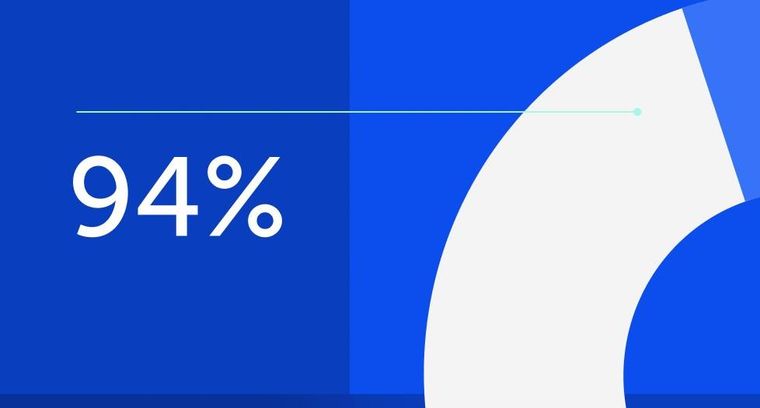
94% of researchers rate our articles as excellent or good
Learn more about the work of our research integrity team to safeguard the quality of each article we publish.
Find out more
ORIGINAL RESEARCH article
Front. Ecol. Evol., 31 October 2022
Sec. Conservation and Restoration Ecology
Volume 10 - 2022 | https://doi.org/10.3389/fevo.2022.1013244
The 2020 COVID-19 lockdown provides an opportunity to assess how the anthropause affected the behavior of birds. Black-headed gulls (Larus ridibundus) wintering at Dianchi Lake (Yunnan Province, southwestern China) prefer to forage on easily accessible human-provided food at various sites along the lake. Following the closure of the lake because of the pandemic, synthetic food was provided at a single location. We expected that the home range size and distribution of gulls would change in response to these changes in food provisioning. A total of 91 gulls were tagged with satellite transmitters in November 2018 and March 2019, and their movements were tracked during the winter months. We analyzed their home range size and spatial distribution in four periods, SCP2019, SOP2019, SCP2020, and SOP2020 (Scenic Opening/Closing Period in 2019/2020), and the difference between SOP2019 and SCP2019 was used as the control group. The eutrophication level in the wintering periods “Nov. 2018–Apr. 2019” and “Oct. 2019–Apr. 2020” was determined using the Normalized Difference Vegetation Index (NDVI), and the coverage ratio of algal blooms and NDVI were used as indicators of the amount of naturally available food. The home range sizes of gulls were reduced in SCP2020 compared with SOP2019, SCP2019, and SOP2020. The gulls were most abundant in the 600–900 m buffer zone and least abundant in the 0–300 m buffer zone in SCP2019; they were most abundant in the 0–300 m buffer zone and least abundant in the 900–2000 m buffer zone in SCP2020. These patterns were consistent with variation in the NDVI and the coverage ratio of algal blooms among buffer zones. Changes in wintering behaviors in SCP2020 relative to other periods suggested that gulls modified their behavior following anthropause-related changes in the distribution and provisioning of food. Our findings provide insights into the role of behavioral plasticity in mediating adaptation to changes in human activities in birds.
Lockdowns that restrict human movements and activities were implemented by various countries at the start of the global COVID-19 pandemic (Karnon, 2020). This dramatic global reduction in human activity has been referred to as “the anthropause” (Bates et al., 2020; Corlett et al., 2020; Rutz et al., 2020). This unprecedented event has provided scientists with an excellent opportunity to study the effects of large-scale shifts in human activities on wildlife (Corlett et al., 2020; Rutz et al., 2020).
Many birds have expanded their home ranges and colonized newly suitable habitats (e.g., urban areas) as a result of decreases in human disturbance during the COVID-19 lockdown, and this has had a positive effect on the survival of birds (Manenti et al., 2020; Schrimpf et al., 2021). However, some birds that prefer to forage on easily accessible human-provided food or build nests in densely populated areas to evade predators, such as barn swallows (Hirundo rustica), rock pigeons (Columba livia), and Torresian crows (Corvus orru), have experienced home range contractions and abandoned previously suitable habitats (Gilby et al., 2020; Hayashi et al., 2020; Soh et al., 2021).
