- 1Departamento de Biologia, Faculdade de Filosofia, Laboratorio de Comportamento e Ecologia de Insetos Sociais, Ciências e Letras de Ribeirão Preto, Universidade de São Paulo, São Paulo, Brazil
- 2Department of Biology, Laboratory of Socioecology and Social Evolution, University of Leuven, Leuven, Belgium
- 3Department of Genetics, Evolution and Environment, University College London, London, United Kingdom
Juvenile hormone (JH) affects multiple physiological traits in insects. In social insects, besides development, JH has been demonstrated to influence caste determination and the production of chemical compounds. In social wasps, JH triggers behavioral maturation, gonadotropic effects, and hydrocarbon modulation. Here, we investigated whether JH displays the same function in fertility and fertility cue production in females of the German wasp Vespula germanica, previously shown in the related species Vespula vulgaris. By experimentally treating workers with JH-analog, an anti-JH, and acetone solvent control, we tested whether JH modulates the cuticular chemical expression (CHCs), the Dufour’s gland chemical composition, and hence the compounds found over the egg’s surface. Additionally, we explored whether JH has a gonadotropic effect on workers. Workers treated with the JH-analog acquired a chemical profile that was intermediate between the queen and other treated workers. Interestingly, the same pattern was also seen in the Dufour’s glands and eggs, although more subtle. Furthermore, workers treated with the JH-analog were more fertile when compared to the controls, supporting the fact that JH acts as a gonadotropic hormone. Our results indicate a similar function of JH in societies of related wasp species V. germanica and V. vulgaris.
Introduction
Juvenile hormone (JH) represents a family of acyclic sesquiterpenoids that are responsible for controlling several physiological traits in insects, from development to sexual maturation (Hartfelder, 2000; Noriega, 2014; Huang, 2020). JH was primarily known to maintain juvenile features in the larval stage during metamorphosis, but later, JH became known for its pleiotropic effects, responsible for regulating immature development, reproduction, aggressive behavior, behavioral maturation, caste determination, diapause, stress response, dispersal and flight activities, and many other polyphenisms (Hartfelder, 2000; Goodman and Cusson, 2012; Jindra et al., 2015). For instance, in solitary insects such as the Bombyx mori, JH plays a role in controlling metamorphosis (Kamimura and Kiuchi, 1998; Zhang et al., 2017). In the fruit flies Drosophila melanogaster, JH controls reproductive traits (Raushenbach et al., 2004; Yamamoto et al., 2013), and in cockroaches, JH likely influences aggressive behavior (Kou et al., 2008). In eusocial insects, as the model honeybee, such as Apis mellifera, JH has acquired a role to directly regulate the age polyethism in workers (Robinson, 1987), whereas in the primitively eusocial bee Bombus terrestris, higher JH affects aggressiveness and dominance rank in females (Pandey et al., 2020). In ant societies, JH displays a variety of roles depending on the species, from reproductive maturation to caste determination. In the ants Harpegnathos saltator and Cardiocondyla obscurior, JH seems to be linked with caste determination, as higher doses of JH induce larvae to become reproductive adults (Schrempf and Heinze, 2006; Penick et al., 2012). In societies of the primitively eusocial ant Dinoponera quadriceps, JH function may have been remodeled during the evolution of the species, instead of triggering gonadotropic effects (increase ovary activity in females), it inhibits reproduction in high-rank workers, as treated workers show a decreased number of vitellogenic eggs and overall smaller ovarioles (Norman et al., 2019). Contrary to the general gonadotropic effect in insects, JH negatively impacts reproduction in some species of ants (Holman, 2012; Azevedo et al., 2016; Pamminger et al., 2016a,b; Norman et al., 2019).
In wasps, JH acquired multiple functions in the species, which vary among the different levels of sociality. In primitively eusocial species, JH displays a gonadotropic effect in females in Polistes annularis (Barth et al., 1975), Ropalidia marginata (Agrahari and Gadagkar, 2003), Polistes metricus (Tibbetts and Sheehan, 2012), Polistes canadensis (Giray et al., 2005), Polistes fuscatus (Walton et al., 2020), and Polistes dominula (Oi et al., 2021b); JH regulates behavior (onset of foraging and aggressive acts) in P. canadensis (Giray et al., 2005) and P. dominula (Shorter and Tibbetts, 2009; Tibbetts and Izzo, 2009; Tibbetts et al., 2013); and JH affects the production of chemical compounds in Mischocyttarus consimilis (Neves et al., 2020), P. dominula (Oi et al., 2021b), Polistes satan (Oi et al., 2021b), Mischocyttarus cassununga (Oi et al., 2021b; Ferreira et al., 2022), and Mischocyttarus cerberus (Ferreira et al., 2022). In swarm-founding species, JH has been reported to control behavioral maturation in Polybia occidentalis (Prato et al., 2021), and ovary activation and chemical expression of CHCs in P. occidentalis (Prato et al., 2021) and Synoeca surinama (Kelstrup et al., 2014). In highly eusocial wasps, JH effects have been well studied so far in a single species, namely Vespula vulgaris, and one of the roles is being associated with regulating reproductive traits in females, leading to modification of certain cuticular compounds over the cuticle (Oliveira et al., 2017; Oi et al., 2020, 2021a). By treating workers topically with a JH analog (methoprene), studies in the past have supported that in addition to the regulation of CHC production, JH may be directly responsible for increasing the proportion of queen pheromones in V. vulgaris (the compounds n-C27, n-C28, n-C29, and 3-MeC29) (Oliveira et al., 2017; Oi et al., 2021a). Furthermore, in V. vulgaris, increasing JH levels may be decisive for reproductive workers to succeed (Oi et al., 2020), as workers treated with methoprene lay eggs that chemically resemble those laid by queens, and in doing so, their eggs were less policed (Oi et al., 2020).
