- Ministry of Education Key Laboratory for Ecology of Tropical Islands, Key Laboratory of Tropical Animal and Plant Ecology of Hainan Province, College of Life Sciences, Hainan Normal University, Haikou, China
The aim of this study was to identify underwater vocalizations in red-eared turtles (Trachemys scripta elegans) and assess differences between sexes and ages. We recorded the underwater vocalizations of the red-eared sliders and identified 12 call types through manual visual and aural inspection of the recordings. Similarity analysis verified that manual classification was relatively reliable. The call types of the turtle were described and displayed as spectrograms and waveforms. The turtles produced fewer high-frequency call types than low-frequency types in all recordings. Statistical analysis revealed significant differences in the frequencies and duration of the calls of red-eared turtles between different sexes and ages. Males vocalized pulse calls very frequently, whereas a high proportion of high-frequency call types was emitted by the female adult group. The male subadult group emitted higher frequencies of Type A, B, and C calls, which is in accordance with the phenomenon that vocal frequency is often inversely proportional to the turtle size. Some call types produced by red-eared turtles were above the frequency range of their known hearing range. This may have been a by-product of the sound production mechanism or it may have adaptive value in mitigating interference to communication from low-frequency noise common in natural waters in communication The behavioral implications of these vocalizations and whether turtles can hear such high sounds warrant further study.
Introduction
Sound communication is one of the most common forms of communication among animals and is important for information transmission between individuals (Gerhardt and Huber, 2002), particularly for species living in dark environments or underwater where light is lacking (Shang, 2014). In the complex underwater environment, natural calls remain discernible at distances where frequency-dependent propagation losses distort the shape of the spectrum (Boatright-Horowitz et al., 1999). More than 300 species of turtles have been identified worldwide; however, vocalizations have been recorded for only a limited number of species. Most studies of turtle vocalizations have focused on air recordings (Cook and Forrest, 2005; Galeotti et al., 2005; McKenna et al., 2019; Monteiro et al., 2019), with only a few underwater recordings for some species (Giles et al., 2009; Ferrara et al., 2014a,2017, 2019). Thus, vocalization studies of various freshwater turtles are needed to improve the understanding of their behavior and ecology.
The red-eared slider (Trachemys scripta elegans) is a semi-aquatic freshwater turtle, native to North America, from the Mississippi River to around the Gulf of Mexico; it exhibits high ecological adaptability (Ma and Shi, 2017). Red-eared sliders are not social animals, but the overlap index of individuals’ home ranges is very high. In terms of behavior, females have larger home range area and line home range than males (Ma et al., 2013). This typical alien invasive species in China poses threats to native freshwater turtles (Wei et al., 2012). Studies of the vocalizations of the red-eared slider are important for conserving China’s native freshwater turtles. In China, 14 native turtle species live in the same environment as red-eared turtles (Shi et al., 2009). In terms of fighting for food, feeding efficiency, fecundity, hunger tolerance, and disease resistance, these turtles are stronger than the native freshwater turtles of China (Shi et al., 2009; Wei et al., 2012; Zhao et al., 2013; Qiu et al., 2015). For vocal animals, sound expulsion and induction are common methods in conservation management (Wang, 2010; Wang et al., 2010; Yuan, 2020). This study on the vocalization of red-eared sliders can provide basic data on the sound expulsion and induction of red-eared turtles in the wild, and provide ideas for the conservation of freshwater turtles in China.
Sex differences in vocalization are common in many vocal animals (Sathyan et al., 2017; Ammie, 2019; Buck et al., 2020) and may be related to differences in the size of vocal organs (Pellitteri, 2003; Galeotti et al., 2005), intraspecific competition, and mate selection pressure (Garcia et al., 2013; Zhu et al., 2017). This sex difference in turtle vocalization provides a foundation for understanding sexual selection in voice communication.
Previous auditory research in the red-eared slider in air indicated that 300–500 Hz is the most sensitive part of hearing range (Christensen-Dalsgaard et al., 2012) and that females show greater sensitivity, because auditory brainstem response thresholds are significantly lower in females than in males for frequencies in the 200–1,100 Hz range (Wang et al., 2019). However, some turtles vocalize at frequencies beyond their hearing range (Cook and Forrest, 2005; Piniak et al., 2012a,b; Ferrara et al., 2014b). Whether the hearing range of the red-eared slider matches its vocal frequency requires further analysis.
Herein, we recorded the underwater vocalizations of red-eared sliders, classified the call types, analyzed the vocalization differences between sex and age groups, and discussed the association between hearing range and vocalization frequency in this species. This study is the first one to describe red-eared slider vocalization and improves our understanding of this invasive species in China.
Materials and methods
Data collection
We used a Song Meter SM4 underwater sound recorder (Wildlife Acoustics, Inc., Maynard, MA, USA) to collect turtles’ calls (sampling rate: 44,100 Hz; sensitivity: −165 dB; Re: 1 V/μPa; omnidirectional hydrophone bandwidth: 2–30,000 Hz ± 3 dB; gain: 16 dB). The hydrophone was calibrated by the company before purchase. Data were stored in WAV format. The recording was conducted in a soundproof room at the College of Life Sciences, Hainan Normal University, to prevent environmental noise from being recorded. The soundproof room is a confined space 1.7 m by 2 m by 2 m. Its walls and ceiling are made of composed of steel and foam sandwich composite board (12 cm thick); the inner side of the composite board is covered with sound absorbing cotton (2.5 cm thick). Prior to the experiment, the water in the tortoise-free tank was recorded for 3 days and found to have no external acoustic signal. The average sound pressure level of the underwater background noise (without turtles in the water) in the tank was 92.35 dB. The energy was mainly concentrated at low frequencies below 50 Hz, with a sound pressure level of up to 70 dB, whereas noise in the frequency band above 200 Hz was approximately 40 dB.
We obtained 16 healthy captive red-eared turtles from a turtle farm, where they were raised in groups of 20–30 in shallow concrete pools in the open air, with a space next to the pool for insolation. We divided them into groups of female adults (n = 4), male adults (n = 4), female subadults (n = 4), and male subadults (n = 4). Males and females were distinguished based on differences in their front claws, cloaca, plastron, and tail notch (Mader, 2006). The eight subadults were 9 months old, and the eight adults were 5 years old. Recordings were performed for each of the five following groups: four female adults, four male adults, four female subadults, four male subadults, and a mix of eight adult turtles. In each recording, the turtles in a group were free to roam in an inflatable plastic circular cistern 1.2 m in diameter, with a 30 cm water depth. The hydrophone was suspended 15 cm below the water surface at the center of the cistern for recording (Figure 1). The cistern was also video recorded, and a researcher monitored the turtles’ behaviors on the video feed in real time. The recording was performed in late December 2020. Each group was continuously recorded from 8:00 to 20:00 of 1 day. In total, we collected 60 h of data in 5 days. To match the video of the turtle’s behavior with the sound recordings, we synchronized the clock in the recorder and the clock in the camera before the recording began.
Data analysis
Acoustic data were analyzed using the Raven Pro 1.5 software (The Cornell Lab of Ornithology, Ithaca, NY, USA). The spectrograms were displayed using a Fast Fourier-Transforms size of 512 points (Hamming window). Two researchers experienced in aquatic vocalizations manually identified sound signals through visual and aural inspection of the recordings. Sounds with similar characteristics (spectrum shape; auditory) as published turtle sounds (Giles et al., 2009; Ferrara et al., 2017; Charrier et al., 2022) and that clearly sounded like animal calls were selected. Only sound signals for which the spectrograms showed high signal-to-noise ratio were selected for further acoustical analyses. The variables extracted from the spectrograms of selected sound signals were low frequency (Hz) (the lower limit of the frequency range of a signal), high frequency (Hz) (the upper limit of the frequency range of a signal), peak frequency (Hz) (the frequency represented by a maximum energy in the wave spectrum), and signal duration (ms). The characteristics of the spectrograms of signals such as the number of harmonic and non-harmonic frequency bands were also noted. The selected sound signals were classified according to audible differences detected by a listening researcher, and the morphological characteristics of the spectrograms (Giles et al., 2009). Two signals were classified as the same type when they showed the same auditory characteristics and highly similar spectral morphologies. The recordings were monitored using Sennheiser IE300 earphones (Wedemark, Germany).
