- 1Laboratorio de Sistemática Filogenética, Red de Biología EvolutivaInstituto de Ecología, A.C., Xalapa, Mexico
- 2Laboratorio de Genética de Poblaciones, Red de Biología EvolutivaInstituto de Ecología, A.C., Xalapa, Mexico
- 3Red de Ecoetología, Instituto de Ecología, A.C., Xalapa, Mexico
To understand the evolutionary history of species, it is necessary to know the mechanisms for reproductive isolation, divergence-time between populations, and the relative action of the evolutionary forces (e.g., mutation, genetic drift, gene flow) within and between populations of the same, or closely related species. Although Canthon is one of the more diverse genera of neotropical beetles, insufficient research has been done to comprehend the divergent patterns that explain its speciation process. The absence of diagnostic morphological characters and the wide geographic variation of qualitative traits in Scarabaeinae obscures species delimitation, genealogical limits between populations, and its taxonomy. Canthon cyanellus is one of the best-known species in ecological and evolutionary aspects. It is a widely distributed species in the tropical forests of America. Also, the current deforestation has facilitated its incursion into open areas. Individuals from different populations have similar morphological characters but show wide variation in body color throughout their distribution, which makes it difficult to delimit the subspecies that comprise it. Recently, studies have been carried out to elucidate the pre-and postzygotic isolation mechanisms between populations and the historical biogeographical processes favoring cladogenesis events during the Pleistocene. Morphological variation of the male genitalia does not correspond to the phylogeographic structure. However, the morphological differences in one of the pieces of the endophallic sclerites have allowed a preliminary delimitation of some genetically differentiated clades. Finally, we consider that the joint analysis of traditional morphological taxonomy and phylogeography is important to understand the speciation process in the C. cyanellus complex.
1. Introduction
Biological evolution is a complex hierarchically structured process encompassing microevolution, speciation, and evolution above the species level (Gould, 2002). Thus, to understand the evolutionary history and the mechanisms involved in the formation of new species is necessary to know the historical biogeographical processes (e.g., the geographical separation between regions), which modify the distribution and population densities of the species (Mayr, 1963; Endler, 1977). Likewise, it is important to know the pre-and post-zygotic reproductive isolation mechanisms (Dobzhansky, 1970; Coyne and Orr, 1998), which are the first step toward the formation of new species.
With this knowledge, it is possible to test different biogeographic scenarios and establish their relationship with the patterns of genetic differentiation among populations and among species (Hewitt, 1996; Irwin, 2002). Reproductive isolation mechanisms are intensified when genetic drift, recurrent point mutation, and inbreeding affect populations with small effective sizes (Wright, 1982). In this regard, some studies have compared divergent patterns of characters (e.g., morphological, genetic, ecological, behavioral, niche, etc.) between populations of the same species, or between populations of phylogenetically close species founded evidence of genetic structure between populations, mechanisms of reproductive isolation, and morphological differences (e.g., in the grasshopper Chorthippus parallelus: Tregenza et al., 2000; in the bumblebees Bombus ephippiatus and Bombus wilmattae: Duennes et al., 2012, 2017; in the cicada Subpsaltria yangi: Liu et al., 2019).
Within the order Coleoptera, the coprophagous beetles Scarabaeinae Latreille, 1802 are very diverse (ca. 6,700 species; Bánki et al., 2022). Their widespread distribution, behavioral complexity, and diverse morphology make them a good model for studying the evolutionary processes that have given raise to them (Mullen and Shaw, 2014). Different analysis and phylogenetic approaches have brought advances in the systematics of Scarabaeinae dung beetles (Monaghan et al., 2007; Tarasov and Génier, 2015; Gunter et al., 2016; Tarasov and Dimitrov, 2016). Phylogenetic studies for taxonomic levels below subfamily are recent (Nolasco-Soto et al., 2017, 2020; Hensen et al., 2018, 2020, 2021; Maldaner et al., 2019; Pardo-Diaz et al., 2019; Mello et al., 2021; Moctezuma et al., 2021; Halffter et al., 2022). Those studies have explored different data sets (e.g., molecular, morphometric, behavioral, ecological).
The Scarabaeinae Deltochilini Lacordaire, 1856 is one of the oldest tribes along with the Dichotomiini (Davis et al., 2002). Canthon Hoffmanssegg, 1817 is the most specious lineage of neotropical Deltochilini (Halffter and Martínez, 1977). The phylogenetic relationships established within Canthon and close related genera from morphological characters indicate that it is a polyphyletic group (Medina et al., 2003). These data led to the creation of putative subgenera; however, it is difficult to set clear boundaries between species and subspecies due to the absence of diagnostic morphological characters (Cupello and Vaz-de-Mello, 2018; Vieira et al., 2019; Nunes et al., 2020; Vaz-de-Mello et al., 2020). In addition, many Scarabaeinae species exhibit intraspecific geographic variation in body color (e.g., Silvicanthon obscurus Schmidt, 1920, Phanaeus tridens Castelnau, 1840, and Canthon quinquemaculatus Castelnau, 1840). Therefore, some authors have designated subspecies based on the patterns of coloration in some species (e.g., Canthon rutilans Harold, 1868; Canthon latipes Blanchard, 1845; Phanaeus pyrois Bates, 1887; Arnaud, 2002, Medina et al., 2003).
Canthon cyanellus LeConte, 1859 is a necrophagous roller beetle from the Neotropics (Favila, 2001), which provides valuable ecosystem services similar to other Scarabaeinae (e.g., nutrient recycling, fly control, carrion removal, secondary seed dispersal, soil aeration; Nichols et al., 2008). It is a well-studied species in ecological and evolutionary aspects related to reproductive behavior (Favila, 2001; Favila et al., 2005; Chamorro-Florescano and Favila, 2008, 2009, 2016; Chamorro-Florescano et al., 2011, 2017), larval development (Hernández-Martínez and Martínez, 2003; Martínez, 2005), chemical communication (Bellés and Favila, 1983; Ortiz-Domínguez et al., 2006a,b; Cortez et al., 2012, 2015; Favila et al., 2012), population genetics and phylogeography (Ortiz-Domínguez et al., 2010; Nolasco-Soto et al., 2017; Arce-Valdés et al., 2021), among others. Traditionally, the taxonomy of C. cyanellus was based in non-informative morphological characters like variations in body color (Halffter, 1961). This has created uncertainty about genealogical limits among populations, which has led some authors not to recognize several of the subspecies (i.e., Canthon cyanellus cyanellus LeConte, 1859; C. c. sallei Harold, 1863, and C. c. violetae Halffter, 1961; Solís and Kohlmann, 2002, 2012).
Here, we will discuss the historical (e.g., cladogenesis, genetic structure) and contemporary processes (e.g., sexual recognition) that explain the evolutionary history and diversity of the C. cyanellus complex in its distribution range. This will allow a better understanding of the historical processes and mechanisms that have contributed to the formation of new species.
2. Evolutionary history of Canthon
The Scarabaeinae are of Gondwanan origin (Gunter et al., 2018). Depending on the author 12 (Smith, 2006) or 11 (Bouchard et al., 2011) tribal names have been proposed. Currently, the tribe Deltochilini encompasses over 100 genera, but according to Tarasov and Dimitrov (2016) it should comprise only 22 genera. Canthon belongs to the rolling beetle’s group, characterized by long middle and hind legs and a head without any tubercles, keels, or horns (Halffter, 1961). Nearly 180 species are included within this genus (Bánki et al., 2022). Recently, Cupello and Vaz-de-Mello (2018) based on a taxonomic review for ‘Canthon sensu lato’ concluded that at least 22 species should be considered incertae sedis as they do not belong to any of the subgenera currently recognized.
Although Canthon is mainly Neotropical, several species have Nearctic distribution (Kohlmann and Halffter, 1990). It is found from southern Canada to Argentina occupying a great diversity of ecosystems in an extremely rugged topography (Medina et al., 2003). It is absent in Chile and on the United States Pacific coast (Halffter, 1961). The hypothesis about the origin of Canthon indicates that the great richness of species that occurs in the north of South America, associated with its absence in Chile and the Patagonian region, suggests a possible center of origin in the Arquibrasil massif (Halffter, 1962). However, there is no hard data that corroborates this hypothesis.
Since 1963, Halffter has made a series of contributions to the biogeography of the Mexican entomofauna, mainly from the beetles Scarabaeidae, which propose hypotheses about its evolutionary history. These contributions suggest the existence of the biogeographic zone known as the Mexican Transition Zone (MTZ), which was invaded by different lineages (i.e., cenocrons) that evolved in the Nearctic and Neotropic zones (Morrone, 2015; Halffter, 2017; Halffter and Morrone, 2017; Morrone, 2020). Apparently, Canthon colonized the neotropical part of the MTZ during different migratory events that occurred from South to North America One migratory movement occurred before or during the Miocene, which included lineages that diversified widely in the Mexican Altiplano and the north of USA (e.g., Canthon obliquus Horn, 1894; humectus Say, 1932 and pilularius Linnaeus, 1758 species groups; Halffter, 1964; Kohlmann and Halffter, 1990; Halffter et al., 2022). A second ongoing migratory movement started after the reconstitution of the Isthmus of Panama (e.g., Canthon viridis Palisot de Beauvois, 1805, C. cyanellus and Canthon indigaceus LeConte, 1866; Halffter, 2017; Halffter and Morrone, 2017; Nolasco-Soto et al., 2017). Also, a current migratory movement associated with the expansion of rainforests in the last 10,000 years (e.g., Canthon subhyalinus Harold, 1867; Canthon euryscelis Bates, 1887, Canthon angustatus Harold, 1867) (Kohlmann and Halffter, 1990; Halffter and Morrone, 2017).
2.1. Canthon cyanellus
The mating of Canthon cyanellus takes place during the rainy season. This species uses small vertebrate carcasses to feed and reproduce (Halffter et al., 1983; Favila and Díaz, 1996). Sexual recognition occurs in the food source through cuticular compounds (Ortiz-Domínguez et al., 2006b). Sexually mature pairs cooperate in building and rolling a food ball; then, both cooperate in nest construction. At a distance of 2 to 3 m from the carcasses, the male buries the ball on which the female is mounted. On the nest, the pair copulates, and the female makes 2–6 brood balls (Favila and Díaz, 1996). One fertilized egg is laid in each brood ball; the larval development takes place in the ball until the adult hatches nearly 25 days later (Favila, 1993). The male remains in the nest with the female protecting the brood balls with chemical compounds that prevent the development of fungi and bacteria (Cortez and Favila, 2007). During his stay, the male also prevents the female from copulating with other males (Favila et al., 2005).
Canthon cyanellus has a wide distribution in the Neotropics (Figure 1): Mexico, Guatemala, Honduras, Nicaragua, Costa Rica, Panama, Trinidad, Colombia, Venezuela, Brasil, Ecuador, and Peru (Solís and Kohlmann, 2002). At its northern limit distribution, it reaches the Nearctic region to the eastern side of the United States in Texas (Halffter, 1961). Different subspecies are recognized according to the variation in body coloration (Robinson, 1948). Halffter (1961), following the ideas of Robinson (1948), places the different color morphs in a single species (i.e., C. cyanellus), but recognizes three subspecies: (1) Canthon cyanellus cyanellus LeConte, 1859 (Figure 2A) with monochromatic green or blue color; this is distributed from Texas and part of the tropical region in Mexico; it is considered synonymous with Canthon speciosus Harold, 1868 and Canthon spinosus Harold, 1863 (Howden, 1966); (2) Canthon cyanellus violetae Halffter, 1961 (Figure 2B) with the elytra dark metallic green, the pronotum, and pygidium disc, except lateral–posterior margins, are orange-reddish; it is found in southwestern Mexico. Howden (1966) suggests that C. c. violetae may be synonymous with C. c. sallei given the great similarity with individuals from Nicaragua, the type locality of C. c. sallei; and (3) Canthon cyanellus sallei Harold, 1863 (Figure 2C) has the dorsal surface largely reddish brown; its distribution ranges from Guatemala to Peru (Blackwelder, 1944). This is considered a synonym with C. sallei triangulatus Schmidt, 1920 and C. sallei gutticollis Schmidt, 1920 (Howden, 1966).
