- Department of Zoology, University of British Columbia, Vancouver, BC, Canada
The introduction of the functional response into population ecology in 1949 by Maurice Solomon was focused on explaining population regulation by density-dependent mortality caused by predators and natural enemies. Like many simple ecological measures originating at the population level, it was soon being used for other purposes at the single species and individual predator level. It is thus necessary when we use this important response function that we have a clear hypothesis in mind that is being tested. Here I provide a capsular summary of the origins of the functional response and suggest five problems with its application in population and community ecology. The functional response has much utility as a critical component of understanding population and community dynamics but must be carefully aimed at specific questions.
Introduction
I present here a brief overview of the history of the functional response concept, having lived through the early work of Maurice Solomon (Solomon, 1949) followed by the pioneering work of Holling (1959), the additions by Murdoch (1971), and the growing literature that has followed all this early work. My purpose here is to provide a capsular history of this early work, and then to explore five general problems that affect the use of functional responses for real world predator prey systems. I present no simple solutions for these problems, but they must be noted and clearly specified.
A short history of predator–prey dynamics
Everyone knew that predators ate prey but in the 1930s and 1940s most questions were about the natural-history interactions of predators and their prey. Interest arose in the 1930s and 1940s almost independently in agricultural pest control studies and in wildlife management problems regarding the conservation of mammals and birds. Solomon (1949) summarized the existing literature on population dynamics and produced a synthesis that brought data from laboratory and field populations into the framework of density-dependence population regulation framed by A.J. Nicholson (Nicholson, 1933). In his synthesis, Solomon (1949) defined the functional response as the number of prey items consumed by an individual predator per unit of time, and he explored how the functional response might change in relation to the density of the prey items. The numerical response was complementary to the functional response and recorded the change in the density of the predator as its prey population rose or fell in density, and it had already been recognized earlier by insect ecologists. There is a great deal of simple theory in Solomon (1949) but the limitations of this pioneering approach as a sufficient tool for understanding the role of predator–prey interactions in population and community dynamics has changed as both theoretical and empirical ecology has developed during the last 70 years.
Holling (1959) elaborated the components of predation described earlier by Solomon and applied these to his analysis in a classic paper of small mammal predation rates on the cocooned pupae of the European pine sawfly in Ontario pine forests. Holling described three types of functional responses (Figure 1), which when combined with three kinds of numerical responses would lead to a variety of rates of prey losses to predators. The principles were clear, the results less so because of statistical problems of obtaining field data on predator feeding rates on sawfly cocoons and the difficulties of scaling laboratory feeding rates to field situations. Holling’s work was a pioneering effort to describe numerical and functional responses of predators via simple mathematical models so that they could be combined to provide an estimate of the total loss of prey to predators, one of the ultimate goals of predation theory (Holling, 1961). But again as in Solomon’s original work, the limitations of these simple models of predator–prey interactions were not clearly identified.
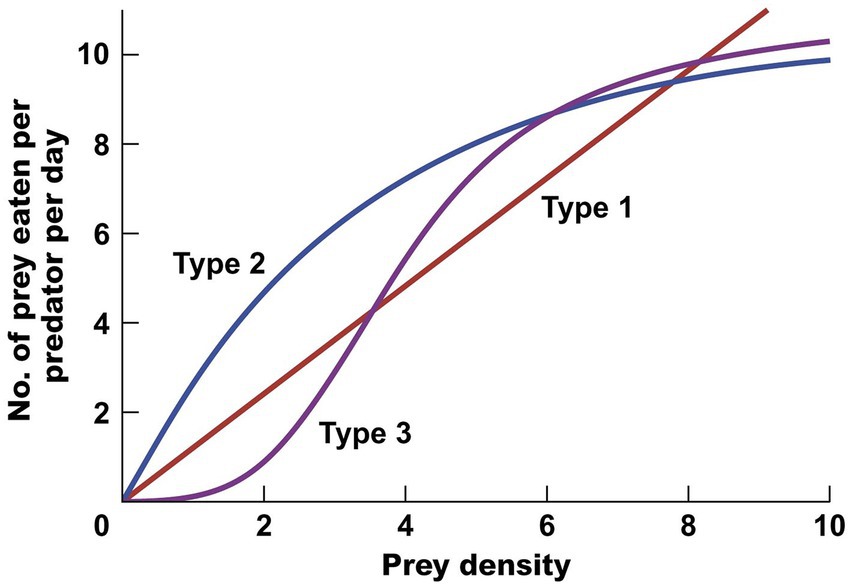
Figure 1. Three types of functional responses of a predator to different levels of prey density. Holling (1959) named these Type 1, 2, and 3, and for any particular predator–prey system, much research has gone into determining which of these 3 curves, if any, describe the data best. [From (Krebs, 2009), Figure 11.14.]
