- 1College of Engineering, Northeast Agricultural University, Harbin, China
- 2College of Art and Science, Northeast Agricultural University, Harbin, China
In order to solve the problem that water resources in a certain province are relatively scarce and the spatial matching effect of resources is poor, which causes the w-e-f system to face the problem of trade-off, the water-energy-food coupling coordination and collaborative optimization system is proposed. By constructing the w-e-f multi-objective collaborative optimization model, the multi-objective optimization schemes under different weight scenarios are compared, and scenario 2 with better effect is selected as the best scheme for collaborative optimization. The results show that, on the basis of meeting the constraint conditions, by adjusting the crop planting structure and making efficient use of resource elements, the water resource consumption of major grain crops can be reduced by 7.3% and the total energy consumption can be reduced by 2.5% by 2030 compared with 2019.
Conclusion: The system provides solutions to alleviate the pressure on water resources and ecological environment to a certain extent. Compared with the single objective optimization scheme, multi-objective optimization can effectively alleviate the trade-off conflict between w-e-f.
Introduction
Responding to the demand for food, energy and water resources has become a major challenge for human social development. In the twenty-first century, the global exploitation and consumption of fresh water are increasing rapidly, and the limited fresh water resources are decreasing day by day. The crisis of water resources has increasingly become an important challenge for the sustainable development of human society. The demand for energy in agriculture, industry, national defense and military affairs as well as the life of domestic residents in various countries has increased rapidly. The supply and demand situation of primary energy represented by crude oil and coal has become increasingly tense, resulting in large fluctuations in energy prices, which have affected all countries in the world to varying degrees. Since 2008, food shortages have occurred in many parts of the world, leading to sharp fluctuations in international food prices and the continuous decline of global food reserves, leading to a large-scale food crisis. As a result, international relations among many countries have become more intense and the political situation has become more tense. With the continuous growth of the population and the impact of global changes, the global demand for water, energy and food in 2050 will nearly double compared with that in 2010 (Xu et al., 2020). Water must be used for energy use, processing and conversion, and energy is also needed to treat, distribute and transform water resources. All the linkages between production and consumption, such as planting, growing, harvesting and transport, are inextricably linked to water and energy use. Our resources have influence and influence. However, fresh water resources are becoming more and more scarce, food supply is becoming more and more uncertain, and social production and processing life needs more and more energy. The new characteristics of convergence and conflict among the three make water, energy and food more connected and more influential. The integration of water development, energy and food security is particularly important under the impact of global population growth, insecurity, environmental degradation and climate change. Therefore, it is particularly important to study the internal relationships and interactions among water, energy and food. It is not enough to focus on just one (Zhong and Zhang, 2020). China uses 7% of the world’s agricultural land to feed about 20 percent of the world’s population. Coal remains the main source of China’s energy. Due to the continuous development of population, economy and people’s living standard, China’s demand for food and energy is increasing year by year, and high consumption is increasing. Natural resources and environmental problems are hindering China’s economic and social development. Food and energy production requires a large amount of water, but China’s water resources are scarce and uneven over time and space. Per capita water supply is only 2,100 m3, less than a third of the world average. The shortage of water resources and the increasing demand for food and energy have brought serious problems to the stability of China’s “water-energy-grain” system. Due to the special climate and geographical location, China’s “water and grain” resources are scarce, and the inconsistency in space and time is obvious, which restricts the economic and population growth from the following aspects. Per capita resources are limited: (1) China is one of the poorest countries in the world in terms of water resources per capita. In 14 provinces, autonomous regions and municipalities directly under the Central Government, the per capita water shortage is less than the internationally recognized 1,750 m3, and in nine regions, the water shortage is less than 500 m3. China consumes less than 90% of rice and as many as 200 m people depend on food. Coal reserves reached 600 billion tons, the third largest in the world. However, per capita resources are about 462 tons, which is lower than the world average. In 2018, China’s dependence on oil increased to 69.8%. (2) The spatial and sectional distribution of water resources are uneven. From the perspective of geographical distribution, it has the characteristics of more in the south and less in the north, and more in the east and less in the west. From the time distribution, summer and autumn rain hot more, winter and spring rain hot less. (3) The distribution of water resources is inconsistent with population, land, energy, economy and society. The northern region (north of Khyangan Basin) accounts for 63.5% of the country, about 46% of the population, 60% of the arable land, 44% of the GDP, only product water accounts for 19%. China’s energy bases are mainly located in Ordos, Shanxi, Xinjiang and other northern and central and western regions of Inner Mongolia. Energy exploitation and processing require a large amount of water resources. At the same time, the amount of water resources required for farmland irrigation in these regions is high, but the local water resources are extremely short, and the water consumption contradiction between different water departments is serious (Cao et al., 2020). (4) Water pollution is becoming more and more serious. Agricultural cultivation, coal mining and other food and energy production processes will aggravate water environment pollution and intensify the contradiction between supply and demand of water resources. The severe contradiction of “food, energy, and water” restricts the sustainable development of China’s economy and society.
