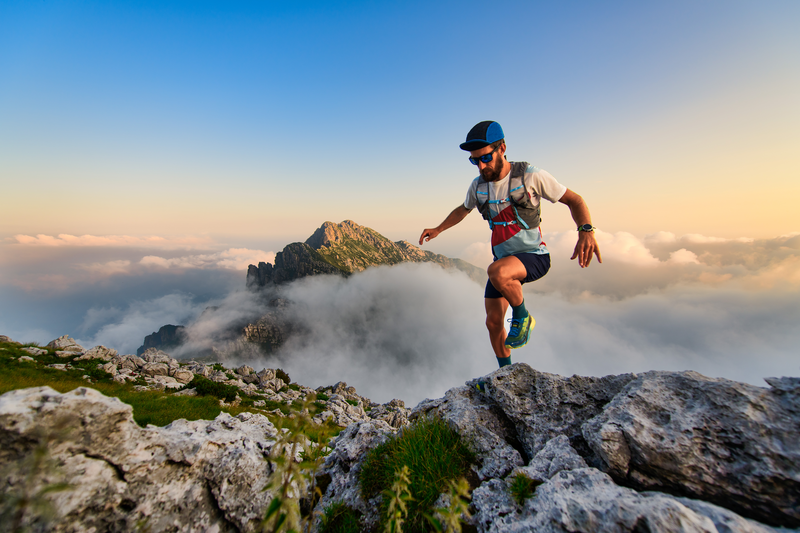
94% of researchers rate our articles as excellent or good
Learn more about the work of our research integrity team to safeguard the quality of each article we publish.
Find out more
EDITORIAL article
Front. Ecol. Evol. , 04 January 2023
Sec. Population, Community, and Ecosystem Dynamics
Volume 10 - 2022 | https://doi.org/10.3389/fevo.2022.1068096
This article is part of the Research Topic Kin Selection and Kin Cooperation in Plants View all 7 articles
Editorial on the Research Topic
Kin selection and kin cooperation in plants
Plants have evolved to make the most of the limited resources they can acquire from their environment. This evolution has led to the wide range of different life-history and growth strategies observed among species occupying the different abiotic environments around the world. Within communities, resources are carved up among individuals in the “economy of nature” also as a function of different growth decisions. Much of the variation we observe among species is the result of genetic divergence in evolved strategies. Within populations, however, phenotypic variation can often be attributed to the expression of phenotypic plasticity. An important component of this plasticity is due to “decisions” taken in the face of competition with neighbors. But all neighbors are not equal. The differ in competitive ability, and, critically, in how closely related they are to one another. Kin-selection theory has long shown how animals should alter their interactions with one another as a function of how closely related they are to one another, but the same principles also apply to all organisms, including microbes and plants (West et al., 2021). In this Research Topic, six papers are brought together that consider how kin relationships among neighbors might affect plants.
The concept of kin selection, a term introduced by Maynard Smith (1964), was appreciated by Darwin (1859), who recognized that the behavior sterile workers in a bee colony could benefit their fitness by increasing the reproductive success of their “stock” or, in terms that Hamilton (1964) explained formally, their “inclusive fitness”. Hamilton (1964) was the first to apply it to plants, pointing to seed dormancy and seed number per ovule as possible mechanisms to increase cooperation and reduce conflict among relatives. For instance, seed dormancy may be seen as a bet-hedging strategy by the mother to have its seeds germinate in different environments at different times (Hamilton, 1964; Penfield, 2017). The mother extends this control through the water-permeability of the seed coat, an entirely maternal tissue. Similarly, seed dispersal can reduce competition among siblings by ensuring the placement of seeds from a mother in different locations. But kin selection could play a role in the evolution of any trait that involves interactions among different individuals in a population. Its scope in plants should be particularly great because plant populations are very often genetically structured as a result of limited dispersal of seeds and pollen (Vekemans and Hardy, 2004).
Kin selection could act on plant populations at any or all stages in the plant life cycle, from gametophytes (the haploid gamete-producing phase) to sporophytes (the usually dominant, diploid phase of plants). Development of many embryos from different pollen parents within the ovary of a maternal parent is widespread in flowering plants. Competitive interactions among such siblings set the stage for gains in inclusive fitness through sacrifice and cooperation depending on their genetic relatedness that can vary with diversity of pollen parents of seeds within single ovaries or fruits (Hamilton, 1964; Kress, 1981; Bawa, 2016). Such interactions may have driven the evolution of many reproductive traits in plants (Bawa, 2016). Hypotheses to explain the evolution of double fertilization and the arcane variation among some lineages of embryo-sac cytology are beautifully illustrative of the power of kin-selection thinking (Haig, 1987).
