- 1Center for Adaptable Western Landscapes, Northern Arizona University, Flagstaff, AZ, United States
- 2Conservation Science Partners, Truckee, CA, United States
- 3College of Agriculture and Natural Resources, University of Maryland, College Park, MD, United States
- 4Environment and Society Department, Utah State University, Logan, UT, United States
- 5Point Blue Conservation Science, Petaluma, CA, United States
- 6Department of Ecology and Evolutionary Biology and Kansas Biological Survey, University of Kansas, Lawrence, KS, United States
Introduction: Ecological conditions at a given site are driven by factors including resource availability, habitat connectivity, and disturbance history. Land managers can influence disturbance history at a site by harvesting resources, creating transportation pathways, introducing new species, and altering the frequency and severity of events such as fires and floods. As a result, locations with different land management histories have also likely experienced different disturbance trajectories that, over time, are likely to result in different ecological characteristics.
Methods: To understand how the presence of different management histories may shape ecological conditions across large landscapes, we examined plant and soil characteristics at matched sampling points across jurisdictional boundaries within four Protected Area-Centered Ecosystems (PACEs) in the western US. We employed Bayesian modeling to explore 1) the extent to which specific ecological variables are linked to disturbance and jurisdiction both among and within individual PACEs, and 2) whether disturbance evidence differs among jurisdictions within each PACE.
Results: Across all jurisdictions we found that disturbances were associated with ecologically meaningful shifts in percent cover of bare ground, forbs, grass, shrubs, and trees, as well as in tree species richness, soil stability, and total carbon. However, the magnitude of shifts varied by PACE. Within PACEs, there were also meaningful associations between some ecological variables and jurisdiction type; the most consistent of these were in soil stability and soil carbon:nitrogen ratios. Disturbance evidence within each PACE was relatively similar across jurisdictions, with strong differences detected between contrast jurisdictions only for the Lassen Volcanic National Park PACE (LAVO).
Discussion: These findings suggest an interaction between management history and geography, such that ecotones appear to manifest at jurisdictional boundaries within some, but not all, contexts of disturbance and location. Additionally, we detected numerous differences between PACEs in the size of disturbance effects on ecological variables, suggesting that while the interplay between disturbance and management explored here appears influential, there remains a large amount of unexplained variance in these landscapes. As continued global change elevates the importance of large landscape habitat connectivity, unaligned management activities among neighboring jurisdictions are likely to influence existing ecological conditions and connectivity, conservation planning, and desired outcomes.
1. Introduction
The ecosystem at any given location is driven in part by history of disturbance and stress (e.g., Pierce et al., 2007; Miller et al., 2011). Events and processes that add, reduce, or rearrange resources are key influences on the diversity and function of species assemblages (Powell et al., 2011; Trivellone et al., 2017; Zhang et al., 2019). Human activity shapes these patterns, even in large undeveloped landscapes. Human management type and activity at a given site can affect resources through harvest, restoration, biological invasion, and other processes (Lampert et al., 2014; Goldmann et al., 2015; Innes et al., 2019). Management also affects the types, intensity, and frequency of disturbance, such as through fire suppression, damming and diversion of water, or grazing (Führer, 2000; Alkemade et al., 2013; Schmutz and Moog, 2018). As a result, sites with similar environmental conditions but different management histories may exhibit different biodiversity, function, and adaptive capacity (Bengtsson et al., 2000; Fischer et al., 2006; Levin et al., 2006; Floren et al., 2014; Nicotra et al., 2015; Teague and Barnes, 2017; Huang et al., 2020). Understanding how management may drive such differences among sites is particularly important in light of global change, the emergence of novel ecosystems, and an increasing need for planners and managers to tailor solutions to changing conditions across landscapes (Hobbs et al., 2006).
If management processes shape ecological patterns, over time ecological similarities between jurisdictions may be predicted by similarities in management history (Aslan et al., 2021a). Conversely, initially intact ecosystems that are subjected to unique management histories across jurisdictional boundaries may, over time, diverge to be ecologically distinct even with similar climate, geology, and geography. A primary mechanism driving these management-driven shifts is likely consistent differences between jurisdictions in anthropogenic disturbance. Sites that have been managed primarily for resource extraction such as logging and mining, for example, will exhibit high occurrence of surface disturbance, resource transportation roads, younger forests on average, and possibly active restoration following extraction (DeLong et al., 2004; Zollner et al., 2005; Andrés and Mateos, 2006; Hartmann et al., 2012; Huang et al., 2015; Nelson et al., 2019). Sites managed for recreation, by contrast, may exhibit disturbances clustered in accessible areas such as campgrounds or attractions, passenger car roads and trails, and active restoration such as revegetation projects (Brown et al., 2008; Marzano and Dandy, 2012; Monz et al., 2013; Gutzwiller et al., 2017). Residential sites, including rangelands or forests with subsistence farms, ranches, or private inholdings, may exhibit disturbances that are clustered around built structures, with surrounding undeveloped areas containing trails and further disturbance through off-road vehicle use, harvesting of non-timber forest products, or firewood collection (Maestas et al., 2001; Havlick, 2002; Hansen et al., 2005; Ponstingel, 2020; Gonçalves et al., 2021). Adjacent sites managed for different goals likely provide a key indicator of the potential for management-driven ecological divergence.
Conservation relies on understanding how differing management on the two sides of a boundary may create discontinuities between protected and adjacent areas. These effects may differ across scales. Conservation planning often relies on large, connected landscapes protected from development. In North America, examples include Paseo Pantera: the Path of the Panther (for jaguar movement between Mexico and the United States) and the Yellowstone to Yukon initiative (protecting large mammal migration between Canada and the United States) (Rabinowitz, 2014; Chester, 2015). Smaller, more numerous efforts seek to preserve local habitat connectivity across multi-jurisdictional landscapes through coordinated restorations, watershed, or fire management (e.g., Schultz et al., 2012; Koontz and Newig, 2014; Schultz and Moseley, 2019). Connected landscapes, in turn, allow for dispersal of individuals, gene flow, seasonal migration, recolonization of sites following disturbance, and distributional shifts of populations as a result of climate change (Rudnick et al., 2012; Baguette et al., 2013). However, large landscapes that are divided into multiple distinct management units—as a result of historical events and decisions, distribution of economically-valuable resources, funding allocations, grandfathered practices, and other drivers—are subject to an assortment of internal decisions that result in a patchwork of management practices (Huggard, 2004; Andrew et al., 2012; Aslan et al., 2021a). The result is a management mosaic (sensu Epanchin-Niell et al., 2010) that can manifest as an ecological mosaic.
In undeveloped landscapes, management mosaics likely maintain some commonalities that span jurisdictions—for example, broad vegetation type responds to elevation, latitude, and topography and is unlikely to shift across boundaries in response to management except where disturbance has removed all vegetation such as at a mine or quarry. By contrast, changes in plant composition and vegetation pattern and disturbance evidence may respond at smaller spatial and temporal scales to varying management activities, tracking the management mosaic. This mix of factors and scales likely generates an ecological continuum between adjacent management units (Duinker et al., 2010; Andrew et al., 2012; Wiersma et al., 2015). Within that continuum, our aim was to understand how ecological conditions vary as a function of the jurisdictional mosaic within landscape-scale ecosystems.
We placed our study in the landscapes containing four large national parks in the western US: Sequoia-Kings Canyon National Park (SEKI), Lassen Volcanic National Park (LAVO), Grand Canyon National Park and protected areas encompassed in the same ecosystem along the Colorado River (CORI), and Rocky Mountain National Park (ROMO). We selected these case study ecosystems to inform our ongoing conversations with land managers in each park regarding the influences of cross-boundary management challenges. We used field-based data collection to measure ecological characteristics and disturbance evidence across jurisdictional boundaries and employed a Bayesian framework to analyze and understand the resulting patterns, in order to determine whether jurisdiction is predictive of certain ecological characteristics within these large landscapes. We hypothesized that variation in disturbance management is a likely mechanism driving such relationships, so we also analyzed first the relationship between disturbance and ecological variables, then the relationship between disturbance and jurisdiction, and finally the relationship between jurisdiction and measured ecological variables. We piloted the methods used here in the CORI landscape (Aslan et al., 2021b) and in this study refined analyses and extended them across all four landscapes to enable comparison among different geographies. Our findings thus enable us to discuss possible social-ecological influences on ecological conditions within and among landscapes.