To test the reliability of the manual classification, we examined the similarity between signals of different call types and the similarity between signals of the same call type (Manolakis et al., 2005; Zheng, 2011). Pearson’s linear correlation coefficient (Gibbons, 1985) was used to detect similarities. The calculation was performed in MATLAB R2021a (MathWorks, Inc., Natick, MA, USA).
Because turtles made mechanical sounds by crawling and moving underwater in the pool, we identified these sounds before recording the turtles’ vocalizations. Turtle behaviors were observed via real time monitoring while underwater sounds were recorded with a hydrophone. The occurrence time of each behavior was recorded, and the frequency spectrum and auditory characteristics of the sounds produced by different behaviors were analyzed in Raven Pro 1.5 software. We then identified the corresponding sounds produced by the common behaviors of turtles in water, and these were not included as turtle vocal sounds. Time-frequency diagrams of these sounds are shown in Supplementary Figure 1.
Statistical analysis
The Kolmogorov–Smirnov test (Dallal and Wilkinson, 1986) was used to estimate whether the sound parameters for each group followed normal distribution (P > 0.05). If the distribution was normal, the differences in parameters among sex–age groups were tested using one-way analysis of variance (least significant difference test) for pairwise comparisons. Otherwise, Kruskal–Wallis analysis (non-parametric tests algorithms) (Siegel, 1956) was used to evaluate the differences among the five groups, and paired comparison was conducted between all pairs of groups. Differences were considered as significant when P < 0.05. All statistical analyses were conducted using IBM SPSS Statistics 26 software (IBM, Inc., Chicago, IL, USA).
Results
We detected 2,241 distinguishable calls of T. scripta elegans in 16 individuals and assigned these calls to 12 types based on their aural character, frequency, and the morphology of their spectrum (Figure 2). More than two-thirds of the calls were made by males (Table 1). The female groups and mixed-sex group produced far fewer calls than the male groups. We observed no specific behaviors of the red-eared turtles throughout the recording; therefore, whether these vocalizations had behavioral implications remains unclear.
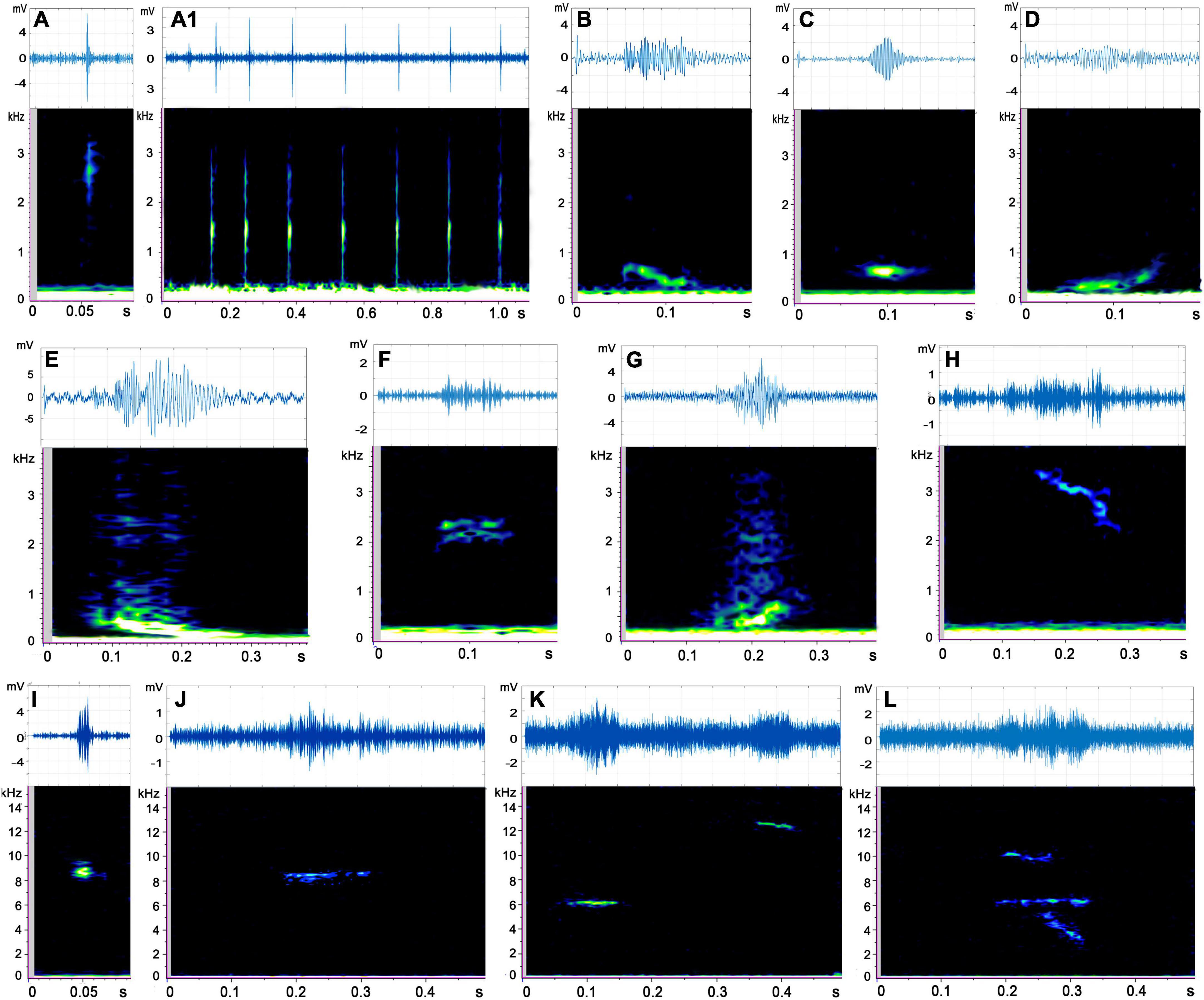
Figure 2. Spectrogram and waveform views of call types produced by Trachemys scripta elegans. (A–L) Corresponds to the 12 call types of red-eared sliders, and A1 represents successive occurrences of A call type. All spectrograms were obtained with Raven Pro 1.5 using Hamming windows with 512 pt fast Fourier transform. The waveforms were drawn in MATLAB R2021a.
Description of turtle calls
The spectrograms and waveforms of each call type are shown in Figure 2. The acoustic parameters of each type are shown in Table 1. Because eight adult turtles were recorded twice, the calculation of the acoustic parameters in Table 1 did not include data from the mixed sex group to avoid the risk of pseudoreplication. Audio samples of each type are available in the Supplementary Audios 1–12.
Type A (n = 1,662) was the most common call type of red-eared sliders and was particularly more frequent in the male groups than in the female groups. This type was characterized by a single pulse and sounded similar to soft tapping on a wooden stick by a fingernail. The mean peak frequency was 2,421 ± 1,821 Hz (mean ± sd). This call type often occurred continuously, sometimes in a series of three to seven calls (intervals ranged from 94 to 167 ms) (Figure 2A1 and Supplementary Audio 1).
Type B (n = 147) was characterized by the spectrum shape of a single down sweep with a peak frequency mean value of 426 ± 482 Hz. It sounded similar to a falling tone (Figure 2B and Supplementary Audio 2).
Type C (n = 98) was a short wave with a flat tone below 1,000 Hz and sounded similar to the single chirp of a mouse. It resembled an elliptical dot on the spectrum (Figure 2C and Supplementary Audio 3).
Type D (n = 75) showed almost mirror symmetry with the Type B spectrum; it was ascending and characterized by the spectrum shape of a single rising sweep tone in the low-frequency band (Figure 2D and Supplementary Audio 4).
Type E (n = 40) resembled a descending tone snorted from the nose and was a broadband signal with harmonics (Figure 2E and Supplementary Audio 5). The mean low and high frequencies were 274 ± 412 and 2,292 ± 1,220 Hz, respectively. However, its peak frequency was concentrated at low frequencies with a mean value of 534 ± 693 Hz.
Type F (n = 31) consisted of two harmonics in the middle frequency band (Figure 2F) and sounded similar to a single cricket chirp (Supplementary Audio 6). This type showed a narrow band signal in the middle frequency.
Type G (n = 23) sounded similar to a rising tone from the nose (Supplementary Audio 7). It was also a broadband signal with harmonics (Figure 2G), and the peak frequency was concentrated at a low-frequency band.
