- Department of Biology, University of Padova, Padova, Italy
By definition, biology is the science of all living beings. However, horizons restricted to either plants or animals have characterized the development of life sciences well beyond the emergence of unified perspectives applying to all forms of life, such as the cell theory or the theory of evolution. Separation between botanical and zoological traditions is not destined to go extinct easily, or shortly. Disciplinary isolation is emphasized by institutional contexts such as scientific societies and their congresses, specialist journals, disciplines recognized as teaching subjects and legitimate and fundable research fields. By shaping the personal agendas of individual scientists, this has a strong impact on the development of biology. In some fields, botanical and zoological contributions have long being effectively intertwined, but in many others plant and animal biology have failed to progress beyond a marginal dialogue. Characteristically, the so-called “general biology” and the philosophy of biology are still zoocentric (and often vertebrato- or even anthropocentric). In this article, I discuss legitimacy and fruitfulness of some old lexical and conceptual exchanges between the two traditions (cell, tissue, and embryo). Finally, moving to recent developments, I compare the contributions of plant vs. animal biology to the establishment of evolutionary developmental biology. We cannot expect that stronger integration between the different strands of life sciences will soon emerge by self-organization, but highlighting this persisting imbalance between plant and animal biology will arguably foster progress.
Very soon after I began to teach Natural History, or what we now call Biology, at the Royal School of Mines, some 20 years ago, I arrived at the conviction that the study of living bodies is really one discipline, which is divided into Zoology and Botany simply as a matter of convenience; and that the scientific Zoologist should no more be ignorant of the fundamental phenomena of vegetable life, than the scientific Botanist of those of animal existence. Huxley and Martin (1875); Preface by T. H. H., p. V
Introduction
A few years before fixing in these words his views on biology, Huxley had published a series of articles that linked discussions on cell, protoplasm, and evolution to proposed changes in medical and science education (Huxley, 1869); soon thereafter he characterized biology as a synthetic and unitary science that makes use of the contribution of all life disciplines (Huxley, 1877).
Nevertheless, a century and a half later, and more than two centuries since the first use of the term biology to indicate the study of living beings (Lamarck, 1802; Treviranus, 1802), biology is still treated today as a huge convenient umbrella for independent research agendas, more than a real unitary science. To be sure, any effort to generalize must necessarily come to terms with the structural and functional diversity of living beings. But the problem does not lie only in the difficulty of finding unity in diversity. Serious problems are also due to the history of the various disciplines that study the living beings, a history reflected in the academic systems of university studies, in the names of scientific societies and in the subjects of their congresses, in the titles and contents of scientific journals. To some extent, the dividing lines can still be traced back to the distance that for centuries separated the zoological from the botanical tradition and to the marginality in which the studies on organisms other than animals and plants were left, often forcibly shoehorned within disciplines that do no justice to their phylogenetic position–such as fungi, “algae,” and different groups of protists, marginalized both by botany and zoology.
Today, it is legitimate to debate if the ambitious program to develop a general biology as a unitary science is still sensible, or useful. Two full centuries since the term biology was first used with a broadly similar meaning, three main dimensions have emerged as critical to define this project: first, the delimitation of biology in respect to the other sciences; second, the integration between the research agendas of the main branches of the study of life, traditional as well as recent ones; third, the broadest taxonomic scope. In the following pages I will briefly examine a number of issues deriving from the still well-entrenched separation between the zoological and botanical traditions, with respect to the reciprocal exchanges between them–a trading arena very often marred by lack of interest for the advances in the other field or, to the contrary, by unwarranted uncritical transfer of research projects and the associated language. For example, in reproductive biology, the use of a very different terminology in the description of phenomena in plants and animals, respectively, continues to hinder comparisons and attempts at generalization (Fusco and Minelli, 2019).
Terminological inconsistence and associated risk of misunderstanding are also frequent within a strictly, or mainly, zoological or botanical tradition. These semantic issues are often evidence for the lack of concern for a truly general approach to biology. Just for the sake of examples from different biological disciplines, consider the inconsistent use of three terms: preformation, cryptic species, and terminal addition.
In biology, preformation is the term for the old view of development, according to which the new individual is already present in the egg, or in the spermatozoon (Farley, 1982; Pinto-Correia, 1997) or, at least, somehow elaborated in the ovary of the mother or in the testes of the father (Pyle, 2006). In a botanical context, however, preformation has been used for the initiation of leaves and inflorescences one or more years prior to maturation and function (e.g., Diggle, 1997, p. 154).
Cryptic species are sets of two or more taxa that are more-or-less indistinguishable morphologically and have been therefore regarded as a single species, until their hidden diversity is eventually discovered through crossing experiments or by charactering diagnostic segments of their genome (e.g., Monro and Mayo, 2022). However, “cryptic” being per se an academic equivalent for “hidden, concealed,” the term has easily found also a different use, as “difficult to observe,” e.g., deep-sea animals (Claridge et al., 2004).
In animal developmental biology, terminal addition is “the process of addition of serial elements in a posterior subterminal growth zone” (Jacobs et al., 2005, p. 498); but the same term has been also used for the “evolutionary change that proceeds by adding stages to the end of ancestral ontogeny” (Gould, 1977, p. 7).
Historical background
The science of life
As noted by Zammito (2018) in his master work on the early German contribution to the emergence of a unitary science of living organisms, three main traditions can be recognized: natural sciences, philosophy, and medicine. The dialogue between them was not necessarily easy during the 18th and early 19th century, and the consequences of this multiple parentage are still visible today.
However, the most critical issue is the contrast between the zoological and the botanical traditions. This has a visible academic aspect, as described in the following section(s), but also a philosophical one. Aristotle credited plants with a vital soul, but not with a sentient one, as present in animals. Aldo Zullini (pers. comm.) has remarked that in the Bible’s narrative Noah was concerned for the survival of all animal species, but the fate of land plants under the Deluge did apparently not concern him at all. Still in modern times, the German philosopher and writer Reimarus (1754) regarded plants as inanimate objects.
The distance between the study of the two kingdoms of living beings was abridged effectively by Linnaeus with his extraordinarily successful Systema Naturae (13 editions), from the first (Linnæus, 1735) later described by the author himself as conspectus tantum operis et quasi mappa geographica (a mere overview and a kind of map of the whole work) to the bulky, multi-volume ones (Linnaeus, 1766/68, 1788/1793). In this work, animals and plants are treated together and similarly. But this cannot be considered a conscious step toward a unitary science of life, because the Systema also covered minerals, the third kingdom of nature, the members of which are classified by Linnaeus per classes, ordines, genera, and species similar to his treatment of animals and plants. A first strong confutation of the three Linnaean kingdoms of nature came from Pallas (1766a), who denounced that, although it is “customary to divide all the objects that make up our globe, and those that it contains, in three kingdoms–animal, plant, and mineral–[…] this distinction accepted until now is arbitrary and imaginary; Nature is organized very differently, within which, if we look at the system of Nature with a spirit free from preconceptions, we must instead recognize the primary distinction between inert and brute bodies and living and organic ones” (Pallas, 1766a, p. 3; text excerpts originally in Latin, French or German have been translated by this article’s author). It must be added that in his later works Pallas contributed important works both to zoology (e.g., Pallas, 1766b,1767/80, 1778) and botany (e.g., Pallas, 1784/88), and also to geology, but not to mineralogy or petrography.
A close affinity between plants and animals was suggested by some 18th century studies on plant and animal reproduction and development. To compare the development of plants and animals, Caspar Friedrich Wolff first tackled the study of the early stages of a plant’s development, then moved on to the chicken embryo and finally outlined a Theoria generationis (Wolff, 1759, 1764), a unitary theory of generation he aimed to apply to all organic life (Zammito, 2018). Indeed, for Spallanzani (1780), from the point of view of the mechanisms of development, there is no fundamental difference between plants and animals. More precisely, the French botanist Charles-François Brisseau de Mirbel stated that animals and plants share many structural aspects and important functional similarities, as both, in fact, are made up of cellular and vascular tissue, have a complex structure articulated in organs with functional divisions and undergo growth, development, and decay (Brisseau de Mirbel, 1802).
But the first works where the term biology was used to indicate the study of living beings (e.g., Lamarck, 1802; Treviranus, 1802) were not immediately followed by the actualization of comprehensive research programs. Exemplary in this respect is Lamarck: despite his acquaintance with both animals and plants [in 1793, when he was assigned the chair of Insects, Worms, and Microscopic Animals at the Muséum d’Histoire Naturelle, he had extensively worked on the French flora (Lamarck, 1778) but had not written a single line on animals] and his early intention to establish the foundations of a science of living beings, he stopped writing Biologie. Considérations sur la nature, les facultés, le développement et l’origine des Corps vivans (Biology. Considerations on the nature, faculties, development and origin of living bodies) soon after the first few pages, penned in 1800, eventually discovered and published in the 20th century (Lamarck, 1944); and a few years later he declared that he would never commit himself to such a project for a unitary science of life (Lamarck, 1809).
Some historians of biology argue that the actual birth of biology as an autonomous science had to wait until the advent of an evolutionary view of life. In particular, Smocovitis (1992, p. 1, n. 1) argued that “Only with evolution, which defied reduction to physics and chemistry […], at the same time that it introduced a causo-mechanical agent for evolutionary change, could biology claim autonomy. This took place in Thomas Henry Huxley’s England, and most likely in the thought of Huxley himself.” Smocovitis cites the following words as a strong support to her view: “the conscious attempt to construct a complete science of Biology hardly dates further back than Treviranus and Lamarck, at the beginning of this century, while it has received its strongest impulse, in our own day, from Darwin” (Huxley, 1884, p. 4), but this sentence can also been taken as a support for an earlier origin of biology, with the “conscious attempt to construct,” or at least to formulate, a program for “a complete science of Biology.”
Smocovitis’ position can be explained as a consequence of the widespread agreement, largely shared by many biologists even today, that nothing in biology makes sense except in the light of evolution (Dobzhansky, 1973, p. 125). This may explain why Mayr and Provine (1980) choose Perspectives on the Unification of Biology as the subtitle for their book on The Evolutionary Synthesis.
However, although it is undeniable that everything in biology can be addressed as explanandum in the light of evolution, it is also true that in biology there are plenty of dimensions other than the evolutionary one: the phenomena of development and reproduction, for example, are molded by evolution, as everything in life, but are also per se worthy of study as autonomous disciplines.
In historical perspective, however, the main consequence of the identification of the actual birth of biology with the advent of a Darwinian view of evolution is the disregard for the role of the cell theory formulated by Schwann (1839) ca. 20 years before the Origin (Darwin, 1859): “it may be asserted, that there is one universal principle of development for the elementary parts of organisms, however different, and that this principle is the formation of cells. […] The development of the proposition, that there exists one general principle for the formation of all organic productions, and that this principle is the formation of cells, as well as the conclusion which may be drawn from this proposition, may be comprised under the term cell-theory” [Schwann (1847, p. 165), italics as in the original; text equivalent to the earlier German version in Schwann (1839, p. 197)]. The specific object of this latter work is “to demonstrate the most intimate connection between the two kingdoms of organic nature, starting from the similarity of the laws of development of the elementary parts of animals and plants” (Schwann, 1847, p. IX).
It is widely known that this view of the cell as the core unit of all life was soon thereafter strengthened by Rudolf Virchow, nevertheless it is worth citing verbatim from the article in which Virchow introduced the aphorism omnis cellula a cellula (Virchow, 1855, p. 23), subsequently modified by Leydig (1857, p. 9) into the more popular but strictly equivalent form omnis cellula e cellula: “This certainly sober view is far from being merely speculative; rather, it is so empirical that it only broke through to me when I was able, by demonstrating the connective tissue corpuscles and by describing the cellular nature of the cartilage and bone corpuscles, to also dissect the body of the adult vertebrate in cell territories in a way that until then had only been known in the embryo, in some lower animals and in plants. Only then was it possible to have a unified view of the entire biological field, and by conceptually combining the various facts, a general principle was found” (Virchow, 1855, p. 24).
Zoology vs. botany: Centuries of academic and intellectual independence
In many institutional contexts, botany has long been excluded from the set of disciplines called natural sciences. Separate chairs for botany vs. natural history (zoology and earth sciences) are found until well into the 19th century at many European universities. For example, at the University of Padua, the study of botany, which began with the foundation of the Botanical Garden in 1545, remains completely separate from that of the other disciplines, starting with the establishment in 1734 of a chair for natural sciences, from which in 1869 will derive a chair of zoology and comparative anatomy and a chair of geology. A meeting point between the study and teaching of zoology and botany took place only with the establishment of the Faculty of Mathematical, Physical, and Natural Sciences, in 1872.