The Dufour’s gland is the main source of egg-maternity cues in social insects, as this gland is associated with the sting apparatus in females of several Hymenoptera (Apocrita) (Mitra, 2013). This gland may have acquired other roles across the evolution of hymenopteran species (Mitra, 2013) and one of the key functions of this gland is to release chemical compounds that cover the eggs during oviposition (Abdalla and Cruz-Landim, 2001). Besides that, Dufour’s gland compounds are used during nest building, nestmate recognition, fertility signaling, brood cell lining, and as sexual pheromones (Mitra, 2013). Given the existing evidence that V. vulgaris workers treated with methoprene lay eggs that chemically resemble those laid by the queens (Oi et al., 2020), it is likely that increasing JH levels have cascade effects, that not only trigger cuticle chemical modification (Oi et al., 2021a) but also trigger a modification in other chemical reservoirs, such as the Dufour’s gland. Hence, the chemical modification in the eggs laid by methoprene-treated workers in V. vulgaris may represent a modification in Dufour’s gland composition.
Here, we evaluated whether JH is responsible for controlling chemical expression and reproduction in the German wasp Vespula germanica. First, we experimentally manipulated the levels of JH in workers by treating them with either JH analog methoprene, anti-JH precocene, or solvent-only acetone as a control. We sampled their CHCs, eggs, and Dufour’s glands to investigate their chemical composition and their level of chemical similarity. Then, treated workers were compared with fertile queens to identify putative fertility cues (chemical cues associated with their reproductive state). Finally, we estimated fertility by counting the number of oocytes in the ovaries of treated workers. Considering that JH acts as a pleiotropic hormone, regulating fertility and fertility signaling in the sister species V. vulgaris (Oi et al., 2021a), we hypothesized that JH effects would be conserved in V. germanica. Because workers have lower JH levels when compared to queens in V. vulgaris (Oliveira et al., 2017), we expected that CHCs, eggs, and Dufour’s glands from methoprene-treated workers would chemically resemble those from fertile queens, and the opposite would be seen for the samples from solvent and precocene-treated workers. Similarly, we expected methoprene-treated workers to be more fertile compared to precocene- and solvent-treated workers.
Materials and methods
Study site and nest sampling
Thirteen nests of V. germanica were collected between August and September 2019 in the surroundings of Leuven, Belgium (50° 52′ 46′′ N; 4° 42′ 3” E, 41 m elevation). Nests were collected from the ground or attics, then they were kept in plastic boxes and taken to the Laboratory of Socioecology and Social Evolution at the University of Leuven, Belgium.
Experimental setup
Once in the lab, the wasp nest was sedated with CO2 and the combs containing pupae were maintained in plastic boxes for 24 to 48 h to sample newly emerged workers. Queens were sampled and stored in the freezer until further analysis. Eggs present in the original combs coming from the field were sampled and categorized as queen-laid eggs in further analysis. Under queen-right conditions, worker-laid eggs are efficiently removed by policing workers (Bonckaert et al., 2008). A total of five queen-laid eggs were sampled and stored in the freezer until further analysis. Newly emerged workers (0 days old) were topically treated on their abdomen with either 5 μl JH analog methoprene (20 μg/μl) (Sigma-Aldrich), 5 ul anti-JH precocene (15 μg/μl) (Sigma-Aldrich), or 5 μl of the solvent acetone (Sigma-Aldrich) as control. Those concentrations and single topical application were shown to be effective for the sister species V. vulgaris (Oliveira et al., 2017; Oi et al., 2020, 2021a). After being treated, the workers (ca. 20 individuals) were settled in experimental wooden boxes corresponding to their treatments. Wooden boxes consisted of two parts (similar to Oi et al., 2021a). The first part (nest box) contained a comb in which the eggs were removed (to allow workers to lay eggs) and ranged with a metal wire. The second part (foraging box) contained falcon tubes with sugar syrup, sugar paste (Apifonda), and pieces of mealworm. The nest and foraging boxes were connected by a hole (Supplementary Information in Supplementary Figure 1). The experiments were kept in a climate room, under controlled conditions for a week (12-h cycle, 28°C). After a week, the wasps were sedated, and the females were individualized in glass vials kept in a freezer −20°C for chemical extractions and dissections. The combs were stored in the freezer (−20°C) to keep the worker-laid eggs preserved until being further chemically analyzed.
Chemical extraction and analyses
We individualized queens and workers in glass vials, and we filled them with 2 ml (queens) or 1 ml (workers) of pentane (Acros organics, HPLC) to extract CHCs. After 1 minute in contact with the solvent, the wasps were removed from the glass vials, and they were all preserved again in the freezer until Dufour’s gland dissections. To sample the Dufour’s gland, the abdominal tagma of each female was dissected under a stereomicroscope. The female was pinned in a Petri dish containing a saline solution, a small incision was made on each side of the terminal part of their abdomen. The abdomen was exposed to sample the Dufour’s gland. Once the Dufour’s gland was located, we used tweezers to sample it and put it in a glass insert filled with 50 μl of pentane. Once in the glass insert, the Dufour’s gland was smashed with the aid of the tweezers. Queen-laid and worker-laid eggs (from each of the three treatments) were individualized in glass inserts. All the eggs were washed with 50 μl of pentane for a minute, after that, all the eggs were removed from the solution. Each sample corresponds to one sampled egg. After the extraction procedure, all the samples were let dry under the fume hood at room temperature. Glass vials containing CHCs were refilled with 1,000 μl (queens) and 200 μl (workers) of hexane (HiPerSolv CHROMANORM, HPLC). Glass vials containing chemical extracts from eggs and Dufour’s gland were refilled with 50 μl of hexane. All the samples were analyzed in a system of Gas Chromatography coupled with Mass Spectrometry (GC-MS) (Thermo