- 1College of Life and Environmental Science, Wenzhou University, Wenzhou, Zhejiang, China
- 2College of Life and Environment Sciences, Engineering Research Center of Food Environment and Public Health, Minzu University of China, Beijing, China
- 3Key Laboratory of Animal Ecology and Conservation Biology, Institute of Zoology, Chinese Academy of Sciences, Beijing, China
Introduction: Global warming has led to frequent heat waves, causing global organisms to face severe survival challenges. However, the way in which heat waves threaten the fitness and survival of animals remains largely unclear. Oxidative damage and immunity are widely considered the link between heat waves and threats to animals.
Methods: To evaluate the oxidative damage caused by heat waves and to reveal the physiological resistance to heat waves by the antioxidant defense of animals from different latitudes, we exposed both high-latitude (Zhejiang) and low-latitude (Hainan) populations of Chinese pond turtle (Mauremys reevesii) to simulate heat waves and a moderate thermal environment for 1 week, respectively. Next, we compared the oxidative damage by malondialdehyde (MDA) and antioxidant capacity by superoxide dismutase (SOD), catalase (CAT), glutathione peroxidase (GPx), and total antioxidant capacity (T-AOC) in the liver tissues and evaluated the innate immunity by serum complement protein levels (C3, C4) and lysozyme activity in plasma of turtles.
Results and discussion: We found that heat waves significantly increased the content of MDA and the activity of CAT, whereas it decreased the activity of SOD, T-AOC, and GSH/GSSG in turtles from low latitudes. Furthermore, heat waves increased CAT activity but decreased GSH/GSSG in turtles from high latitudes. Although the turtles from high latitudes had higher levels of innate immunity, the heat waves did not affect the innate immunity of C3, C4, or lysozyme in either population. These results indicate that the low-latitude population suffered higher oxidative damage with lower antioxidant capacities. Therefore, we predict that Chinese pond turtles from low latitudes may be more vulnerable to heat waves caused by climate warming. This study reveals the physiological and biochemical resistance to heat waves in Chinese pond turtles from different latitudes and highlights the importance of integrative determination of fitness-related responses in evaluating the vulnerability of ectotherms from different latitudes to climate warming.
Introduction
A serious consequence of climate warming is the occurrence of more frequent and extreme heat waves. In recent years, the warmest summers and continuous heat events have been recorded, even in polar areas (Hansen et al., 2012; Witze, 2022). Therefore, understanding the effects of heat waves on species has been more critical and urgent than ever (Stillman, 2019). Heat events (e.g., summer heat waves) make summer a terrible season for animals, during which they face the challenges of stressful heat (Burggren, 2018; Vinagre et al., 2018). Both terrestrial and marine ecosystems experience biodiversity loss due to heat waves (Rogers-Bennett and Catton, 2019). Heat waves can have disastrous effects on animals, including direct mortality, depressing population growth, and declining individual fitness (see details in Stillman, 2019). For example, mortality has been widely observed in individuals or populations that are unable to escape or resist heat waves (Morignat et al., 2018). Some birds cannot maintain their body temperatures under heat waves by cool evaporating, and thus die of dehydration (McKechnie and Wolf, 2010; Xie et al., 2017). Furthermore, heat waves are associated with functional decline and infectious disease outbreaks in some species, leading to large-scale population collapse (Harvell et al., 2019; Rogers-Bennett and Catton, 2019). For instance, the innate immunity of common musk turtles (Sternotherus odoratus) is modified by heat events, thus modulating their resistance to heat waves (Goessling et al., 2019). Extremely high air temperatures can also cause a decline in cognitive and locomotor performance and decreased fitness (Danner et al., 2021). However, the mechanism by which animals resist heat waves is largely unclear (Stillman, 2019; Logan and Cox, 2020).
The antioxidant defense may be the most important physiological adjustment when animals are exposed to heat waves (Troschinski et al., 2014; Baker et al., 2020). Extremely high temperatures would seriously increase the metabolic rates and synchronously produce reactive oxygen species (ROS; Ben Ameur et al., 2012), which would react with the molecular components of cells, such as lipids, proteins, and nucleic acids, leading to oxidative damage in cell machinery, indicated by the content of malondialdehyde (MDA), carbonylated protein, and DNA injury (Li et al., 2021). The accumulation of oxidative damage then induces the collapse of functions such as immunity, and even leads to mortality of the individuals; hence, depressed immunity can serve as a proxy for oxidative damage. For instance, a simulated heat wave induced oxidative damage in the corn snake (Pantherophis guttatus) and depressed its innate immunity (Stahlschmidt et al., 2017). Heat waves also decrease body mass, lysozyme activity, and individual fitness in tropical butterflies (Bicyclus anynana; Fischer et al., 2014). In animals, the antioxidant defense can eliminate ROS production through a series of reactions catalyzed by antioxidant enzymes, including superoxide dismutase (SOD), catalase (CAT), and glutathione peroxidase (GPx; Munro and Treberg, 2017). In summary, heat waves induce oxidative damage (e.g., MDA) and affect immunity; however, individuals can enhance their antioxidant defense to reduce the potential threat of heat waves (Speakman and Garratt, 2014). For example, our previous study demonstrated that when yellow pond turtles (Mauremys mutica) faced heat waves, enhanced GPx facilitated antioxidant defense and plausibly reduced the production of MDA (i.e., oxidative damage), thereby sustaining innate immunity, including increased lysozyme activity and serum complement C4 levels (Li et al., 2021).
Although the latitudinal vulnerability of animals to heat waves under climate warming is largely unclear, heat waves are predicted to increase toward low latitudes (IPCC, 2021), indicating that species from these areas would face more challenges. Nonetheless, heat tolerance increases toward low latitudes (i.e., tropical areas) in insects, fish, turtles, and birds (Sunday et al., 2011, 2012). The latitudinal vulnerability of animals to heat waves is determined by the interaction between heat waves and how local animals physiologically resist them (Hall and Sun, 2021). Therefore, revealing the physiological resistance to heat waves across latitudes is critical for understanding the thermal adaptation to environments and for evaluating the vulnerability of animals to climate warming geographically (Carlo et al., 2018; Dahlke et al., 2020; Li et al., 2021).
As ectotherms, the physiological functions and fitness of turtles are significantly affected by their thermal environment (Ackerman, 1981; Attaway et al., 1998; Binckley et al., 1998; Ashmore and Janzen, 2003; Bostrom et al., 2010). Turtles are becoming increasingly vulnerable to ongoing climate warming (Taylor et al., 2020). The Chinese three-keeled pond turtle (Mauremys reevesii) is an endangered and unique species that is widespread in mainland China (IUCN, 2022). The species biology has been widely investigated, including life-history cycle, sex determination, and thermal biological traits (Du et al., 2009; Ye et al., 2009; Zhang et al., 2019; Wu et al., 2022), making it an appropriate system to study physiological resistance to heat waves.
In this study, we used M. reevesii from low latitudes (Hainan) and high latitudes (Zhejiang) as the study system. We first exposed the two populations of turtles to a simulated heat wave and then determined oxidative damage with MDA; anti-oxidant ability with the activity of SOD, CAT, GPx; and total antioxidant capacity (T-AOC) in the liver. We also evaluated the functional damage by determining the innate immunity of serum complement protein levels (C3, C4) and lysozyme activity in plasma. By comparing the traits of each population between the control and heat treatments, we aimed to reveal the effects of heat waves on the antioxidant responses and innate immune function of M. reevesii across latitudes. Based on the heat tolerance pattern in turtles across latitudes (Sunday et al., 2011), we predicted that heat waves would induce oxidative stress and depress immune function in M. reevesii at high latitudes rather than at low latitudes.
