- 1College of Life Sciences, Xinjiang Agricultural University, Ürümqi, China
- 2College of Forestry, Nanjing Forestry University, Nanjing, Jiangsu, China
Both natural and sexual selection can shape sexual dimorphism. However, determination of the contribution of these selection pressures is challenging. In lizards, sexual selection can contribute to the larger head size of males than that of females. However, males and females can also diverge in their head size to prey on different food resources under conditions of limited resources (and/or high competitors). Here, 109 individuals from two sympatric desert racerunners (Eremias grammica: 28 males and 30 females; Eremias velox: 25 males and 26 females) were studied to determine their sexual head shape (head length, width, and depth). Additionally, 191 and 169 feces samples of E. grammica and E. velox, respectively, were collected to assess the niche divergence hypothesis (a proxy for natural selection). We found that both species had dimorphic head shapes; male heads (i.e., length, width, and depth) were significantly larger than female heads (P < 0.05, in all cases) in E. grammica, and male heads of E. velox were significantly longer than those of females (P < 0.05). Chi-square test revealed that there were significant differences in the proportion (Hymenopteran and Orthopteran) and sizes of prey type between the two sexes of E. grammica; conspecific males and females of E. velox differed in the proportion of Coleopteran and Hymenopteran prey. Both males and females of these two species had a high niche overlap index (range from ∼ 0.78 to 0.99) with each other. There were also significant differences in the sizes of the heads and prey between the two species (P < 0.05). However, the interspecific differences were mainly caused by interspecific male–male differences in morphological and prey traits. In summary, we believe that both natural (pressures from resource competition) and sexual selection drive sexual head shape dimorphism in these two sympatric lizards, owing to high food resource competition in arid regions. Therefore, head trait divergence can reduce competition by resulting in a preference for different prey types.
Introduction
Both natural and sexual selection can shape sexual dimorphism, which is described as a systematic difference in life-history traits between conspecific males and females (Darwin, 1871; Andersson, 1994). This phenomenon is widespread in reptiles (Cox et al., 2003, 2007; Liang et al., 2021a,b). However, determining the contribution of these selections remains a challenge because head trait variations correspond to differences in bite force (i.e., sexual selection) and prey consumption (i.e., natural selection) (Herrel et al., 2007). Specifically, sexual selection explains that males have larger heads than do females, to increase bite force and competition success during male–male combat (Darwin, 1871; Trivers, 1972). The niche divergence hypothesis (a proxy for natural selection) states that trait divergence between the two sexes (and hence using different microhabitats or food) is the outcome of competition for resources (i.e., larger sizes can eat larger prey, see Schoener, 1967, 1977; Preest, 1994). This hypothesis does not expect male-or female-biased sexual dimorphism but only focuses on trait differences between the sexes. Larger heads may provide access to larger and harder prey, resulting in an enlarged niche. However, we could not easily identify the roles of sexual and natural selection, and the extent of their contribution to males having larger heads. In particular, for some species with pronounced male–male competition, male heads were significantly larger than those of females; however, there was no difference in bite force between the two sexes (e.g., Trapelus sanguinolenta, see Wang et al., 2020). Ecological sexual dimorphisms are therefore thought to result either as a by-product of sexual selection and divergent gamete investment or through competition-driven niche partitioning between the sexes (i.e., ecological character displacement, Dayan and Simberloff, 1998; Lisle, 2019). However, studies on the relationship between head size and the niche divergence hypothesis are lacking (but see Herrel et al., 1999).
Under the niche divergence hypothesis, sexual size dimorphism can arise when males and females compete for limited resources (Dayan and Simberloff, 1998; Bolnick and Doebeli, 2003; Pincheira-Donoso et al., 2018). This can happen when food (MacArthur and Wilson, 1967) is limited and/or the number of potential competitors is high (a proxy of limited resources, see Pincheira-Donoso et al., 2009). However, global island analysis failed to detect a correlation between sexual size dimorphism and competitor number in reptiles (Meiri et al., 2014). The head is the only organ used by lizards to feed; if there is indeed potential resource competition, conspecific males and females would respond with more pronounced head shape divergence than body size (Andrews, 1979).
Phylogenetically correlated species have similar niches, and hence have potentially stronger interspecific competition (Pacala and Roughgarden, 1982; Hawlena and Pérez-Mellado, 2009). In particular, in regions with few resources (e.g., food) but diverse sympatric reptiles (e.g., in desert, see Roll et al., 2017), potential interspecific competition would be high. Therefore, the head divergence between the two sexes could reduce both intraspecific and interspecific competition for food resources (Nel et al., 2015). However, other studies have found that prey size does not correlate with the degree of head dimorphism (Zhao and Liu, 2013; Taverne et al., 2019). Among the several methods used to investigate species’ dietary habits, fecal samples are a widely accepted method [see reviewed in Pérez-Mellado et al. (2011)], because it does not require killing the individuals to obtain stomach or gut contents. Therefore, many studies (e.g., Capizzi, 1999; Hawlena and Pérez-Mellado, 2009) have used fecal pellets for dietary studies in lizards (but see Pincheira-Donoso, 2008).