Fisher Scientific Trace 1,300 connected to a Thermo Fisher Scientific ISQ mass spectrometer). In the GC, a column of 30 meters with a diameter of 0.25 mm and a 0.25 um film was used (Restek MXT-5). From each sample, 1 μl was injected at a temperature of 320°C using a split-lees injection mode (similar conditions used by Oi et al., 2020, 2021a,b). The initial temperature was set to 40°C and kept for 2 min. Then, the temperature was increased to 120°C with an increased rate of 20°C per min. After that, the temperature increased to 200°C at a rate of 10°C per minute, and at a rate of 7°C per min until 250°C. Finally, the last increase of temperature consisted of 5°C per minute until 350°C, which was held for 4 min. The GC-MS parameters used in our work were based on previously published studies (Oi et al., 2020, 2021a,2021b). We used helium as carrier gas under a constant flow rate of 0.9 ml per minute. The temperature of the MS electric ionization and MS transfer line was kept at 300°C. To identify the hydrocarbons and calculate retention indexes, samples of external alkane ladders (Sulpeco) ranging from n-C7 to n-C40 were run. Ion chromatograms were integrated using R studio Version 1.4.1717 (R version 4.1.0) with a script provided in the Supplementary material. After integration, we extracted the peak area which was converted into relative abundances to be used in the statistical analysis. We performed multivariate and univariate analyses to check whether the dependent variables (chemical compounds) were affected according to the different treatments. Groups of females (queens, methoprene, precocene, and acetone) belonging to the three types of samples (CHCs, eggs, and Dufour’s gland) were compared using a PERMANOVA test (9,999 permutations) to check whether they varied according to their chemical composition. For that, we used the package vegan (Oksanen et al., 2015) in R. The data corresponding to CHCs, eggs, and Dufour’s gland were plotted using a principal component analysis (PCA) to visualize data distribution and group formation. To check which compounds contributed the most to group differentiation, a percentage similarity test (SIMPER) using a Bray–Curtis index was performed with the package vegan (Oksanen et al., 2015). To check the level of similarity among samples of CHCs, eggs, and Dufour’s gland from the four groups of females, the differences in compound abundancy were analyzed by using ANOVA on the log-transformed relative peak areas per compound. The ANOVA test was followed by a Tukey post hoc test with Bonferroni corrections to compare the abundance of compounds between groups of samples per group of females. For that, the function contrast from the package lsmeans (Bates et al., 2015) was used. To observe clustering based on their chemical information, the function pheatmap from the package pheatmap (Kolde, 2015) was used and UPGMA was utilized as the clustering method using 1-Pearson correlation for the distance matrix (adapted from Oi et al., 2021a).
Dissections
Workers were dissected in ringer solution under a stereomicroscope (Leica, model: MSV266). The total number of oocytes was counted and was used as a proxy for investigating the effects of treatments on the females’ reproduction. We included the measurements of head size as a proxy of size for the individuals. We measured head width at their widest part from the head frontal view. Head measurements were conducted using the software Image J. We ran a Spearman correlation test to evaluate if head width and number of oocytes were correlated. Head width and the number of oocytes were not significantly correlated (rho = −0.036; p-value = 0.770), thus head width was not included as a factor in the following analysis. To analyze the effects of the treatments on the reproductive traits of females, data were analyzed by using a generalized linear mixed model with Poisson distribution, compared to the glmer function from the lme4 (Bates et al., 2015) package. As we checked overdispersion in our data, using the function dispersion_glmer from blmeco package (Korner-Nievergelt et al., 2015), we fitted a negative binomial model that gave the best AIC value. The model was fitted with the total number of counted oocytes as a fixed effect and nest origin as a random factor. For pairwise comparison among the treatments, we used the function emmeans adopting fdr correction (Bates et al., 2015). All analyses and graphics were done in R studio version 1.4.1717 (R version 4.1.0) (R Core Team, 2021).
Results
The number of samples that were included in chemical analyses were as follows: CHCs – 4 queens, 25 workers treated with acetone, 21 workers treated with methoprene, and 25 workers treated with precocene; Eggs – 27 queen-laid eggs, 20 eggs laid by workers treated with acetone, 23 eggs laid by workers treated with methoprene, and 24 eggs laid by workers treated with precocene; Dufour’s gland – 8 samples for queens, 25 samples for workers treated with acetone, 15 samples for workers treated with methoprene, and 24 samples for workers treated with precocene. Some samples of CHCs and Dufour’s gland were removed due to the quality of the chromatograms.
Cuticular hydrocarbons
A total of 31 chemical compounds were detected in the samples (Supplementary Table 1). The permutation analysis showed that the CHCs from different groups of females were statistically different (F value = 27.000, p < 0.001***) (Table 1). The post-doc permutation analysis showed that the CHCs differed significantly when the groups were compared in pairs (Table 1). According to the SIMPER analysis, among the 10 first compounds, several differed between the pairs of females (Supplementary Table 4). However, the most contrasting variation was detected between queens versus all the groups of treated workers (Figure 1A and Supplementary Figure 2). Looking at the first 10 compounds highlighted by SIMPER analyses, workers treated with methoprene were the most different group if compared with workers treated with acetone or precocene. Interestingly, the pattern also exists including or excluding non-treated workers (Supplementary material in Supplementary Figure 2). A total of four and three compounds were statistically different in the pairs “acetone × methoprene” and “methoprene × precocene,” respectively. On the other hand, none of the first 10 compounds differed between workers treated with acetone and precocene.
