- 1Biology Department, Trent University, Peterborough, ON, Canada
- 2Department of Biological Sciences, University of Manitoba, Winnipeg, MB, Canada
Ecological studies investigating niche breadth and overlap often have limited spatial and temporal scale, preventing generalizations across varying environments and communities. For example, it is not clear whether species having restricted diets maintain such patterns relative to closely related species and across their geographic range of co-occurrence. We used stable isotope analysis of hair and fur samples collected from four regions of sympatry for Canada lynx (Lynx canadensis) and bobcat (Lynx rufus) spanning southern Canada and the northern United States, to test the prediction that the more generalist species (bobcat) exhibits a wider dietary niche than the more specialist species (Canada lynx) and that this pattern is consistent across different regions. We further predicted that Canada lynx diet would consistently exhibit greater overlap with that of bobcat compared to overlap of bobcat diet with Canada lynx. We found that Canada lynx had a narrower dietary niche than bobcat, with a high probability of overlap (85–95%) with bobcat, whereas the bobcat dietary niche had up to a 50% probability of overlap with Canada lynx. These patterns of dietary niche breadth and overlap were consistent across geographic regions despite some regional variation in diet breadth and position, for both species. Such consistent patterns could reflect a lack of plasticity in species dietary niches. Given the increasingly recognized importance of understanding dietary niche breadth and overlap across large spatial scales, further research is needed to investigate the mechanisms by which broad-scale patterns are maintained across species and systems.
Introduction
Variation in dietary niche breadth has been associated with various aspects of species distributions, including range size and expansion (Lanszki et al., 2022), heterogeneity of spatial distributions (Alberdi et al., 2020) and environments occupied (Walsh and Tucker, 2020; Fargallo et al., 2022), and responses to landscape change (Kellner et al., 2019). This diversity of outcomes highlights that many factors likely contribute to shaping dietary niche breadth. It also emphasizes the relevance of broad-scale patterns in dietary niche breadth to understanding community and ecosystem dynamics, predicting species responses to global change, and addressing biodiversity declines. Given the various factors that can influence dietary niche breadth and overlap, regional variation could affect broad-scale patterns of niche breadth within a species and overlap between species. However, these uncertainties have rarely been investigated across broad spatial scales, especially among species that occupy large geographic ranges and occur at low densities across the landscape.
Niche breadth is influenced by the availability of preferred resources, which may be impacted by community interactions, and proclivity to exploit alternate resources (Mac Arthur and Levins, 1964; Rozenweig, 1991; McGill et al., 2006). Foraging theory predicts that, owing to their higher proficiency to acquire preferred resources, species with restricted food preferences will have narrow dietary niches that overlap with those of sympatric species having more generalized feeding patterns (Stephens and Krebs, 1986). Alternatively, species with broad food preferences and that consume alternate food types when preferred resources are scarce will be reflected by wider dietary niches (Stephens and Krebs, 1986). However, local conditions or circumstances such as the frequency with which resources, environments, or communities fluctuate spatially and/or temporally can alter foraging strategies (Mac Arthur and Levins, 1964; Devictor et al., 2010). Individual specialization further contributes to the population-level diet breadth and variation observed across different spatial and temporal scales (Bolnick et al., 2003; Devictor et al., 2010; Costa-Pereira et al., 2019). Given the challenges of collecting data on trophic interactions across large scales in the field, the prediction that patterns of dietary niche position, breadth, and overlap are maintained across geographic ranges has rarely been tested. It follows that stable isotope analysis offers a novel and informative means for assessing this prediction and potentially overcoming challenges associated with conducting expansive research.
We assessed the dietary niche of two closely related carnivores, known to consume similar prey, across multiple regions spanning a broad geographic scale. Canada lynx (Lynx canadensis) and bobcat (Lynx rufus) are typically classified as “specialist” and “generalist” consumers, respectively (Anderson and Lovallo, 2003), although these classifications are recognized as being over-simplifications when considered across temporal or spatial scales e.g., (see Roth et al., 2007; Burstahler et al., 2016). Canada lynx primarily occupy the North American boreal forest, Rocky Mountains, and northeast United States, whereas bobcat occur in southern Canada, throughout the contiguous United States and extend into Mexico (Anderson and Lovallo, 2003). The two species thus occur in sympatry only across the Canada-United States border and in the Rocky Mountains (Peers et al., 2013). Increased abundance of bobcats as well as their recent range expansion (Lavoie et al., 2009; Roberts and Crimmins, 2010), and reductions in lynx abundance and range retraction (Koen et al., 2014b; Marrotte and Bowman, 2021) could also be related to dietary niche variation for one or both species. We evaluated dietary niche position, breadth and overlap for Canada lynx and bobcat using stable isotope analysis of hair and fur samples collected from multiple regions spanning their sympatric range. We predicted that: (1) dietary niche position of both species would vary regionally (e.g., due to regional differences in prey availability and/or isotopic signatures); (2) Canada lynx dietary niche would consistently be narrower than that of bobcat; and (3) dietary niche of Canada lynx would be more similar to that of bobcat (i.e., have a higher probability of overlap) than vice versa.
Materials and methods
Sample collection and isotopic analysis
Canada lynx and bobcat hair and fur samples were collected between 2009 and 2012 from the North American Fur Auctions (Toronto, ON) and Fur Harvester Auction Inc. (North Bay, ON). We analyzed samples available for areas of sympatry between Canada lynx and bobcat in four regions: (1) southeastern British Columbia (Canada lynx, n = 28; bobcat, n = 54), (2) west of Lake Superior in Ontario and Minnesota (Canada lynx, n = 63; bobcat, n = 19), (3) the interlake region of Sault Ste. Marie, Ontario and Michigan (Canada lynx, n = 18; bobcat, n = 8), and (4) southern Québec along the St. Lawrence river (Canada lynx, n = 32; bobcat, n = 23), hereafter referred to as British Columbia, western Ontario, central Ontario, and Québec, respectively. Geolocations for harvested pelts represent the centroid of the trapline or management unit from which each animal was harvested (Row et al., 2014; Figure 1). Note that despite these regions of sympatry, there is limited evidence that the two species hybridize (Koen et al., 2014a).
We measured stable isotope ratios (δ13C and δ15N) of fur samples to assess niche position, breadth and overlap for the two species. Canada lynx and bobcat share similar hair growth patterns, moulting once in the spring (April–May) and once in the autumn (October–November; Quinn and Parker, 1987; Anderson and Lovallo, 2003). Canada lynx and bobcat fur samples were taken from the hind leg of pelts from winter-harvested animals and therefore, stable isotope measurements reflect the autumn diet for both species. All fur samples were washed with soap and water, rinsed thoroughly, and then oven-dried at 60°C for 48 h. Samples were homogenized into a fine powder using a ball mill, wrapped in tin capsules, and sent to the Chemical Tracers Laboratory, Windsor, ON, Canada for δ13C and δ15N measurement using a continuous-flow isotope ratio mass spectrometer.