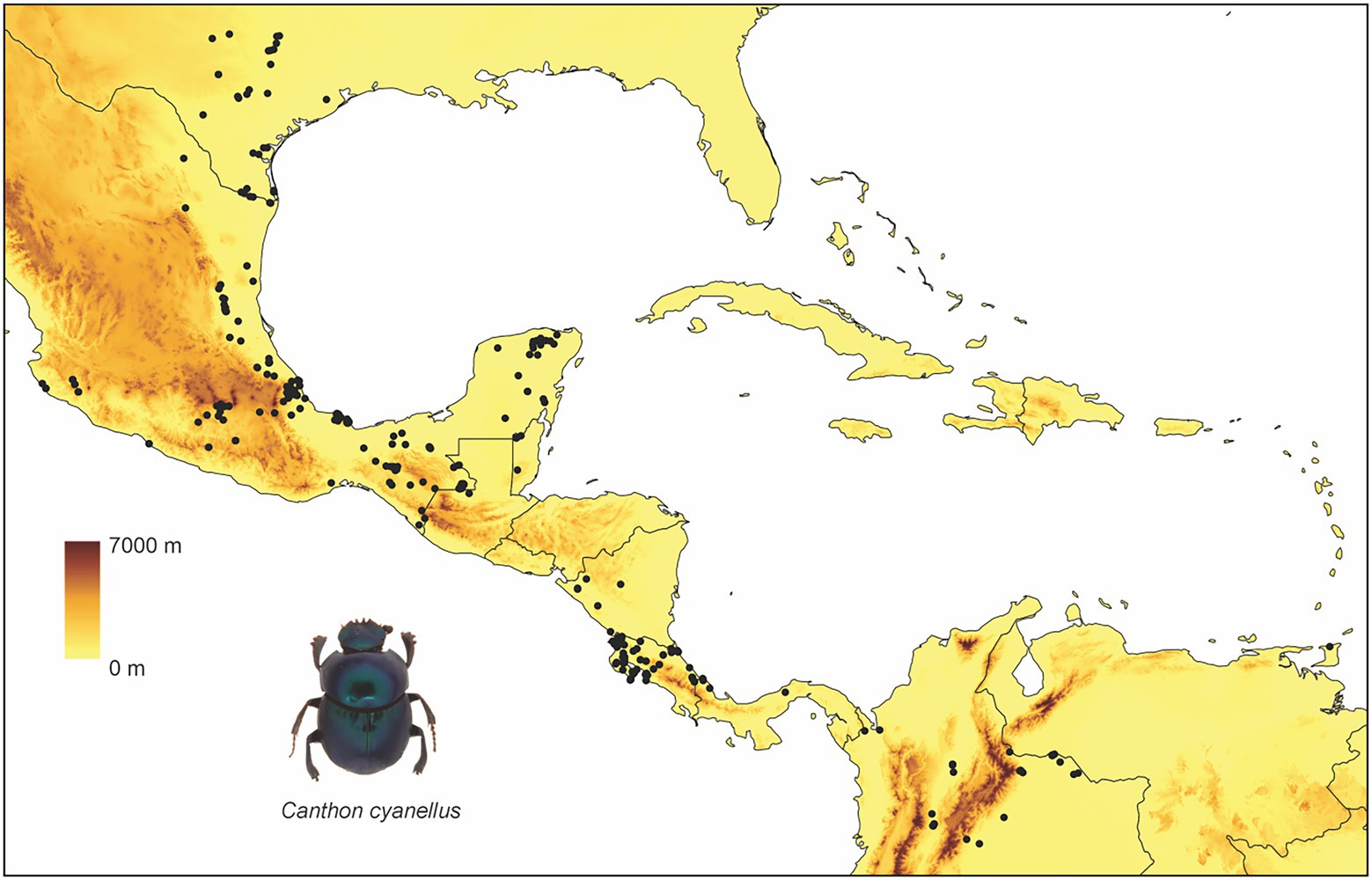
Figure 1. Distribution of Canthon cyanellus. This is how GBIF suggests citing records from different datasets: https://www.gbif.org/ occurrence download, Global Biodiversity Information Facility (2020).
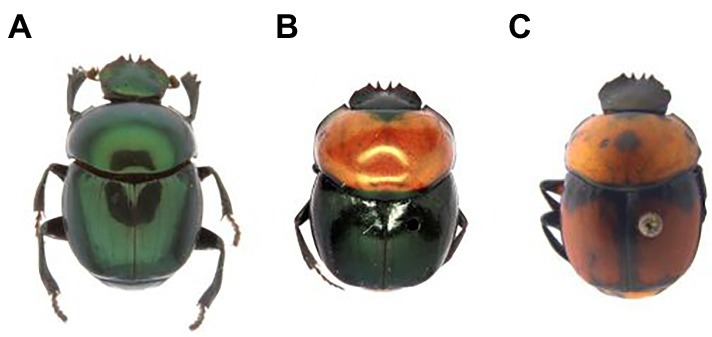
Figure 2. Canthon cyanellus subspecies sensu Halffter (1961). (A) C. c. cyanellus; (B) C. c. violetae, and (C) C. c. sallei.
Canthon cyanellus is part of the entomofauna that was established in the MTZ during the Plio-Pleistocene, following a dispersion path from South America to southern and southeastern Mexico through the Gulf slope of Mexico and the Pacific Ocean (Kohlmann and Halffter, 1990; Morrone, 2015; Halffter and Morrone, 2017). This distribution corresponds to a Typical Neotropical pattern (sensu Halffter, 1962) that is associated with tropical dry forests, cloud forests, and tropical forests with an altitudinal distribution from 0 to 2,000 m (Halffter and Morrone, 2017).
Nolasco-Soto et al. (2017, 2020) concluded that at the MTZ C. cyanellus has gone throughout at least seven cladogenetic events (i.e., GF, ChaCal, Ixt, SEM, NGM, Hua, SPS + SGM; Table 1 and Figure 3) during the Pleistocene (Figure 4). The ancestor of C. cyanellus gets in the MTZ during the Plio-Pleistocene when the main mountain systems had already formed. The most likely ancestral area for C. cyanellus in Mexico was on the Pacific slope, later the ancestor could follow an invasion route through the foothills of the Trans Mexican Volcanic Belt and then to northern and southern Gulf of Mexico (Nolasco-Soto et al., 2017). Subsequently, cladogenesis might have occurred in a north–south spatial sequence, initially separating the Chamela populations in Jalisco (Cha clade) and Goméz-Farías (GF clade) in the Sierra Madre Oriental from the rest of the southern populations (Nolasco-Soto et al., 2017). The GF population could be a relict at “El Cielo” Biosphere Reserve in Tamaulipas (Nunes, 2019). The next cladogenetic event is dated at ca. 1.43 Myr splitting the northern Gulf of Mexico populations (NGM clade), and the populations in southern Mexico. The Huatulco populations (Hua clade) split from the remaining southern ones (SGM + SPS clades) around 1.26 Myr. One more cladogenetic event that occurred at ca. 0.97 Myr separated the Chamela and GF clades. Finally, the estimated divergence time between the SGM and SPS clades occurred at ca. 0.91 Myr.
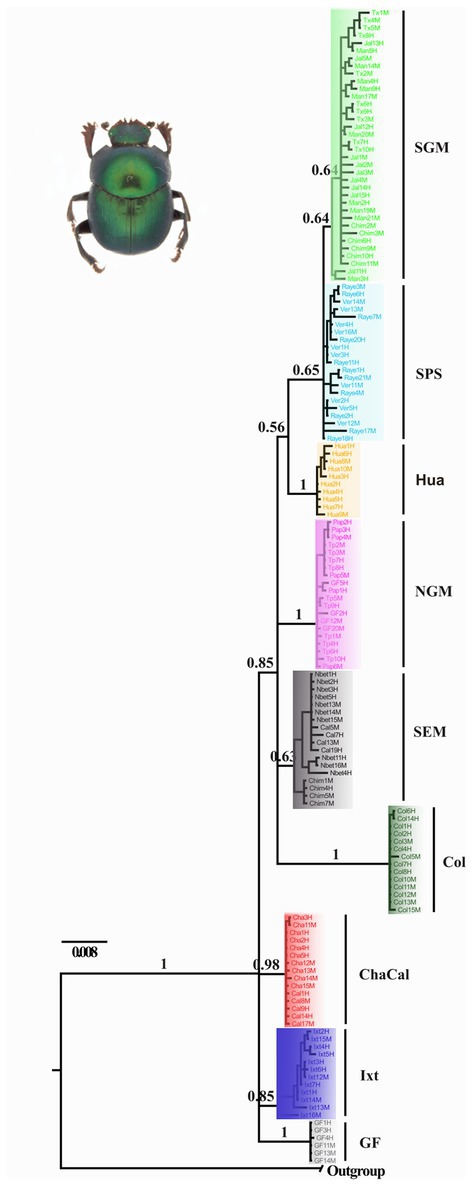
Figure 3. Bayesian consensus tree obtained by Nolasco-Soto et al. (2020) inferred from a multilocus dataset (ITS2 + 16S + COI). SGM, Jalcomulco, Los Tuxtlas, La Mancha, Los Chimalapas; SPS, El Vergel and Raymundo Enríquez; Hua, Huatulco; NGM, Papantla, Tuxpan and Gómez-Farías; SEM, Nueva Betania, Calakmul and Los Chimalapas; Col, Colombia; ChaCal, Chamela and Calakmul; Ixt, Ixtapa; GF, Gómez Farías.