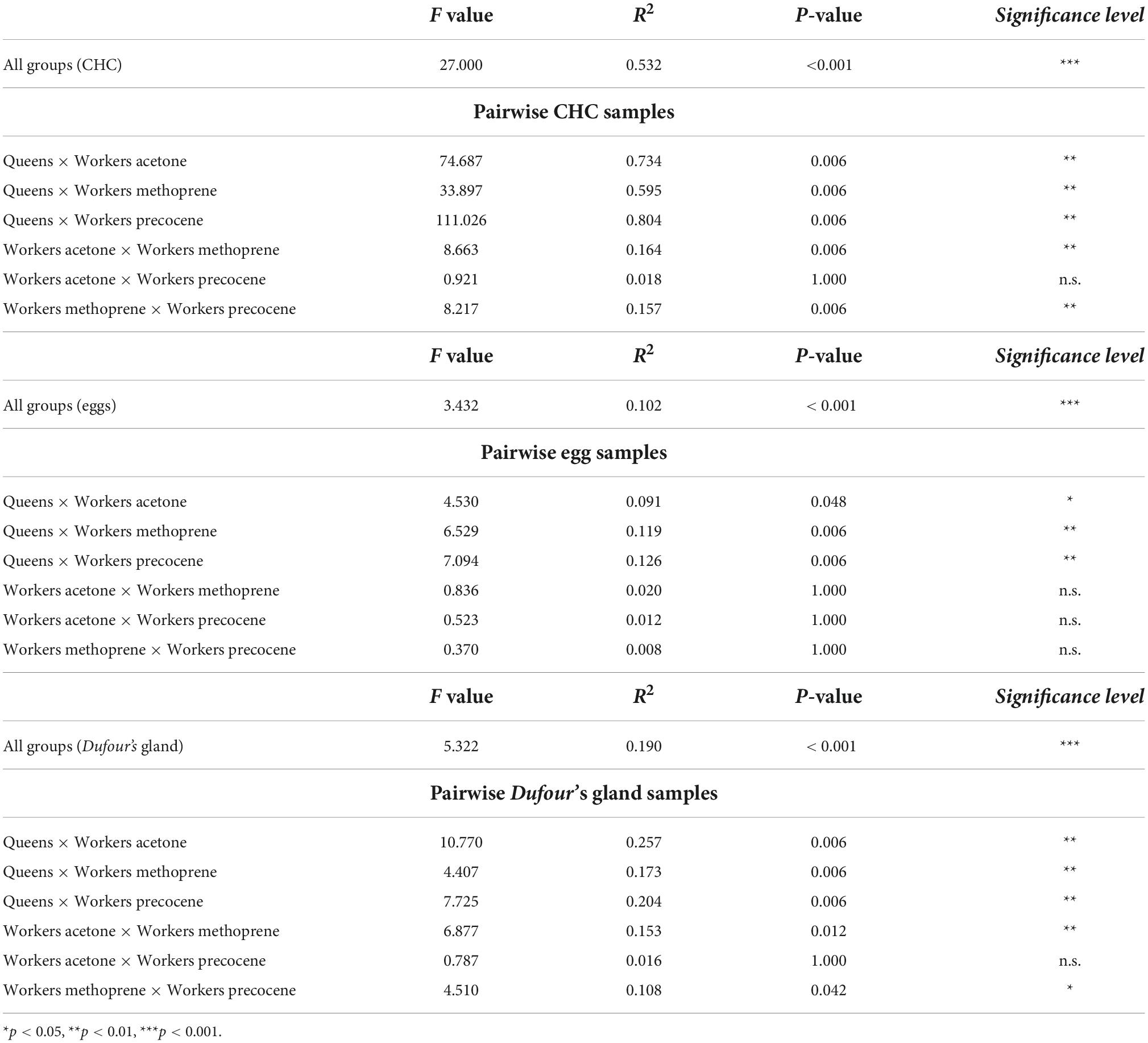
Table 1. Permutation analysis (PERMANOVA) represented by a group of samples in Vespula germanica: cuticular hydrocarbons (CHC), eggs, and Dufour’s gland.
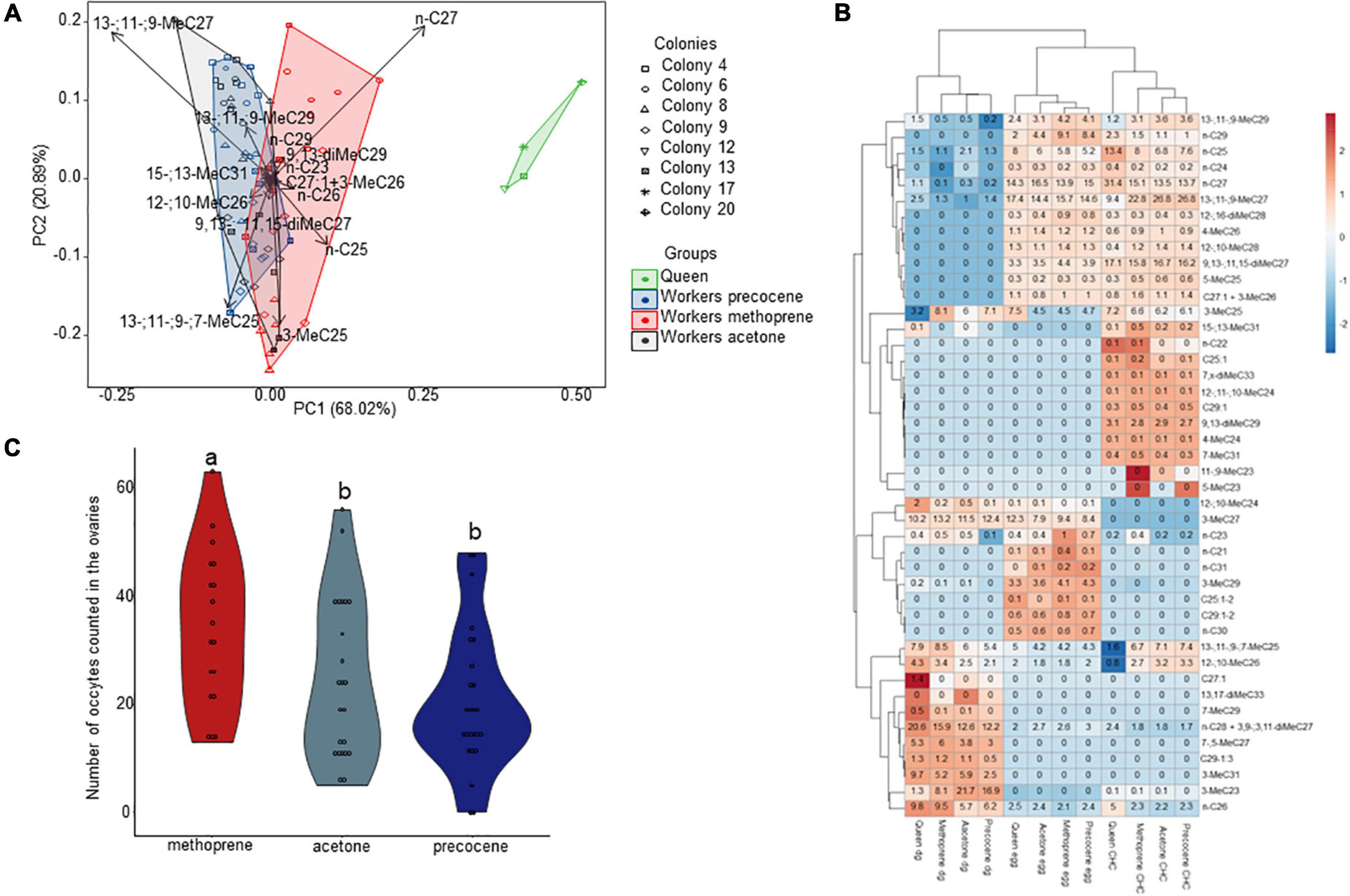
Figure 1. (A) Principal component analysis (PCA) showing cuticular hydrocarbons (CHCs) differences in Vespula germanica. The symbols indicate the origin of the colonies and colors correspond to groups of females, queens, or treated workers. (B) Heatmap based on the chemical compounds of the different groups of samples, cuticular hydrocarbons (CHC), eggs, or Dufour’s gland (dg) for all the groups of females (queens, workers treated with methoprene, workers treated with acetone, and workers treated with precocene). Numbers in the heatmap represent the log-transformed relative peak areas per compound and the background colors represent the z-scores. (C) The total number of oocytes in the ovaries of Vespula germanica workers was greater in methoprene-treated workers compared to acetone- and precocene-treated workers. Different letters represent statistical differences among treated workers.