Type H (n = 17) was characterized by the spectrum shape of a single down sweep in the middle frequency band (Figure 2H and Supplementary Audio 8). It resembled the single cry of a chick. The duration of this signal was very long, averaging 161.10 ± 159.09 ms. This call type was not observed in male adult turtles.
Type I (n = 79) was a high-frequency call that sounded similar to a single sparrow call. The mean value of the peak frequency was 7,758 ± 2,459 Hz (Figure 2I and Supplementary Audio 9).
Type J (n = 54) was a high-frequency call in the shape of a flat sweep on the spectrum and sounded similar to a slow and long birdsong (Figure 2J and Supplementary Audio 10). This was a narrow band signal concentrated at around 7,000 Hz. Additionally, 74.07% of these sounds were produced by the female adult group.
Type K (n = 12) consisted of two parts on spectrogram: the first part was low and the second part was high. It sounded similar to two birdsongs in a row (Figure 2K and Supplementary Audio 11). The duration of this signal was very long, averaging 279.84 ± 100.80 ms. Furthermore, 83.33% of these calls were produced by the female adult group, and the male adult group did not make such sounds.
Type L (n = 3) was the most complex call recorded and was composed of high-, medium-, and low-frequency parts overlapping in time (Figure 2L). This call sounded similar to a single bright bird call. Only the female adult group made this sound (Supplementary Audio 12).
Similarity calculation
The average correlation coefficient among the 12 call types was 0.11 and that among the internal signals of each call type was 0.92. The specific correlation coefficients are shown in Table 2. According to these results, the similarity between call types was small and the difference was large, whereas the signal similarity within each type was large and the difference was small. Thus, the manual classification was relatively reliable.
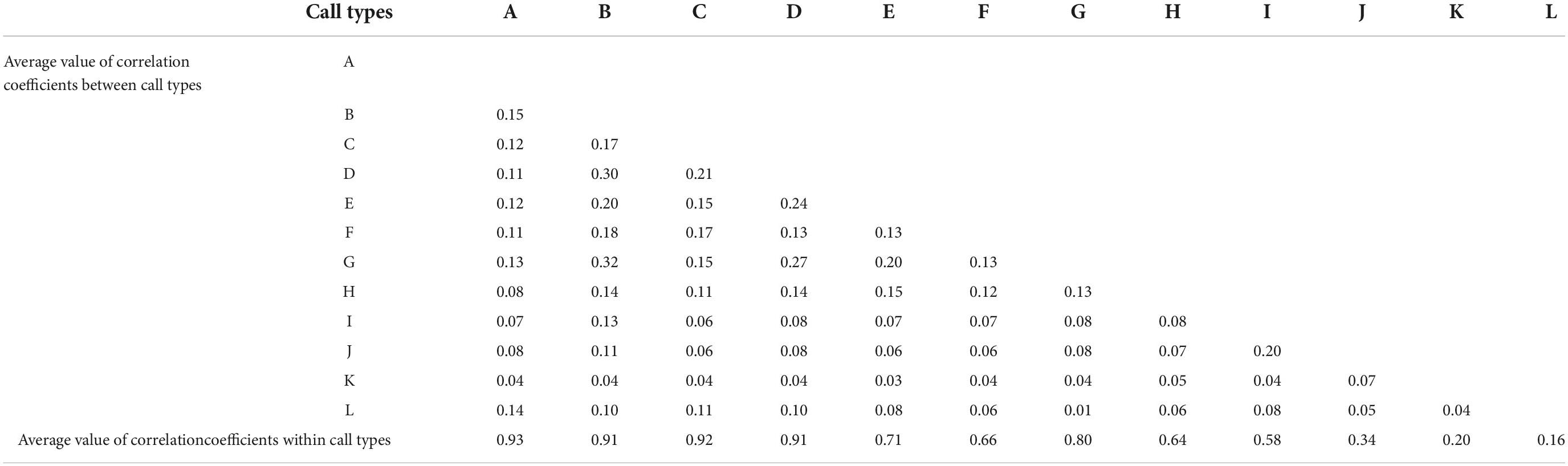
Table 2. Average value of correlation coefficients for signals within each call type and between call types.
Differences in calls among different sex and age groups
Kruskal–Wallis analysis was used to evaluate differences among different sex and age groups in acoustic parameters, as these parameters did not follow a normal distribution based on the Kolmogorov–Smirnov test (P > 0.05). Five call types showed significant differences among groups in frequency parameters, and four call types showed significant differences among groups in duration. For each call type, the differences between groups for low, high, and peak frequencies were very similar. The acoustic parameters of the three types (Type A, B, and C) with the highest rate of emittance in the recordings showed significant differences among different groups.
Peak frequency
Of the 12 call types, only four showed significant differences in their peak frequencies among the sex–age groups. The other eight call types showed no significant differences in their peak frequency among groups (Table 3, Figure 3, and Supplementary Table 1).
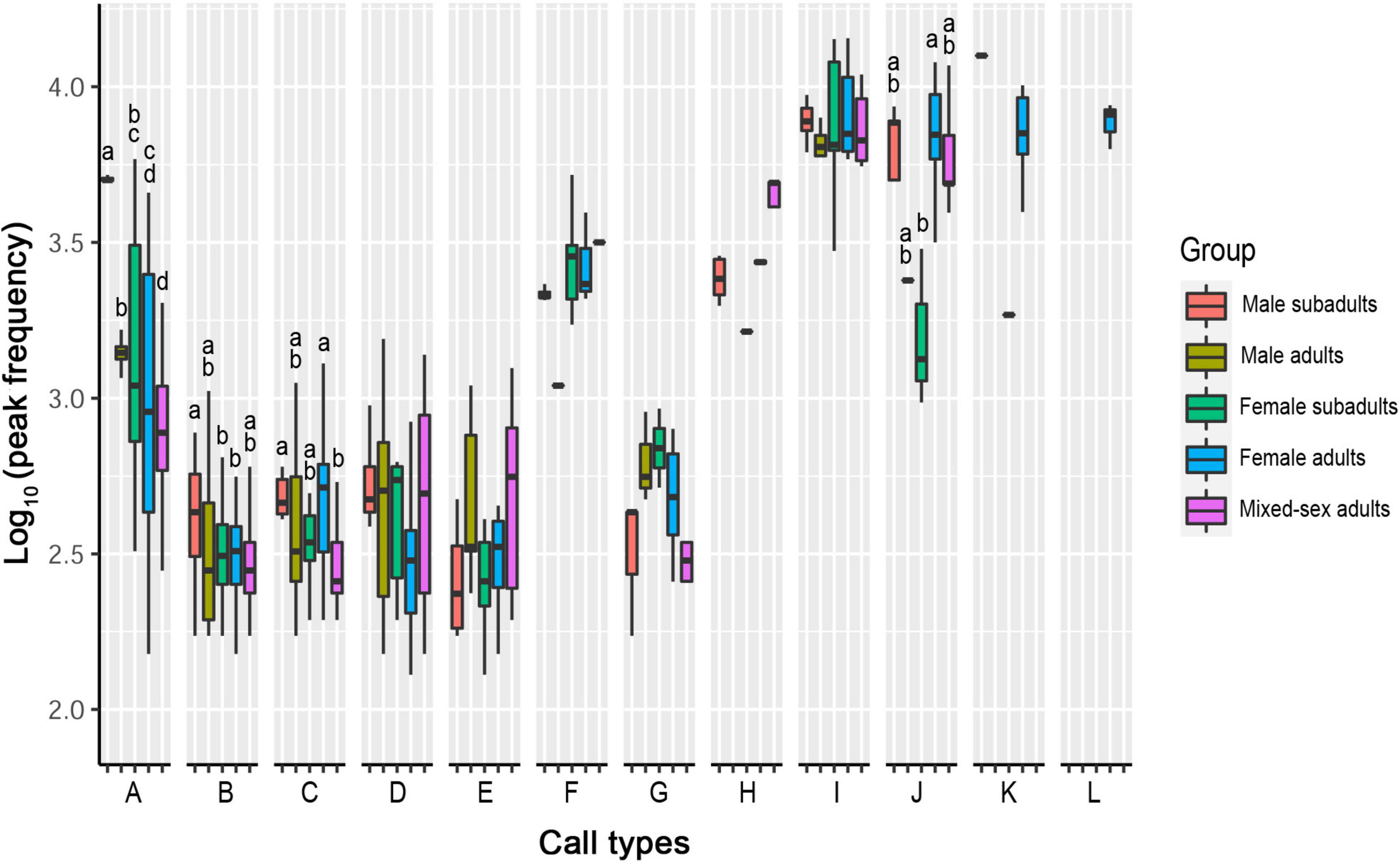
Figure 3. Differences in peak frequencies among five sex–age groups for all call types. The y-axis is the log-transformed value of peak frequency. The x-axis represents the call types. Boxplots with different lowercase letters refer to Kruskal–Wallis comparison tests yielding significant results (P < 0.05), and the relationship among the letters is a > b > c > d. Boxplots with the same lowercase letters did not significantly differ (P > 0.05). Call types without letters on the boxplots showed no significant differences among sex–age groups.