Data analysis
Based on previous studies that found little annual variation in the diets of Canada lynx in the southern part of their range (Szumski et al., in review), we assumed limited temporal variation in Canada lynx and bobcat diets during the 4 years of our study and therefore pooled observations within each region across years. Note that isotopic signatures did not differ qualitatively according to year in which samples were taken for region (Supplementary Figure 1). To further confirm our assumption, we fit a multivariate generalized linear mixed effects model using the MCMCglmm package in R (Hadfield, 2010) with species and region as fixed effects and random intercepts for year. The random year effect was almost zero (posterior mean = 0.0017, lower 95% CI = 1.6e-17, upper 95% CI = 0.0026) and explained <1% of the total variation in δ13C and δ15N ratios, confirming the negligible effect of year.
We compared niche breadth and overlap of lynx and bobcat within each region using the R packages “SIBER” (Jackson et al., 2011) and “nicheROVER” (Lysy et al., 2015; Swanson et al., 2015). The isotopic niche region is defined by each niche axis (δ13C and δ15N) and treated as a trait probability density function that can be compared against other groups (Carmona et al., 2016). Uncertainty is incorporated into niche region estimates using a Bayesian framework, whereby the 95% highest density interval for each niche axis is used as a continuous distribution from which to sample iteratively. We estimated niche regions based on the mean and variance of δ13C and δ15N for each species using an uninformative, normal Inverse Wishart prior (α = 95%). We ran two chains of 10,000 iterations, burning the first 1,000 draws and thinning every 10 draws.
The breadth of resource use along both diet axes (δ13C and δ15N) can be summarized by standard ellipse area (SEA) in SIBER, and further broken down into each niche axis for analysis of niche position and overlap in nicheROVER. Posterior distributions represent the full range of feasible parameter values, given observed data and prior probabilities (which we kept vague to minimize the influence on the posterior). Thus, probabilistic difference in niche position and variance between species is inferred from overlap of posterior distributions. As probability density functions integrate to 1, density of posterior solutions is directly proportional to relative proportion of that trait value in the population (Carmona et al., 2016) and thus may be used as a proxy for exploitation proficiency of the population (Devictor et al., 2010). Niche overlap is then calculated as the probability that the isotopic ratio of a random individual from one species would occur within the 95% highest density niche region of the competitor species.
Multivariate normality is assumed for both SIBER and nicheROVER, which we verified graphically using qq-plots of Mahalanobis distances (Legendre and Legendre, 2012) for each species and region. Three bobcat samples were identified as statistical outliers that were excluded from analysis: one from British Columbia with very low δ15N (−0.32‰), one from British Columbia with very high δ13C (−17.5‰), and one from Québec that was enriched in both 13C and 15N (δ13C = −18.5‰, δ15N = 9.14‰). Given that these stable isotope ratios are nonetheless consistent with the scope of values reported for North American mammals (Roth et al., 2007), we repeated the analysis with these outliers included and found that the results (Supplementary Figures 2–5) remained consistent with those presented here.
Results
As expected, niche position of each species varied regionally (Figures 2, 3). Mean δ13C of Canada lynx varied by up to 0.66‰ across regions, and less than that of bobcat, which varied by up to 1.90‰. Alternatively, mean δ15N of bobcat varied by up to 1.09‰, and less than that of Canada lynx, which varied by up to 1.83‰. The relative niche position of Canada lynx and bobcat also differed along one niche axis (δ13C or δ15N) across all study regions (Figures 2, 3). In all regions except British Columbia, the mean δ15N for bobcat was 0.7–0.9‰ higher than that for lynx, but mean δ13C values were similar for both species. In British Columbia, mean δ13C for bobcat was 1.7‰ higher than that for lynx and mean δ15N was similar for both species. Variance in δ13C and δ15N were generally higher for bobcat than lynx, reflecting the presumed broader range of prey consumed by bobcat, except for δ13C in central Ontario and δ15N in western Ontario, where estimates for both species were similar. Covariances of δ13C and δ15N were generally positive, except for lynx in central Ontario that was negative, suggesting that isotopic ratios of δ13C and δ15N tend to vary together.
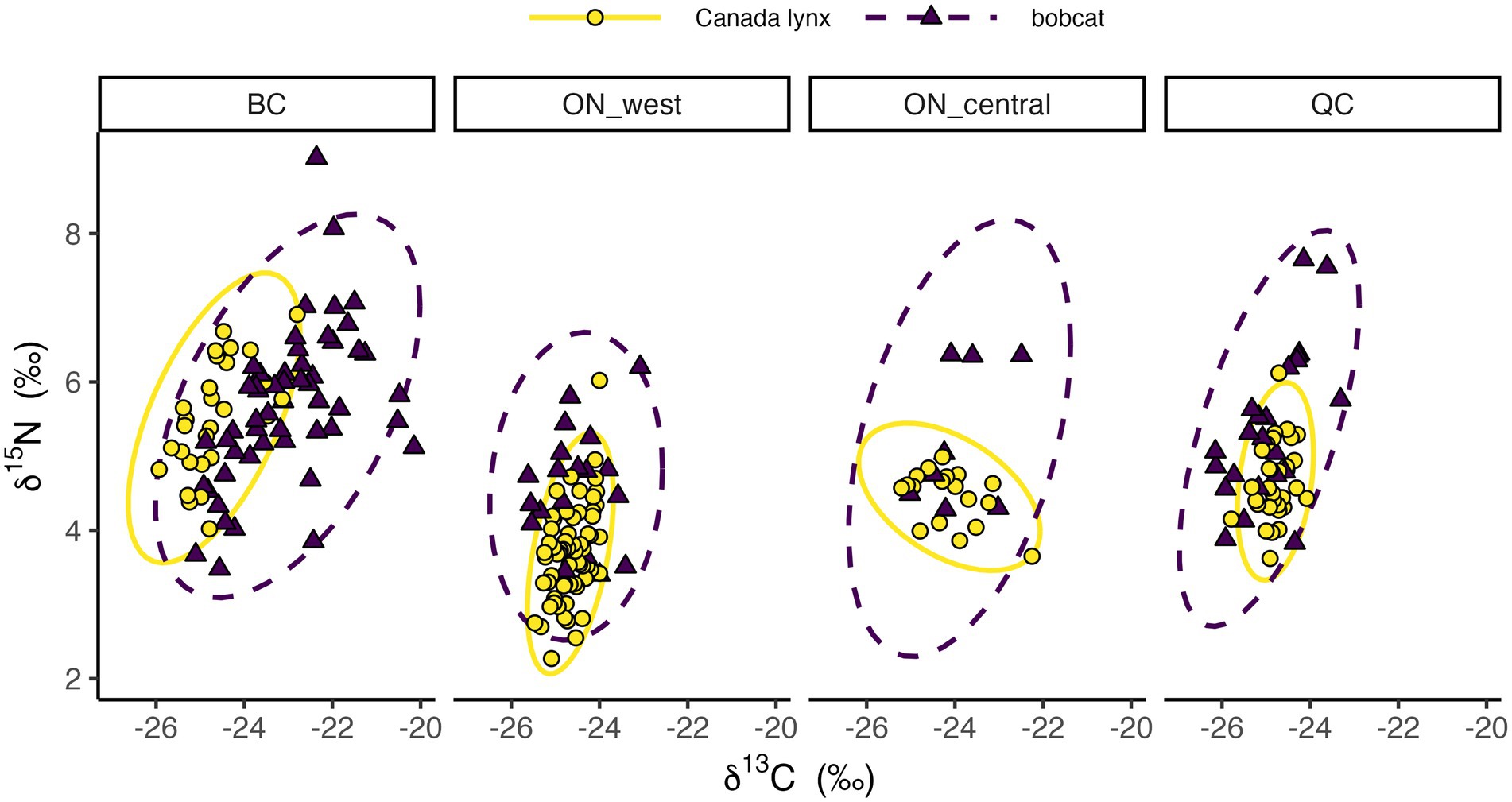
Figure 2. Stable isotope ratios of Canada lynx and bobcat in each study region: British Columbia (BC), western Ontario (ON_west), central Ontario (ON_central), and Québec (QC). Ellipses depict 95% confidence levels.