Eggs
A total of 27 chemical compounds were detected on the surface of eggs (Supplementary Table 2). The permutation analysis indicated that the hydrocarbons covering the eggs from different groups were statistically different (F value = 3.432, p < 0.001***) (Table 1). The SIMPER analysis showed that linear, methylated, and dimethylated compounds were responsible for group differentiation (Supplementary Table 4). The hydrocarbons n-C25, 3-MeC25 and 3-MeC27 were seen to be abundant over the surface of queen-laid eggs, which make them putative queen egg-marking compounds (Supplementary Table 2). Overall, the chemical composition of eggs laid by the different groups of females overlapped in the PCA analyses (Supplementary Figure 3), thus the chemical variation in eggs laid by different groups of females is subtle and restricted to certain compounds.
Dufour’s gland
A total of 23 chemical compounds were detected in samples of Dufour’s glands (Supplementary Table 3). The permutation analysis showed that the hydrocarbons found in the Dufour’s glands of females were statistically different (F value = 5.322, p < 0.001***) (Table 1). The post hoc permutation analysis revealed that almost all the six pairs were statistically different (p < 0.05*) (Table 1). Hydrocarbons from the four classes of compounds detected in samples of Dufour’s gland (linear, methylated, dimethylated alkanes, and alkenes) were found to contribute to group differentiation (Supplementary Table 4). The variation among females for the Dufour’s gland is restricted to specific chemical compounds (high overlap among samples) as shown for the eggs (Supplementary Figure 4).
Similarity among samples
Samples were primarily clustered based on their original groups regardless of female category; however, a higher similarity was detected between CHCs and egg-marking cues (Figure 1B). CHC and egg samples clustered together in the same upper branch, whereas Dufour’s gland samples clustered in a different branch. Pairwise comparison per compound showed that several compounds were statistically different among samples (Supplementary Table 5).
Effects on reproduction
A total of 66 workers (acetone = 23; methoprene = 18; precocene = 25) of V. germanica were dissected. Two pairwise comparisons were significantly different. Methoprene-treated workers had more oocytes in their ovaries and were significantly different from either acetone-treated workers (z ratio = −2.487, p = 0.019*) or precocene-treated workers (z ratio = 3.782, p < 0.001***). Precocene-treated workers and acetone-treated workers did not have different numbers of oocytes in their ovaries (z ratio = 1.402, p = 0.161). Different letters represent statistical differences among treated workers.
Discussion
Our initial hypothesis that JH has a conserved function underlying chemical modulation and reproduction in Vespula wasps was corroborated. CHCs varied according to caste as queens showed to be different from workers. Furthermore, we showed that workers treated with the JH analog methoprene expressed chemical profiles that were intermediate between queens and the other two worker groups (acetone- and precocene-treated workers). We detected a subtle colony effect over the CHC dataset, which could be due to the fact that CHCs also play a role in nestmate recognition. We also demonstrated a higher chemical similarity between CHC and egg samples, meaning the same compounds are present in the two sources. Additionally, workers treated with methoprene had more developed oocytes in their ovaries compared to workers from the other two groups. The chemical changes were more pronounced in the CHC samples, whereas the variation for egg and Dufour’s gland samples were more subtle. These results provide additional support for the hypothesis that JH has pleiotropic properties, being responsible for regulating several processes in social wasps, such as ovary activation, behavioral maturation, oviposition, and the modulation of chemical compounds (Oliveira et al., 2017; Oi et al., 2021a,b; Prato et al., 2021). JH not only modulates the production of CHCs, as it has been previously demonstrated for wasp species (Oliveira et al., 2017; Oi et al., 2021a,b; Prato et al., 2021), but also can potentially modulate other components, such as the Dufour’s gland composition.
Our results are in line with previous reports of chemical composition in V. germanica (queens, workers, virgin queens, and males), that CHCs vary in quantity and are probably important to mediate intranidal interactions (da Silva et al., 2021). One key hydrocarbon that has been reported to be queen-characteristic was the linear alkane n-C27, which was also found in higher proportions in our queen samples, and can potentially be a candidate for the queen pheromone in V. germanica (da Silva et al., 2021). This compound was also higher in methoprene-treated workers. Previously, the linear alkane n-C27 was shown to be overexpressed in the cuticle of queens of four other Vespine specie, Vespula squamosa, Vespula pensylvanica, Vespula alascensis, and Dolichovespula maculata (Derstine et al., 2018) and to act as a queen pheromone in V. vulgaris (Van Oystaeyen et al., 2014). Empirical evidence is still lacking to confirm if this compound possibly works as a conserved queen signal in V. germanica or other Vespine wasps.