Literature review
Yan and Qin (2020) proposed that coordination is mainly manifested as a benign cycle of system development, that is, a development process in which each subsystem interacts and cooperates with each other, supports and cooperates with each other under the condition of the overall goal evolution of the system. On this basis, Yang (2020) further pointed out the process mechanism and conditions for the system to transform from disordered state to ordered state in synergetics. She believes that there are four elements that are very important to the operation and development of the system. The first element is the order parameter. The order parameter always accompanies and has a continuous effect on the operation of the system during the development and operation of the system. The order parameter plays a leading role in the development of the subsystem. The second element is the organic connection and mutual cooperation among subsystems, which is mainly manifested in that each subsystem can form synergy in the development process. When the correlation between subsystems plays a dominant role, the overall system will certainly develop in an orderly direction. The third element is the control parameter. The internal development of the system cannot be separated from the influence of the external environment. The external environment provides certain control parameters to ensure the operation and development of the system. The last element is the feedback mechanism. The system always advances in continuous development. Feedback regulation is an important guarantee for the system to achieve development (Yang, 2020). Qi et al. (2022) believe that in the social system, the interaction and self-organization of subsystems will contribute to the whole system. This contribution is synergy, which will promote the continuous development of the system. In general, the emergence of synergetics has brought a new direction to the development of our research system and provided us with new ideas to deal with the system problems of various disciplines (Qi et al., 2022). Lu et al. (2021) took Dalian City as an empirical research object to evaluate its intensive use of land resources, and applied synergetics theory to select 30 order parameter indicators to build an intensive use evaluation index system on the basis of expert consultation method and literature method. Lv et al. (2020) first constructed the state parameters of the subsystem and carried out gray correlation analysis with the entropy of the land use structure. On this basis, the state parameters representing the subsystem were selected, and then the evolution model of the land use system was constructed. The order parameters were obtained by applying the gray system modeling method of nonlinear differential equations. Yangzhou city was taken as an empirical object to carry out coordination on the land use system (Lv et al., 2020). Wang et al. (2021) conducted collaborative research on subsystems. He divided the rural system into economic subsystem, social subsystem, ecological subsystem and scientific and technological subsystem. Through the coupling of each subsystem in four aspects, including space-time coupling, functional coupling, structural coupling and target coupling (Wang et al., 2021). Meng (2021_ evaluated the environmental risk of the land use system in Beijing mountain basin, established the coevolution model of the land use system using the gray system modeling method, and used MATLAB software to calculate the matrix to obtain the relevant order parameters. To sum up, considering the collaborative evaluation of land use structure in this essay, the land use system is divided into three subsystems, namely, the land use economic subsystem, the land use ecological subsystem and the land use social subsystem, and the state parameters are selected in each subsystem for research. According to the selected state parameters and the entropy of land use structure, the gray correlation analysis is carried out. The obtained state parameter with high correlation is the order parameter representing the change of land use structure (Meng, 2021). Luo et al. (2021) described the complex relationship and core elements of WEF nexus in different regions through case studies. Chikozho et al. (2020) studied the basic relationship of the WEF nexus system based on historical data using multivariate statistical methods. Forbes et al. (2020) selected the Tonle Sap River and Mekong River Basin as the research object. The water, energy and food security in the basin are closely related, and the relationship method has good applicability in promoting stakeholder cooperation. At present, the understanding of the relationship between wefs mainly has three characteristics, namely, multi center complex network, local characteristics and a large number of feedback loops. The research of Chinese scholars in this aspect is still at the exploratory stage. Most of them are in the primary stage of water-energy-food. The research is mainly about the theoretical framework or other aspects based on historical statistical data, and the qualitative research on the impact of water, energy and food on society, economy and environment (Forbes et al., 2020).
Based on a systematic review of relevant literature and a deep understanding of the connotation of w-e-fnexus, this essay takes a province as the research area for empirical research. First of all, by constructing the w-e-f system evaluation index system, using the comprehensive evaluation index and the coupling coordination model, the w-e-f system evaluation index and the coupling co scheduling of the whole province and various cities from 2008 to 2018 were calculated, the characteristics of their spatial and temporal differences were analyzed, and the system correlation effect was explored (Jiang et al., 2020). On this basis, the factor analysis method is used to explore the main influencing factors of the change of w-e-f coordination level, and the driving mechanism is analyzed from the dimensions of supply-demand relationship, resource consumption structure and sustainability.
Research method
Concept and connotation of collaborative security of water-energy-food related system
Concept of collaborative security of related systems
Water, energy and food are inseparable from each other. Changes in the situation of one resource can always have an impact on the quantity, quality and even price fluctuation of the other two resources. As the basic resources of social development, water, energy and food affect and restrict each other, and control the development of the system through the mutual transmission of various relationships among resources. The three constitute a closely linked and inseparable whole (Gnanadesikan et al., 2020). In 2011, the world economic forum took the three resources as a whole and explained their meanings from the perspective of resource security. The safety of water resources is briefly summarized as the health of drinking water and the provision of basic water conservancy facilities. Energy security is manifested in the security and stability of energy supply and the realization of clean production and services. Food security is the sufficiency of food supply and the guarantee of nutrition.