In northwest China, there is a large area of arid desert with poor vegetation but with the second highest lizard richness in China (Liang et al., 2022). For example, there are five sympatric diurnal species in the Tukai Desert: two Eremias lizards (E. grammica and Eremias velox, see Figure 1), two Phrynocephalus lizards (P. mystaceus and P. guttatus), and one steppe agama lizard (Trapelus sanguinolenta) (Wang et al., 2020). Among these five species, T. sanguinolenta is the largest, inhabiting the shrub-ground niche, whereas the other four species are strictly ground-dwelling. For Phrynocephalus, these two species have different populations (i.e., P. mystaceus is rarely observed, while P. guttatus is widespread in the region) and with slight overlap in daily activity time (P. mystaceus is active between 12:00 and 16:00; authors’ personal observation). P. mystaceus is the largest species of this genus, while P. guttatus is a medium-sized species, implying potentially weak competition in Phrynocephalus. In contrast, both species of Eremias were abundant in this desert with similar daily activity times and body sizes. Therefore, coexistence of these phylogenetically related sympatric species is a notable phenomenon; assessing both inter- and intraspecific differences in morphology and prey between these two species may aid in a better understanding. In this study, we examined sexual head dimorphism and its correlation with the niche divergence hypothesis in two Eremias lizards. We aimed to address the following questions:
(1) What are the sexual head shape dimorphism patterns in these two species? Both studied species of Eremias exhibit pronounced male–male combat in the wild (field observation, also see Liang et al., 2018 for E. arguta); therefore, we predicted that males have larger heads than females because of sexual selection. Sexual selection contributes to larger male heads, which is correlated with male–male competition for mating (Gvozdik and Damme, 2003; Lailvaux and Irschick, 2007; Wang et al., 2020).
(2) Does sex-specific divergence in prey composition and size exist in these two species? We predicted that both prey composition and size would differ between the sexes of these two species as it would reduce sexual resource competition within species.
(3) As these two lizards are sympatric and phylogenetically related, the potential food competition between these two species is high. Therefore, we also predicted that there would be differences in prey between the two species to reduce interspecific competition.
Materials and methods
Morphology and feces collection
From July 2018, to August 2019, 61 and 51 E. grammica (females: 30, males: 31) and E. velox (females: 26, males: 25) individuals were captured from the Tukai Desert (WGS 84, longitude: 80.76°, latitude: 43.97°), Xinjiang, China, respectively. Sexual maturity sizes were 36.17 mm and 42.00 mm for E. velox (Wang et al., 2014) and E. grammica (Zhao et al., 1999), respectively. We measured the snout-vent length (SVL), head length (HL), head width (HW), and head depth (HD) using digital calipers (Shanggong calipers, 0–150 mm). All measurements were accurate within 0.01 mm.
The lizards were housed in plastic cages (30 × 10 × 15 cm), specifically to collect fecal samples (some individuals had more than one fecal sample). Each cage housed two or three individuals with the same gender, these cages were placed in a room with ambient temperature that varied from 20 to 28°C with a 12-h light/12-h dark cycle. A 250 W light bulb was suspended at one end of each cage, 20 cm above the cage floor, and the lizards could freely move to warmer and cooler places within the cage. No feeding was performed until the fecal sample collection was completed (one to three days). We observed lizards every 4 h (but not at night) to collect the sample. A total of 191 and 169 fecal samples from E. grammica (female:111, male:80) and E. velox (female:82, male:87), respectively, were collected.
Prey composition and size
Dried fecal sample was placed in petri dishes filled with water for 12–24 h, in order to soften them. Subsequently, we used forceps to separate and collect prey remains (antenna, chelicerae, telson, elytron, etc.). The prey remains were preserved in the same plastic sealing bags of the fecal samples (i.e., the number of plastic sealing bags was equal to the number of feces). One of us (L.S.) used a stereo microscope and identification keys to identify taxa at the order or family level based on the remains of these prey (Xin et al., 1985). Both the left- and right-sided elements were considered effective individuals (see Results section). For Coleoptera, tergites were well maintained in feces, which could be a good proxy for insect intake sizes. As the bigger head has a bigger bite force, it can prey on bigger and harder prey (especially Coleoptera). Therefore, we measured the length and width of the tergites for further purposes (see below).
Statistical analyses
First, we assessed the normality and homogeneity of the variance of the data. We used the Student’s t-test to assess the SVL difference between males and females, and further used the analysis of covariance to assess the difference in head sizes (HL, HW, HD), with SVL as the covariance because heads were correlated with lizard body sizes (Liang et al., 2018). Fisher’s exact test was used to assess the differences in prey composition between the two sexes. We also performed a Student’s t-test to assess the difference in tergite size (length and width) between the two sexes for these two species. We used the “space” R-package to assess the niche width of males and females and the niche overlap index (Zhang, 2016). We also repeated all analyses (morphology, prey, and niche) between the two species to assess the potential interspecific competition. We analyzed the differences in: (1) all individuals regardless of sexual dimorphism and (2) sex-specific differences between the two species. Data were log-10 transformed to normalize residuals and reduce heteroscedasticity (King, 2000). Graphs were created using “ggplot2” (Wickham, 2016). Descriptive statistics were presented as mean ± standard error (SE).
Results
Sexual dimorphism and allometry of morphology
There was a significant difference in SVL between males (mean: 60.05 ± 0.86 mm) and females (mean: 56.73 ± 0.90 mm) of E. grammica (t = 2.69, df = 56, P = 0.009; Figure 2 and Table 1). Males had significantly larger length, width, and depth of the head than did females (P < 0.05 in all cases; Table 1). In E. velox, males were significantly larger than females in the SVL (t = 0.913, df = 48, P < 0.0001; Figure 2); further, males had significantly longer heads than females (P < 0.05, Table 1).