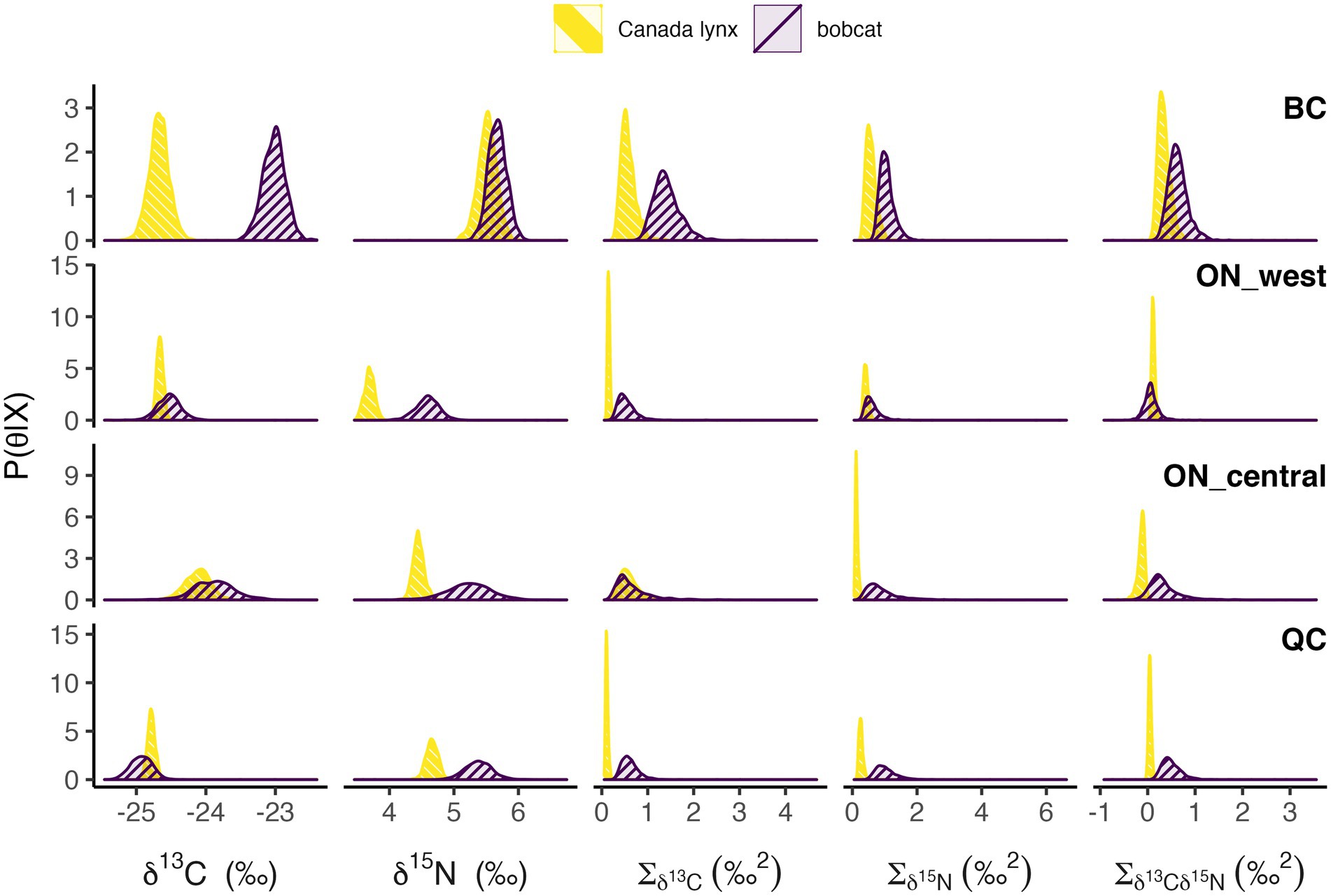
Figure 3. Posterior distributions of mean and variance/covariance (Σ) of δ13C and δ15N stable isotope ratios for Canada lynx and bobcat in each study region: British Columbia (BC), western Ontario (ON_west), central Ontario (ON_central), and Québec (QC).
Stable isotope ratios revealed greater variation in bobcat diet, reflecting consumption of a greater diversity of prey compared to Canada lynx. This observation was consistent across all four regions, supporting the prediction that the dietary niche of lynx would consistently be narrower than that of bobcat (Figures 2, 4). Mean estimates of isotopic niche breadth (measured as the standard ellipse area) for bobcat ranged between 1.8–3.3‰2 and were consistently higher than that of Canada lynx (0.5–1.5‰2) by a factor of 2.3 (British Columbia) to 3.8 (Québec; Figure 4).