According to the synergetic theory, the water energy grain correlation system, as a complex system with open characteristics, constantly exchanges material and energy with the outside world, which is unstable. The change of the original structure mode affected by the conditions will lead to its departure from the stable state. For example, the emergence and application of new technology, the change of resource demand caused by various factors, and the adjustment of national industrial policies will all promote the system to change its original stable state and establish a new system structure. According to the servo principle, the internal subsystems and subsystems of the correlation system can form mutual synergy through self-cooperation, self-regulation and self-organization, and form the order parameter (slow variable) with a dominant role. This variable dominates other variables and becomes the dominant variable for the coordinated development of the system. When each subsystem in the system can form a positive feedback state that promotes each other, it is considered that the system has a good level of coordination (Li et al., 2021). In general, the essence of the water-energy-food related system is to consider the interaction among the three from the perspective of the overall system, alleviate the problems of insufficient supply, waste and imbalance of resources, improve the coordination and management ability of the related departments of resources, and promote the common sustainable development of society, economy, environment and resources.
Therefore, the definition of collaborative security of water-energy-food related system is proposed. That is, on the premise of ensuring the harmonious development of the regional economy, society and ecological environment, through the improvement of the internal security of the energy, food and water resources subsystems and the close interaction and feedback between the subsystems, a benign mutual promotion is formed to achieve the improvement of the overall security and the coordinated progress.
The connotation of collaborative security of related systems
The water-energy-food correlation system can be broadly summarized into two relationships: the first is the dependency relationship, that is, the energy consumption generated in the process of water resources extraction, purification, regulation, storage, transportation, distribution and reuse. The development, production and cooling of energy are supported by water resources. Energy and water resources are also important resource bases for grain irrigation. The energy crops planted provide biomass energy. The second is the constraint relationship caused by the dependency between resources. Because the production and utilization of food and energy depend on water resources, the water resources conditions will limit the local food and energy production, and the production of energy and food may also cause changes in the local water environment, disrupt the original resource status, cause water pollution, and increase the purification load of water resources. Figure 1A shows the dynamic framework for the collaborative safety of hydropower food-related systems, and Figure 1B shows the work flow chart of this paper. The relationship of dependence and restriction determines that water-energy-food is always an interconnected whole system. In this essay, the dynamic framework of collaborative security of water-energy-food related system is constructed based on the synergy theory, as shown in Figure 1A.
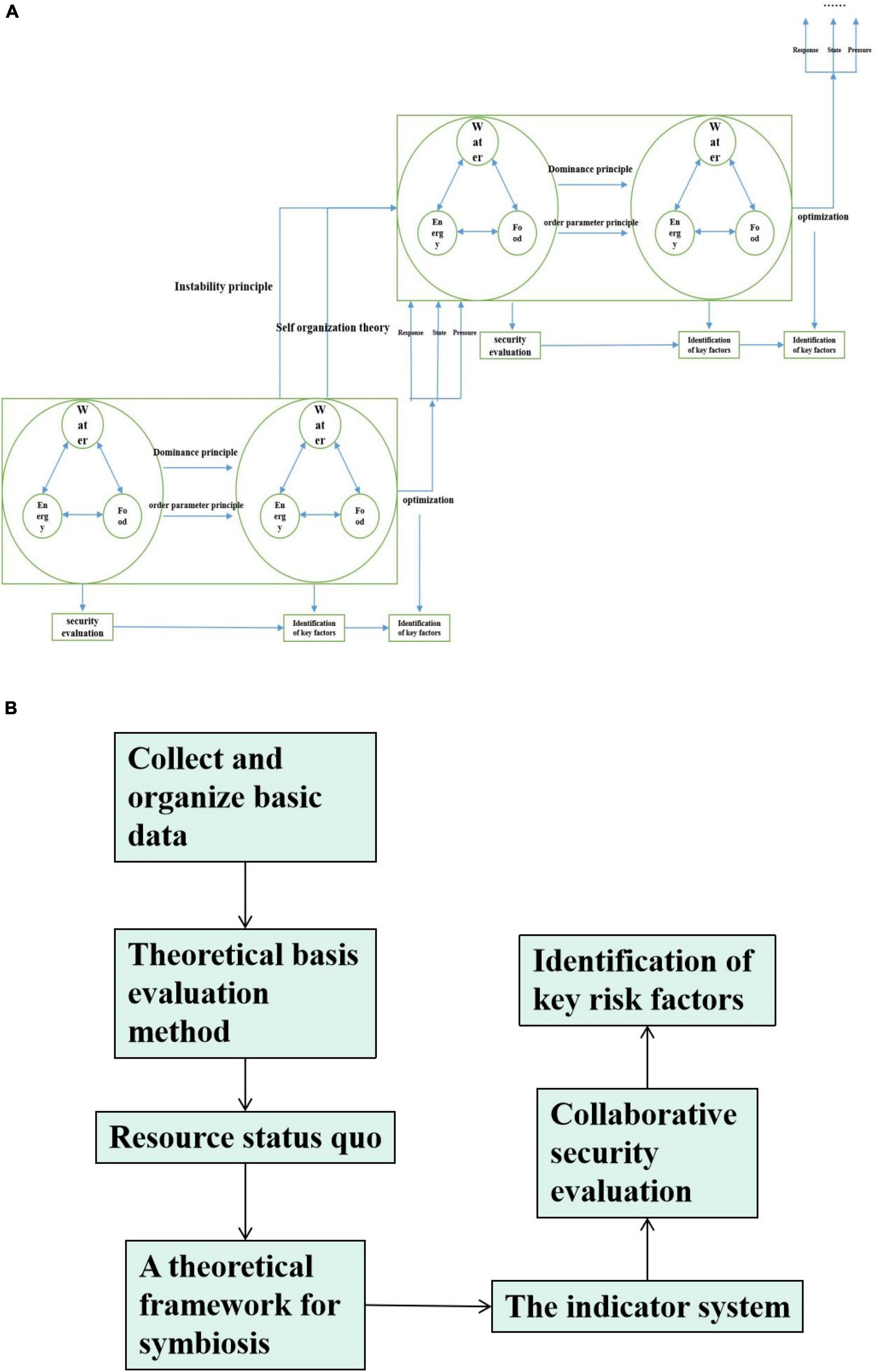
Figure 1. (A) dynamic frame of collaborative security of water-energy-food related system. (B) Technology roadmap.