For Type A, the peak frequency in the male subadult group was higher than that in all other groups (P < 0.05). The peak frequency in the male adult group was significantly higher than that in the female adult and mixed-sex groups (P < 0.05). The peak frequency of the female subadult group was significantly higher than that in the mixed-sex group (P < 0.05). There was no significant difference between the female adult and female subadult, between the female subadult and male adult groups, or between the female adult and mixed-sex adult groups (P > 0.05).
For Type B, the peak frequency in the male subadult group was significantly higher than that in the female subadult and female adult groups (P < 0.05). There was no significant difference in the peak frequency among the male adult, female subadult, female adult, and mixed-sex adult groups (P > 0.05).
For Type C, the peak frequency in the mixed-sex adult group was significantly lower than that in the male subadult and female adult groups (P < 0.05). There was no significant difference among male subadult, male adult, and female subadult groups (P > 0.05).
For Type J, the peak frequency in the female adult group was higher than that in the female subadult group (P < 0.05). There was no significant difference in the peak frequency among male subadult, male adult, female subadult, and mixed-sex adult groups (P > 0.05).
Low frequency
Five call types showed significant differences in their low frequencies among the sex–age groups. The other seven call types showed no significant differences in low frequency among groups (Table 3, Figure 4, and Supplementary Table 2).
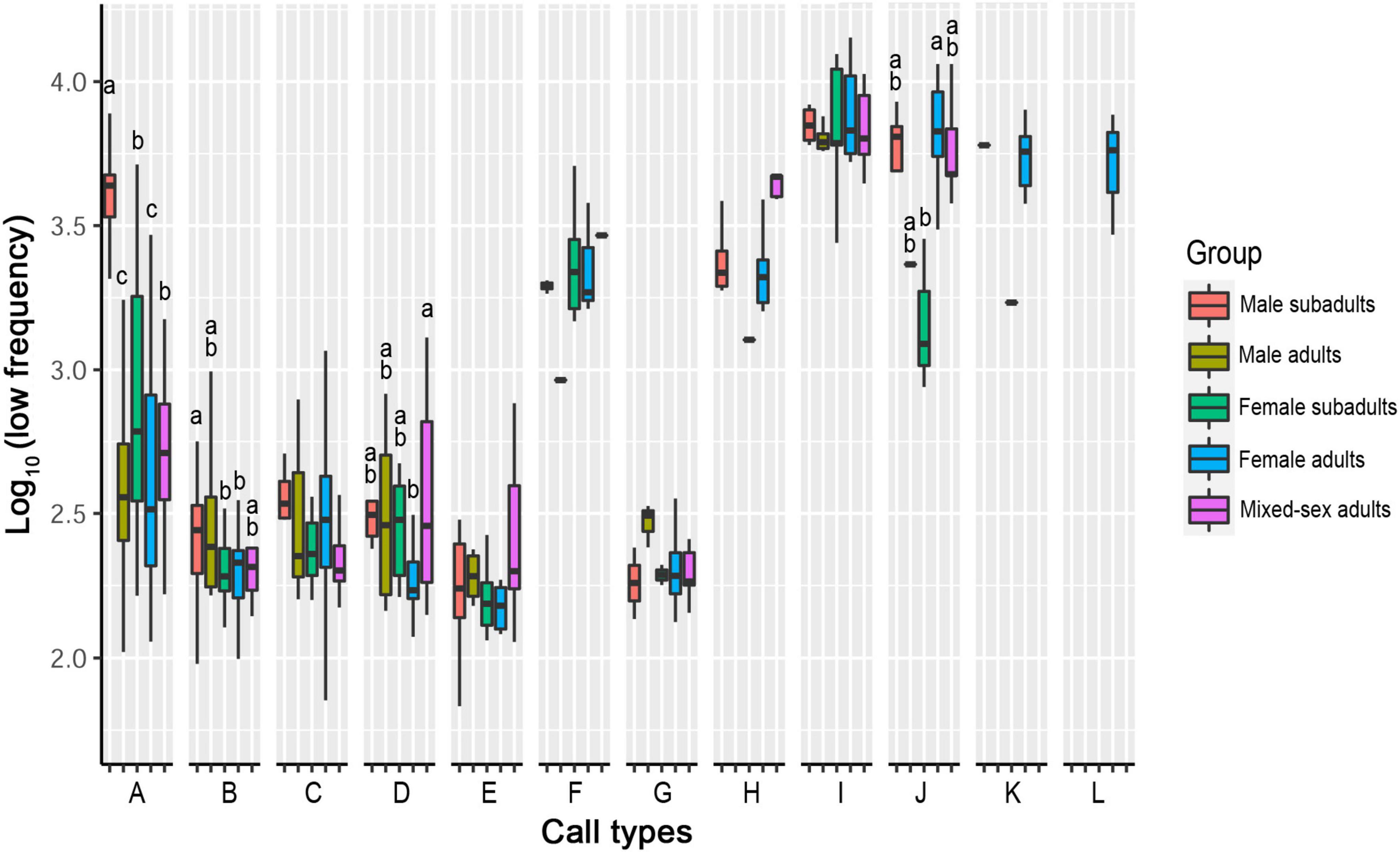
Figure 4. Differences in low frequencies among five sex–age groups for all call types. The y-axis is the log-transformed value of low frequency. The x-axis represents the call types. Boxplots with different lowercase letters refer to Kruskal–Wallis comparison tests yielding significant results (P < 0.05), and the relationship among the letters is a > b > c > d. Boxplots with the same lowercase letters did not significantly differ (P > 0.05). Call types without letters on the boxplots showed no significant differences among sex–age groups.
For Type A, the low frequency in the male subadult group was higher than that in all other groups (P < 0.05). The low frequencies of the female subadult and mixed-sex adult groups were significantly higher than those of the female adult and male adult groups (P < 0.05). There was no significant difference between the female adult and male adult groups or between the female subadult and mixed-sex adult groups (P > 0.05).
For Type B, the low frequency in the male subadult group was significantly higher than that in the female subadult and female adult groups (P < 0.05). There was no significant difference in low frequency among the male adult, female subadult, female adult, and mixed-sex adult groups (P > 0.05).
For Type D, the low frequency in the mixed-sex adult group was significantly higher than that in the female adult group (P < 0.05). There was no significant difference among the male adult, female adult, female subadult, and male subadult groups (P > 0.05), or among the male adult, male subadult, female subadult, and mix-sex adult groups (P > 0.05).
For Type J, the low frequency in the female adult group was higher than that in the female subadult group (P < 0.05). There was no significant difference in the low frequency among the male subadult, male adult, female subadult, and mixed-sex adult groups (P > 0.05).
High frequency
Of the 12 call types, only four showed significant differences in their high frequencies among sex–age groups. The other eight call types showed no significant differences in the peak high frequency among groups (Table 3, Figure 5, and Supplementary Table 3).