With this study, we aimed to examine differences in ecological variables at a point in time that are reflective of mechanisms that span temporal and spatial scales. Knowing that the vegetation and soils at a sampling location are reflective of broad biogeographical influences, geology, historical events, and days to decades to centuries of species interactions and biological processes, we aimed to investigate whether differences in management can manifest in a consistent way detectable in spite of such broad natural variability.
2. Materials and methods
2.1. Study areas
We collected ecological data across jurisdictional boundaries within four focal Protected Area-Centered Ecosystems (PACEs), which served as case study systems for this work. All four PACEs are located in the western US. ROMO occupies a section of the eastern slope and high elevations of the Rocky Mountains in Colorado, spanning vegetation types including oak grasslands, coniferous forests, and tundra. CORI occurs in northern Arizona, southern Utah, and southeastern Nevada and includes sagebrush desert, oak and pinyon-juniper woodlands, and coniferous forests. SEKI occupies a stretch of ridgeline and both western and eastern slopes of the Sierra Nevada in south-central California, ranging from mesic oak grasslands to tundra to high desert. LAVO occurs in temperate coniferous forest in northern California.
2.2. Framework and hypothesis development
Our field sampling protocols and analyses were guided by an a priori set of hypotheses linking ecological variables to disturbance and disturbance to jurisdiction (Table 1; Figure 1). At our field sites, we examined the frequency of evidence of disturbance in the form of fire, forest management, grazing, and general human presence. We predicted that vegetation structure would respond to these disturbances. Specifically, we hypothesized that disturbances would be associated with diminished tree cover, and, due to increased light penetration, would be associated with increasing cover of bare ground, grasses, forbs, and, over time, shrubs (Goosem, 2007; Shatford et al., 2007; Schwilk et al., 2009; Stephens et al., 2012; Crotteau et al., 2013; Miller et al., 2014). We also hypothesized that disturbances would reduce soil stability and alter soil chemistry (Kutiel and Shaviv, 1989; Manley et al., 1995; Neff et al., 2005; James et al., 2021). Our detailed hypotheses are presented in Table 1.
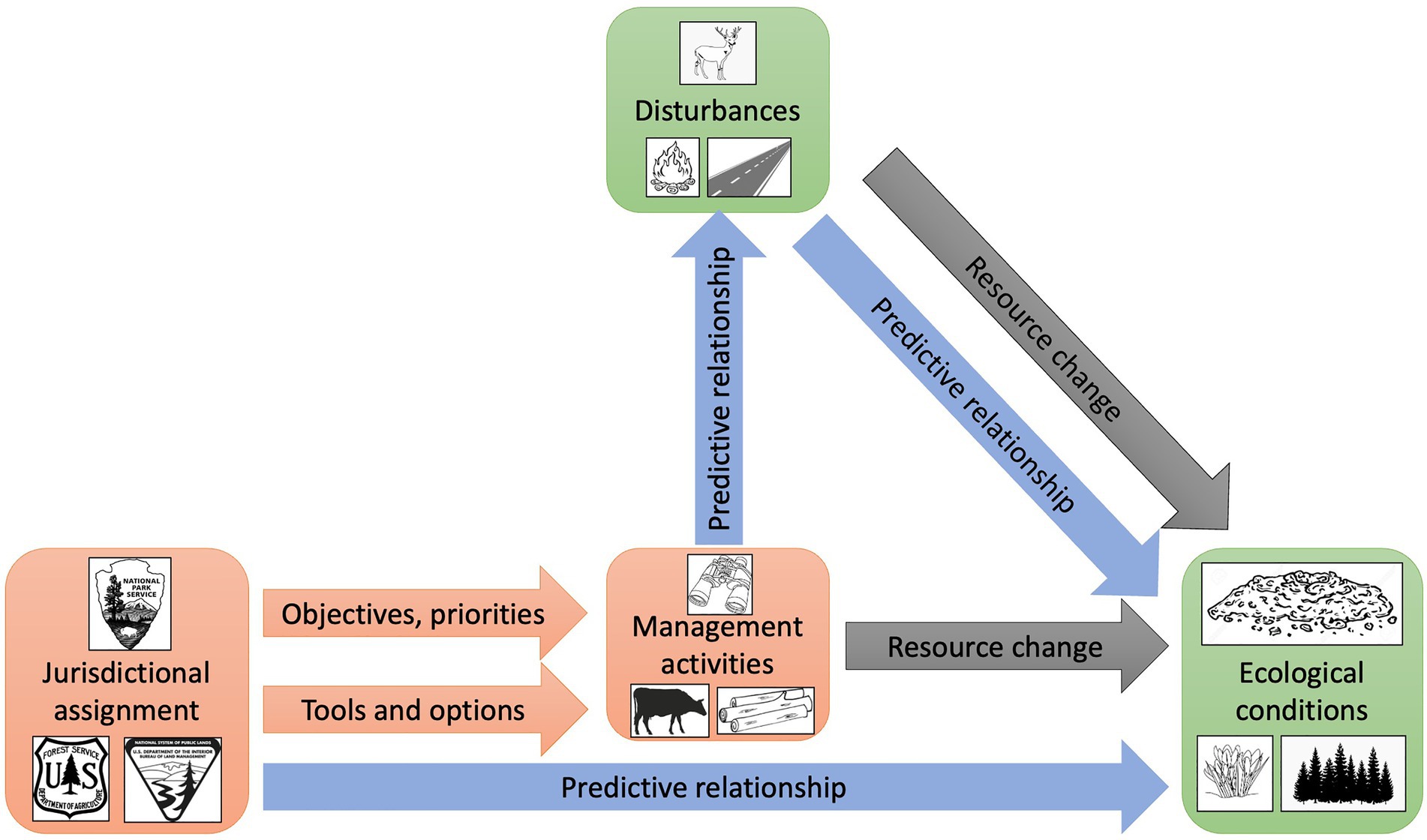
Figure 1. Experimental design and analytical flow. Green boxes = measured empirically during this study. Orange boxes and arrows = inferred from established policy and documents; drivers of hypotheses. Gray arrows = hypothesized mechanisms for relationships. Blue arrows = analyzed relationships.
We hypothesized that disturbances would vary in frequency and severity across different jurisdictional types (National Park Service-NPS, US Forest Service-USFS-Wilderness and Nonwilderness, and Bureau of Land Management-BLM) in our case study landscapes. Wilderness areas in the US are managed to be “untrammeled,” with as little human disturbance as possible (Parsons and Landres, 1996; Zellmer, 2014); as a result, general forest management activities are rare, as are prescribed burns, although natural wildfires may be particularly frequent and extensive. With their dual missions of conservation and recreation, national parks may employ burns and forest management to restore biodiversity or reduce fire hazard, but also aim to support natural processes and patterns, likely resulting in an intermediate level of fire and forest management in such units. National forests are managed to produce the nation’s timber crop and thus most likely to use intensive management techniques. Grazing occurs in all sites if grandfathered into NPS or USFS Wilderness (Pinto, 2014; Squillace, 2014), but is excluded from such units if not practiced prior to their protection. Grazing is a primary land use in BLM and USFS Nonwilderness areas. General human presence is likely to be particularly high in those areas as well, since they are considered multiuse (Havlick, 2002; Koontz and Bodine, 2008; Monz et al., 2013; Theobald, 2013; Payne, 2016). In our analytical methods, described below, we examined the strength of relationships between disturbance variables and ecological responses and identified instances in which ecological responses and disturbance evidence contrasted between jurisdictional units within the focal landscapes.
2.3. Field data collection
In 2017, 2018, and 2019 data were collected from public lands under different jurisdictions in all four PACEs. Data were collected from randomly selected sites near jurisdictional boundaries and, at each site, from clustered points such that two points lay on each side of the boundary and points formed a square with sides of 200 m (Supplementary Figure S1). Distance between points was selected to minimize natural differences in elevation and general vegetation type between the points within each square, in order to hold constant sources of natural variation as much as possible. At each point, researchers established two 50 m-long, 6 m-wide belt transects directed away from the jurisdictional boundary and angled 45 and 135 degrees from the boundary line (Supplementary Figure S1). Disturbance was recorded as present/absent within 1-m intervals along each belt transect. Groundcover was recorded by line-point intercept at 0.5-m intervals along the midline of the two transects. Abundance, size, and species richness of adult and sapling trees were recorded within a 100m2 quadrat established between 20 m and 30 m along each transect and centered on the transect’s 25 m mark. Soil stability was assessed at 5-m intervals along each of the transects, using a field soil slake test kit (Herrick et al., 2001). Soil cores to 20 cm depth were collected from three locations per transect and homogenized to allow later lab-based chemical and physical analysis (after Aslan et al., 2021b).
