- Department of Integrative Biology, The University of Texas, Austin, TX, United States
Introduction: Evidence of animal personality and behavioral syndromes is widespread across animals, yet the development of these traits remains poorly understood. Previous research has shown that exposure to predators, heterospecifics, and urbanized environments can influence personality and behavioral syndromes. Yet, to date, the influence of early social experiences with conspecifics on the development of adult behavioral traits is far less known. We use swordtail fish (Xiphophorus nigrensis), a species with three genetically-determined male mating strategies (courtship display, coercion, or mixed strategy) to assess how different early-life social experiences shape adult behavioral development.
Methods: We raised female swordtails from birth to adulthood in density-controlled sexual-social treatments that varied in the presence of the type of male mating tactics (coercers only, displayers only, coercers and displayers, and mixed-strategists only). At adulthood, we tested females’ boldness, shyness, aggression, sociality, and activity.
Results: We found that the number of different mating strategies females were raised with (social complexity) shaped behavioral development more than any individual mating strategy. Females reared in complex environments with two male mating tactics were bolder, less shy, and less aggressive than females reared with a single male mating tactic (either courtship only or coercion only). Complex sexual-social environments produced females with behavioral syndromes (correlations between aggression and activity, shyness and aggression, and social interaction and activity), whereas simple environments did not.
Discussion: Importantly, the characteristics of these socially-induced behavioral syndromes differ from those driven by predation, but converge on characteristics emerging from animals found in urban environments. Our findings suggest that complexity of the sexual-social environment shapes the development of personality and behavioral syndromes to facilitate social information gathering. Furthermore, our research highlights the previously overlooked influence of sexual selection as a significant contributing factor to diverse behavioral development.
Introduction
Early social experiences are known to have powerful effects on adult physiology (stress-response, Murthy and Gould, 2018; Laubach et al., 2021) and behavior (e.g., mate preferences, ten Cate et al., 2006), and are expected to be important factors shaping a multitude of adult behaviors (Stamps and Groothuis, 2010a,b; Taborsky, 2016; Cabrera et al., 2021). Researchers have manipulated early-life experience with variation in food availability (Groothuis et al., 2005; Edenbrow and Croft, 2013), immune stress (Butler et al., 2012; DiRienzo et al., 2015), predation (Bell and Sih, 2007; Niemelä et al., 2012; Edenbrow and Croft, 2013; Castellano and Friard, 2021), heterospecific exposure (Delclos et al., 2020), social isolation (Naguib et al., 2011; Liedtke et al., 2015), and physical enrichment (Liedtke et al., 2015; Xu et al., 2021) —all showing that these developmental experiences can shape traits like boldness, activity, aggression, and exploration. These traits are often studied in the context of animal personality [i.e., consistent inter-individual variation in behavior (Bergmüller and Taborsky, 2010; Stamps and Groothuis, 2010a,b)] and behavioral syndromes [i.e., correlated behaviors across time and context (Sih et al., 2004a,b)]. Yet, there are few empirical studies that investigate how early-life social experiences affect the development of personality and behavioral syndromes in adults (Stamps and Groothuis, 2010a,b; Taborsky, 2016; Cabrera et al., 2021).
Surprisingly, one of the untapped areas of research into personality and behavioral syndromes is how they are shaped by social interactions between the sexes. Sexual behavior is known to lead to selection for different behavioral traits. For instance, in mating systems that rely on male coercion, females tend to adopt behavioral strategies to evade sexual harassment (Rowe et al., 1994; Pilastro et al., 2003; Darden and Croft, 2008; Dadda, 2015). Meanwhile, in mating systems that rely on male display or courtship, females adopt cooperative behaviors [e.g., visiting leks (Uy et al., 2001; DuVal, 2013; Rosenthal, 2017); solicitation displays (Searcy and Capp, 1997)]. Differences in mating systems and specific male mating tactics are expected to lead to changes in non-reproductive female behaviors such as shoaling (Magurran and Garcia, 2000) as well as cognition (Cummings, 2018). However, whether experience with different mating tactics influences the development of female personality or behavioral syndromes has not yet been investigated.
In addition, the complexity of the social environment itself may shape personality and behavioral syndromes. Social complexity has been hypothesized to drive the evolution of cognition (Dunbar, 1998) and communication (Freeberg et al., 2012), and correlates with brain evolution in primates (Dunbar, 2009), birds (Burish et al., 2004), and ants (Kamhi et al., 2016). How might complexity relate to personality and behavioral syndromes? It has been posited that in complex physical environments (Bengston et al., 2014) constraints on how individuals behave towards the complex environment have pleiotropic effects on other types of behaviors. Less is known about how social complexity affects these behavioral traits. At this point, we know that group-housed animals develop different behaviors as adults than those reared in isolation (Ballen et al., 2014; Liedtke et al., 2015). However, it may not simply be the presence or number of conspecifics that alters behavioral development, but the diversity of interactions individuals have with different types of conspecifics (Hobson et al., 2019).
Species with complex mating systems and alternative reproductive tactics allow us to begin to explore how specific social interactions alter behavioral development. Here, we manipulate lifetime social experiences (from birth to adulthood) of female El Abra swordtails (Xiphophorus nigrensis) to test how exposure to specific mating tactics (e.g., coercion, courtship display, or some combination of the two) as well as differing degrees of social complexity affect the development of personality and behavioral syndromes. Males of this species exhibit 1 of 3 genetically determined sexual phenotypes: large males are ornamented and court females with swimming displays, small males are drab and coerce females by chasing after them, and intermediate-sized males are moderately ornamented and exhibit a mixed strategy that includes both courtship and coercion (Ryan and Causey, 1989).
We raised female swordtails from birth to adulthood (generally >1 year) in one of five different social-rearing environments that maintained similar social densities but varied the combination of different male phenotypes in order to isolate the role of specific male tactics and the number of male tactics that females were exposed to in shaping female personality and behavioral syndromes. We ask whether females raised in different sexual-social environments (i) differ in their activity, sociability, boldness, shyness, and aggression; (ii) whether these behaviors are repeatable (form personality traits); and (iii) whether they correlate with one another (form a behavioral syndrome). Furthermore, our design allows us to compare the effects of (iv) specific sexual-social experiences (courtship only vs. coercion only experiences), and (v) simple relative to complex mating environments (e.g., treatments with a single mating tactic vs. treatments with two mating tactics) on the development of personality and behavioral syndromes.
Materials and methods
Sexual-social rearing treatments
We raised female Xiphophorus nigrensis from birth to adulthood (1–1.5 years) in five socially controlled rearing treatments followed by behavioral testing in early adulthood. Experimental females were first introduced into experimental 30 gallon aquaria as fry (<10 mm) from broods produced in community tanks with individual broods split across all treatments to control for genetic effects. The 15 experimental aquaria (5 treatments x 3 replicates each) were initially stocked with 30–40 fry of unknown sex along with 4 adult model females (identified with elastomer tags) and 4 adult males (see below for more details). Fish were fed Cargill and TetraMin flakes once daily and were supplemented with brine shrimp periodically. Tanks were enriched with plastic plants, flower pots, and gravel, and were illuminated with full spectrum aquarium lights. Experimental tanks were visually inspected 3 times a week to quantify juvenile densities and remove developing males. Developing males can be identified by a thickening of the anal fin as it develops into a gonopodium. Juvenile densities increased over time due to additional brood production by adult model females; and fluctuations in tank densities over time were due to changes in births, deaths, and removal of developing males. To minimize the variation in social densities across treatments and replicates over time, fry would be added to or removed from replicate tanks to maintain similar densities. Adult male stimuli were also moved between replicate tanks within a social treatment every 3 months to ensure developing females experienced multiple individual males of a given phenotype.
Our experimental females were reared in one of five social rearing treatments that varied by the type of male sexual behavior females experienced. The rearing treatments varied by the different types of stimulus males present: large courtship displaying males only (D), small coercive males only (C), mixed strategy intermediate-sized males only (M), combination of small coercive and large displaying males (C&D), and females only (F). To control for adult density, each tank had 8 stimulus adults including four male types described above (D, C or M males) and four adult model females. The C&D group contained two small, coercive males and two large, displaying males. The M, D, and C groups each had 4 males of their respective phenotype (see Figures 1A–D); and the F group had 8 adult model females. Adult stimulus (or model) females were selected from a community tank where they had experience with all types of X. nigrensis males and served as social learning models for developing females. We selected large adult females (usually >30 mm) to serve as models in our treatment tanks as avoidance of small males and preference for courting males increases with female size which is a proxy for age (Rios-Cardenas et al., 2007; Wong et al., 2011), with preference for associating with large courting males existing even in the absence of coercive males (Reding and Cummings, 2017). Adult model females were dorsally tagged with white elastomer markings to differentiate them from the developing experimental females. It takes approximately one year for X. nigrensis females to reach sexual maturity, and sexually mature females are distinguishable from immature females by a melanized brood patch on the ventral area near the gonopore. We maintained experimental females in treatment tanks for 15–18 months in order to characterize developmental effects from the combined influences of social learning (from observing model females) and their own direct sexual-social experiences as adults. As mate choice copying has been documented in other poeciliids (Dugatkin and Godin, 1993; Briggs et al., 1996), we expect social learning of mate preferences to strongly shape developing female preferences in adulthood (Dugatkin, 1996; Davies et al., 2020). We conducted multiple rounds of this social rearing experiment from mid-2016 to 2019. Juvenile females that were introduced into experimental tanks in mid-2016 were tested as adults in 2018, and juvenile females introduced into social treatments in 2017–2018 were tested as adults in 2019.