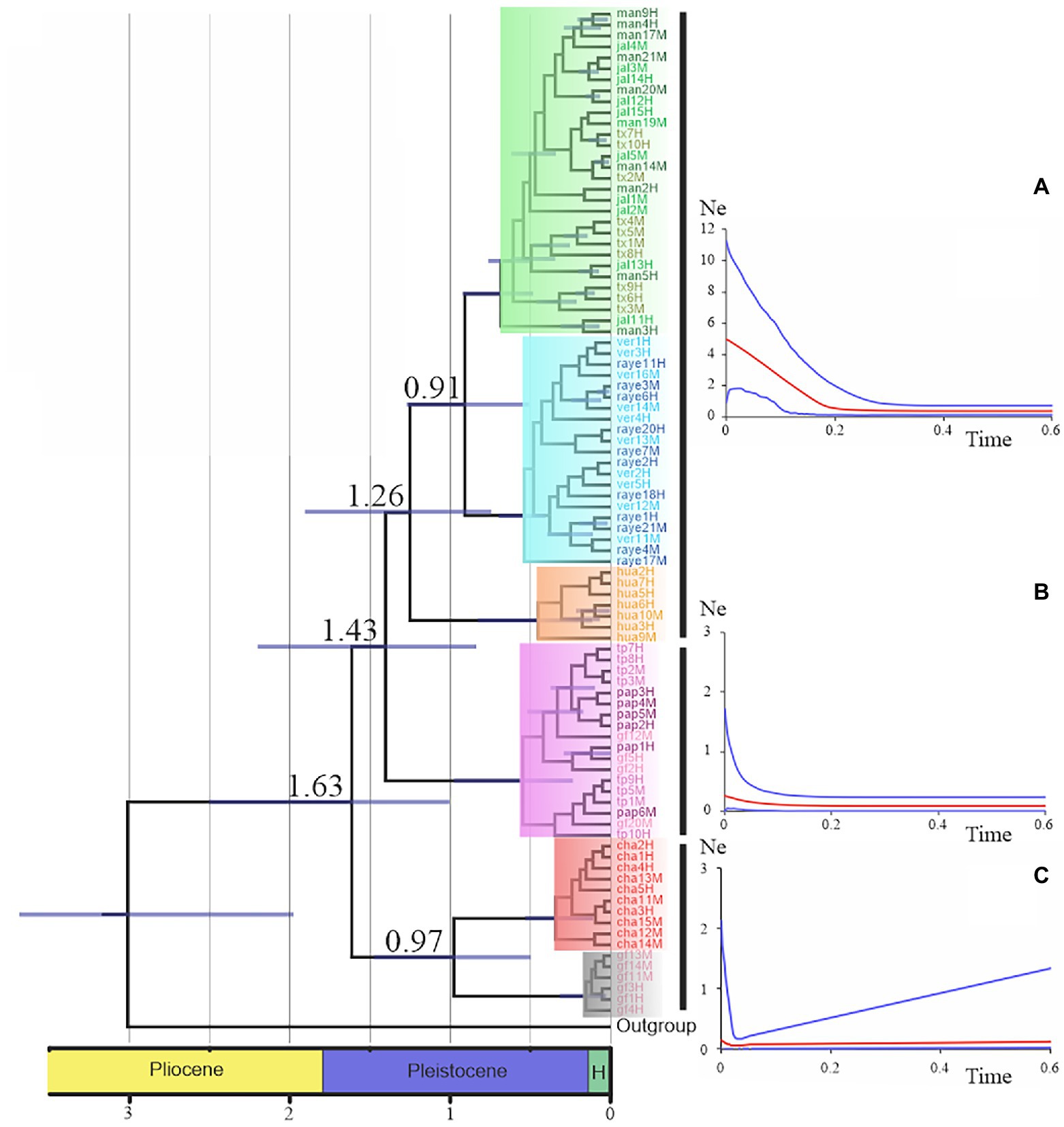
Figure 4. Chronogram and demographic history for the major clades were obtained by Nolasco-Soto et al. (2017). Time is in millions of years. Nodes with posterior probabilities above 0.9 show time uncertainty by means of 95% HPD bars. Skyland plots display the historical demographic tendency of the mayor lineages. The red line indicates the trend of the median Ne, whereas the blue lines represent the 95% confidence limits. (A) “Great lineage” demographic trend (Fu’s Fs = −31.01, p < 0.0001), (B) NGM clade demographic trend (Fu’s Fs = −4.75, p < 0.01), and (C) GF-Cha clade demographic trend (Fu’s Fs = 4.99, p < 0.01).
Those Cladogenetic events could be related to the contraction and expansion of tropical forests associated with glacial and interglacial cycles during the Pleistocene. A cladogenetic event that separated the NGM and SGM clades might be associated with the geological activity that occurred ca. 1.5 Myr east of the Trans Mexican Volcanic Belt in the state of Veracruz (Ferrari et al., 2005). Similarly, Ornelas et al. (2013) concluded that several cloud forest taxa distributed in Mexico diverge during the Pleistocene. These divergences suggest that the genetic differentiation of those species can be explained by the dynamics of the forests influenced by the climatic fluctuations of the Quaternary. On the other hand, the Isthmus of Tehuantepec may represent a biological corridor for C. cyanellus similarly as what has been observed for other taxa (e.g., fruit flies Anastrepha: Antonio-Hernández et al., 2018; Sturnira hondurensis bats: Torres-Morales, 2019).
The demographic history of the C. cyanellus populations in the MTZ indicates that they remained in stasis during most of the Pleistocene (Nolasco-Soto et al., 2017). Postglacial demographic expansions began ca. 180,000–20,000 years and continue to the present (Figure 4). Currently, these expansions can be associated with landscape changes due to tropical deforestation and intensive farming, favoring their incursion into open areas such as grasslands (Arellano et al., 2008; Salomão et al., 2018).
3. Incipient speciation
3.1. Reproductive barriers
During the speciation process, prezygotic (e.g., ecological, behavioral, reproductive seasonality, gametic incompatibility) or postzygotic (e.g., sterility or hybrid unviability) barriers are formed which restrict gene flow between populations, which leads to their differentiation (Tregenza et al., 2000; Sánchez-Guillén et al., 2012). During the divergence process between sister species, it is possible to evaluate the selection forces that act on the reproductive barriers because they are acquired during this process (grey area sensu de Queiroz, 2007).
Cuticular compounds (CHCs) mediate recognition and sexual attraction in several insect species (Chung and Carroll, 2015). In C. cyanellus, the composition of CHCs varies between sexes and allopatric populations distributed along the Gulf slope of Mexico (Ortiz-Domínguez et al., 2006a, 2006b). In the beetle populations of Gómez-Farías such variation has been associated with a change in food preferences for Diplopoda carcasses (Ortiz-Domínguez et al., 2006a). The diet affects the quantity and quality of CHCs preventing sexual recognition and mate selection (Chung and Carroll, 2015). In C. cyanellus, the differences in the composition of CHCs are not strong enough to prevent interpopulation matting. Aggressive behavior does occur during sexual recognition at least under laboratory conditions. Interpopulation crosses had low fecundity and fertility, being more evident in couples from populations separated by ≥600 km (Ortiz-Domínguez et al., 2006a). This suggested that the populations throughout its distribution are in an incipient speciation process. Similarly, Grimaldi et al. (1992) observed in heterospecific experimental crosses of allopatric populations of the Drosophila testacea that reproductive isolation is asymmetric between some crosses. This is determined by differences in sexual recognition, the lack of transfer of sperm, and hybrid unviability.
In damselflies of the genus Ischnura (Sánchez-Guillén et al., 2012, 2014), Drosophila flies (Kamimura and Mitsumoto, 2012), and Carabidae beetles (Ishikawa, 1987; Kubota and Sota, 1998), reproductive isolation it is determined by mechanical prezygotic barriers, which prevent the formation of hybrids. Even though the populations of C. cyanellus are genetically structured (Nolasco-Soto et al., 2020), the morphology of the aedeagus is similar between individuals and populations (Figures 5, 6). Instead, the morphological differences in the peripheral medial sclerite allowed a possible separation between the different subspecies of C. cyanellus (Nunes, 2019; Figure 7). In contrast, in species of beetles: Phanaeus tridens group, the morphology of the phallobase, parameres, and endophallite copulatrix are attributes that apparently are in a state of evolutionary stasis (Moctezuma et al., 2021). In Onthopagus taurus Schreber, 1759 morphology variation in four of the five endophallic sclerites influenced male’s fertilization success in the context of sperm competition (House and Simmons, 2003). In C. cyanellus, sperm competition influences paternity success (Favila et al., 2005). Therefore, it is possible, that in C. cyanellus the endophallic sclerites may be subject to sexual selection.
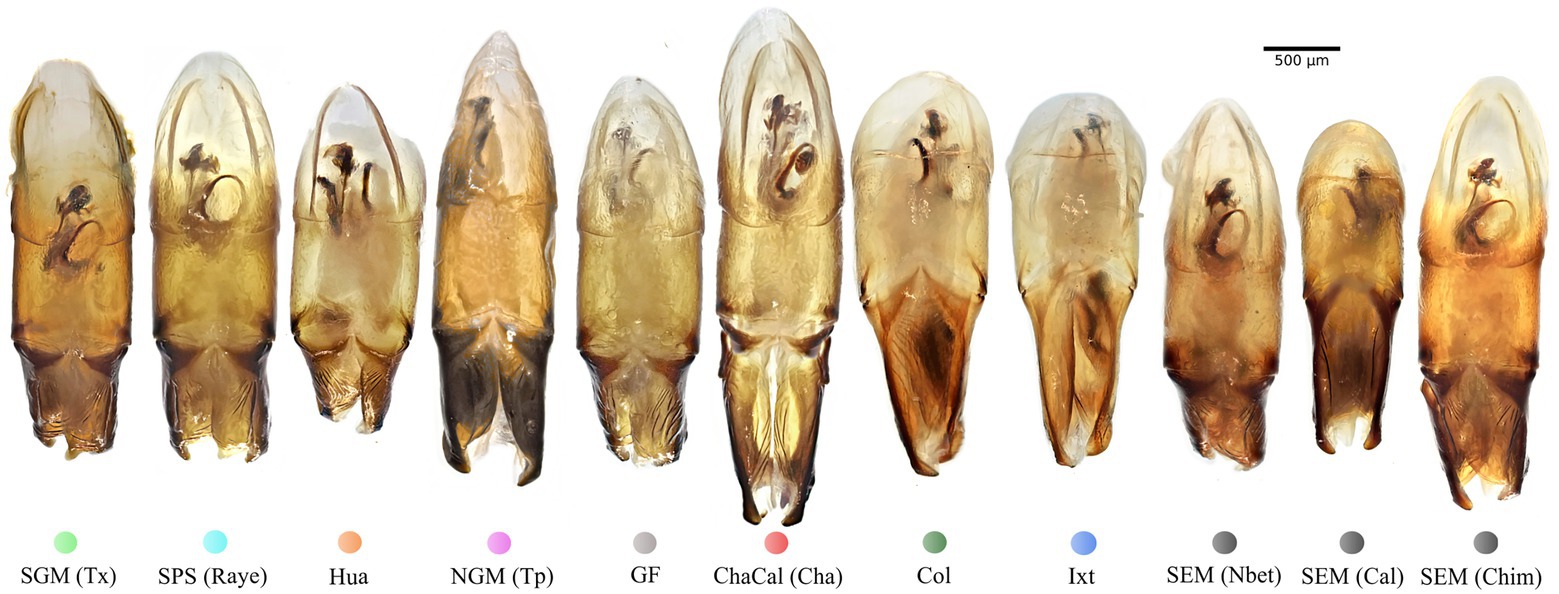
Figure 5. Aedeagus lateral view of specimens from the seven Mexican clades and the Colombian clade: Colored dots correspond to the clade color showed in the Bayesian tree on Figure 3.