Adding to the complexity, Murdoch (1971) pointed out that the functional response could be altered by growth or changes in size of the predators, so that a count of predator numbers would not of necessity be an accurate measure of the functional response. He called this the developmental response and pointed out that predators could also change their diets in a feeding response to prey abundance changes. The functional response thus became more decomposed and thus more complicated (Figure 2).
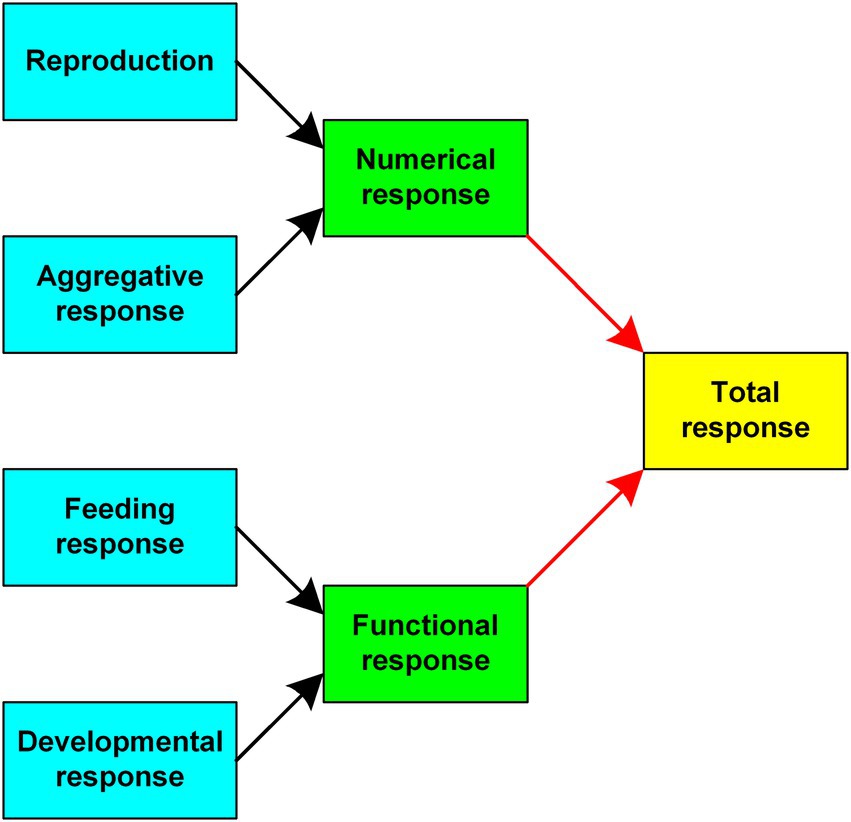
Figure 2. The components of predation that combine to give the total response of a predator to changes in the density of its prey species. [From Krebs, 2009, Figure 11.18.]
There has been much change in understanding predator–prey dynamics since the early days of the 1950s to 1970s, and the remainder of this collection of papers will bring you up to date on these advances. A larger picture has now evolved that recognizes multiple predator-multiple prey species interactions with the involved time lags are critical to understanding how natural communities operate and thus added a layer of complexity to understanding how predator–prey dynamics fits within the global view of ecology at the present time.