Materials and methods
Ethics statement
We experimented with animals under the supervision of the Animal Ethical and Welfare Committee of Wenzhou University (Approval No. WZU-049).
Experimental design
We used 16 yearling turtles for each population, which were purchased from the turtle farm in Jiaxing, Zhejiang Province (120°53′E, 30°46′N) for the high-latitude population and from Haikou, Hainan province (110°19′E, 20°2′N) for the low-latitude population in 2019. The phylogenetic relationship of these two populations with CytB indicated that they were from one species without differentiation (Supplementary Figure S1). The turtles were transferred to the laboratory at Wenzhou University by train in 2 days, where they were reared in individual terraria (length × width × height = 45 × 35 × 10 cm) in temperature-controlled incubators (KB400, Binder GmbH, Tuttlingen, Germany). The temperature was modified daily, mimicking the field thermal environment of July and August in in Jiaxing and Haikou (28 ± 4°C, Supplementary Figure S2; China Meteorological Data Service Center, http://data.cma.cn). After 1 week, we used a 2 × 2 experimental design to acclimate the turtles: population origin (high latitude vs. low latitude) × thermal treatment (control vs. heat waves). Accordingly, turtles from each population were randomly and evenly assigned to two treatments: control and heat wave. Animals under the control treatment were reared at 28 ± 4°C continuously, and their counterparts under the heat wave treatment were reared under a fluctuating thermal regime of 35 ± 4°C, which mimicked the heat waves of Jiaxing in July and August from 2011 to 2017 (China Meteorological Data Service Center, http://data.cma.cn; Figure 1). Accordingly, we acclimated eight turtles to each treatment for each population. During acclimation, we provided commercial food to the turtles daily. The light cycle inside the incubator was set at 14 h light:10 h dark (6 a.m.–8 p.m.) using fluorescent lamps. The water was changed daily when the light was turned off.
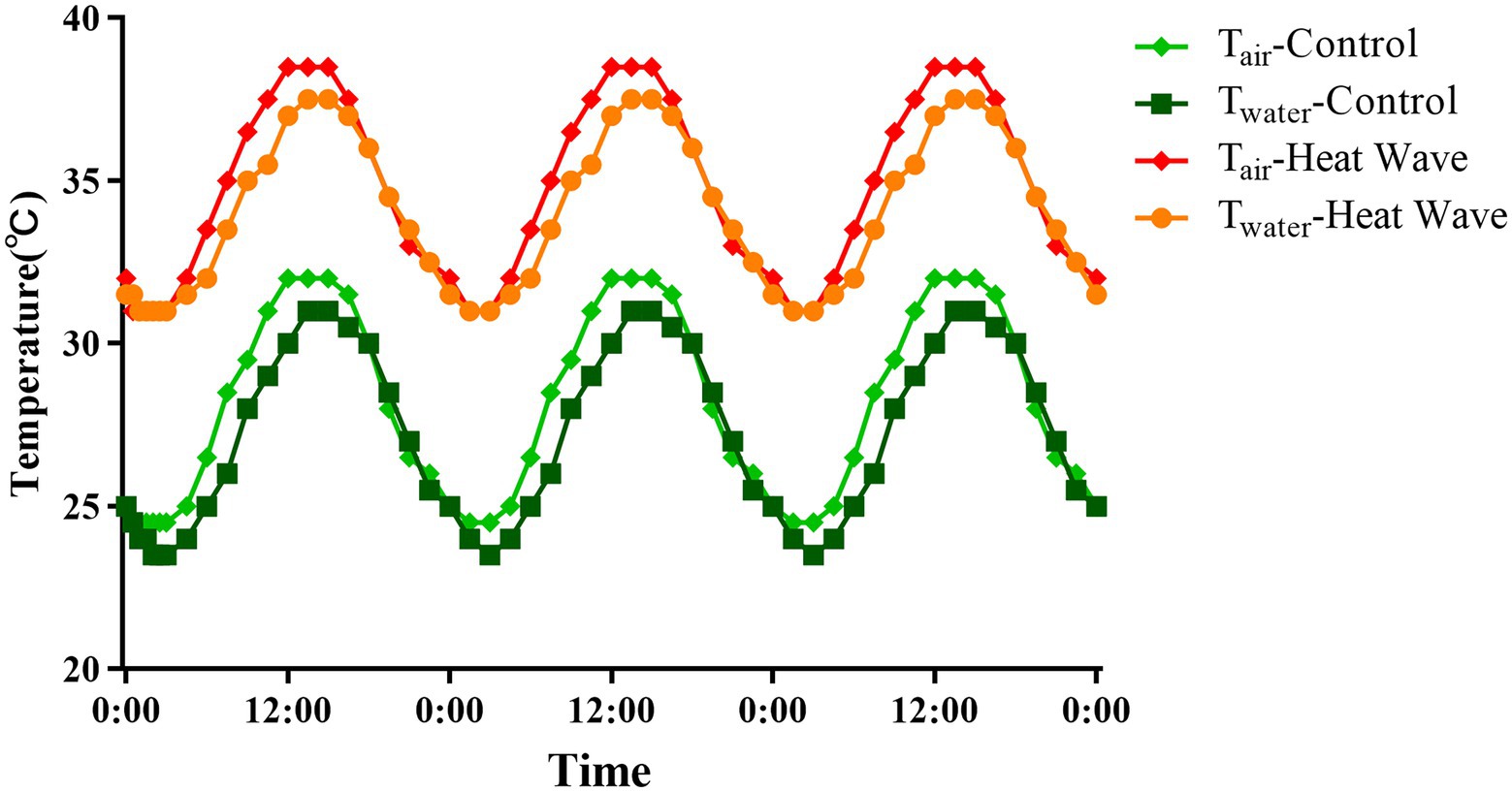
Figure 1. Thermal treatments for turtles under control and heat waves, respectively. The red and light green lines indicate the air temperatures under heat waves and control treatments, while orange and dark green lines indicate the water temperatures under heat waves and control treatments, respectively.
Oxidative damage, antioxidant defense, and immunity indices
After 1 week of acclimation, the turtles were used to determine oxidative damage, antioxidant ability, and innate immunity. Following an established method (Li et al., 2021), we sampled the liver tissues for oxidative damage and antioxidant ability determination, and the blood for innate immunity determination. Eight turtles from each population in each treatment were used in this study. In brief, the turtles were first sacrificed by decapitation, followed by pithing using heavy shears according to the AVMA Guidelines for the Euthanasia of Animals (2013 Edition). Then, we collected the liver tissue on ice. Blood-free livers were weighed immediately (±0.0001 g) and frozen in liquid nitrogen. Blood was collected from the carotid artery and centrifuged at 3,000 rpm for 15 min (Fresco 21, Thermo Fisher Scientific, Waltham, MA, United States) to obtain serum and plasma.