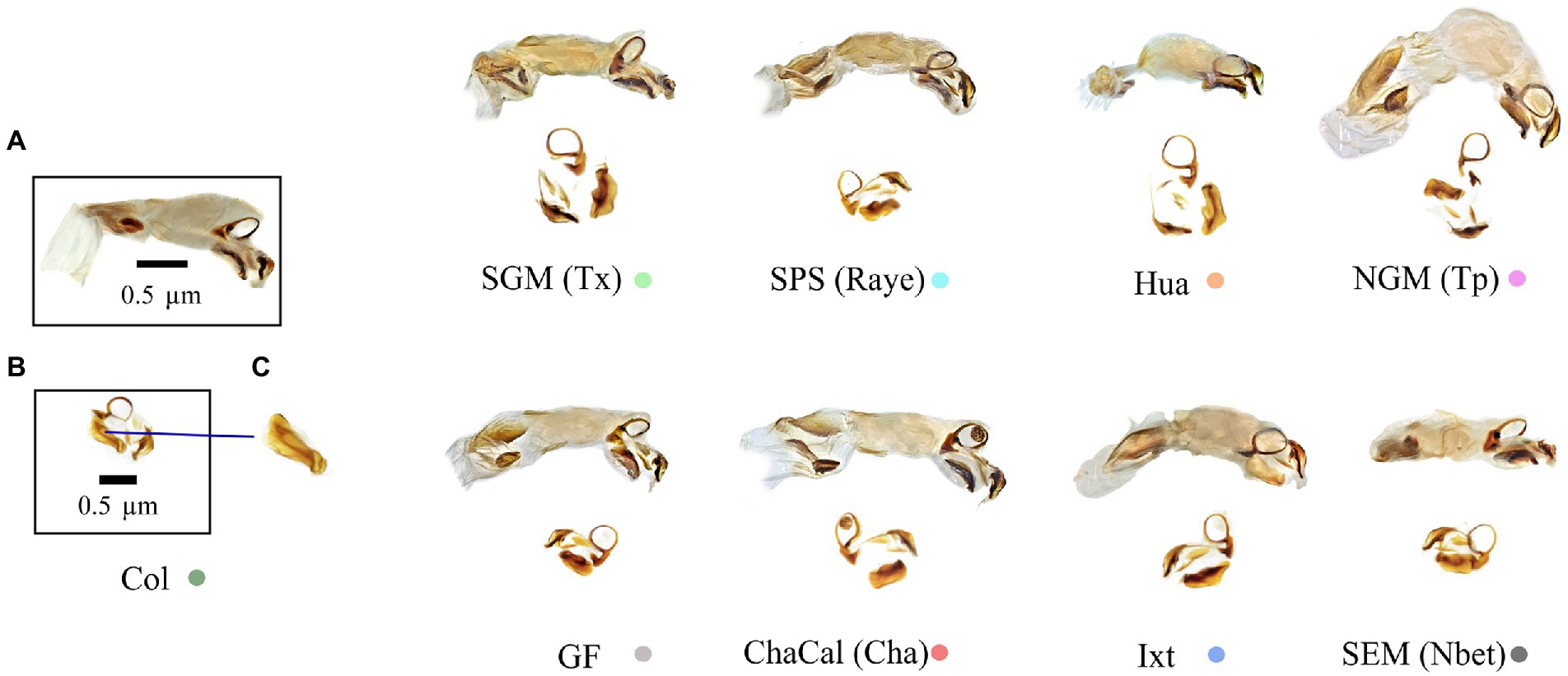
Figure 7. (A) Endophallus, (B) Endohpallites, and (C) Peripheral medial sclerite sensu Nunes (2019). The morphology of this structure allows the recognition of Canthon cyanellus clades sensu Nolasco-Soto et al. (2017, 2020). Colored dots correspond to the clade color shown in the Bayesian tree in Figure 3.
3.2. Diversity and genetic structure
Ortiz-Domínguez et al. (2010) quantified the diversity and genetic structure of five allopatric populations of C. cyanellus from tropical-forests located on the slope of the Gulf of Mexico. Those populations were structured in two groups, one to the north and one to the south. According to Nolasco-Soto et al. (2017), these groups correspond to the NGM and SGM clades. This might explain the problems of sexual recognition, low fecundity, and fertility, as well as the aggressive behavior, observed between couples from different populations (Ortiz-Domínguez et al., 2006a, 2006b). This genetic structure could be explained by isolation by distance, which restricts gene flow between them (Nolasco-Soto et al., 2017; Figure 8).
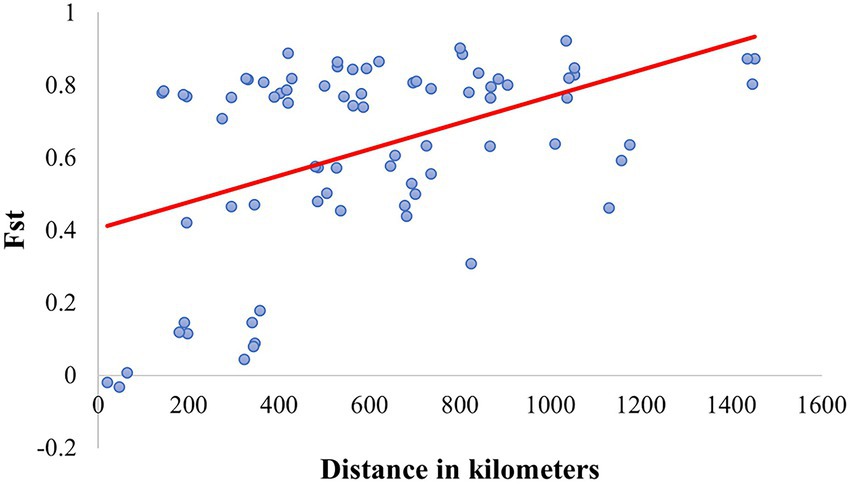
Figure 8. Linear regression between Fst and the geographic distance (R2 = 0.212, p < 0.0001) in the Mexican populations of Canthon cyanellus. This analysis was carried out based on the paired linear geographic distances between populations and the paired Fst values were obtained with DnaSP v6.12.03 (Rozas et al., 2017).
Similarly, in the species Canthon staigi Pereira, 1953 with wide distribution in the Atlantic forests in Brazil, Paraguay, and Argentina, the effect of habitat loss and fragmentation decreases the genetic diversity of its populations. However, they do not have a fragmented genetic structure due to a high gene flow between populations (Ferreira-Neto et al., 2017). This suggests that the current diversity and genetic structure of neotropical Scarabaeinae populations may be influenced by changes in the landscape caused by anthropic activities. Other factors that can lead to speciation are related to the size of the occupied area, the type of habitat, population demography, life history attributes of the species, as well as historical processes (Nolasco-Soto et al., 2017, Maldaner et al., 2019).
Microsatellite markers indicated that populations of Canthon staigi, which have been altered by anthropic activities, presented inbreeding depression and less allelic richness compared to populations in conserved areas (Cruz et al., 2017). Currently, 14 polymorphic microsatellites have been developed (2–16 alleles each) for the populations of C. cyanellus that belong to the different clades identified by Nolasco-Soto et al. (2017, 2020). These microsatellites contain private alleles that suggest their potential use to assess the genetic structure and more precise geographic delimitation of clades within C. cyanellus (Arce-Valdés et al., 2021).
4. Chromatic variation and cryptic species
In C. cyanellus the cuticular color variation does not match with the genetic structure of the populations or with the taxonomic division sensu Halffter (1961) (Nolasco-Soto et al., 2017, 2020; Figure 9). Populations with genetically differentiated metallic green individuals along the Pacific slope and the Gulf of Mexico reveal cryptic species in allopatry. The only genetically differentiated populations, which are distinguished by cuticular color, are in the region of Tuxpan and Papantla (i.e., very dark blue and green), and in Gómez-Farías (i.e., bright light green). In the state of Chiapas, there are populations with dark green individuals genetically differentiated from other populations in which individuals with polychromatic and monochromatic colors coexist (Nolasco-Soto et al., 2017, 2020). Solís and Kohlmann (2002) carried out a detailed study for C. cyanellus in various locations in Costa Rica, demonstrating the coexistence of several cuticular colors, being an example of a polytopic species, and suggesting genetic differences between populations. Based on that fact, Solís and Kohlmann (2002) do not recognize subspecies. The same coloration patterns as those described by Solís and Kohlmann (2002) can be found in beetles from Chiapas, Mexico. The same can be observed in Nicaragua and Honduras for C. c. sallei, but light and dark colors also occur in South American populations (Vaz-de-Mello and Cupello, 2018).
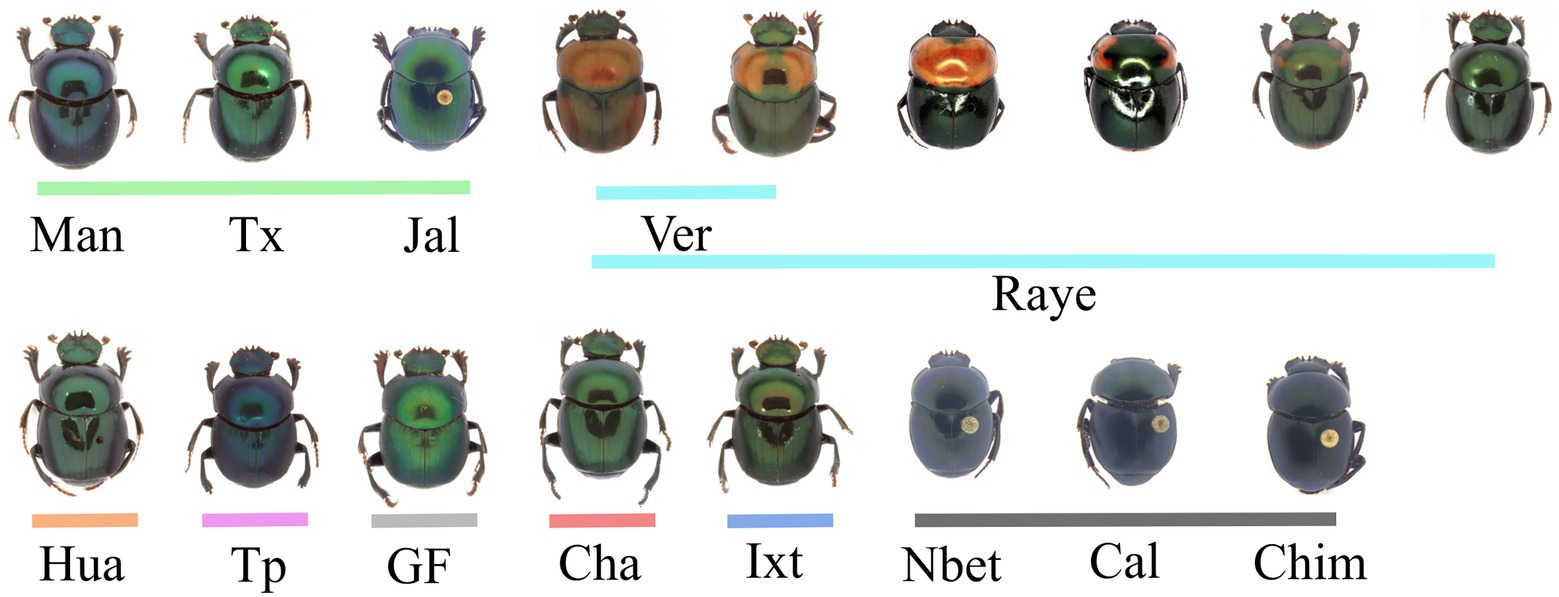
Figure 9. Morphological variation of color pattern in Canthon cyanellus populations. Colored lines correspond to the clade color shown in the Bayesian tree in Figure 3, (Nolasco-Soto et al., 2020). The genealogy was estimated from Bayesian inference (BI) analysis using the software MrBayes v.3.2.6 (Ronquist et al., 2012) available in the CIPRES portal (Miller et al., 2010).