We found that sexual head dimorphism was correlated with sex-specific allometric patterns of the head in E. grammica; head length (slope: 0.027 ± 0.011, t = 2.42, P = 0.018), width (slope: 0.021 ± 0.009, t = 2.17, P = 0.034), and depth (slope: 0.034 ± 0.01, t = 3.1, P = 0.003) increased more rapidly in males than in females with increasing body size (Figure 3). However, E. velox exhibited less support for allometric patterns; head length, but not width or depth, increased more quickly in males than in females (slope: – 0.022 ± 0.011, t = –2.03, P = 0.047), with increasing body size (Figure 3).
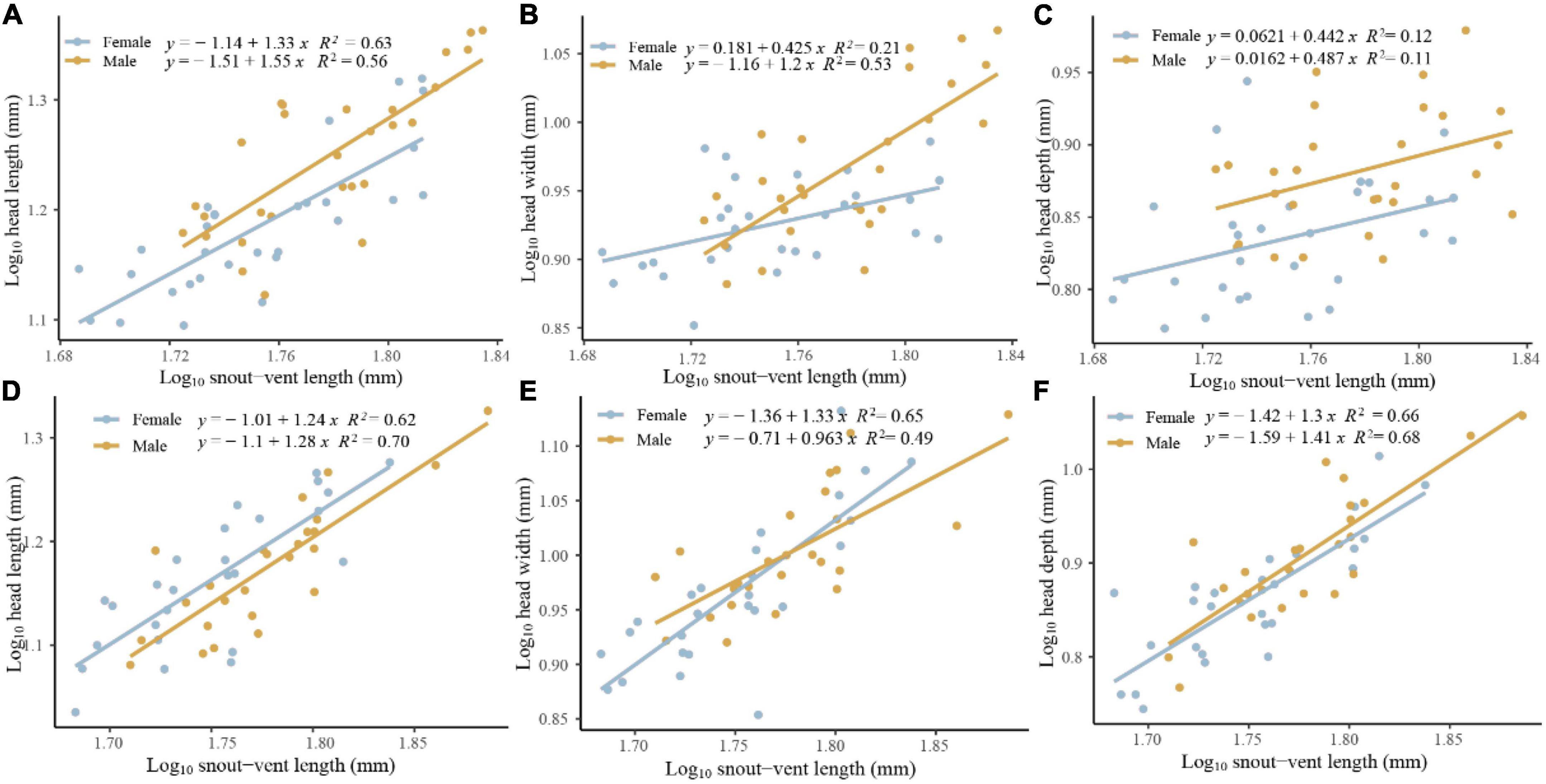
Figure 3. Allometric pattern of head length (A,D), width (B,E), and depth (C,F) for Eremias grammica (A–C) and E. velox (D–F) males and females.
Diet composition, size, and sexual dimorphism
In total, 527 prey items (299 in E. grammica and 228 in E. velox) belonged to 11 categories, all of which were insects (Table 2). The diet of E. grammica (299) comprised primarily Coleoptera larvae (30.4%), Acrididae (23.1%), and Formicidae (14.4%), and the remaining nine categories ranged from 0.3 to 10%, whereas E. velox comprised primarily Acrididae (41.6%), Coleoptera larvae (14.9%), and Buprestidae (13.5%), with the remaining nine categories ranging from 1.32 to 8.77% (Figure 4 and Table 2).