Things were not different in America, e.g., at Harvard, where in 1847 Louis Agassiz became Professor of Geology and Zoology. In the following years, Agassiz published, together with Hugh E. Strickland, a bulky Bibliographia zoologiae et geologiae. A General Catalog of All Books, Tracts, and Memoirs on Zoology and Geology (Agassiz and Strickland, 1848). This disciplinary association, somehow odd from the perspective of today, was actually the same as a previous literature repertoire published by Gronovius (1760a), who also published a Bibliotheca botanica as a separate volume (Gronovius, 1760b). In America at least, a close academic association between zoology and geology, to the exclusion of botany, continued to more recent times. For example, Alpheus Spring Packard Jr., one of the founders of The American Naturalist (1867), taught zoology and geology at Brown University in Providence, Rhode Island, until his death in 1905 (Cockerell, 1920). The academic coupling of geology and zoology extends into our times: between 1967 and 2002, Stephen Jay Gould had the distinction to be, at the same time, Alexander Agassiz Professor of Zoology and Professor of Geology at Harvard University.
As documented by Caron (1988), new disciplinary arrangements involving botany and zoology emerge in England soon after the publication of the Origin. In this institutional sense, the catalytic role of evolutionary theory is evident. In 1860 Thomas Henry Huxley and the botanist Joseph Dalton Hooker succeeded having biology among the disciplines taught at the University of London. In 1866, the British Association for the Advancement of Science (BAAS) created, albeit with difficulty, a biology section. But these improvements toward a unification of the two separate traditions in the study of animals and plants did not last long. Before the end of the century, the biology section of BAAS was dismembered. At the University of London, with Huxley’s retirement the chair of Biology was replaced by a chair of animal biology and one of plant biology; in 1898 the Faculty of Science replaced the biology exam with two separate exams, one in zoology and one in botany.
Similar contrasts affected the academic burgeoning of a unified biology in America, as documented, for instance, by a debate published by the Botanical Gazette in 1890–91. Here are a few insightful excerpts: “one of the foremost of our universities has two unusually able men teaching zoology, and practically no botanical instruction […] the instruction in botany in many of the colleges is yet of the high school grade, recognizing the existence of no plants but the Phanerogams” (Anon, 1890a, p. 180); “The one-sided method of teaching biology pursued in one of our great universities and emphasized in more than one text-book is distinctly deplorable. One even notes in certain circles a tendency to read botany out of the scientific party altogether […]. Men are sent out from the university referred to in the GAZETTE editorial, and from other American institutions, who are totally devoid of any botanical training and totally pervaded with an uncontrollable yearning to label their zoological courses with the word “biology.” […] Doubtless this uncritical use of terminology is fostered by the uncritical study of biology which obtains whenever the great coordinate branch of botany is lopped off and thrown in the fire as a preliminary. Possibly, too, it is due to diffidence and possibly to sheer ignorance. But principally, I am inclined to think, it is the child of shrinking one-sidedness, the progeny of ill-balanced courses of study and of past iniquity in methods of zoological instruction which makes the very word “zoölogy” distasteful to the teacher of to-day” (Anon, 1890b, p. 236–7).
Zoological vs. botanical versions of biological disciplines
In the study of man and, more generally, of animals, it has proved useful to recognize a science of cells (cytology), a science of tissues (histology), and a science of embryonic development (embryology). Many biologists take it for granted that this disciplinary articulation can be generalized to all multicellular organisms; in particular, corresponding disciplines are recognized in plant biology, but the legitimacy of this parallelism between animal biology and plant biology deserves a critical review. Substantial exchange between the researches on plant and animal systems has accompanied the development of cell biology, at least until the first years of the 19th century. Reciprocal illumination, however, has been much lower at higher level of biological organization, in developmental biology especially, as briefly surveyed in the next sections.
Fruitful exchange in cell biology
The botanist Carl Wilhelm von Naegeli (1842) first observed in the nucleus of plant cells elongated bodies, to which the human anatomist Waldeyer (1888) will give the name of chromosomes.
Two zoologists introduced the terms karyokinesis (Schleicher, 1878) and mitosis (Flemming, 1882), but three of the phases of mitosis (prophase, metaphase, and anaphase) have been named by a botanist (Strasburger, 1884), the fourth (telophase) by the comparative anatomist, embryologist, and histologist Heidenhain (1894).
The alternation between the haploid number and the diploid number of chromosomes during the life cycle was also clarified with the joint contribution of zoologists and botanists.
The zoologist Van Beneden (1883) observed in the roundworm that fertilization leads to a doubling of the chromosomal number, since the chromosomes carried by the nucleus of the spermatozoon are added to those carried by the nucleus of the egg, while all cell divisions give rise to daughter cells with a number of chromosomes identical to each other and equal to that of the mother cell. Therefore, the life cycle must include a reductional division.
Meiosis was first described in sea urchin eggs by Hertwig (1876). Boveri (1887) studied the division processes that lead to the formation of the egg cell in the roundworm Ascaris, with the emission of polar cells. Farmer and Moore (1905), a botanist and a zoologist respectively, gave a name to this reductional division, using the now forgotten spelling “maiosis”–soon changed to “meiosis” by Koernicke (1905).
In some of the major comprehensive works on cytology published in the last years of the 19th century, both plant and animal cells (sometimes also “protozoans”) were considered, despite the obviously sounder confidence of the author with the first (Strasburger, 1877, 1907) or second kingdom (Hertwig, 1893; actually, a book on both cytology and histology: Die Zelle und die Gewebe; Wilson, 1896). However, Maienschein’s (1991, pp. 46–47) characterization of Edmund Beecher Wilson’s volume as the last monumental, synthetic and all inclusive cytology textbook to have been written by an individual author betrays the historian’s zoocentric perspective. To be sure, Wilson’s book included a few scattered paragraphs on plants, but their weight and extent can be measured by the number of corresponding illustrations: out of a total of 142, there are only six of vascular plants and five of “algae” (two of Volvox and one each of Chara, Spirogyra, and Closterium).
This taxonomically broad perspective was rapidly lost, however, in the cytology textbooks published thereafter. A significant example is the General Cytology edited by Cowdry (1924), for two different but equally important reasons. First, in this 754-pages book, the plant cell is considered, marginally, in two chapters only, on the permeability of the cell (sic) to diffusing substances (Jacobs, 1924) and, a bit more extensively, on the Mendelian heredity in relation to cytology (Morgan, 1924). This coverage clearly does not fulfill the volume’s intended aim as stated in the Editor’s Preface, where botany is the first biological science to the service of which the book should have been written: “During the summer of 1922 several meetings were held at the Marine Biological Laboratory at Woods Hole, Massachusetts, in order to consider the advisability of making a co-operative attempt to state in general terms what is known or conjectured regarding the principles which govern cellular structure and function; or, in other words, to present briefly for the first time within the scope of a single volume data concerning the cell fundamental, alike, to the sciences of botany, zoology, physiology, and pathology.” Second, by delivering to the printer the General Cytology textbook, Cowdry announced the forthcoming publication of a Special Cytology (Cowdry, 1928), intended as A Companion and Supplementary Volume to General Cytology. By “special,” however, the volume editor did not mean the biology of plant and animal cells: “On the basis of the fundamental data concerning cells of all kinds given in General Cytology, this second volume will, it is hoped, bear a superstructure of information regarding the chief types of cells that make up the human body, the blood cells, nerve cells, gland cells, and others.” Thus, eventually, nothing more than cell biology in its ancillary role to medicine.
Tissues and embryos
Parallelism between zoology and botany is quite obvious in some disciplines, especially those with longer tradition such as taxonomy: “Botanists were the first to give an example to zoologists of the true disposition to give to a general distribution in order to represent the very order of nature […]. What botanists have done with regard to plants, we must finally do with regard to the animal kingdom” (Lamarck, 1809, 1: 271–2). The same was true for phylogenetics, since the first comprehensive efforts of Haeckel (1866), which include, alongside a graphical depiction of the affinities among the major animal groups, also the first use of tree metaphor for plant phylogeny (Tassy, 2011). In disciplines conceived in times close to us, such as genetics and molecular biology, a separation between animal and plant versions appears since the beginning less justified and less useful.
More problematic are the different aspects of organismic biology. Although the term “plant histology” is not as popular as its zoological (or medical) equivalent, and quite often–see, for example, the essays on the history of histology by Zanobio (1971) and Bracegirdle (1977), both of which deal exclusively with human histology–tissues are commonly described also in the botanical literature. There is, however, much to be discussed on the appropriateness of using the same term for the “tissues” of animals and plants. Let’s just mention the space reserved in plant histology for tissues formed by dead cells, where all the cellular material has disappeared, leaving only the walls, such as those forming the little cells found by Hooke (1665, p. 114) in the cork.
Another problematic area is a plant embryology understood as the botanical equivalent of animal embryology. Still within zoology, the meaning of the term “embryo” is not universally obvious, e.g., in sponges. In botany, at least until the beginning of the 18th century it is still uncertain whether “embryo” is used to indicate the ovary or its contents: “If the calyx crowns the ovary or the embryo of the fruit, which is one and the same, it is certain that it is monophyllous, and that it constitutes one body with the embryo” (Vaillant, 1718, p. 29). This is a significant quote, since Vaillant was among the first to be convinced that sexuality existed in plants. Less clear is Linnaeus (“when the seed sprouts from the earth, it also grows and puts out roots […], so much so that I would gladly have it as certain and indubitable, that the embryo before fertilization is hidden in its bud like marrow”; Linnaeus, 1756, p. 31), also because elsewhere he uses the term “embryo” even in the description of minerals. For example, among the Fossilia Concreta, Linnæus (1744) recognized a genus Ætites with species like Ætites Geodes, diagnosed as Ætites embryone libero terrestri.
Very different was the description of plant reproductive structures by Boerhaave (1727), who borrowed from medicine a number of terms: “Those plants are called Dicotyledons, whose seeds, when sown, come out of the ground with two anomalous seminal leaves, or are divided into two lobes, […] these plants always have an Embryo between the little placentas, from which we see that every seed is a uterus, and the plant is stored within it” (Boerhaave, 1727, p. 21). Eventually terms and concepts borrowed from human (and animal) embryology are redefined and extensively used by Gaertner (1788) in his large treatise De fructibus et seminibus plantarum (On Plant Fruits and Seeds). Some of these terms remain in use today for both plants and animals, but nobody would venture to say, for example, that the placenta of plants is homologous to the placenta of mammals. Unfortunately, however, the idea of an equivalence between what is called an embryo in either kingdom is still widespread, even among professionals.
The comparative method: Contrasting traditions
Until the advent of cladistics, the approach to the comparative method was somewhat different in zoology and botany. To clarify the main differences between the two traditions, it is useful to take as a reference the triple distinction introduced by Owen (1847, 1848) between special homology (correspondence between organs of different animals), serial homology (correspondence between parts of the same animal) and general homology (correspondence between parts of an animal and elements of the archetype). Before the formulation of these definitions and the introduction, by himself Owen (1843), of the definitions of analog and homolog that are generally considered the reference point in these matters, animal comparative anatomy was mainly based on what will be later called special homology. This applies to the admirable comparison between the human skeleton and the skeleton of a bird proposed by Belon (1555), with specification of the correspondences between the two, bone by bone; it applies to the comparative anatomy of Georges Cuvier and Étienne Geoffroy Saint-Hilaire’s (cf. Appel, 1987), although for what Owen will call special homology Geoffroy Saint-Hilaire used the term analogie, while he used homologie for what later will be called serial homology (Geoffroy Saint-Hilaire, 1830). Owen’s archetype (actually limited to the vertebrate skeleton) has an antecedent in typus Goethe’s (1790b) and it is precisely in Goethe, who turned his attention to both plant and animal forms, that we find a fundamental difference between plant comparative morphology vs. its zoological equivalent. For plants, Goethe (1790a) did not imagine a typus, but proposed an ideal process of continuous transformation in which alles ist Blatt [everything is leaf; actually first spelled by Goethe alles ist Blat in a manuscript note eventually published in Goethe (1892)]. In the following decades, while in the comparative anatomy of animals the fundamental notions traced by Owen in terms of special homology and serial homology will be both developed and revised [e.g., by Lankester (1870)], in botany the corresponding developments–especially but not exclusively in the German tradition–will be influenced by the Goethian approach at least until Wilhelm Troll’s major works (e.g., Troll, 1937/43; see Kaplan, 2001).