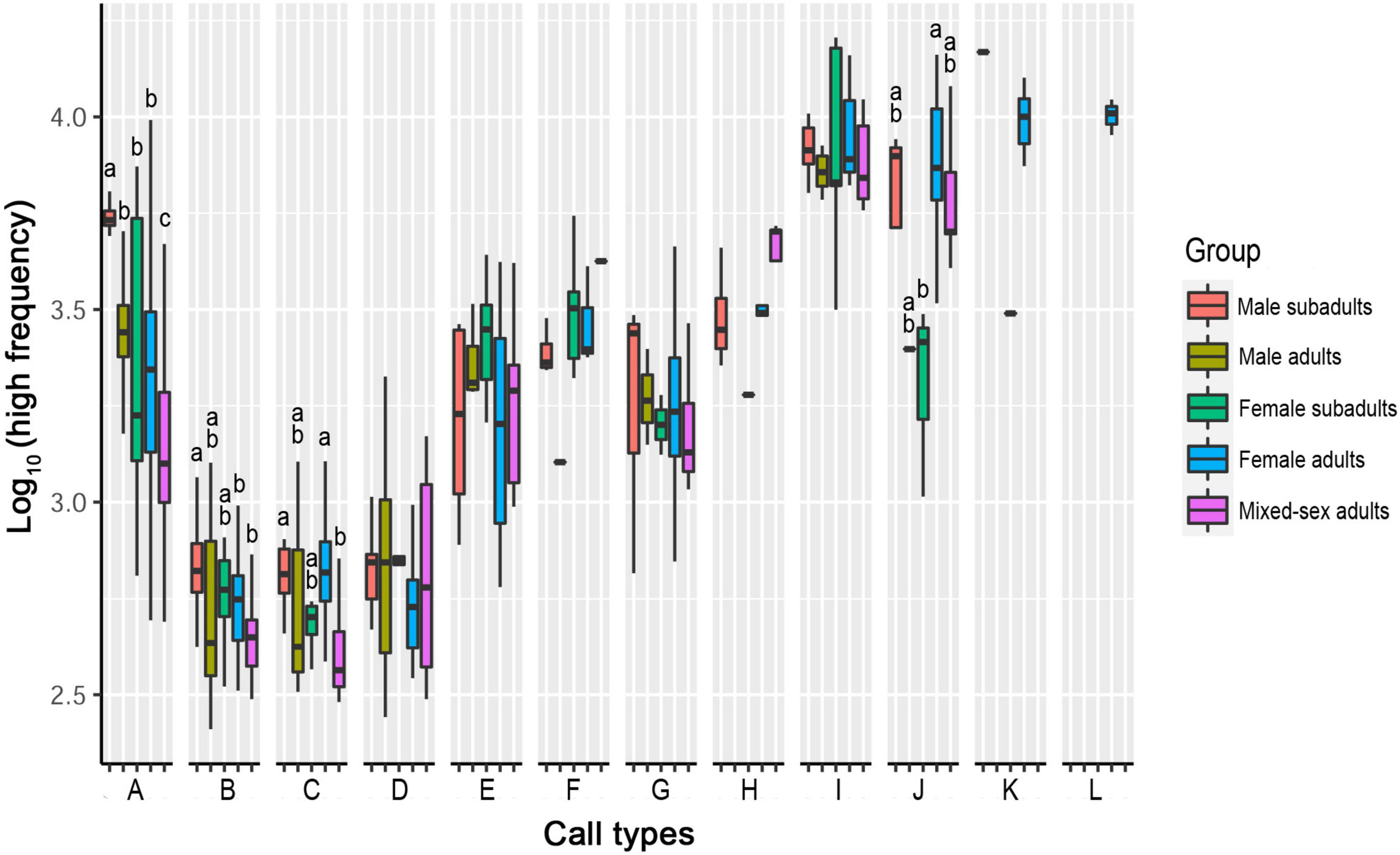
Figure 5. Differences in high frequencies among five sex–age groups for all call types. The y-axis is the log-transformed value of high frequency. The x-axis represents the call types. Boxplots with different lowercase letters refer to Kruskal–Wallis comparison tests yielding significant results (P < 0.05), and the relationship among the letters is a > b > c > d. Boxplots with the same lowercase letters did not significantly differ (P > 0.05). Call types without letters on the boxplots showed no significant differences among sex–age groups.
For Type A, the high frequency in the male subadult group was higher than that in all other groups (P < 0.05). The high frequencies in the mixed-sex adult groups were significantly lower than that in all other groups (P < 0.05). There was no significant difference among the female adult, male adult, female, subadult groups, or between the male subadult and mixed-sex adult groups (P > 0.05).
For Type B, the high frequency in the male subadult group was significantly higher than that in the female adult and mixed-sex adult groups (P < 0.05). There was no significant difference in the high frequency among the male subadult, female subadult, and male adult groups, or among the female adult, female subadult, male adult, and mixed-sex adult groups (P > 0.05).
For Type C, the high frequency in the mixed-sex adult group was significantly lower than that in the male subadult and female adult groups (P < 0.05). There was no significant difference among the male subadult, male adult, and female subadult groups (P > 0.05).
For Type J, the high frequency in the female adult group was higher than that in the female subadult group (P < 0.05). There was no significant difference in the high frequency among the male subadult, male adult, female subadult, and mixed-sex adult groups (P > 0.05).
Call duration
Four call types showed significant differences in durations among the sex–age groups. The other eight call types showed no significant differences in duration among groups (Table 3, Figure 6, and Supplementary Table 4).
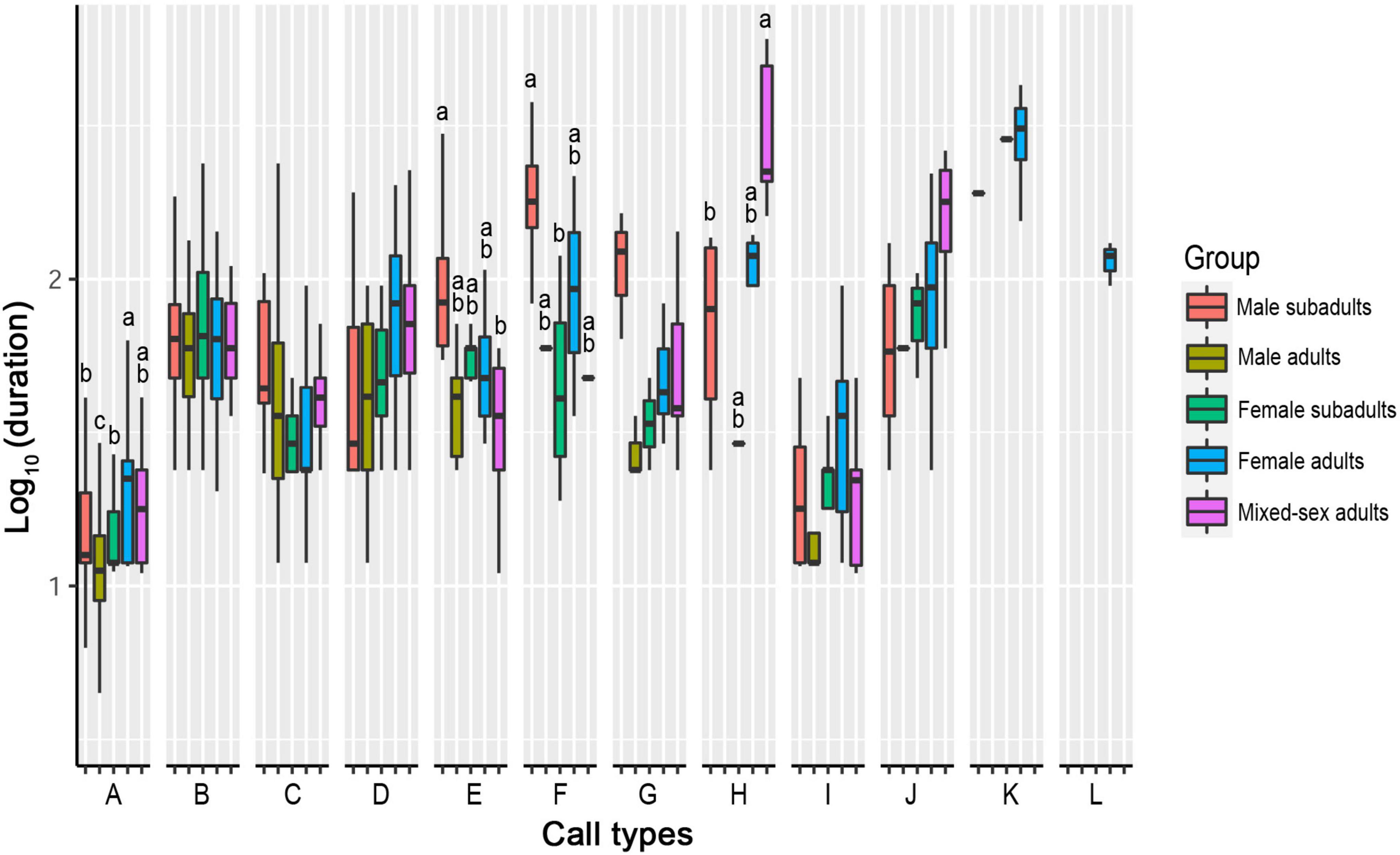
Figure 6. Differences in call duration among five sex–age groups for all call types. The y-axis is the log-transformed value of duration. The x-axis represents the call types. Boxplots with different lowercase letters refer to Kruskal–Wallis comparison tests yielding significant results (P < 0.05), and the relationship among the letters is a > b > c > d. Boxplots with the same lowercase letters did not differ significantly (P > 0.05). Call types without letters on the boxplots showed no significant differences among sex–age groups.