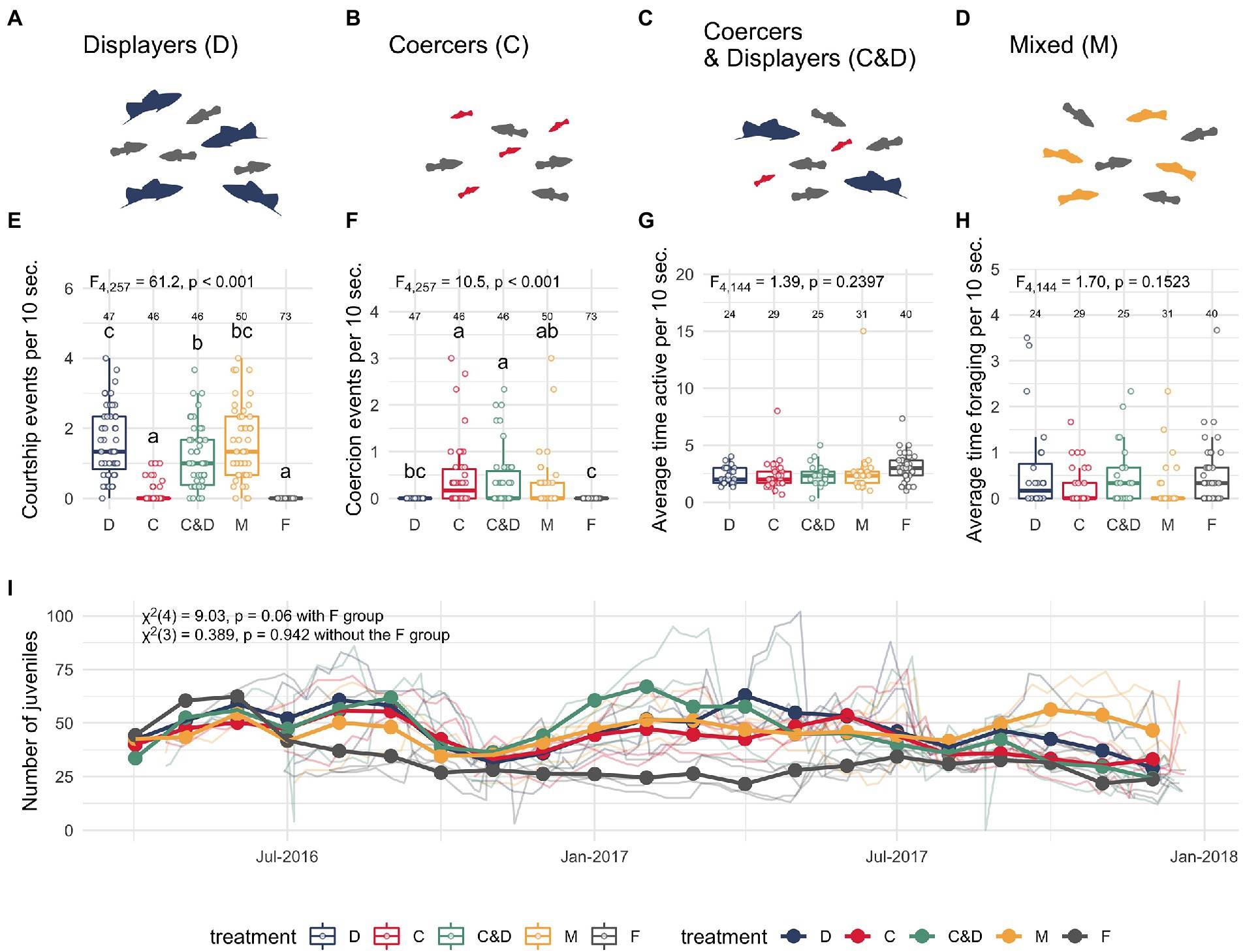
Figure 1. Social rearing environment conditions. Schematic of different social rearing environments: D, displayers only (A); C, Coercers only (B); C&D, coercers and displayers together (C); M, mixed strategy males (D); and F, female only environments (not shown). Females from different rearing environments experienced different rates of male courtship (E) and coercion (F), while exhibiting no differences in their activity (time spent moving) (G) or foraging (H) rates. Box and whisker plots show median (center line) and interquartile range. Letters demonstrate similarity from Tukey post hoc analysis. Data were from 411 video clips (10 s each) collected from the treatment tanks during the developmental rearing period (> 1 year) prior to behavioral testing of the experimental females at adulthood (number of videos per treatment shown above box and whisker plots). Weekly density measurements across replicate tanks for each treatment (I) reveal that females in the F (female only treatment) showed a near-significant difference in juvenile densities over time relative to the male-exposed treatments (p = 0.06). Excluding the female-only treatment group revealed that females from male-exposure treatments (D, C, C&D, and M) experienced similar density levels over time (p = 0.94). Bold colored lines represent the average densities across replicate tanks within a social treatment: (D) blue, (C) red, (C&D) green, (M) yellow, (F) grey, while faint lines show density counts from individual replicate tanks (represented as lighter colors than the treatment average line).
To quantify the sexual-social interactions females from different social treatments experienced during their developmental period, we recorded weekly 15 min video recordings of all social rearing tanks between March 2016 to June 2017 for a total of 149 videos. From each video, we selected three 10 s video clips (from 1, 6, and 11 min. timestamps) that were then hand-scored by 7 undergraduate researchers naïve to the studies objectives (for a total of 411 scored video clips). Observers scored rates of coercive chases and courtship displays oriented toward adult females in each treatment group, along with average number of female foraging bouts and activity levels (time spent moving) across the rearing treatments. As expected in a system with genotypically-determined alternative male reproductive strategies, we were able to verify that females experienced different rates of coercion and courtship attempts across the different treatments (Figures 1E,F). However, we were not able to quantify adult female responses to these attempts (e.g., successful copulations) due to constraints of the video-recordings (see Supplemental videos 1–5 for representative videos of our social treatment tanks). Nonetheless, an extensive literature on Xiphophorus female preferences for large displaying males and aversion of small coercive males leads us to reasonably expect female responses in our experiment to be consistent with patterns of past research (Ryan and Causey, 1989; Cummings and Mollaghan, 2006; Rios-Cardenas et al., 2007; Wong et al., 2011; Reding and Cummings, 2017).
Behavioral assessment
After experimental females reached adulthood, we evaluated their shyness, boldness, aggression, sociability, and activity levels by testing them in 3 assays (scototaxis, mirror aggression, and shoaling assays described below, see Table 1 for information on which behaviors came from which assays). In total, we tested 60 experimental females with sample sizes from each treatment including: D, display-only (n = 15); C, coercive-only (n = 9); C&D, coercive and displayers (n = 20); M, mixed-strategy (n = 10); and F female-only (n = 6). For information on sample sizes from replicate tanks see Supplementary Table S1 (Supplementary Information). We used the initial trials from all experimental females to evaluate how treatment influenced specific behaviors. To assess consistency of these behavioral traits (e.g., personality), we measured repeatability of these behaviors from experimental fish tested twice on each of the three assays across a 17 day period with an interval of 14 days between scototaxis trials, 13 days between mirror/aggression trials, and 16 days between sociability trials. After removal from treatment tanks for testing, focal females were individually-housed in adjacent 25 by 24 by 30 cm aquaria that allowed fish to see and interact with the neighboring fish but remain separate for continuous identification of focal fish. The visual social interaction was intended to reduce any stress associated with social isolation. We ran repeat assays for 60 fish with initial sociability assay run on Day 1 while the initial mirror and scototaxis trials run on Day 2 with a minimum resting interval of 2 h between their 1st (aggression) and 2nd (scototaxis) assay of the day (see Supplementary Table S2 in Supplementary Information for complete schedule). For 18 fish, we ran females through the three assays only once (with 12 following the same initial assay schedule as above, and 6 fish having longer intervals between trials: Day 1 sociability, Day 2 aggression; Day 4 scototaxis). Due to technical problems with videos, along with some mortality of our experimental fish (n = 4), our final samples sizes for initial behavioral trials included sociability (n = 54), scototaxis (n = 58), and aggression (n = 54); and final sample sizes for repeat trials included sociability (n = 41), scototaxis (n = 41), and aggression (n = 26). All videos were recorded using Nikon D3300 cameras with 18-55 mm lenses.
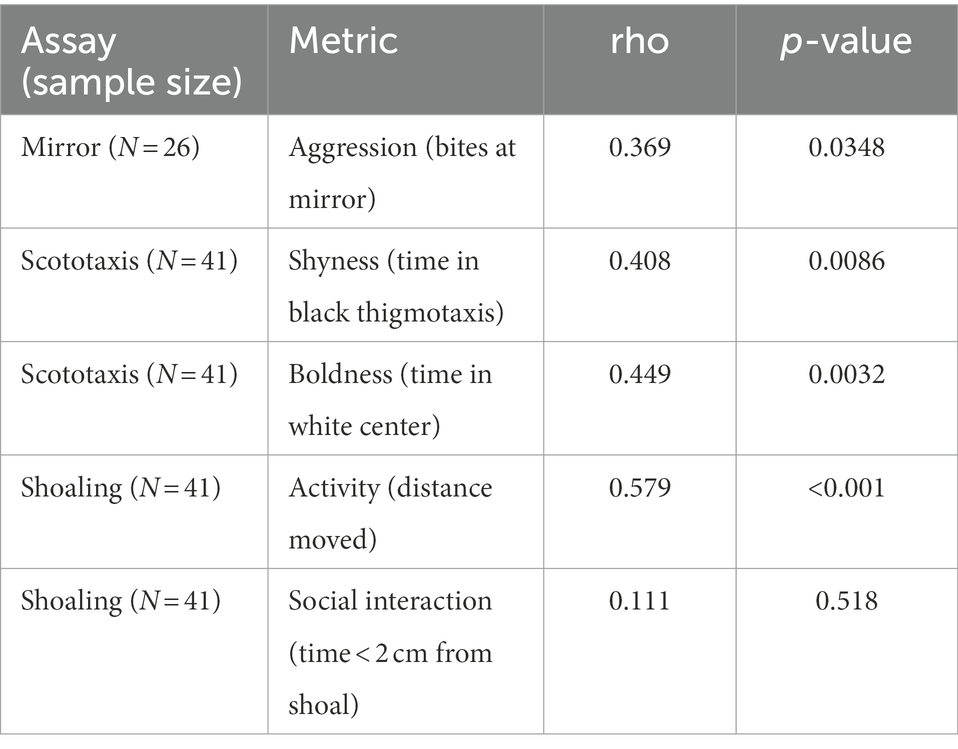
Table 1. Spearman’s rank correlation between the two repeat measurements for each behavior from each assay.