We aimed to sample 15 sites (90 points) from each of the following contrasts within each PACE: NPS/USFS Wilderness; NPS/USFS Nonwilderness; NPS/BLM; USFS Wilderness/USFS Nonwilderness; USFS Nonwilderness/BLM; and USFS Wilderness/BLM. In practice, not all contrasts occur within all PACEs, and due to access issues, we were not able to reach the full 15 sites for each contrast. Nevertheless, all management types and at least four contrasts were sampled in each PACE and our final set of sampled sites included 28 (112 points; 224 transects) in ROMO (4 contrasts), 50 (200 points; 400 transects) in LAVO (5 contrasts), 51 (204 points; 416 transects) in SEKI (4 contrasts), and 64 (256 points; 512 transects) in CORI (6 contrasts) (Figure 2).
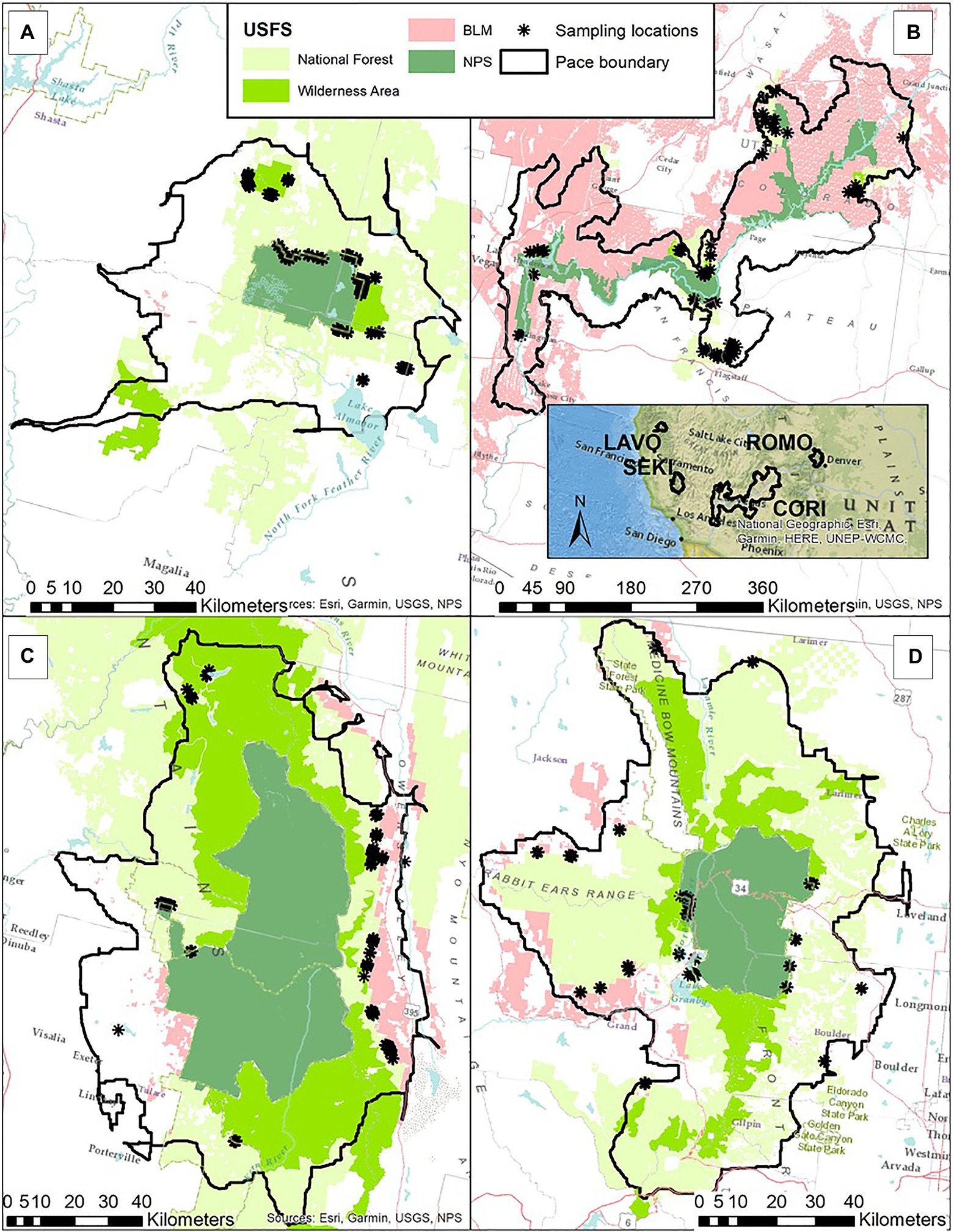
Figure 2. Map showing the four protected area centered ecosystems (PACEs) where we collected field samples; (A) Lassen LAVO, (B) Grand Canyon CORI, (C) Sequoia Kings Canyon SEKI, and (D) Rocky Mountain ROMO. Colors in the map indicate the different jurisdictions we examined; United States Fish and Wildlife Service (USFWS) wilderness and non-wilderness areas, Bureau of Land Management (BLM) and National Park Service (NPS). The solid black line indicates the boundary of each PACE.
2.4. Overall modeling and data analysis
To examine the relationships between disturbance and ecological variables (Table 1), disturbance and jurisdiction, and jurisdiction and ecological variables, we modeled data from sites within each PACE using a general, hierarchical formulation for the posterior and joint distribution of unobserved quantities:
Bracket notation (Gelfand and Smith, 1990), [a | b,c], reads the probability of a conditional on b and c and implies that any distribution appropriate for the support of the random variable yij could be used (Supplementary Table S1). Generality in notation is achieved using the moment matching function h() that returns the parameters of a distribution given its first and second central moments (Hobbs and Hooten, 2015). The subscript i = 1, 2, nj indexes observations within site j; j = 1, 2, J indexes sites within the PACE. The observations come from either of two transects at each of two points in each jurisdiction at a site (Supplementary Figure S1).
Observations were modeled with site-level intercept and, usually, site-specific variance terms. Intercepts for each site, αj, were modeled as a random variable arising from a normal distribution with mean μα and variance : αj ∼ N(μα, ). Site-level variances, , were modeled as a random variable arising from a gamma distribution with parameters matched to moments and : ∼ gamma( / , / ). We also considered models with.
a simple fixed error term, σ2, for observations. β are jurisdictional effects, and γ are other disturbance effects in a generalized linear model (linear, exponential, or logit−1) appropriate for the data, notated by the link function g() (Supplementary Table S1).
The model described above assumes that jurisdictional effects are fixed at the scale of the PACE. Although simple and sensible, such a model does not allow for the possibility that jurisdictional effects are random, and may co-vary with other terms (the intercept, for instance). For example, the influence of jurisdiction in a model of bare ground cover may depend on the proportion of bare ground cover at a site, with more barren sites exhibiting different jurisdictional effects than highly vegetated sites. Thus, we also considered models in which jurisdictional effects were treated as random using random slope terms, βj. In this case, αj and βj are distributed multivariate normal. Their covariance was modeled using the scaled inverse-Wishart distribution with degree of freedom parameter set to L + 1 to induce a uniform prior distribution on parameter correlations (Gelman and Hill, 2006). L is the dimension of the covariance matrix.
The covariate vector xij encodes the jurisdiction within which each sample falls. Specifically, jurisdiction was “effect coded.” Effect coding uses ones, zeros, and minus ones to convey group membership (BLM, NPS, USFS non-wilderness, or USFS wilderness). With k = 1, 2, K groups there are K − 1 effect-coded variables. The Kth effect variable is not needed because the other K − 1 variables contain all of the information needed to determine the group into which an observation falls. With effect coding, the intercept, αj, is equal to the overall mean at site j. It is the grand mean of all observations at site j holding all other covariates (disturbance variables, wij) at their means. The coefficients, β, are equal to the difference between the mean of the jurisdiction and the overall mean at the site (αj). The coefficient for the Kth variable can be computed as a derived quantity using -
The covariates, wij, were chosen to explain spatial variation in the response as a result of disturbance factors, including fire, human disturbance, forest management, and grazing. Disturbance factors varied by transect and were defined as the number of point intercepts at which a given disturbance sign was detected. Such sign included indicators of fire (ash, charring, etc.), human disturbance (trail, chainsaw, trash, etc.), forest management (chainsaw, other cutting, etc.), and grazing (cattle prints, scat, etc.; included only for models of CORI and ROMO). Additional covariates in wij were derived using remotely sensed or gridded data products, including elevation, and typically varied by point within site according to the spatial resolution of the product from which it was came.