For Type A, the call duration in the male adult group was shorter than that in all other groups (P < 0.05). The call duration in the female adult group was significantly longer than that in the female subadult and male subadult groups (P < 0.05). There was no significant difference among the male subadult, female subadult, and mixed-sex adult groups or between the female adult and mixed-sex adult groups (P > 0.05).
For Type E, the call duration in the male subadult group was longer than that in the mixed-sex adult group (P < 0.05). There was no significant difference in call duration among the male adult, female adult, female subadult, and mixed-sex adult groups or among the male adult, female adult, female subadult, and male subadult groups (P > 0.05).
For Type F, the call duration in the male subadult group was longer than that in the female subadult group (P < 0.05). There was no significant difference in call duration among the male adult, female adult, female subadult, and mixed-sex adult groups or among the male adult, female adult, male subadult, and mixed-sex adult groups (P > 0.05).
For Type H, the call duration in the male subadult group was shorter than that in the mixed-sex adult group (P < 0.05). There was no significant difference in call duration among the male adult, female adult, female subadult, and male subadult groups or among the male adult, female adult, female subadult, and mixed-sex adult groups (P > 0.05).
Discussion
Vocalization differences between different sex and age groups
In many species with sexually dimorphic body sizes, the smaller of the two sexes produces higher-frequency vocalizations (Maurer et al., 2008). Studies of tortoises showed that vocal frequency is inversely proportional to the animal size (Galeotti et al., 2005). Similar patterns were found in birds and amphibians (Bowman, 1983; Ryan, 1985; Ryan and Brenovitz, 1985). Males of two species of jacana produce higher-frequency calls compared to those produced by larger-bodied females (Buck et al., 2020). The peak frequency of the calls of different tree frog species may decrease with increasing body size (Sun et al., 2017). Male subadult red-eared sliders emitted higher frequencies of Type A, B, and C calls compared to the other groups (Figure 3), but male subadults were the smallest in size among the four sex–age groups. A larger body size typically indicates a larger vocal organ, which produces lower frequencies (Narins et al., 2006; Garcia et al., 2013). In the same way, smaller red-eared sliders have smaller vocal organs and therefore produce higher frequencies of sound.
However, body size may not always be inverse to vocal frequency. Females of several owl species have smaller syringes and higher-pitched vocalizations than males but are larger than males (Miller, 1934). Male chimpanzees have higher peak frequencies than females in two call types (males are larger than females) (Ammie, 2019). Baboons (Papio cynocephalus) (Fischer et al., 2004) and gibbons (Hylobates lar) (Barelli et al., 2013) show similar patterns. The Jacana spinosa, which is larger than Jacana jacana, has a higher peak frequency of calls than J. jacana (Buck et al., 2020). Compared to the other groups, the female adult red-eared sliders produced more high-frequency calls, whereas female adults had the largest body size among all groups. High-frequency vocalizations are produced by the Amazonian River turtle (Podocnemis expansa) mainly during nesting (Ferrara et al., 2014a). We did not perform the recording during breeding season; however, the ability of adult females to produce high-frequency calls may be related to their reproductive behaviors.
Turtles’ calls may be related to mating success (Galeotti et al., 2005), which can reflect the preferences of female turtles (Galeotti et al., 2004) and transmit individual information (Sacchi et al., 2003). In our recordings, each sex–age group presented unique characteristics (Table 1). Turtles’ individual-specific vocalizations are used for individual recognition (Charrier et al., 2022). Red-eared sliders exhibit sex- and age-specific vocalizations, indicating their communication function.
Range of hearing and frequency of sound
Some studies have shown that the hearing threshold in air of red-eared sliders is 200–1,100 Hz (Christensen-Dalsgaard et al., 2012; Wang et al., 2019). Our records showed that most of the underwater calls produced by red-eared sliders were around or below the frequency range of their known hearing range (Table 1). However, the peak frequencies of four types (Type I, J, K, and L) were beyond the known hearing range of these turtles.
Similar results have been observed for the acoustics of green sea turtles (Chelonia mydas) and leatherback (Dermochelys coriacea), whose in air hearing ranges are lower than their peak frequency of vocalization (Cook and Forrest, 2005; Piniak et al., 2012a,b; Ferrara et al., 2014b). Yangtze finless porpoise (Neophocaena phocaenoides asiaeorientalis) is highly sensitive to sounds that are far below the frequency of signals they emit (Li et al., 2005; Wang et al., 2020). Amphibians were thought to be unable to hear high-frequency sounds (Pettigrew et al., 1981), but some frogs have recently been shown to use ultrasonic vocalizations for communication (Feng et al., 2006; Arch et al., 2008, 2009).
The high-frequency signals may be byproducts of the sound production mechanism (Roverud, 1989) or they may be important components of tonal quality (Thorpe and Griffin, 1962; Wang et al., 2009). However, high-frequency signals can be advantageous under certain conditions. High-frequency calls contribute to resisting interference to communication from low-frequency noise common in natural rivers or lakes (Wang et al., 2020; Zhou et al., 2021).
Variety of call types
The pig-nosed turtle (Carettochelys insculpta) produces only three simple call types underwater (Ferrara et al., 2017). The South American giant river turtle (P. expansa) can produce 11 complex call types with many harmonics (Ferrara et al., 2013, 2014a). Lepidochelys kempii emits six types of calls (Ferrara et al., 2019). The Oblong turtle (C. oblonga) has 17 types of vocalizations, with the peak frequency less than 1,800 Hz (Giles et al., 2009). Compared with these aquatic turtles, red-eared sliders have a large variety of vocalizations, with a wide frequency range and various forms of calls include bout, pulse, harmonics, flat/up/down-sweep, and complex signal combinations.
As an invasive alien species, the red-eared slider is a threat to many native species. The varied vocalizations and wide-range frequency of its calls are indicative of high social skills as an aquatic species (Morton, 1977; Bednáøová et al., 2013). This is not only beneficial to their predation and positioning abilities (Gelfand and McCracken, 1986) but also their mating and reproductive behaviors, as diversiform vocalizations can transmit more individual information (Sacchi et al., 2003) and easily attract the female’s attention (Galeotti et al., 2005). These acoustical advantages may help the species better adapt to their environment and outcompete other aquatic turtles. As the calls were recorded in an artificial environment, not all acoustic descriptions reflect free-field recordings, particularly in their duration (Giles et al., 2009).
The average values of the correlation coefficients within most call types were high, but the average correlation coefficients within the three high-frequency types were low. This is due to the fact that all three call types have one characteristic in common: the frequency range and duration of calls within a type vary considerably. Hence, two calls with similar auditory sense and similar spectral shape will show lower correlation scores in the algorithm when they occupy different frequency ranges and different durations. Improved methods may be needed to solve this problem in the future.
Conclusion
We identified 12 underwater call types produced by red-eared sliders. These vocalizations differed significantly among turtles of different ages and sexes. Males vocalized pulse calls very frequently, whereas a high proportion of high-frequency call types was emitted by the female adult group. Some call types produced by red-eared sliders exceeded the frequency range of their known hearing range. The varied vocalizations of red-eared sliders may help the species to better adapt to their environment and outcompete other aquatic turtles. Further studies are needed to determine the function of these high-frequency calls and whether turtles can hear such high-frequency sounds.
Data availability statement
The raw data supporting the conclusions of this article will be made available by the authors, without undue reservation.
Ethics statement
The animal study was reviewed and approved by Animal Research Ethics Committee of Hainan Provincial Education Center for Ecology and Environment, Hainan Normal University.
Author contributions
LZ wrote the manuscript with support from L-HZ, HL, TW, HS, and JW. LZ and JW conceptualized the study. LZ, HL, and TW conducted the experiments. LZ performed the final data analysis with suggestions of L-HZ, HS, and JW. All authors contributed to the article and approved the submitted version.