The water-energy-food correlation system is an open nonlinear system, which has close relationship with the external environment, and there are always exchanges of material, energy and information. According to the order parameter principle and the servo principle, in the process of continuous development of the system and evolution to the ordered state, the synergy of the internal subsystems will become more and more significant, and can form the key variables that dominate the evolution of the system. Such variables are order parameters. In the water energy grain correlation system, this dominant parameter may be any subsystem of water, energy and grain, or may be two subsystems. Different types of interaction relations may be formed between subsystems, which may promote each other, or one of them may restrict the other. When each subsystem in the system cannot form a mutually promoting relationship (the unbalanced relationship is represented by circles of different sizes of subsystems in the figure), the system can be optimized by changing external factors such as pressure, state and response, so as to make the system develop in a cooperative direction.
According to the instability principle and self-organization theory, the system will form a new structure and state (Liu et al., 2020). The evolution process of the new system will develop a new interaction relationship between the order parameters and the internal subsystems. When the subsystems do not reach the ideal state of mutual promotion, the system will be optimized through the change of external forces to make the system reach a higher level of coordination. It should be specially pointed out that the state of the system is constantly changing, so the process of system collaborative development is dynamic. Even if the system reaches the short-term collaborative goal, it will not keep the state unchanged all the time. It is necessary to continuously optimize the system by using external factors, so that the system can continuously evolve toward higher-level collaboration.
This essay believes that the following issues should be considered when studying the collaborative security of water-energy-food related system:
① The evaluation content should consider not only the condition attribute of the resource itself, but also the correlation attribute of the socio-economic environment and resources.
② The evaluation methods and results should reflect the security status and development trend of water, energy, food subsystems and the overall related system in the research area.
③ The evaluation methods and results should reflect the variables that play a leading role in the system collaborative security in the research area, as well as the interaction and coordination between subsystems, and whether there is a good promotion relationship. The collaborative security of the water-energy-food related system depends on the security situation of the subsystems. Therefore, the representation of the security situation of each subsystem can be decomposed, and the interrelationship with society and economy needs to be reflected. Therefore, the pressure state response model framework is adopted to construct the subsystem indicators as the research basis. Based on this, the research framework of the collaborative security of the water-energy-food related system is constructed, as shown in Figure 2.
Construction of multi-objective optimization model
Based on the analysis of the spatial-temporal change and driving mechanism of w-e-f coupling coordination level in a province, it is found that the primary factor affecting the change of coordination level is the relationship between supply and demand of resources, especially the pressure of water resources and energy caused by food demand. Therefore, in order to promote the coordinated development of w-e-f relationship in a province, this essay intends to carry out collaborative optimization of water resources and energy consumption in agricultural production. Considering that the irrigation water demand and energy consumption of various crops are obviously different, and the demand of crops is also replaceable to a certain extent, the resource pressure can be reduced by adjusting the crop planting structure (Chai and Yang, 2020; Song et al., 2021). Based on this, on the basis of reference to relevant research, this essay establishes a multi-objective optimization model to synergistically optimize the water and energy consumption of the agricultural system, and redistributes the limited available irrigation water and energy among various crops on the premise of meeting various constraints.
Objective function
According to the agricultural planting structure, major crops such as wheat, corn, potato and beans are planned. The planning period is 10 years, corresponding to 2021–2030. The objective function in this essay includes the minimization of irrigation water, the minimization of energy consumption and the maximization of crop yield. The formula is as follows (1), (2), and (3):
Where, t represents the planning period, t = 1−10; v is the crop type, v = 1−4 is wheat, corn, beans and potatoes; P(W),P(E) and P(F) are agricultural irrigation water, energy consumption and crop yield, respectively. SAMt,v is the sowing area of crop v in yeart, AWQtt,v is the crop water quota, θ is the effective utilization coefficient of irrigation water; PAMt,v is the energy consumption per unit area in the process of crop production, mainly composed of chemical fertilizer and pesticide energy consumption, agricultural machinery energy consumption and irrigation power consumption; ϕ is the agricultural energy utilization efficiency; OMFPt,v is the yield per unit area of crops.