Scototaxis
We measured shyness and boldness in females using a scototaxis assay (Maximino et al., 2010). Our scototaxis tank involved a 25 × 50 cm aquarium with one half lined with white felt and the other half lined with black felt (Figure 2A). The black side is a darker environment and can serve as a refuge, while the white side is more exposed and activities in this region have been associated with boldness (Maximino et al., 2010; Ramsey et al., 2014; Etheredge et al., 2018). We evaluated ‘shyness’ as time spent in the edges of the black half (black thigmotaxis) as it represents the most sheltered portion of the tank, and we characterized ‘boldness’ as the time spent in the white center because it represented the most exposed zone (Figure 2A). To start the trial, the tank is filled with 10 cm of water and the overhead fluorescent lights turned on. The fish is then introduced to the center of the arena and constrained to a 4 cm space by white barrier on the white side and a black barrier on the black side. After a 5 min habituation period, the barriers are removed and the fish’s behavior was recorded for 10 min. Full water changes were conducted between experimental fish to control for accumulation of cortisol in the water.
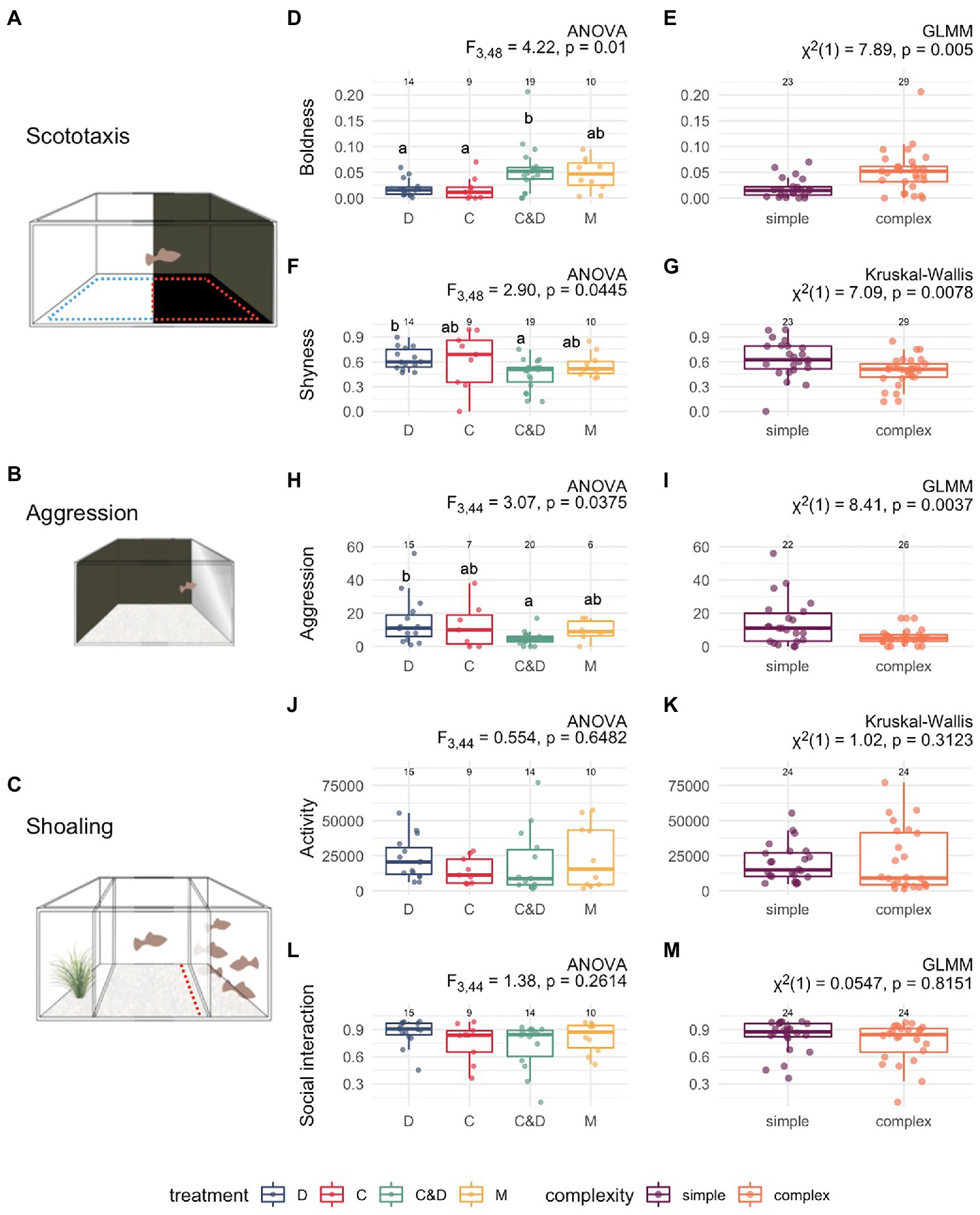
Figure 2. Behavioral effects of social rearing environment on adult behavior. At adulthood, females were tested in (A) scototaxis, (B) aggression, and (C) shoaling assays. We measured (D) boldness (white center, proportion of time in white center—denoted by dashed blue line), and (F) shyness (black thigmotaxis, proportion of time on black side within 2 cm of wall—denoted by dashed red line) in the scototaxis assay. We assessed (H) aggression as the number of bites at the mirror; and activity (J) as the total movement (pixels traversed on video screen: arbitrary units) during the shoaling assay; and social interaction (L) as the proportion of time individuals spent in the social interaction zone [2 cm from barrier, see red line in (C)]. We used ANOVA to test for differences across groups for each behavioral metric with associated p value shown for each test, and significant differences between pair wise comparisons using Tukey-post hoc test denoted with different letters above each treatment group. We further examined behavioral developmental differences by social complexity (E,G,I,K,M) by using GLMM (and Kruskal–Wallis for non-normal data) between “simple” (pooled rearing environments with a single male mating tactic (C,D) shown in purple) and “complex” (pooled rearing environments with two male mating tactics (C&D, M) shown in orange).
To quantify boldness and shyness in this assay, we divided the tank into 4 zones: black center, white center, black thigmotaxis (2 cm nearest the walls on the black side, see red dashed area in Figure 2A), and white thigmotaxis (2 cm nearest the walls on the white side, see blue dashed area in Figure 2A). We superimposed grids onto scototaxis videos using Microsoft Powerpoint and iMovie to create the 4 zones and time spent in each zone was scored using cowlog (Hänninen and Pastell, 2009) by a trained undergraduate (RB). Video names were blinded such that the scorer was uninformed which treatment the subject female was raised within.
Mirror assay
We tested females’ aggression towards their mirror image following a similar protocol to other poeciliid studies (Franck et al., 1985; Moretz and Morris, 2003; Wilson et al., 2011). Three sides of a 28 cm x 9 cm tank were lined with grey felt and a mirror was secured to the unfelted end (Figure 2B), with a video camera recordings collected directly above the tank. A focal female was introduced to a PVC tube on the opposite end as the mirror for a 5 min habituation period. Afterwards, the PVC tube is removed and the fish allowed to explore the tank for 5 min with an opaque barrier covering the mirror end (movement control). The fish is then gently constrained back into the PVC tube for 1 min, during which the opaque barrier is removed and the mirror exposed. After 1 min, the camera is turned on and the trial recorded for 5 min. All water is changed between fish to control for accumulation of cortisol. A single trained undergraduate (NJ) scored all videos for aggressive behavior towards the mirror by counting the total number of bites focal fish directed toward their mirror image. Video names were blinded such that the scorer was uninformed which treatment the subject female was raised within.
Shoaling assay
We measured two behaviors from the shoaling assay: activity and sociability. Activity was measured in the shoaling assay because it was the least stressful assay and movements in less risky environments are considered to be better measures of general activity that are unconfounded by stress (Brown et al., 2005; Etheredge et al., 2018). The assay was conducted in a rectangular glass aquarium measuring 120 cm in length and 30 cm in width and filled with water to a height of 18 cm (Figure 2C). The walls of the aquarium were lined with blue felt to reduce stress and maximize video tracking success. The felt also prevents the fish from becoming distracted by its reflection in the glass. A clear plexiglass partition was inserted 24 cm from the wall on each side of the tank (lengthwise), creating a 72 cm arena between the barriers. The tank was illuminated by four fluorescent aquarium bulbs covered with teal and dark blue filters. A shoal group, consisting of 5 females was placed on the side of the glass partition opposite of the focal fish and allowed 30 min to acclimate prior to testing. On the other side of the tank, an artificial plant was inserted on the same side as the focal fish, and the glass partition covered with brown felt opposite the plant. Between tests, the shoal side and plant side were switched to prevent side biases.
To begin each assay, the focal fish was placed in a 10 cm diameter PVC habituation tube for 5-min, and then released and recorded for 10-min. Following this initial observation period, black felted plastic dividers were used to corral the focal fish back into the center of the arena. The fish was then held between the dividers (separated by 10 cm) for an additional 5-min habituation period. During this time the shoal of five females was moved to the opposite side of the aquarium and vice versa with the plant. The black felted dividers were then removed, and video recordings conducted again for 10-min. Focal fish were then subsequently returned to their home aquaria.
We analyzed the video recordings using EthovisionXT15 (Noldus) behavioral tracking software to quantify time spent 2 cm adjacent to the plexiglass partition on the shoal side (see red dashed line in Figure 2C). We quantified female behaviors during the middle 5-min portion for each 10 min observation period for a total of 10 min of behavioral tracking per subject (5 min from each observation period). Tracking began approximately 2 min after the start of each observation period to allow fish time to adjust to the new environment following removal of the habituation tube or black felted dividers. For each observation period, we collected total time spent in the social interaction zone as well as total distance moved as a measure of activity.