At the beginning of the 20th century, Russell (1916) will only deal with animals in his history of morphology and the comparative method; similarly, plants are not mentioned in the essays by Naef (1927) and Boyden (1943) on homology or in Remane’s (1952) volume on Die Grundlagen des natürlichen Systems, der vergleichenden Anatomie und der Phylogenetik (Foundations of the natural system, comparative anatomy and phylogenetics). Same for Jardine (1967), except for mentioning Goethe and Owen. By contrast, only plants are considered by most botanists who have been writing about the comparative method since the mid-20th century (e.g., Mason, 1957; Sattler, 1966, 2022; Kaplan, 1971; Tomlinson, 1984; Sokoloff and Remizowa, 2021). Infrequent are the articles in which both plants and animals were considered (e.g., Sattler, 1984, 1988). This has changed with the advent of cladistics, whose methods and theoretical problems have been developed with contributions by both zoologists and botanists. Meanwhile, the early, important contribution of the botanist Walter Zimmermann to the birth of phylogenetic systematics (Zimmermann, 1959a) has turned to the light (Donoghue and Kadereit, 1992): his work had been long obscured by the success of Hennig’s works (especially Hennig, 1966).
An assessment of the relative contributions of zoologists and botanists to the development of the concept of homology can be found in Hoßfeld and Olsson (2005) who reconstruct this history without citing botanical papers except for Bachmann (1989); and Kleisner’s (2007) on The Formation of the Theory of Homology in Biological Sciences cites only eight botanical publications in a bibliography of 154 titles.
An original contribution of plant comparative morphology, anticipated by several works by Arber (1941, 1950) and Zimmermann (1959b) is the Continuum Morphology or Fuzzy Arberian Morphology (e.g., Sattler and Jeune, 1992; Rutishauser, 1995, 1999; Rutishauser and Isler, 2001; Rutishauser et al., 2008), intended as an alternative to Classical Morphology. In the latter, the traditional items of vascular plant organization, e.g., root, shoot, and leaf, are regarded as concepts with clear-cut borders and, in principle, without intermediates; in Fuzzy Arberian Morphology, these structural categories are treated as concepts with fuzzy borders, allowing for the occurrence of virtually any intermediate. This theoretical pluralism in plant sciences “provides an instructive, complementary perspective on what evo-devo modularity could be, [but] this fact has been overlooked quite often” (Vergara-Silva, 2003, p. 260–1) in botany, up to our days (Sattler, 2022) and virtually ignored in zoology.
Plants and animals in evo-devo–exchange and sharing
The taxonomic scope
As noted by Bateman and DiMichele (1994, p. 63), “the remarkable repertoire of the most prominent [then] living evolutionary essayist, S. J. Gould, is notable for a dearth of botanical examples. Reviews of evolutionary mechanisms in the kingdoms Plantae and Fungi […] are far fewer and have coopted with minor modifications theories that are essentially zoocentric.”
Taxonomic coverage in academic journals
“[M]ost of the current efforts to establish the boundaries and internal structure of [evo-devo] rely on information pertaining to only one of the kingdoms in which ontogenetic development occurs: animals” (Vergara-Silva, 2003, p. 250).
A bibliometric analysis of the contents of a representative sample of journals whose disciplinary coverage spans between developmental biology and evolutionary developmental biology demonstrates the strong bias in these areas in favor of animals and the very limited exchange between mainstream studies focusing on plants and animals, respectively. The sample (Table 1) includes 22 journals, of which 19 have been selected among the 40 titles in the current list of titles covered by the Web of Science1 for the Developmental Biology category. Of the remaining titles, one (Journal of Evolutionary Biology) has been selected from the Web of Science journal list for Evolutionary Biology (a list of 60 titles, among which four are also present in the list for Developmental Biology and are all included in this article’s analysis); the two remaining titles (BMC Developmental Biology, Frontiers in Ecology and Evolution–Evolutionary Developmental Biology) have been added because of their contents, despite their absence from the current Web of Science coverage. The table specifies the range of years for which the journal’s content has been sampled. For some journals with very high number of published papers, the analysis has been limited to one year in three during the covered time span. Overall, the total number of papers published in the sampled journals/years is 66,667 articles. Except for the Journal of Experimental Zoology Part B, no journal title suggest a bias in favor of animals as study objects, nevertheless the botanical coverage is over 5% only in five journals: Journal of Evolutionary Biology (16.1% before 2000, but only 7.7% thereafter), the section on Evolutionary Developmental Biology of Frontiers in Ecology and Evolution (9.9%), EvoDevo (8.4%), Genesis (8.3% before 2000, but only 1.1% thereafter), Current Topics in Developmental Biology (5.2%). Still more meager is the presence of articles on organisms other than animals and plants, except for Dictyostelium, more frequently treated than plants in BMC Developmental Biology, Development, Growth and Differentiation, Developmental Biology, Differentiation, and especially in the pre-2000 volumes of Genesis.
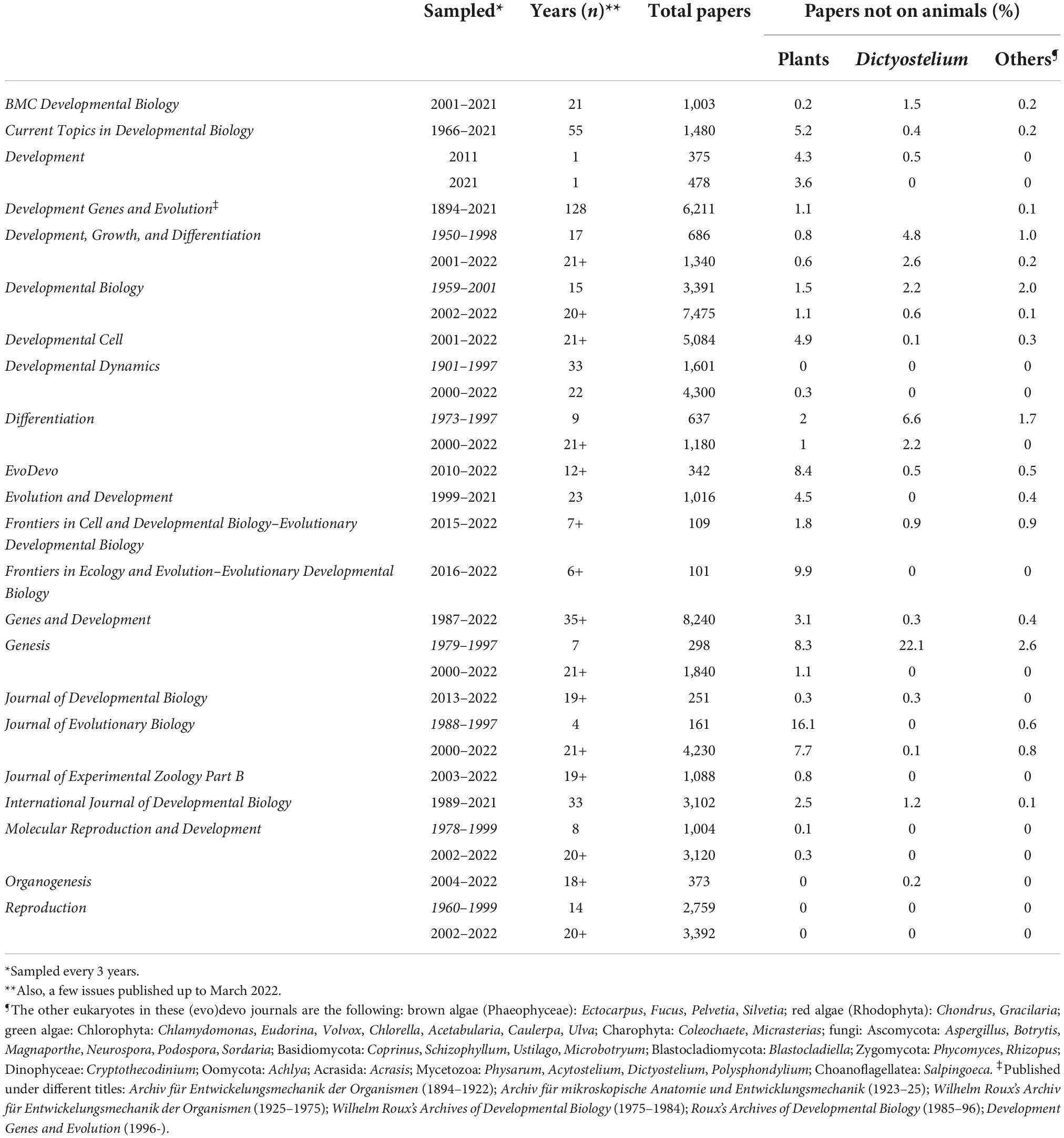
Table 1. Quantitative analysis of papers on organisms other than animals (plants, Dictyostelium, others) in the major journals on developmental biology and evolutionary developmental biology, and in the Journal of Evolutionary Biology.
Some eukaryotes other than animals and plants
Volvox
Described by Linnaeus (1758) as the last genus of the Vermes Zoophyta, Volvox remained within the province of zoology for about one century. For example, we find it in Grant’s (1841) Outlines of Comparative Anatomy, presenting a sketch of the present state of knowledge, and of the progress of discovery, in that science; and designed to serve as an introduction to animal physiology, and to the principles of classification in zoology, where it is described as a loricated animalcule (p. 3), or a compound or aggregate animalcule (p. 249).
Arguments suggesting its placement in the plant kingdom where first floated around 1850, but were not promptly accepted. This explains Cohn’s choice to describe a related genus Stephanosphaera, (currently in Haematococcaceae, a family of the Chlamydomonadales like the Volvocaceae within which Volvox is classified) in the Zeitschrift für wissenschaftliche Zoologie “for zoologists have hitherto been the only ones to take an interest in the forms of the Volvocines, and even now they seem reluctant to leave to the botanists this interesting family, to which, as will be hereafter developed, they nevertheless wrongly lay claim” (Cohn, 1852, p. 91).
The zoologists’ interest in Volvox has lasted quite longer. The digitized copy of Cohn’s (1875) Die Entwickelungsgeschichte der Gattung Volvox (The Development of the Genus Volvox) available through the Biodiversity Heritage Library belong(ed) to a zoological library.
Comparisons between animals and Volvox have been fashionable for a century at least, and are still occasionally proposed. Volvox has been long used as a model system for studying the ontogeny and phylogeny of multicellularity and cellular differentiation, often stressing the circumstance that the comparatively simple organization of this colonial green alga makes it easy to study developmental processes (e.g., embryogenesis, tissue remodeling, and cell differentiation) similar to those of more complex organisms, both animals and plants (e.g., Bonner, 1950; Kirk, 2000; Schmitt, 2003; Desnitskiy, 2009; Nishii and Miller, 2010; Herron, 2016; Matt and Umen, 2016). A number of studies have focused on traits unique to Volvox, e.g., the cytoplasmic bridge, a type of cell-to-cell attachment that takes place during this alga’s morphogenesis, different from both the junctions found in animal cells and the plasmodesmata of plants (Green and Kirk, 1981; Green et al., 1981). For a long while, zoologists have been puzzled by the similarity between the inversion of cell layers observed in early (“embryonic”) stages of Volvox morphogenesis and similar behavior in sponges–similar but unrelated (Bonner, 1950; Höhn et al., 2015; Herron, 2016; Desnitskiy, 2018).
Unfortunately, rather than including comparisons based on a reasonable phylogenetic background, some studies use Volvox as a key step along a formal morphocline, e.g., from isogamy to oogamy (Herron, 2016).
The claim (e.g., von der Heyde and Hallmann, 2022) that volvocine algae show common characteristics with both vascular plants and animals is uninteresting, unless placed in the correct phylogenetic context, which reveals that these common characteristics are mainly the result of parallel evolution. For sure, it does not help to use, in a broad comparative context, old-fashion taxonomic terms such as “the large group of algae (including green, red, and brown algae, diatoms, and dinoflagellates)” (p. 1327). For example, contrasting it with the unicellular relative Chlamydomonas reinhardtii, also an established model organism, von der Heyde and Hallmann (2022) stress the interest of Volvox carteri as an early example of organism with germ-soma differentiation. This is an interesting feature per se and justifies comparative molecular genetic analysis to understand how different genes regulate germ-soma differentiation (Schmitt, 2003; Herron, 2016; Matt and Umen, 2018). However, in phylogenetic perspective it is does not help explaining the evolution of germ-soma distinction in either animals (whose lineage split off from the green algae much earlier than the emergence of germ-soma distinction in the volvocines) or plants, which evolved from a different group of green algae where germ-soma distinction is apparently absent (but there have been a few suggestions to the existence of early segregated cell lineages, e.g., Lanfear, 2018; Ren et al., 2021).