Similarly, in the beetle Coprophanaeus encifer Germar, 1821, color variation is not related to cryptic speciation or genetic structure throughout its distribution in South America (Maldaner et al., 2019). On the other hand, Canthon rutilans different color morphs were assigned to the subspecies C. r. rutilans Castelnau, 1840 and C. r. cyanescens Harold, 1868; furthermore, recent studies found that these two subspecies diverge in thermal adaptations (Hensen et al., 2018), reproductive behavior (Hensen et al., 2020), and ecological niche (Hensen et al., 2021). This species indicates that the geographical variation of cuticular color in some species of Scarabaeinae might be related to cryptic speciation or species divergence, nonetheless the assignment of subspecies considering color as the only differentiation character (Solís and Kohlmann, 2002; Cupello et al., 2021).
In some species of Scarabaeinae, the color has been related to the period of activity (Hernández, 2002), thermoregulation (Amore et al., 2017; Alves et al., 2018; Cuesta and Lobo, 2019), phenotypic plasticity (Stanbrook et al., 2021), and Batesian mimicry (Alves et al., 2018). In the geotrupid beetle, Phelotrupes auratus local selection affects individuals of different colors that are regulated by multiple loci and correlated with barriers to gene flow (Araki and Sota, 2021). In C. cyanellus the metallic green color has a genetic basis (Favila et al., 2000), but it is possible that environmental factors also determine color variation throughout its distribution as has been suggested for other Scarabaeinae (Davis et al., 2008; Scholtz, 2009). Therefore, we do not rule out that stochastic processes such as genetic drift and genetic control by multiple loci may influence geographic color variation in this species.
5. Discussion
The evolutionary scenario that explains the diversification of C. cyanellus is associated with historical isolation by distance, which restricted the genetic flow between populations, causing their speciation in allopatry during the Pleistocene. The eight historically separate units recovered by Nolasco-Soto et al. (2020) can be identified by nucleotide synapomorphies. Traditional morphological taxonomy for this group was based on the middle peripheral sclerite character. However, based on a phylogenetic species concept, those eight units can be recognized as different species, in which pre-and postzygotic reproductive barriers are developing.
The observed geographic variation in the aedeagus morphology and the body color of C. cyanellus is not related to the inferred genetic structure of the populations nor the current taxonomic classification. The latitudinal distribution of color variation in Mexico tends toward monochromatic green. Although the adaptive significance of color variation is unclear, this may suggest some thermoregulatory function or selective pressure imposed by different local environmental conditions (Amore et al., 2017; Alves et al., 2018; Cuesta and Lobo, 2019). It is possible that different evolutionary mechanisms interact to produce geographic variation in body color in this group of beetles (Araki and Sota, 2021).
Studies carried out with microsatellite molecular markers, suggest that the diversity and current genetic structure of populations can be shaped by changes in the landscape caused by human activities (Cruz et al., 2017). This may occur due to habitat fragmentation, favoring in some cases the possibility of allopatric speciation due to geographic isolation and the effect of genetic drift. It is necessary to evaluate to what degree the current genetic structure of C. cyanellus populations might be shaped by the effects of fragmentation, extensive use of agriculture, and livestock management. This knowledge will make it possible to elucidate if some populations might be susceptible to ecological changes (e.g., Sierra Madre Oriental and in Tapachula, Chiapas).
The current review is part of an effort to understand the evolutionary history of Scarabaeinae beetles in the Mexican Transition Zone. To sort out the cryptic diversity present in this beetle complex represents an important step in understanding its evolutionary interrelationships. New lines of research must be opened to test hypotheses on the evolution of the genitalia, as well as to explore the importance of pre-and postzygotic reproductive barriers in this species.
6. Taxonomic status
Key to the determination of the subspecies of Canthon cyanellus (sensu Halffter, 1961).
1. Quadridentate clypeus. Hind tibiae markedly arched. The separation between proepisternae and proepimera only with an indication of a keel, limited to the internal part, close to the coxa. Teeth of the anterior tibiae, especially the two most apical ones, notably directed forwards, obliquely: the three tibial teeth are grouped in the most apical part of the piece C. cyanellus LeConte.
2. Dorsal surface green, blackish-green or dark blue. Pronotum with fine to evident and dense punctuation. With antescutelar impression. Hind tibiae markedly arched, forming an angle with the point of inflection toward the middle of the tibia. Texas and Mexico. C. cyanellus cyanellus LeConte.
Head and periphery of the pronotum dark green, the pronotum, and pygidium disc, except the latero-posterior margins, orange-reddish.
3. With antescutelar impression. Pronotum with very fine but dense punctuation. Dark metallic green elytra. Southwest Mexico C. cyanellus violetae n. subsp. Posterior edge of pronotum without antescutelar impression. Pronotum with slight punctuation, perceptible only on both sides and posterior margin. The anterior edge of the elytra, elytral suture line, and elytral epipleura are bright green, the elytral disc is orange-reddish; this coloring manifestly predominates. Central and South America C. cyanellus sallei Harold.
4. Pronotum with a dark triangular spot whose tip reaches the base of the elytra, lateral pronotum spots, separate or absent C. cyanellus sallei var. triangulatus Schmidt. Upper and lower surface dark green; pronotum with four light spots, one at each angle; pygidium usually dark C. cyanellus sallei var. gutticollis Schmidt.
On the other hand, Nunes (2019) carried out the most recent taxonomic review for the C. cyanellus group. Nunes (2019) assigned a new (but not yet named) subgenus to the group “cyanellus” based on the diagnostic character of the clypeal teeth of the anterior margin of the head. In addition, the morphology of the middle peripheral endophallic sclerite allowed him to separate and give preliminary validity to five of the chromatic forms that had been considered synonyms of C. cyanellus to a new species and a subspecies. Some of these chromatic forms have their correspondence with the clades (e.g., GF, NGM) inferred by Nolasco-Soto et al. (2017, 2020). Phylogeography, combined with traditional morphological taxonomy, provides information on species boundaries, populations, and other intraspecific groups; thus, a better understanding of the speciation processes (Table 2).
1. Canthon cyanellus LeConte, 1859 sensu stricto is the chromatic form used to describe the species. Nunes (2019) designed this as the subspecies, C. c. cyanellus. It corresponds to the clade NGM (Nolasco-Soto et al., 2017). A very dark blue with a rough dorsal surface characterizes it. It is distributed at the northern area of Veracruz in Tuxpan, Papantla, and Tancoco. This species may reach its distribution as far as Texas (Robinson, 1948). However, the genealogical relationship among the populations from Texas and Mexico is unknown.
2. Canthon speciosus Harold, 1868. This species was considered a synonym of C. cyanellus, and it was assigned to the subspecies C. c. cyanellus by Halffter (1961). Harold described it as having a bright green and bluish-green body color, with a smooth dorsal surface. This corresponds to the SGM clade sensu Nolasco-Soto et al. (2017), which includes populations from the southern area of Veracruz. Nunes (2019) assigned it as a synonym of C. spinosus and delimited its distribution in the eastern coastal zone of Mexico from Veracruz, Yucatan, Quintana Roo, Campeche, Chiapas to the border with Guatemala at the Petén area. Some specimens from Los Chimalapas in Oaxaca, all from Nueva Betania in Chiapas, and some from Calakmul in Campeche sequenced by Nolasco-Soto et al. (2020) were recovered within the SEM clade, therefore, C. speciosus would be a polyphyletic group. The SEM clade seems to correspond to the material reviewed by Harold referring to C. spinosus from southern Mexico. To resolve this problem material from the localities examined by Nunes should be sequenced, as well as a detailed review of the endophallic sclerites of the SEM clade individuals must be performed.
3. Canthon violetae Halffter, 1961. It is characterized by dark-green metallic elytra, as well as reddish-orange pronotum, and pygidium disc, except the lateral–posterior margins. The ventral part of the body is blackish-green and with some abdominal segments reddish-orange. It is endemic to the center and southwest area of the state of Chiapas. This is the SPS clade sensu Nolasco-Soto et al. (2017, 2020) that includes individuals from localities as El Vergel (also reviewed by Nunes, 2019), and Raymundo Enríquez, which are genetically differentiated from other populations in Chiapas. At the Raymundo Enríquez locality, there are individuals with completely green or reddish-orange cuticular colors (or a mixed pattern of these colors in different parts of the body; i.e., head, pronotum, abdomen, and pygidium). These color forms have been reported by Solís and Kohlmann (2002) in Costa Rica. This may suggest a possible hybridization zone or a species in which color variation is controlled by several codominant genes.
4. Canthon sallei Harold, 1863. Orange color at the cuticula, body, and elytra with black outline, may have a triangular-shaped spot in the center of the pronotum, sometimes it appears as a longitudinal line (Nunes, 2019). Nunes (2019) assigned Canthon dentiger Harold, 1968 as a synonym of C. sallei. This species is distributed from northwestern Colombia to southern Mexico and may occur in sympatry with C. triangulatus at its southern limit and with C. violetae at its northern limit (Nunes, 2019). The material reviewed by Nunes came from Nicaragua, Costa Rica, Colombia, and Panama. He also reviewed beetles from El Vergel (Chiapas, Mexico), which corresponds to the SPS clade sensu Nolasco-Soto et al. (2017). This clade contains large part of the color variation reported for the “cyanellus” group (e.g., metallic green, orange, and combinations of these colors in different parts of the body). Further molecular analyses of specimens from the localities reviewed by Nunes (2019) should be included, as well as a detailed review of the middle peripheral sclerite morphology must be carried out to determine if all the observed variation represents a polymorphic population or if this is a species complex.
5. Canthon triangulatus Schmidt, 1920. Synonyms: C. sallei guticollis Schmidt, 1920 and C. cyanellus havranekae Martínez, 1988 (Nunes, 2019). The species is distinguished by an opaque dorsal surface, pronotum, and elytra of orange color and black outline. On the pronotum, it has a longitudinal central spot. The pygidium is orange (Nunes, 2019). According to Nolasco-Soto et al. (2020), it corresponds to the Colombian clade. The phenotype corresponded to the one described for the variety that Schmidt called C. sallei triangulatus, which Halffter (1961) synonymized with C. cyanellus sallei. It is possible that populations from the Natagaima region in Tolima have their own identity and evolutionary history (Nolasco-Soto et al., 2020). These populations are enclosed between the central and eastern Andes Mountains in Colombia.
6. Canthon cyanellus (undescribed subspecies) Nunes & Vaz-de-Mello. It is characterized by a bright green color with a rough dorsal surface. According to Nunes (2019), it is an endemic subspecies in the region of “El Cielo” Biosphere Reserve in Tamaulipas (Mexico). This taxon corresponds to the GF clade sensu Nolasco-Soto et al. (2017).
7. Canthon (undescribed species) Nunes & Vaz-de-Mello. It has bright dark green color. This species corresponds to the clade Ixt located in Ixtapa, Zihuatanejo in the Pacific (Nolasco-Soto et al., 2020). However, it is easily confused with specimens recovered in the SGM, SEM, Hua, and ChaCal clades (Nolasco-Soto et al., 2020). Based on the material reviewed by Nunes (2019), which included specimens from Ixtapa, Zihuatanejo in Guerrero, El Aguacero, and El Vergel in Chiapas, the author concluded that this new species of Canthon might be in sympatry with C. violetae y C. sallei.
8. Other cryptic species. The localities of Huatulco in Oaxaca (Clade Hua) and Chamela Biosphere Reserve (Clade Cha) in Jalisco are monophyletic clades that may represent distinct and new species (Nolasco-Soto et al., 2017).