Many studies of the effects of the COVID-19 lockdown on wildlife have confirmed that behavioral plasticity in birds has facilitated adaptation to the unpredictable changes associated with anthropogenic activities (Gilby et al., 2020; Hayashi et al., 2020; Manenti et al., 2020; Schrimpf et al., 2021; Soh et al., 2021). However, our current understanding of how behavioral plasticity has mediated adaptation in birds during the COVID-19 lockdown (e.g., distribution shifts from urban areas to natural environments) is mostly based on studies at large spatial scales; by contrast, few studies have quantitatively characterized changes in home ranges and the distribution of populations. This likely stems from the difficulty of obtaining detailed ecological data on the movements of bird populations. However, such data could greatly enhance our understanding of how behavioral plasticity mediates adaptation to environmental changes in birds.
Black-headed gulls (Larus ridibundus) greatly benefit from human activities and often occur in popular tourist destinations (Li and Liu, 2012). More than 40,000 gulls winter every year at Dianchi Lake in southwestern Kunming City, Yunnan Province, southwestern China. Haigeng Dam (HGD) in Dianchi Lake, a wetland park in Kunming, is a popular destination for tourists during the winter. The total length of HGD is 2.6 km, and the gulls are fed by tourists daily at various points scattered along the dam. Black-headed gulls began foraging at Dianchi Lake because of food shortages in 1985, and local residents provided them with buns and bread for many years (Li and Liu, 2012). Human-provided food has become an important food source for wintering gulls (Li and Liu, 2012). Gulls often wait to be fed at HGD during the daytime, are attracted to noises made by tourists, and sometimes even snatch food from tourists’ hands (Feng and Liang, 2020). Indeed, the gulls at this site prefer foraging on easily accessible human-provided food (Scott et al., 2015).
Dianchi Lake was closed on January 27, 2020, because of a COVID-19 outbreak. After the closure, gulls began to be fed at several fixed points along HGD (i.e., fixed-point provisioning; hereafter FPM) rather than at various scattered points (i.e., scattered provisioning; hereafter SPM) because the entry of tourists was prohibited. Gulls were also fed a synthetic feed with multiple nutrients after the closure but were fed fattier foods (e.g., bread, biscuits, and sausage) before the pandemic. These changes in feed provisioning might have affected their wintering movements near HGD. We expected that black-headed gulls would undergo an adaptive shift in their home range sizes and distribution following the closure of HGD. To test this hypothesis, we tracked the movements of black-headed gulls wintering at Dianchi Lake by satellite tracking and analyzed the home range size and distribution of gulls, as well as the relationship between behavioral changes and the distribution and amount of food during the COVID-19 lockdown in 2020.
Dianchi Lake (24°40′–25°02’N, 102°37′–102°48′E) is located on the Yunnan–Guizhou Plateau in southwestern China (Mao et al., 2019; Figure 1); it has an area of 308.6 km2 and an average elevation of 1,888 m a.s.l. (Wang et al., 2021). The lake is nearly semicircular, the shoreline is approximately 150 km in length, and the lake is 40.4 km long with an average width of 7.0 km (Wang et al., 2021). The climate is subtropical, with a mean temperature of 14.7°C, an average annual precipitation of 797–1,007 mm, and 227 frost-free days per year (Yang et al., 2010). Over 40,000 black-headed gulls forage at Dianchi Lake and nearby parks in Kunming City in the winter every year. Large amounts of food such as bread, biscuits, and sausage are provided by tourists and urban residents (Feng and Liang, 2020).