Funding
This work was supported by the National Natural Science Foundation of China program (Grant No. 31860608) and the Postdoctoral Research Project of Hainan Province (Grant No. RC2100007855).
Acknowledgments
We appreciated graduate students Jinhong Lei and Bo Chen of Hainan Normal University, who helped a lot by assisting with the data recording.
Conflict of interest
The authors declare that the research was conducted in the absence of any commercial or financial relationships that could be construed as a potential conflict of interest.
Publisher’s note
All claims expressed in this article are solely those of the authors and do not necessarily represent those of their affiliated organizations, or those of the publisher, the editors and the reviewers. Any product that may be evaluated in this article, or claim that may be made by its manufacturer, is not guaranteed or endorsed by the publisher.
Supplementary material
The Supplementary Material for this article can be found online at: https://www.frontiersin.org/articles/10.3389/fevo.2022.1022052/full#supplementary-material
References
Ammie, K. K. (2019). “Evidence for sexual dimorphism in chimpanzee vocalizations: A comparison of male and female call production and acoustic parameters,” in The Chimpanzees of the Taï forest 40 years of research, eds C. Boesch and R. Wittig (Cambridge: Cambridge University Press), 410–421. doi: 10.1017/9781108674218.026
Arch, V., Grafe, T., and Narins, P. (2008). Ultrasonic signalling by a Bornean frog. Biol. Lett. 4, 19–22. doi: 10.1098/rsbl.2007.0494
Arch, V., Grafe, T., Gridi-Papp, M., and Narins, P. (2009). Pure ultrasonic communication in an endemic Bornean frog. PLoS One 4:e5413. doi: 10.1371/journal.pone.0005413
Barelli, C., Mundry, R., Heistermann, M., and Hammerschmidt, K. (2013). Cues to androgens and quality in male gibbon songs. PLoS One 8:e82748. doi: 10.1371/journal.pone.0082748
Bednáøová, R., Hrouzková-Knotková, E., Burda, H., Sedláèek, F., and Šumbera, R. (2013). Vocalizations of the giant mole-rat (Fukomys mechowii), a subterranean rodent with the richest vocal repertoire. Bioacoustics 22, 87–107. doi: 10.1080/09524622.2012.712749
Boatright-Horowitz, S. S., Cheney, C. A., and Simmons, A. M. (1999). Atmospheric and underwater propagation of bullfrog vocalizations. Bioacoustics 9, 257–280. doi: 10.1080/09524622.1999.9753404
Bowman, R. I. (1983). “The evolution of song in Darwin’s finches,” in Patterns of evolution in Galapagos organisms, eds R. I. Bowman, M. Berson, and A. E. Leviton (San Francisco, CA: American Association for the Advancement of Science), 237–537.
Buck, E. J., Monroe, T., Zwicky, G., Derryberry, E. P., and Lipshutz, S. E. (2020). Species and sex differences in vocalizations between sex-role reversed shorebirds, Northern Jacana (Jacana spinosa) and Wattled Jacana (J. jacana). Wilson J. Ornithol. 132, 343–351. doi: 10.1676/1559-4491-132.2.343
Charrier, I., Jeantet, L., Maucourt, L., Régis, S., Lecerf, N., Benhalilou, A., et al. (2022). First evidence of underwater vocalizations in green sea turtles Chelonia mydas. Endanger. Species Res. 48, 31–41. doi: 10.3354/esr01185
Christensen-Dalsgaard, J., Brandt, C., Willis, K. L., Christensen, C. B., Ketten, D., Edds-Walton, P., et al. (2012). Specialization for underwater hearing by the tympanic middle ear of the turtle, Trachemys scripta elegans. Proc. R. Soc. B 279, 2816–2824. doi: 10.1098/rspb.2012.0290
Cook, S. L., and Forrest, T. G. (2005). Sounds produced by nesting leatherback sea turtles (Dermochelys coriacea). Herpetol. Rev. 36, 387–390.
Dallal, G. E., and Wilkinson, L. (1986). An analytic approximation to the distribution of Lilliefor’s test statistic for normality. Am. Stat. 40, 294–296. doi: 10.1080/00031305.1986.10475419
Feng, A., Narins, P., Xu, C., Lin, W., Yu, Z., Qiu, Q., et al. (2006). Ultrasonic communication in frogs. Nature 440, 333–336. doi: 10.1038/nature04416
Ferrara, C. R., Vogt, R. C., Sousa-Lima, R., Tardio, B., and Bernardes, V. (2014a). Sound communication and social behavior in an Amazonian river turtle (Podocnemis expansa). Herpetologica 70, 149–156. doi: 10.1655/HERPETOLOGICA-D-13-00050R2
Ferrara, C. R., Mortimer, J. A., and Vogt, R. C. (2014b). First evidence that hatchlings of Chelonia mydas emit sounds. Copeia 2, 245–247. doi: 10.1643/CE-13-087
Ferrara, C. R., Vogt, R. C., and Sousa-Lima, R. S. (2013). Turtle vocalizations as the first evidence of posthatching parental care in chelonians. J. Comp. Psychol. 127, 24–32. doi: 10.1037/a0029656
Ferrara, C. R., Vogt, R. C., Eisemberg, C. C., and Doody, J. S. (2017). First evidence of the pig-nosed turtle (Carettochelys insculpta) vocalizing underwater. Copeia 105, 29–32. doi: 10.1643/CE-16-407
Ferrara, C. R., Vogt, R. C., Sousa-Lima, R. S., Lenz, A., and Morales-Mávil, J. E. (2019). Sound communication in embryos and hatchlings of Lepidochelys kempii. Chelon. Conserv. Biol. 18, 1–6. doi: 10.2744/CCB-1386.1
Fischer, J., Kitchen, D. M., Seyfarth, R. M., and Cheney, D. L. (2004). Baboon loud calls advertise male quality: Acoustic features and their relation to rank, age, and exhaustion. Behav. Ecol. Sociobiol. 56, 140–148. doi: 10.1007/s00265-003-0739-4
Galeotti, P., Sacchi, R., Fasola, M., and Ballasina, D. (2005). Do mounting vocalisations in tortoises have a communication function? A comparative analysis. Br. J. Herpetol. 15, 61–71.
Galeotti, P., Sacchi, R., Rosa, D. P., and Fasola, M. (2004). Female preference for fast-rate, high-pitched calls in Hermann’s tortoises (Testudo hermanni). Behav. Ecol. 16, 301–308. doi: 10.1093/beheco/arh165
Garcia, M., Charlton, B. D., Wyman, M. T., Fitch, W. T., and Reby, D. (2013). Do red deer stags (Cervus elaphus) use roar fundamental frequency (F0) to assess rivals? PLoS One 8:e83946. doi: 10.1371/journal.pone.0083946
Gelfand, D. L., and McCracken, G. F. (1986). Individual variation in the isolation calls of Mexican free-tailed bat pups (Tadarida brasiliensis Mexicana). Anim. Behav. 34, 1078–1086. doi: 10.1016/S0003-3472(86)80167-1
Gerhardt, H. C., and Huber, F. (2002). Acoustic communication in insects and anurans: Common problems and diverse solutions. Chicago, IL: The University of Chicago Press.
Gibbons, J. D. (1985). Nonparametric statistical inference, 2nd Edn. New York, NY: Marcel Dekker. doi: 10.2307/2531272
Giles, J. C., Davis, J. A., Mccauley, R. D., and Kuchling, G. (2009). Voice of the turtle: The underwater acoustic repertoire of the long-necked freshwater turtle, Chelodina oblonga. J. Acoust. Soc. Am. 126, 434–443.
Li, S., Wang, K., Wang, D., and Akamatsu, T. (2005). Echolocation signals of the free-ranging Yangtze finless porpoise (Neophocaena phocaenoides asiaeorientialis). J. Acoust. Soc. Am. 117, 3288–3296. doi: 10.1121/1.1882945
Ma, K., and Shi, H. (2017). “Red-eared slider Trachemys scripta elegans (Wied-Neuwied),” in Biological invasions and its management in China, eds F. Wan, M. Jiang, and A. Zhan (Dordrecht: Springer), 49–76. doi: 10.1007/978-981-10-3427-5_4
Ma, K., Li, C., Shi, H. T., Wang, J., Li, D., and Wang, J. (2013). Home range comparison of exotic species Trachemys scripta elegans and native species Mauremys sinensis in the Qionghai section of Wanquan river, Hainan Island, China. Chin. J. Zool. 48, 331–337.