Constraint condition
The constraints of W-E-F optimization include water, energy, food, land and environment.
(1) Land use restriction: During the planning period, the land area allocated to various crops must be smaller than the arable land area, and must meet the requirements of large crops.
(2) Irrigation guarantee quota: the total irrigation water required for normal crop growth shall not exceed the water requirement stipulated by law.
(3) Environmental limitations of fertilizers: excessive use of fertilizers (depending on the use of fertilizers and the use of high-quality fertilizers for each crop) can cause nitrogen, phosphorus and other pollution from non-agricultural sources (Ruan et al., 2020).
(4) Pesticide environmental constraints: excessive use of pesticides (depending on the pesticide demand of each crop and the effective utilization coefficient of sprayed pesticides) will produce environmental problems such as cod and eutrophication, so it needs to be controlled.
(5) Food security constraints: grain production should be stable and meet the basic food needs of residents.
(6) Crop planting area constraint: the total planting area of all crops shall not exceed the maximum sowable area.
(7) Non negative constraint: the decision variables in the solution process should be positive.
Determination of model parameters
In this essay, the basic data such as crop output and agricultural water resource consumption structure are obtained from the statistical yearbook and water resources bulletin. The demand for energy, water and agricultural products is determined according to the 14th 5 year plan of a province and the population change trend. The irrigation water demand of each crop is calculated according to the agricultural water quota of a province published in 2020. As the proportion of water-saving irrigation has been increasing in recent years, the effective utilization coefficient of irrigation water is calculated as per the increase of 0.02 every 5 years in the 13th 5 year plan for agriculture. The effective irrigation area in the planning period is calculated according to the average growth rate in the past 5 years. Figures 3–8 list some parameters related to crops, including crop water quota, crop yield per unit area under different conditions, crop pesticide, fertilizer demand and mechanical energy consumption.
Model solving
This essay adopts the weighted minimum deviation method to solve the multi-objective optimization model. The decision variable of the model is the sown area of different crops in the planning period, and the formula is as follows (4):
Among them, P(W), P(E), and P(F), respectively, represent the three objective functions in the model. P(W)max,P(F)maxandP(F)max, respectively, represent the maximum value of the three objective functions; P(W)min,P(E)minandP(F)min, respectively, represent the minimum values of the three objective functions; ω1,ω2, and ω3 are the weights of W-E-F objective function,ω1 + ω2 + ω3=1.
Model test
In order to test the reliability of the multi-objective optimization model, this essay takes 2019 as the comparative year. According to the agricultural planting structure of that year, various parameters are substituted for calculation. Finally, the deviation between the simulation results and the actual value of that year is compared (Dogra et al., 2020). At the same time, due to the lack of separate energy consumption data of the planting industry and the fact that this study does not cover all crops, the total energy consumption of the planting industry (direct energy consumption) is calculated according to the composition of the added value of the primary industry, and then the energy consumption of other crops is excluded according to the proportion of the sown area of various crops and the calculation method of the energy consumption of some crops in the existing literature, so as to obtain the total energy consumption of the research crops. Similarly, the actual amount of irrigation water, fertilizer and pesticide is also calculated by subtracting the consumption of other crops from the total amount. The results show that the errors between the model fitting value and the actual value are all within 5.34% (see Figure 9). Among them, the grain output reached 12.9 million tons, and the irrigation water consumption was slightly lower than the actual value. Therefore, it can be determined that the error of the model constructed in this essay is controllable and the optimization results have high reliability.
Optimization scheme design
Since each subsystem of w-e-f has different optimization requirements, and the optimization results produced by different target weight combinations are obviously different, three groups of weight scenarios are set in this essay (Figures 10–12). Scenario 1 aims to carry out balanced collaborative optimization. Accordingly, the three objective functions have the same weight. Scenario 2 focuses more on water resources objectives, with the weights of w-e-f objectives being 0.50, 0.25, and 0.25, respectively. Scenario 3 focuses on energy objectives, with the weights of 0.25, 0.50, and 0.25, respectively.
In addition, in order to show the advantages of the multi-objective optimization method, the water, energy and grain subsystems are, respectively optimized with single objective and solved with the same constraints as the multi-objective optimization.