A total of 23 and 68 gulls were captured at Dianchi Lake in November 2018 and March 2019, respectively. During each sampling event, we captured gulls at the center of Dianchi Lake by boat. Gulls were captured by holding food in one hand and catching them quickly with the other hand. We tagged each gull with a GPS transmitter (YH–GTG0306, Hangzhou Yuehai Technology Ltd., China) using the backpack method (Nagendran et al., 1994; Supplementary Figures S1, S2). Each transmitter weighed 5–7 g, and the Teflon harness weighed approximately 4 g, which is equivalent to 2.6–3.2% of the gull’s average body mass (342.09 ± 4.42 g, range 290–444 g, n = 68). Permission to capture black-headed gulls was granted by the Forestry and Grassland Bureau of Yunnan Province, China [No. 33, KunLin Protection (2018)].
The solar-powered transmitters were programmed to record a GPS position every 2 h, and data were transferred via the China Mobile Communication System. The data, which were decoded and downloaded through the web client, included time, longitude, latitude, and location accuracy. The accuracy of the GPS transmitters was categorized into five classes: A (±5 m), B (±10 m), C (±20 m), D (± 0 m), and invalid data. Only data in classes A, B, and C were used in analyses. Signal interruption and recovery were assumed to occur when the signals of transmitters were interrupted for periods of days to months at one location but then recovered elsewhere after the interruption. Gulls were assumed to have died or the transmitters were assumed to have fallen off if the transmitters gave persistent signals for days or months at a fixed location and the temperature of the equipment was abnormal.
According to the date when the gulls arrived at and departed from the wintering ground, the two consecutive wintering periods for the gulls were defined as “Nov. 2018–Apr. 2019” and “Oct. 2019–Apr. 2020.” The HGD closure date “Jan. 27, 2020” was used as the cut-off date for the wintering period “Oct. 2019–Apr. 2020.” The period from November 2019 to the closure date of HGD was defined as the “Scenic Opening Period in 2020” (SOP2020); the period from the closure date of HGD to the last day that the gull was present on the wintering ground was defined as the “Scenic Closing Period in 2020” (SCP2020). Similarly, “Jan. 27, 2019” was used as the cut-off date for the wintering period “Nov. 2018–Apr. 2019,” which was used as the control group, and this period was divided into two stages: the “Scenic Opening Period in 2019” (SOP2019) and the “Scenic Closing Period in 2019” (SCP2019).
HGD is a popular tourist site, where gulls are fed large volumes of food. The spatial distribution of gulls at different distances from HGD may be affected by food provisioning. To analyze the effect of differences in the magnitude of human interventions on the spatial distribution of the gulls, the “Multiple Ring Buffer” tool was used in ArcGIS10.7 (ESRI, Redlands, CA, United States) to create a multi-ring buffer centered on HGD. Given that the linear distance between HGD to the west bank of Dianchi Lake (where gulls occur) is approximately 2 km, we used HGD as the center, and the different buffer zones were divided into equal intervals of 300 m (0–300 m, 300–600 m, and 600–900 m), with the exception of the fourth buffer zone (900–2000 m), which was larger to ensure that all possible locations of the gulls within the study site were captured.
Dianchi is a eutrophic lake; although the overall water quality has improved in recent years, eutrophication still occurs in certain parts of the lake (Li et al., 2019; Wang et al., 2020, 2021). The distribution and amount of aquatic organisms such as fish and shrimp are related to eutrophication, and serious eutrophication can result in water quality deterioration and decreases in the abundance of aquatic organisms (Zhao et al., 2005; Ismael, 2012; Landsberg et al., 2020). Eutrophication also results in algal blooms, and the extent of eutrophication can be estimated using the Normalized Difference Vegetation Index (NDVI) and the coverage ratio of algal blooms (Hu et al., 2021). To characterize the distribution and amount of natural food in different buffer zones in the wintering periods “Nov. 2018–Apr. 2019” and “Oct. 2019–Apr. 2020,” Landsat8 OLI images were downloaded from Geospatial Data Cloud, and remote sensing images of Kunming City with cloud cover less than 10% on January 31, 2019, February 16, 2019, January 18, 2020, and February 19, 2020, were used (Geospatial Data Cloud, 2022). The “Radiometric Calibration” and “FLAASH Atmospheric Correction” tools were used in ENVI 5.2 (Exelis VIS, NY, United States) to eliminate the effect of the atmosphere and obtain the reflectance of ground objects.