Dictyostelium discoideum
At the time of the first studies of cellular slime molds from the point of view of developmental biology, it was long clear that these organisms are not close relatives of either plants or animals. For a long time they were classified with (or “near”) the fungi, only to end up in the universe of eukaryotes that do not belong to any of these three great lineages, but to an enormous radiation of forms, unicellular or multicellular, subject to increasingly complex and often radical taxonomic changes.
The increasingly frequent presence of articles on the biological cycle and developmental biology of Dictyostelium starting from the middle of the last century therefore invites us to clarify the position of these studies with respect to zoological and botanical traditions. As shown in Table 1, the presence of articles on the cellular slime molds in development biology journals has always been recognizable, sometimes even better than for articles on plants. In fact, this line of research emerges from journals on agriculture (e.g., Raper, 1937) and bacteriology (Raper and Smith, 1939) to pass to those of botany (Bonner, 1944) and, finally, to journals on developmental biology without specific animal or plant focus (Strmecki et al., 2005), retaining to this day a position of privileged organism in studies on the transition from the unicellular to the multicellular condition (e.g., Kessin, 2001; Schaap, 2011).
Evo-devo’s core concepts and research agenda
Since the first emergence of evolutionary developmental biology as a disciplinary sector of biology, a debate has opened, and is still open, on what can be considered its core issues. A certain consensus seems to have been reached at the moment on the priority position of three concepts: modularity, evolvability, and innovation.
As for modularity, we can refer to Klingenberg’s (2005, p. 6) definition: “Modules are assemblages of parts that are tightly integrated internally by relatively many and strong interactions but relatively independent of one another because there are only relatively few or weak interactions between modules.”
This notion has found greater space in the zoological literature, for example, in reference to the serial units (vertebrae, segments, etc.) recognizable along the main body axis of vertebrates, annelids, and arthropods, rather than in botany, e.g., in terms of phytomers, basic building block of the plant body composed of a lateral determinate organ, an axillary meristem, and an internode (Jaramillo and Kramer, 2007; Bell, 2008).
However, even in zoology, “more than two millennia of studying segmentation in animals have failed to produce a definition of segmentation that is applicable in even a majority of cases. Moreover, discussions on segmentation are often reduced to debates over the definition of segmentation and whether the animal or system described is actually segmented, rather than to debates over the developmental mechanisms and evolutionary processes” (Hannibal and Patel, 2013, p. 8). In the case of arthropods, the traditional notion that their bodies are made of segments must be replaced (Budd, 2001; Fusco, 2005, 2008) with the notion that the body of these animals has a multiplicity of units repeated in series, in part but not necessarily or universally integrated into blocks corresponding to the segments of traditional morphology.
The controversial nature of the notion of module has often been the subject of discussion (Schlosser, 2002; Schlosser and Wagner, 2004; Callebaut and Rasskin-Gutman, 2005; Omont and Képes, 2005; Van Speybroeck, 2005; Mitchell, 2006; Wagner et al., 2007; Esteve-Altava, 2017).
From an evolutionary perspective, however, most interesting is the contrast between the evolutionary changes limited to parts of the organism that correspond to well-recognizable morphological modules and the instances of systemic change disrupting a pre-existing modular organization (Minelli, 2015b). Of plants, the best known cases are the riverweeds and the duckweeds. The whole vegetative structure of the riverweeds (Podostemaceae; e.g., Rutishauser, 1995) deviates strongly in comparison with all other flowering plants. The most derived forms are more reminiscent of an alga than of a plant with roots, stem, branches, and leaves. In duckweeds, a basally splitting lineage within the arum family (Araceae) (Henriquez et al., 2014), the whole photosynthetic structure is reduced to a floating disk-shaped body, with one or more roots: extreme simplification is achieved in Wolffia arrhiza, a subspherical green blob less than one millimeter in diameter. Similar systemic changes are not uncommon among parasitic animals. Exemplary are three strongly modified lineages of cnidarians, i.e., Buddenbrockia (a wormlike parasite of freshwater bryozoans; Jiménez-Guri et al., 2007), Polypodium (an irregular jelly blob with a number of threadlike projections living on sturgeons’ eggs; Siddall et al., 1995), and the Myxozoa, a whole group (753 species currently listed in the Catalogue of Life, 2022) of organisms with complex life cycle classified in the past in two different protozoan classes (Wolf and Markiw, 1984; Siddall et al., 1995).
As for evolvability, it still seems difficult to fix a definition acceptable to the majority of scholars (see for example, Minelli, 2017b; Payne and Wagner, 2019; and literature therein). Let’s just mention West-Eberhard’s (1998, p. 8417) definition of evolvability as “the ability of particular features of systems to facilitate change,” and Klingenberg’s (2005, p. 221) one, focusing on “the potential of a population to respond to selection or to undergo non-adaptive evolution by drift.” Important contributions on this topic have come from both animal (e.g., Hendrikse et al., 2007) and plant evo-devo (e.g., Schlichting and Murren, 2004; Pigliucci, 2008).
Finally, innovations and novelties emerged as topics worth of targeted studies in evolutionary biology first in zoology (e.g., Mayr and Vaurie, 1948; Mayr, 1954, 1960; Bock, 1965; Fretter, 1969), later in botany (e.g., Lewis, 1962; Khanna, 1964). The basic literature is still mainly zoological (e.g., Moczek, 2008; Wagner, 2011; Peterson and Müller, 2013; Erwin, 2017, 2021; Oakley, 2017), but a number of botanical examples have been the target of intense study in recent years, e.g., nectar spurs of Aquilegia (Hodges, 1997; Fernández-Mazuecos et al., 2018). Both zoological and botanical examples are discussed together from a macroevolutionary perspective in Minelli (2016b).
Discussion and conclusion
In an article written on the occasion of the launch of the Frontiers in Evolutionary Developmental Biology section of Frontiers in Ecology and Evolution (Minelli, 2015a), I outlined a list of the grand challenges that evo-devo is facing. It is possible to take up that list again, identifying the contributions given to each of these so far by the tradition of research centered on animals, compared to that of the botanical tradition, and identify what in the less developed sector can be advantageously taken as a stimulus and example from the tradition centered on the other kingdom of the living. The list includes both evolution-centered and development-centered questions. Some of them have been pursued thus far mainly in a zoological context, e.g., constraints on developmental evolution, reversibility of evolution, exaptation of developmental processes, adultocentrism (Minelli, 2003), the developmental hourglass (see Section “Developmental hourglass”), and the internalization of releasing inputs transforming polyphenism into polymorphism; or in a botanical one, e.g., novelty following horizontal gene transfer; paramorphism (plant: M’Cosh, 1851; Uittien, 1928; cf. also the Fuzzy Arberian Morphology mentioned above in Section “The comparative method: contrasting tradition”; animal: Minelli, 2000); the remaining ones in both fields, e.g., saltational evolution (see Section “Saltational evolution”), morphological stasis, and epigenetics.
What is development–Pattern vs. process
As noted by Pradeu et al. (2016, p. 176), “including or excluding plants from the study of development changes our vision of development: it questions whether development continues in adulthood (Steeves and Sussex, 1989), whether regeneration is part of development (Birnbaum and Sánchez Alvarado, 2008), and whether development should be defined molecularly (in which case “development” would mean two different things for plants and for animals) or rather at the level of general principles (in which case there may exist a unified meaning of development, adequate for both plants and animals) (Meyerowitz, 2002; Vervoort, 2014).” Our perspective on these fundamental questions will require additional adjustments if we broaden further our taxonomic scope, to include at least the remaining multicellular organisms (Bonner, 2003), and possibly also the unicellular ones. Fungi, in particular “have historically received less attention from biologists than their plant and animal counterparts. This lack of attention […] is also evident in the philosophy of biology literature, where fungi are often completely ignored or mentioned only as pathogens of plants and animals” (Molter, 2017, p. 1117). Another group of multicellular organisms that is at last gaining attention from the perspective of broad-scope comparative developmental biology and life history studies is the brown algae (e.g., Coelho et al., 2020; Heesch et al., 2021), whereas a corresponding progress is still lacking for the red algae.
According to a traditional perspective, “to study development is to study multicellularity” (Bonner, 2001). However, the exclusion of unicells from developmental biology is unwarranted (Minelli, 2020; Mani and Tlusty, 2021; Minelli and Valero-Gracia, 2022). Along their life cycles, several unicells undergo dramatic and predictable transitions, besides those corresponding to progression along the mitotic cycle (e.g., Bozdech et al., 2003; Matthews, 2005; Ehrenkaufer et al., 2007; Kabani et al., 2009; Dayel et al., 2011; Fairclough et al., 2013; Sebé-Pedrós et al., 2016). In addition to the eukaryotic unicellulars, it may even be argued that developmental processes are also recognizable among the prokaryotes (e.g., Flemming et al., 2016; Schwartzman et al., 2022).
A robust attenuation of the barriers that still persist between the disciplinary traditions linked to the large taxonomic groups can give an important push toward a new way of facing, from the point of view of developmental biology and possibly in an evo-devo perspective, a very particular class of scaffolded systems, i.e., plant galls.
Scaffolded developmental systems (Minelli, 2016a) rely on resources that do not feed their metabolism, but are strictly required for the emergence of forms. The most popular class of scaffolded systems are multigenomic organisms such as humans with their microbiota. In this case, all but one of the partners are usually treated as scaffolding partners, involved in the development of what is considered the only partner actually undergoing development.
The only major exception to this typically unipolar approach is lichens, but plant galls also deserve attention in this respect. The morphogenesis of the symbiotic thallus form has found a (minor) place in developmental biology (e.g., Honegger, 1996). Galls have very rarely been considered as products of development processes, something they seriously deserve. Many plant galls, particularly those involving an insect, have a very specific and often complex shape, comparable to the specific and often complex shape of organisms capable of reproducing. At last, we begin to read some studies on gene expression (Bailey et al., 2015; Nabity, 2016; Takeda et al., 2019; Korgaonkar et al., 2021) eventually demonstrating that gall tissues are as different transcriptionally as they are anatomically (Martinson et al., 2021; cf. Schultz and Stone, 2022). In galls as “extended phenotypes” (Schultz and Stone, 2022) we find examples of reciprocal scaffolding (Minelli, 2017a), phenotypic plasticity, paramorphism, and combinatorial homology of exemplary importance for a general biology.
The developmental hourglass
Within several major lineages of metazoans, e.g., vertebrates and arthropods, the earliest stages of a conventional egg-to-adult developmental trajectory are often strongly divergent (e.g., a bird egg vs. a human zygote), but ontogenies subsequently converge toward a stage (the phylotypic stage; Sander, 1983) at which similarity is greatest, to diverge again toward their increasingly different shapes. This first convergent, then divergent pattern of embryo morphology is known as the developmental hourglass, or egg-timer (Duboule, 1994). This pattern is apparently not restricted to animals. A couple of studies (Quint et al., 2012; Drost et al., 2015) suggest that plant embryogenesis also follows an hourglass pattern of development at the molecular level. Irrespective of the questionable use of the same term “embryo” in both plants and animals, the convergent hourglass pattern suggests the greatest conservation in the middle stages of embryonic development as a general regularity in the evo-devo of multicellulars (Cridge et al., 2019).
Saltational evolution
Contrasting with the generalized acceptance of a view of evolution ruled by continuous insensible change, with major changes only resulting from the accumulation of minor modification over long sequences of generations, a few instances of saltational evolution have been reported, both in the animal and the plant kingdom. Until recently, zoologists have ignored the contributions of botanists to the description and interpretation of saltational patterns of evolution, e.g., Bateman and DiMichele (1994, 2002), see also Vergara-Silva (2003). At last, reciprocal illumination has been advocated to support examples of saltational phenotypic evolution described in plants and animals, respectively, when a zoological example (Chagas-Junior et al., 2008) has been referred to in a botanical article (Theißen, 2009) and botanical examples (Theißen, 2006) in a zoological publication (Minelli et al., 2009).