The models of disturbances are meant to reveal whether the frequency/intensity of disturbances is more pronounced on specific jurisdictions. Because disturbance factors are the focus here (appearing on the lefthand side of the model equation) we simplified the model in Eq. 1 by removing w′ijγ and removed all references to γ in the expression for the posterior and joint distribution (Eq. 2).
Priors on all parameters were specified to be vague. Priors on model coefficients were normal centered on zero. Variance of these priors was set to assure that dispersion of the prior was much larger than the dispersion of the marginal posterior of the coefficients, except in the case of inverse logit models, where the variance was set to assure a flat distribution of the prediction of proportions (Hobbs and Hooten, 2015). Priors on variances were broad uniform or gamma distributions. Analysis of sensitivity to priors revealed no meaningful effects of priors on marginal posterior distributions of model parameters.
2.5. Model checking and selection
We selected only models that converged using statistics of Brooks and Gelman (1988) and used posterior predictive checks to remove models that were not capable of generating the observed data (Hobbs and Hooten, 2015). We used the minimum posterior predictive loss approach (Gelfand and Ghosh, 1998) to select among the remaining candidate models for each response variable in each PACE.
2.6. Inference
We used the posterior distribution of the coefficients in each model to test our hypotheses about jurisdictional effects and the influence of disturbance factors on each of our ecological variables. Effects were considered positive if estimates of the posterior distributions of their corresponding coefficients had probability density > 0.75 to the right of zero and negative if their coefficients had probability density > 0.75 left of zero.
Contrasts between jurisdictions were computed in the following way. First, at each Markov chain Monte Carlo (MCMC) iteration, m, we make a draw for the intercept term for hypothetical, out-of-sample site from the underlying distribution of intercepts–e.g., ∼ normal (μα, ). In the model invoking random point jurisdiction effects, draws for the intercept and jurisdiction effects were made concurrently using the multivariate normal. In this case, the intercept and jurisdictional effects will co-vary according to the covariance matrix, Σ. Next, we computed the expected value of the response, , for each jurisdiction, holding elevation and all disturbance variables at their respective means. Contrasts are formed by computing the difference, , in the means between two jurisdictions at each MCMC iteration. For instance, . We summarize the differences between jurisdictions using all M samples in the converged output of the MCMC algorithm. We implemented the algorithm in JAGS (Plummer, 2003), using the R programming language (R Core Team, 2017) to fit all models.
3. Results
Our analysis examined, first, whether field-collected evidence of focal disturbance types was associated with measured ecological groundcover, tree, and soil characteristics across all PACEs; second, whether disturbance evidence varied by jurisdiction and where; and third, whether ecological variables varied by jurisdiction and where. In combination, these outputs allowed us to evaluate the contexts in which jurisdiction was predictive of ecological condition across our focal landscapes, and whether these patterns were consistent with our hypotheses surrounding disturbance variability by management type. We considered relationships to have ecological meaning if a disturbance or jurisdiction type was associated with at least a 75% probability of a shift in an ecological or disturbance variable, and if the magnitude of that shift was at least 10% of the range of that variable, excluding the most extreme observations (Table 2; Supplementary Figures S2, S3).
3.1. Relationships between focal disturbance types and ecological variables
Consistent with our hypotheses (Table 1), we found that each focal disturbance type was related to shifts in groundcover type, soil stability, and soil carbon. However, the magnitude of these relationships varied strongly by PACE. Fire evidence was related to increases in bare ground cover in ROMO, LAVO, and SEKI and a decrease in bare ground in CORI; decreases in soil stability in ROMO and LAVO; increased forb cover in SEKI; and increased shrub cover, decreased tree cover, and decreased tree species richness in ROMO (Table 2). Evidence of forest management (e.g., chainsaw scars) was related to decreased bare ground cover in ROMO and SEKI; increased soil stability in ROMO, SEKI, and CORI; decreased grass cover in ROMO; and decreased shrub cover in SEKI (Table 2). Grazing was associated with both increased bare ground cover and forb cover in ROMO and CORI (Table 2). Finally, evidence of human activity in general (e.g., roads, trails, and disturbance from active forest management) was associated with increased cover of bare ground in CORI, ROMO, and LAVO; decreased soil stability in ROMO and LAVO; increased grass cover in SEKI and LAVO; decreased shrub cover in ROMO; and soil carbon in SEKI (Table 2).
3.2. Relationships between focal disturbance type and jurisdiction
Our analysis detected differences in the occurrence of disturbance evidence between jurisdictional contrasts only for forest management overall and only in LAVO (Table 3).
3.3. Relationships between jurisdiction and ecological variables
We compared pairs of adjacent jurisdictions within each PACE for meaningful differences in ecological variables. Two ecological variables, soil stability and soil carbon: nitrogen ratios, differed between jurisdictions in multiple contrast pairs. Soil stability differed between USFS Wilderness and USFS Nonwilderness in LAVO and SEKI, between NPS and USFS Wilderness in ROMO, and between NPS and USFS Nonwilderness in ROMO and LAVO (Table 4). Carbon: nitrogen ratios differed between BLM and USFS Nonwilderness in LAVO and between NPS and both USFS Nonwilderness and USFS Wilderness in LAVO.
4. Discussion
This study detected two scales of ecological patterns (plant community structure and soil properties) that were predicted by the evidence of disturbances and by the site’s jurisdiction. Disturbance was linked to a site’s ecological variables, including groundcover type, soil stability, and soil carbon, although these relationships differed among systems. Jurisdictional relationships with soil stability were clear, with additional but less consistent relationships emerging between jurisdiction and soil carbon: nitrogen ratios, bare ground cover, and tree diameter at breast height. Two take-home messages emerge from our findings: first, relationships between ecological variables and disturbance and between ecological variables and jurisdiction varied by PACE. This finding suggests that context is important, with ecotones manifesting at jurisdictional boundaries in certain environmental settings but not in others. Secondly, soil properties showed the strongest and most consistent patterns, both to jurisdiction type and disturbance. Interestingly, then, the signals of jurisdictional boundaries on ecological properties across large landscapes were strongest at the finest spatial scale examined.
Although all disturbance types were linked to ecological responses, no such link was consistent across all the examined PACEs. The greatest number of meaningful shifts in ecological variables associated with disturbance occurred in ROMO, where effects were detected in cover of bare ground, grass, shrubs, and trees, as well as in soil stability. Spanning the continental divide with a national park established in 1915, the ROMO PACE includes wide variation in temperature and precipitation as well as wide variation in human population density, land use, and recreation impacts (Maestas et al., 2001; Kumar et al., 2009; Hansen et al., 2011). From historic cattle ranches to amenity migrants, a blend of human occupants can be found across the Rocky Mountain region (Riebsame et al., 1996; Gosnell and Travis, 2005; Hansen et al., 2014). Furthermore, with a large metropolitan area nearby and steady growth in visitation (national park records report 4 million visitors per year since 2015), it may be that the ecosystems of the ROMO PACE are particularly subject to a relatively constant diversity of anthropogenic disturbances. On the other end of the spectrum, few links between disturbance and ecological responses were observed in the CORI PACE. Although the park itself (established in 1919) receives the highest visitation of those we examined (the National Park Service reported 6.4 million visitors in 2018), visitation is concentrated into a small area and CORI PACE as a whole has low human population density. The large majority of the landscape has been subject to a century and a half of intensive grazing. Fires and forest management are mainly restricted to the forested portions of the PACE, with current forest management trying to mimic the historic fire regime (Holcomb et al., 2011). As a whole, the PACE is arid within only a few high moisture pockets (on the Kaibab Plateau). Aridity as a common environmental stress may play a homogenizing role in the ecology of all jurisdictions across the PACE. That is, the small number of ecological responses to disturbance and jurisdictions likely reflects a consistent effect of low water availability. Fire and grazing have been a consistent part of the landscape so long and may have interacted with water stress to apply strong selective pressures on vegetation communities, such that grazing-intolerant and fire-intolerant species are no longer common in any jurisdiction on the landscape (Moore et al., 1999; Simpson, 2020). A lack of jurisdictional responses may be consistent in areas where disturbance adaptation is consistent across habitat types, including grasslands and savannas (Bowman et al., 2009).