We imported the preprocessed images into ArcGIS10.7 and used the vector data of the buffer zone and the “Extract by Mask” tool to obtain the images of the different buffer zones. The NDVI of different buffer zones was calculated using the “Raster Calculator” tool and converted into vector data using the “Raster to Point” tool to obtain the number of locations distributed within a 30 × 30 m grid in each buffer and the corresponding NDVI values (0–300 m: 896 samples; 300–600 m: 1303 samples; 600–900 m: 1,615 samples; and 900–2000 m: 5,227 samples). Each buffer zone was divided into “Algal blooms” and “No algal blooms” using the “Reclassification” tool according to NDVI ≥ −0.1, which has previously been used as a criterion to infer the presence of algal blooms (Xie et al., 2010). The area of algal blooms and the total area of each buffer zone were calculated using the “Field Calculator” tool. The coverage ratio of algal blooms in each buffer zone was calculated as the area of algal blooms in each buffer zone divided by the total area of each buffer zone.
Tourists mainly feed gulls at HGD during the day; therefore, location data for SOP2019, SCP2019, SOP2020, and SCP2020 during the daytime were used to analyze the home range size and distribution of gulls. Dawn and twilight data for Kunming City were obtained from a Chinese website with publicly available data (Chinese convenient inquiry website for the masses, 2021). We used an intermediate time for each month (based on all data over each month) to indicate representative dawn and twilight hours for each month. We then defined day and night cycles for each month (Supplementary Table S1).
The kernel density estimation (KDE; Calenge, 2011) method in R (version 3.3, ‘adehabitatHR’ package) was used to analyze the home range sizes (90% KDE) of the gulls in the wintering period. To analyze the spatial distribution of the gulls near the HGD, the “Spatial Join” tool was used to obtain the number of locations in each buffer zone (SOP2019: 403 samples; SCP2019: 247 samples; SOP2020: 665 samples; and SCP2020: 812 samples). A total of 200 samples were randomly selected from SOP2019 and SCP2019, and 500 samples were randomly selected from SOP2020 and SCP2020 using the “Random case sample” tool in SPSS Statistics 26.0 (IBM Inc., Armonk, NY, United States) to determine whether the gulls were randomly distributed in the buffer zone. Next, we counted the number of gull locations in each buffer zone and calculated the ratio of gull locations in each buffer zone to the total number of locations to remove the effect of differences in the number of locations between individuals; we also determined the area of each buffer zone and calculated the ratio of the area of each buffer zone to the total area of the buffer zone (i.e., all buffer zones combined) to remove the effect of differences in the area of each buffer zone. Finally, the spatial distribution of the gulls near HGD was characterized using the Spatial Distribution Index (SDI) according to the following formula: number of locations in buffer zone i/the total number of locations)/(area of buffer zone i/total area of buffer zone).
Finally, to characterize the spatial distribution of the gulls among the different buffer zones, we simulated the spatial distribution of gulls assuming a total of 1,000 locations across all buffer zones. The number of locations in buffer zone i was determined using the following formula: [SDI × (area of buffer zone i/total area of buffer zone)]/1,000 (Supplementary Table S2). The “Create Random Points” tool in ArcGIS10.7 was used to create random points, and the “Kernel Density” tool was used to create heat maps on the basis of random points in buffer zone i.