Functional vs. morphological perspective
In the comparative anatomy of animals, the lack of a clear distinction between body parts defined in strictly morphological terms and organs defined on the basis of functional criteria has been a source of inconsistencies and contradictions. The difficulty of separating the two reading criteria led, for example, to semantic complications in Anton Dohrn’s enunciation of the principle of change of function (Princip des Funktionswechsels; Dohrn, 1875; Minelli, 2021). Problems of the same type have also troubled botany, for example, in the evolutionary interpretation and the naming of the parts of the flower (e.g., Minelli, 2018). For example, in distinguishing between sepals and petals, the usual criteria, e.g., color, number of vascular bundles and developmental timing, cannot be applied universally (Ronse De Craene, 2007; Ronse De Craene and Brockington, 2013). In plants with two whorls of perianth organs, an external whorl of sepals can often be distinguished from an internal whorl of petals, but this is problematic when both whorls are sepaloid or petaloid. This circumstance apparently brings support to Warner et al.’s (2009) “mosaic theory” according to which the association between quality (sepaloid or petaloid features) and position is itself a product of evolution, and a single flower organ can also include left and right sectors with different qualities.
Creative disciplinary integration
Integration between the different strands of the life sciences will hardly emerge by auto-organization. Searching engines such as Google Scholar may help exploring the literature in fields other than the specialist province within which an individual researcher has been accustomed to work, but the logics of the AI guiding the following navigation need at least a motivated, perhaps creative start with the launch of suitable searching strings. Interdisciplinary journals, such as the sample discussed above, may offer opportunity to open our attention onto less familiar topics and, specifically, stimulate a researcher in animal developmental biology or evolutionary developmental biology to look for potentially parallel stories among the plants, and vice versa. More useful, however, is the opportunity offered by webinars and especially by congresses in the program of which talks or sessions covering both plants and animals can be accommodated. But these welcome opportunities will hardly improve the reciprocal illumination that the zoological and botanical traditions would be able to produce, unless a growing number of scientists become more and more accustomed to follow developments in both fields.
This may not be easy. In 1966, the American Society of Zoologists changed its name to Society for Integrative and Comparative Biology; 6 years later, its journal (formerly, American Zoologist) became Integrative and Comparative Biology. This was a deliberate effort to stimulate broader intellectual exchange. However, up to date, this initiative’s success has been quite meager: more than 20 years after the change to the society’s name, “SICB has remained principally a society that supports integrative zoological research” (Ogburn and Edwards, 2019).
The researchers attending a large international congress are likely to be much more inspired by couple of plenary lectures roaming through disparate branches of the tree of life than by a few dozen session of the same congress, part of which strictly focusing on plants, others on animals, not to mention narrower taxonomic partitions. Twenty years since these words were written, Vergara-Silva’s (2003, p. 269; italics as in the original) admonition still maintains its full validity: “not only should animal evo-devo biologists acknowledge discoveries achieved in plants: both camps should also go deeper in their sampling of objects of study, both processes or taxa. There is currently no way to ensure that the developmental-genetic mechanisms known to date, in both animals and plants, are the only possible processes determining the potential for evolution–evolvability–existing in natural populations.”
At last, encouraging signs of a more open attitude are emerging, at least since the foundation (2006) of the European Society for Evolutionary Developmental Biology, followed 9 years later by an American sister, the Pan-American Society for Evolutionary Developmental Biology. At the lively congresses of these two societies, opportunities for mixing experiences between scholars working on plants and animals, respectively, are increasingly frequent, despite the fact that most of the contributed talks are still encased in exclusively zoological or botanical symposia. But in the crammed spaces between long rows of posters, the nearest neighbors of plant evo-devoists stationing close to their work are very often young researchers from zoological labs. Here, exchanges are much easier than in the lecture halls that host the formally structured symposia. These young researchers belong to the same population as those who have attended one or more editions of the Summer School in Evolutionary Developmental Biology held in Venice since 2009. It is from this type of events that a new generation of researchers will hopefully emerge, capable of integrating the best of two academic traditions that have been too separate thus far.
Author contributions
AM: conceptualization, investigation, writing – original draft and review and editing.
Acknowledgments
The author is grateful to Mario Coiro for his invitation to contribute an article to the Research Topic “Reciprocal Illumination and Consilience in Plant Evolutionary-Development,” to Arianna Gualdi for her precious help in mining the bibliometric information summarized in Table 1, and to two reviewers for their constructive criticism of an earlier version.
Conflict of interest
The author declares that the research was conducted in the absence of any commercial or financial relationships that could be construed as a potential conflict of interest.
Publisher’s note
All claims expressed in this article are solely those of the authors and do not necessarily represent those of their affiliated organizations, or those of the publisher, the editors and the reviewers. Any product that may be evaluated in this article, or claim that may be made by its manufacturer, is not guaranteed or endorsed by the publisher.
Footnotes
References
Agassiz, L., and Strickland, H. E. (1848). Bibliographia zoologiae et geologiae. A general catalogue of all books, tracts, and memoirs on zoology and geology. London: Ray Society. doi: 10.5962/bhl.title.56049
Appel, T. A. (1987). The Cuvier-Geoffroy debate: French biology in the decades before Darwin. Oxford: Oxford University Press.
Arber, A. (1941). The interpretation of leaf and root in the angiosperms. Biol. Rev. 16, 81–105. doi: 10.1111/j.1469-185X.1941.tb01096.x
Bailey, S., Percy, D. M., Heifer, C. A., and Cronk, Q. C. B. (2015). The transcriptional landscape of insect galls: Psyllid (Hemiptera) gall formation in Hawaiian Metrosideros polymorpha (Myrtaceae). BMC Genomics 16:943. doi: 10.1186/s12864-015-2109-9
Bateman, R. M., and DiMichele, W. A. (1994). “Saltational evolution of form in vascular plants: A neoGoldschmidtian synthesis,” in Shape and form in plants and fungi, eds D. S. Ingram and A. Hudson (Cambridge, MA: Academic Press), 63–102.
Bateman, R. M., and DiMichele, W. A. (2002). “Generating and filtering major phenotypic novelties: NeoGoldschmidtian saltation revisited,” in Developmental genetics and plant evolution, eds Q. C. B. Cronk, R. M. Bateman, and J. A. Hawkins (London: Taylor & Francis), 109–159. doi: 10.1201/9781420024982.ch7
Bell, A. (2008). Plant form: An illustrated guide to flowering plant morphology. Portland, OR: Timber Press.
Birnbaum, K. D., and Sánchez Alvarado, A. (2008). Slicing across kingdoms: Regeneration in plants and animals. Cell 132, 697–710. doi: 10.1016/j.cell.2008.01.040
Bock, W. J. (1965). The role of adaptive mechanisms in the origin of higher levels of organization. Syst. Zool. 14, 272–287. doi: 10.2307/sysbio/14.4.272
Boerhaave, H. (1727). Historia plantarum, quæ in horto academico Lugduni-Batavorum crescunt cum earum characteribus, & medicinalibus virtutibus. Pars Prima. Romae: Apud Franciscum Gonzagam. doi: 10.5962/bhl.title.109913
Bonner, J. T. (1944). A descriptive study of the development of the slime mold Dictyostelium discoideum. Am. J. Bot. 31, 175–182. doi: 10.1002/j.1537-2197.1944.tb08016.x
Bonner, J. T. (2001). First signals: The evolution of multicellular development. Princeton, NJ: Princeton University Press. doi: 10.1515/9781400830589
Bonner, J. T. (2003). On the origin of differentiation. J. Biosci. 28, 523–528. doi: 10.1007/BF02705126
Boveri, T. (1887). Über die Befruchtung der Eier von Ascaris megalocephala. Sitzber. Ges. Morphol. Physiol. 3, 71–80.
Boyden, A. (1943). Homology and analogy: A century after the definitions of “homologue” and “analogue” of Richard Owen. Q. Rev. Biol. 18, 228–241. doi: 10.1086/394676
Bozdech, Z., Llinas, M., Pulliam, B. L., Wong, E. D., Zhu, J., and DeRisi, J. L. (2003). The transcriptome of the intraerythrocytic developmental cycle of Plasmodium falciparum. PLoS Biol. 1:E5. doi: 10.1371/journal.pbio.0000005
Bracegirdle, B. (1977). The history of histology: A brief survey of sources. Hist. Sci. 15, 77–101. doi: 10.1177/007327537701500201
Brisseau de Mirbel, C. F. (1802). Traité d’anatomie et de physiologie végétale: Suivi de la nomenclature méthodique ou raisonnée des parties extérieures des plantes, et un exposé succinct des systèmes de botanique les plus généralement adoptés: Ouvrage servant d’introduction à l’étude de la botanique. Paris: Dufar.
Budd, G. E. (2001). Why are arthropods segmented? Evol. Dev. 3, 332–342. doi: 10.1046/j.1525-142X.2001.01041.x
Callebaut, W., and Rasskin-Gutman, D. (2005). Modularity: Understanding the development and evolution of natural complex systems. Cambridge, MA: MIT Press. doi: 10.7551/mitpress/4734.001.0001
Caron, J. A. (1988). ‘Biology’ in the life sciences: A historiographical contribution. Hist. Sci. 26, 223–268. doi: 10.1177/007327538802600301
Catalogue of Life (2022). Catalogue of life. Available online at: https://www.catalogueoflife.org/ (accessed September 24, 2022).
Chagas-Junior, A., Edgecombe, G. D., and Minelli, A. (2008). Variability in trunk segmentation in the centipede order Scolopendromorpha: A remarkable new species of Scolopendropsis Brandt (Chilopoda: Scolopendridae) from Brazil. Zootaxa 1888, 36–46. doi: 10.11646/zootaxa.1888.1.2
Claridge, A. W., Mifsud, G., Dawson, J., and Saxon, M. J. (2004). Use of infrared digital cameras to investigate the behaviour of cryptic species. Wildl. Res. 31, 645–650. doi: 10.1071/WR03072
Cockerell, T. D. A. (1920). Biographical memoir of Alpheus Spring Packard 1839-1905. Biogr. Mem. Natl. Acad. Sci. 9, 181–236.
Coelho, S. M., Peters, A. F., Müller, D., and Cock, J. M. (2020). Ectocarpus: An evo-devo model for the brown algae. EvoDevo 11, 1–9. doi: 10.1186/s13227-020-00164-9
Cohn, F. (1852). Ueber eine neue Gattung aus der Familie der Volvocinen. Ztschr. wiss. Zool. 4, 77–116.
Cohn, F. (1875). Die Entwickelungsgeschichte der Gattung Volvox. Breslau: Philosophische Facultät der Königlichen Universität.
Cowdry, E. V. (1924). General cytology. Chicago, IL: The University of Chicago Press. doi: 10.7208/chicago/9780226251257.001.0001
Cridge, A. G., Dearden, P. K., and Brownfield, L. R. (2019). “The developmental hourglass in the evolution of embryogenesis,” in Evolutionary developmental biology, eds L. N. de la Rosa and G. B. Müller (Cham: Springer), 111–120. doi: 10.1007/978-3-319-33038-9_185-1
Darwin, C. (1859). On the origin of species by means of natural selection, or the preservation of favored races in the struggle for life. London: John Murray. doi: 10.5962/bhl.title.82303
Dayel, M. J., Alegado, R. A., Fairclough, S. R., Nichols, S. A., McDonald, K., and King, N. (2011). Cell differentiation and morphogenesis in the colony-forming choanoflagellate Salpingoeca rosetta. Dev. Biol. 357, 73–82. doi: 10.1016/j.ydbio.2011.06.003
Desnitskiy, A. G. (2009). Volvox (Chlorophyta, Volvocales) as a model organism in developmental biology. Russ. J. Dev. Biol. 40, 238–241. doi: 10.1134/S1062360409040079
Desnitskiy, A. G. (2018). Comparative analysis of embryonic inversion in algae of the genus Volvox (Volvocales, Chlorophyta). Russ. J. Dev. Biol. 49, 129–133. doi: 10.1134/S1062360418030025
Diggle, P. K. (1997). Extreme preformation in an alpine Polygonum viviparum: An architectural and developmental analysis. Am. J. Bot. 84, 154–169. doi: 10.2307/2446077
Dobzhansky, T. (1973). Nothing in biology makes sense except in the light of evolution. Am. Biol. Teach. 35, 125–129. doi: 10.2307/4444260
Dohrn, A. (1875). Der Ursprung der Wirbelthiere und das Princip des Funktionswechsels. Leipzig: Engelmann.