Pre-existing ecological differences among jurisdictions are an important confounding variable that may obscure the relationships we aimed to examine here. The historical assignment of management units to specific jurisdictions was driven by their characteristics—for example, BLM lands are generally rangelands with high forage incidence, and forested landscapes are generally managed by the USFS. Differences in grass or tree cover, therefore, may have driven the assignment of jurisdiction, rather than the other way around. This study, however, was designed with the expectation that both are true—that regions with certain characteristics are indeed more likely to be assigned to certain jurisdictions, but also that the management differences can reinforce divergence of neighboring parcels, such that ecotones may also be products of management itself. Jurisdictional boundaries, drawn on a map at coarse scale, are unlikely to precisely track natural ecotones such as shifts from forests to woodlands to grasslands. By sampling very close to boundaries, at sites matched by elevation and vegetation type, we aimed to keep sources of natural variation as constant as possible in order to discern any divergence emerging at fine scale and directly at the boundary, and thus possibly as a result of management, if it occurs. Our findings that some ecological characteristics do vary in some cases by jurisdiction, but also by PACE, suggest an interplay between the social construct of jurisdictions, the response time of individual ecological characteristics, and the biophysical and geographical characteristics across and between landscapes.
Importantly, we observed relationships between disturbances and ecological variables at the level of full PACEs, as well as between jurisdictions and ecological variables. Our methods only detected disturbances recent enough to leave visible traces on the landscape – i.e., charred or downed wood, chainsaw cuts, cattle scat and prints, trails and campsites, etc. High incidences of a disturbance may indicate recent disturbance, but a lack of visible disturbance may indicate either no disturbance or a past disturbance that is simply no longer visible. Future research in which investigations such as these are performed in collaboration with environmental historians might enable longer-term or historical drivers of current conditions to be elucidated, perhaps deepening our understanding of ecological heterogeneity across management mosaics. Furthermore, because we were interested in the degree to which administrative boundaries manifested as ecological boundaries, our empirical data collection took place within 100 m of each jurisdictional boundary. Some disturbance types likely track boundaries closely; for example, livestock grazing in a fenced unit is likely to exert maximum impact immediately along and up to the fenceline and to be absent across the boundary. However, some disturbances (e.g., recreation or fire) and management activities (e.g., fuels or invasives management) may be more spatially diffuse and may become more visible at greater distances from those boundaries. Most of the administrative boundaries we observed in our sampling areas are unmarked or are designated only with rare signage or bits of unmaintained fencing, suggesting that management activities and disturbance effects may not respond to sharp barriers but may instead dissipate more diffusely as they near or cross a boundary. Thus, the temporal persistence and spatial heterogeneity of management effects and disturbance evidence vary in ways that may additionally impede detection of the relationships we examined.
Soil properties, and soil stability particularly, showed the most consistent and well-supported relationships to disturbance or jurisdiction. Soil properties can vary over short distances, due to a combination of parent material, vegetation type, and disturbance (Manley et al., 1995; Lamarche et al., 2004; Neff et al., 2005; Hartmann et al., 2012; Verma and Jayakumar, 2012; Pellegrini et al., 2018). As such, soil properties represent ecological variables at the smallest spatial scale we examined for this study. Soil changes, particularly those in response to disturbances, may be relatively long-lived (Neff et al., 2005; Hartmann et al., 2012; Kuske et al., 2012; Pellegrini et al., 2018), such that their “recovery” may well outlast visible evidence of disturbance. Combined, these facets may make soils the most durable ecological indicators of disturbance and jurisdictional differences. Given their foundational role in ecosystems, divergence in soil properties may have indirect effects that affect the resilience of other components over longer time scales. Different soil properties, however, varied in their responsiveness to the factors investigated here. Despite well-known effects of fire, grazing, and forestry on soil chemistry (Kutiel and Shaviv, 1989; Manley et al., 1995; Neff et al., 2005; Verma and Jayakumar, 2012; Pellegrini et al., 2018), total soil C and N were not related to evidence of these disturbances in our PACEs. In contrast, soil stability was linked to both fire and forest management evidence, and often coincided with changes in ground cover. Soil stability is directly reduced by disturbances that remove plant cover (Belnap, 1995; Duchicela et al., 2012; Chandler et al., 2019) so these patterns are almost certainly mechanistically linked and soil stability changes may continue even after vegetation recovers (Duchicela et al., 2012; Pohl et al., 2012). The broader jurisdictional differences in both soil chemistry and stability may reflect either (1) the gap between visible disturbance sign and past management impacts, (2) soil differences that contributed to different land uses and jurisdictional designations, or (3) a combination of both. Ultimately, though, jurisdictional differences in soil stability and soil fertility (C: N ratio) may impact erosion, hydrology, and vegetation representing both livestock forage and fuels for fire. Better understanding these jurisdictional differences in soils can help with conservation planning and predicting ecosystem resilience.
Large landscape conservation is an ongoing challenge in light of global change drivers, which impact large areas and drive rapid shifts in species composition and distribution, biological invasions, and large-scale disturbances such as megafires and floods (Rudnick et al., 2012; Baldwin et al., 2018). However, such landscapes inevitably encompass multiple jurisdictions, requiring planning and predictions that incorporate cross-boundary effects and multijurisdictional decision-making (Locke, 2011; Bixler et al., 2016; Imperial et al., 2016; Scarlett and McKinney, 2016). Understanding how differing management approaches may lead to ecological differences and thus ecotones, and the scale and context of these effects, will be critical for identifying areas of collaboration and prioritization for cross-boundary decision-making. Our work suggests that anthropogenic disturbances are structuring forces across landscapes, but that their legacies may present in unexpected ways and unequally in different regions. As managers and policymakers aim to support resilient landscapes, it will be important to incorporate history, social landscapes, and the interplay of ecological stress and disturbance into truly interdisciplinary planning, going forward.
Data availability statement
The raw data supporting the conclusions of this article will be made available by the authors, without undue reservation.
Author contributions
CA, RE-N, MB, SV, and BS designed the study and received the funding. LZ analyzed the data. CA led the manuscript writing. CA, LZ, RE-N, MB, SV, and BS contributed to manuscript revisions. All authors contributed to the article and approved the submitted version.
Funding
Funding for this work was provided by National Science Foundation Award #1617309.
Acknowledgments
We thank the National Park Service, US Forest Service, and Bureau of Land Management personnel who provided permits, guidance, and feedback in each of the sampled protected area-centered ecosystems. We are deeply grateful for the hard work of our tireless field crewmembers, including: M. Sample, B. Tiffany, S. Sterner, M. Sotelo, G. Conley, M. Barasha, M. Andrews, J. Collier, M. Bannard, R. Lee, S. Lehman, B. Oxford, and W. Topete.
Conflict of interest
The authors declare that the research was conducted in the absence of any commercial or financial relationships that could be construed as a potential conflict of interest.
Publisher’s note
All claims expressed in this article are solely those of the authors and do not necessarily represent those of their affiliated organizations, or those of the publisher, the editors and the reviewers. Any product that may be evaluated in this article, or claim that may be made by its manufacturer, is not guaranteed or endorsed by the publisher.