Mader, D. R. (2006). Reptile medicine and surgery, 2nd Edn. St. Louis, FL: Saunders. doi: 10.1016/B0-7216-9327-X/X5001-9
Manolakis, D. G., Ingle, V. K., and Kogon, S. M. (2005). Statistical and adaptive signal processing: Spectral estimation, signal modeling, adaptive filtering and array processing (artech house signal processing library). Norwood, MA: Artech House.
Maurer, G., Smith, C., Süsser, M., and Magrath, R. D. (2008). Solo and duet calling in the pheasant coucal: Sex and individual call differences in a nesting cuckoo with reversed size dimorphism. Aust. J. Zool. 56, 143–149. doi: 10.1071/ZO08049
McKenna, L. N., Paladino, F. V., Tomillo, P. S., and Robinson, N. J. (2019). Do sea turtles vocalize to synchronize hatching or nest emergence? Copeia 107, 120–123. doi: 10.1643/ce-18-069
Miller, A. H. (1934). The vocal apparatus of North American owls. Condor 36, 204–213. doi: 10.2307/1363856
Monteiro, C. C., Carmo, H., Santos, A., Corso, G., and Sousa-Lima, R. S. (2019). First record of bioacoustic emission in embryos and hatchlings of hawksbill sea turtles (Eretmochelys imbricata). Chelon. Conserv. Biol. 18, 273–278. doi: 10.2744/CCB-1382.1
Morton, S. (1977). On the occurrence and significance of motivation–structural rules in some birds and mammal sounds. Am. Nat. 111, 855–869. doi: 10.1086/283219
Narins, P. M., Feng, A. S., and Fay, R. R. (2006). Hearing and sound communication in amphibians, Vol. 28. New York, NY: Springer Science and Business Media. doi: 10.1007/978-0-387-47796-1
Pellitteri, R. D. (2003). Sexual selection of Testudo Hermanni (Reptilia, Testudinidae): The relationship between morphology-physiological conditions, courtship, singing, and mating success. Master’s thesis. Pavia: University of Pavia.
Pettigrew, A., Anson, M., and Chung, S. (1981). Hearing in the frog: A neurophysiological study of the auditory response in the midbrain. Proc. R. Soc. Lond. Ser. B 212, 433–457. doi: 10.1098/rspb.1981.0047
Piniak, W. E. D., Eckert, S. A., Harms, C. A., and Stringer, E. M. (2012a). Underwater hearing sensitivity of the leatherback sea turtle (Dermochelys coriacea): Assessing the potential effect of anthropogenic noise. Herndon, VA: U.S. Department of the Interior and Bureau of Ocean Energy Management, 8–13.
Piniak, W. E. D., Mann, D. A., Eckert, S. A., and Harms, C. A. (2012b). “Amphibious hearing in sea turtles,” in The effects of noise on aquatic life (advances in experimental medicine and biology), eds A. N. Popper and A. Hawkins (New York City, NY: Springer), 83–87. doi: 10.1007/978-1-4419-7311-5_18
Qiu, Z., Hong, M., Zhang, H., Chen, P., and Shi, H. (2015). A comparative study of anti-oxidative ability between Trachemys scripta elegans and Mauremys sinensis under acute nitrite stress. Ecol. Sci. 34, 99–104.
Roverud, R. (1989). Harmonic and frequency structure used for echolocation sound pattern recognition and distance information processing in the rufous horseshoe bat. J. Comp. Physiol. A 166, 251–255. doi: 10.1007/BF00193469
Ryan, M. J., and Brenovitz, E. A. (1985). The role of body size, phylogeny, and ambient noise in the evolution of bird song. Am. Nat. 126, 87–100. doi: 10.1086/284398
Sacchi, R., Galeotti, P., Fasola, M., and Ballasina, D. (2003). Vocalizations and courtship intensity correlate with mounting success in marginated tortoises Testudo marginata. Behav. Ecol. Sociobiol. 55, 95–102. doi: 10.1007/s00265-003-0685-1
Sathyan, R., Engelbrecht, A., and Couldridge, V. C. K. (2017). Morphological, acoustic and genetic divergence in the bladder grasshopper Bullacris unicolor. Ethol. Ecol. Evol. 29, 1–22. doi: 10.1080/03949370.2017.1287915
Shi, H., Gong, S., Liang, W., Hong, M., Fu, L., and Wang, J. (2009). Control of the spread of exotic red-eared turtles in China’s wild environment. Bull. Biol. 44, 1–3.
Sun, Z., Wang, T., Zhu, B., and Wang, J. (2017). Characteristics and rhythm of the song of the red webbed tree frog during reproduction. Chin. J. Ecol. 36, 1672–1677.
Thorpe, W., and Griffin, D. (1962). Ultrasonic frequencies in bird song. Nature 193:595. doi: 10.1038/193595a0
Wang, J. (2010). A kind of sound bait. Chinese Patent No CN201860653U. Qingdao: China National Intellectual Property Administration.
Wang, K., Wang, D., Fang, L., Wang, Z., Wu, Y., Duan, G., et al. (2010). Acoustic driving and protecting method for Sousa Chinensis in construction sea area. Chinese Patent CN 201410423815. Wuhan: China National Intellectual Property Administration.
Wang, T., Li, H., Cui, J., Zhai, X., Shi, H., and Wang, J. (2019). Auditory brainstem responses in the red-eared slider Trachemys scripta elegans (Testudoformes: Emydidae) reveal sexually dimorphic hearing sensitivity. J. Comp. Physiol. A 205, 847–854. doi: 10.1007/s00359-019-01372-y
Wang, X., Wang, D., Wu, X., Wang, C., Wang, R., and Xia, T. (2009). Response specificity to advertisement vocalization in the Chinese alligator (Alligator sinensis). Ethology 115, 832–839. doi: 10.1111/j.1439-0310.2009.01671.x
Wang, Z., Li, J., Duan, P., Mei, Z., Niu, F., Akamatsu, T., et al. (2020). Evoked-potential audiogram variability in a group of wild Yangtze finless porpoises (Neophocaena asiaeorientalis asiaeorientalis). J. Comp. Physiol. A 206, 527–541. doi: 10.1007/s00359-020-01426-6
Wei, C., Yang, Z., Hong, M., Bo, T., Zhang, J., He, B., et al. (2012). Comparative study on hunger tolerance between exotic species of red-eared turtle and native species of Chinese striped turtle. Sichuan J. Zool. 31, 430–434.
Yuan, C. (2020). A frog food trap. Chinese Patent No CN211458562U. Chengdu: China National Intellectual Property Administration.
Zhao, L., Jie, L., Wei, C., Wang, J., Hong, M., and Shi, H. (2013). Comparisons on the competitive ability for food between trachemys scripta elegans and Mauremys sinensis. Sichuan J. Zool. 32, 873–878. doi: 10.3969/j.issn.1000-7083.2013.06.015
Zhou, L., Chen, X., Duan, P., Wang, D., Wang, Z., and Wang, K. (2021). Spatial-temporal variations in biosonar activity of Yangtze finless porpoise in the lower reaches of the Yangtze River and its correlation with underwater noise: Are quieter non-shipping branches the remaining shelters? Aquat. Conserv. 31, 964–978. doi: 10.1002/aqc.3535
Keywords: red-eared slider, vocalization, sex-age difference, underwater recording, call type
Citation: Zhou L, Zhao L-H, Li H, Wang T, Shi H and Wang J (2022) Underwater vocalizations of Trachemys scripta elegans and their differences among sex–age groups. Front. Ecol. Evol. 10:1022052. doi: 10.3389/fevo.2022.1022052
Received: 18 August 2022; Accepted: 06 October 2022;
Published: 14 November 2022.
Edited by:
Guo-Hua Ding, Lishui University, ChinaReviewed by:
Liang Fang, South China Sea Fisheries Research Institute (CAFS), ChinaGhulam Nabi, Hebei Normal University, China
Copyright © 2022 Zhou, Zhao, Li, Wang, Shi and Wang. This is an open-access article distributed under the terms of the Creative Commons Attribution License (CC BY). The use, distribution or reproduction in other forums is permitted, provided the original author(s) and the copyright owner(s) are credited and that the original publication in this journal is cited, in accordance with accepted academic practice. No use, distribution or reproduction is permitted which does not comply with these terms.
*Correspondence: Jichao Wang, wjc@hainnu.edu.cn