Optimization scheme selection
Figure 13 shows the comparison of the results of different scenario optimization schemes under the credibility level of 0.8. Energy and water resources are expressed as the saving rate, while grain is expressed as the growth rate. It can be found that the objective function values under various scenarios have different characteristics. For scenario 2, water resource consumption can be significantly reduced, by 7.3% compared with 2019. However, in order to meet the constraint of grain production, energy saving is slightly lower than that in scenario 1. Under the condition of scenario 3, although the decline of energy consumption is the best, the advantages of the other two scenarios are not obvious, and the water resource saving will also reach the lowest value under the three scenarios (Shriram et al., 2021). The three objective function values of scenario 1 are in the middle position except for the high grain yield increase, which indicates that a certain coordination has been achieved among multiple objectives. Finally, scenario 2 ensures the growth of grain output on the basis of effectively reducing water resource consumption. Compared with scenario 3, the difference in energy consumption and grain output is smaller than the advantage brought by water resource saving. Compared with scenario 1, the effective saving of water resources is realized under the condition that the difference in energy and grain improvement is small.
Figure 14 shows that compared to the multi-objective optimization, the objective values obtained by the three optimization objectives all exceed the values of the multi-objective function. This shows that if the decision-making department does not consider the balance between resources, it can achieve the best single goal, but the other two goals will decline significantly. For example, taking water resources as a single objective will lead to a significant increase in energy consumption and a decline in grain production, which indicates that the single objective optimization effect is not ideal and should be optimized under the multi-objective scenario.
To sum up, since scenario 2 performs best in the multi-objective scheme and is more conducive to promoting the coordination of resources than single objective optimization, this essay studies the optimization scheme of w-e-f system in a province based on scenario 2.
Result analysis
Based on the above discussion, this essay will analyze the optimization results from the aspects of arable land resource allocation, water resource allocation and pesticide and fertilizer consumption, as follows:
(1) Allocation of arable land resources
During the planning period, the planting area of various crops will have different change trends. First, by 2030, the planting area of beans and potato crops will increase by 33.7 and 15.4%, respectively (Figure 15). Meanwhile, as the main food crops, the planting area of wheat will increase first and then decrease with the change of population, and will eventually decrease by 22.9%. The planting area of the main crop corn will increase by 1.2%. This is caused by the difference in water energy consumption of different crops. Among them, the water energy consumption of wheat production is significantly higher than that of other crops, but the wheat yield of a province is significantly lower than that of Northern Henan at the same latitude. At the same time, the improvement of living standards and the decline of population will also reduce the demand for rations. Therefore, the decline of wheat planting area is relatively high (Zhao et al., 2019). At the same time, with the diversification of food sources, the demand for potato crops may continue to increase, and the strategy of import substitution will inevitably require increasing the sown area of legumes, and increasing the rotation area will also help to maintain soil fertility. Therefore, in the future, policymakers need to adjust planting strategies according to resource constraints, climate conditions and agricultural infrastructure conditions.
(2) Water resources allocation
Therefore, it is particularly urgent to optimize the distribution of limited agricultural irrigation water resources on the basis of ensuring the basic food demand. Due to the adjustment of crop planting structure and the improvement of water resource utilization efficiency, the water resource consumption of various crops will change significantly by 2030. The optimization results show that the irrigation water consumption of most crops shows a downward trend in the planning period. Among them, the irrigation water consumption of wheat will be reduced from 1.25 billion in 2019 to 860 million, and the irrigation water consumption of corn will be reduced from 2.46 billion to 2.297 billion, which will help reduce the pressure of agricultural production on the water resources system, especially for Yuncheng City, the main wheat production area.
(3) Fertilizer and pesticide consumption
At present, the policies of soil testing, formula fertilization and organic fertilizer application that have been promoted can effectively reduce the use of chemical fertilizer. Due to the decrease of wheat planting area with high fertilizer demand and the increase of bean and potato planting area, the demand for agricultural fertilizer will also decrease significantly. In addition, the environmental protection policy will also limit the excessive use of chemical fertilizer, and promote the improvement of the utilization rate and purity of chemical fertilizer, thus contributing to the reduction of agricultural non-point source pollution (Xie et al., 2021; Huang and Yang, 2022). The optimization results show that by 2030, the amount of chemical fertilizer applied to various crops will decrease to varying degrees, with an overall decrease of 16.4%, among which, the amount of chemical fertilizer used for corn and wheat will decrease to 596,000 and 159,000 tons, respectively.
The existing policies show that the government departments strictly control the amount of pesticides by formulating pollutant emission policies to ensure food and ecological safety. Due to the planting structure and economic benefits, corn and wheat have become the main consumption of pesticides, accounting for 83.4% of the grain crops. By 2030, the improvement of fertilizer and pesticide utilization efficiency and the promotion of policies will reduce the pesticide consumption of crop production by 9.3%. At the same time, the large-scale construction of high-standard farmland and the emphasis on dry farming will reduce the impact of climate change on grain production and effectively increase the grain yield of dry land, thus offsetting the impact of the decline in wheat planting area. Therefore, the multi-objective planning scheme can effectively alleviate the conflicts caused by different pesticide use preferences in water resources, energy and agricultural sectors.