Donoghue, M. J., and Kadereit, J. W. (1992). Walter Zimmermann and the growth of phylogenetic theory. Syst. Biol. 41, 74–85. doi: 10.1093/sysbio/41.1.74
Drost, H.-G., Gabel, A., Grosse, I., and Quint, M. (2015). Evidence for active maintenance of phylotranscriptomic hourglass patterns in animal and plant embryogenesis. Mol. Biol. Evol. 32, 1221–1231. doi: 10.1093/molbev/msv012
Duboule, D. (1994). Temporal colinearity and the phylotypic progression: A basis for the stability of a vertebrate Bauplan and the evolution of morphologies through heterochrony. Dev. Suppl. 1994, 135–142. doi: 10.1242/dev.1994.Supplement.135
Ehrenkaufer, G. M., Haque, R., Hackney, J. A., Eichinger, D. J., and Singh, U. (2007). Identification of developmentally regulated genes in Entamoeba histolytica: Insights into mechanisms of stage conversion in a protozoan parasite. Cell Microbiol. 9, 1426–1444. doi: 10.1111/j.1462-5822.2006.00882.x
Erwin, D. H. (2017). The topology of evolutionary novelty and innovation in macroevolution. Phil. Trans. R. Soc. Lond. B Biol. Sci. 372:20160422. doi: 10.1098/rstb.2016.0422
Erwin, D. H. (2021). A conceptual framework of evolutionary novelty and innovation. Biol. Rev. 96, 1–15. doi: 10.1111/brv.12643
Esteve-Altava, B. (2017). Challenges in identifying and interpreting organizational modules in morphology. J. Morphol. 278, 960–974. doi: 10.1002/jmor.20690
Fairclough, S. R., Chen, Z., Kramer, E., Zeng, Q., Young, S., Robertson, H. M., et al. (2013). Premetazoan genome evolution and the regulation of cell differentiation in the choanoflagellate Salpingoeca rosetta. Genome Biol. 14:R15. doi: 10.1186/gb-2013-14-2-r15
Farley, J. (1982). Gametes and spores. Ideas about sexual reproduction 1750–1914. Baltimore, MD: Johns Hopkins University Press.
Farmer, J. B., and Moore, J. E. S. (1905). On the maiotic phase (reduction divisions) in animals and plants. Q. Jour. Microsc. Sci. 48, 489–557. doi: 10.1242/jcs.s2-48.192.489
Fernández-Mazuecos, M., Blanco-Pastor, J. L., Juan, A., Carnicero, P., Forrest, A., Alarcon, M., et al. (2018). Macroevolutionary dynamics of nectar spurs, a key evolutionary innovation. New Phytol. 222, 1122–1138. doi: 10.1111/nph.15654
Flemming, H.-C., Wingender, J., Szewzyk, U., Steinberg, P., Rice, S. A., and Kjelleberg, S. (2016). Biofilms: An emergent form of bacterial life. Nat. Rev. Microbiol. 14, 563–575. doi: 10.1038/nrmicro.2016.94
Flemming, W. (1882). Zellsubstanz, Kern und Zelltheilung. Leipzig: F. C. W. Vogel. doi: 10.5962/bhl.title.168645
Fretter, V. (1969). Aspects of metamorphosis in prosobranch gastropods. Proc. Malacol. Soc. London 38, 375–386.
Fusco, G. (2005). Trunk segment numbers and sequential segmentation in myriapods. Evol. Dev. 7, 608–617. doi: 10.1111/j.1525-142X.2005.05064.x
Fusco, G. (2008). Morphological nomenclature, between patterns and processes: Segments and segmentation as a paradigmatic case. Zootaxa 1950, 96–102. doi: 10.11646/zootaxa.1950.1.9
Fusco, G., and Minelli, A. (2019). The biology of reproduction. Cambridge: Cambridge University Press. doi: 10.1017/9781108758970
Gaertner, J. (1788). De fructibus et seminibus plantarum. Stutgardiæ: Typis Academiae Carolinae. doi: 10.5962/bhl.title.102753
Geoffroy Saint-Hilaire, E. (1830). Principles de philosophie zoologique discutés en mars 1830, au sein de I’academie royale des sciences. Paris: Pichon et Didier. doi: 10.5962/bhl.title.2163
Goethe, J. W. (1790b). “Versuch über die Gestalt der Tiere [Fragment 1790],” in Schriften zur Morphologie, (Stuttgart: J.G. Cotta’sche Buchhandlung Nachfolger), 604–614.
Goethe, J. W. (1790a). Versuch die Metamorphose der Pflanzen zu erklären. Gotha: Ettinger. doi: 10.5962/bhl.title.127448
Goethe, W. (1892). “Paralipomena I, Morphologische Studien in Italien,” in Goethe’s Werke. Abteilung 2. Naturwissenschaftliche Schriften, (Weimar: Hermann Böhlau), 273–288.
Grant, R. E. (1841). Outlines of comparative anatomy. London: Hippolyte Baillière. doi: 10.5962/bhl.title.28330
Green, K. J., and Kirk, D. L. (1981). Cleavage patterns, cell lineages, and development of a cytoplasmic bridge system in Volvox embryos. J. Cell Biol. 91, 743–755. doi: 10.1083/jcb.91.3.743
Green, K. J., Viamontes, G. I., and Kirk, D. L. (1981). Mechanism of formation, ultrastructure, and function of the cytoplasmic bridge system during morphogenesis in Volvox. J. Cell Biol. 91, 756–769. doi: 10.1083/jcb.91.3.756
Gronovius, L. T. (1760a). Bibliotheca regni animalis atque lapidei, seu recensio auctorum et librorum qui de regno animali & lapideo methodice, physice, medice, chymice, philologice, vel theologice tractant, in usum naturalis historiae studiosorum. Leiden, NL: Sumptibus auctoris. doi: 10.5962/bhl.title.39608
Gronovius, L. T. (1760b). Bibliotheca botanica, sive, catalogus auctorum et librorum qui de re botanica, de medicamentis ex vegetabilibus paratis, de re rustica, & de horticultura tractant, a Joanne Francisco Seguierio Nemausense digestus, accessit Bibliotheca botanica Jo. Ant. Bumaldi, seu potius Ovidii Montalbani Bononiensis, nec non auctuarium in Bibliothecam botanicam Cl. Seguierii. Leiden, NL: Cornelius Haak.
Haeckel, E. (1866). Generelle Morphologie der Organismen: Allgemeine Grundzüge der organischen Formen-Wissenschaft, mechanisch begründet durch die von Charles Darwin reformirte Descendenz-Theorie. Berlin: Reimer. doi: 10.5962/bhl.title.3953
Hannibal, R. L., and Patel, N. H. (2013). What is a segment? EvoDevo 4:35. doi: 10.1186/2041-9139-4-35
Heesch, S., Serrano-Serrano, M., Barrera-Redondo, J., Luthringer, R., Peters, A. F., Destombe, C., et al. (2021). Evolution of life cycles and reproductive traits: Insights from the brown algae. J. Evol. Biol. 34, 992–1009. doi: 10.1111/jeb.13880
Heidenhain, M. (1894). Neue Untersuchungen über die Centralkörper und ihre Beziehungen zum Kern- und Zellenprotoplasma. Arch. Mikr. Anat. 43, 423–758. doi: 10.1007/BF02933880
Hendrikse, J. L., Parsons, T. E., and Hallgrímsson, B. (2007). Evolvability as the proper focus of evolutionary developmental biology. Evol. Dev. 9, 393–401. doi: 10.1111/j.1525-142X.2007.00176.x
Henriquez, C. L., Arias, T., Pires, J. C., Croat, T. B., and Schaal, B. A. (2014). Phylogenomics of the plant family Araceae. Mol. Phyl. Evol. 75, 91–102. doi: 10.1016/j.ympev.2014.02.017
Herron, M. D. (2016). Origins of multicellular complexity: Volvox and the volvocine algae. Mol. Ecol. 25, 1213–1223. doi: 10.1111/mec.13551
Hertwig, O. (1876). Beiträge zur Kenntniss der Bildung, Befruchtung und Theilung des thierischen Eies. Morph. Jb. 1, 347–432.
Hertwig, O. (1893). Die Zelle und die Gewebe. Grundzüge der allgemeinen Anatomie und Physiologie. Jena: Fischer. doi: 10.5962/bhl.title.1992
Hodges, S. A. (1997). “Rapid radiation due to a key innovation in columbines (Ranunculaceae: Aquilegia,” in Molecular evolution and adaptive radiation, eds T. J. Givnish and K. J. Sytsma (Cambridge: Cambridge University Press), 391–405.
Höhn, S., Honerkamp-Smith, A. R., Haas, P. A., Khuc Trong, P., and Goldstein, R. E. (2015). Dynamics of a Volvox embryo turning itself inside out. Phys. Rev. Lett. 114:178101. doi: 10.1103/PhysRevLett.114.178101
Honegger, R. (1996). “Morphogenesis,” in Lichen biology, ed. T. H. Nash (Cambridge: Cambridge University Press), 65–87.
Hoßfeld, U., and Olsson, L. (2005). The history of the homology concept and the Phylogenetisches Symposium. Theor. Biosci. 124, 243–253. doi: 10.1007/BF02814486
Huxley, T. H. (1869). On the physical basis of life. Fortn. Rev. 5, 129–145. doi: 10.5962/bhl.title.24707
Huxley, T. H. (1884). The crayfish: An introduction to the study of zoology, 4th Edn. London: Kegan Paul, Trench & Co. doi: 10.5962/bhl.title.152013
Huxley, T. H., and Martin, H. N. (1875). A course of practical instruction in elementary biology. London: Macmillan. doi: 10.5962/bhl.title.57803
Jacobs, D. K., Hughes, N. C., Fitz-Gibbon, S. T., and Winchell, C. J. (2005). Terminal addition, the Cambrian radiation and the Phanerozoic evolution of bilaterian form. Evol. Dev. 7, 498–514. doi: 10.1111/j.1525-142X.2005.05055.x
Jacobs, M. H. (1924). “Permeability of the cell to diffusing substances,” in General cytology, ed. E. V. Cowdry (Chicago, IL: The University of Chicago Press), 97–164.
Jaramillo, M. A., and Kramer, E. M. (2007). The role of developmental genetics in understanding homology and morphological evolution in plants. Int. J. Plant Sci. 168, 61–72. doi: 10.1086/509078
Jardine, N. (1967). The concept of homology in biology. Biol. J. Linn. Soc. 1, 327–361. doi: 10.1111/j.1095-8312.1969.tb00125.x
Jiménez-Guri, E., Okamura, B., and Holland, P. W. H. (2007). Origin and evolution of a myxozoan worm. Integr. Comp. Biol. 47, 752–758. doi: 10.1093/icb/icm026
Kabani, S., Fenn, K., Ross, A., Ivens, A., Smith, T. K., Ghazal, P., et al. (2009). Genome-wide expression profiling of in vivo-derived bloodstream parasite stages and dynamic analysis of mRNA alterations during synchronous differentiation in Trypanosoma brucei. BMC Genomics 10:427. doi: 10.1186/1471-2164-10-427
Kaplan, D. R. (1971). On the value of comparative development in phylogenetic studies – a rejoinder. Phytomorphology 21, 134–140.
Kaplan, D. R. (2001). The science of plant morphology: Definition, history, and role in modern biology. Am. J. Bot. 88, 1711–1741. doi: 10.2307/3558347
Kessin, R. H. (2001). Dictyostelium: Evolution, cell biology, and the development of multicellularity. Cambridge: Cambridge University Press. doi: 10.1017/CBO9780511525315
Khanna, K. R. (1964). Differential evolutionary activity in bryophytes. Evolution 18, 652–670. doi: 10.2307/2406218
Kirk, D. L. (2000). Volvox as a model system for studying the ontogeny and phylogeny of multicellularity and cellular differentiation. J. Plant Growth Regul. 19, 265–274. doi: 10.1007/s003440000039
Kleisner, K. (2007). The formation of the theory of homology in biological sciences. Acta Biotheor. 55, 317–340. doi: 10.1007/s10441-007-9023-8
Klingenberg, C. P. (2005). “Developmental constraints, modules and evolvability,” in Variation: A central concept in biology, eds B. Hallgrímsson and B. K. Hall (Burlington, VT: Elsevier), 219–247. doi: 10.1016/B978-012088777-4/50013-2
Koernicke, M. (1905). Die neueren Arbeiten über die Chromosomenreduktion im Pflanzenreich und daran anschliessende karyokinetische Probleme. Zweiter Bericht. Bot. Ztg 63, 289–307.