Double fertilization refers to the union of the second sperm of the male gametophyte (issued from the pollen tube when it enters the ovule) with (usually) two haploid polar nuclei to form a triploid endosperm, a tissue analogous to placenta of mammals, that nourishes the developing embryo. Kin selection provides an explanation for this bizarre phenomenon. As Haig explains in his contribution to this Research Topic, the evolution of double fertilization is plausibly the resolution of discrepancies between the genetic interests of the mother and father of developing embryos in an ovary (Charnov, 1979; Queller, 1983; Friedman, 1998). While the father gains some control over investment by contributing his genes to the nourishing endosperm, the mother also retains control by contributing two copies. Genetic imprinting further contributes to this maternal control (Costa et al., 2012).
In his review, Haig lays out the details of the genetic events that lead to the production of the embryo sac in which fertilization takes place. Such events do vary among some lineages (and he exhorts us to study these cases), but the almost ubiquitous maintenance across flowering plants of endosperm with three rather than more copies of the plant genome suggests that it might be a “‘sweet spot' that reduces sibling competition for maternal resources within the constraints of conserved features of angiosperm developmental and molecular biology”. Indeed, Haig's article emphasizes the need to interpret embryo-sac cytology in developmental terms, with a view to how the details of meiosis and downstream organization of its products determine relatedness among embryo-sac cells. Haig revisits ideas that he first published as products of his doctoral work over 30 years ago, but now with the benefit of subsequent work, including observations on the effects of genome dosage that have confirmed early intuitions based on kin selection. His work also advances a kin-conflict interpretation of a number of unusual cases, such as where more than one haploid product of meiosis generates the multicellular embryo sac.
Whereas, Haig addresses variation within the developing seed coat as a stage for kin selection, Shivaprakash and Bawa examine the topological evolution of seeds in the ovary—their placentation. Placentation characterizes the number and spatial arrangement of ovules. In multi-ovulated ovaries, growing embryos with different degree of relatedness to each other and to the mother through the intervening endosperm can the set the stage for competition and cooperation among embryos. Thus, kin selection has the potential to influence the evolution of the number of ovules developing in the ovary and the ways such ovules are isolated from each other. The authors show that evolutionary trends in placentation could be explained in the context of kin selection.
Much work invoking kin selection in plants has been directed at interactions between growing and competing sporophytes. Kin selection in this context would thus seem to be between individuals that are more separated from one another than cells of an embryo sac or seeds within an ovary. As noted above, the strong patterns of relatedness in plant populations give us every reason to expect selection to shape interactions as a function of kin, but an obvious pre-requisite is for interacting individuals to recognize, and to determine how closely they are related to, their neighbors. In recent years, many studies have implicated kin recognition when plants interact as plants establishing and competing for resources in soil or for light. The ratio of shoot:root allocation and the arrangement of leaves have been found to vary with relatedness among neighbors (Li et al., 2017). The remaining four articles in the Research Topic deal with this aspect of kin selection in plants, notably in terms of now plants might modify their behavior in response to communication among roots.
Biedrzycki and Bais offer us a review of this literature. They define kin recognition in terms of “differences in plant growth patterns […] or physiological processes […] between the plants when grown with related (kin) versus non-related (stranger or non-kin) plants”. This view is common, but we also need to remember that kin may differ from non-kin in an experiment also because of genetically different competitive abilities (e.g., Klemens, 2008). Biedrzycki and Bais consider evidence for the effect of neighbor identity on uptake by plants of nutrients, citing a number of studies that indicate that the way plants respond to kin vs. non-kin may also depend on the abiotic context, i.e., on the extent to which nutrients are limiting, or on the presence or concentration of stressors such as heavy metal ions or drought. For instance, while kin responses of plants of Sorghum vulgare appear to depend on nutrient conditions and cadmium contamination (Li et al., 2018), a study of root growth patterns in the clonally propagating species Glechoma heteracea failed to record interactions between responses to kin and drought as a stressor (Goddard et al.).