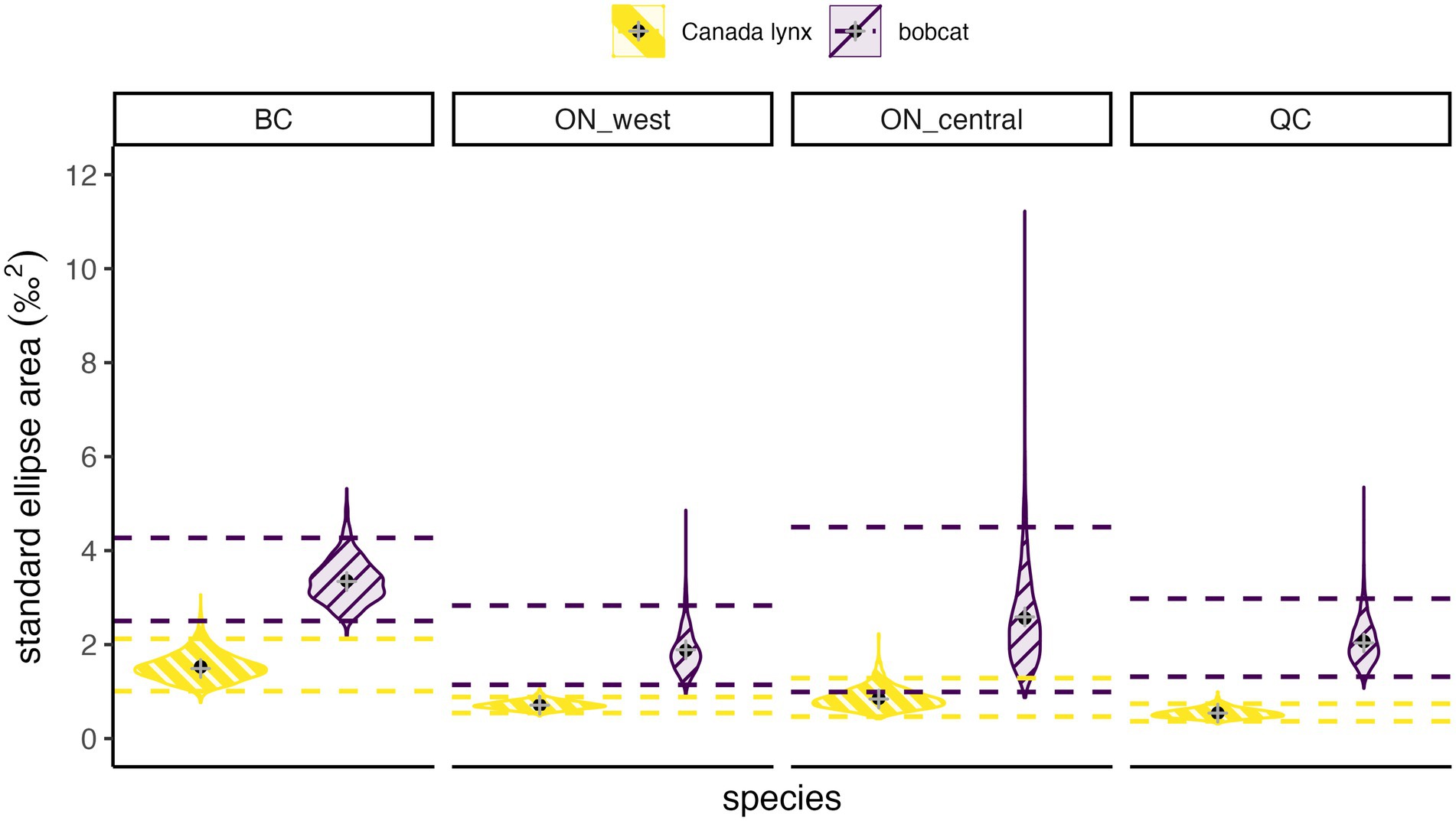
Figure 4. Posterior distributions of dietary niche breadth estimates measured as standard ellipse area for lynx and bobcat in each study region: British Columbia (BC), western Ontario (ON_west), central Ontario (ON_central), and Québec (QC). Black points show the mean, gray plus signs show the sample-corrected standard ellipse area, and dashed lines show 95% credible intervals of the posterior distribution.
Our findings further support the prediction that dietary niche of Canada lynx would exhibit a higher probability of overlap with that of bobcat than vice versa (Figure 5). The probabilities of a lynx’s isotopic ratios occurring within the dietary niche region of bobcat were consistently skewed toward 100% (mean estimates ranging between 85% and 95% across study regions), indicating the likelihood of complete niche overlap of lynx with sympatric bobcat populations. In contrast, the probability of an individual bobcat’s diet overlapping with dietary niche of the sympatric lynx population was considerably lower in all regions (mean estimates of 35–50%; Figure 5). Credible intervals for estimates of mean diet overlap between species were broader for central and western Ontario, likely due to smaller sample sizes (n = 19 bobcat in western Ontario; n = 8 bobcat and n = 18 Canada lynx in central Ontario). Nonetheless, the remarkable consistency in these species’ dietary niche breadth and overlap across regions suggests that general patterns of diet breadth and differentiation operate at large spatial scales.
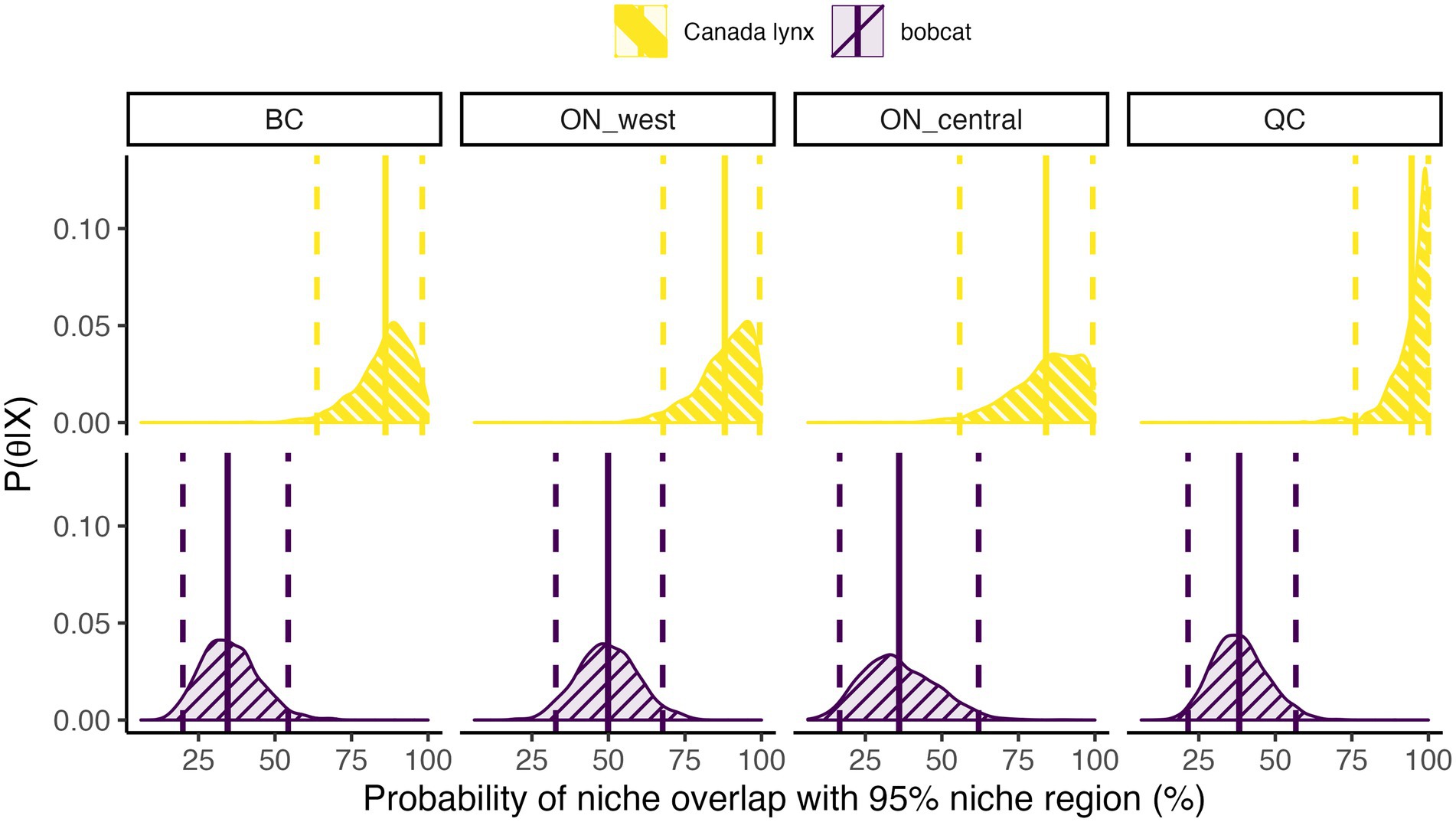
Figure 5. Posterior distributions of the probability that an individual of one species falls within the 95% niche region of the competitor species in each study region: British Columbia (BC), western Ontario (ON_west), central Ontario (ON_central), and Québec (QC). Vertical lines show the mean (solid) and 95% credible intervals (dashed) of the posterior distribution.