Supplementary material
The Supplementary material for this article can be found online at: https://www.frontiersin.org/articles/10.3389/fevo.2022.1053548/full#supplementary-material
References
Alkemade, R., Reid, R. S., van Den Berg, M., De Leeuw, J., and Jeuken, M. (2013). Assessing the impacts of livestock production on biodiversity in rangeland ecosystems. Proc. Natl. Acad. Sci. U. S. A. 110, 20900–20905. doi: 10.1073/pnas.1011013108
Andrés, P., and Mateos, E. (2006). Soil mesofaunal responses to post-mining restoration treatments. Appl. Soil Ecol. 33, 67–78. doi: 10.1016/j.apsoil.2005.08.007
Andrew, M. E., Wulder, M. A., and Coops, N. C. (2012). Identification of de facto protected areas in boreal Canada. Biol. Conserv. 146, 97–107. doi: 10.1016/j.biocon.2011.11.029
Aslan, C. E., Brunson, M. W., Sikes, B. A., Epanchin-Niell, R. S., Veloz, S., Theobald, D. M., et al. (2021a). Coupled ecological and management connectivity across administrative boundaries in undeveloped landscapes. Ecosphere 12:e03329. doi: 10.1002/ecs2.3329
Aslan, C. E., Zachmann, L., McClure, M., Sikes, B. A., Veloz, S., Brunson, M. W., et al. (2021b). Quantifying ecological variation across jurisdictional boundaries in a management mosaic landscape. Landsc. Ecol. 36, 1215–1233. doi: 10.1007/s10980-021-01198-7
Augustine, D. J., Booth, D. T., Cox, S. E., and Derner, J. D. (2012). Grazing intensity and spatial heterogeneity in bare soil in a grazing-resistant grassland. Rangel. Ecol. Manag. 65, 39–46. doi: 10.2111/REM-D-11-00005.1
Baguette, M., Blanchet, S., Legrand, D., Stevens, V. M., and Turlure, C. (2013). Individual dispersal, landscape connectivity and ecological networks. Biol. Rev. 88, 310–326. doi: 10.1111/brv.12000
Baldwin, R. F., Trombulak, S. C., Leonard, P. B., Noss, R. F., Hilty, J. A., Possingham, H. P., et al. (2018). The future of landscape conservation. Bio Sci. 68, 60–63. doi: 10.1093/biosci/bix142
Belnap, J. (1995). Surface disturbances: their role in accelerating desertification. Environ. Monit. Assess. 37, 39–57. doi: 10.1007/BF00546879
Bengtsson, J., Nilsson, S. G., Franc, A., and Menozzi, P. (2000). Biodiversity, disturbances, ecosystem function and management of European forests. For. Ecol. Manag. 132, 39–50. doi: 10.1016/S0378-1127(00)00378-9
Best, R. J., and Arcese, P. (2009). Exotic herbivores directly facilitate the exotic grasses they graze: mechanisms for an unexpected positive feedback between invaders. Oecologia 159, 139–150. doi: 10.1007/s00442-008-1172-1
Bixler, R. P., Johnson, S., Emerson, K., Nabatchi, T., Reuling, M., Curtin, C., et al. (2016). Networks and landscapes: a framework for setting goals and evaluating performance at the large landscape scale. Front. Ecol. Environ. 14, 145–153. doi: 10.1002/fee.1250
Bowman, D. M. J. S., Balch, J. K., Artaxo, P., Bond, W. J., Carlson, J. M., Cochrane, M. A., et al. (2009). Fire in the earth system. Science. 324, 481–484. doi: 10.1126/science.1163886
Brooks, S. P., and Gelman, A. (1988). Alternative methods for monitoring convergence of iterative simulations. J. Comput. Graph. Stat. 7, 434–455.
Brown, R. N. K., Rosenberger, R. S., Kline, J. D., Hall, T. E., and Needham, M. D. (2008). Visitor preferences for managing wilderness recreation after wildfire. J. For. 106, 9–16. doi: 10.1093/jof/106.1.9
Chandler, D. G., Day, N., Madsen, M. D., and Belnap, J. (2019). Amendments fail to hasten biocrust recovery or soil stability at a disturbed dryland sandy site. Restor. Ecol. 27, 289–297. doi: 10.1111/rec.12870
Chester, C. C. (2015). Yellowstone to Yukon: Transborder conservation across a vast international landscape. Environ. Sci. Pol. 49, 75–84. doi: 10.1016/j.envsci.2014.08.009
Crotteau, J. S., Morgan Varner, J., and Ritchie, M. W. (2013). Post-fire regeneration across a fire severity gradient in the southern cascades. For. Ecol. Manag. 287, 103–112. doi: 10.1016/j.foreco.2012.09.022
D’Odorico, P., Okin, G. S., and Bestelmeyer, B. T. (2012). A synthetic review of feedbacks and drivers of shrub encroachment in arid grasslands. Ecohydrology 5, 520–530. doi: 10.1002/eco.259
DeLong, S. C., Fall, S. A., and Sutherland, G. D. (2004). Estimating the impacts of harvest distribution on road-building and snag abundance. Can. J. For. Res. 34, 323–331. doi: 10.1139/x03-184
DiTomaso, J. M. (2000). Invasive weeds in rangelands: species, impacts, and management. Weed Sci. 48, 255–265. doi: 10.1614/0043-1745(2000)048[0255,IWIRSI]2.0.CO;2
Duchicela, J., Vogelsang, K. M., Schultz, P. A., Kaonongbua, W., Middleton, E. L., and Bever, J. D. (2012). Non-native plants and soil microbes: potential contributors to the consistent reduction in soil aggregate stability caused by the disturbance of north American grasslands. New Phytol. 196, 212–222. doi: 10.1111/j.1469-8137.2012.04233.x
Duinker, P. N., Wiersma, Y., Haider, W., Hvenegaard, G. T., and Schmiegelow, F. K. (2010). Protected areas and sustainable forest management: what are we talking about? For. Chron. 86, 173–177. doi: 10.5558/tfc86173-2
Epanchin-Niell, R. S., Hufford, M. B., Aslan, C. E., Sexton, J. P., Port, J. D., and Waring, T. M. (2010). Controlling invasive species in complex social landscapes. Front. Ecol. Environ. 8, 210–216. doi: 10.1890/090029
Fischer, J., Lindenmayer, D. B., and Manning, A. D. (2006). Biodiversity, ecosystem function, and resilience: ten guiding principles for commodity production landscapes. Front. Ecol. Environ. 4, 80–86. doi: 10.1890/1540-9295(2006)004[0080:BEFART]2.0.CO;2
Floren, A., Müller, T., Dittrich, M., Weiss, M., and Linsenmair, K. E. (2014). The influence of tree species, stratum and forest management on beetle assemblages responding to deadwood enrichment. For. Ecol. Manag. 323, 57–64. doi: 10.1016/j.foreco.2014.03.028
Führer, E. (2000). Forest functions, ecosystem stability and management. For. Ecol. Manag. 132, 29–38. doi: 10.1016/S0378-1127(00)00377-7
Gelfand, A. E., and Ghosh, S. K. (1998). Model choice: a minimum posterior predictive loss approach. Biometrika 85, 1–11.
Gelfand, A. E., and Smith, A. F. M. (1990). Sampling-based approaches to calculating marginal densities. J. Amer. Stat. Assoc. 85, 398–409.
Gelman, A., and Hill, J. (2006). Data Analysis Using Regression and Multilevel/Hierarchical Models. Cambridge, MA, USA: Cambridge University Press.
Goldmann, K., Schöning, I., Buscot, F., and Wubet, T. (2015). Forest management type influences diversity and community composition of soil fungi across temperate Forest ecosystems. Front. Microbiol. 6:1300. doi: 10.3389/fmicb.2015.01300
Gonçalves, P. H. S., De Medeiros, P. M., and Albuquerque, U. P. (2021). Effects of domestic wood collection on tree community structure in a human-dominated seasonally dry tropical forest. J. Arid Environ. 193:104554. doi: 10.1016/j.jaridenv.2021.104554
Goosem, M. (2007). Fragmentation impacts caused by roads through rainforests. Curr. Sci. 93, 1587–1595.
Gosnell, H., and Travis, W. R. (2005). Ranchland ownership dynamics in the Rocky Mountain west. Rangel. Ecol. Manag. 58, 191–198. doi: 10.2111/1551-5028(2005)58<191:RODITR>2.0.CO;2
Gutzwiller, K. J., D’Antonio, A. L., and Monz, C. A. (2017). Wildland recreation disturbance: broad-scale spatial analysis and management. Front. Ecol. Environ. 15, 517–524. doi: 10.1002/fee.1631
Hansen, A. J., Davis, C. R., Piekielek, N., Gross, J., Theobald, D. M., Goetz, S., et al. (2011). Delineating the ecosystems containing protected areas for monitoring and management. Bioscience 61, 363–373. doi: 10.1525/bio.2011.61.5.5
Hansen, A. J., Knight, R. L., Marzluff, J. M., Powell, S., Brown, K., Gude, P. H., et al. (2005). Effects of exurban development on biodiversity: patterns, mechanisms, and research needs. Ecol. Appl. 15, 1893–1905. doi: 10.1890/05-5221
Hansen, A. J., Piekielek, N., Davis, C., Haas, J., Theobald, D. M., Gross, J. E., et al. (2014). Exposure of U.S. National Parks to land use and climate change 1900–2100. Ecol. Appl. 24, 484–502. doi: 10.1890/13-0905.1
Hartmann, M., Howes, C. G., VanInsberghe, D., Yu, H., Bachar, D., Christen, R., et al. (2012). Significant and persistent impact of timber harvesting on soil microbial communities in Northern coniferous forests. ISME J. 6, 2199–2218. doi: 10.1038/ismej.2012.84
Havlick, D. (2002). No Place Distant: Roads and Motorized Recreation on America’s Public Lands. Chicago: Island Press.