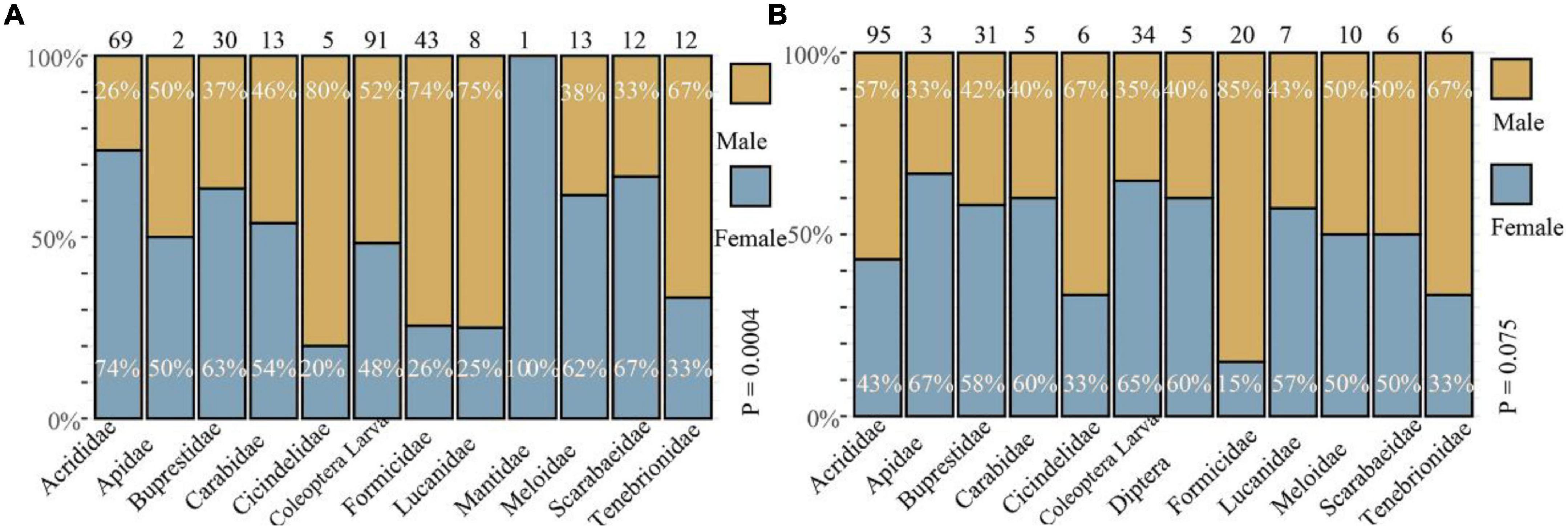
Figure 4. Frequency of prey composition (%) between the two sexes of Eremias grammica (A) and E. velox (B). The number in each histogram represents the total number of prey items. The top values indicate the proportion of males, while the bottom values indicate that of females.
Fisher’s exact test (Figure 4) revealed that male and female E. grammica were significantly different in prey composition (X2 = 35.84, P = 0.0005). Males had a higher proportion of Formicidae, Lucanidae, and Cicindelidae (74–80%) in their diet, and females had a higher proportion of Acrididae, Buprestidae, Meloidae, and Scarabaeidae (62–74%). The niche width of males and females was 5.21 and 4.72, respectively; while the overlap index was 0.78 between males and females of E. grammica. However, there was no significant difference in the proportion of prey between the two sexes of E. velox (X2 = 16.95, P = 0.097), although females had a high proportion of Apidae, Carabidae, and Diptera (60–67%). Male and female niche widths were 3.99 and 4.52, respectively, with a high niche overlap index of 0.99.
A total of 129 tergites belonging to E. grammica (21 female and 23 male) and E. velox (45 female and 40 male) were collected. We found significant differences in both length (males: 3.61 ± 0.28, females: 2.75 ± 0.16, t = 2.49, df = 42, P < 0.05) and width (males: 2.12 ± 0.16, females: 1.7 ± 0.08, t = 2.23, df = 42, P < 0.05) of the tergites between both sexes of E. grammica. However, differences were only found in the length (males: 3.07 ± 0.1, females: 2.73 ± 0.11, t = 2.64, df = 83, P < 0.05) of tergites between male and female E. velox (Figure 5).