Much controversy involving functional responses remains to be resolved. One controversy has occurred over the competing concepts of prey-dependent, ratio-dependent, or predator dependent, measuring the effects of predation by ratios of predator density to prey density (reviewed by Abrams, 2015). Many additional papers have discussed which type of functional response model is to be preferred (e.g., Barraquand, 2014; Ginzburg and Damuth, 2022), and these controversies over new functional forms of predator–prey models, and how to deal with the non-consumptive effects of predators are what has spurred much broader interest in the analysis of predator–prey dynamics.
From an empirical point of view I would like to outline here some of my thoughts on the current state of predator–prey studies with concentration on functional responses of predators to prey. There are a variety of issues in population and community ecology for which functional ecology ideas are useful. I concentrate here on the traditional use of the functional response to assess how a particular predator can influence changes in population density of one or more prey species. I will use the food web of the Boreal Forest ecosystem at Kluane, Yukon (Figure 3) as a touchstone for my comments. I recognise five problems in providing answers to what would seem to be a simple problem in prey population dynamics.
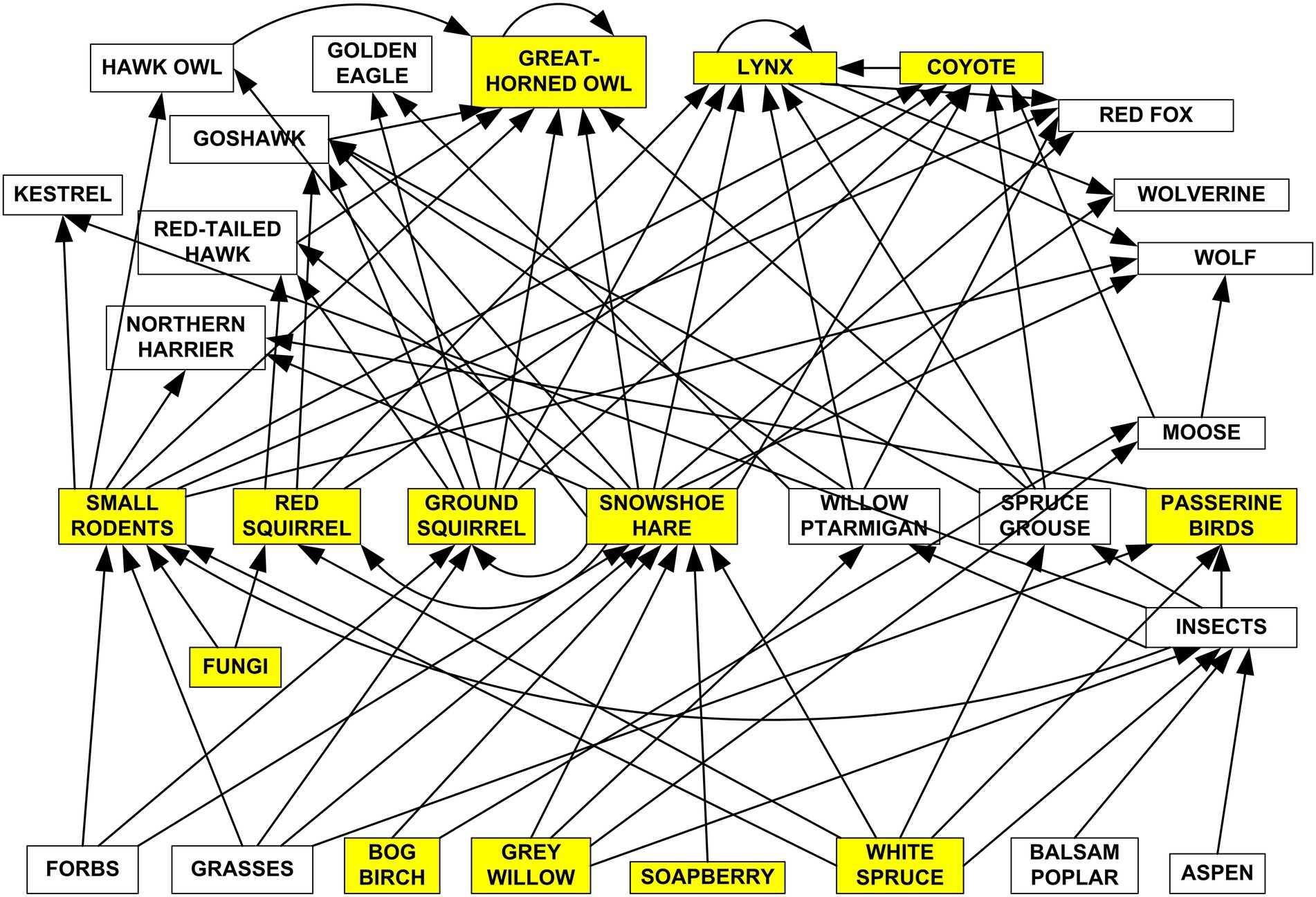
Figure 3. Simplified food web for the boreal forest at Kluane Lake, Yukon. All these species and species groups have been studied but the shaded taxa have had the majority of the research focus [revised from Krebs et al., 2001, Figure 2.8]. The complexities implied in this relatively simple food web is challenging.
Many predators may be more specialist than generalist
There is much discussion in the mammalian literature on this topic (Lambin et al., 2000; Graham and Lambin, 2002; Klemola et al., 2002; Ylönen et al., 2003; Smout et al., 2010; Peers et al., 2012). Many complications are introduced when a particular predator feeds on a variety of prey, or when several predators concentrate feeding on one species of prey. At Kluane Lake most predators are focused on snowshoe hares (Figure 3) but virtually all the predators in this ecosystem are generalists that survive by consuming many prey species. What species is a generalist predator in some ecosystems may be classed as a specialist in other ecosystems, and what species is a specialist predator in winter can be a generalist predator in summer, so that simple conclusions that the Canada lynx (Lynx canadensis) in this system in a specialist predator is an oversimplification. For both vertebrate and invertebrate predators this is the first hurdle – to avoid the trap of one predator-one prey modelling, which simplifies the mathematics but ignores the real world.