Following the protocols of commercial kits (Nanjing Jiancheng Bioengineering Institute, Nanjing, China) and our previously established methods, we determined the MDA content and the activity of the antioxidant enzymes SOD, CAT, GPx, and T-AOC in the liver tissue (Li et al., 2021). In brief, the MDA content was determined by measuring the absorbance of the compound produced by MDA and thiobarbituric acid at 532 nm. SOD activity was then determined at 550 nm in the xanthine-xanthine oxidase system, and the rate of cytochrome c reduction by superoxide ions was monitored and calculated. CAT activity was estimated at 405 nm by monitoring the consumption of H2O2. The GSH consumption rate at 412 nm was used to determine GPx. Total antioxidant capacity (T-AOC) was measured based on the absorbance of the Fe2+-o-phenanthroline complex at 520 nm.
Similar to oxidative damage and antioxidant defense, we followed established methods and enzyme-linked immunosorbent assay (ELISA) kits (Shanghai MLBIO Biotechnology Co., LTD, Shanghai, China) to determine the concentrations of serum C3, C4, and plasma lysozyme, as part of innate immunity (Li et al., 2021). Samples were incubated with anti-fish horseradish peroxidase-linked antibodies and after thorough washing, and color was developed using 3,3′,5,5′-tetramethylbenzidine. Sulfuric acid was added to terminate the reaction and absorbance was measured at 450 nm.
Statistical analysis
Data were analyzed using Statistica 6.0. Before the analysis, the residuals of the variance were checked for normality and homogeneity using Shapiro–Wilk and Levene’s tests, respectively. Data were normalized by exponential or Ln-transformation if necessary. Two-way ANOVAs were used to test the differences between treatments and populations in MDA, SOD, CAT, GPx, T-AOC, C3, C4, and lysozyme levels, followed by the post hoc Tukey’s HSD test. Data were expressed as the mean ± SE. The significance level was set at α = 0.05.
Results
Oxidative damage and antioxidant defense
The MDA content was significantly higher in the turtles from low latitudes than in those from high latitudes (F1, 28 = 32.307, p < 0.001), and was higher in animals exposed to heat waves than in the controls (F1, 28 = 25.541, p = 0.002). However, there was no interaction in determining the MDA content between the population origin and thermal treatments (F1, 28 = 2.728, p = 0.110; Figure 2A).
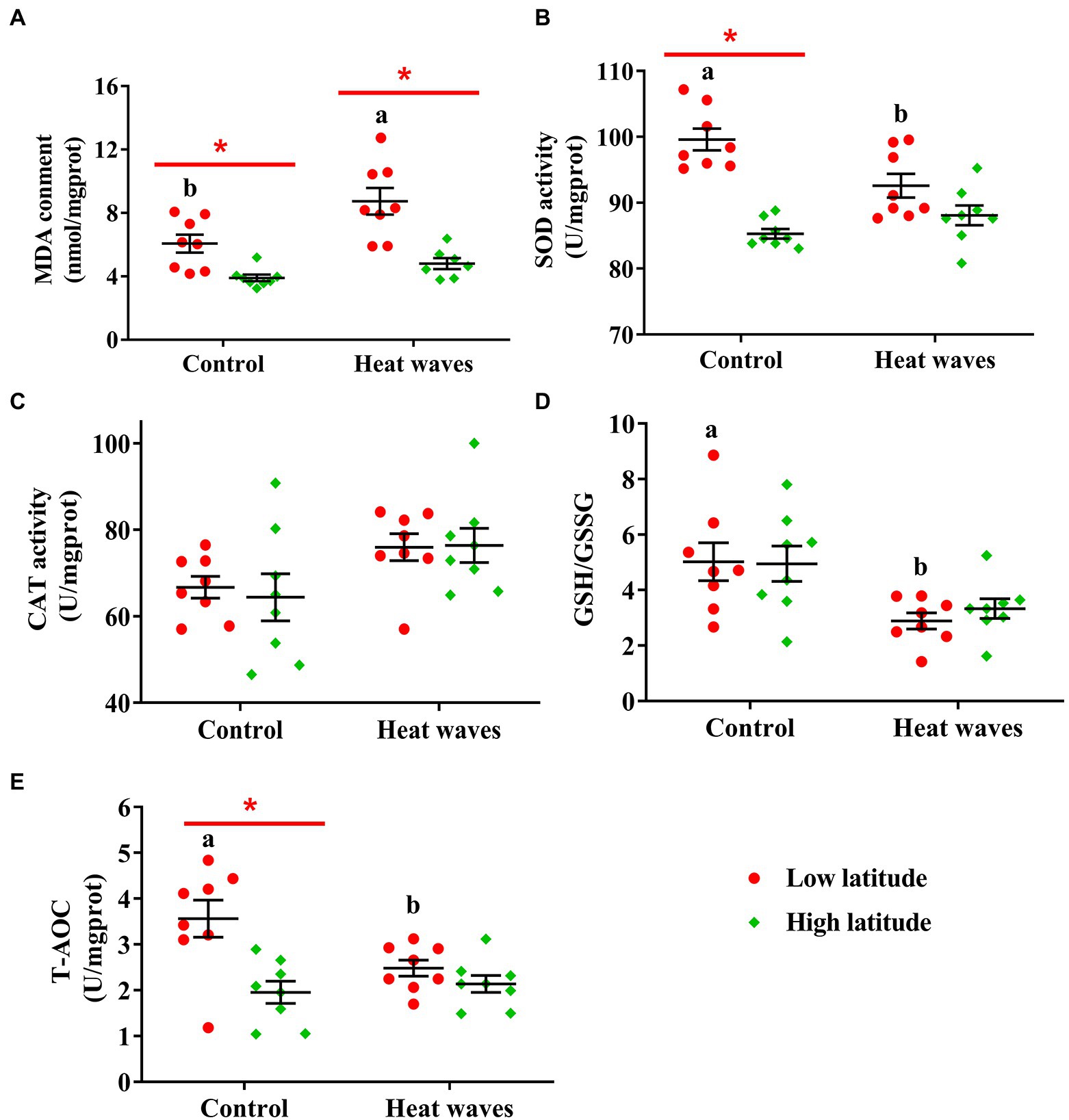
Figure 2. The content of MDA (A), and the activity of SOD (B), CAT (C), GPx (D), and T-AOC (E) in liver tissues of the turtles from low and high latitudes. The red spots and green diamonds indicate the turtles from low and high latitudes, respectively. Each dot indicates an individual, and the error bars indicate the mean ± SE. Red asterisks indicate significant differences between latitudes under the same treatment. Lowercases indicate significant differences between treatments in turtles from low latitude.
The activity of SOD was affected by population origin (F1, 28 = 40.330, p < 0.001) and the interaction between population origin and thermal treatment (F1, 28 = 10.960, p = 0.003). Thermal treatment did not affect the SOD activity (F1, 28 = 2.010, p = 0.168). The turtles from low latitudes had higher SOD activity than those from high latitudes, mainly induced by the significantly higher activity of SOD in turtles under the control treatment. However, SOD activity in turtles from different populations did not differ under the heat wave treatment (Figure 2B).