Herrick, J., Whitford, W., Soyza, A., Van Zee, J., Havstad, K., Seybold, C., et al. (2001). Field soil aggregate stability kit for soil quality and rangeland health evaluations. Catena 44, 27–35. doi: 10.1016/S0341-8162(00)00173-9
Hobbs, R. J., Arico, S., Aronson, J., Baron, J. S., Bridgewater, P., Cramer, V. A., et al. (2006). Novel ecosystems: theoretical and management aspects of the new ecological world order. Glob. Ecol. Biogeogr. 15, 1–7. doi: 10.1111/j.1466-822X.2006.00212.x
Hobbs, N. T., and Hooten, M. B. (2015). Bayesian Models: A Statistical Primer for Ecologists. Princeton, NJ, USA: Princeton University Press.
Holcomb, C. M., Sisk, T. D., Dickson, B. D., Sesnie, S. E., and Aumack, E. N. (2011). Administrative Boundaries and Ecological Divergence: The Divided History and Coordinated Future of Land Management on the Kaibab plateau, Arizona, USA. Arizona, USA: University of Arizona press.
Huang, L., Jin, C., Zhen, M., Zhou, L., Qian, S., Jim, C. Y., et al. (2020). Biogeographic and anthropogenic factors shaping the distribution and species assemblage of heritage trees in China. Urban For. Urban Green. 50:126652. doi: 10.1016/j.ufug.2020.126652
Huang, Y., Tian, F., Wang, Y., Wang, M., and Hu, Z. (2015). Effect of coal mining on vegetation disturbance and associated carbon loss. Environ. Earth Sci. 73, 2329–2342. doi: 10.1007/s12665-014-3584-z
Huggard, D. (2004). Establishing representative ecosystems within a managed landscape: an approach to assessment of non-harvestable areas. Report to the sustainable Forest management network, knowledge exchange and technology extension program.
Imperial, M. T., Ospina, S., Johnston, E., O’Leary, R., Thomsen, J., Williams, P., et al. (2016). Understanding leadership in a world of shared problems: advancing network governance in large landscape conservation. Front. Ecol. Environ. 14, 126–134. doi: 10.1002/fee.1248
Innes, J., Fitzgerald, N., Binny, R., Byrom, A., Pech, R., Watts, C., et al. (2019). New Zealand ecosanctuaries: types, attributes and outcomes. J. R. Soc. N. Z. 49, 370–393. doi: 10.1080/03036758.2019.1620297
James, J., Page-Dumroese, D., Busse, M., Palik, B., Zhang, J., Eaton, B., et al. (2021). Effects of forest harvesting and biomass removal on soil carbon and nitrogen: two complementary meta-analyses. For. Ecol. Manag. 485:118935. doi: 10.1016/j.foreco.2021.118935
Koontz, T. M., and Bodine, J. (2008). Implementing ecosystem Management in Public Agencies: lessons from the U.S. Bureau of Land Management and the Forest Service. Conserv. Biol. 22, 60–69. doi: 10.1111/j.1523-1739.2007.00860.x
Koontz, T. M., and Newig, J. (2014). From planning to implementation: top-down and bottom-up approaches for collaborative watershed management. Policy Stud. J. 42, 416–442. doi: 10.1111/psj.12067
Kumar, S., Simonson, S. E., and Stohlgren, T. J. (2009). Effects of spatial heterogeneity on butterfly species richness in Rocky Mountain National Park, CO USA. Biodivers. Conserv. 18, 739–763. doi: 10.1007/s10531-008-9536-8
Kuske, C. R., Yeager, C. M., Johnson, S., Ticknor, L. O., and Belnap, J. (2012). Response and resilience of soil biocrust bacterial communities to chronic physical disturbance in arid shrublands. ISME J. 6, 886–897. doi: 10.1038/ismej.2011.153
Kutiel, P., and Shaviv, A. (1989). Effect of simulated forest fire on the availability of N and P in mediterranean soils. Plant Soil 120, 57–63. doi: 10.1007/BF02370290
Lamarche, J., Bradley, R. L., Paré, D., Légaré, S., and Bergeron, Y. (2004). Soil parent material may control forest floor properties more than stand type or stand age in mixedwood boreal forests. Écoscience 11, 228–237. doi: 10.1080/11956860.2004.11682828
Lampert, A., Hastings, A., Grosholz, E. D., Jardine, S. L., and Sanchirico, J. N. (2014). Optimal approaches for balancing invasive species eradication and endangered species management. Science 344, 1028–1031. doi: 10.1126/science.1250763
Levin, P. S., Holmes, E. E., Piner, K. R., and Harvey, C. J. (2006). Shifts in a Pacific Ocean fish assemblage: the potential influence of exploitation. Conserv. Biol. 20, 1181–1190. doi: 10.1111/j.1523-1739.2006.00400.x
Locke, H. (2011). Transboundary cooperation to achieve wilderness protection and large landscape conservation. Park. Sci. 28, 24–28.
Maestas, J. D., Knight, R. L., and Gilgert, W. C. (2001). Biodiversity and land-use change in the American Mountain west. Geogr. Rev. 91, 509–524. doi: 10.1111/j.1931-0846.2001.tb00238.x
Manley, J. T., Schuman, G. E., Reeder, J. D., and Hart, R. H. (1995). Rangeland soil carbon and nitrogen responses to grazing. J. Soil Water Conserv. 50, 294–298.
Marzano, M., and Dandy, N. (2012). Recreationist behaviour in forests and the disturbance of wildlife. Biodivers. Conserv. 21, 2967–2986. doi: 10.1007/s10531-012-0350-y
Miller, R. F., Ratchford, J., Roundy, B. A., Tausch, R. J., Hulet, A., and Chambers, J. (2014). Response of conifer-encroached Shrublands in the Great Basin to prescribed fire and mechanical treatments. Rangel. Ecol. Manag. 67, 468–481. doi: 10.2111/REM-D-13-00003.1
Miller, A. D., Roxburgh, S. H., and Shea, K. (2011). How frequency and intensity shape diversity–disturbance relationships. Proc. Natl. Acad. Sci. 108, 5643–5648. doi: 10.1073/pnas.1018594108
Monz, C. A., Pickering, C. M., and Hadwen, W. L. (2013). Recent advances in recreation ecology and the implications of different relationships between recreation use and ecological impacts. Front. Ecol. Environ. 11, 441–446. doi: 10.1890/120358
Moore, M. M., Covington, W. W., and Fulé, P. Z. (1999). Reference conditions and ecological restoration: a southwestern ponderosa pine perspective. Ecol. Appl. 9, 1266–1277. doi: 10.1890/1051-0761(1999)009[1266:RCAERA]2.0.CO;2
Neff, J. C., Reynolds, R. L., Belnap, J., and Lamothe, P. (2005). Multi-decadal impacts of grazing on soil physical and biogeochemical properties in Southeast Utah. Ecol. Appl. 15, 87–95. doi: 10.1890/04-0268
Nelson, D. L., Kellner, K. F., and Swihart, R. K. (2019). Rodent population density and survival respond to disturbance induced by timber harvest. J. Mammal. 100, 1253–1262. doi: 10.1093/jmammal/gyz094
Nicotra, A. B., Beever, E. A., Robertson, A. L., Hofmann, G. E., and O’Leary, J. (2015). Assessing the components of adaptive capacity to improve conservation and management efforts under global change. Conserv. Biol. 29, 1268–1278. doi: 10.1111/cobi.12522
Parsons, D. J., and Landres, P. B. (1996). “Restoring natural fire to wilderness: how are we doing?” in Fire in Ecosystem Management: Shifting the Paradigm from Suppression to Prescription. eds. L. P. Theresa and A. B. Leonard (Lawrence, Kansas, USA: Allen Press), 366–373.
Payne, W. A. (2016). Tragedy of the commons revisited grazing, land degradation and desertification on multi-use. Public Lands of Nevada. 沙漠研究 26, 121–128. doi: 10.14976/jals.26.3_121
Pellegrini, A. F. A., Ahlström, A., Hobbie, S. E., Reich, P. B., Nieradzik, L. P., Staver, A. C., et al. (2018). Fire frequency drives decadal changes in soil carbon and nitrogen and ecosystem productivity. Nature 553, 194–198. doi: 10.1038/nature24668
Pierce, S., Luzzaro, A., Caccianiga, M., Ceriani, R. M., and Cerabolini, B. (2007). Disturbance is the principal α-scale filter determining niche differentiation, coexistence and biodiversity in an alpine community. J. Ecol. 95, 698–706. doi: 10.1111/j.1365-2745.2007.01242.x
Pinto, R. L. (2014). Cattle grazing in the national parks: historical development and history of management in three Southern Arizona parks. Available at: https://search.proquest.com/docview/1556771557/abstract/ABC83D75101B4C48PQ/1 (Accessed March 24, 2020).
