruxton, G. D., and Krause, S. (2010). Trends Ecol. Trends Ecol. Evol. 25, 28–34. doi: 10.1016/j.tree.2009.06.016
Krickel, B. (2018). Saving the mutual manipulability account of constitutive relevance. Stud. Hist. Phil. Sci. 68, 58–67. doi: 10.1016/j.shpsa.2018.01.003
Latty, T., and Beekman, M. (2015). Slime moulds use heuristics based on within-patch experience to decide when to leave. J. Exp. Biol. 218, 1175–1179. doi: 10.1242/jeb.116533
Ludwig, D. (2015). Extended cognition and the explosion of knowledge. Philos. Psychol. 28, 355–368. doi: 10.1080/09515089.2013.867319
Lyon, P. (2006). The biogenic approach to cognition. Cogn. Process. 7, 11–29. doi: 10.1007/s10339-005-0016-8
Lyon, P., Keijzer, F., Arendt, D., and Levin, M. (2021). Reframing cognition: getting down to biological basics. Philos. Trans. R. Soc. Lond. Ser. B Biol. Sci. 376:20190750. doi: 10.1098/rstb.2019.0750
Mahall, B. E., and Callaway, R. M. (1991). Root communication among desert shrubs. Proc. Natl. Acad. Sci. U. S. A. 88, 874–876. doi: 10.1073/pnas.88.3.874
Mahall, B. E., and Callaway, R. M. (1992). Root communication mechanisms and intracommunity distributions of two Mojave Desert shrubs. Ecology 73, 2145–2151. doi: 10.2307/1941462
Mathel, J. A., and Dickel, L. (2017). Complex cephalopod cognition. Curr. Opin. Behav. Sci. 16, 131–137. doi: 10.1016/j.cobeha.2017.06.008
Maturana, H. R., and Varela, F. J. (1980). Autopoiesis and Cognition: The Realization of the Living. Dordrecht, Holland: D. Reidel Publishing Company
Menary, R. (2010a). “Cognitive integration and the extended mind” in The Extended Mind. ed. R. Menary (Cambridge, MA: The MIT Press), 189–225.
Mortimer, B., Soler, A., Siviour, C. R., and Vollrath, F. (2018). Remote monitoring of vibrational information in spider webs. Sci. Nat. 105, 37–39. doi: 10.1007/s00114-018-1561-1
Nakata, K. (2010). Attention focusing in a sit-and-wait forager: a spider controls its prey-detection ability in different web sectors by adjusting thread tension. Proc. R. Soc. B Biol. Sci. 277, 29–33. doi: 10.1098/rspb.2009.1583
Nakata, K. (2013). Spatial learning affects thread tension control in orb-web spiders. Biol. Lett. 9, 20130052–20130054. doi: 10.1098/rsbl.2013.0052
Onishi, S., Tobita, K., and Makioka, S. (2022). Hand constraint reduces brain activity and affects the speed of verbal responses on semantic tasks. Sci. Rep. 12:13545. doi: 10.1038/s41598-022-17702-1
Parise, A. G., Gagliano, M., and Souza, G. (2020). Extended cognition in plants: is it possible? Plant Signal. Behav. 15:1710661. doi: 10.1080/15592324.2019.1710661
Perry, C. J., Barron, A. B., and Chittka, L. (2017). The frontiers of insect cognition. Curr. Opin. Behav. Sci. 16, 111–118. doi: 10.1016/j.cobeha.2017.05.011
Reid, C. R., Latty, T., Dussutour, A., and Beekman, M. (2012). Slime mold uses an externalized spatial “memory” to navigate in complex environments. Proc. Natl. Acad. Sci. U. S. A. 109, 17490–17494. doi: 10.1073/pnas.1215037109
Rupert, R. D. (2004). Challenges to the hypothesis of extended cognition. J. Philos. 101, 389–428. doi: 10.5840/jphil2004101826
Scott, G. A., Terstege, D. J., Vu, A. P., Law, S., Evans, A., and Epp, J. R. (2020). Disrupted neurogenesis in germ-free mice: effects of age and sex. Front. Cell Dev. Biol. 8:407. doi: 10.3389/fcell.2020.00407
Shannon, C. E. (1948). A mathematical theory of communication. Bell Syst. Tech. J. 27, 379–423. doi: 10.1002/j.1538-7305.1948.tb01338.x
Shapiro, J. A. (2007). Bacteria are small but not stupid: cognition, natural genetic engineering and socio-bacteriology. Stud. Hist. Phil. Biol. Biomed. Sci. 38, 807–819. doi: 10.1016/j.shpsc.2007.09.010
Shapiro, J. A. (2021). All living cells are cognitive. Biochem. Biophys. Res. Commun. 564, 134–149. doi: 10.1016/j.bbrc.2020.08.120
Simon, H. (1955). A behavioral model of rational choice. Q. J. Econ. 69, 99–118. doi: 10.2307/1884852
Sims, M., and Kiverstein, J. (2022). Externalized memory in slime mould and the extended (non-neural) mind. Cogn. Syst. Res. 73, 26–35. doi: 10.1016/j.cogsys.2021.12.001
Smart, P. R. (2022). Toward a mechanistic account of extended cognition. Philos. Psychol. 35, 1107–1135. doi: 10.1080/09515089.2021.2023123
Smart, P., Clowes, R. W., and Heersmink, R. (2017). Minds online: the interface between web science, cognitive science and the philosophy of mind. Foundat. Trends Web Sci. 6, 1–232. doi: 10.1561/1800000026
Souza, G. M., Toledo, G. R. A., and Saraiva, G. F. R. (2018). “Towards systemic view for plant learning: ecophysiological perspective” in Memory and Learning in Plants. eds. F. Baluška, M. Gagliano, and G. Witzany (Cham: Springer), 163–190.