Korgaonkar, A., Han, C., Lemire, A. L., Siwanowicz, I., Bennouna, D., Kopec, R. E., et al. (2021). A novel family of secreted insect proteins linked to plant gall development. Curr. Biol. 31, 1836–1849. doi: 10.1016/j.cub.2021.01.104
Lamarck, J. B. (1802). Hydrogéologie, ou Recherches sur l’influence qu’ont les eaux sur la surface du globe terrestre, sur les causes de l’existence du bassin des mers, de son déplacement et de son transport successif sur les différens points de la surface de ce globe, enfin sur les changemens que les corps vivans exercent sur la nature et l’état de cette surface. Paris: Chez l’Auteur.
Lamarck, J. B. (1809). Philosophie zoologique, ou exposition des considérations relatives à l’histoire naturelle des animaux. Paris: Dentu.
Lanfear, R. (2018). Do plants have a segregated germline? PLoS Biol. 16:e2005439. doi: 10.1371/journal.pbio.2005439
Lankester, E. R. (1870). On the use of the term homology in modern zoology, and the distinction between homogenetic and homoplastic agreements. Ann. Mag. Nat. Hist. 6, 34–43. doi: 10.1080/00222937008696201
Lewis, H. (1962). Catastrophic selection as a factor in speciation. Evolution 16, 257–271. doi: 10.1111/j.1558-5646.1962.tb03218.x
Leydig, F. (1857). Lehrbuch der Histologie des Menschen und der Tiere. Frankfurt: Meidinger. doi: 10.5962/bhl.title.22838
Linnæus, C. (1735). Systema naturæ, sive regna tria naturæ systematice proposita per classes, ordines, genera, & species. Lugduni Batavorum: Haak.
Linnæus, C. (1744). Systema naturae in quo proponuntur naturae regna trio secundum classes, ordines, genera & species. Editio quarta. Parisiis: Michaelis-Antonii David. doi: 10.5962/bhl.title.119572
Linnaeus, C. (1756). Plantæ hybridæ quas, […] disquisitioni sistit Joh. J. Haartman. Amoenitates Academicae 3. Holmiæ: Laurentius Salvius, 28–62.
Linnaeus, C. (1758). Systema natura per regna tria naturae secundum classes, ordines, genera, species, cum characteribus, differentiis, synonymis, locis. Editio decima, reformata. Holmiæ: Laurentius Salvius. doi: 10.5962/bhl.title.542
Linnaeus, C. (1766/68). Systema naturae per regna tria naturae, secundum classes, ordines, genera, species, cum characteribus, differentiis, synonymis, locis. Editio duodecima, reformata. Holmiae: Laurentii Salvii.
Linnaeus, C. (1788/1793). Systema naturae per regna tria naturae, secundum classes, ordines, genera, species, cum characteribus, differentiis, synonymis, locis. Lipsiae: Beer.
M’Cosh, J. (1851). Some remarks on the plant morphologically considered. Trans. Bot. Soc. 4, 127–132. doi: 10.1080/03746605309467602
Maienschein, J. (1991). “Cytology in 1924: Expansion and collaboration,” in The expansion of American biology, eds K. R. Benson, J. Maienschein, and R. Rainiger (London: Rutgers University Press), 23–51.
Mani, S., and Tlusty, T. (2021). A topological look into the evolution of developmental programs. Biophys. J. 120, 4193–4201. doi: 10.1016/j.bpj.2021.08.044
Martinson, E. O., Werren, J. H., and Egan, S. P. (2021). Tissue-specific gene expression shows a cynipid wasp repurposes oak host gene networks to create a complex and novel parasite-specific organ. Mol. Ecol. 31, 3228–3240. doi: 10.1111/mec.16159
Mason, H. L. (1957). The concept of the flower and the theory of homology. Madroño 14, 81–95. doi: 10.1016/j.thbio.2005.08.003
Matt, G. Y., and Umen, J. G. (2016). Volvox: A simple algal model for embryogenesis, morphogenesis and cellular differentiation. Plant Dev. 419, 99–113. doi: 10.1016/j.ydbio.2016.07.014
Matt, G. Y., and Umen, J. G. (2018). Cell-type transcriptomes of the multicellular green alga Volvox carteri yield insights into the evolutionary origins of germ and somatic differentiation programs. G3 (Bethesda) 8, 531–550. doi: 10.1534/g3.117.300253
Matthews, K. R. (2005). The developmental cell biology of Trypanosoma brucei. J. Cell Sci. 118, 283–290. doi: 10.1242/jcs.01649
Mayr, E. (1954). “Change of genetic environment and evolution,” in Evolution as process, eds J. Huxley, A. C. Hardy, and E. B. Ford (London: Allen and Unwin), 157–180.
Mayr, E. (1960). “The emergence of evolutionary novelties,” in Evolution after Darwin. I. The evolution of life: Its origin, history, and future, ed. S. Tax (Chicago, IL: The University of Chicago Press), 349–380.
Mayr, E., and Provine, W. B. (1980). The evolutionary synthesis: Perspectives on the unification of biology. Cambridge, MA: Harvard Univ. Press. doi: 10.4159/harvard.9780674865389
Mayr, E., and Vaurie, C. (1948). Evolution in the family Dicruridae (birds). Evolution 2, 238–265. doi: 10.1111/j.1558-5646.1948.tb02744.x
Meyerowitz, E. M. (2002). Plants compared to animals: The broadest comparative study of development. Science 295, 1482–1485. doi: 10.1126/science.1066609
Minelli, A. (2000). Limbs and tail as evolutionarily diverging duplicates of the main body axis. Evol. Dev. 2, 157–165. doi: 10.1046/j.1525-142x.2000.00054.x
Minelli, A. (2003). The development of animal form. Cambridge: Cambridge University Press. doi: 10.1017/CBO9780511541476
Minelli, A. (2015b). “Morphological misfits and the architecture of development,” in Macroevolution. Explanation, interpretation and evidence, eds E. Serrelli and N. Gontier (Cham: Springer), 329–343. doi: 10.1007/978-3-319-15045-1_10
Minelli, A. (2015a). Grand challenges in evolutionary developmental biology. Front. Ecol. Evol. 2:85. doi: 10.3389/fevo.2014.00085
Minelli, A. (2016b). Species diversity vs. morphological disparity in the light of evolutionary developmental biology. Ann. Bot. 117, 781–794. doi: 10.1093/aob/mcv134
Minelli, A. (2016a). Scaffolded biology. Theor. Biosci. 135, 163–173. doi: 10.1007/s12064-016-0230-1
Minelli, A. (2017b). “Evolvability and its evolvability,” in Challenging the modern synthesis: Adaptation, development, and inheritance, eds P. Huneman and D. Walsh (Oxford: Oxford University Press), 211–238. doi: 10.1093/oso/9780199377176.003.0007
Minelli, A. (2017a). Lichens and galls. Two families of chimeras in the space of form. Azafea. Rev. Filos. 19, 91–105.
Minelli, A. (2018). Plant evolutionary developmental biology. Cambridge: Cambridge University Press. doi: 10.1017/9781139542364
Minelli, A. (2020). Not just mammals – epigenetics in a science of all living beings. Paradigmi 38, 495–512.
Minelli, A. (2021). On the nature of organs and organ systems – a chapter in the history and philosophy of biology. Front. Ecol. Evol. 9:745564. doi: 10.3389/fevo.2021.745564
Minelli, A., and Valero-Gracia, A. (2022). Spatially and temporally distributed complexity–a refreshed framework for the study of GRN evolution. Cells 11:1790. doi: 10.3390/cells11111790
Minelli, A., Chagas, A. J., and Edgecombe, G. D. (2009). Saltational evolution of trunk segment number in centipedes. Evol. Dev. 11, 318–322. doi: 10.1111/j.1525-142X.2009.00334.x
Mitchell, S. D. (2006). Modularity: More than a buzzword? Biol. Theor. 1, 98–101. doi: 10.1162/biot.2006.1.1.98
Moczek, A. P. (2008). On the origins of novelty in development and evolution. BioEssays 30, 432–447. doi: 10.1002/bies.20754
Monro, A. K., and Mayo, S. J. (2022). Cryptic species: Morphological stasis, circumscription, and hidden diversity. Cambridge: Cambridge University Press. doi: 10.1017/9781009070553
Morgan, T. H. (1924). “Mendelian heredity in relation to cytology,” in General cytology, ed. E. V. Cowdry (Chicago, IL: The University of Chicago Press), 691–734.
Nabity, P. D. (2016). Insect-induced plant phenotypes: Revealing mechanisms through comparative genomics of galling insects and their hosts. Am. J. Bot. 103, 979–981. doi: 10.3732/ajb.1600111
Nishii, I., and Miller, S. M. (2010). Volvox: Simple steps to developmental complexity? Curr. Opin. Plant Biol. 13, 646–653. doi: 10.1016/j.pbi.2010.10.005
Oakley, T. H. (2017). Furcation and fusion: The phylogenetics of evolutionary novelty. Dev. Biol. 431, 69–76. doi: 10.1016/j.ydbio.2017.09.015
Ogburn, R. M., and Edwards, E. J. (2019). Celebrating a new division of botany at SICB: An introduction to the Integrative Plant Biology Symposium. Integr. Comp. Biol. 59, 489–492. doi: 10.1093/icb/icz114
Omont, N., and Képes, F. (2005). Book review: Modularity in development and evolution. BioEssays 27, 667–668. doi: 10.1002/bies.20243
Owen, R. (1843). Lectures on comparative anatomy and physiology of the invertebrate animals, delivered at the royal college of surgeons in 1843. London: Longman, Brown, Green & Longmans. doi: 10.5962/bhl.title.11742
Owen, R. (1847). Report on the archetype and homologies of the vertebrate skeleton. Report of the sixteenth meeting of the British association for the advancement of science, held at Southampton in september 1846. London: Murray, 169–340. doi: 10.5962/bhl.title.61890
Owen, R. (1848). On the archetype and homologies of the vertebrate skeleton. London: Richard and John Taylor. doi: 10.5962/bhl.title.118611
Pallas, P. S. (1766a). Elenchus zoophytorum, sistens generum adumbrationes generaliores et specierum cognitarum succinctas descriptiones, cum selectis auctorum synonymis. Frankfurt: Varrentrapp. doi: 10.5962/bhl.title.6595
Pallas, P. S. (1766b). Miscellanea zoologica, quibus novæ imprimis atque obscuræ animalium species describuntur et observationibus iconibusque illustrantur. Hague, NL: Petrum van Cleef. doi: 10.5962/bhl.title.69851
Pallas, P. S. (1767/80). Spicilegia zoologica: Quibus novae imprimis et obscurae animalium species iconibus, descriptionibus atque commentariis illustrantur. Berolini: Prostant apud Gottl. doi: 10.5962/bhl.title.39832
Pallas, P. S. (1778). Novae species quadrupedum e glirium ordine cum illustrationibus variis complurium ex hoc ordine animalium. Erlangen: Walther. doi: 10.5962/bhl.title.15686
Pallas, P. S. (1784/88). Flora rossica seu stirpium imperii rossici per europam et asiam indigenarum descriptiones et icones. Petropoli: J. J. Weitbrecht.
Payne, J. L., and Wagner, A. (2019). The causes of evolvability and their evolution. Nat. Rev. Genet. 20, 24–38. doi: 10.1038/s41576-018-0069-z
Peterson, T., and Müller, G. B. (2013). What is evolutionary novelty? Process versus character based definitions. J. Exp. Zool. 320B, 345–350. doi: 10.1002/jez.b.22508
Pinto-Correia, C. (1997). The ovary of Eve. Chicago, IL: The University of Chicago Press. doi: 10.7208/chicago/9780226669502.001.0001
Pradeu, T., Laplane, L., Prévot, K., Hoquet, T., Reynaud, V., Fusco, G., et al. (2016). Defining “development”. Curr. Top. Dev. Biol. 117, 171–183. doi: 10.1016/bs.ctdb.2015.10.012
Pyle, A. (2006). “Malebranche on animal generation. Preexistence and the microscope,” in The problem of animal generation in early modern philosophy, ed. J. E. H. Smith (Cambridge: Cambridge University Press), 194–214. doi: 10.1017/CBO9780511498572.010
Quint, M., Drost, H.-G., Gabel, A., Ullrich, K. K., Markus Bönn, M., and Grosse, I. (2012). A transcriptomic hourglass in plant embryogenesis. Nature 490, 98–101. doi: 10.1038/nature11394
Raper, K. B. (1937). Growth and development of Dictyostelium discoideum with different bacterial associates. J. Agr. Res. 55, 289–316.