Plummer, M. (2003). JAGS: a program for analysis of Bayesian graphical models using Gibbs sampling. Work. Pap. 8, 1–10.
Pocock, Z., and Lawrence, R. E. (2005). “How far into a forest does the effect of a road extend? Defining road edge effect in eucalypt forests of South-Eastern Australia.” in Proceedings of the 2005 International Conference on Ecology and Transportation (North Carolina State University, Raleigh, North Carolina, USA: Center for Transportation and the Environment), 397–405.
Pohl, M., Graf, F., Buttler, A., and Rixen, C. (2012). The relationship between plant species richness and soil aggregate stability can depend on disturbance. Plant Soil 355, 87–102. doi: 10.1007/s11104-011-1083-5
Ponstingel, D. (2020). The impact of exurban development on forested areas in Kurgan City, Russia. Land Use Policy 94:104485. doi: 10.1016/j.landusepol.2020.104485
Powell, S., Costa, A. N., Lopes, C. T., and Vasconcelos, H. L. (2011). Canopy connectivity and the availability of diverse nesting resources affect species coexistence in arboreal ants. J. Anim. Ecol. 80, 352–360. doi: 10.1111/j.1365-2656.2010.01779.x
R Core Team (2017). R: A Language and Environment for Statistical Computing. Vienna, Austria: R Foundation for Statistical Computing.
Rabinowitz, A. (2014). “The underground railway of the jaguar,” in An Indomitable Beast. ed. A. Rabinowitz (Berlin: Springer), 133–149.
Riebsame, W. E., Gosnell, H., and Theobald, D. M. (1996). Land use and landscape change in the Colorado Mountains I: theory, scale, and pattern. Mt. Res. Dev. 16, 395–405. doi: 10.2307/3673989
Rudnick, D., Ryan, S., Beier, P., Cushman, S., Dieffenbach, F., Epps, C. W., et al. (2012). The role of landscape connectivity in planning and implementing conservation and restoration priorities. Issues in ecology. undefined. Available at: https://www.semanticscholar.org/paper/The-Role-of-Landscape-Connectivity-in-Planning-and-Rudnick-Ryan/e0ea58e8cbc592d5e0c9c704334b9f2334483355 (Accessed August 9, 2021).
Scarlett, L., and McKinney, M. (2016). Connecting people and places: the emerging role of network governance in large landscape conservation. Front. Ecol. Environ. 14, 116–125. doi: 10.1002/fee.1247
Schmutz, S., and Moog, O. (2018). “Dams: ecological impacts and management,” in Riverine Ecosystem Management. eds. S. Shmutz and J. Sendzimir (Cham: Springer), 111–127.
Schultz, C. A., Jedd, T., and Beam, R. D. (2012). The collaborative Forest landscape restoration program: a history and overview of the first projects. J. For. 110, 381–391. doi: 10.5849/jof.11-082
Schultz, C. A., and Moseley, C. (2019). Collaborations and capacities to transform fire management. Science 366, 38–40. doi: 10.1126/science.aay3727
Schwilk, D. W., Keeley, J. E., Knapp, E. E., McIver, J., Bailey, J. D., Fettig, C. J., et al. (2009). The national fire and fire surrogate study: effects of fuel reduction methods on forest vegetation structure and fuels. Ecol. Appl. 19, 285–304. doi: 10.1890/07-1747.1
Shatford, J. P. A., Hibbs, D. E., and Puettmann, K. J. (2007). Conifer regeneration after Forest fire in the Klamath-Siskiyous: how much, how soon? J. For. 105, 139–146. doi: 10.1093/jof/105.3.139
Simpson, A. C. (2020). Evidence for state change in an arid grassland-steppe: limited recovery of vegetation after intermediate to long-term cattle exclusion in the southwestern U.S. Available at: https://www.proquest.com/docview/2415358997/abstract/5ED27A5099DF4FB1PQ/1 (Accessed February 2, 2022).
Souther, S., Loeser, M., Crews, T. E., and Sisk, T. (2019). Complex response of vegetation to grazing suggests need for coordinated, landscape-level approaches to grazing management. Glob. Ecol. Conserv. 20:e00770. doi: 10.1016/j.gecco.2019.e00770
Stephens, S. L., McIver, J. D., Boerner, R. E. J., Fettig, C. J., Fontaine, J. B., Hartsough, B. R., et al. (2012). The effects of Forest fuel-reduction treatments in the United States. Bioscience 62, 549–560. doi: 10.1525/bio.2012.62.6.6
Taboada, M. A., Rubio, G., and Chaneton, E. J. (2015). “Grazing impacts on soil physical, chemical, and ecological properties in forage production systems,” in Soil Management: Building a Stable Base for Agriculture (New York, John Wiley & Sons, Ltd), 301–320.
Teague, R., and Barnes, M. (2017). Grazing management that regenerates ecosystem function and grazingland livelihoods. Afr. J. Range Forage Sci. 34, 77–86. doi: 10.2989/10220119.2017.1334706
Teague, W. R., Dowhower, S. L., and Waggoner, J. A. (2004). Drought and grazing patch dynamics under different grazing management. J. Arid Environ. 58, 97–117. doi: 10.1016/S0140-1963(03)00122-8
Theobald, D. M. (2013). A general model to quantify ecological integrity for landscape assessments and US application. Landsc. Ecol. 28, 1859–1874. doi: 10.1007/s10980-013-9941-6
Trivellone, V., Bougeard, S., Giavi, S., Krebs, P., Balseiro, D., Dray, S., et al. (2017). Factors shaping community assemblages and species co-occurrence of different trophic levels. Ecol. Evol. 7, 4745–4754. doi: 10.1002/ece3.3061
Verma, S., and Jayakumar, S. (2012). Impact of forest fire on physical, chemical and biological properties of soil: a review. Proc. Int. Acad. Ecol. Environ. Sci. 2:168.
Wiersma, Y. F., Duinker, P. N., Haider, W., Hvenegaard, G. T., and Schmiegelow, F. K. A. (2015). Introduction: relationships between protected areas and sustainable Forest management: where are we heading? Conserv. Soc. 13, 1–12. doi: 10.4103/0972-4923.161206
Zellmer, S. (2014). Wilderness imperatives and untrammeled nature. College of Law Faculty Publications. Available at: https://digitalcommons.unl.edu/lawfacpub/193 (Accessed December 11, 2022).
Zhang, C., Settele, J., Sun, W., Wiemers, M., Zhang, Y., and Schweiger, O. (2019). Resource availability drives trait composition of butterfly assemblages. Oecologia 190, 913–926. doi: 10.1007/s00442-019-04454-5
Zheng, Y., Zhou, G., Zhuang, Q., and Shimizu, H. (2020). Long-term elimination of grazing reverses the effects of shrub encroachment on soil and vegetation on the Ordos plateau. J. Geophys. Res. Biogeosciences 125:e2019JG005439. doi: 10.1029/2019JG005439
Keywords: anthropogenic disturbance, coupled natural-human systems, cross-boundary management, ecological variability, fire, forest management, grazing, groundcover
Citation: Aslan CE, Zachmann L, Epanchin-Niell RS, Brunson MW, Veloz S and Sikes BA (2022) Soil characteristics and bare ground cover differ among jurisdictions and disturbance histories in Western US protected area-centered ecosystems. Front. Ecol. Evol. 10:1053548. doi: 10.3389/fevo.2022.1053548
Edited by:
Luís Borda-de-Água, Centro de Investigacao em Biodiversidade e Recursos Geneticos (CIBIO-InBIO), PortugalReviewed by:
Anvar Sanaei, Leipzig University, GermanyYolanda F. Wiersma, Memorial University of Newfoundland, Canada
Copyright © 2022 Aslan, Zachmann, Epanchin-Niell, Brunson, Veloz and Sikes. This is an open-access article distributed under the terms of the Creative Commons Attribution License (CC BY). The use, distribution or reproduction in other forums is permitted, provided the original author(s) and the copyright owner(s) are credited and that the original publication in this journal is cited, in accordance with accepted academic practice. No use, distribution or reproduction is permitted which does not comply with these terms.
*Correspondence: Clare E. Aslan, Y2xhcmUuYXNsYW5AbmF1LmVkdQ==