Discussion
Our results revealed consistent patterns in dietary niche breadth and overlap of two sympatric carnivores, across a broad spatial scale. The dietary niche of Canada lynx was narrower and had a high probability of overlapping with the niche region of bobcat, whose dietary niche was broader and had a lower probability of overlapping with that of Canada lynx, across all four regions. Consistency in these patterns occurred despite regional variation in the niche position and breadth of each carnivore, providing compelling evidence that patterns in niche breadth and overlap are maintained across broad geographic scales.
Previous investigations into broad geographic patterns in dietary niches and their drivers have mainly focused on interspecific variation (e.g., Papacostas and Freestone, 2016; Gainsbury and Meiri, 2017; Granot and Belmaker, 2019), whereas studies investigating intraspecific variation have largely focused on individual specialization and niche characteristics in the context of environmental variation occurring at more localized scales within a single region or population (e.g., Woo et al., 2008; Abbas et al., 2011; Navarro-López and Fargallo, 2015). Therefore, the current study is unique in that we investigate intraspecific variation in niche position and breadth across a broad spatial scale, and show that despite regional variation, interspecific patterns in niche breadth and overlap appear to be maintained for two closely related species. As predicted by the ecological theory, the niche region of the species with more restricted feeding (Canada lynx) consistently exhibited a narrower dietary niche with a high probability of overlap with the more generalist consumer (bobcat), whereas the broader niche region of bobcat exhibited much lower probability of overlap with Canada lynx. Although we did not infer the specific drivers of this consistency, observed patterns could arise from interspecific variation in dietary preferences or competitive interactions. Previous studies examining spatial and temporal variation in niche breadth of Canada lynx found that it appears to vary with the dynamics of its primary prey (snowshoe hare) both over time (Burstahler et al., 2016) and spatially along a latitudinal gradient (Roth et al., 2007; Szumski et al., in review), suggesting that prey availability might be key in determining feeding strategy. If this is the case for both bobcat and Canada lynx, we might expect regional variation in niche breadth to be positively correlated for the two species. While we observed this trend overall (Supplementary Figure 6), we lack sufficient data to formally test this prediction. Yurkowski et al. (2016) is one of the few other examples of studies having characterized dietary niches across a broad spatial scale. That study detected lower population-level niche breadth of both ringed seals and beluga whales at higher latitudes, presumably due to lower prey diversity in the high Arctic (Yurkowski et al., 2016). They further revealed dietary generalization in beluga whales and individual specialization in ringed seal that was higher in populations with wider niche breadth (Yurkowski et al., 2016), implying that latitudinal variation in population-level niche breadth is driven by different foraging strategies at the individual level for each species. The above findings highlight the need for further research investigating intraspecific variation in niche breadth over broad geographic scales, its role in population-level and interspecific patterns, and the underlying driving mechanisms. Such efforts will be especially important to better understand the implications of observed broad-scale changes in species distributions and biodiversity loss.
Many factors could influence spatial variation in niche breadth. Niche breadth is predicted to vary with prey diversity and availability (Yurkowski et al., 2016; Carvalho and Davoren, 2020). Likewise, predator densities can also influence feeding strategies via intraspecific interactions (Svanbäck and Bolnick, 2007). Environments that are either extreme or stable could promote dietary restriction due to lower ecological opportunity or local adaptation of narrow ecological niches (Jocque et al., 2010; Bonetti and Wiens, 2014; Rivas-Salvador et al., 2019). Ecological specialization is also widely predicted to arise in environments with greater species richness owing to more opportunity for community-level interactions (Kartzinel et al., 2015; Granot and Belmaker, 2019). Mechanisms underlying patterns in niche breadth and overlap we observed could be important for understanding range expansion and retraction of bobcat and Canada lynx, respectively (Koen et al., 2014; Marrotte and Bowman, 2021). A recent study suggested that trophic niches based on resource use (i.e., Eltonian niche) may be more relevant to the distribution of species at fine spatial scales, while habitat niches based on climate or environmental conditions (i.e., Grinnellian niche) may be more important across large geographic scales (Stevens, 2022). However, due to challenges associated with collecting necessary field data, few studies have characterized trophic niches across broad spatial scales thus limiting their incorporation into species distribution models (Austin, 2002; Araújo and Guisan, 2006). Peers et al. (2013) investigated the Grinnellian niche of Canada lynx and bobcat, concluding that bobcat may displace Canada lynx when both species overlap. However, they also found that bobcat habitat niche breadth expanded in regions of sympatry with lynx (Peers et al., 2013), suggesting that the former species might also be displaced by Canada lynx. We do not know whether these habitat and space use dynamics translate to our observations of Canada lynx and bobcat dietary breadth and overlap. It is notable that Grinnellian and Eltonian niches are not independent of each other, as co-occurring species have access to similar resources. However, the mechanisms influencing breadth of one type of niche does not imply that the same mechanisms act on the other. Further investigation into spatial variation in niche breadth and the relative importance of environmental conditions and resource use in species distributions will therefore be valuable, especially for those species undergoing range shifts, expansions, and contractions, like Canada lynx and bobcat. Stable isotope analysis of fur or other samples provides an efficient and robust method for addressing these questions, compared to more traditional approaches involving intensive field research.
When trying to assess dietary niches using stable isotopes, spatial and temporal variation in isotopic ratios of both consumers and their food sources therefore need be considered, as isotopic signatures are expected to vary with carbon sources, assimilation, as well as community trophic structure (Farquhar et al., 1989; Layman et al., 2012). Other studies have measured isotope ratio variation across regions for both snowshoe hare and red squirrel (Tamiasciurus hudsonicus), the primary prey for lynx (Roth et al., 2007; Merkle et al., 2017) and bobcat (Newbury and Hodges, 2018) in our study regions. Red squirrels (and other rodents) are typically enriched in both δ13C and δ15N compared to snowshoe hares (Roth et al., 2007; Merkle et al., 2017; Szumski et al., submitted). The mean values of δ13C and δ15N of Canada lynx in our study are consistent with values reflecting high consumption of snowshoe hares, whereas mean δ13C or δ15N of bobcat were consistently elevated. Our findings are also consistent with previous studies showing general patterns of prey consumption for both species (e.g., O’Donoghue et al., 2001; Witczuk et al., 2015; Ivan and Shenk, 2016; Newbury and Hodges, 2018). Consequently, it is likely that Canada lynx in our study consumed a greater proportion of snowshoe hares, whereas the broader dietary niche of bobcat reflects a greater reliance on different prey types such as red squirrels and other rodents.