Raper, K. B., and Smith, N. R. (1939). The growth of Dictyostelium discoideum upon pathogenic bacteria. J. Bact. 38, 431–444. doi: 10.1128/jb.38.4.431-445.1939
Remane, A. (1952). Die Grundlagen des natürlichen Systems, der vergleichenden Anatomie und der Phylogenetik. Zweite Auflage. Leipzig: Akademische Verlagsgesellschaft Geest und Portig.
Ren, Y., He, Z., Liu, P., Traw, B., Sun, S., Tian, D., et al. (2021). Somatic mutation analysis in Salix suchowensis reveals early-segregated cell lineages. Mol. Biol. Evol. 38, 5292–5308. doi: 10.1093/molbev/msab286
Ronse De Craene, L. P. (2007). Are petals sterile stamens or bracts? The origin and evolution of petals in the core eudicots. Ann. Bot. 100, 621–630. doi: 10.1093/aob/mcm076
Ronse De Craene, L. P., and Brockington, S. F. (2013). Origin and evolution of petals in angiosperms. Plant Ecol. Evol. 146, 5–25. doi: 10.5091/plecevo.2013.738
Rutishauser, R. (1995). Developmental patterns of leaves in Podostemonaceae as compared to more typical flowering plants: Saltational evolution and fuzzy morphology. Can. J. Bot. 73, 1305–1317. doi: 10.1139/b95-142
Rutishauser, R. (1999). Polymerous leaf whorls in vascular plants: Developmental morphology and fuzziness of organ identity. Int. J. Plant Sci. 160, S81–S103. doi: 10.1086/314221
Rutishauser, R., and Isler, B. (2001). Developmental genetics and morphological evolution of flowering plants, especially bladderworts (Utricularia): Fuzzy Arberian morphology complements classical morphology. Ann. Bot. 88, 1173–1202. doi: 10.1006/anbo.2001.1498
Rutishauser, R., Grob, V., and Pfeifer, E. (2008). “Plants are used to having identity crises,” in Evolving pathways. Key themes in evolutionary developmental biology, eds A. Minelli and G. Fusco (Cambridge: Cambridge University Press), 194–213. doi: 10.1017/CBO9780511541582.015
Sander, K. (1983). “The evolution of patterning mechanisms: Gleanings from insect embryogenesis and spermatogenesis,” in Development and evolution: The sixth symposium of the British society for developmental biology, eds B. C. Goodwin, N. Holder, and C. C. Wylie (Cambridge: Cambridge University Press), 137–160.
Sattler, R. (1966). Towards a more adequate approach to comparative morphology. Phytomorphology 16, 417–429.
Sattler, R. (2022). Kaplan’s principles of plant morphology: A critical review. Bot. Rev. 88, 257–270. doi: 10.1007/s12229-022-09280-8
Sattler, R., and Jeune, B. (1992). Multivariate analysis confirms the continuum view of plant form. Ann. Bot. 69, 249–262. doi: 10.1093/oxfordjournals.aob.a088338
Schaap, P. (2011). Evolutionary crossroads in developmental biology: Dictyostelium discoideum. Development 138, 387–396. doi: 10.1242/dev.048934
Schleicher, W. (1878). Die Knorpelzellteilung. Arch. Mikr. Anat. 16, 248–300. doi: 10.1007/BF02956384
Schlichting, C. D., and Murren, C. J. (2004). “Evolvability and the raw materials for adaptation,” in Plant adaptation: Molecular genetics and ecology, eds Q. C. B. Cronk, J. Whitton, R. H. Ree, and I. E. P. Taylor (Ottawa: NRC Research Press), 18–29.
Schlosser, G. (2002). Modularity and the units of evolution. Theor. Biosci. 121, 1–80. doi: 10.1078/1431-7613-00049
Schlosser, G., and Wagner, G. P. (2004). Modularity in development and evolution. Chicago, IL: University of Chicago Press.
Schmitt, R. (2003). Differentiation of germinal and somatic cells in Volvox carteri. Curr. Opin. Microbiol. 6, 608–613. doi: 10.1016/j.mib.2003.10.007
Schultz, J. C., and Stone, G. N. (2022). A tale of two tissues: Probing gene expression in a complex insect-induced gall. Mol. Ecol. 31, 3031–3034. doi: 10.1111/mec.16482
Schwann, T. (1839). Mikroskopische Untersuchungen über die Uebereinstimmung in der Struktur und dem Wachsthum der Thiere und Pflanzen. Berlin: Sander’sche Buchhandlung.
Schwann, T. (1847). Microscopical researches into the accordance in the structure and growth of animals and plants. London: Seydenham Society. doi: 10.5962/bhl.title.11431
Schwartzman, J. A., Ebrahimi, A., Chadwick, G., Sato, Y., Roller, B. R. K., Orphan, V. J., et al. (2022). Bacterial growth in multicellular aggregates leads to the emergence of complex life cycles. Curr. Biol. 32, 3059–3069. doi: 10.1016/j.cub.2022.06.011
Sebé-Pedrós, A., Peña, M. I., Capella-Gutiérrez, S., Antó, M., Gabaldón, T., Ruiz-Trillo, I., et al. (2016). High-throughput proteomics reveals the unicellular roots of animal phosphosignaling and cell differentiation. Dev. Cell 39, 186–197. doi: 10.1016/j.devcel.2016.09.019
Siddall, M. E., Martin, D. S., Bridge, D., Desser, S. S., and Cone, D. K. (1995). The demise of a phylum of protists: Phylogeny of Myxozoa and other parasitic Cnidaria. J. Parasitol. 81, 961–967. doi: 10.2307/3284049
Smocovitis, V. B. (1992). Unifying biology: The evolutionary synthesis and evolutionary biology. J. Hist. Biol. 25, 1–65. doi: 10.1007/BF01947504
Sokoloff, D. D., and Remizowa, M. V. (2021). The use of plant ontologies in comparative and evolutionary studies should be flexible. Am. J. Bot. 108, 909–911. doi: 10.1002/ajb2.1692
Spallanzani, L. (1780). Della generazione di diverse piante, in Id. Dissertazioni di fisica animale e vegetabile, tomo secondo. Modena: Società Tipografica.
Steeves, T. A., and Sussex, I. M. (1989). Patterns in plant development. Cambridge: Cambridge University Press. doi: 10.1017/CBO9780511626227
Strasburger, E. (1884). Die Controversen der indirecten Kerntheilung. Arch. Mikr. Anat. 23, 246–304. doi: 10.1007/BF02952312
Strmecki, L., Greene, D. M., and Pear, C. J. (2005). Developmental decisions in Dictyostelium discoideum. Dev. Biol. 284, 25–36. doi: 10.1016/j.ydbio.2005.05.011
Takeda, S., Yoza, M., Amano, T., Ohshima, I., Hirano, T., Sato, M. H., et al. (2019). Comparative transcriptome analysis of galls from four different host plants suggests the molecular mechanism of gall development. PLoS One 14:e0223686. doi: 10.1371/journal.pone.0223686
Tassy, P. (2011). Trees before and after Darwin. J. Zool. Syst. Evol. Res. 49, 89–101. doi: 10.1111/j.1439-0469.2010.00585.x
Theißen, G. (2006). The proper place of hopeful monsters in evolutionary biology. Theor. Biosci. 124, 349–369. doi: 10.1016/j.thbio.2005.11.002
Theißen, G. (2009). Saltational evolution: Hopeful monsters are here to stay. Theor. Biosci. 128, 43–51. doi: 10.1007/s12064-009-0058-z
Treviranus, G. R. (1802). Biologie oder Philosophie der lebenden Natur für Naturforscher und Aertzte, Erster Band. Göttingen: Röwer. doi: 10.5962/bhl.title.10546
Uittien, H. (1928). Über den Zusammenhang zwischen Blattnervatur und Sprossverzweigung. Recueil Trav. Bot. Néerl. 25, 390–412.
Vaillant, S. (1718). Sermo de structura florum, horum differentia, usuque partium eos constituentium. Leiden, NL: P. van der Aa.
Van Beneden, E. (1883). Recherches sur la maturation de l’oeuf et la fécondation. Ascaris megalocephala. Arch. Biol. 4, 265–640.
Van Speybroeck, L. (2005). Modularity. Understanding the development and evolution of natural complex systems. Cambridge, MA: MIT Press. doi: 10.21825/philosophica.82207
Vergara-Silva, F. (2003). Plants and the conceptual articulation of evolutionary developmental biology. Biol. Philos. 18, 249–284. doi: 10.1023/A:1023936102602
Vervoort, M. (2014). “Comparison of animal and plant development: A right track to establish a theory of development?,” in Towards a theory of development, eds A. Minelli and T. Pradeu (Oxford: Oxford University Press), 203–217. doi: 10.1093/acprof:oso/9780199671427.003.0013
von der Heyde, E. L., and Hallmann, A. (2022). Molecular and cellular dynamics of early embryonic cell divisions in Volvox carteri. Plant Cell 34, 1326–1353. doi: 10.1093/plcell/koac004
von Naegeli, K. (1842). Zur Entwicklungsgeschichte des Pollens bei den Phanerogamen. Zürich: Orell, Füssli.
Wagner, A. (2011). The origins of evolutionary innovations. Oxford: Oxford University Press. doi: 10.1093/acprof:oso/9780199692590.001.0001
Wagner, G. P., Pavlicev, M., and Cheverud, J. M. (2007). The road to modularity. Nat. Rev. Genet. 8, 921–931. doi: 10.1038/nrg2267
Waldeyer, W. (1888). Über Karyokinese und ihre Beziehungen zu den Befruchtungsvorgängen. Arch. Mikrosk. Anat. 32, 1–122. doi: 10.1007/BF02956988
Warner, K. A., Rudall, P. J., and Frohlich, M. W. (2009). Environmental control of sepalness and petalness in perianth organs of waterlilies: A new mosaic theory on the evolutionary origin of a differentiated perianth. J. Exp. Bot. 60, 3559–3574. doi: 10.1093/jxb/erp202
West-Eberhard, M. J. (1998). Evolution in the light of developmental and cell biology, and vice versa. Proc. Natl. Acad. Sci. U.S.A. 95, 8417–8419. doi: 10.1073/pnas.95.15.8417
Wilson, E. B. (1896). The cell in development and inheritance. New York, NY: Macmillan. doi: 10.5962/bhl.title.46211
Wolf, K., and Markiw, M. E. (1984). Biology contravenes taxonomy in the Myxozoa: New discoveries show alternation of invertebrate and vertebrate hosts. Science 225, 1449–1452. doi: 10.1126/science.225.4669.1449
Zammito, J. H. (2018). ). The Gestation of German biology. Philosophy and physiology from Stahl to Schelling. Chicago, IL: The University of Chicago Press. doi: 10.7208/chicago/9780226520827.001.0001
Zanobio, B. (1971). Micrographie illusoire et théories sur la structure de la matière vivante. Clio Med. 6, 25–40.
Keywords: developmental biology journals, Dictyostelium, evolutionary biology journals, history of biology, hourglass model, paramorphism, saltational evolution, Volvox
Citation: Minelli A (2022) Two-way exchanges between animal and plant biology, with focus on evo-devo. Front. Ecol. Evol. 10:1057355. doi: 10.3389/fevo.2022.1057355
Received: 29 September 2022; Accepted: 14 November 2022;
Published: 01 December 2022.
Edited by:
Mario Coiro, University of Vienna, AustriaReviewed by:
Karl J. Niklas, Cornell University, United StatesPaula J. Rudall, Royal Botanic Gardens, Kew, United Kingdom
Copyright © 2022 Minelli. This is an open-access article distributed under the terms of the Creative Commons Attribution License (CC BY). The use, distribution or reproduction in other forums is permitted, provided the original author(s) and the copyright owner(s) are credited and that the original publication in this journal is cited, in accordance with accepted academic practice. No use, distribution or reproduction is permitted which does not comply with these terms.
*Correspondence: Alessandro Minelli, YWxlc3NhbmRyby5taW5lbGxpQHVuaXBkLml0