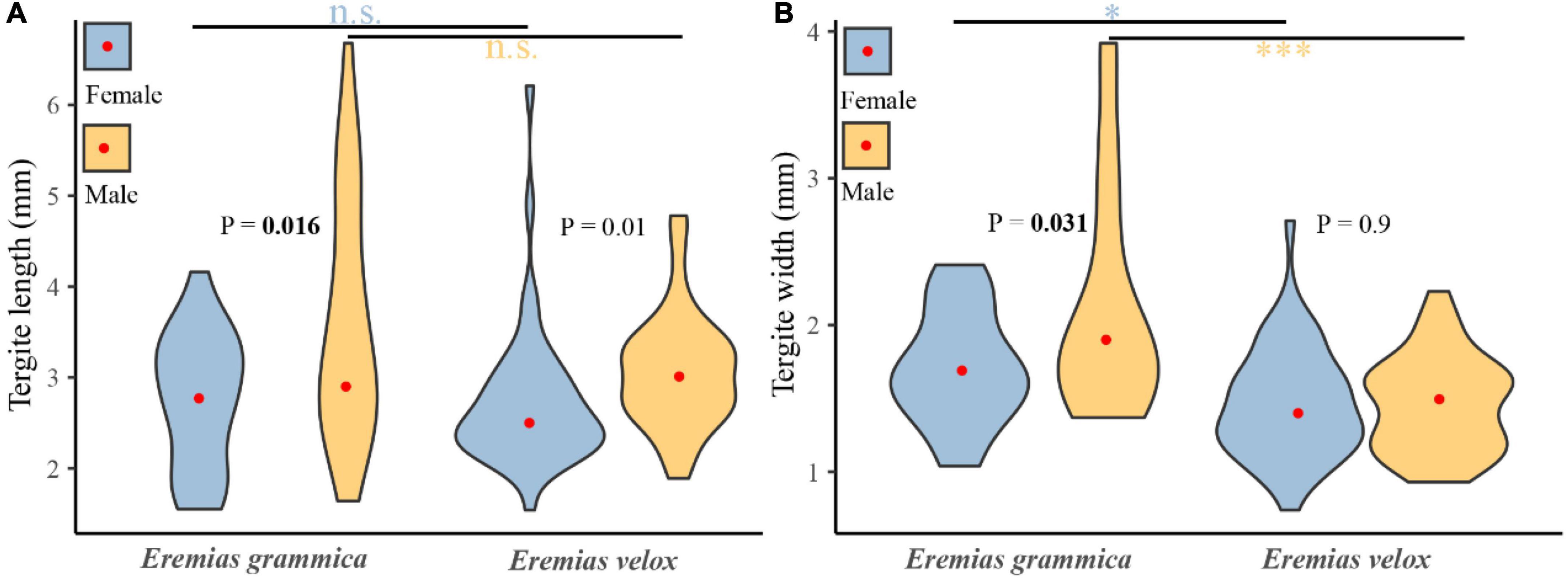
Figure 5. Differences of tergite length (A) and width (B) between males and females for Eremias grammica and E. velox. The red points are the mean values of size for each group. P–values represent the differences in prey sizes between males and females, and the line represents the differences in sizes between two species of the same sex. *P < 0.05, ***P < 0.001.
Interspecific variation
We found no significant differences in snout-vent length between E. grammica and E. velox (t = –0.111, df = 107, P = 0.912). However, there were significant differences between the heads of these two species: E. grammica had a larger head length (slope: – 0.045 ± 0.007, t = – 5.682, P < 0.001), whereas E. velox had a larger head width and depth (width: 0.038 ± 0.007, t = 5.23, P < 0.001, depth: 0.027 ± 0.008, t = 3.24, P = 0.002). Variations in interspecific males were similar to the overall results (P < 0.05, Table 1) within the sexes; however, for females, there was no interspecific variation in head length (P > 0.05, Table 1).
There was a significant difference in prey composition between the two species (P = 0.0004), and the differences between them were significant, with P = 0.0004 for males and P = 0.227 for females. Niche widths of E. grammica and E. velox were 5.41 and 4.38, respectively, while the niche overlap index was 0.76 between the two species. Females of these two species had a high niche overlap (0.99) compared to males (0.55). There was no significant difference in prey length between the two species; however, E. grammica had significantly wider prey than did E. volex (t = –0.111 ± 0.021, t = –5.31, P < 0.001). The results were still valid when we compared prey sizes, specifically within the sexes (Figure 5).
Discussion
Sexual dimorphism is a widespread phenomenon in lizards (e.g., body size, Cox et al., 2003; Liang et al., 2021a,b), which is thought to reflect the different reproductive roles between males and females (Orme et al., 2002; Bolnick and Doebeli, 2003). The head is important for feeding, locomotion, defensive behavior, among other vital functions; however, understanding the evolution of head variation is a complex task (Meyer et al., 2019; Wang et al., 2020). In the present study, the body and head sizes of male Eremias were larger than those of females (Table 1), and male lizards with relatively larger heads exhibited more rapid growth. Additionally, we found that males had different prey compositions and sizes in contrast to those of females. The differences in head traits and prey between these two species were mainly caused by interspecific male–male differences.
Sexual selection drives male-biased dimorphism
Previous studies have revealed that, in reptiles, males generally have relatively larger heads than do females after accounting for body size; this pattern can be found at both global (Scharf and Meiri, 2013) and local regional scales (see Liang et al., 2018 for E. arguta). This pattern was also observed in this study. A larger head in males allows for higher bite force than that in females, which is important during intersexual competition (Meyer et al., 2019; Cruz-Elizalde et al., 2020; but see Wang et al., 2020). Additionally, the larger heads of males could also possess larger vomeronasal organs, which is potentially beneficial for detecting females (e.g., Marvin, 2009).
All dimorphic traits of the head were correlated with the growth dynamics between males and females, implying that males exhibit more rapid growth than do females, and consequently larger head sizes (Figure 3). Both species (also see E. arguta, Liang et al., 2018) exhibited territorial behaviors (e.g., male–male competition) during our field observations. Males are aggressive, head and body sizes are likely important traits for signaling the fighting ability of Eremias males. Therefore, we suggest that sexual selection may result in sexual head dimorphism in both species.