To sum up, during the planning period, due to the priority of water resources and energy systems, the increase of water-saving irrigation area and the control of environmental impact, the consumption of irrigation water and pesticides and fertilizers will show a downward trend. By 2030, the irrigation water consumption of major grain crops can be reduced by 7.3% compared with 2019, but the total grain output will still maintain a small growth. In addition, as the improvement of agricultural production mechanization will offset the contribution of chemical fertilizer reduction to the reduction of energy consumption, the reduction of energy consumption of grain crops is relatively small, and it is expected to be reduced by 2.5% by 2030. In short, with the application of advanced technology, the grain production capacity will be significantly improved, and the ability to resist risks will also be significantly enhanced. For example, due to the promotion of degradable plastic film and other technical means, the grain yield of dry land will also be improved. However, in consideration of the need to reduce the pressure on water resources and energy, the increase of the actual grain output will be limited, and it is expected to remain at about 14.04 million tons.
Conclusion
In short, in order to realize the collaborative optimization of local w-e-f, we should not only pay attention to the resource system itself, but also start from the external factors such as economy, society and environment to improve the sustainable utilization ability of w-e-f resources. Social factors such as population, the gap between the rich and the poor, dietary preference and urbanization, as well as economic factors such as industrial structure, wealth distribution and infrastructure, are important initial conditions that affect the resource demand of w-e-f, while ecological carrying capacity is a necessary condition to ensure the reproduction of w-e-f. In the future, urbanization and economic development may still promote energy and food demand, while the decline of the total population and the optimization of the industrial structure will help offset this effect. Meanwhile, the continuous optimization of the ecological environment is conducive to ensuring the stable supply of w-e-f resources. Therefore, on the basis of accurately grasping the internal and external relations of w-e-f, it is necessary to formulate reasonable action plans for possible changes in various fields, especially to improve the ability to resist various risks. Therefore, it may be necessary to carry out cross departmental policy formulation and coordination. According to the multi-objective collaborative optimization system, decision-makers can reduce water resources and agricultural effort to a certain extent by adjusting planting methods and improving optimal water use while satisfying various constraints. At the same time, reduce the use of chemical fertilizers and pesticides to reduce the excessive pressure on resources and the environment. In addition, compared with single-objective optimization, multi-objective optimization can reduce the tradeoff of resources.
Data availability statement
The original contributions presented in this study are included in the article/supplementary material, further inquiries can be directed to the corresponding author.
Author contributions
YR and WP helped XR complete all the process of essay. All authors contributed to the article and approved the submitted version.
Funding
This research was supported by funds from the National Natural Science Foundation of China (No. 52009019) and the Natural Science Foundation of Heilongjiang Province (No. LH2019E012).
Conflict of interest
The authors declare that the research was conducted in the absence of any commercial or financial relationships that could be construed as a potential conflict of interest.
Publisher’s note
All claims expressed in this article are solely those of the authors and do not necessarily represent those of their affiliated organizations, or those of the publisher, the editors and the reviewers. Any product that may be evaluated in this article, or claim that may be made by its manufacturer, is not guaranteed or endorsed by the publisher.
References
Gnanadesikan, A., Speller, C. M., Ringlein, G., Soucie, J. S., and Pradal, M. A. (2020). Feedbacks driving interdecadal variability in southern ocean convection in climate models: A coupled oscillator mechanism. J. Phys. Oceanogr. 50, 2227–2249. doi: 10.1175/JPO-D-20-0037.1
Liu, B., Zu, S., Wang, H., Xia, J., and Ding, N. (2020). Influence mechanism of visual perception of edge rate lines cycle length on driver’s speed. Transport 36, 38–45. doi: 10.3846/transport.2020.12372
Chikozho, C., Managa, L. R., and Dabata, T. (2020). Ensuring access to water for food production by emerging farmers in south africa: What are the missing ingredients? Water S.A 46, 225–233. doi: 10.17159//wsa/2020.v46.i2.8237
Lu, C., Hong, W., Wang, Y., and Zhao, D. (2021). Study on the coupling coordination of urban infrastructure and population in the perspective of urban integration. IEEE Access 9, 124070–124086. doi: 10.1109/ACCESS.2021.3110368
Forbes, D. L., Meneely, K. M., Chilton, A. S., Lamb, A. L., and Ellis, H. R. (2020). The 3his metal coordination site Promotes the coupling of oxygen activation to cysteine oxidation in cysteine dioxygenase. Biochemistry 59, 2022–2031. doi: 10.1021/acs.biochem.9b01085