Predators often switch prey items seasonally or in relation to shifts in multiple prey abundances within the same ecosystem
A large literature exists on the variable diets of predators, and handling time varies among different prey types. In these cases there is no simple one functional response needed to define predator capture rates if you wish to use functional responses as part of a model for prey and predator dynamics (Murdoch, 1969; Kjellander and Nordström, 2003; Peers et al., 2014). Multiple prey abundances can be dealt with in a model (e.g., Chan et al., 2017). Seasonality and diurnal activity patterns both introduce an array of complications to understanding the effects of predation on prey population dynamics (Studd et al., 2020). At Kluane Lake Canada lynx diet in summer is very poorly known and we cannot assume it is a specialist predator without further data on seasonal diets.
Wide-ranging predators can operate in many distinct prey communities
Spatial variation in the food webs of habitats occupied confound generalizations for predators that have a sub-continental scale geographic range. Roth et al. (2007) illustrated this for Canada lynx, and Peers et al. (2012, 2014) provide further data on the ecological niches of bobcat (Lynx rufus) and lynx in Canada. Recognizing the variation in the food webs of communities is a necessary start to untangling these differences among multiple predators operating in the same ecosystem. At Kluane Lake for example, our main study area covered 350 sq. km but the movements of individual lynx go from to 100–1,100 km (Slough and Mowat, 1996).
The statistical fits of specific models used to define functional responses are never perfect
Intraspecific variation will cause a lack of fit to any specific response model. We tend to hope that ecological relationships ought to fit some simple mathematical function. This is in my opinion a throwback to the early years of studies on predator–prey ecology and is typified in Holling’s papers (1959) and many papers since. All variation around the fitted curve is described as ‘error’ and yet one suspects that most of the interesting ecology (such as surplus killing, social grouping, behavioural aggression) is contained in those deviations from the expected curve. An example raised by this issue is the paper by Chan et al. (2017) from the Kluane ecosystem study. It is not clear how to model prey depletion or what is the appropriate unit of measure (single kill rate for the whole winter), and the broader issue of which model is least wrong (Abrams and Ginzburg, 2000; Ginzburg and Damuth, 2022).