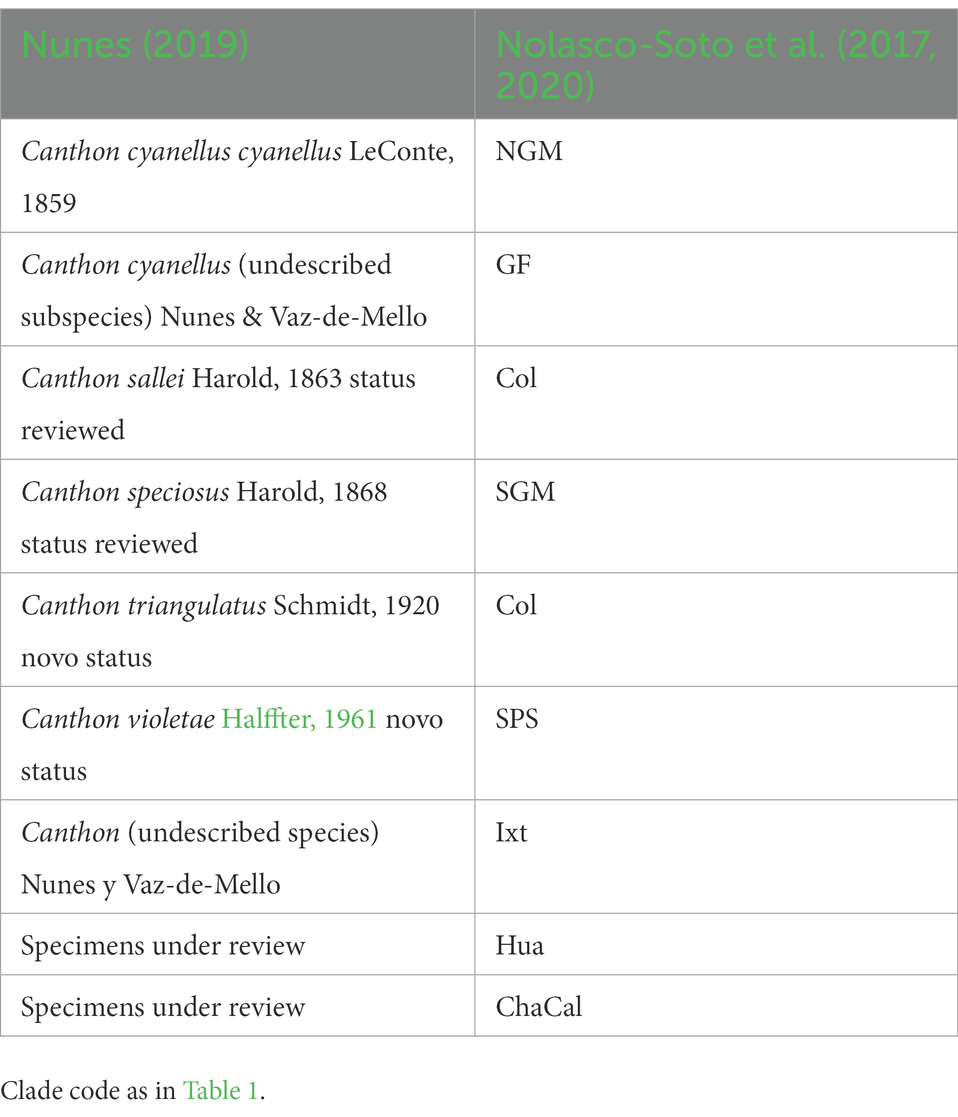
Table 2. Taxonomic division of Canthon cyanellus carried out by Nunes (2019) compared to the clades inferred by Nolasco-Soto et al. (2017, 2020)
Author contributions
All authors listed have made a substantial, direct, and intellectual contribution to the work and approved it for publication.
Acknowledgments
The authors would like to thank Dr. Jorge Valdez Carrasco for his support in the preparation and photographs of the endophallic sclerite de C. cyanellus. Figure 1 was a contribution by Claudio Mota Vargas.
Conflict of interest
The authors declare that the research was conducted in the absence of any commercial or financial relationships that could be construed as a potential conflict of interest.
Publisher’s note
All claims expressed in this article are solely those of the authors and do not necessarily represent those of their affiliated organizations, or those of the publisher, the editors and the reviewers. Any product that may be evaluated in this article, or claim that may be made by its manufacturer, is not guaranteed or endorsed by the publisher.
References
Alves, V. M., Hernández, M. I. M., and Lobo, J. M. (2018). Elytra absorb ultraviolet radiation but transmit infrared radiation in Neotropical Canthon species (Coleoptera, Scarabaeinae). Photochem. Photobiol. 94, 532–539. doi: 10.1111/php.12889
Amore, V., Hernández, M. I. V., Carrascal, L. M., and Lobo, J. M. (2017). Exoskeleton may influence the internal body temperatures of Neotropical dung beetles (Col. Scarabaeinae). PeerJ 5:e3349. doi: 10.7717/peerj.3349
Antonio-Hernández, E., García-Ramírez, M. J., and Fong-Lara, D. (2018). New records of the genus Anastrepha Schiner, 1868 (Diptera: Tephritidae) in the isthmus of Tehuantepec, Oaxaca, México. Biocyt. Biol. Cienc. y Tecnol. 11, 824–833. doi: 10.22201/fesi.20072082.2018.11.67517
Araki, Y., and Sota, T. (2021). Population genetic structure underlying the geographic variation in beetle structural colour with multiple transition zones. Mol. Ecol. 30, 670–684. doi: 10.1111/mec.15758
Arce-Valdés, L. R., Sánchez-Guillén, R. A., Nolasco-Soto, J., and Favila, M. E. (2021). Next-generation sequencing, isolation and characterization of 14 microsatellite loci of Canthon cyanellus (Coleoptera: Scarabaeidae). Mol. Biol. Rep. 48, 7433–7441. doi: 10.1007/s11033-021-06761-8
Arellano, L., León-Cortés, J., and Ovaskainen, O. (2008). Patterns of abundance and movement in relation to landscape structure: a study of a common scarab (Canthon cyanellus cyanellus) in Southern Mexico. Landsc. Ecol. 23, 69–78. doi: 10.1007/s10980-007-9165-8
Arnaud, P. (2002). Phanaeini. Dendropaemon, Tetramereia, Homalotarsus, Megatharsis, Diabroctis, Coprophanaeus, Oxysternon, Phanaeus, Sulcophanaeus. England: Hillside Books.
Bánki, O., Roskov, Y., Döring, M., Ower, G., Vandepitte, L., Hobern, D., et al. (2022). Catalogue of Life Checklist (Version 2022-01-14).
Bellés, X., and Favila, M. (1983). Protection chimique du nid chez Canthon cyanellus cyanellus LeConte [Col. Scarabaeidae]. Bull. Soc. Entomol. Fr. 88, 602–607. doi: 10.3406/bsef.1983.18090
Blackwelder, R. E. (1944). Checklist of the coleopterous insects of Mexico, Central America, the West Indies and South America. Bull. U. S. Natl. Mus. 185, 189–341. doi: 10.5479/si.03629236.185.2
Bouchard, P., Bousquet, Y., Davies, A. E., Alonso-Zarazaga, M. A., Lawrence, J. F., Lyal, C. H. C., et al. (2011). Family-group names in Coleoptera (Insecta). ZooKeys 88, 1–972. doi: 10.3897/zookeys.88.807
Chamorro-Florescano, I. A., and Favila, M. E. (2008). Male reproductive status affects contest outcome during nidification in Canthon cyanellus cyanellus LeConte (Coleoptera: Scarabaeidae). Behaviour 145, 1811–1821. doi: 10.1163/156853908786279637
Chamorro-Florescano, I. A., and Favila, M. E. (2009). The reproductive status of both sexes affects the frequency of mating and the reproductive success of males in the ball roller beetle Canthon cyanellus cyanellus (Coleoptera: Scarabaeidae). Behaviour 146, 1499–1512. doi: 10.1163/156853909X445560
Chamorro-Florescano, I. A., and Favila, M. E. (2016). Male success in intrasexual contests extends to the level of sperm competition in a species of dung roller beetle. Ethology 122, 53–60. doi: 10.1111/eth.12443
Chamorro-Florescano, I. A., Favila, M. E., and Macías-Ordóñez, R. (2011). Ownership, size and reproductive status affect the outcome of food ball contests in a dung roller beetle: when do enemies share? Evol. Ecol. 25, 277–289. doi: 10.1007/s10682-010-9428-8
Chamorro-Florescano, I. A., Favila, M. E., and Macías-Ordóñez, R. (2017). Contests over reproductive resources in female roller beetles: outcome predictors and sharing as an option. PLoS One 12:e0182931. doi: 10.1371/journal.pone.0182931
Chung, H., and Carroll, S. B. (2015). Wax, sex and the origin of species: dual roles of insect cuticular hydrocarbons in adaptation and mating. Bio Essays 37, 822–830. doi: 10.1002/bies.201500014
Cortez, V., and Favila, M. E. (2007). Actividad antifúngica del ácido 4-metoxi fenilácetico producido en las glándulas esternales de machos del escarabajo rodador Canthon cyanellus cyanellus (Coleoptera: Scarabaeidae). Entomol. Mex. 6, 355–359.
Cortez, V., Favila, M. E., Verdú, J. R., Ortiz, A. J., and Trigos, R. (2012). Behavioral and antennal electrophysiological responses of a predator ant to the pygidial gland secretions of two species of Neotropical dung roller beetles. Chemoecology 22, 29–38. doi: 10.1007/s00049-011-0095-1
Cortez, V., Verdú, J. R., Ortiz, A. J., Trigos, R., and Favila, M. E. (2015). Chemical diversity and potential biological functions of the pygidial gland secretions in two species of Neotropical dung roller beetles. Chemoecology 25, 201–213. doi: 10.1007/s00049-015-0189-2
Coyne, J. A., and Orr, H. A. (1998). The evolutionary genetics of speciation. Philos. Trans. R. Soc. Lond. B 353, 287–305. doi: 10.1098/rstb.1998.0210
Cruz, G. A. S., Ferreira-Neto, C. A., Lira-Neto, A. C., and Moura, R. C. (2017). Isolation and characterization of microsatellite markers in Canthon (Peltecanthon) staigi (Coleoptera: Scarabaeidae) and cross-amplification in related species. Int. J. Agric. Sc. Food Technol. 3, 32–35. doi: 10.17352/2455-815X.000019
Cuesta, E., and Lobo, J. M. (2019). Visible and near-infrared radiation may be transmitted or absorbed differently by beetle elytra according to habitat preference. PeerJ 7:e8104. doi: 10.7717/peerj.8104
Cupello, M., Ribeiro-Costa, C. S., and Vaz-de-Mello, F. Z. (2021). The evolution of Bolbites onitoides (Coleoptera: Scarabaeidae: Phanaeini): its phylogenetic significance, geographical polychromatism and the subspecies problem. Zool. J. Linnean Soc. 194, 973–1034. doi: 10.1093/ZOOLINNEAN/ZLAB015
Cupello, M., and Vaz-de-Mello, F. Z. (2018). A monographic revision of the Neotropical dung beetle genus Sylvicanthon Halffter & Martínez, 1977 (Coleoptera: Scarabaeidae: Scarabaeinae: Deltochilini), including a reappraisal of the taxonomic history of ‘Canthon sensu lato’. Eur. J. Taxon. 467, 1–205. doi: 10.5852/ejt.2018.467
Davis, A. L. V., Brink, D. J., Sholtz, C. H., Prinsloo, L. C., and Deschodt, C. M. (2008). Functional implications of temperature-correlated color polymorphism in an iridescent, scarabaeine dung beetle. Ecol. Entomol. 33, 771–779. doi: 10.1111/j.1365-2311.2008.01033.x
Davis, A. L. V., Scholtz, C. H., and Philips, T. K. (2002). Historical biogeography of scarabaeine dung beetles. J. Biogeogr. 29, 1217–1256. doi: 10.1046/j.1365-2699.2002.00776.x
de Queiroz, K. (2007). Species concepts and species delimitation. Syst. Biol. 56, 879–886. doi: 10.1080/10635150701701083
Duennes, M. A., Lozier, J. D., Hines, H. M., and Cameron, S. A. (2012). Geographical patterns of genetic divergence in the widespread Mesoamerican bumble bee Bombus ephippiatus (Hymenoptera: Apidae). Mol. Phylog. Evol. 64, 219–231. doi: 10.1016/j.ympev.2012.03.018
Duennes, M. A., Petranek, C., Diez, P., de Bonilla, E., Mérida-Rivas, J., Martínez-López, O., et al. (2017). Population genetics and geometric morphometrics of the Bombus ephippiatus species complex with implications for its use as a commercial pollinator. Conserv. Genet. 18, 553–572. doi: 10.1007/s10592-016-0903-9
Endler, J. A. (1977). Geographic Variation, Speciation, and Clines. Princeton, NJ: Princeton University Press.