Statistics
All analyses were conducted in R version 4.1.2. To test for personality, we used Spearman’s Rank correlation to test for repeatability between first and second measures of each behavior across the females that experienced repeat scototaxis, aggression and shoaling assays (Nakagawa and Schielzeth, 2010). To test for effects of rearing environment on behavioral development, we used the initial measurements for all subject females’ behavioral data as input into a Principal Component Analysis (PCA). To understand how rearing environment affected the mean values of each behavior, we also used ANOVA, using social treatment as the explanatory variable and behavioral response as the response variable. To evaluate whether mating system complexity drove differences in behavioral development, we combined measures from simple mating environments (treatments with only 1 male tactic: C and D) and combined measures from complex mating environments (treatments with 2 male tactics: C&D and M). We used generalized linear mixed effect models (GLMMs) with complexity as a fixed effect and treatment nested within complexity as a random effect to control for the non-independence between observations from treatment within each level of complexity. We also included replicate tank ID as a random effect to control for tank effects. Our complexity GLMM model did not converge for shyness and activity data, so for these analyses we used a non-parametric Kruskal-Wallis test. To evaluate behavioral syndromes (correlations between different behavior measures), we used bivariate correlation matrices as well as PCA coupled with ANOVA to test whether differences in loadings on each of the first two axes of variation differed across social complexity levels.
Results
Sexual-social rearing conditions
Video recordings collected during the developmental period from the experimental tanks revealed that females from different rearing environments experienced different levels of courtship (Figure 1E, ANOVA: F = 61.2, p < 0.001) and coercion (Figure 1F, ANOVA: F = 10.5, p < 0.001). Tukey post hoc analysis reveals that females raised in the D, M, and C&D social treatments experienced higher courtship than females raised in C and F treatments. Conversely, females raised in C, M, and C&D treatments experienced more coercion than females raised in D and F treatments, as expected. We also ran these analyses without the F treatment to make sure the absence of courtship and coercion in these females did not skew our results. Removal of this treatment confirmed that the male-exposed rearing environments differed in the amount of courtship (ANOVA: F = 28.8, p < 0.001) and coercion (ANOVA: F = 5.91, p < 0.001). Tukey post hoc analysis without the F-females reveals females raised in D, C&D, and M treatments experienced more courtship than females reared in C treatments, with no difference in courtship rates between D, C&D, and M females. Conversely, females raised in C treatments experienced more coercion than females in M or D, but not C&D treatments (See Supplementary Figure S1). Measurements of adult female models in each of the social treatments (while swimming freely within these experimental tanks) exhibited no significant difference in general activity (e.g., time spent moving, Figure 1G, p = 0.2397) or foraging rates (Figure 1H, p= 0.1523).
Density measurements (n = 1,184 tri-weekly counts over 18 months across replicate tanks) of juveniles in the rearing tanks showed that females reared in different sexual-social treatments experienced similar levels of social densities across their 13–15 month developmental period (Figure 1I). To assess whether treatments differed in juvenile density, we used linear mixed effect models using lme4 package in R with treatment as a fixed effect. Across all treatments (C, D, C&D, M and F groups), there was a borderline statistically significant effect of treatment on juvenile densities (Χ2 = 9.03, df = 4, p = 0.060 across all treatments), though this effect was mainly due to the female-only group (F, see Figure 1I, Χ2 = 0.39, df = 3, p = 0.94 for only male-exposed treatments). Multiple regression analysis revealed no significant difference in slopes between treatments (p value of slope estimated differences for each treatment relative to the C social rearing group: p = 0.727 (C&D), p = 0.601 (D), p = 0.668 (M), and p = 0.246 (F)).
Personality
Following the developmental rearing period (13–15 months), experimental females were assessed in repeat trials of individual aggression, scototaxis and shoaling assays that revealed significant correlations across trials for some behavior metrics. We found that bites (rho = 0.37, p = 0.03), shyness (rho = 0.40, p = 0.008), boldness (rho = 0.45, p = 0.003), and activity (distance traveled in shoaling assay, rho = 0.58, p < 0.0001, Table 1) exhibited significant correlations across repeat trials. However, we found no statistically significant correlations between scored social interaction time between repeat shoaling assay trials (Table 1).
Sexual-social rearing environment shaping behavioral traits
Given the female-only social treatment had the smallest sample size (n = 6) and a marginally significant trend for lower densities (Figure 1I), we excluded the female-only group from subsequent analyses. Restricting our analyses to male-exposed rearing treatments (D, C, C&D, and M) enables us to compare how exposure to diverse mating types during development influences female behavioral development, while developing a balanced comparison between groups that involved a single mating tactic (C, D treatments) and groups that involved two mating tactics (C&D, M treatments). Comparisons including the F group can be found in Supplementary Figure S2 in Supplementary Information.
Boldness and shyness
The sexual-social rearing environment had significant effects on boldness (proportion of time spent in white center, Figure 2D, ANOVA: F = 4.22 p = 0.01) and shyness (proportion of time spent in black thigmotaxis zone; Figure 2F, ANOVA: F = 2.90, p = 0.04). Tukey post hoc analysis shows that females raised in the C&D treatment were bolder than females in the C (p = 0.04) and D (p = 0.02) treatments. In addition, C&D females were less shy than D females (p = 0.05). When pooling treatment groups based on the number of mating tactics females were exposed to, we found that females reared with two mating tactics (complex environments: C&D and M treatments) were significantly bolder (Figure 2E: GLMM: Χ2 = 7.89, p = 0.005) and less shy (Figure 2G: Kruskal-Wallis: Χ2 = 7.09, p < 0.008) than females raised with only a single mating tactic (pooled C and D treatments).
Aggression
We found that sexual-social rearing experience affected intrasexual aggression (number of bites performed at mirror, Figure 2H, ANOVA: F = 3.07, p = 0.04). Tukey post hoc analysis shows females experienced with courtship displaying male types only (D treatment) showed significantly higher number of bites than C&D females (p = 0.03). Overall, females from multiple male tactic environments (complex environments) performed fewer bites than females from single male tactic environments (simple environments; Figure 2I, GLMM: Χ2 = 8.41, p = 0.004).
Activity
There were no significant differences across treatments in the distance moved during the sociability assay (Figure 2J, ANOVA: F = 0.55, p = 0.65), nor were there differences between simple and complex groups (Figure 2K, Kruskal-Wallis: Χ2 = 1.02, p = 0.31).
Sociability
There were no significant differences across treatments in the amount of social interaction (Figure 2L, ANOVA: F = 1.38, p = 0.26), nor were there differences between simple and complex groups (Figure 2M, GLMM: Χ2 = 0.05, p = 0.82).
Sexual-social-rearing environment shaping behavioral syndromes
When we explored individual bi-variate correlations across the five behavioral metrics, we found several significant correlations affected by social rearing conditions (Figure 3). The one consistent trend shared across all treatment groups was a negative relationship between shyness and boldness (e.g., spearman correlation rho = −0.86, p = 0.003 for C females (Figure 3B); rho = −0.7, p = 0.031 for M females (Figure 3D); with similarly non-significant trends in D (p = 0.08, Figure 3A) and C&D (p = 0.13, Figure 3C) females). This correlation is expected because these traits represent opposing ends of the boldness/shyness spectrum, but we interpret this correlation with caution due to the non-independence of these measures that come from the same assay. However, we observed variation in correlations between behaviors measured in different contexts based on sexual-social rearing environment. Among females reared with displaying courters (D females), we found no significant correlations between behavioral traits across contexts. Females raised with coercive males only © had a positive trend between social interaction and aggression (Figure 3B, rho = 0.74, p = 0.058). Meanwhile, females raised with both types of phenotypes (C&D) revealed a positive correlation between aggression and shyness (Figure 3C, rho = 0.48 p = 0.039). And females raised with mixed strategy males (M females) and both of these phenotypes (C&D females) exhibited a positive correlation between activity and social interaction (Figure 3D, rho = 0.78, p = 0.012; Figure 3C, rho = 0.56 p = 0.038).