Long-term tracking of the movements of gulls is a major challenge because of gull mortality as well as the vulnerability of the transmitters to falling off, which results in signal interruption. Satellite data included in analyses met the three following criteria: (i) data for the wintering period were complete; (ii) gulls had returned to Dianchi Lake in the winters of 2018 and 2019; and (iii) the number of locations in each wintering stage was at least 30 for gulls used in home range size analyses (Supplementary Table S3). Thirty individuals met the above criteria. In addition, three individuals tracked in 2018 successfully returned to Dianchi Lake and then departed successfully for 2 consecutive years. Data on these three individuals during the wintering period in the second year were also used for the analysis of the wintering period “Oct. 2019–Apr. 2020.” Data in location classes A, B, and C were used, which corresponded to an accuracy of <10 m, and a velocity of <3 km/h was used to calculate the home range (Jia et al., 2020) and spatial distribution. A total of 4,279 and 4,434 GPS locations were used to analyze the home range and spatial distribution of the gulls, respectively.
A Levene’s test and Kolmogorov–Smirnov test was used to test the homogeneity of variance and normality of the data separately. Given that home range size (90%KED) and NDVI data were normal and variance uniform, differences in NDVI of the four buffer zones were tested using one-way analysis of variance (ANOVA) and least significant difference (LSD) t-tests. Differences in the wintering behaviors of gulls in SOP2019, SCP2019, SOP2020, and SCP2020 could be caused by changes in the provisioning method and vary among wintering stages (early, middle, and later periods) and years. To detect the effects of different provisioning methods on the wintering behavior of gulls, the effect of the interaction between year and wintering stage on the home range sizes of gulls was tested using two-factor ANOVA, then post hos comparison using LSD t-tests. Given that SDI data were not normal, differences in the SDI of the gulls in different stages were tested using non-parametric Mann–Whitney U-tests, and differences in the SDI of the four buffer zones were tested using k-independent samples Kruskal–Wallis tests in SPSS Statistics 26.0 (IBM Inc., Armonk, NY, United States). Data were presented as the mean ± standard error (SE).
Different years had significant effect on the home range sizes of the gulls (F1,58 = 8.19, p = 0.01). Different stages and the interaction between wintering stage and year had no significant effect on that (Stages: F1,58 = 3.01, p = 0.09; Interaction: F1,58 = 0.75, p = 0.39). The home range sizes of the gulls were significantly smaller in SCP2020 (73.23 ± 8.83 km2) than in SOP2019 (188.33 ± 32.70 km2; p = 0.001), SCP2019 (174.29 ± 42.80 km2; p = 0.01), and SOP2020 (139.83 ± 24.22 km2; p = 0.03; Figures 2, 3).
Figure 2. Wintering home range sizes (90%KDE) of black-headed gulls in different stages (SOP2019, SCP2019, COP2020, and SCP2020) at Dianchi Lake, Kunming City, Yunnan Province, southwestern China. Different letters (a, b) indicate significant differences in home range sizes among stages (p ≤ 0.05), and the same letters indicate no differen ces (p > 0.05).
Figure 3. Wintering home range sizes (90%KDE) of black-headed gulls at different stages in Dianchi Lake, Kunming City, Yunnan Province, southwestern China. (A-SOP2019, n = 11; B-SCP2019, n = 11; C-SOP2020, n = 20; D-SCP2020, n = 20).
During the wintering period “Nov. 2018–Apr. 2019”, gulls were more common in the 600–900 m buffer zone (Z18 = −2.70, p = 0.01) and less common in the 0–300 m buffer zone (Z18 = −2.41, p = 0.02) in SCP2019 compared with SOP2019 (Table 1; Figure 4). The SDI of the gulls in the 300–600 m (Z18 = −0.19, p = 0.85) and 900–2000 m (Z18 = −0.23, p = 0.82) buffer zones did not significantly differ between SOP2019 and SCP2019 (Table 1; Figure 4).
Table 1. SDI, NDVI, and coverage ratio of algal blooms in different buffer zones at Dianchi Lake, Kunming City, Yunnan Province, southwestern China.
Figure 4. Spatial distribution of black-headed gulls in different buffer zones during four stages at Dianchi Lake, Kunming City, Yunnan Province, southwestern China (A-SOP2019; B-SCP2019; C-SOP2020; D-SCP2020).