The use of functional and numerical responses as a sufficient explanation for prey population regulation is bound to fail
Behavioural ecologists have documented many attributes of individual predators, as well as the plethora of variables that affect their food choice, so that it is now impossible to think of a universal functional response relationship that could be used for a predictive model. Work on the snowshoe hare – Canada lynx functional response in the Yukon boreal forest (Figure 4) has been replicated with remarkably similar results over two 10-year cycles (O’Donoghue et al., 1998; Studd et al., 2021, Studd, pers.comm.). These encouraging results with replication we must recognize do not recognize or include the non-lethal effects of predators on their prey (Boudreau et al., 2019; Lavergne et al., 2021). Measurement of functional and numerical responses outside of the laboratory is uncommon yet necessary to solve this problem. New technology involving GPS collars and accelerometers may help to answer these questions.
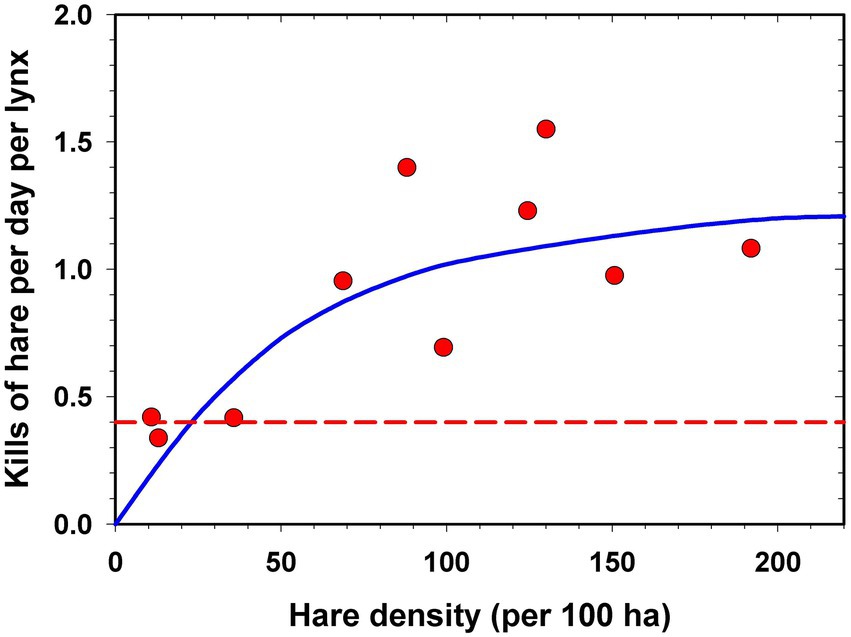
Figure 4. One example of a Type 2 functional response shown by Canada lynx to the density of snowshoe hares averaged over 10 winters at Kluane Lake, Yukon. The horizontal dashed line is the estimated energy requirement needs of an adult lynx per day in winter. These 10 data points originated from 2,232 km of snow tracking of individual lynx over these years. (From O’Donoghue et al., 1998).
I have spent over 50 years of research on the terrestrial arctic and the boreal forest ecosystems of northern Canada, and over that time many ecologists have studied and commented on the importance or lack of importance of predator–prey interactions. My impression is that the real world of understanding the impacts of multiple predators attacking prey like lemmings and snowshoe hares is slowly becoming visible, but there is still a gap between the conceptual models and the real-world impacts of predators on their prey. We need to move from averages over many predators to the details of how individual predators forage to further our understanding of the role of predation in population regulation (Studd et al., 2021).