CAT activity was enhanced by heat waves in both populations (F1, 28 = 7.354, p = 0.011). However, neither population origin (F1, 28 = 0.058, p = 0.812) nor the interaction between population origin and thermal treatment (F1, 28 = 0.121, p = 0.730) affected CAT activity (Figure 2C). Similarly, neither population origin (F1, 28 = 0.127, p = 0.724) nor the interaction between population and treatment (F1, 28 = 0.244, p = 0.625) affected the GSH/GSSG; however, the turtles from both populations had lower GSH/GSSG if they were under heat wave treatment (F1, 28 = 12.953, p = 0.001; Figure 2D). The total antioxidant capacity (T-AOC) was affected by population origin (F1, 28 = 13.305, p = 0.001) and the interaction between population origin and thermal treatment (F1, 28 = 5.555, p = 0.026). However, heat waves did not affect the T-AOC (F1, 28 = 2.791, p = 0.106). Turtles from low latitudes had higher T-AOC than those from high latitudes, mainly induced by significantly higher T-AOC under the control treatment. Interestingly, the T-AOC of the two populations did not differ under the heat-wave treatment (Figure 2E).
Innate immunity responses
Levels of C3 (F1, 28 = 13.683, p < 0.001) and C4 (F1, 28 = 5.324, p = 0.029) were significantly higher in turtles from high latitudes than in turtles from low latitudes. However, neither the thermal treatment (C3: F1, 28 = 0.260, p = 0.614; C4: F1, 28 = 1.443, p = 0.240) nor the interaction between population origin and thermal treatment (C3: F1, 28 = 2.354, p = 0.136; C4: F1, 28 = 4.008, p = 0.056) affected the C3 or C4 levels (Figures 3A,B).
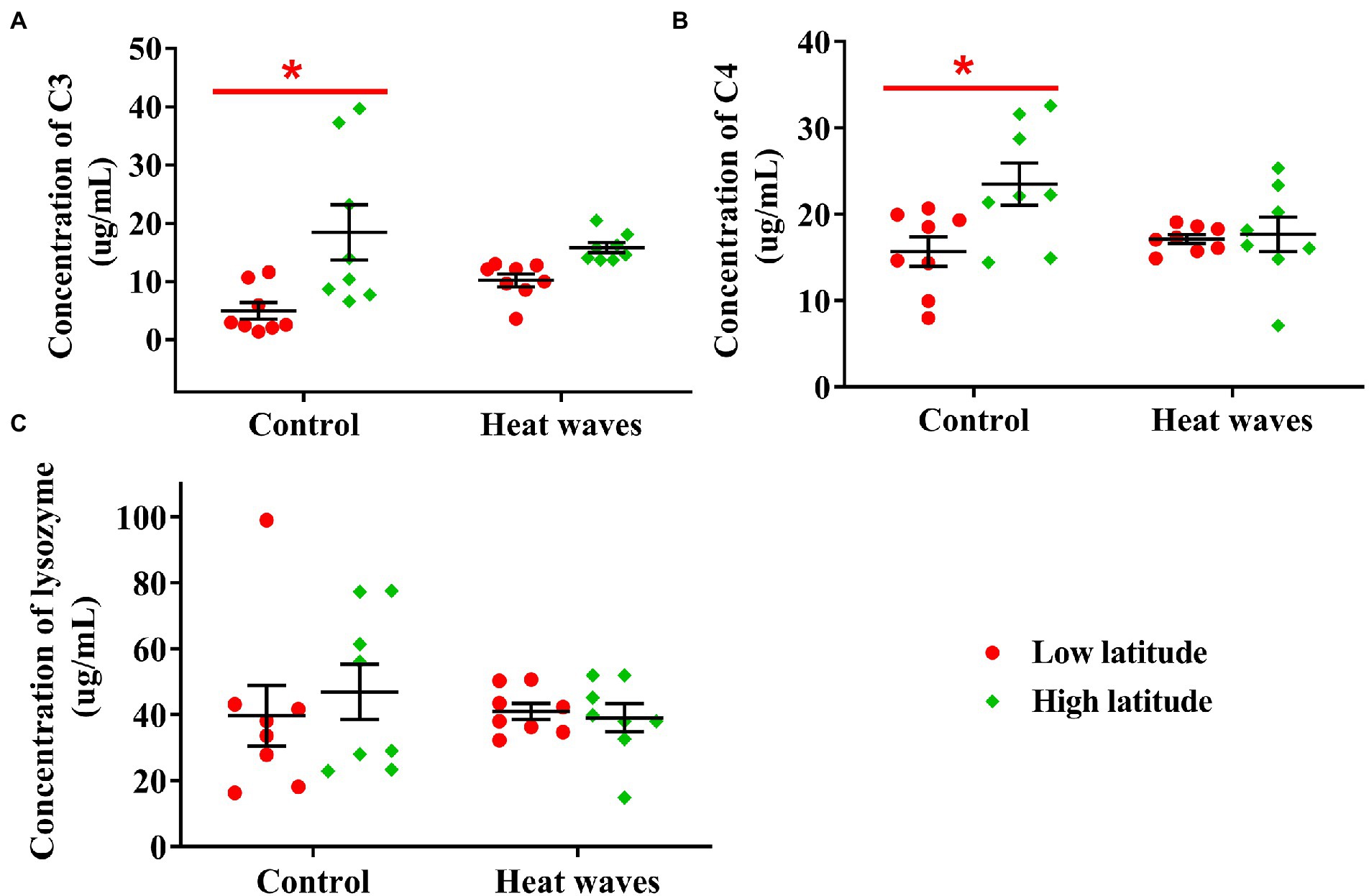
Figure 3. The concentration of serum C3 (A), C4 (B), and lysozyme (C) in plasma of the turtles from low and high latitudes. The red spots and green diamonds indicate the turtles from low and high latitudes, respectively. Each dot indicates an individual, and the error bars indicate the mean ± SE. Red asterisks indicate significant differences between latitudes under the same treatment. Lowercases indicate significant differences between treatments in turtles from low latitude.
In contrast, lysozyme activity was not affected by population origin (F1, 28 = 0.155, p = 0.697), thermal treatment (F1, 28 = 0.243, p = 0.626), or their interactions (F1, 28 = 470, p = 0.499; Figure 3C).
Discussion
Summer heatwaves caused by climate warming have been demonstrated to impose increasing threats to animals (Stillman, 2019; Witze, 2022). However, how heat waves affect animals is still largely unknown, especially at the physiological and biochemical levels (Somero, 2011; Campbell-Staton et al., 2020). This study demonstrated that the effects of simulated heat waves on the biochemistry and physiology of the Chinese pond turtle, M. reevesii, were complex and varied across latitudes. We found that 1-week heat waves caused significant damage at the cellular level by increasing MDA levels, together with a depressed antioxidant defense in turtles from low latitudes, but not affecting the turtles from high latitudes (Figure 2). Although innate immunity-related traits were not depressed by heat waves, the lower daily level of immunity combined with higher oxidative damage may still predict vulnerability to climate warming in turtles from low latitudes.
Higher oxidative damage by heat waves in low-latitude turtles
When ROS are not quenched by antioxidant defenses, they cause oxidative damage such as lipid peroxidation, protein carbonylation, and DNA damage (Ben Ameur et al., 2012). As one of the final products of lipid peroxidation, MDA content reflects the degree of oxidative damage suffered by important biomacromolecules, including phospholipids, glycolipids, and cholesterol (Parrish, 2013; Ayala et al., 2014). This damage leads to functional loss and disruption of homeostasis (Dong et al., 2021).