During the wintering period “Oct. 2019–Apr. 2020”, gulls were more common in the 0–300 m buffer zone (Z41 = −2.03, p = 0.04) and less common in the 900–2000 m buffer zone (Z41 = −2.10, p = 0.04) in SCP2020 compared with SOP2020 (Table 1; Figure 4). The SDI of the gulls in the 300–600 m (Z41 = −0.55, p = 0.58) and 600–900 m (Z41 = −1.70, p = 0.09) buffer zones did not significantly differ between SOP2020 and SCP2020 (Table 1; Figure 4).
The NDVI of algal blooms in the four buffer zones significantly differed in both SCP2019 (F3,9,037 = 62.15, p < 0.001) and SCP2020 (F3,9,037 = 135.85, p < 0.001). The NDVI of the 300–600 m and 600–900 m buffer zones was lower than that of the 0–300 m and 900–2000 m buffer zones in SCP2019 and SCP2020; NDVI was lowest in the 600–900 m buffer zone (Table 1). The same patterns among the four buffer zones were observed in the algal bloom coverage ratio data (Table 1). Eutrophication was thus stronger at the edge of Caohai than at the center of HGD in both SCP2019 and SCP2020, and eutrophication was lowest in the 600–900 m buffer zone.
Human activities have pervasive effects on the distributions of animals (Morrison et al., 2007; Molinos et al., 2016). The gulls at HGD prefer to forage on easily accessible human-provided food; thus, their distribution might be affected by the location and density of tourists (Li and Liu, 2012; Scott et al., 2015). In our study, the home ranges and spatial distribution of black-headed gulls were altered in response to changes in food provisioning caused by the pandemic-induced anthropause, which indicated that gulls changed their behavior during the COVID-19 lockdown.
The home range sizes of gulls decreased significantly following the closure of HGD in 2020, and the most pronounced decreases were observed from Waihai and Cuihu to Caohai near HGD; these reductions might be related to the anthropause caused by the COVID-19 lockdown. At the FPM site, which is located at the central platform area of HGD, approximately 300 kg of synthetic feed with multiple nutrients was provided to the gulls daily to meet their energy demands during the closure period in 2020 (Xinhua news agency, 2020; Supplementary Table S4). Under FPM, gulls needed to wait to receive food near HGD, and this resulted in reductions in home range sizes.
These findings contrast with the results of previous research on Torresian crows and rock pigeons, which both prefer to forage on easily accessible human-provided food (Gilby et al., 2020; Soh et al., 2021). After crows and pigeons stopped receiving food from humans during the COVID-19 lockdown, they fled their previous habitats, expanded their home ranges, and spent more time foraging. Our findings revealed that black-headed gulls preferred to forage on easily accessible human-provided food similar to Torresian crows and rock pigeons, but changes in their behavior following the COVID-19 lockdown differed. This might be explained by differences in the magnitude of human interventions. The gulls mainly occur in an urban wetland park, and the food provisioning method was altered after the closure of HGD; by contrast, the crows and pigeons mainly occur in urban settlements and were unable to obtain food following the suspension of human activities, which forced them to travel further to find food and meet their energy requirements. We predict that a complete cessation in FPM during the closure of HGD in 2020 would have forced the gulls to expand their home ranges and spend more time foraging in a manner similar to that documented in rock pigeons and Torresian crows.
During the wintering period “Nov. 2018–Apr. 2019” when HGD was open to tourists, the daily energy demand of the gulls was met via SPM and natural food sources. Black-headed gulls are long-distance migratory birds and select habitats that maximize their intake of nutrients to ensure a successful spring migration (Ushine et al., 2017; Gan et al., 2019). Therefore, with the exception of foods rich in fat provided by tourists (e.g., bread and biscuits), the gulls preferred natural foods rich in proteins and vitamins to meet their nutritional needs during SCP2019. We speculated that natural foods such as fish and shrimp were less abundant given the greater level of eutrophication in the 0–300 m buffer zone near HGD, and natural foods were more abundant in the 600–900 m buffer zone because of the weaker level of eutrophication; consequently, the gulls were less common in the 0–300 m buffer zone and more common in the 600–900 m buffer zone in SCP2019.