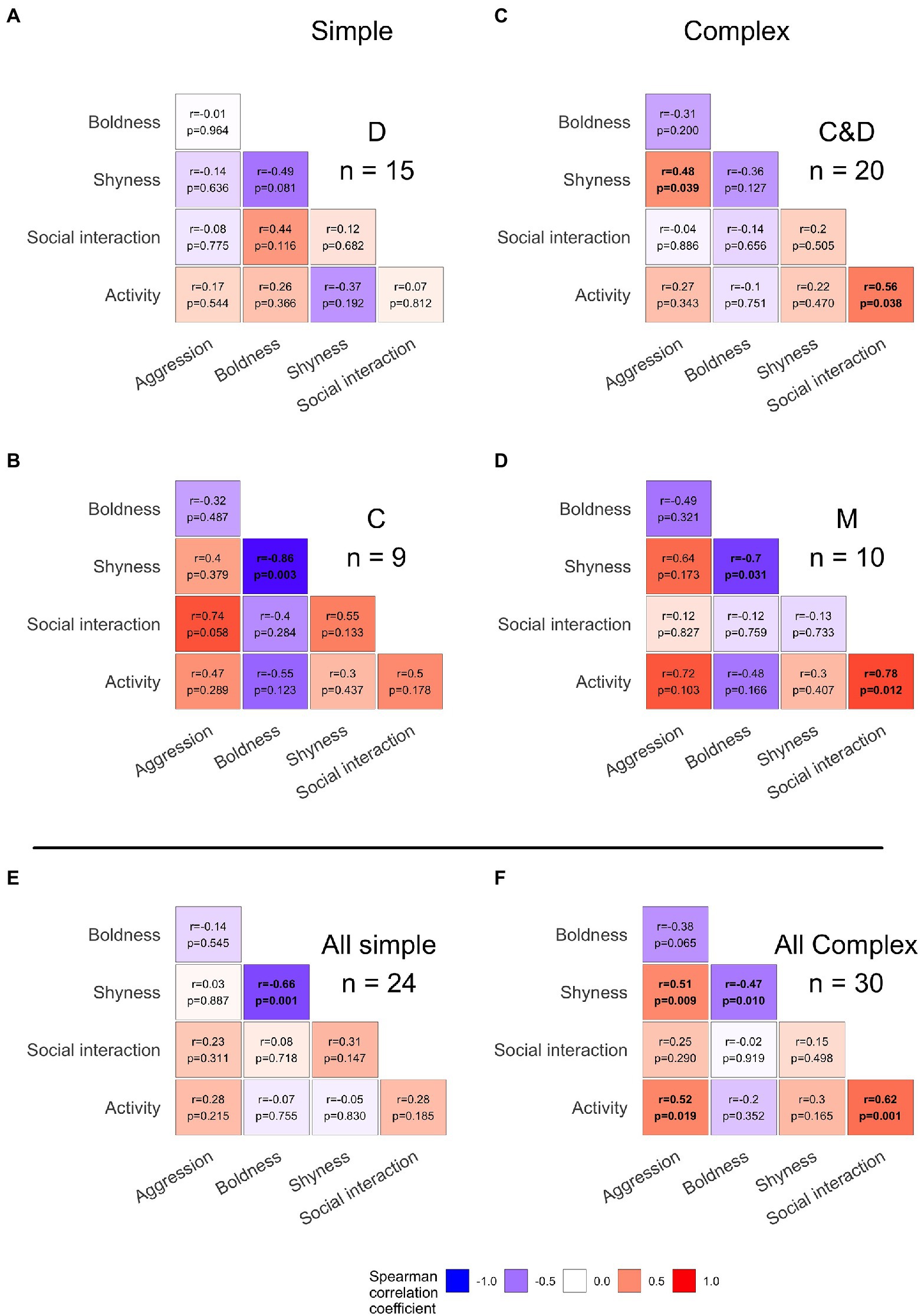
Figure 3. Heatmap of behavioral correlations by treatment and complexity. Spearman’s rank correlation of behaviors by treatment (A–D) and sexual-social complexity (E,F) with negative correlations shown in cooler colors and positive correlations in warmer colors. Significant spearman’s rank correlations (p < 0.05) are denoted with bold font. A significant or near-significant trend for a negative relationship was observed between shyness and boldness which is expected given the non-independence of these two behavioral characteristics (measured within the same assay). Evidence for behavioral syndromes (correlations of behaviors measured between contexts, sensu Sih et al., 2004b) were found only in complex sexual-social rearing environments [(F), aggression and shyness (p = 0.009), aggression and activity (p = 0.019), activity and social interaction (p = 0.001), and near-significant boldness and aggression (p = 0.065)] but not in simple sexual-social rearing environments (E).
More broadly, we found that females reared in environments with two mating tactics showed multiple significant correlations between behaviors whereas females reared in simple environments with a single mating tactic largely did not. Pooling females from simple sexual-social environments (C and D treatments) showed the expected negative correlation between boldness and shyness (Figure 3E, rho = −0.66, p = 0.001). Meanwhile, pooling females from complex sexual-social environments with two mating tactics (C&D and M treatments) showed positive correlations between aggression and activity (Figure 3F, rho = 0.52 p = 0.019), aggression and shyness (rho = 0.51 p = 0.009), social interaction and activity (rho = 0.62 p = 0.001), along with a near-significant negative correlation between boldness and aggression (rho = −0.38, p = 0.065) and the expected negative correlation between shyness and boldness (rho = −0.47, p = 0.01).
Principal component analysis
Incorporating all behavior metrics as inputs into a PCA, we found that the number of mating tactics females were exposed to during development significantly predicted individual variation along the primary axis in this multidimensional reduction (Figure 4A). The first two principal components explain 66.79% of the multivariate variation (PC1 = 42.86%, PC2 = 23.93%). PC1 primarily captures variation associated with boldness, shyness and aggression while PC2 captures variation associated with social interaction and activity (Figure 4B). Females from complex sexual-social environments (two mating tactics) had significantly higher PC1 scores than females from simple, single mating tactic environments (Figure 4C, ANOVA: F = 14.32, p = 0.0006). There was also a non-significant trend for females from complex environments to score higher on PC2 than those from simple environments (Figure 4D, ANOVA: F = 2.689, p = 0.0835).
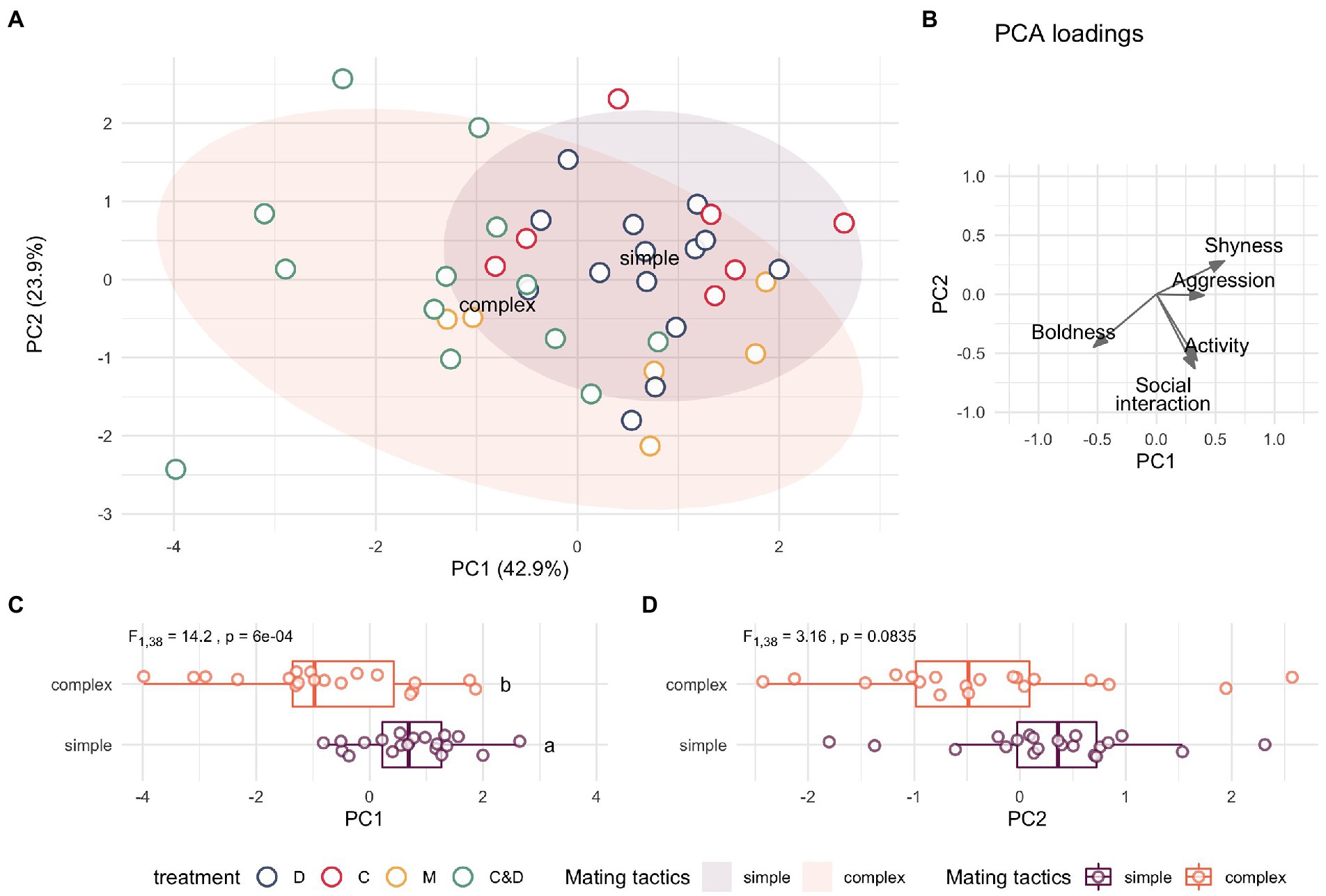
Figure 4. Multivariate analysis of behavioral traits by social complexity. Principal component analysis (PCA) including all 5 behavioral metrics revealed females reared in simple (purple) and complex (orange) mating environments show behavioral differences in multidimensional space. (A) The first two principal components explain 66.79% of the multivariate variation, with PC1 explaining 42.9% of the variance. Points are colored by treatment and ellipses represent 95% confidence intervals. (B) Vector-plot of PC loadings reveal that boldness, shyness, and aggression load most prominently on PC1, whereas activity and shoaling tendencies load more strongly onto PC2. (C) An ANOVA reveals females from complex environments (C&D and M groups) score lower on PC1 than females from simple environments (C and D groups, p = 0.0006); with (D) non-significant trend for complex-reared females to have lower PC2 scores than simple-reared females (p = 0.08).
Discussion
In this study, we successfully created discrete sexual-social experiences that females encountered for the entirety of their developmental period (from parturition to adulthood) by manipulating female exposure to different combinations of alternative male reproductive phenotypes. Importantly, females raised in these different social groups experienced similar densities (Figure 1I), enabling us to examine the effect of male mating tactic and sexual-social complexity on female development independent of social density effects.
Personality
We found that aggression, activity, boldness, and shyness were stable over repeat measurements (Table 1). These findings demonstrate that X. nigrensis possess similarly stable personality traits as have been reported in other poeciliids. For instance, aggression (Wilson et al., 2011), boldness (Boulton et al., 2014), activity (Cote et al., 2010; Biro and Adriaenssens, 2013; Blake and Gabor, 2014), and exploration (Blake and Gabor, 2014; Heinen-Kay et al., 2016) have been established as personality traits in other swordtail and mosquitofish species. Variation in some of these personality traits such as boldness and aggression has previously been shown to correlate with predation (Huntingford, 1976; Bell, 2005; Dingemanse et al., 2007; Blake and Gabor, 2014; Heinen-Kay et al., 2016; Castellano and Friard, 2021), but this is among the first to explore how specific social experiences shape the development of these traits.