Roles of niche divergence hypothesis in head dimorphism
Head size or shape dimorphism can arise if the sexes evolve appropriate adaptations for different prey: the head is an important organ in obtaining, processing, and ingesting food, and animals with larger (i.e., wider, taller) heads can prey on larger, and harder prey because of larger bite forces (Jiménez-Arcos et al., 2017). This is probably because of the known positive relationship between prey size and hardness (Herrel et al., 1999; Meyer et al., 2019). Consequently, sexual head dimorphism can reduce sexual resource competition by resulting in dietary differences between males and females, as has been reported in many species (Halloy et al., 2006; Losos, 2009). In this study, we found a relationship between the niche divergence hypothesis and male-biased sexual head dimorphism. Specifically: (1) larger-headed males of E. grammica had a higher proportion of Cicindelidae, Formicidae, and Lucanidae in the prey composition than did females (Figure 4), which was related to sexual head dimorphism; (2) males consumed larger sizes of Coleoptera than did females (measured by tergite sizes, Figure 5), suggesting that males could prey on larger insects.
We found weak support for niche divergence in E. velox because both prey composition and size showed marginal differences between the two sexes. Males and females had a high niche overlap index (0.99), which was higher than that of E. grammica (0.78). This pattern is consistent with that of sexual head dimorphism because neither width nor depth was different between males and females in E. velox. Similar results have been reported for other arid lizards (Liu et al., 2011; Zhao and Liu, 2013). This is regardless of the species exhibiting sexual head dimorphism without prey divergence (Zhao and Liu, 2013; Taverne et al., 2019). It is still not easy to determine the contribution of sexual and natural selection in this study, because if sexual selection primarily contributes to sexual head dimorphism, males and females differ in their consumption of available prey. Therefore, this would also result in niche segregation between the two sexes (i.e., niche divergence is the result of sexual selection). Males and females can also inhabit different microhabitats to avoid potential competition; however, this was not tested in our study, which should be assessed in the future.
Interspecific variation
Sexual dimorphism can arise when males and females compete for limited resources (Dayan and Simberloff, 1998; Bolnick and Doebeli, 2003; Pincheira-Donoso et al., 2018). Phylogenetically related sympatric species have the same phenotypes in similar ecological circumstances (e.g., anoles, Losos, 2009), and therefore face high interspecific competition for resources. In this study, there was no difference between the body sizes of the two Eremias species, but significant differences were observed in both the head and prey between the two species. Moreover, the magnitude and forms of intersexual head trait divergence varied between these two species (e.g., E. velox showed less dimorphic head traits). Therefore, a potential explanation would be that, as resources are rare in arid regions, these two sympatric species have evolved different functional traits (head shape) and inhabited different niches to avoid interspecies and intraspecific competition.
Interplay of sexual and natural selections
We acknowledge that we still cannot determine the contribution of sexual and natural selection from the observations of this study, because niche divergence is sometimes a by-product of sexual selection. However, we found that the niche overlap index of the males of these two species (0.55) was much lower than that of the females (0.99), which suggested that interspecific competition was higher in males than in females. When resources are limited, pressures on males are extremely high in these two species; both need resources to meet their fitness requirements, and head divergence could help them inhabit different niches and avoid potential interspecific competition. Sexual head dimorphism and niche divergence may occur simultaneously. Thus, we suggest that both sexual and natural selection resulted in sexual head dimorphism in these two species.
Conclusion
In this study, we found both head shape dimorphism and prey divergence in two sympatric Eremias species, with noticeable head differences between the two sexes corresponding to significant differences in prey composition and size in E. grammica and vice versa in E. velox. Interspecific differences in the heads and preys of these two species were mainly due to male–male variations. We suggest that both sexual and natural selection resulted in sexual head dimorphism in these two sympatric species.
Data availability statement
The data presented in this article can be found online at www.datadryad.org (https://doi.org/10.5061/dryad.ht76hdrk3). Further inquiries can be directed to the corresponding author.
Author contributions
TL, LW, and LS participated in data collection as well as in the conception and writing of the article. TL analyzed the data. All authors contributed to the article and approved the submitted version.
Funding
We gratefully acknowledge funding from the National Natural Science Foundation of China (31660613).
Acknowledgments
We thank Jing An, Xinqi Wang, and Peng Zheng for their fieldwork help. We thank Guo-hua Ding, and two referees for commenting on an earlier draft of the manuscript. We would like to thank Editage (www.editage.cn) for English language editing.
Conflict of interest
The authors declare that the research was conducted in the absence of any commercial or financial relationships that could be construed as a potential conflict of interest.
Publisher’s note
All claims expressed in this article are solely those of the authors and do not necessarily represent those of their affiliated organizations, or those of the publisher, the editors and the reviewers. Any product that may be evaluated in this article, or claim that may be made by its manufacturer, is not guaranteed or endorsed by the publisher.
References
Andrews, R. M. (1979). Evolution of life histories: a comparison of Anolis lizards from matched island and mainland habitats. Breviora 454, 1–51.
Bolnick, D. I., and Doebeli, M. (2003). Sexual dimorphism and adaptive speciation: two sides of the same ecological coin. Evolution 57, 2433–2449. doi: 10.1111/j.0014-3820.2003.tb01489.x
Capizzi, D. (1999). Preliminary data on food habits of an Alpine population of Horvath’s Rock Lizard Lacerta horvathi MÉHELY, 1904. Herpetozoa 11, 117–120.