In this study, we found that the MDA content in the liver of turtles from low latitudes (tropical areas) increased significantly after 1 week of exposure to heat waves (Figure 2A), indicating significant negative effects. Heat waves could be increasing the metabolism by which ROS are produced, and consequently, oxidative damage (e.g., MDA) would accumulate (Jena et al., 2013). This result is similar to the adverse effects observed on a lizard (Eremias multiocellata; Han et al., 2020). However, heat waves did not induce significant oxidative damage in the Chinese soft-shell turtle (Pelodiscus sinensis; Zhang et al., 2019), or the yellow pond turtle (Mauremys mutica; Li et al., 2021). This discrepancy may be due to the population origin of the studied individuals. Previous studies on P. sinensis (Hebei, 39°58′N) and M. mutica (Zhejiang, 30°46′N) have been conducted on turtles from high latitudes (Zhang et al., 2019; Li et al., 2021). As it may be that high-latitude reptiles live at temperatures lower than their physiological optimum (Hao et al., 2020; Liu et al., 2022), higher environmental temperatures would be beneficial for their functions (Huey et al., 2009), and heat waves may not lead to significant oxidative damage. Similarly, in the present study, the MDA content of high latitude specimens did not increase after exposure to heat waves.
Alternatively, the MDA content is also regulated by the balance between oxidative stress and antioxidant defense (Halliwell, 2007). In this study, all the antioxidant defense-related traits, except CAT, were downregulated (i.e., SOD, GSH/GSSG, and T-AOC) in turtles from low latitudes after being exposed to heat waves (Figure 2). Widely depressed antioxidant defenses (i.e., SOD, GPx, and T-AOC) in low-latitude turtles may limit their ability to eliminate ROS and prevent oxidative damage, such as the production of MDA (Došenović et al., 2021; Pinya et al., 2021). The effects of SOD, CAT, and GPx activity on the elimination of MDA have been widely demonstrated in vertebrates, including fishes (Fakhereddin and Doğan, 2021), reptiles (Han et al., 2020; Kou et al., 2021; Pinya et al., 2021), birds (Altan et al., 2003), and mammals (Wei et al., 2016). For instance, oxidative damage (i.e., MDA content) caused by seawater in rats is higher in individuals with lower SOD activity (Wei et al., 2016). In this study, the high-latitude population of pond turtles showed conflicting ability in antioxidant defense, exhibiting decreased GSH/GSSG but increased CAT after exposure to heat waves (Figures 2C,D).
Another important finding in this study was that under the control treatment, the MDA content, and the activity of SOD and T-AOC, in low-latitude turtles were higher than those in their counterparts from high latitudes (Figures 2A,B,E). The MDA content can be increased by chronic warming as reported in the mussel Mytilus coruscus (Khan et al., 2021), and the octocoral Veretillum cynomorium (Lopes et al., 2018). As the low-latitude population of pond turtles in this study experienced higher ambient temperatures than the high-latitude population, the higher MDA content may reflect a chronic accumulation of oxidative damage induced by average warmer temperatures similar to other reptiles (Reguera et al., 2014). Accordingly, the higher activities of SOD and T-AOC may also reflect that the antioxidant defense was chronically triggered by oxidative stress in low-latitude pond turtles.
Heat waves did not depress innate immunity
As an initial response to pathogens, innate immunity is nonspecific and essential for individuals to survive in stressful surroundings (Lafferty, 2009). In addition, the immune response of organisms relies on the production of pro-oxidant substances and is regulated by oxidative stress (Espelid et al., 1996; Sorci and Faivre, 2009). Therefore, innate immunity is a robust index for evaluating the fitness consequences of oxidative damage caused by heat waves (Han et al., 2020). Innate immunity, universal to reptiles, has been demonstrated to be thermally sensitive in turtles (Zimmerman et al., 2017).
In this study, the concentrations of C3, C4, and lysozyme did not differ among thermal treatments in either high- or low-latitude turtles, implying that innate immunity was not adjusted by heat waves. These results contradict our assumption that oxidative damage would induce decreased immunity. Although innate immunity is widely demonstrated to be inhibited by heat waves in ectotherms, including snails (Leicht et al., 2013), fish (Dittmar et al., 2014), and reptiles (Stahlschmidt et al., 2017), it was also found to be enhanced (Adamo and Lovett, 2011; Li et al., 2021), or unaltered (Zimmerman et al., 2017). This study provides further evidence that the effects of heat waves on innate immunity are complex in ectotherms. Although we did not find decreased C3, C4, or lysozyme levels, heat waves might decline some other traits of innate immunity. For example, heat waves induce oxidative stress and depress innate immunity by low IgM and white blood cells (WBC) in racerunners (Eremias multiocellata; Han et al., 2020).
Interestingly, this study found higher concentrations of C3 and C4 in high-latitude pond turtles under the control treatment, indicating a higher ability to kill bacteria or digest pathogens than those in low-latitude turtles under moderate temperatures (Baker et al., 2019). Although heat waves did not depress the innate immunity of turtles from low latitudes, their lower C3 and C4 concentrations under moderate temperatures may imply weaker immunity.
In summary, this study revealed oxidative damage in the liver of low-latitude pond turtles (M. reeviesii) after experiencing 1-week heat waves, relative to their high-latitude counterparts. The innate immunity of C3, C4, and lysozyme were not affected in either latitudinal population; however, we found lower daily C3 and C4 levels in the low-latitude turtles. By integrating the above physiological and biochemical responses, we predicted that pond turtles would be more vulnerable to climate warming. Nonetheless, it is notable that in this study, we only focused on oxidative damage and related immunity at physiological and biochemical levels. As the frequency of heat waves has increased in recent years (Stillman, 2019; Witze, 2022), the consequences on fitness-related traits of individuals and populations, including reproduction, survival, and population dynamics, should be given more attention (Deutsch et al., 2008; Stahlschmidt et al., 2017; Sun et al., 2021). In addition, oxidative stress, damage, and antioxidative defense are complex networks, and before we can draw a comprehensive picture, more indices should be added in future research, such as carbonylated protein, 8-hydroxy-2-deoxy Guanosine (8-OH-dG), IgA, IgM, and WBC, to evaluate the damage to biomacromolecules and immunities (Espelid et al., 1996; Zimmerman et al., 2010, 2017). Furthermore, most current studies have evaluated the effects of heat waves under climate warming by immediate or short-term responses of the species, as in this study. Aging and longevity should be included in future research to provide a chronic and long-lasting view for investigating the effects of climate warming on animals (Zhang et al., 2018; Zhu et al., 2019; Dupoué et al., 2022).
Data availability statement
The raw data supporting the conclusions of this article will be made available by the authors, without undue reservation.
Ethics statement
The animal study was reviewed and approved by Animal Ethical and Welfare Committee of Wenzhou University (Approval No. WZU-049).
Author contributions
YZ and SL designed the study, analyzed the data, and wrote the manuscript with comments from XH, QZ, and LX. SL, WT, JO, and DW conducted the experiments. All authors contributed to the article and approved the submitted version.
Funding
This research was supported by grants from the National Natural Science Foundation of China (nos. 31971419, 32171486, and 31801977), and the Natural Science Foundation of Zhejiang Province (no. LY16C030001).
Acknowledgments
We appreciate Luyi Han, Hongya Huang, Mengting Jin, and Jiayuan Huang for their assistance in turtle husbandry and data collection during the study.
Conflict of interest
The authors declare that the research was conducted in the absence of any commercial or financial relationships that could be construed as a potential conflict of interest.
Publisher’s note
All claims expressed in this article are solely those of the authors and do not necessarily represent those of their affiliated organizations, or those of the publisher, the editors and the reviewers. Any product that may be evaluated in this article, or claim that may be made by its manufacturer, is not guaranteed or endorsed by the publisher.