Sexual-social rearing environment shaping personality
We show that social experience during ontogeny leads to long lasting and repeatable changes in personality during adulthood, adding to a growing body of literature on the ontogeny of personality (Stamps and Groothuis, 2010a,b; Hedrick, 2017; Cabrera et al., 2021). Importantly, we quantified behavior in the rearing environment (Figure 1) as well as during adulthood allowing a direct comparison of what type or combination of early-life experiences affect adult personality (Taborsky, 2016). Why would developmental exposure to both courtship and coercion lead to bolder and less aggressive females? One possible interpretation is that responding to multiple male sexual behaviors is more cognitively challenging than responding to males that predictably employ the same tactic. In a complex sexual-social environment, an increase in boldness and a reduction in aggression may increase females’ exposure to important social information and thereby allow females to appropriately respond. Boldness may be required to explore the sexual-social environment, while too much intrasexual aggression may detract attention away from male behavior and thereby decrease information gathering related to male conspecifics. Our results are consistent with findings in other taxa where social isolation leads to lower exploration in individuals relative to individuals raised with others (Liedtke et al., 2015). Indeed, boldness has been shown to positively correlate with a number of cognitive traits such as spatial learning in lizards (Carazo et al., 2014) and learning speed in chickadees (Guillette et al., 2009). Access to information likely explains differences in aggression in dominance hierarchies (Hobson, 2020), and our results suggest that aggression may be modulated to increase social information gathering when social contexts are complex. In this study, experience with multiple male mating behaviors leads to bolder and less aggressive females, which may lead to increased information acquisition.
Sexual-social rearing environment shaping behavioral syndromes
Why might behavioral syndromes emerge from a complex, but not simple, mating environment during development? According to the social niche specialization hypotheses, behavioral syndromes may emerge from animals optimizing their behavior in a socioecological context through state-behavior feedbacks (Bergmüller and Taborsky, 2010; Sih, 2011; Sih et al., 2015). Perhaps sexual-social complexity that reflects the natural social conditions of X. nigrensis requires some constraint on the development of female behavior. If, for example, an increase in female boldness in response to social complexity aids in information processing and improved social decision making, behaviors such as aggression might be constrained to lower levels to facilitate this relationship. In support of this view, social complexity is associated with increased social competence across taxa (Taborsky and Oliveira, 2012). Female behavior from a simple social environment may be less constrained, with reduced pressure to form behavioral syndromes. In addition, research on pace-of-life syndromes suggests ecological conditions drive differences in resource allocation to survival and reproduction that may also affect personality and behavioral syndromes (Polverino et al., 2018). In this experiment, social complexity may have shaped female behavior through its effect on pace-of-life syndromes. Physical complexity in the rearing environment has been shown to affect behavioral syndromes (Liedtke et al., 2015; Xu et al., 2021), and here we show that sexual-social complexity is also an important factor in shaping behavioral syndromes.
Previous research in sticklebacks (Bell and Sih, 2007; Dingemanse et al., 2007) has shown that exposure to predators leads to the formation of behavioral syndromes, whereas predator-naïve sticklebacks lack behavioral syndromes. Here we find that exposure to multiple mating tactics leads to the formation of female behavioral syndromes, which are absent in females from environments with lower mating tactic complexity. Our results suggest that sexual selection pressures might be just as powerful as natural selection pressures at forming behavioral syndromes. Interestingly, the correlations we report are in opposite directions as what is commonly reported in studies where predation pressure is implicated in behavioral syndrome formation (Huntingford, 1976; Bell, 2005; Dingemanse et al., 2007). Whereas many predation studies report a positive correlation between boldness, aggression and activity in focal prey taxa, we found negative correlations between activity and aggression and between aggression and boldness in females raised with multiple male mating tactics. This unusual behavioral syndrome in females reared in complex mating environments may reflect a constraint females face in the more complex social environment that link together sets of behaviors possibly involved in social information gathering. Interestingly, this same pattern of low aggression linked with high boldness is found among animals experiencing urbanization [e.g., northern cardinals (Huang et al., 2020), lizards (Batabyal and Thaker, 2019) and several other taxa (Sadoul et al., 2021)]. Researchers have suggested that boldness may emerge in the more complex urban environments as a result of habituation to humans (Samia et al., 2015); and that lower aggression may facilitate greater cognitive flexibility required in these more complex urban environments (Sadoul et al., 2021). The convergence of the same behavioral syndrome traits emerging from socially complex conspecific environments as those that emerge from exposure to humans may suggest that complexity in a number of different forms can lead to selection for a specific suite of behavioral traits.
An alternative, non-mutually exclusive, explanation for how the social environment affects the development of behavioral syndromes is that diverse social experiences represent different social stressors that produce different coping styles. Coping styles refers to variation in how individuals respond behaviorally and physiologically to stressful events with ‘proactive’ animals tending to be more bold and aggressive, and ‘reactive’ animals tending to be the opposite (Koolhaas et al., 1999; Bensky et al., 2017). The environment is expected to shape behavioral syndromes through shaping the stress axes in early development (Sih, 2011; Killen et al., 2013; Careau et al., 2014; Guenther et al., 2014; Farine et al., 2015). For example, in the cavy (Cavia aperea), variation in birth season (associated with different stressors) leads to behavioral syndrome differences in adults (Guenther et al., 2014). In crickets, agonistic experience during development shapes individual differences in approach-avoidance behaviors, aggressiveness, and motility, which the authors argue supports a proactive and reactive coping style syndromes in that species (Balsam and Stevenson, 2021). The social environment can represent different levels of stress, and certainly variation in the type of mating interactions is likely to be a large source of stress variation. Further research may be able to determine if these differences in behavioral syndromes correlate with differences in social information processing and/or differences in stress physiology (coping styles).
Conclusion
In summary, we found that sexual-social experiences during development affect the emergence of behavioral syndromes. We were able to manipulate the type of social interactions females experienced across development while keeping social group size the same— allowing us to differentiate the type of social interactions from the quantity. Our results have broad implications on the power of sexual selection on the evolution and maintenance of behavioral syndromes and personality. If female experience with single or multiple male mating tactics in the laboratory has the power to shape behavioral syndromes, then we should also see this pattern in the wild. Comparative work should examine whether species with single mating tactics repeatedly diverge in personality and behavioral syndromes from taxa with multiple alternative male mating tactics; and whether species with multiple mating tactics exhibit stronger evidence for behavioral syndromes (and whether a boldness-low aggression syndrome is the most dominant). Moreover, future studies should consider multiple sources of environmental variation on behavioral syndrome development including both sexual selection (mating system, social complexity) and natural selection (predation, habitat complexity) pressures.
Data availability statement
The raw data supporting the conclusions of this article will be made available by the authors, without undue reservation.
Ethics statement
The animal study was reviewed and approved by Institutional Animal Care and Use Committee (protocol AUP-2019-00230).
Author contributions
MC: conceptualized research and funding acquisition and resources. PQ, YS, KW, RD, and VY: conducted research. PQ and MC: wrote manuscript. LR and PQ: data curation and formal analysis. PQ, LR, and MC: visualization. All authors contributed to the article and approved the submitted version.
Funding
This research was funded by the National Science Foundation (grant number NSF-IOS-1911826 to MC).
Acknowledgments
We are grateful to the Brackenridge Field Laboratory at UT Austin which provided a semi-natural outdoor setting for our seed populations of X. nigrensis. We heartily thank all of the undergraduate students who helped to maintain social environments, conduct density counts, and score data including Rob Bailey, Niveya James, Kathryn Kaihlanen, Camille Akins, Amogh Kashyap, Adam Redmer, Jessika McFarland, and Caleb Fleischer.
Conflict of interest
The authors declare that the research was conducted in the absence of any commercial or financial relationships that could be construed as a potential conflict of interest.
Publisher’s note
All claims expressed in this article are solely those of the authors and do not necessarily represent those of their affiliated organizations, or those of the publisher, the editors and the reviewers. Any product that may be evaluated in this article, or claim that may be made by its manufacturer, is not guaranteed or endorsed by the publisher.