Policing behavior is an effective mechanism against worker reproduction of the German wasp V. germanica (Bonckaert et al., 2008). Our results showed that the eggs laid by methoprene-treated workers chemically resembled the ones laid by queens. Although not significant, methoprene-treated workers overexpressed the hydrocarbons 3-MeC25, n-C25, and 3-MeC27 on their eggs, compounds that are characteristic of queen-laid eggs. In the sister species, V. vulgaris, two methylated hydrocarbons, namely 3-MeC27 and 3-MeC29, are also deposited in higher proportions in queen-laid eggs (Oi et al., 2015). Policing behavioral assays confirmed that 3-MeC29, besides working as a queen pheromone (Van Oystaeyen et al., 2014), also works as an egg maternity signal in V. vulgaris (Oi et al., 2015). Behavioral assays are needed to certify whether one or a combination of 3-MeC25, n-C25, and 3-MeC27 play a role as queen egg-maternity compounds in V. germanica. The higher abundance of these compounds in the eggs of methoprene-treated workers may be an indication of high fertility. Although the Dufour’s gland has been hypothesized as the main source of egg-marking cues (Mitra, 2013), here we show that egg samples were more chemically similar to CHC samples. Some compounds still overlap between Dufour’s gland and eggs; however, the chemical composition of eggs may change after oviposition due to their direct interaction with the nest or other components (e.g., when the eggs are licked by females, when females touch them with their antennae, or even from the contact with the paper cell).
Besides regulating chemical production, here we also report that JH has likely conserved gonadotropic effects in V. germanica, similar to its sister species V. vulgaris (Oliveira et al., 2017; Oi et al., 2020, 2021b). JH is responsible for regulating reproductive traits in primitively and swarm-founding wasp species (Tibbetts and Sheehan, 2012; Kelstrup et al., 2014; Walton et al., 2020; Prato et al., 2021) and in highly eusocial species. Altogether, JH may have a gonadotropic-conserved function across the lineage of wasps. However, this hypothesis still must be considered with caution because comparative data are needed, which also requires including the inspection of JH circulating levels in females accordingly. Furthermore, given its pleiotropic roles, females are affected by JH along their nest ontogeny (Kelstrup et al., 2015; Oi et al., 2021b), and future experimental design need to take this into consideration.
In conclusion, our results strengthen the hormonal pleiotropy hypothesis, as we show that both traits, chemical production and reproduction, are under shared endocrine control in V. germanica. JH may be responsible for controlling the chemical production of other sources, such as the chemical composition of Dufour’s gland which is posteriorly deposited on the surface of eggs. The hydrocarbons that are found over the eggs may work as cues to allow policing in V. germanica. Future research is necessary to confirm whether the queen-characteristic compounds that we highlighted in our results indeed act as queen pheromones or egg-marking pheromones in V. germanica and other Vespinae wasps.
Data availability statement
The original contributions presented in this study are included in the article/Supplementary material, further inquiries can be directed to the corresponding author.
Author contributions
RCS and CAO contributed to the conception and design of the study, performed the material preparation, data collection, and analysis. RCS wrote the first draft of the manuscript. All authors commented on previous versions of the manuscript, read, and approved the final manuscript version.
Funding
This study was financially supported by Coordenação de Aperfeiçoamento de Pessoal de Nível Superior, Brazil (CAPES) – Finance Code 001 and grant 2018/22461-3 São Paulo Research Foundation (FAPESP) to RCS. Funding was provided from Bilateral grant FWO-FAPESP to CAO, FSN, and TW (process: 2018/10996-0; 2021/05598-8 FAPESP; and FWO: GOF8319N), Research Foundation Flanders to CAO (postdoctoral fellowship FWO-12V6318N and research grant FWO-1513219N), and Conselho Nacional de Desenvolvimento Científico e Tecnológico to FSN (307702/2018-9).
Acknowledgments
We thank an Vandoren for running the samples and Clint Varnemen to help with the experiments. We also thank the two reviewers for their helpful comments in the earlier version of the present work.
Conflict of interest
The authors declare that the research was conducted in the absence of any commercial or financial relationships that could be construed as a potential conflict of interest.
Publisher’s note
All claims expressed in this article are solely those of the authors and do not necessarily represent those of their affiliated organizations, or those of the publisher, the editors and the reviewers. Any product that may be evaluated in this article, or claim that may be made by its manufacturer, is not guaranteed or endorsed by the publisher.
Supplementary material
The Supplementary Material for this article can be found online at: https://www.frontiersin.org/articles/10.3389/fevo.2022.1024580/full#supplementary-material
References
Abdalla, F. C., and Cruz-Landim, C. D. (2001). Dufour’s glands in the hymenopterans (Apidae, Formicidae, Vespidae): A review. Revista Brasil. Biol. 61, 95–106. doi: 10.1590/S0034-71082001000100013
Agrahari, M., and Gadagkar, R. (2003). Juvenile hormone accelerates ovarian development and does not affect age polyethism in the primitively eusocial wasp, Ropalidia marginata. J. Insect Physiol. 49, 217–222. doi: 10.1016/S0022-1910(02)00268-8
Azevedo, D. O., de Paula, S. O., Zanuncio, J. C., Martinez, L. C., and Serrão, J. E. (2016). Juvenile hormone downregulates vitellogenin production in Ectatomma tuberculatum (Hymenoptera: Formicidae) sterile workers. J. Exp. Biol. 219, 103–108. doi: 10.1242/jeb.127712
Barth, R. H., Lester, L. J., Sroka, P., Kessler, T., and Hearn, R. (1975). Juvenile hormone promotes dominance behavior and ovarian development in social wasps (Polistes annularis). Experientia 31, 691–692. doi: 10.1007/BF01944632
Bates, D., Mächler, M., Bolker, B., and Walker, S. (2015). Fitting linear mixed-effects models using lme4. J. Stat. Softw. 67, 1–48. doi: 10.18637/jss.v067.i01
Bonckaert, W., Vuerinckx, K., Billen, J., Hammond, R. L., Keller, L., and Wenseleers, T. (2008). Worker policing in the German wasp Vespula germanica. Behav. Ecol. 19, 272–278. doi: 10.1093/beheco/arm128
da Silva, R. C., Brown, R. L., do Nascimento, F. S., Wenseelers, T., and Oi, C. A. (2021). Cuticular hydrocarbons as cues of caste and sex in the German wasp Vespula germanica. Insectes Soc. 68, 261–276. doi: 10.1007/s00040-021-00817-5
Derstine, N. T., Gries, R., Zhai, H., Jimenez, S. I., and Gries, G. (2018). Cuticular hydrocarbons determine sex, caste, and nest membership in each of four species of yellowjackets (Hymenoptera: Vespidae). Insectes Soc. 65, 581–591. doi: 10.1007/s00040-018-0649-0
Ferreira, H. M., da Silva, R. C., do Nascimento, F. S., Wenseleers, T., and Oi, C. A. (2022). Reproduction and fertility signalling under joint juvenile hormone control in primitively eusocial Mischocyttarus wasps. Chemoecology 32, 105–116. doi: 10.1007/s00049-022-00370-y
Giray, T., Giovanetti, M., and West-Eberhard, M. J. (2005). Juvenile hormone, reproduction, and worker behavior in the neotropical social wasp Polistes canadensis. Proc. Natl. Acad. Sci. U.S.A. 102, 3330–3335. doi: 10.1073/pnas.0409560102
Goodman, W. G., and Cusson, M. L. I. G. (2012). “The juvenile hormones,” in Insect endocrinology, ed. L. I. Gilbert (New York, NY: Academic Press), 310–365.