These five thoughts presented here should be interpreted to mean we need much more research on both the theory and the reality of functional responses in many different ecosystems. This research however must be grounded in the problems that have been identified during the last 60 years that show how complex functional responses can be, how they can vary among individual predators, among different ecosystems, so that we cannot assume that one-size-fits-all. The interaction of food preferences of a particular predator, individual variation, chance, other species in the food web, and now changing climate will stimulate much more research on the broad issue of how predators interact with their prey, how flexible they are, and what new species interactions will impinge on what we now assume to be a stable community with stable interactions and constant functional relationships.
Author contributions
The author confirms being the sole contributor of this work and has approved it for publication.
Conflict of interest
The author declares that the research was conducted in the absence of any commercial or financial relationships that could be construed as a potential conflict of interest.
Publisher’s note
All claims expressed in this article are solely those of the authors and do not necessarily represent those of their affiliated organizations, or those of the publisher, the editors and the reviewers. Any product that may be evaluated in this article, or claim that may be made by its manufacturer, is not guaranteed or endorsed by the publisher.
References
Abrams, P. A. (2015). Why ratio dependence is (still) a bad model of predation. Biol. Rev. 90, 794–814. doi: 10.1111/brv.12134
Abrams, P. A., and Ginzburg, L. R. (2000). The nature of predation: prey dependent, ratio dependent or neither? Trends Ecol. Evol. 15, 337–341. doi: 10.1016/S0169-5347(00)01908-X
Barraquand, F. (2014). Functional responses and predator–prey models: a critique of ratio dependence. Theor. Ecol. 7, 3–20. doi: 10.1007/s12080-013-0201-9
Boudreau, M. R., Seguin, J., Boonstra, R., Palme, R., Boutin, S., Krebs, C. J., et al. (2019). Experimental increase in predation risk causes a cascading stress response in free-ranging snowshoe hares. Oecologia 191, 311–323. doi: 10.1007/s00442-019-04500-2
Chan, K. W., Boutin, S., Hossie, T. J., Krebs, C. J., O’Donoghue, M., and Murray, D. L. (2017). Improving the assessment of predator functional responses by considering alternate prey and predator interactions. Ecology 98, 1787–1796. doi: 10.1002/ecy.1828
Ginzburg, L. R., and Damuth, J. (2022). The issue isn’t which model of consumer interference is right, but which one is least wrong. Front. Ecol. Evol. 10:860542. doi: 10.3389/fevo.2022.860542
Graham, I. M., and Lambin, X. (2002). The impact of weasel predation on cyclic field-vole survival: the specialist predator hypothesis contradicted. J. Anim. Ecol. 71, 946–956. doi: 10.1046/j.1365-2656.2002.00657.x
Holling, C. S. (1959). The components of predation as revealed by a study of small mammal predation of the European pine sawfly. Can. Entomol. 91, 293–320. doi: 10.4039/Ent91293-5
Holling, C. S. (1961). Principles of insect predation. Annu. Rev. Entomol. 6, 163–182. doi: 10.1146/annurev.en.06.010161.001115
Kjellander, P., and Nordström, J. (2003). Cyclic voles, prey switching in red fox, and roe deer dynamics - a test of the alternative prey hypothesis. Oikos 101, 338–344. doi: 10.1034/j.1600-0706.2003.11986.x
Klemola, T., Tanhuanpaa, M., Korpimäki, E., and Ruohomaki, K. (2002). Specialist and generalist natural enemies as an explanation for geographical gradients in population cycles of northern herbivores. Oikos 99, 83–94. doi: 10.1034/j.1600-0706.2002.990109.x
Krebs, C. J. (2009). 'Ecology: The experimental analysis of distribution and abundance.’ 6th edn. (Benjamin Cummings: San Francisco.)
Krebs, C. J., Boutin, S., and Boonstra, R. (2001). Ecosystem dynamics of the boreal Forest: The Kluane project. Oxford University Press, New York.