Yan, G., and Qin, Q. (2020). The application of edge computing technology in the collaborative optimization of intelligent transportation system based on information physical fusion. IEEE Access 8, 153264–153272. doi: 10.1109/ACCESS.2020.3008780
Wang, G. B., Zhao, Y. F., Wang, C., Wang, X. C., Ma, J., Li, X. D., et al. (2021). Multi-level peer-to-peer collaborative optimization of smart energy system based on big data analysis. J. Phys. Conf. Ser. 1965, 79–85. doi: 10.1088/1742-6596/1965/1/012145
Song, H., Zhou, S., Chang, Z., Su, Y., and Yang, J. (2021). Collaborative processing and data optimization of environmental perception technologies for autonomous vehicles. Assembly Autom. 41, 283–291. doi: 10.1108/AA-01-2021-0007
Xie, H., Wang, Y., Gao, Z., Ganthia, B., and Truong, C. (2021). Research on frequency parameter detection of frequency shifted track circuit based on nonlinear algorithm. Nonlinear Eng. 10, 592–599. doi: 10.1515/nleng-2021-0050
Dogra, J., Jain, S., Sharma, A., Kumar, R., and Sood, M. (2020). Brain tumor detection from MR images employing fuzzy graph cut technique. Recent Adv. Comput. Sci. Commun. 13, 362–369. doi: 10.2174/2213275912666181207152633
Li, J., Wang, W., Li, B., Tan, J., and Peng, B. (2021). Directionality of butterfly leaves and nonuniform deformation mechanism in gob-side entry driving roadway. J. Mech. 37, 291–301. doi: 10.1093/jom/ufab004
Lv, J., Li, Y. P., Huang, G. H., Suo, C., and Li, Y. (2020). Quantifying the impact of water availability on china’s energy system under uncertainties: A perceptive of energy-water nexus. Renew. Sustain. Energy Rev. 134:110321. doi: 10.1016/j.rser.2020.110321
Ruan, L., Yan, Y., Guo, S., Wen, F., and Qiu, X. (2020). Priority-based residential energy management with collaborative edge and cloud computing. IEEE Trans. Industr. Inform. 16, 1848–1857. doi: 10.1109/TII.2019.2933631
Luo, Q., Wang, Y., Liu, L., Chen, N., and Wei, J. (2021). The optimization of hydraulic and electric power system considering the multi-particle swarm optimization algorithm. J. Phys. Conf. Ser. 1992:032130. doi: 10.1088/1742-6596/1992/3/032130
Huang, R., and Yang, X. (2022). Analysis and research hotspots of ceramic materials in textile application. J. Ceramic Process. Res. 23, 312–319.
Shriram, S., Jaya, J., Shankar, S., and Ajay, P. (2021). Deep learning-based real-time AI virtual mouse system using computer vision to avoid COVID-19 spread. J. Healthc. Eng. 2021:8133076. doi: 10.1155/2021/8133076
Yang, S. (2020). Regional coupling coordination degree between new urbanization and water ecological civilization in china, 2009-2018. Ecol. Econ. 16, 18–27.
Chai, T. Y., and Yang, T. (2020). Research status and prospects of distributed collaborative optimization. Sci. Sin. Technol. 50, 1414–1425. doi: 10.1360/SST-2020-0040
Jiang, W., Zou, D., Zhou, X., Zuo, G., and Li, H. J. (2020). Research on key technologies of multi-task-oriented live maintenance robots for ultra high voltage multi-split transmission lines. Industr. Robot 48, 17–28. doi: 10.1108/IR-03-2020-0066
Xu, W., Zhang, X., Xu, Q., Gong, H., Li, Q., Liu, B., et al. (2020). Study on the coupling coordination relationship between water-use efficiency and economic development. Sustainability 12:1246. doi: 10.3390/su12031246
Zhong, W., and Zhang, D. (2020). Study on coupling mechanism of carbon-water symbiosis system and regulation and control of water resources in the context of regional collaborative development. IOP Conf. Ser. Earth Environ. Sci. 546:032036. doi: 10.1088/1755-1315/546/3/032036
Zhao, X. L., Liu, X., Liu, J., Chen, J., Fu, S., and Zhong, F. (2019). The effect of ionization energy and hydrogen weight fraction on the non-thermal plasma vocs removal efficiency. J. Phys. D Appl. Phys. 52:145201. doi: 10.1088/1361-6463/aafe8b
Cao, Y., Wang, L., Jiang, S., Yang, W., and Guo, X. (2020). Optimal operation of cold–heat–electricity multi-energy collaborative system based on price demand response. Glob. Energy Interconnection 3, 430–441. doi: 10.1016/j.gloei.2020.11.003
Qi, Y., Farnoosh, A., Lin, L., and Liu, H. (2022). Coupling coordination analysis of china’s provincial water-energy-food nexus. Environ. Sci. Pollut. Res. 29, 23303–23313. doi: 10.1007/s11356-021-17036-x
Keywords: water-energy-food, coupling coordination, driving mechanism, collaborative optimization, energy consumption
Citation: Ren X, Ren Y and Pei W (2022) Study on coupling coordination and collaborative optimization system of water-energy-grain. Front. Ecol. Evol. 10:1028463. doi: 10.3389/fevo.2022.1028463
Received: 26 August 2022; Accepted: 08 September 2022;
Published: 03 November 2022.
Edited by:
Hamzeh Ghorbani, Islamic Azad University, IranReviewed by:
Sahar Lajmorak, Institute for Advanced Studies in Basic Sciences (IASBS), IranNima Mohamadian, Islamic Azad University, Iran
Copyright © 2022 Ren, Ren and Pei. This is an open-access article distributed under the terms of the Creative Commons Attribution License (CC BY). The use, distribution or reproduction in other forums is permitted, provided the original author(s) and the copyright owner(s) are credited and that the original publication in this journal is cited, in accordance with accepted academic practice. No use, distribution or reproduction is permitted which does not comply with these terms.
*Correspondence: YongTai Ren, ytren@neau.edu.cn