Hartfelder, K. (2000). Insect juvenile hormone: From “status quo” to high society. Braz. J. Med. Biol. Res. 33, 157–177. doi: 10.1590/s0100-879x2000000200003
Holman, L. (2012). Costs and constraints conspire to produce honest signaling: Insights from an ant queen pheromone. Evolution 66, 2094–2105. doi: 10.1111/j.1558-5646.2012.01603.x
Huang, Z. Y. (2020). “Juvenile Hormone,” in Encyclopedia of social insects, ed. C. K. Starr (Berlin: Springer), 1–3. doi: 10.1007/978-3-319-90306-4_68-1
Jindra, M., Belles, X., and Shinoda, T. (2015). Molecular basis of juvenile hormone signaling. Curr. Opin. Insect Sci. 11, 39–46. doi: 10.1016/j.cois.2015.08.004
Kamimura, M., and Kiuchi, M. (1998). Effects of a juvenile hormone analog, fenoxycarb, on 5th stadium larvae of the silkworm, Bombyx mori (Lepidoptera: Bombycidae). Appl. Entomol. Zool. 33, 333–338. doi: 10.1303/aez.33.333
Kelstrup, H. C., Hartfelder, K., and Wossler, T. C. (2015). Polistes smithii vs. Polistes dominula: The contrasting endocrinology and epicuticular signaling of sympatric paper wasps in the field. Behav. Ecol. Sociobiol. 69, 2043–2058. doi: 10.1007/s00265-015-2015-9
Kelstrup, H. C., Hartfelder, K., Nascimento, F. S., and Riddiford, L. M. (2014). The role of juvenile hormone in dominance behavior, reproduction and cuticular pheromone signaling in the caste-flexible epiponine wasp, Synoeca surinama. Front. Zool. 11:78. doi: 10.1186/s12983-014-0078-5
Kolde, R. (2015). pheatmap: Pretty heatmaps. R package. Available online at: https://CRAN.R-project.org/package=pheatmap
Korner-Nievergelt, F., Roth, T., von Felten, S., Guelat, J., Almasi, B., and Korner-Nievergelt, P. (2015). Bayesian data analysis in ecology using linear models with R, BUGS and Stan. Amsterdam: Elsevier.
Kou, R., Chou, S. Y., Huang, Z. Y., and Yang, R. L. (2008). Juvenile hormone levels are increased in winners of cockroach fights. Horm. Behav. 54, 521–527. doi: 10.1016/j.yhbeh.2008.05.011
Mitra, A. (2013). Function of the Dufour’s’s gland in solitary and social Hymenoptera. J. Hymenopt. Res. 35:33. doi: 10.3897/jhr.35.4783
Neves, E. F., Montagna, T. S., Junior, L. C. S., Michelutti, K. B., Cardoso, C. A. L., Andrade, L. H. C., et al. (2020). Effect of larval topical application of juvenile hormone on cuticular chemical composition of Mischocyttarus consimilis (Vespidae: Polistinae) females. Sociobiology 67, 433–443. doi: 10.13102/sociobiology.v67i3.4366
Noriega, F. G. (2014). Juvenile hormone biosynthesis in insects: What is new, what do we know, and what questions remain? Int. Sch. Res. Notices 2014:967361. doi: 10.1155/2014/967361
Norman, V. C., Pamminger, T., Nascimento, F., and Hughes, W. O. (2019). The role of juvenile hormone in regulating reproductive physiology and dominance in Dinoponera quadriceps ants. PeerJ 7:e6512. doi: 10.7717/peerj.6512/supp-1
Oi, C. A., Brown, R. L., da Silva, R. C., and Wenseleers, T. (2020). Reproduction and signals regulating worker policing under identical hormonal control in social wasps. Sci. Rep. 10:18971. doi: 10.1038/s41598-020-76084-4
Oi, C. A., da Silva, R. C., Stevens, I., Ferreira, H. M., Nascimento, F. S., and Wenseleers, T. (2021b). Hormonal modulation of reproduction and fertility signaling in polistine wasps. Curr. Zool. 67, 519–530. doi: 10.1093/cz/zoab026
Oi, C. A., Ferreira, H. M., da Silva, R. C., Bienstman, A., do Nascimento, F. S., and Wenseleers, T. (2021a). Effects of juvenile hormone in fertility and fertility-signaling in workers of the common wasp Vespula vulgaris. PLoS One 16:e0250720. doi: 10.1371/journal.pone.0250720
Oi, C. A., Van Oystaeyen, A., Oliveira, R. C., Millar, J. G., Verstrepen, K. J., van Zweden, J. S., et al. (2015). Dual effect of wasp queen pheromone in regulating insect sociality. Curr. Biol. 25, 1638–1640. doi: 10.1016/j.cub.2015.04.040
Oksanen, J., Blanchet, F. G., Kindt, R., Legendre, P., Minchin, P. R., O’Hara, R. B., et al. (2015). Vegan community ecology package: Ordination methods, diversity analysis and other functions for community and vegetation ecologists. R package ver, 2-3.