Supplementary material
The Supplementary material for this article can be found online at: https://www.frontiersin.org/articles/10.3389/fevo.2022.1050569/full#supplementary-material
References
Ballen, C., Shine, R., and Olsson, M. (2014). Effects of early social isolation on the behaviour and performance of juvenile lizards, Chamaeleo calyptratus. Anim. Behav. 88, 1–6. doi: 10.1016/j.anbehav.2013.11.010
Balsam, J. S., and Stevenson, P. A. (2021). Agonistic experience during development establishes inter-individual differences in approach-avoidance behaviour of crickets. Sci. Rep. 11, 16702–16713. doi: 10.1038/s41598-021-96201-1
Batabyal, A., and Thaker, M. (2019). Social coping styles of lizards are reactive and not proactive in urban areas. Gen. Comp. Endocrinol. 270, 67–74. doi: 10.1016/j.ygcen.2018.10.007
Bell, A. M. (2005). Differences between individuals and populations of threespined stickleback. J. Evol. Biol. 18, 464–473. doi: 10.1111/j.1420-9101.2004.00817.x
Bell, A. M., and Sih, A. (2007). Exposure to predation generates personality in threespined sticklebacks (Gasterosteus aculeatus). Ecol. Lett. 10, 828–834. doi: 10.1111/j.1461-0248.2007.01081.x
Bengston, S. E., Pruitt, J. N., and Riechert, S. E. (2014). Differences in environmental enrichment generate contrasting behavioural syndromes in a basal spider lineage. Anim. Behav. 93, 105–110. doi: 10.1016/j.anbehav.2014.04.022
Bensky, M. K., Paitz, R., Pereira, L., and Bell, A. M. (2017). Testing the predictions of coping styles theory in threespined sticklebacks. Behav. Process. 136, 1–10. doi: 10.1016/j.beproc.2016.12.011
Bergmüller, R., and Taborsky, M. (2010). Animal personality due to social niche specialisation. Trends Ecol. Evol. 25, 504–511. doi: 10.1016/j.tree.2010.06.012
Biro, P. A., and Adriaenssens, B. (2013). Predictability as a personality trait: consistent differences in intraindividual behavioral variation. Am. Nat. 182, 621–629. doi: 10.1086/673213
Blake, C. A., and Gabor, C. R. (2014). Effect of prey personality depends on predator species. Behav. Ecol. 25, 871–877. doi: 10.1093/beheco/aru041
Boulton, K., Grimmer, A. J., Rosenthal, G. G., Walling, C. A., and Wilson, A. J. (2014). How stable are personalities? A multivariate view of behavioural variation over long and short timescales in the sheepshead swordtail, Xiphophorus birchmanni. Behav. Ecol. Sociobiol. 68, 791–803. doi: 10.1007/s00265-014-1692-0
Briggs, S. E., Godin, J. G. J., and Dugatkin, L. A. (1996). Mate-choice copying under predation risk in the Trinigadian guppy (Poecilia reticulata). Behav. Ecol. 7, 151–157. doi: 10.1093/beheco/7.2.151
Brown, C., Gardner, C., and Braithwaite, V. A. (2005). Differential stress responses in fish from areas of high-and low-predation pressure. J. Comp. Physiol. B. 175, 305–312. doi: 10.1007/s00360-005-0486-0
Burish, M. J., Kueh, H. Y., and Wang, S. S. H. (2004). Brain architecture and social complexity in modern and ancient birds. Brain Behav. Evol. 63, 107–124. doi: 10.1159/000075674
Butler, M. W., Toomey, M. B., McGraw, K. J., and Rowe, M. (2012). Ontogenetic immune challenges shape adult personality in mallard ducks. Proc. R. Soc. B Biol. Sci. 279, 326–333. doi: 10.1098/rspb.2011.0842
Cabrera, D., Nilsson, J. R., and Griffen, B. D. (2021). The development of animal personality across ontogeny: a cross-species review. Anim. Behav. 173, 137–144. doi: 10.1016/j.anbehav.2021.01.003
Carazo, P., Noble, D. W., Chandrasoma, D., and Whiting, M. J. (2014). Sex and boldness explain individual differences in spatial learning in a lizard. Proc. R. Soc. B Biol. Sci. 281:20133275. doi: 10.1098/rspb.2013.3275
Careau, V., Buttemer, W. A., and Buchanan, K. L. (2014). Early-developmental stress, repeatability, and canalization in a suite of physiological and behavioral traits in female zebra finches. Integr. Comp. Biol. 54, 539–554. doi: 10.1093/icb/icu095
Castellano, S., and Friard, O. (2021). Environmental effects on the ontogenesis of tadpole personality. Anim. Behav. 175, 153–161. doi: 10.1016/j.anbehav.2021.03.002
Cote, J., Fogarty, S., Weinersmith, K., Brodin, T., and Sih, A. (2010). Personality traits and dispersal tendency in the invasive mosquitofish (Gambusia affinis). Proc. R. Soc. B Biol. Sci. 277, 1571–1579. doi: 10.1098/rspb.2009.2128
Cummings, M. E. (2018). Sexual conflict and sexually dimorphic cognition—reviewing their relationship in poeciliid fishes. Behav. Ecol. Sociobiol. 72, 1–13. doi: 10.1007/s00265-018-2483-9
Cummings, M., and Mollaghan, D. (2006). Repeatability and consistency of female preference behaviours in a northern swordtail Xiphophorus nigrensis. Anim. Behav. 72, 217–224. doi: 10.1016/j.anbehav.2006.01.009
Dadda, M. (2015). Female social response to male sexual harassment in poeciliid fish: a comparison of six species. Front. Psychol. 6:1453. doi: 10.3389/fpsyg.2015.01453
Darden, S. K., and Croft, D. P. (2008). Male harassment drives females to alter habitat use and leads to segregation of the sexes. Biol. Lett. 4, 449–451. doi: 10.1098/rsbl.2008.0308
Davies, A. D., Lewis, Z., and Dougherty, L. R. (2020). A meta-analysis of factors influencing the strength of mate-choice copying in animals. Behav. Ecol. 31, 1279–1290. doi: 10.1093/beheco/araa064
Delclos, P. J., Forero, S. A., and Rosenthal, G. G. (2020). Divergent neurogenomic responses shape social learning of both personality and mate preference. J. Exp. Biol. 223:jeb.220707. doi: 10.1242/jeb.220707
Dingemanse, N. J., Wright, J., Kazem, A. J., Thomas, D. K., Hickling, R., and Dawnay, N. (2007). Behavioural syndromes differ predictably between 12 populations of three-spined stickleback. J. Anim. Ecol. 76, 1128–1138. doi: 10.1111/j.1365-2656.2007.01284.x
DiRienzo, N., Niemelä, P. T., Skog, A., Vainikka, A., and Kortet, R. (2015). Juvenile pathogen exposure affects the presence of personality in adult field crickets. Front. Ecol. Evol. 3:36. doi: 10.3389/fevo.2015.00036
Dugatkin, L. A. (1996). Copying and mate choice. In C. M. Heyes and B. G. Galef, Jr. (Eds.) Social learning in animals: The roots of culture, (pp 85–105. Academic Press. doi: 10.1016/B978-012273965-1/50006-6
Dugatkin, L. A., and Godin, J. G. J. (1993). Female mate copying in the guppy (Poecilia reticulata): age-dependent effects. Behav. Ecol. 4, 289–292. doi: 10.1093/beheco/4.4.289
Dunbar, R. I. (1998). The social brain hypothesis. Evol. Anthropol. 6, 178–190. doi: 10.1002/(SICI)1520-6505(1998)6:5<178::AID-EVAN5>3.0.CO;2-8
Dunbar, R. I. (2009). The social brain hypothesis and its implications for social evolution. Ann. Hum. Biol. 36, 562–572. doi: 10.1080/03014460902960289
DuVal, E. H. (2013). Female mate fidelity in a lek mating system and its implications for the evolution of cooperative lekking behavior. Am. Nat. 181, 213–222. doi: 10.1086/668830
Edenbrow, M., and Croft, D. P. (2013). Environmental and genetic effects shape the development of personality traits in the mangrove killifish Kryptolebias marmoratus. Oikos 122, 667–681. doi: 10.1111/j.1600-0706.2012.20556.x
Etheredge, R. I., Avenas, C., Armstrong, M. J., and Cummings, M. E. (2018). Sex-specific cognitive–behavioural profiles emerging from individual variation in numerosity discrimination in Gambusia affinis. Anim. Cogn. 21, 37–53. doi: 10.1007/s10071-017-1134-2
Farine, D. R., Spencer, K. A., and Boogert, N. J. (2015). Early-life stress triggers juvenile zebra finches to switch social learning strategies. Curr. Biol. 25, 2184–2188. doi: 10.1016/j.cub.2015.06.071
Franck, D., Hannes, R. P., Lanffermann, H., and Ribowski, A. (1985). Effects of social isolation on aggressiveness in fish with special reference to the swordtail (Xiphophorus helleri). Behav. Process. 10, 415–427. doi: 10.1016/0376-6357(85)90041-5
Freeberg, T. M., Dunbar, R. I., and Ord, T. J. (2012). Social complexity as a proximate and ultimate factor in communicative complexity. Philos. Trans. R. Soc. B Biol. Sci. 367, 1785–1801. doi: 10.1098/rstb.2011.0213
Groothuis, T. G., Koolhaas, J. M., Drent, P. J., and Carere, C. (2005). Epigenetic effects on personality traits: early food provisioning and sibling competition. Behaviour 142, 1329–1355. doi: 10.1163/156853905774539328
Guillette, L. M., Reddon, A. R., Hurd, P. L., and Sturdy, C. B. (2009). Exploration of a novel space is associated with individual differences in learning speed in black-capped chickadees Poecile atricapillus. Behav. Process. 82, 265–270. doi: 10.1016/j.beproc.2009.07.005
Guenther, A., Finkemeier, M. A., and Trillmich, F. (2014). The ontogeny of personality in the wild Guinea pig. Anim. Behav. 90, 131–139. doi: 10.1016/j.anbehav.2014.01.032
Hänninen, L., and Pastell, M. (2009). CowLog: Open-source software for coding behaviors from digital video. Beh. Res. Meth. 41, 472–476. doi: 10.3758/BRM.41.2.472
Hedrick, A. V. (2017). The development of animal personality. Front. Ecol. Evol. 5:14. doi: 10.3389/fevo.2017.00014
Heinen-Kay, J. L., Schmidt, D. A., Stafford, A. T., Costa, M. T., Peterson, M. N., Kern, E. M., et al. (2016). Predicting multifarious behavioural divergence in the wild. Anim. Behav. 121, 3–10. doi: 10.1016/j.anbehav.2016.08.016
Hobson, E. A. (2020). Differences in social information are critical to understanding aggressive behavior in animal dominance hierarchies. Curr. Opin. Psychol. 33, 209–215. doi: 10.1016/j.copsyc.2019.09.010