Supplementary material
The Supplementary material for this article can be found online at: https://www.frontiersin.org/articles/10.3389/fevo.2022.1053260/full#supplementary-material
SUPPLEMENTARY FIGURE S1 | Identification of phylogenetic relationship between the turtles from Zhejiang and Hainan populations using CytB gene as marker, and Pelodiscus sinensis as the outgroup. ‘S’ indicates low latitude population and ‘N’ indicates high latitude population.
SUPPLEMENTARY FIGURE S2 | Mean monthly air temperatures from Zhejiang and Hainan populations (1981-2010). Data were obtained from China Meteorological Data Service Center.
References
Ackerman, R. A. (1981). Growth and gas exchange of embryonic sea turtles (Chelonia caretta). Copeia 1981, 757–765. doi: 10.2307/1444175
Adamo, S. A., and Lovett, M. M. (2011). Some like it hot: the effects of climate change on reproduction, immune function and disease resistance in the cricket Gryllus texensis. J. Exp. Biol. 214, 1997–2004. doi: 10.1242/jeb.056531
Altan, O., Pabuçcuoğlu, A., Altan, A., Konyalioğlu, S., and Bayraktar, H. (2003). Effect of heat stress on oxidative stress, lipid peroxidation and some stress parameters in broilers. Br. Poult. Sci. 44, 545–550. doi: 10.1080/00071660310001618334
Ashmore, G. M., and Janzen, F. J. (2003). Phenotypic variation in smooth softshell turtles (Apalone mutica) from eggs incubated in constant versus fluctuating temperatures. Oecologia 134, 182–188. doi: 10.1007/s00442-002-1109-z
Attaway, M. B., Packard, G. C., and Packard, M. J. (1998). Hatchling painted turtles (Chrysemys picta) survive only brief freezing of their bodily fluids. Comp. Biochem. Physiol. A Mol. Integr. Physiol. 120, 405–408. doi: 10.1016/S1095-6433(98)00031-2
Ayala, A., Muñoz, M. F., and Argüelles, S. (2014). Lipid peroxidation: production, metabolism, and signaling mechanisms of malondialdehyde and 4-hydroxy-2-nonenal. Oxidative Med. Cell. Longev. 2014:360438. doi: 10.1155/2014/360438
Baker, S. J., Kessler, E. J., and Merchant, M. E. (2019). Antibacterial activities of plasma from the common (Chelydra serpentina) and alligator snapping turtle (Macrochelys temminckii). J. Exp. Zool. Part A Ecol. Integr. Physiol. 331, 85–92. doi: 10.1002/jez.2237
Baker, B. P., Van Wie, I., Braun, E., and Jimenez, A. G. (2020). Thermal stability vs. variability: insights in oxidative stress from a eurytolerant fish. Comp. Biochem. Physiol. A Mol.-Integr. Physiol. 249:110767. doi: 10.1016/j.cbpa.2020.110767
Ben Ameur, W., De Lapuente, J., El Megdiche, Y., Barhoumi, B., Trabelsi, S., Camps, L., et al. (2012). Oxidative stress, genotoxicity and histopathology biomarker responses in mullet (Mugil cephalus) and sea bass (Dicentrarchus labrax) liver from Bizerte lagoon (Tunisia). Mar. Pollut. Bull. 64, 241–251. doi: 10.1016/j.marpolbul.2011.11.026
Binckley, C. A., Spotila, J. R., Wilson, K. S., and Paladino, F. V. (1998). Sex determination and sex ratios of Pacific leatherback turtles. Copeia 1998, 291–300. doi: 10.2307/1447425
Bostrom, B. L., Jones, T. T., Hastings, M., and Jones, D. R. (2010). Behaviour and physiology: the thermal strategy of leatherback turtles. PLoS One 5:e13925. doi: 10.1371/journal.pone.0013925
Burggren, W. (2018). Developmental phenotypic plasticity helps bridge stochastic weather events associated with climate change. J. Exp. Biol. 221:jeb161984. doi: 10.1242/jeb.161984
Campbell-Staton, S. C., Winchell, K. M., Rochette, N. C., Fredette, J., Maayan, I., Schweizer, R. M., et al. (2020). Parallel selection on thermal physiology facilitates repeated adaptation of city lizards to urban heat islands. Nat. Ecol. Evol. 4, 652–658. doi: 10.1038/s41559-020-1131-8
Carlo, M. A., Riddell, E. A., Levy, O., and Sears, M. W. (2018). Recurrent sublethal warming reduces embryonic survival, inhibits juvenile growth, and alters species distribution projections under climate change. Ecol. Lett. 21, 104–116. doi: 10.1111/ele.12877
Dahlke, F. T., Wohlrab, S., Butzin, M., and Pörtner, H. O. (2020). Thermal bottlenecks in the life cycle define climate vulnerability of fish. Science 369:65. doi: 10.1126/science.aaz3658
Danner, R. M., Coomes, C. M., and Derryberry, E. P. (2021). Simulated heat waves reduce cognitive and motor performance of an endotherm. Ecol. Evol. 11, 2261–2272. doi: 10.1002/ece3.7194
Deutsch, C. A., Tewksbury, J. J., Huey, R. B., Sheldon, K. S., Ghalambor, C. K., Haak, D. C., et al. (2008). Impacts of climate warming on terrestrial ectotherms across latitude. Proc. Natl. Acad. Sci. U. S. A. 105, 6668–6672. doi: 10.1073/pnas.0709472105
Dittmar, J., Janssen, H., Kuske, A., Kurtz, J., and Scharsack, J. P. (2014). Heat and immunity: an experimental heat wave alters immune functions in three-spined sticklebacks (Gasterosteus aculeatus). J. Anim. Ecol. 83, 744–757. doi: 10.1111/1365-2656.12175
Dong, A. G., Huo, J. F., Yan, J. J., Dong, A. L., and Liu, B. W. (2021). Lipid peroxidation of kidney of the turtle Mauremys reevesii caused by cadmium. Environ. Sci. Pollut. Res. 28, 6811–6817. doi: 10.1007/s11356-020-11054-x
Došenović, M., Radaković, M., Vučićević, M., Vejnović, B., Vasiljević, M., Marinković, D., et al. (2021). Evaluation of the effects of two anaesthetic protocols on oxidative status and DNA damage in red-eared sliders (Trachemys scripta elegans) undergoing endoscopic coeliotomy. Acta Vet. Hung. 68, 337–344. doi: 10.1556/004.2020.00058
Du, W. G., Shen, J. W., and Wang, L. (2009). Embryonic development rate and hatchling phenotypes in the Chinese three-keeled pond turtle (Chinemys reevesii): the influence of fluctuating temperature versus constant temperature. J. Therm. Biol. 34, 250–255. doi: 10.1016/j.jtherbio.2009.03.002
Dupoué, A., Blaimont, P., Angelier, F., Ribout, C., Rozen-Rechels, D., Richard, M., et al. (2022). Lizards from warm and declining populations are born with extremely short telomeres. Proc. Natl. Acad. Sci. U. S. A. 119:e2201371119. doi: 10.1073/pnas.2201371119