Although dietary niches may be related to isotopic niches, they are not the same. Variation in isotopic niches arises not only from changes in diet but also from variation in isotopic baselines and diet-tissue discrimination factors (Wolf et al., 2009; Hette-Tronquart, 2019). For example, food availability was shown to influence individual isotopic signatures through differences in diet-consumer isotope fractionation associated with variation in growth rates (Gorokhova, 2018). Various stress factors (e.g., malnutrition, parasitism, exposure to toxic substances) were also found to influence isotopic signatures, which could also be misinterpreted as variation in dietary niches (Karlson et al., 2018). Isotope ratios like those used in our study reflect a mean value of an individual’s diet and, consequently, measures of dietary niches (i.e., position, breadth, and overlap) reflect between-individual variation rather than within-individual variation (Bearhop et al., 2004; Jackson et al., 2011). Within-individual variation in dietary niches can be measured by sampling various tissues from the same individual that vary in the time with which dietary signatures are integrated (Bearhop et al., 2004) or by performing stable isotope analysis on serially sectioned tissues (Rogers et al., 2020). Demographic factors such as age/size structure and sex ratios are also expected to influence intra- and interpopulation variation in isotopic signatures (Bolnick et al., 2003; Burstahler et al., 2016). In one lynx population, age classes responded differently to changes in hare density, with diet breadth of yearlings increasing at low hare densities, while adults and dependent juveniles maintained a constant diet through the initial decline in hare density (Burstahler et al., 2016). Although these data were not available in the current study, future studies investigating mechanisms underlying patterns in niche breadth and overlap would therefore benefit from ancillary data collected at the level of individuals (e.g., age, sex, health status) and stable isotope analyses that permit estimation of both between- and within-individual variation to assess the role of individual specialization across broad geographic scales.
To conclude, we recognize that organisms can adjust foraging strategies in response to a range of ecological conditions and constraints (e.g., Díaz-Ruiz et al., 2013, Manenti et al., 2013, Karanth et al., 2017). Despite this, Canada lynx and bobcat exhibit a remarkably consistent pattern of niche breadth and overlap across regions spanning a broad spatial scale, for which the underlying mechanisms remain unknown. However, this finding provides evidence that could suggest a lack of plasticity in species’ diet features, implying that many community-level trophic interactions and ecosystem dynamics may be shaped largely by evolutionary processes that operate at the scale of the species rather than the population. Connecting mechanisms driving niche breadth and overlap at fine scales to those resulting in maintenance of consistent patterns across broad spatial scales will be important for furthering our understanding of factors influencing distribution of species and biodiversity loss, as well as incorporating trophic interactions into models aimed at predicting responses to climate change, range shifts, species invasions, habitat loss and fragmentation, and other sources of environmental variation.
Data availability statement
The datasets presented in this study can be found in online repositories. The names of the repository/repositories and accession number(s) can be found at: https://github.com/JenileeGobin/lynx-bobcat-diet.
Author contributions
CS, JR, and DM conceived the study. CS processed samples and sent them for analysis, performed preliminary data analysis, and wrote an initial draft of the manuscript. JG conducted the data analyses presented in the current manuscript and supplementary materials, generated figures, and wrote the final draft of the manuscript with input from CS, JR, and DM. All authors contributed to the article and approved the submitted version.
Funding
Funding was provided by a Strategic NSERC grant awarded to DM and co-authors.
Acknowledgments
We would like to thank the North American Fur Auctions Inc. who made this work possible.
Conflict of interest
The authors declare that the research was conducted in the absence of any commercial or financial relationships that could be construed as a potential conflict of interest.
Publisher’s note
All claims expressed in this article are solely those of the authors and do not necessarily represent those of their affiliated organizations, or those of the publisher, the editors and the reviewers. Any product that may be evaluated in this article, or claim that may be made by its manufacturer, is not guaranteed or endorsed by the publisher.
Supplementary material
The Supplementary material for this article can be found online at: https://www.frontiersin.org/articles/10.3389/fevo.2022.1059155/full#supplementary-material
References
Abbas, F., Morellet, N., Hewison, A. J. M., Merlet, J., Cargnelutti, B., Lourtet, B., et al. (2011). Landscape fragmentation generates spatial variation of diet composition and quality in a generalist herbivore. Oecologia 167, 401–411. doi: 10.1007/s00442-011-1994-0
Alberdi, A., Razgour, O., Aizpurua, O., Novella-Fernandez, R., Aihartza, J., Budinski, I., et al. (2020). DNA metabarcoding and spatial modelling link diet diversification with distribution homogeneity in European bats. Nat. Commun. 11, 1154–1162. doi: 10.1038/s41467-020-14961-2
Anderson, E. M., and Lovallo, M. J. (2003). “Bobcat and lynx,” in Wild mammals of North America. eds. G. A. Feldhamer and B. Thompson (Baltimore, MD: Johns Hopkins University Press), 758–786.