Cox, R. M., Butler, M., and John-Alder, H. B. (2007). The Evolution of Sexual Size Dimorphism in Reptiles. Oxford: Oxford University Press.
Cox, R. M., Skelly, S. L., and John-Alder, H. B. (2003). A comparative test of adaptive hypotheses for sexual size dimorphism in lizards. Evolution 57, 1653–1669. doi: 10.1111/j.0014-3820.2003.tb00371.x
Cruz-Elizalde, R., Villamar-Duque, T. E., and Ramírez-Bautista, A. (2020). Sexual dimorphism in size and shape in the viviparous lizard Abronia taeniata (Squamata: Anguidae) in central Mexico. Acta Zool. 102, 220–226. doi: 10.1111/azo.12329
Dayan, T., and Simberloff, D. (1998). Size patterns among competitors: ecological character displacement and character release in mammals, with special reference to island populations. Mamm. Rev. 28, 99–124. doi: 10.1046/j.1365-2907.1998.00029.x
Gvozdik, L., and Damme, R. V. (2003). Evolutionary maintenance of sexual dimorphism in head size in the lizard Zootoca vivipara: a test of two hypotheses. J. Zool. 259, 7–13. doi: 10.1017/S0952836902003308
Halloy, M., Robles, C., and Cuezzo, F. (2006). Diet in two syntopic neotropical lizard species of Liolaemus (Liolaemidae): interspecific and intersexual differences. Rev. Espaola Herpetol. 20, 47–56.
Hawlena, D., and Pérez-Mellado, V. (2009). Change your diet or die: predator-induced shifts in insectivorous lizard feeding ecology. Oecologia 161, 411–419. doi: 10.1007/s00442-009-1375-0
Herrel, A., Mcbrayer, L. D., and Larson, P. M. (2007). Functional basis for sexual differences in bite force in the lizard Anolis lineatopus. Biol. J. Linn. Soc. 91, 111–119. doi: 10.1111/j.1095-8312.2007.00772.x
Herrel, A., Spithoven, L., Damme, R. V., and Vree, F. D. (1999). Sexual dimorphism of head size in Gallotia galloti: testing the niche divergence hypothesis by functional analyses. Funct. Ecol. 13, 289–297. doi: 10.1046/j.1365-2435.1999.00305.x
Jiménez-Arcos, V. H., Sanabria-Urbán, S., and Castillo, R. C. (2017). The interplay between natural and sexual selection in the evolution of sexual size dimorphism in Sceloporus lizards (Squamata: Phrynosomatidae). Ecol. Evol. 7, 905–917. doi: 10.1002/ece3.2572
King, R. B. (2000). Analyzing the relationship between clutch size and female body size in reptiles. J. Herpetol. 34, 148–150. doi: 10.1111/j.0014-3820.2006.tb01089.x
Lailvaux, S. P., and Irschick, D. J. (2007). The evolution of performance-based male fighting ability in Caribbean Anolis lizards. Am. Nat. 170, 573–586. doi: 10.1086/521234
Liang, T., Li, Y., Zheng, P., and Shi, L. (2018). Sexual dimorphism and female reproduction of Eremias arguta. Arid Zone Res. 35, 1160–1166.
Liang, T., Meiri, S., and Shi, L. (2021a). Sexual size dimorphism in lizards: Rensch’s rule, reproductive mode, clutch size, and line fitting method effects. Integr. Zool. 17, 787–803. doi: 10.1111/1749-4877.12569
Liang, T., Shi, L., Bempah, G., and Lu, C. H. (2021b). Sexual size dimorphism and its allometry in Chinese lizards. Evol. Ecol. 35, 323–335. doi: 10.1007/s10682-021-10104-1
Liang, T., Zhou, L., Dai, W., Zhang, Z., and Shi, L. (2022). Spatial patterns and drivers of Chinese lizard richness among multiple scales. Asian Herpetol. Res. 13, 117–124. doi: 10.16373/j.cnki.ahr.210056
Lisle, S. P. D. (2019). Understanding the evolution of ecological sex differences: integrating character displacement and the Darwin-Bateman paradigm. Evol. Lett. 3, 434–447. doi: 10.1002/evl3.134
Liu, Y., Wang, S., and Shi, L. (2011). Effects of age and sex on diet composition of Phrynocephalus grumgrzimailoi. Chinese J. Zool. 46, 111–117.
Losos, J. B. (2009). Lizards in an Evolutionary Tree: Ecology and Adaptive Radiation of Anoles. Berkeley, CA: University of California Press.
MacArthur, R. H., and Wilson, E. O. (1967). The Theory of Island Biogeography. Princeton NJ: Princeton University Press.