Espelid, S., LØkken, G. B., Steiro, K., and BØgwald, J. (1996). Effects of cortisol and stress on the immune system in Atlantic Salmon (Salmo salar L.). Fish Shellfish Immunol. 6, 95–110. doi: 10.1006/fsim.1996.0011
Fakhereddin, T., and Doğan, D. (2021). Pro-oxidant potency of clothianidin in rainbow trout. Arh. Hig. Rada Toksikol. 72, 164–172. doi: 10.2478/aiht-2021-72-3522
Fischer, K., Klockmann, M., and Reim, E. (2014). Strong negative effects of simulated heat waves in a tropical butterfly. J. Exp. Biol. 217, 2892–2898. doi: 10.1242/jeb.106245
Goessling, J. M., Ward, C., and Mendonça, M. T. (2019). Rapid thermal immune acclimation in common musk turtles (Sternotherus odoratus). J. Exp. Zool. Part A Ecol. Integr. Physiol. 331, 185–191. doi: 10.1002/jez.2252
Hall, J. M., and Sun, B. J. (2021). Heat tolerance of reptile embryos: current knowledge, methodological considerations, and future directions. J. Exp. Zool. Part A Ecol. Integr. Physiol. 335, 45–58. doi: 10.1002/jez.2402
Halliwell, B. (2007). Biochemistry of oxidative stress. Biochem. Soc. Trans. 35, 1147–1150. doi: 10.1002/anie.198610581
Han, X. Z., Hao, X., Wang, Y., Wang, X. F., Teng, L. W., Liu, Z. S., et al. (2020). Experimental warming induces oxidative stress and immunosuppression in a viviparous lizard, Eremias multiocellata. J. Therm. Biol. 90:102595. doi: 10.1016/j.jtherbio.2020.102595
Hansen, J., Sato, M., and Ruedy, R. (2012). Perception of climate change. Proc. Natl. Acad. Sci. U. S. A. 109, E2415–E2423. doi: 10.1073/pnas.1205276109
Hao, X., Tao, S. A., Meng, Y., Liu, J. Y., Cui, L. X., Liu, W. L., et al. (2020). Thermal biology of cold-climate distributed Heilongjiang grass lizard, Takydromus amurensis. Asian Herpetol. Res. 11, 350–359. doi: 10.16373/j.cnki.ahr.200020
Harvell, C. D., Montecino-Latorre, D., Caldwell, J. M., Burt, J. M., Bosley, K., Keller, A., et al. (2019). Disease epidemic and a marine heat wave are associated with the continental-scale collapse of a pivotal predator (Pycnopodia helianthoides). Sci. Adv. 5:eaau7042. doi: 10.1126/sciadv.aau7042
Huey, R. B., Deutsch, C. A., Tewksbury, J. J., Vitt, L. J., Hertz, P. E., Álvarez Pérez, H. J., et al. (2009). Why tropical forest lizards are vulnerable to climate warming. Proc. R. Soc. B Biol. Sci. 276, 1939–1948. doi: 10.1098/rspb.2008.1957
IPCC (2021). “Climate change 2021: the physical science basis” in Contribution of Working Group I to the Sixth Assessment Report of the Intergovernmental Panel on Climate Change, eds. V. Masson-Delmotte, P. Zhai, A. Pirani, S. L. Connors, C. Péan, S. Berger, N. Caud, Y. Chen, L. Goldfarb, M. I. Gomis, M. Huang, K. Leitzell, E. Lonnoy, J. B. R. Matthews, T. K. Maycock, T. Waterfield, O. Yelekçi, R. Yu, and B. Zhou (Cambridge, United Kingdom and New York, NY, USA: Cambridge University Press).
IUCN (2022). The IUCN Red List of Threatened Species. Version 2022-1. Available at: https://www.iucnredlist.org
Jena, K., Kumar Kar, P., Kausar, Z., and Babu, C. S. (2013). Effects of temperature on modulation of oxidative stress and antioxidant defenses in testes of tropical tasar silkworm Antheraea mylitta. J. Therm. Biol. 38, 199–204. doi: 10.1016/j.jtherbio.2013.02.008
Khan, F. U., Chen, H., Gu, H. X., Wang, T., Dupont, S., Kong, H., et al. (2021). Antioxidant responses of the mussel Mytilus coruscus co-exposed to ocean acidification, hypoxia and warming. Mar. Pollut. Bull. 162:111869. doi: 10.1016/j.marpolbul.2020.111869
Kou, H. Y., Hu, J. R., Vijayaraman, S. B., Wang, A. L., Zheng, Y. Y., Chen, J. J., et al. (2021). Evaluation of dietary zinc on antioxidant-related gene expression, antioxidant capability and immunity of soft-shelled turtles Pelodiscus sinensis. Fish Shellfish Immunol. 118, 303–312. doi: 10.1016/j.fsi.2021.08.033
Lafferty, K. D. (2009). The ecology of climate change and infectious diseases. Ecology 90, 888–900. doi: 10.1890/08-0079.1
Leicht, K., Jokela, J., and Seppälä, O. (2013). An experimental heat wave changes immune defense and life history traits in a freshwater snail. Ecol. Evol. 3, 4861–4871. doi: 10.1002/ece3.874
Li, S. R., Li, J. H., Chen, W., Xu, Z. W., Xie, L., and Zhang, Y. P. (2021). Effects of simulated heat wave on oxidative physiology and immunity in Asian yellow pond turtle (Mauremys mutica). Front. Ecol. Evol. 9:704105. doi: 10.3389/fevo.2021.704105
Liu, W. L., Liu, P., Cui, L. X., Meng, Y., Tao, S. A., Han, X. Z., et al. (2022). Moderate climate warming scenarios during embryonic and post-embryonic stages benefit a cold-climate lizard. Funct. Ecol. 36, 1137–1150. doi: 10.1111/1365-2435.14032
Logan, M. L., and Cox, C. L. (2020). Genetic constraints, transcriptome plasticity, and the evolutionary response to climate change. Front. Genet. 11:1088. doi: 10.3389/fgene.2020.538226
Lopes, A. R., Faleiro, F., Rosa, I. C., Pimentel, M. S., Trubenbach, K., Repolho, T., et al. (2018). Physiological resilience of a temperate soft coral to ocean warming and acidification. Cell Stress Chaperone. 23, 1093–1100. doi: 10.1007/s12192-018-0919-9
Mckechnie, A. E., and Wolf, B. O. (2010). Climate change increases the likelihood of catastrophic avian mortality events during extreme heat waves. Biol. Lett. 6, 253–256. doi: 10.1098/rsbl.2009.0702
Morignat, E., Gay, E., Vinard, J. L., Sala, C., Calavas, D., and Hénaux, V. (2018). Impact of heat and cold waves on female cattle mortality beyond the effect of extreme temperatures. J. Therm. Biol. 78, 374–380. doi: 10.1016/j.jtherbio.2018.11.001
Munro, D., and Treberg, J. R. (2017). A radical shift in perspective: mitochondria as regulators of reactive oxygen species. J. Exp. Biol. 220, 1170–1180. doi: 10.1242/jeb.132142
Parrish, C. C. (2013). Lipids in marine ecosystems. ISRN Oceanogr. 2013:604045. doi: 10.5402/2013/604045
Pinya, S., Renga, E., Fernández, G., Mateu-Vicens, G., Tejada, S., Capó, X., et al. (2021). Physiological biomarkers in loggerhead turtles (Caretta caretta) as a tool for monitoring sanitary evolution in marine recovery centres. Sci. Total Environ. 757:143930. doi: 10.1016/j.scitotenv.2020.143930