Lambin, X., Petty, S. J., and MacKinnon, J. L. (2000). Cyclic dynamics in field vole populations and generalist predators. J. Anim. Ecol. 69, 106–119. doi: 10.1046/j.1365-2656.2002.00657.x
Lavergne, S. G., Krebs, C. J., Kenney, A. J., Boutin, S., Murray, D., Palme, R., et al. (2021). The impact of variable predation risk on stress in snowshoe hares over the cycle in North America’s boreal forest: adjusting to change. Oecologia 197, 71–88. doi: 10.1007/s00442-021-05019-1
Murdoch, W. W. (1969). Switching in general predators: experiments on predator specificity and stability of prey populations. Ecol. Monogr. 39, 335–354. doi: 10.2307/1942352
Murdoch, W. W. (1971). The developmental response of predators to changes in prey density. Ecology 52, 132–137. doi: 10.2307/1934744
Nicholson, A. J. (1933). The balance of animal populations. J. Anim. Ecol. 2, 131–178. doi: 10.2307/954
O’Donoghue, M., Boutin, S., Krebs, C. J., Zuleta, G., Murray, D. L., and Hofer, E. J. (1998). Functional responses of coyotes and lynx to the snowshoe hare cycle. Ecology 79, 1193–1208. doi: 10.2307/176736
Peers, M. J. L., Thornton, D. H., and Murray, D. L. (2012). Reconsidering the specialist-generalist paradigm in niche breadth dynamics: resource gradient selection by Canada lynx and bobcat. PLoS One 7:e51488. doi: 10.1371/journal.pone.0051488
Peers, M. J. L., Wehtje, M., Thornton, D. H., and Murray, D. L. (2014). Prey switching as a means of enhancing persistence in predators at the trailing southern edge. Glob. Chang. Biol. 20, 1126–1135. doi: 10.1111/gcb.12469
Roth, J. D., Marshall, J. D., Murray, D. L., Nickerson, D. M., and Steury, T. D. (2007). Geographical gradients in diet affect population dynamics of Canada lynx. Ecology 88, 2736–2743. doi: 10.1890/07-0147.1
Slough, B. G., and Mowat, G. (1996). Lynx population dynamics in an untrapped refugium. J. Wildl. Manag. 60, 946–961. doi: 10.2307/3802397
Smout, S., Asseburg, C., Matthiopoulos, J., Fernández, C., Redpath, S., Thirgood, S., et al. (2010). The functional response of a generalist predator. PLoS One 5, 1–7. doi: 10.1371/journal.pone.0010761
Solomon, M. E. (1949). The natural control of animal populations. J. Anim. Ecol. 18, 1–35. doi: 10.2307/1578
Studd, E. K., Derbyshire, R. E., Menzies, A. K., Simms, J. F., Humphries, M. M., Murray, D. L., et al. (2021). The purr-fect catch: using accelerometers and audio recorders to document kill rates and hunting behaviour of a small prey specialist. Methods Ecol. Evol. 12, 1277–1287. doi: 10.1111/2041-210X.13605
Studd, E. K., Menzies, A. K., Siracusa, E. R., Dantzer, B., Lane, J. E., McAdam, A. G., et al. (2020). Optimisation of energetic and reproductive gains explains behavioural responses to environmental variation across seasons and years. Ecol. Lett. 23, 841–850. doi: 10.1111/ele.13494
Keywords: population dynamics, functional responses, predator prey interactions, C.S. Holling, models in ecology
Citation: Krebs CJ (2022) Some historical thoughts on the functional responses of predators to prey density. Front. Ecol. Evol. 10:1052289. doi: 10.3389/fevo.2022.1052289
Edited by:
Thomas John Hossie, Trent University, CanadaReviewed by:
Peter Arnold Abrams, University of Toronto, CanadaLev Ginzburg, Stony Brook University, United States
Copyright © 2022 Krebs. This is an open-access article distributed under the terms of the Creative Commons Attribution License (CC BY). The use, distribution or reproduction in other forums is permitted, provided the original author(s) and the copyright owner(s) are credited and that the original publication in this journal is cited, in accordance with accepted academic practice. No use, distribution or reproduction is permitted which does not comply with these terms.
*Correspondence: Charles J. Krebs, krebs@zoology.ubc.ca