Oliveira, R. C., Vollet-Neto, A., Oi, C. A., van Zweden, J. S., Nascimento, F., Brent, C. S., et al. (2017). Hormonal pleiotropy helps maintain queen signal honesty in a highly eusocial wasp. Sci. Rep. 7:1654. doi: 10.1038/s41598-017-01794-1
Pamminger, T., Buttstedt, A., Norman, V., Schierhorn, A., Botías, C., Jones, J. C., et al. (2016a). The effects of juvenile hormone on Lasius niger reproduction. J. Insect Physiol. 95, 1–7. doi: 10.1016/j.jinsphys.2016.09.004
Pamminger, T., Treanor, D., and Hughes, W. O. (2016b). Pleiotropic effects of juvenile hormone in ant queens and the escape from the reproduction–immunocompetence trade-off. Proc. R. Soc. B 283:20152409. doi: 10.1098/rspb.2015.2409
Pandey, A., Motro, U., and Bloch, G. (2020). Juvenile hormone interacts with multiple factors to modulate aggression and dominance in groups of orphan bumble bee (Bombus terrestris) workers. Horm. Behav. 117:104602. doi: 10.1016/j.yhbeh.2019.104602
Penick, C. A., Prager, S. S., and Liebig, J. (2012). Juvenile hormone induces queen development in late-stage larvae of the ant Harpegnathos saltator. J. Insect Physiol. 58, 1643–1649. doi: 10.1016/j.jinsphys.2012.10.004
Prato, A., da Silva, R. C., Assis, D. S., Mateus, S., Hartfelder, K., and Nascimento, F. S. (2021). Juvenile hormone affects age polyethism, ovarian status, and cuticular hydrocarbon profile in workers of a Polybia occidentalis wasp. J. Exp. Biol. 224, jeb240200. doi: 10.1242/jeb.240200
R Core Team (2021). R: A language and environment for statistical computing. Vienna: R Foundation for Statistical Computing.
Raushenbach, I. Y., Gruntenko, N. E., Bownes, M., Adonieva, N. V., Terashima, J., Karpova, E. K., et al. (2004). The role of juvenile hormone in the control of reproductive function in Drosophila virilis under nutritional stress. J. Insect Physiol. 50, 323–330. doi: 10.1016/j.jinsphys.2004.02.001
Robinson, G. E. (1987). Regulation of honey bee age polyethism by juvenile hormone. Behav. Ecol. Sociobiol. 20, 329–338. doi: 10.1007/BF00300679
Schrempf, A., and Heinze, J. (2006). Proximate mechanisms of male morph determination in the ant Cardiocondyla obscurior. Evol. Dev. 8, 266–272. doi: 10.1111/j.1525-142X.2006.00097.x
Shorter, J. R., and Tibbetts, E. A. (2009). The effect of juvenile hormone on temporal polyethism in the paper wasp Polistes dominulus. Insectes Soc. 56, 7–13. doi: 10.1007/s00040-008-1026-1
Tibbetts, E. A., and Izzo, A. S. (2009). Endocrine mediated phenotypic plasticity: Condition-dependent effects of juvenile hormone on dominance and fertility of wasp queens. Horm. Behav. 56, 527–531. doi: 10.1016/j.yhbeh.2009.09.003
Tibbetts, E. A., and Sheehan, M. J. (2012). The effect of juvenile hormone on Polistes wasp fertility varies with cooperative behavior. Horm. Behav. 61, 559–564. doi: 10.1016/j.yhbeh.2012.02.002
Tibbetts, E. A., Vernier, C., and Jinn, J. (2013). Juvenile hormone influences precontest assessment behaviour in Polistes dominulus paper wasps. Anim. Behav. 85, 1177–1181. doi: 10.1016/j.anbehav.2013.03.003
Van Oystaeyen, A., Oliveira, R. C., Holman, L., van Zweden, J. S., Romero, C., Oi, C. A., et al. (2014). Conserved class of queen pheromones stops social insect workers from reproducing. Science 343, 287–290. doi: 10.1126/science.1244899
Walton, A., Tumulty, J. P., Toth, A. L., and Sheehan, M. J. (2020). Hormonal modulation of reproduction in Polistes fuscatus social wasps: Dual functions in both ovary development and sexual receptivity. J. Insect Physiol. 120, 103972. doi: 10.1016/j.jinsphys.2019.103972
Yamamoto, R., Bai, H., Dolezal, A. G., Amdam, G., and Tatar, M. (2013). Juvenile hormone regulation of Drosophila aging. BMC Biol. 11:85. doi: 10.1186/1741-7007-11-85
Keywords: fertility cues, hydrocarbons, Dufour’s gland, Vespula germanica, Vespinae
Citation: da Silva RC, do Nascimento FS, Wenseleers T and Oi CA (2022) Juvenile hormone modulates hydrocarbon expression and reproduction in the german wasp Vespula germanica. Front. Ecol. Evol. 10:1024580. doi: 10.3389/fevo.2022.1024580
Received: 21 August 2022; Accepted: 18 October 2022;
Published: 09 November 2022.
Edited by:
Michael John Greene, University of Colorado Denver, United StatesReviewed by:
Joël Meunier, UMR 7261 Institut de Recherche sur la Biologie de l’Insecte (IRBI), FranceUlrich Rainer Ernst, University of Hohenheim, Germany
Copyright © 2022 da Silva, do Nascimento, Wenseleers and Oi. This is an open-access article distributed under the terms of the Creative Commons Attribution License (CC BY). The use, distribution or reproduction in other forums is permitted, provided the original author(s) and the copyright owner(s) are credited and that the original publication in this journal is cited, in accordance with accepted academic practice. No use, distribution or reproduction is permitted which does not comply with these terms.
*Correspondence: Rafael Carvalho da Silva, cmNzaWx2YTI4MTJAdXNwLmJy