Araújo, M. B., and Guisan, A. (2006). Five (or so) challenges for species distribution modelling. J. Biogeogr. 33, 1677–1688. doi: 10.1111/j.1365-2699.2006.01584.x
Austin, M. P. (2002). Spatial prediction of species distribution: an interface between ecological theory and statistical modelling. Ecol. Model. 157, 101–118. doi: 10.1016/S0304-3800(02)00205-3
Bearhop, S., Adams, C. E., Waldron, S., Fuller, R. A., and Macleod, H. (2004). Determining trophic niche width: a novel approach using stable isotope analysis. J. Anim. Ecol. 73, 1007–1012. doi: 10.1111/j.0021-8790.2004.00861.x
Bolnick, D. I., Svanbäck, R., Fordyce, J. A., Yang, L. H., Davis, J. M., Hulsey, C. D., et al. (2003). The ecology of individuals: incidence and implications of individual specialization. Am. Nat. 161, 1–28. doi: 10.1086/343878
Bonetti, M. F., and Wiens, J. J. (2014). Evolution of climatic niche specialization: a phylogenetic analysis in amphibians. Proc. R. Soc. B 281:20133229. doi: 10.1098/rspb.2013.3229
Burstahler, C. M., Roth, J. D., Gau, R. J., and Murray, D. L. (2016). Demographic differences in diet breadth of Canada lynx during a fluctuation in prey availability. Ecol. Evol. 6, 6366–6375. doi: 10.1002/ece3.2115
Carmona, C. P., de Bello, F., Mason, N. W. H., and Leps, J. (2016). Traits without borders: integrating functional diversity across scales. Trends Ecol. Evol. 31, 382–394. doi: 10.1016/j.tree.2016.02.003
Carvalho, P. C., and Davoren, G. K. (2020). Niche dynamics of sympatric non-breeding shearwaters under varying prey availability. IBIS 162, 701–712. doi: 10.1111/ibi.12783
Costa-Pereira, R., Araújo, M. S., Souza, F. L., and Ingram, T. (2019). Competition and resource breadth shape niche variation and overlap in multiple trophic dimensions. Proc. R. Soc. B 286:20190369. doi: 10.1098/rspb.2019.0369
Devictor, V., Clavel, J., Julliard, R., Lavergne, S., Mouillot, D., Thuiller, W., et al. (2010). Defining and measuring ecological specialization. J. Appl. Ecol. 47, 15–25. doi: 10.1111/j.1365-2664.2009.01744.x
Díaz-Ruiz, F., Delibes-Mateos, M., García-Moreno, J. L., López-Martín, J. M., Ferreira, C., and Ferreras, P. (2013). Biogeographical patterns in the diet of an opportunistic predator: the red fox Vulpes vulpesin the Iberian Peninsula. Mammal Rev. 43, 59–70. doi: 10.1111/j.1365-2907.2011.00206.x
Fargallo, J. A., Navarro-López, J., Cantalapiedra, J. L., Pelegrin, J. S., and Fernández, M. H. (2022). Trophic niche breadth of Falconidae species predicts biomic specialization but not range size. Biology 11, 522–537. doi: 10.3390/biology11040522
Farquhar, G. D., Ehleringer, J. R., and Hubick, K. T. (1989). Carbon isotope discrimination and photosynthesis. Annu. Rev. Plant Physiol. Plant Mol. Biol. 40, 503–537. doi: 10.1146/annurev.pp.40.060189.002443
Gainsbury, A., and Meiri, S. (2017). The latitudinal diversity gradient and interspecific competition: no global relationship between lizard dietary niche breadth and species richness. Glob. Ecol. Biogeogr. 26, 536–572. doi: 10.1111/geb.12560
Gorokhova, E. (2018). Individual growth as a non-dietary determinant of the isotopic niche metrics. Methods Ecol. Evol. 9, 269–277. doi: 10.1111/2041-210X.12887
Granot, I., and Belmaker, J. (2019). Niche breadth and species richness: correlation strength, scale and mechanisms. Glob. Ecol. Biogeogr. 29, 159–170. doi: 10.1111/geb.13011
Hadfield, J. D. (2010). MCMC methods for multiresponse generalised linear mixed models: the MCMCglmm R package. J. Stat. Softw. 33, 1–22. doi: 10.18637/jss.v033.i02
Hette-Tronquart, N. (2019). Isotopic niche is not equal to trophic niche. Ecol. Lett. 22, 1987–1989. doi: 10.1111/ele.13218
Ivan, J. S., and Shenk, T. M. (2016). Winter diet and hunting success of Canada lynx in Colorado. J. Wildl. Manag. 80, 1049–1058. doi: 10.1002/jwmg.21101
Jackson, A. L., Inger, R., Parnell, A. C., and Bearhop, S. (2011). Comparing isotopic niche widths among and within communities: SIBER-Stable Isotope Bayesian Ellipses in R. J. Anim. Ecol. 80, 595–602. doi: 10.1111/j.1365-2656.2011.01806.x
Jocque, M., Field, R., Brendonck, L., and De Meester, L. (2010). Climatic control of dispersal-ecological specialization trade-offs: a metacommunity process at the heart of the latitudinal diversity gradient? Glob. Ecol. Biogeogr. 19, 244–252. doi: 10.1111/j.1466-8238.2009.00510.x
Karanth, K. U., Srivathsa, A., Vasudev, D., Puri, M., Parameshwaran, R., and Kumar, S. (2017). Spatio-temporal interactions facilitate large carnivore sympatry across a resource gradient. Proc. R. Soc. B 284:20161860. doi: 10.1098/rspb.2016.1860
Karlson, A. M., Reutgard, M., Garbaras, A., and Gorokhova, E. (2018). Isotopic niche reflects stress-induced variability in physiological status. R. Soc. Open Sci. 5:171398. doi: 10.1098/rsos.171398
Kartzinel, T. R., Chen, P. A., Coverdale, T. C., Erickson, D. L., Kress, W. J., Kuzmina, M. L., et al. (2015). DNA metabarcoding illuminates dietary niche partitioning by African large herbivores. Proc. Natl. Acad. Sci. 112, 8019–8024. doi: 10.1073/pnas.1503283112
Kellner, K. F., Duchamp, J. E., and Swihart, R. K. (2019). Niche breadth and vertebrate sensitivity to habitat modification: signals from multiple taxa across replicated landscapes. Biodivers. Conserv. 28, 2647–2667. doi: 10.1007/s10531-019-01785-w
Koen, E. L., Bowman, J., Lalor, J. L., and Wilson, P. J. (2014a). Continental-scale assessment of the hybrid zone between bobcat and Canada lynx. Biol. Conserv. 178, 107–115. doi: 10.1016/j.biocon.2014.07.016
Koen, E. L., Bowman, J., Murray, D. L., and Wilson, P. J. (2014b). Climate change reduces genetic diversity of Canada lynx at the trailing edge range. Ecography 37, 754–762. doi: 10.1111/j.1600-0587.2013.00629.x
Lanszki, J., Hayward, M. W., Ranc, N., and Zalewski, A. (2022). Dietary flexibility promotes range expansion: the case of golden jackals in Eurasia. J. Biogeogr. 49, 993–1005. doi: 10.1111/jbi.14372
Lavoie, M., Collin, P.-Y., Lemieux, F., Jolicoeur, H., Canac-Marquis, P., and Larivière, S. (2009). Understanding fluctuations in bobcat harvest at the northern limit of their range. J. Wildl. Manag. 73, 870–875. doi: 10.2193/2008-275
Layman, C. A., Araujo, M. S., Boucek, R., Hammerschlag-Peyer, C. M., Harrison, E., Jud, Z. R., et al. (2012). Applying stable isotopes to examine food-web structure: an overview of analytical tools. Biol. Rev. 87, 545–562. doi: 10.1111/j.1469-185X.2011.00208.x
Legendre, P., and Legendre, L. (2012). “Tests of normality and multinormality,” in Numerical Ecology. eds: P. Legendre and L. Legendre (Oxford: Elsevier B.V), 187–194.
Lysy, M., Stasko, A. D., and Swanson, H. K.. (2015). R package ‘niche ROVER’: (Niche) (R)egion and Niche (Over)lap Metrics for Multidimensional Ecological Niches, v 1.0.