Reguera, S., Zamora-Camacho, F. J., Trenzado, C. E., Sanz, A., and Moreno-Rueda, G. (2014). Oxidative stress decreases with elevation in the lizard Psammodromus algirus. Comp. Biochem. Physiol. A Mol. Integr. Physiol. 172, 52–56. doi: 10.1016/j.cbpa.2014.02.018
Rogers-Bennett, L., and Catton, C. A. (2019). Marine heat wave and multiple stressors tip bull kelp forest to sea urchin barrens. Sci. Rep. 9:15050. doi: 10.1038/s41598-019-51114-y
Somero, G. N. (2011). Comparative physiology: a “crystal ball” for predicting consequences of global change. Am. J. Physiol. Regul. Integr. Comp. Physiol. 301, R1–R14. doi: 10.1152/ajpregu.00719.2010
Sorci, G., and Faivre, B. (2009). Inflammation and oxidative stress in vertebrate host–parasite systems. Philos. Trans. R. Soc. B Biol. Sci. 364, 71–83. doi: 10.1098/rstb.2008.0151
Speakman, J. R., and Garratt, M. (2014). Oxidative stress as a cost of reproduction: beyond the simplistic trade-off model. BioEssays 36, 93–106. doi: 10.1002/bies.201300108
Stahlschmidt, Z. R., French, S. S., Ahn, A., Webb, A., and Butler, M. W. (2017). A simulated heat wave has diverse effects on immune function and oxidative physiology in the corn snake (Pantherophis guttatus). Physiol. Biochem. Zool. 90, 434–444. doi: 10.1086/691315
Stillman, J. H. (2019). Heat waves, the new normal: summertime temperature extremes will impact animals, ecosystems, and human communities. Physiology 34, 86–100. doi: 10.1152/physiol.00040.2018
Sun, B. J., Ma, L., Wang, Y., Mi, C. R., Buckley, L. B., Levy, O., et al. (2021). Latitudinal embryonic thermal tolerance and plasticity shape the vulnerability of oviparous species to climate change. Ecol. Monogr. 91:e01468. doi: 10.1002/ecm.1468
Sunday, J. M., Bates, A. E., and Dulvy, N. K. (2011). Global analysis of thermal tolerance and latitude in ectotherms. Proc. R. Soc. B Biol. Sci. 278, 1823–1830. doi: 10.1098/rspb.2010.1295
Sunday, J. M., Bates, A. E., and Dulvy, N. K. (2012). Thermal tolerance and the global redistribution of animals. Nat. Clim. Chang. 2, 686–690. doi: 10.1038/Nclimate1539
Taylor, E., Diele-Viegas, L., Gangloff, E., Hall, J., Halpern, B., Massey, M., et al. (2020). The thermal ecology and physiology of reptiles and amphibians: a user's guide. J. Exp. Zool. A Ecol. Integr. Physiol. 335, 13–44. doi: 10.1002/jez.2396
Troschinski, S., Dieterich, A., Krais, S., Triebskorn, R., and Köhler, H. R. (2014). Antioxidant defence and stress protein induction following heat stress in the Mediterranean snail Xeropicta derbentina. J. Exp. Biol. 217, 4399–4405. doi: 10.1242/jeb.113167
Vinagre, C., Mendonça, V., Cereja, R., Abreu-Afonso, F., Dias, M., and Mizrahi, D. (2018). Ecological traps in shallow coastal waters—potential effect of heat-waves in tropical and temperate organisms. PLoS One 13:e0192700. doi: 10.1371/journal.pone.0192700
Wei, L. F., Zhang, H. M., Wang, S. S., Jing, J. J., Zheng, Z. C., and Gao, J. X. (2016). Changes of MDA and SOD in brain tissue after secondary brain injury with seawater immersion in rats. Turk. Neurosurg. 26, 384–388. doi: 10.5137/1019-5149.Jtn.8265-13.1
Witze, A. (2022). Extreme heatwaves: surprising lessons from the record warmth. Nature 608, 464–465. doi: 10.1038/d41586-022-02114-y
Wu, P. F., Wang, X. F., Gao, F., and Du, W. G. (2022). Role of Cyp19a1 in the female pathway of a freshwater turtle species (Mauremys reevesii) with temperature-dependent sex determination. Zool. Res. 43, 81–84. doi: 10.24272/j.issn.2095-8137.2021.287
Xie, S. Z., Romero, L. M., Htut, Z. W., and Mcwhorter, T. J. (2017). Stress responses to heat exposure in three species of Australian desert birds. Physiol. Biochem. Zool. 90, 348–358. doi: 10.1086/690484
Ye, R. H., Zheng, R. Q., Wang, L., and Du, W. G. (2009). Polymorphic microsatellite loci in the Chinese pond turtle (Chinemys reevesii). Conserv. Genet. 10, 1045–1048. doi: 10.1007/s10592-008-9684-0
Zhang, Q., Han, X. Z., Hao, X., Ma, L., Li, S. R., and Du, W. G. (2018). A simulated heat wave shortens the telomere length and lifespan of a desert lizard. J. Therm. Biol. 72, 94–100. doi: 10.1016/j.jtherbio.2018.01.004
Zhang, W. Y., Niu, C. J., Liu, Y. K., and Storey, K. B. (2019). Positive or negative? The shell alters the relationship among behavioral defense strategy, energy metabolic levels and antioxidant capacity in freshwater turtles. Front. Zool. 16:3. doi: 10.1186/s12983-019-0301-5
Zhu, L., Wang, L., and Ma, C. S. (2019). Sporadic short temperature events cannot be neglected in predicting impacts of climate change on small insects. J. Insect Physiol. 112, 48–56. doi: 10.1016/j.jinsphys.2018.12.003
Zimmerman, L. M., Carter, A. W., Bowden, R. M., and Vogel, L. A. (2017). Immunocompetence in a long-lived ectothermic vertebrate is temperature dependent but shows no decline in older adults. Funct. Ecol. 31, 1383–1389. doi: 10.1111/1365-2435.12867
Keywords: climate warming, heat waves, oxidative stress, antioxidant defense, innate immunity, turtle
Citation: Tao W, Ou J, Wu D, Zhang Q, Han X, Xie L, Li S and Zhang Y (2022) Heat wave induces oxidative damage in the Chinese pond turtle (Mauremys reevesii) from low latitudes. Front. Ecol. Evol. 10:1053260. doi: 10.3389/fevo.2022.1053260
Edited by:
Lin Zhang, Hubei University of Chinese Medicine, ChinaReviewed by:
Wenyi Zhang, Nanjing Normal University, ChinaHong-Liang Lu, Hangzhou Normal University, China
Li Ding, Hainan Normal University, China
Copyright © 2022 Tao, Ou, Wu, Zhang, Han, Xie, Li and Zhang. This is an open-access article distributed under the terms of the Creative Commons Attribution License (CC BY). The use, distribution or reproduction in other forums is permitted, provided the original author(s) and the copyright owner(s) are credited and that the original publication in this journal is cited, in accordance with accepted academic practice. No use, distribution or reproduction is permitted which does not comply with these terms.
*Correspondence: Shuran Li, bGlzaHVyYW5Ad3p1LmVkdS5jbg==; Yongpu Zhang, emhhbmd5cEB3enUuZWR1LmNu
†These authors have contributed equally to this work