The gulls were more common in the 0–300 m buffer zone and less common in the 900–2000 m buffer zone in SCP2020 compared with SOP2020, which was different from the pattern observed in SCP2019, and these differences are likely associated with changes in the food provisioning method and the type of food provided. Before the closure of HGD, gulls were provided fatty foods at various scattered points; after the closure of HGD, gulls were provided synthetic feed with multiple nutrients, which could serve as a replacement for fish and shrimp as main food sources before spring migration (Kunming Information Port, 2016). Furthermore, the relatively high spatial and temporal predictability of synthetic feed, coupled with the ease with which it can be acquired, compared with natural food sources, can enhance the body condition and fitness of gulls and provide key energy reserves for gulls before making their spring migration (Bartumeus et al., 2010; Cortes-Avizanda et al., 2012; Oro et al., 2013).
The distance to the site with synthetic feed was long in the 900–2000 m buffer zone, and the amount of natural foods such as fish and shrimp was lower in this zone because of the higher level of eutrophication; in the other three buffer zones, synthetic feed and natural food sources are available to gulls. Therefore, we speculated that gulls could obtain food quickly in the 0–300 m buffer zone because the distance to the food provisioning site was shorter; in the 300–600 m and 600–900 m buffer zones, the gulls could conveniently obtain synthetic feed as well as natural food, which is consistent with the conclusions of the trade-off between maximizing energy intake and minimizing energy expenditure (Schaefer and Schaefer, 2006; Thiel et al., 2007; Wang et al., 2012).
Anthropogenic food subsidies can improve individual fitness and promote increases in the population size of opportunistic species, which may affect communities, food webs, and ecosystems by altering processes such as competition, predator–prey interactions, and nutrient transfer between biotopes and ecosystems (Oro et al., 2013). Therefore, we speculated that changes in the distribution and provisioning of human-provided food caused by the anthropause not only have a direct effect on the home range and spatial distribution of gulls but also might have indirect effects on the population sizes of gulls, biological communities, and ecosystems.
Although we did not document the amount of natural food resources in Dianchi Lake, we analyzed eutrophication levels, which provide an indirect measure of the amount and distribution of natural resources such as fish and shrimp, given that Dianchi Lake has been a eutrophic lake since the 1980s (Guo et al., 2013). We also used wintering period data for 1 year before the COVID-19 outbreak as a control group. Differences in the wintering behaviors of gulls at different years and stages (early, middle, and later periods) were determined by evaluating the effect of the year × stage interaction on the home range sizes of gulls. This allowed us to isolate the effect of food provisioning method on the wintering behavior of gulls.
We studied plasticity in the wintering behavior of black-headed gulls, including their home range size and distribution in different buffers, in two consecutive wintering periods, which coincided with an anthropause caused by the COVID-19 pandemic. Gulls were able to promptly adapt to changes in food provisioning method; the home range sizes of gulls at Dianchi Lake decreased significantly during this period, and the number of gulls in the region located furthest from the HGD was significantly reduced in 2020, which might be related to the abundance of natural food sources. These behavioral changes support the hypothesis that the gulls promptly altered their behavior in response to the unpredictable changes in the distribution and provisioning of food caused by the anthropause, and this resulted in substantial changes in their home ranges and distribution at our study site. Our findings provide new insights into how behavioral plasticity mediates adaptation to changes in human activities in birds.
The original contributions presented in the study are included in the article/Supplementary material, further inquiries can be directed to the corresponding author.
HX: manuscript preparing and revising and field work performing. XZ and ZY: field work performing and writing contributions. RJ and LC: field work performing. GZ: research conceiving and designing, field work performing, and writing contributions. All authors read the text, provided comments, suggestions and corrections, and approved the final version. All authors contributed to the article and approved the submitted version.
This work was supported by the National Key Research and Development Program of China (No. 2019YFA0607103) and Program of National Forestry and Grassland Administration (Nos. 213023721203 and 202999922001).