Mac Arthur, R. H., and Levins, R. (1964). Competition, habitat selection, and character displacement in a patchy environment. Proc. Natl. Acad. Sci. U. S. A. 51, 1207–1210. doi: 10.1073/pnas.51.6.1207
Manenti, R., Denoël, M., and Ficetola, G. F. (2013). Foraging plasticity favours adaptation to new habitats in fire salamanders. Anim. Behav. 86, 375–382. doi: 10.1016/j.anbehav.2013.05.028
Marrotte, R. R., and Bowman, J. (2021). Seven decades of southern range dynamics of Canada lynx. Ecol. Evol. 11, 4644–4655. doi: 10.1002/ece3.7364
McGill, B. J., Enquist, B. J., Weiher, E., and Westoby, M. (2006). Rebuilding community ecology from functional traits. Trends Ecol. Evol. 21, 178–185. doi: 10.1016/j.tree.2006.02.002
Merkle, J. A., Polfus, J. L., Debridge, J. J., and Heinemeyer, K. S. (2017). Dietary niche partitioning among black bears, grizzly bears, and wolves in a multiprey ecosystem. Can. J. Zool. 95, 663–671. doi: 10.1139/cjz-2016-0258
Navarro-López, J., and Fargallo, J. A. (2015). Trophic niche in a raptor species: the relationship between diet diversity, habitat diversity and territory quality. PLoS One 10:e0128855. doi: 10.1371/journal.pone.0128855
Newbury, R. K., and Hodges, K. E. (2018). Regional differences in winter diets of bobcats in their northern range. Ecol. Evol. 8, 11100–11110. doi: 10.1002/ece3.4576
O’Donoghue, M., Boutin, S., Murray, D. L., Krebs, C. J., Hofer, E. J., Breittenmoser, U., et al. (2001). Coyotes and Lynx in Ecosystem Dynamics of the Boreal Forest: The Kluane Project. eds. C. J. Krebs, S. Boutin, and R. Boonstra (New York: Oxford University Press), 275–323.
Papacostas, K. J., and Freestone, A. L. (2016). Latitudinal gradient in niche breadth of brachyuran crabs. Glob. Ecol. Biogeogr. 25, 207–217. doi: 10.1111/geb.12400
Peers, M. J. L., Thornton, D. H., and Murray, D. L. (2013). Evidence for large-scale effects of competition: niche displacement in Canada lynx and bobcat. Proc. R. Soc. B Biol. Sci. 280:20132495. doi: 10.1098/rspb.2013.2495
Quinn, N. W. S., and Parker, G. (1987). “Lynx,” in Wild furbearer management and conservation in North America. eds. M. Novak, J. A. Baker, M. E. Obbard, and B. Malloch (Peterborough, ON: Ontario Fur Managers Federation), 682–694.
Rivas-Salvador, J., Hořák, D., and Reif, J. (2019). Spatial patterns in habitat specialization of European bird communities. Ecol. Indic. 105, 57–69. doi: 10.1016/j.ecolind.2019.05.063
Roberts, N. M., and Crimmins, S. M. (2010). Bobcat population status and management in North America: evidence of large-scale population increase. J. Fish Wildl. Manag. 1, 169–174. doi: 10.3996/122009-JFWM-026
Rogers, M. C., Hilderbrand, G. V., Gustine, D. D., Joly, K., Leacock, W. B., Mangipane, B. A., et al. (2020). Splitting hairs: dietary niche breadth modelling using stable isotope analysis of sequentially grown tissue. Isot. Environ. Health Stud. 56, 358–369. doi: 10.1080/10256016.2020.1787404
Rosenzweig, M. L. (1991). Habitat selection and population interactions: The search for mechanism. Am. Nat. 137:S5-S28. doi: 10.1086/285137
Roth, J. D., Marshall, J. D., Murray, D. L., Nickerson, D. M., and Steury, T. D. (2007). Geographical gradients in diet affect population dynamics of Canada lynx. Ecology 88, 2736–2743. doi: 10.1890/07-0147.1
Row, J. R., Wilson, P. J., Gomez, C., Koen, E. L., Bowman, J., Thornton, J., et al. (2014). The subtle role of climate change on population genetic structure in Canada lynx. Glob. Chang. Biol. 20, 2076–2086. doi: 10.1111/gcb.12526
Stevens, R. D. (2022). Reflections of Grinnellian and Eltonian niches on the distribution of phyllostomid bats in the Atlantic Forest. J. Biogeogr. 49, 94–103. doi: 10.1111/jbi.14284
Svanbäck, R., and Bolnick, D. I. (2007). Intraspecific competition drives increased resourxe use diversity. Within a natural population. Proc. R. Soc. B 274, 839–844. doi: 10.1098/rspb.2006.0198
Swanson, H. K., Lysy, M., Power, M., Stasko, A. D., Johnson, J. D., and Reist, J. D. (2015). A new probabilistic method for quantifying n-dimensional ecological niches and niche overlap. Ecology 96, 318–324. doi: 10.1890/14-0235.1
Walsh, L. L., and Tucker, P. K. (2020). Isotopic niche breadth of a generalist mesopredator increases with habitat heterogeneity across its range. Ecosphere 11:e03314. doi: 10.1002/ecs2.3314
Witczuk, J., Pagacz, S., Gliwicz, J., and Mills, S. (2015). Niche overlap between sympatric coyotes and bobcats in highland zones of Olympic Mountains, Washington. J. Zool. 297, 176–183. doi: 10.1111/jzo.12270
Wolf, N., Carleton, S. A., and Martinez del Rio, C. (2009). Ten years of experimental animal isotopic ecology. Funct. Ecol. 23, 17–26. doi: 10.1111/j.1365-2435.2009.01529.x
Woo, K. J., Elliot, K. H., Davidson, M., Gaston, A. J., and Davoren, G. K. (2008). Individual specialization in diet by a generalist marine predator reflects specialization in foraging behaviour. J. Anim. Ecol. 77, 1082–1091. doi: 10.1111/j.1365-2656.2008.01429.x
Yurkowski, D. J., Ferguson, S., Choy, E. S., Loseto, L. L., Brown, T. M., Muir, D. C. G., et al. (2016). Latitudinal variation in ecological opportunity and intraspecific competition indicates differences in niche variability and diet specialization of Arctic marine predators. Ecol. Evol. 6, 1666–1678. doi: 10.1002/ece3.1980
Keywords: Lynx canadensis, Lynx rufus, specialist-generalist paradigm, niche breadth, niche overlap, broad-scale patterns
Citation: Gobin J, Szumski CM, Roth JD and Murray DL (2022) Patterns of dietary niche breadth and overlap are maintained for two closely related carnivores across broad geographic scales. Front. Ecol. Evol. 10:1059155. doi: 10.3389/fevo.2022.1059155
Edited by:
Jason Newton, University of Glasgow, United KingdomReviewed by:
Daniel Ernesto Naya, Universidad de la República, UruguayLeandro Bergamino, Universidad de la República, Uruguay
Copyright © 2022 Gobin, Szumski, Roth and Murray. This is an open-access article distributed under the terms of the Creative Commons Attribution License (CC BY). The use, distribution or reproduction in other forums is permitted, provided the original author(s) and the copyright owner(s) are credited and that the original publication in this journal is cited, in accordance with accepted academic practice. No use, distribution or reproduction is permitted which does not comply with these terms.
*Correspondence: Jenilee Gobin, amVuaWxlZWdvYmluQHRyZW50dS5jYQ==