Favila, M. E. (1993). Some ecological factors affecting the life-style of Canthon cyanellus cyanellus (Coleoptera: Scarabaeidae): an experimental approach. Ethol. Ecol. Evol. 5, 319–328. doi: 10.1080/08927014.1993.9523019
Favila, M. E. (2001). Historia de vida y comportamiento de un escarabajo necrófago: Canthon cyanellus cyanellus LeConte (Coleoptera: Scarabaeinae). Folia Entomol. Mex. 40, 245–278.
Favila, M. E., and Díaz, A. (1996). Canthon cyanellus cyanellus LeConte (Coleoptera: Scarabaeidae) makes a nest in the field with several brood balls. The Coleopt. Bull. 50, 52–60.
Favila, M. E., Nolasco, J., Chamorro Florescano, I., and Equihua, M. (2005). Sperm competition and evidence of sperm fertilization patterns in the carrion ball-roller beetle Canthon cyanellus cyanellus LeConte (Scarabaeidae: Scarabaeinae). Behav. Ecol. Sociobiol. 59, 38–43. doi: 10.1007/s00265-005-0006-y
Favila, M. E., Ortiz-Domínguez, M., Chamorro-Florescano, I., and Cortez-Gallardo, V. (2012). ““Comunicación química y comportamiento reproductor de los escarabajos rodadores de estiércol (Scarabaeinae: Scarabaeini)”: aspectos ecológicos y evolutivos, y sus posibles causas” in Temas Selectos en Ecología Química de Insectos. eds. J. C. Rojas and E. A. Malo (México: El Colegio de la Frontera Sur), 141–164.
Favila, M. E., Ruiz-Lizarraga, G., and Nolasco, J. (2000). Inheritance of a red cuticular color mutation in the scarab beetle Canthon cyanellus cyanellus LeConte (Coleoptera: Scarabaeidae). Coleop. Bull. 54, 541–545. doi: 10.1649/0010-065X(2000)054[0541:IOARCC]2.0.CO;2
Ferrari, L., Tagami, T., Eguchi, M., Orozco-Esquivel, M. T., Petrone, C. M., Jacobo-Albarrán, J., et al. (2005). Geology, geochronology and tectonic setting of late Cenozoic volcanism along the Southwestern Gulf of Mexico: the Eastern Alkaline Province revisited. J. Vol. Geothe. Res. 146, 284–306. doi: 10.1016/j.jvolgeores.2005.02.004
Ferreira-Neto, C. A., Cruz, G. A. S., Costa de Amorim, I., Queiroz Balbino, V., and Moura, R. C. (2017). Effects of fragmentation and anthropic pressure on the genetic structure of Canthon (Peltecanthon) staigi (Coleoptera: Scarabaeidae) populations in the Atlantic Forest domain. J. Insect Conserv. 21, 267–276. doi: 10.1007/s10841-017-9980-4
Global Biodiversity Information Facility. (2020). GBIF.org Occurrence Download. Available at: https://doi.org/10.15468/dl.mj7ese (Accessed August 12, 2020).
Grimaldi, D., James, A. C., and Jaenike, J. (1992). Systematics and modes of reproductive isolation in the Holarctic Drosophila testacea species group (Diptera: Drosophilidae). Ann. Entomol. Soc. Am. 85, 671–685. doi: 10.1093/aesa/85.6.671
Gunter, N. L., Monteith, G. B., Cameron, S. L., and Weir, T. A. (2018). Evidence from Australian mesic zone dung beetles supports their Gondwanan origin and Mesozoic diversification of the Scarabaeinae. Insect Syst. Evol. 50, 162–188. doi: 10.1163/1876312X-00002171
Gunter, N. L., Weir, T. A., Slipinksi, A., Bocak, L., and Cameron, S. L. (2016). If dung beetles Scarabaeidae: Scarabaeinae arose in association with dinosaurs, did they also suffer a mass co-extinction at the KPg boundary? PLoS One 11:e0153570. doi: 10.1371/journal.pone.0153570
Halffter, G. (1961). Monografía de las especies norteamericanas del género Canthon Hoffsg. (Coleop., Scarab.). Ciencia 20, 225–320.
Halffter, G. (1962). Explicación preliminar de la distribución geográfica de los Scarabaeidae mexicanos. Acta Zool. Mex. 5, 1–17.
Halffter, G. (1964). La entomofauna americana, ideas acerca de su origen y distribución. Folia Entomol. Mex. 6, 1–108.
Halffter, G. (2017). La Zona de Transición Mexicana y la megadiversidad de México: del marco histórico a la riqueza actual. Dugesiana 24, 77–89.
Halffter, G., Espinosa de los, M. A., Nolasco-Soto, J., Arriaga-Jiménez, A., and Rivera-Gasperín, S. (2022). Bajacanthon, a new subgenus for the Mexican Deltochilini (Coleoptera: Scarabaeidae: Scarabaeinae) Fauna. Diversity 14:109. doi: 10.3390/d14020109
Halffter, G., Halffter, V., and Huerta, C. (1983). Comportement sexual et nidification chez Canthon cyanellus cyanellus LeConte. Bull. Soc. Entomol. Mex. 88, 585–594. doi: 10.3406/bsef.1983.18093
Halffter, G., and Martínez, A. (1977). Revisión monográfica de los Canthonina Americanos IV clave para géneros y subgéneros. Folia Entomol. Mex. 38, 29–107.
Halffter, G., and Morrone, J. J. (2017). An analytical review of Halffter's Mexican transition zone, and its relevance for evolutionary biogeography, ecology and biogeographical regionalization. Zootaxa 4226, 1–46. doi: 10.11646/zootaxa.4226.1.1
Hensen, M. C., Hernández, M. I. M., da Silva, P. G., Amore, V., and Lobo, J. M. (2018). Distribution of Canthon rutilans rutilans and Canthon rutilans cyanescens along spatio-temporal and temperature gradients. Insects 9:124. doi: 10.3390/insects9040124
Hensen, M. C., Hernández, M. I. M., and Lobo, J. M. (2021). Predicted distributions could suggest regional sympatry in spite of local allopatry: the case of the dung beetle Canthon rutilans. Entomol. Exp. Appl. 168, 827–835. doi: 10.1111/ens.12462
Hensen, M. C., Lobo, J. M., and Hernández, M. I. M. (2020). Differences in the reproductive behaviour and larval development of two Canthon rutilans subspecies reinforce their thermal regional segregation. Entomol. Exp. Appl. 168, 827–835. doi: 10.1111/eea.12984
Hernández, M. I. M. (2002). The night and day of dung beetles (Coleoptera, Scarabaeidae) in the Serra do Japi, Brazil: elytra colour related to daily activity. Rev. Bras. Entomol. 46, 597–600. doi: 10.1590/s0085-56262002000400015
Hernández-Martínez, G., and Martínez, M. I. (2003). Desarrollo larval de Canthon cyanellus cyanellus LeConte 1859 (Coleoptera: Scarabaeidae). Acta Zool. Mex. 89, 185–200. doi: 10.21829/azm.2003.89891783
Hewitt, G. M. (1996). Some genetic consequences of the ice ages, and their role in divergence and speciation. Biol. J. Linn. Soc. 58, 247–276. doi: 10.1111/j.1095-8312.1996.tb01434.x
House, C. M., and Simmons, L. W. (2003). Genital morphology and fertilization success in the dung beetle Onthophagus taurus: an example of sexually selected male genitalia. Proc. R. Soc. Lond. B 270, 447–455. doi: 10.1098/rspb.2002.2266
Howden, H. F. (1966). Notes on Canthonini of the “Biologia Centrali-Americana” and descriptions of new species (Coleoptera: Scarabaeidae). Can. Entomol. 98, 725–741. doi: 10.4039/Ent98725-7
Irwin, D. E. (2002). Phylogeographic breaks without geographic barriers to gene flow. Evolution 56, 2383–2394. doi: 10.1111/j.0014-3820.2002.tb00164.x
Ishikawa, R. (1987). On the function of the copulatory organs of Ohomopterus (Coleoptera, Carabidae, Genus Carabus). Entomol. Soc. Jap. 55, 202–206.
Kamimura, Y., and Mitsumoto, H. (2012). Lock-and-key structural isolation between sibling Drosophila species. Entomol. Sci. 15, 197–201. doi: 10.1111/j.1479-8298.2011.00490.x
Kohlmann, B., and Halffter, G. (1990). Reconstruction of a specific example of insect invasion waves: the cladistic analysis of Canthon (Coleoptera: Scarabaeidae) and related genera in north America. Quaest. Entomol. 26, 1–20.