Sutton, J. (2010). “Exograms and interdisciplinarity: history, the extended mind, and the civilizing process” in The Extended Mind. ed. R. Menary (Cambridge, MA: The MIT Press), 189–225.
Tillisch, K., Labus, J., Kilpatrick, L., Jiang, Z., Stains, J., Ebrat, B., et al. (2013). Consumption of fermented milk product with probiotic modulates brain activity. Gastroenterology 144, 1394–1401.e4. doi: 10.1053/j.gastro.2013.02.043
Trewavas, A. (2016). Intelligence, cognition, and language of green plants. Front. Psychol. 7:588. doi: 10.3389/fpsyg.2016.00588
Tweedy, L., Susanto, O., and Insall, R. H. (2016). Self-generated chemotactic gradients—cells steering themselves. Curr. Opin. Cell Biol. 42, 46–51. doi: 10.1016/j.ceb.2016.04.003
Tweedy, L., Thomason, P. A., Paschke, P. I., Martin, K., Machesky, L. M., Zagnoni, M., et al. (2020). Seeing around corners: cells solve mazes and respond at a distance using attractand breakdown. Science 369:eaay9792. doi: 10.1126/science.aay9792
Varela, F., Thompson, E., and Rosch, E. (2016). The Embodied Mind: Cognitive Science and Human Experience. Cambridge, MA: MIT Press.
Vogel, D., and Dussutour, A. (2016). Direct transfer of learned behaviour via cell fusion in non-neural organisms. Proc. R. Soc. Lond. B Biol. Sci. 283:20162382. doi: 10.1098/rspb.2016.2382
Watanabe, T. (2000). Web tuning of an orb-web spider, Octonoba sybotides, regulates prey-catching behaviour. Proc. R. Soc. Lond. B Biol. Sci. 267, 565–569. doi: 10.1098/rspb.2000.1038
Weiner, N. (1948). Cybernetics or the Control and Communication in the Animal and the Machine. Cambridge, USA: MIT Press
Wheeldon, C. D., Walker, C. H., Hamon-Josse, M., and Bennett, T. (2021). Wheat plants sense substrate volume and root density to proactively modulate shoot growth. Plant Cell Environ. 44, 1202–1214. doi: 10.1111/pce.13984
Witt, P. N. (1969). Behavioral consequences of laser lesions in the central nervous system of Araneus diadematus cl. Am. Zool. 9, 121–131. doi: 10.1093/icb/9.1.121
Witt, P. N. (1971). Drugs alter web-building of spiders: a review and evaluation. Behav. Sci. 16, 98–113. doi: 10.1002/bs.3830160109
Witzany, G., and Baluška, F. (2012). Life’s code script does not code itself: the machine metaphor for living organisms is outdated. EMBO Rep. 13, 1054–1056. doi: 10.1038/embor.2012.166
Yamagushi, T., Wang, H.-L., Li, X., Ng, T. H., and Morales, M. (2011). Mesocorticolimbic glutamatergic pathway. J. Neurosci. 31, 8476–8490. doi: 10.1523/JNEUROSCI.1598-11.2011
Yuan, J., Zhao, J., Wen, T., Zhao, M., Li, R., Goossens, P., et al. (2018). Root exudates drive the soil-borne legacy of aboveground pathogen infection. Microbiome 6, 1–12. doi: 10.1186/s40168-018
Keywords: extended cognition, behavioural ecology, cognitive traits, niche construction, navigation, memory, spider behaviour, plant behaviour
Citation: Parise AG, Gubert GF, Whalan S and Gagliano M (2023) Ariadne’s thread and the extension of cognition: A common but overlooked phenomenon in nature? Front. Ecol. Evol. 10:1069349. doi: 10.3389/fevo.2022.1069349
Edited by:
José F. Fontanari, University of São Paulo, BrazilReviewed by:
Hilton F. Japyassú, Federal University of Bahia, BrazilEnrico Petracca, University of Bologna, Italy
Copyright © 2023 Parise, Gubert, Whalan and Gagliano. This is an open-access article distributed under the terms of the Creative Commons Attribution License (CC BY). The use, distribution or reproduction in other forums is permitted, provided the original author(s) and the copyright owner(s) are credited and that the original publication in this journal is cited, in accordance with accepted academic practice. No use, distribution or reproduction is permitted which does not comply with these terms.
*Correspondence: André Geremia Parise, ✉ YS5wYXJpc2VAcGdyLnJlYWRpbmcuYWMudWs=