The work on G. heteracea cited by Biedrzycki and Bais in their review features in the Research Topic, too. In this paper, the authors report on experiments in which they grew plants either alone or with kin (a clone of the same genotype), with roots penetrating soil in only one pot or divided between two (Biedrzycki and Bais). They compared root growth both in terms of length extension following harvest and in terms of where the roots were growing in the pots—in order to discern potential patterns of avoidance of a neighbor's roots—and the extent to which sensitivity of root growth to neighbor relatedness depended on water availability. Their study confirmed previous evidence for kin recognition in G. heteracea, especially in terms of neighbor avoidance behavior, though not in an interaction with water availability.
In a second empirical study on sporophyte growth, Asay et al. present a study on the effects of kin recognition on Douglas fir (Pseudotsuga menziesii var. glauca) in the context of the presence or absence of a different conifer species. Whereas, research on root-root recognition has largely been conducted in monospecific situations, the experiments reported by Asay et al. represent the important necessary step toward considering the evolution and expression of kin selection in more biotically complex environments.
Another article that considers interspecific interactions is contributed by Torices et al., who present the results of an intriguing study of the way kinship relations in the context of interactions with pollinators influence flower color and pollination success. They show that in wild populations of Moricandia moricandioides, a self-incompatible annual herb, in the arid regions of south-eastern Spain, individuals growing with kin did not have flowers with more intense purple color but had greater and larger number of flowers as well as greater nectar volume and concentration of sugar in individuals not growing with kin. They speculate on whether genetic composition patches might also affect other flower signals such as odors.
The six papers drawn together in this Research Topic represent just the tip of an iceberg in terms of how interplant relatedness might affect plant growth and development. The patterns or results reported or reviewed are tantalizing and suggestive, but we have a long way to go in framing and testing hypotheses that emerge from theory, and in providing a complete explanation of causation in what we observe. Exact mechanisms of kin recognition especially among individuals are not known (Dudley and File, 2007). Photoreceptors (Crepy and Casal, 2014), root exudates (Palmer et al., 2016; Anten and Chen, 2021), phenotype matching (Crepy and Casal, 2016) and volatile compounds emitted by above-ground tissues have been implicated. In forest trees, there is evidence of mycorrhizal mediated recognition of individuals of the same species (Gorzelak et al., 2015), but it is not known if the kin are differentially recognized (though see Asay et al.). As implied by a closing comment in Haig's article, while we may have made some progress toward kin-focused explanations in terms of “final causation” (the “why?” of evolution), we will also need to do the hard work in finding explanations in terms of “efficient” causes (the “how?”)—such as identifying the molecular and chemical basis of interplant communication. But more fundamental challenges remain, too, not least in devising experiments that exclude potentially confounding explanations that might not involve kin relationships at all (e.g., Klemens, 2008). That task will not be easy.
All authors listed have made a substantial, direct, and intellectual contribution to the work and approved it for publication.
The authors declare that the research was conducted in the absence of any commercial or financial relationships that could be construed as a potential conflict of interest.
All claims expressed in this article are solely those of the authors and do not necessarily represent those of their affiliated organizations, or those of the publisher, the editors and the reviewers. Any product that may be evaluated in this article, or claim that may be made by its manufacturer, is not guaranteed or endorsed by the publisher.
Anten, N. P., and Chen, B. J. (2021). Detect thy family: mechanisms, ecology and agricultural aspects of kin recognition in plants. Plant Cell Environ. 44, 1059–1071. doi: 10.1111/pce.14011
Bawa, K. S. (2016). Kin selection and the evolution of plant reproductive traits. Proc. R. Soc. London B 283, 20160789. doi: 10.1098/rspb.2016.0789
Charnov, E. L. (1979). Simultaneous hermaphroditism and sexual selection. Proc. Natl. Acad. Sci. U. S. A. 76, 2480–2484 doi: 10.1073/pnas.76.5.2480
Costa, L. M., Yuan, J., Rouster, J., Paul, W., Dicknson, H., and Gutierrez-Marcos, J. F. (2012). Maternal control of nutrient allocation in plant seeds by genomic imprinting. Curr. Biol. 22, 160–165. doi: 10.1016/j.cub.2011.11.059
Crepy, M. A., and Casal, J. J. (2014). Photoreceptor-mediated kin recognition in plants. New Phytol. 205, 329–338. doi: 10.1111/nph.13040
Crepy, M. A., and Casal, J. J. (2016). Kin recognition by self-referent phenotype matching in plants. New Phytol. 209, 15–16. doi: 10.1111/nph.13638
Darwin, C. (1859). On the Origin of Species by Means of Natural Selection, or the Preservation of Favoured Races in the Struggle for Life. 1st Edn. London: John Murray.