Kubota, K., and Sota, T. (1998). Hybridization and speciation in the carabid beetles of the subgenus Ohomopterus (Coleoptera, Carabidae, Genus Carabus). Res. Popul. Ecol. 40, 213–222. doi: 10.1007/BF02763406
Liu, Y., Dietrich, C. H., and Wei, C. (2019). Genetic divergence, population differentiation and phylogeography of the cicada Subpsaltria yangi based on molecular and acoustic data: an example of the early stage of speciation. BMC Evol. Biol. 19:5. doi: 10.1186/s12862-018-1317-8
Maldaner, M. E., Vaz-de-Mello, F. Z., Takiya, D. M., and Ferreira, D. C. (2019). Genetic and chromatic variation of Coprophanaeus (Megaphanaeus) ensifer (Germar, 1821) (Coleoptera: Scarabaeidae). Zool. Anz. 283, 150–160. doi: 10.1016/j.jcz.2019.09.001
Martínez, M. I. (2005). Structure of the terminal ampulla in male larvae of Canthon cyanellus LeConte (Coleoptera: Scarabaeidae: Scarabaeinae). Coleop. Bull. 59, 35–39. doi: 10.1649/681
Medina, C. A., Scholtz, C. H., and Gill, B. D. (2003). Morphological variation and systematics of Canthon Hoffmansegg 1817, and related genera of new world Canthonini dung beetles (Coleoptera, Scarabaeinae). Mitt. Mus. Natkd. Berl. Dtsch. Entomol. Z. 50, 23–68. doi: 10.1002/mmnd.4810500105
Mello, A. A. C., Costa Amorim, I., Freitas da Silva, A., Rocha de Medeiros, G., Wallau, G. L., and Moura, R. C. (2021). Mitogenome of Coprophanaeus ensifer and phylogenetic analysis of the Scarabaeidae family (Coleoptera). Genet. Mol. Biol. 44:e20200417. doi: 10.1590/1678-4685-GMB-2020-0417
Miller, M., Pfeiffer, W., and Schwartz, T. (2010). Creating the CIPRES science gateway for inference of large phylogenetic trees. Gateway Computing Environments Workshop 2010, 1–8. doi: 10.1109/gce.2010.5676129
Moctezuma, V., Halffter, G., and Lizardo, V. (2021). The Phanaeus tridens species group (Coleoptera: Scarabaeoidea): a dung beetle group with genital morphological stasis but a changing ecological niche. Acta Entomol. Mus. Natl. Pragae. 61, 447–482. doi: 10.37520/aemnp.2021.025
Monaghan, M. T., Inward, D. J. G., Hunt, T., and Vogler, A. P. (2007). A molecular phylogenetic analysis of the Scarabaeinae (dung beetles). Mol. Phylogenet. Evol. 45, 674–692. doi: 10.1016/j.ympev.2007.06.009
Morrone, J. J. (2015). Halffter’s Mexican transition zone (1962–2014), cenocrons and evolutionary biogeography. J. Zool. Syst. Evol. Res. 53, 249–257. doi: 10.1111/jzs.12098
Morrone, J. J. (2020). The Mexican Transition Zone: A Natural Biogeographic Laboratory to Study Biotic Assembly. Switzerland: Springer.
Mullen, S. P., and Shaw, K. L. (2014). Insect speciation rules: unifying concepts in speciation research. Annu. Rev. Entomol. 59, 339–361. doi: 10.1146/annurev-ento-120710-100621
Nichols, E., Spector, S., Louzada, J., Larsen, T., Amezquita, S., and Favila, M. E. (2008). The Scarabaeinae research network ecological functions and ecosystem services provided by Scarabaeinae dung beetles. Biol. Conserv. 141, 1461–1474. doi: 10.1016/j.biocon.2008.04.011
Nolasco-Soto, J., Favila, M. E., Espinosa De Los, M. A., González-Astorga, J., Halffter, G., Valdez-Carrasco, J., et al. (2020). Variations in genetic structure and male genitalia suggest recent lineage diversification in the Neotropical dung beetle complex Canthon cyanellus (Scarabaeidae: Scarabaeinae). Biol. J. Linn. Soc. 131, 505–520. doi: 10.1093/biolinnean/blaa131
Nolasco-Soto, J., González-Astorga, J., Espinosa De Los, M. A., Galante-Patiño, E., and Favila, M. E. (2017). Phylogeographic structure of Canthon cyanellus (Coleoptera: Scarabaeidae), a Neotropical dung beetle in the Mexican transition zone: insights on its origin and the impacts of Pleistocene climatic fluctuations on population dynamics. Mol. Phylogenet. Evol. 109, 180–190. doi: 10.1016/j.ympev.2017.01.004
Nunes, L. G. O. A. (2019). Revisão taxonômica e definição de alguns grupos de espécies do gênero Canthon Hoffmannsegg (Coleoptera: Scarabaeidae: Scarabaeinae: Deltochilini). Doctoral Thesis. Brazil: Universidade Federal de Pernambuco.
Nunes, L. G. D. O. A., Nunes, R. V., and Vaz-de-Mello, F. Z. (2020). Taxonomic revision of the South American subgenus Canthon (Peltecanthon) Pereira, 1953 (Coleoptera: Scarabaeidae: Scarabaeinae: Deltochilini). Eur. J. Taxon. 594, 1–27. doi: 10.5852/ejt.2020.594
Ornelas, J. F., Sosa, V., Soltis, D. E., Daza, J. M., González, C., Soltis, P. S., et al. (2013). Comparative phylogeographic analyzes illustrate the complex evolutionary history of threatened cloud forests of northern Mesoamerica. PLoS One 8:e53283. doi: 10.1371/journal.pone.0056283
Ortiz-Domínguez, M., Favila, M. E., González, D., and Zuñiga, D. (2010). Genetic differences in populations of the ball roller scarab Canthon cyanellus cyanellus (Coleoptera: Scarabaeidae): a preliminary analysis. Elytron 24, 99–106.
Ortiz-Domínguez, M., Favila, M. E., and Mendoza-López, M. R. (2006a). Mate recognition differences among allopatric populations of the Scarab Canthon cyanellus cyanellus (Coleoptera: Scarabaeidae). Ann. Entomol. Soc. Am. 99, 1248–1256. doi: 10.1603/0013-8746(2006)99[1248:MRDAAP]2.0.CO;2
Ortiz-Domínguez, M., Favila, M. E., Mendoza-López, M. R., García-Barradas, O., and Cruz-Sánchez, J. S. (2006b). Epicuticular compounds and sexual recognition in the ball roller scarab, Canthon cyanellus cyanellus. Entomol. Exp. Appl. 119, 23–27. doi: 10.1111/j.1570-7458.2006.00388.x
Pardo-Diaz, C., Lopera Toro, A., Peña Tovar, S. A., Sarmiento-Garcés, R., Sánchez Herrera, M., and Salazar, C. (2019). Taxonomic reassessment of the genus Dichotomius (Coleoptera: Scarabaeinae) through integrative taxonomy. PeerJ 7:e7332. doi: 10.7717/peerj.7332
Robinson, M. (1948). A review of the species of Canthon inhabiting the United States (Scarabaeidae: Coleoptera). Trans. Am. Entomol. Soc. 74, 83–100.
Ronquist, F., Teslenko, M., van der Mark, P., Ayres, D. L., Darling, A., Höhna, S., et al. (2012). MrBayes 3.2: efficient Bayesian phylogenetic inference and model choice across a large model space. Syst. Biol. 61, 539–542. doi: 10.1093/sysbio/sys029
Rozas, J., Ferrer-Mata, A., Sánchez-DelBarrio, J. C., Guirao-Rico, S., Librado, P., Ramos-Onsins, S. E., et al. (2017). DnaSP 6: DNA sequence polymorphism analysis of large datasets. Mol. Biol. Evol. 34, 3299–3302. doi: 10.1093/molbev/msx248
Salomão, P. R., González-Tokman, D., Dáttilo, W., López-Acosta, J. C., and Favila, M. E. (2018). Landscape structure and composition define the body condition of dung beetles (Coleoptera: Scarabaeinae) in a fragmented tropical rainforest. Ecol. Indic. 88, 144–151. doi: 10.1016/j.ecolind.2018.01.033
Sánchez-Guillén, R. A., Córdoba-Aguilar, A., Cordero-Rivera, A., and Wellenreuther, M. (2014). Rapid evolution of prezygotic barriers in non-territorial damselflies. Biol. J. Linn. Soc. 113, 485–496. doi: 10.1111/jeb.12274
Sánchez-Guillén, R. A., Wellenreuther, M., and Cordero-Rivera, A. (2012). Strong asymmetry in the relative strengths of prezygotic and postzygotic barriers between two damselfly sister species. Evolution 66, 690–707. doi: 10.1111/j.1558-5646.2011.01469.x
Scholtz, C. H. (2009). “Environmental influence on the development of color” in Evolutionary biology and conservation of dung beetles. eds. C. H. Scholtz, A. L. V. Davis, and U. Kryger (Sofia-Moscow: Pensoft Pub), 147–154.
Smith, A. G. (2006). A review of the family-group names for the superfamily Scarabaeoidea with corrections to nomenclature and a current classification. Coleopt. Bull. 60, 144–204. doi: 10.1649/0010-065X(2006)60[144:AROTFN]2.0.CO;2
Solís, A., and Kohlmann, B. (2012). Checklist and distribution atlas of the Scarabaeinae (Coleoptera: Scarabaeidae) of Costa Rica. Zootaxa 3482, 1–32.
Stanbrook, R. A., Harris, W. E., Wheater, C. P., and Jones, M. (2021). Evidence of phenotypic plasticity along an altitudinal gradient in the dung beetle Onthophagus proteus. PeerJ 9:e10798. doi: 10.7717/peerj.10798
Tarasov, S., and Dimitrov, D. (2016). Multigene phylogenetic analysis redefines dung beetles relationships and classification (Coleoptera: Scarabaeidae: Scarabaeinae). BMC Evol. Biol. 16, 257–219. doi: 10.1186/s12862-016-0822-x
Tarasov, S., and Génier, F. (2015). Innovative Bayesian and parsimony phylogeny of dung beetles (Coleoptera, Scarabaeidae, Scarabaeinae) enhanced by ontology-based partitioning of morphological characters. PLoS One 10:e0116671. doi: 10.1371/journal.pone.0116671
Torres-Morales, L. (2019). Límites de distribución actual de Sturnira hondurensis. Rev. Mex. Biodivers 90:e902644. doi: 10.22201/ib.20078706e.2019.90.2644
Tregenza, T., Pritchard, V. L., and Butlin, R. K. (2000). Patterns of trait divergence between populations of the meadow grasshopper, Chorthippus parallelus. Evolution 54, 574–585.
Vaz-de-Mello, F. Z., and Cupello, M. (2018). The type specimens of South American dung beetles. Part I: On the species described in the genus Canthon Hoffmannsegg, 1817 by the German entomologist Adolf Schmidt (1856-1923) (Coleoptera, Scarabaeidae, Scarabaeinae). Spixiana 41, 33–76.
Vaz-de-Mello, F., Nunes, L. G. D. O. A., and Costa-Silva, V. (2020). A new species of the genus Canthon Hoffmannsegg (Coleoptera, Scarabaeidae, Scarabaeinae, Deltochilini) from Central Brazil. Pap. Avulsos Zool. 60:e202060. doi: 10.11606/1807-0205/2020.60.special-issue.04
Vieira, M. K., Vaz-de-Mello, F. Z., and Silva, F. A. (2019). A taxonomic revision of the Canthon subgenus Pseudepilissus Martínez, 1954 (Coleoptera: Scarabaeidae: Scarabaeinae). Insect Syst. Evol. 51, 696–752. doi: 10.1163/1876312X-00001023
Keywords: aedeagus, allopatric populations, color polymorphism, genetic structure, incipient speciation, Mexican Transition Zone, reproductive barriers, taxonomic status
Citation: Nolasco-Soto J, González-Astorga J, Espinosa de los Monteros A and Favila ME (2023) Evolutionary history and diversity in the ball roller beetle Canthon cyanellus. Front. Ecol. Evol. 10:1066439. doi: 10.3389/fevo.2022.1066439
Edited by:
Diego Baldo, CONICET Institute of Subtropical Biology (IBS), ArgentinaReviewed by:
Bjarte Henry Jordal, University of Bergen, NorwayMartín Alejandro Montes, Federal Rural University of Pernambuco, Brazil
Copyright © 2023 Nolasco-Soto, González-Astorga, Espinosa de los Monteros and Favila. This is an open-access article distributed under the terms of the Creative Commons Attribution License (CC BY). The use, distribution or reproduction in other forums is permitted, provided the original author(s) and the copyright owner(s) are credited and that the original publication in this journal is cited, in accordance with accepted academic practice. No use, distribution or reproduction is permitted which does not comply with these terms.
*Correspondence: Jorge González-Astorga, ✉ jorge.gonzalez@inecol.mx