Hobson, E. A., Ferdinand, V., Kolchinsky, A., and Garland, J. (2019). Rethinking animal social complexity measures with the help of complex systems concepts. Anim. Behav. 155, 287–296. doi: 10.1016/j.anbehav.2019.05.016
Huang, P., St. Mary, C. M., and Kimball, R. T. (2020). Habitat urbanization and stress response are primary predictors of personality variation in northern cardinals (Cardinalis cardinalis). J. Urban Ecol. 6:juaa 015. doi: 10.1093/jue/juaa015
Huntingford, F. A. (1976). The relationship between anti-predator behaviour and aggression among conspecifics in the three-spined stickleback, Gasterosteus aculeatus. Anim. Behav. 24, 245–260. doi: 10.1016/S0003-3472(76)80034-6
Kamhi, J. F., Gronenberg, W., Robson, S. K., and Traniello, J. F. (2016). Social complexity influences brain investment and neural operation costs in ants. Proc. R. Soc. B Biol. Sci. 283:20161949. doi: 10.1098/rspb.2016.1949
Killen, S. S., Marras, S., Metcalfe, N. B., McKenzie, D. J., and Domenici, P. (2013). Environmental stressors alter relationships between physiology and behaviour. Trends Ecol. Evol. 28, 651–658. doi: 10.1016/j.tree.2013.05.005
Koolhaas, J. M., Korte, S. M., De Boer, S. F., Van Der Vegt, B. J., Van Reenen, C. G., Hopster, H., et al. (1999). Coping styles in animals: current status in behavior and stress-physiology. Neurosci. Biobehav. Rev. 23, 925–935. doi: 10.1016/S0149-7634(99)00026-3
Laubach, Z. M., Greenberg, J. R., Turner, J. W., Montgomery, T. M., Pioon, M. O., Sawdy, M. A., et al. (2021). Early-life social experience affects offspring DNA methylation and later life stress phenotype. Nat. Commun. 12, 1–15. doi: 10.1038/s41467-021-24583-x
Liedtke, J., Redekop, D., Schneider, J. M., and Schuett, W. (2015). Early environmental conditions shape personality types in a jumping spider. Front. Ecol. Evol. 3:134. doi: 10.3389/fevo.2015.00134
Magurran, A. E., and Garcia, C. M. (2000). Sex differences in behaviour as an indirect consequence of mating system. J. Fish Biol. 57, 839–857. doi: 10.1111/j.1095-8649.2000.tb02196.x
Maximino, C., Marques de Brito, T., Dias, C. A. G. D. M., Gouveia, A., and Morato, S. (2010). Scototaxis as anxiety-like behavior in fish. Nat. Protoc. 5, 209–216. doi: 10.1038/nprot.2009.225
Moretz, J. A., and Morris, M. R. (2003). Evolutionarily labile responses to a signal of aggressive intent. Proc. R. Soc. Lond. Ser. B Biol. Sci. 270, 2271–2277. doi: 10.1098/rspb.2003.2510
Murthy, S., and Gould, E. (2018). Early life stress in rodents: animal models of illness or resilience? Front. Behav. Neurosci. 12:157. doi: 10.3389/fnbeh.2018.00157
Naguib, M., Flörcke, C., and van Oers, K. (2011). Effects of social conditions during early development on stress response and personality traits in great tits (Parus major). Dev. Psychobiol. 53, 592–600. doi: 10.1002/dev.20533
Nakagawa, S., and Schielzeth, H. (2010). Repeatability for Gaussian and non-Gaussian data: a practical guide for biologists. Biol. Rev. 85, 935–956. doi: 10.1111/j.1469-185X.2010.00141.x
Niemelä, P. T., DiRienzo, N., and Hedrick, A. V. (2012). Predator-induced changes in the boldness of naïve field crickets, Gryllus integer, depends on behavioural type. Anim. Behav. 84, 129–135. doi: 10.1016/j.anbehav.2012.04.019
Pilastro, A., Benetton, S., and Bisazza, A. (2003). Female aggregation and male competition reduce costs of sexual harassment in the mosquitofish Gambusia holbrooki. Anim. Behav. 65, 1161–1167. doi: 10.1006/anbe.2003.2118
Polverino, G., Santostefano, F., Díaz-Gil, C., and Mehner, T. (2018). Ecological conditions drive pace-of-life syndromes by shaping relationships between life history, physiology and behaviour in two populations of eastern mosquitofish. Sci. Rep. 8, 1–10. doi: 10.1038/s41598-018-33047-0
Ramsey, M. E., Vu, W., and Cummings, M. E. (2014). Testing synaptic plasticity in dynamic mate choice decisions: N-methyl d-aspartate receptor blockade disrupts female preference. Proc. R. Soc. B Biol. Sci. 281:20140047. doi: 10.1098/rspb.2014.0047
Reding, L., and Cummings, M. E. (2017). Context-dependent preferences vary by multicomponent signals in a swordtail. Anim. Behav. 129, 237–247. doi: 10.1016/j.anbehav.2017.05.017
Rios-Cardenas, O., Tudor, M. S., and Morris, M. R. (2007). Female preference variation has implications for the maintenance of an alternative mating strategy in a swordtail fish. Anim. Behav. 74, 633–640. doi: 10.1016/j.anbehav.2007.01.002
Rosenthal, G. G. (2017). Mate Choice: The Evolution of Sexual Decision Making from Microbes to Humans. Princeton: Princeton University Press.
Rowe, L., Arnqvist, G., Sih, A., and Krupa, J. J. (1994). Sexual conflict and the evolutionary ecology of mating patterns: water striders as a model system. Trends Ecol. Evol. 9, 289–293. doi: 10.1016/0169-5347(94)90032-9
Ryan, M. J., and Causey, B. A. (1989). “Alternative” mating behavior in the swordtails Xiphophorus nigrensis and Xiphophorus pygmaeus (Pisces: Poeciliidae). Behav. Ecol. Sociobiol. 24, 341–348. doi: 10.1007/BF00293262
Sadoul, B., Blumstein, D. T., Alfonso, S., and Geffroy, B. (2021). Human protection drives the emergence of a new coping style in animals. PLoS Biol. 19:e3001186. doi: 10.1371/journal.pbio.3001186
Samia, D. S., Nakagawa, S., Nomura, F., Rangel, T. F., and Blumstein, D. T. (2015). Increased tolerance to humans among disturbed wildlife. Nat. Commun. 6, 1–8. doi: 10.1038/ncomms9877
Searcy, W. A., and Capp, M. S. (1997). Estradiol dosage and the solicitation display assay in red-winged blackbirds. Condor 99, 826–828. doi: 10.2307/1370497
Sih, A. (2011). Effects of early stress on behavioral syndromes: an integrated adaptive perspective. Neurosci. Biobehav. Rev. 35, 1452–1465. doi: 10.1016/j.neubiorev.2011.03.015
Sih, A., Bell, A., and Johnson, J. C. (2004a). Behavioral syndromes: an ecological and evolutionary overview. Trends Ecol. Evol. 19, 372–378. doi: 10.1016/j.tree.2004.04.009
Sih, A., Bell, A. M., Johnson, J. C., and Ziemba, R. E. (2004b). Behavioral syndromes: an integrative overview. Q. Rev. Biol. 79, 241–277. doi: 10.1086/422893
Sih, A., Mathot, K. J., Moirón, M., Montiglio, P. O., Wolf, M., and Dingemanse, N. J. (2015). Animal personality and state–behaviour feedbacks: a review and guide for empiricists. Trends Ecol. Evol. 30, 50–60. doi: 10.1016/j.tree.2014.11.004
Stamps, J. A., and Groothuis, T. G. (2010a). Developmental perspectives on personality: implications for ecological and evolutionary studies of individual differences. Philos. Trans. R. Soc. B Biol. Sci. 365, 4029–4041. doi: 10.1098/rstb.2010.0218
Stamps, J., and Groothuis, T. G. (2010b). The development of animal personality: relevance, concepts and perspectives. Biol. Rev. 85, 301–325. doi: 10.1111/j.1469-185X.2009.00103.x
Taborsky, B. (2016). Opening the black box of developmental experiments: behavioural mechanisms underlying long-term effects of early social experience. Ethology 122, 267–283. doi: 10.1111/eth.12473
Taborsky, B., and Oliveira, R. F. (2012). Social competence: an evolutionary approach. Trends Ecol. Evol. 27, 679–688. doi: 10.1016/j.tree.2012.09.003
ten Cate, C., Verzijden, M. N., and Etman, E. (2006). Sexual imprinting can induce sexual preferences for exaggerated parental traits. Curr. Biol. 16, 1128–1132. doi: 10.1016/j.cub.2006.03.068
Uy, J. A. C., Patricelli, G. L., and Borgia, G. (2001). Complex mate searching in the satin bowerbird Ptilonorhynchus violaceus. Am. Nat. 158, 530–542. doi: 10.1086/323118
Wilson, A. J., de Boer, M., Arnott, G., and Grimmer, A. (2011). Integrating personality research and animal contest theory: aggressiveness in the green swordtail Xiphophorus helleri. PLoS One 6:e28024. doi: 10.1371/journal.pone.0028024
Wong, R. Y., So, P., and Cummings, M. E. (2011). How female size and male displays influence mate preference in a swordtail. Anim. Behav. 82, 691–697. doi: 10.1016/j.anbehav.2011.06.024
Keywords: social complexity, behavioral development, coping styles, mating systems, behavioral syndromes, animal personality
Citation: Queller PS, Shirali Y, Wallace KJ, DeAngelis RS, Yurt V, Reding LP and Cummings ME (2022) Complex sexual-social environments produce high boldness and low aggression behavioral syndromes. Front. Ecol. Evol. 10:1050569. doi: 10.3389/fevo.2022.1050569
Edited by:
Ann Valerie Hedrick, University of California, Davis, United StatesReviewed by:
Susanne R. K. Zajitschek, Liverpool John Moores University, United KingdomKlaudia Witte, University of Siegen, Germany
Copyright © 2022 Queller, Shirali, Wallace, DeAngelis, Yurt, Reding and Cummings. This is an open-access article distributed under the terms of the Creative Commons Attribution License (CC BY). The use, distribution or reproduction in other forums is permitted, provided the original author(s) and the copyright owner(s) are credited and that the original publication in this journal is cited, in accordance with accepted academic practice. No use, distribution or reproduction is permitted which does not comply with these terms.
*Correspondence: Philip S. Queller, pqueller@utexas.edu; Molly E. Cummings, mcummings@austin.utexas.edu
†Present address: ESYasmin Shirali, Texas Department of State Health Services, Austin, TX, United States
Kelly J. Wallace, Department of Psychology, Emory University, Atlanta, GA, United States
Vural Yurt, HAS University of Applied Sciences, s-Hertogenbosch, Netherlands