Dudley, S. A., and File, A. L. (2007). Kin recognition in an annual plant. Biol. Lett. 3, 435–438. doi: 10.1098/rsbl.2007.0232
Friedman, W. E. (1998). The evolution of double fertilization and endosperm: an “historical” perspective. Sex Plant Reprod. 11, 6–16. doi: 10.1007/s004970050114
Gorzelak, M. A., Asay, A. K, Pickles, B. G., and Simarad, S. W. (2015). Inter-plant communication through mycorrhizal networks mediates complex adaptive behaviour in plant communities. AoB Plants 7, plv050. doi: 10.1093/aobpla/plv050
Haig, D. (1987). Kin conflict in seed plants. Trends Ecol. Evol. 2, 337–340. doi: 10.1016/0169-5347(87)90110-8
Hamilton, W. D. (1964). The genetical evolution of social behavior I and II. J Theor Biol. 7, 1–52. doi: 10.1016/0022-5193(64)90039-6
Kress, J. W. (1981). Sibling competition and evolution of pollen unit, ovule number, and pollen vector in angiosperms. Systemat. Bot. 6, 101–112. doi: 10.2307/2418541
Li, J., Xu, X., and Feng, R. (2018). Soil fertility and heavy metal pollution (Pb and Cd) alter kin interaction of Sorghum vulgare. Environ. Exp. Bot. 155, 368–377. doi: 10.1016/j.envexpbot.2018.05.009
Li, J., Xu, X., and Liu, Y. (2017). Kin recognition in plants with distinct lifestyles: implications of biomass and nutrient niches. Plant Grow. Reg. 84, 333–339. doi: 10.1007/s10725-017-0343-7
Maynard Smith, J. (1964). Kin selection and group selection. Nature 201, 145–1147. doi: 10.1038/2011145a0
Palmer, A. G., Ali, M., Yang, S., Parchami, N., Bento, T., Mazzella, M., et al. (2016). Kin recognition is a nutrient-dependent inducible phenomenon. Plant Signal Behav. 11:e1224045. doi: 10.1080/15592324.2016.1224045
Penfield, S. (2017). Seed dormancy and germination. Curr. Biol. 17, R874–R878. doi: 10.1016/j.cub.2017.05.050
Queller, D. C. (1983). Kin selection and conflict in seed maturation. J. Theor. Biol. 100, 153–172. doi: 10.1016/0022-5193(83)90099-1
Vekemans, X., and Hardy, O. J. (2004). New insights from fine-scale spatial genetic structure analyses in plant populations. Mol. Ecol. 13, 921–935. doi: 10.1046/j.1365-294X.2004.02076.x
Keywords: kin selection, kin cooperation, pollination, plant evolution, plant reproduction
Citation: Bawa K and Pannell JR (2023) Editorial: Kin selection and kin cooperation in plants. Front. Ecol. Evol. 10:1068096. doi: 10.3389/fevo.2022.1068096
Received: 12 October 2022; Accepted: 29 November 2022;
Published: 04 January 2023.
Edited and reviewed by: Susan Aline Dudley, McMaster University, Canada
Copyright © 2023 Bawa and Pannell. This is an open-access article distributed under the terms of the Creative Commons Attribution License (CC BY). The use, distribution or reproduction in other forums is permitted, provided the original author(s) and the copyright owner(s) are credited and that the original publication in this journal is cited, in accordance with accepted academic practice. No use, distribution or reproduction is permitted which does not comply with these terms.
*Correspondence: Kamal Bawa, a2FtYWwuYmF3YUBnbWFpbC5jb20=
Disclaimer: All claims expressed in this article are solely those of the authors and do not necessarily represent those of their affiliated organizations, or those of the publisher, the editors and the reviewers. Any product that may be evaluated in this article or claim that may be made by its manufacturer is not guaranteed or endorsed by the publisher.
Research integrity at Frontiers
Learn more about the work of our research integrity team to safeguard the quality of each article we publish.