Marvin, G. A. (2009). Sexual and seasonal dimorphism in the cumberland plateau woodland salamander, Plethodon kentucki (Caudata: Plethodontidae). Copeia 2009, 227–232. doi: 10.1643/ch-08-116
Meiri, S., Kadison, A. E., Novosolov, M., Pafilis, P., Foufopoulos, J., Itescu, Y., et al. (2014). The number of competitor species is unlinked to sexual dimorphism. J. Anim. Ecol. 83, 1302–1312. doi: 10.1111/1365-2656.12248
Meyer, J. D., Irschick, D. J., Vanhooydonck, B., Losos, J. B., Adriaens, D., and Herrel, A. (2019). The role of bite force in the evolution of head shape and head shape dimorphism in Anolis lizards. Funct. Ecol. 33, 2191–2202. doi: 10.1111/1365-2435.13438
Nel, K., Rimbach, R., and Pillay, N. (2015). Dietary protein influences the life-history characteristics across generations in the African striped mouse Rhabdomys. J. Exp. Zool. Ecol. Integr. Physiol. 323, 97–108. doi: 10.1002/jez.1903
Orme, C. D. L., Quicke, D. L. J., Cook, J. M., and Purvis, A. (2002). Body size does not predict species richness among the metazoan phyla. J. Evol. Biol. 15, 235–247. doi: 10.1046/j.1420-9101.2002.00379.x
Pacala, S., and Roughgarden, J. (1982). Resource partitioning and interspecific competition in two two-species insular Anolis lizard communities. Science 217, 444–446. doi: 10.1126/science.217.4558.444
Pérez-Mellado, V., Pérez-Cembranos, A., Garrido, M., Luiselli, L., and Corti, C. (2011). Using faecal samples in lizard dietary studies. Amphibia-Reptilia 32, 1–7. doi: 10.1163/017353710X530212
Pincheira-Donoso, D. (2008). Testing the accuracy of fecal-based analyses in studies of trophic ecology of lizards. Copeia 2008, 322–325. doi: 10.1643/CE-06-214
Pincheira-Donoso, D., Tregenza, T., Butlin, R. K., and Hodgson, D. J. (2018). Sexes and species as rival units of niche saturation during community assembly. Glob. Ecol. Biogeogr. 27, 593–603. doi: 10.1111/geb.12722
Pincheira-Donoso, D., Hodgson, D. J., Stipala, J., and Tregenza, T. (2009). A phylogenetic analysis of sex-specific evolution of ecological morphology in Liolaemus lizards. Ecol. Res. 24, 1223–1231. doi: 10.1007/s11284-009-0607-4
Preest, M. R. (1994). Sexual size dimorphism and feeding energetics in Anolis carolinensis: why do females take smaller prey than males? J. Herpetol. 28, 292–298. doi: 10.2307/1564527
Roll, U., Feldman, A., Novosolov, M., Allison, A., Bauer, A. M., Bernard, R., et al. (2017). The global distribution of tetrapods reveals a need for targeted reptile conservation. Nat. Ecol. Evol. 1, 1677–1682. doi: 10.1038/s41559-017-0332-2
Scharf, I., and Meiri, S. (2013). Sexual dimorphism of heads and abdomens: different approaches to ‘being large’ in female and male lizards. Biol. J. Linn. Soc. 110, 665–673. doi: 10.1111/bij.12147
Schoener, T. W. (1967). The ecological significance of sexual size dimorphism in the lizard Anolis conspersus. Science 155, 474–477.
Taverne, M., Fabre, A., King-Gillies, N., Krajnovi, M., Lisičić, D., Martin, L., et al. (2019). Diet variability among insular populations of Podarcis lizards reveals diverse strategies to face resource-limited environments. Ecol. Evol. 9, 12408–12420. doi: 10.1002/ece3.5626
Trivers, R. (1972). Parental Investment and Sexual Selection. Chicago, IL: Aldine Publishing Company.
Wang, X. Q., Liang, T., An, J., and Shi, L. (2020). Comparison of bite force performance and head shape between males and females of Trapelus sanguinolenta. Chinese J. Zool. 55, 29–36. doi: 10.13859/j.cjz.202001004
Wang, Y., Zhou, L., and Shi, L. (2014). Scalation variation and subspecies classification status of rapid racerunner. Sichuan J. Zool. 33, 13–18.
Xin, J., Yang, Q., and Hu, C. (1985). Insects Morphological Taxonomy. Shanghai: Fudan University Press.
Zhang, J. (2016). Spaa: Species Association Analysis. R Package Version 0.2.2. Available online at: https://CRAN.R-project.org/package=spaa (accessed July 15, 2022).
Zhao, E. M., Zhao, K. T., and Zhou, K. Y. (1999). Fauna Sinica, Reptilia (Squamata: Lacertilia). Beijing: Science Press.
Keywords: arid region, feces, niche divergence hypothesis, niche overlap index, preys, limited resources
Citation: Liang T, Wang L and Shi L (2022) Sexual and natural selection interplay in sexual head shape dimorphism of two sympatric racerunners (Squamata: Lacertidae). Front. Ecol. Evol. 10:1016885. doi: 10.3389/fevo.2022.1016885
Received: 11 August 2022; Accepted: 20 September 2022;
Published: 13 October 2022.
Edited by:
Guo-Hua Ding, Lishui University, ChinaReviewed by:
Lin Zhang, Hubei University of Chinese Medicine, ChinaJianli Xiong, Mianyang Normal University, China
Copyright © 2022 Liang, Wang and Shi. This is an open-access article distributed under the terms of the Creative Commons Attribution License (CC BY). The use, distribution or reproduction in other forums is permitted, provided the original author(s) and the copyright owner(s) are credited and that the original publication in this journal is cited, in accordance with accepted academic practice. No use, distribution or reproduction is permitted which does not comply with these terms.
*Correspondence: Lei Shi, bGVpc0B4amF1LmVkdS5jbg==
†These authors have contributed equally to this work and share first authorship