The authors declare that the research was conducted in the absence of any commercial or financial relationships that could be construed as a potential conflict of interest.
All claims expressed in this article are solely those of the authors and do not necessarily represent those of their affiliated organizations, or those of the publisher, the editors and the reviewers. Any product that may be evaluated in this article, or claim that may be made by its manufacturer, is not guaranteed or endorsed by the publisher.
The Supplementary material for this article can be found online at: https://www.frontiersin.org/articles/10.3389/fevo.2022.1013244/full#supplementary-material
SOP2019, Scenic Opening Period in 2019; SCP2019, Scenic Closing Period in 2019; SOP2020, Scenic Opening Period in 2020; SCP2020, Scenic Closing Period in 2020; SPM, Scattered provisioning; FPM, Fixed-point provisioning; HGD, Haigeng Dam; NDVI, Normalized Difference Vegetation Index; SDI, Spatial Distribution Index.
Bartumeus, F., Giuggioli, L., Louzao, M., Bretagnolle, V., Oro, D., and Levin, S. A. (2010). Fishery discards impact on seabird movement patterns at regional scales. Curr. Biol. 20, 215–222. doi: 10.1016/j.cub.2009.11.073
Bates, A. E., Primack, R. B., Moraga, P., and Duarte, C. M. (2020). COVID-19 pandemic and associated lockdown as a “global human confinement experiment,” to investigate biodiversity conservation. Biol. Conserv. 248:108665. doi: 10.1016/j.biocon.2020.108665
Calenge, C. (2011). Home range estimation in R: the adehabitatHR package. Available at: https://cran.r-project.org/web/packages/adehabitatHR/vignettes/adehabitatHR.pdf (Accessed August 11, 2021).
Chinese convenient inquiry website for the masses. (2021). https://richurimo.bmcx.com/kunming__richurimo/ [Accessed November 20, 2021].
Corlett, R. T., Primack, R. B., Devictor, V., Maas, B., Goswami, V. R., Bates, A. E., et al. (2020). Impacts of the coronavirus pandemic on biodiversity conservation. Biol. Conserv. 246:108571. doi: 10.1016/j.biocon.2020.108571
Cortes-Avizanda, A., Jovani, R., Carrete, M., and Donazar, J. A. (2012). Resource unpredictability promotes species diversity and coexistence in an avian scavenger guild: a fifield experiment. Ecology 93, 2570–2579. doi: 10.2307/41739615
Feng, C. Z., and Liang, W. (2020). Behavioral responses of black-headed gulls (Chroicocephalus ridibundus) to artificial provisioning in China. Glob. Ecol. Conserv. 21:e00873. doi: 10.1016/j.gecco.2019.e00873
Gan, L., Cao, X. L., Wang, Y., Wang, Y. Q., Jiang, H. Y., Lan, R. T., et al. (2019). Carriage and potential long distance transmission of listeria monocytogenes by migratory black-headed gulls in Dianchi Lake, Kunming. Emerg. Microbes Infec. 8, 1195–1204. doi: 10.1080/22221751.2019.1647764
Geospatial Data Cloud. (2022). http://www.gscloud.cn/search [Accessed Aril 15, 2022].
Gilby, B. L., Henderson, C. J., Olds, A. D., Ballantyne, J. A., Bingham, E. L., Elliott, B. B., et al. (2020). Potentially negative ecological consequences of animal redistribution on beaches during COVID-19 lockdown. Biol. Conserv. 253:108926. doi: 10.1016/j.biocon.2020.108926
Guo, H. C., Wang, X. Y., and Yi, X. (2013). Study on eutrophication control strategy based on the succession of water ecosystem in the Dianchi Lake. Geogr. Res. 6, 998–1006. doi: 10.11821/yj2013060003
Hayashi, T., Yaegashi, N., and Konishi, I. (2020). Impact of COVID-19: Decrease in the number of fledging barn swallow chicks in Tokyo. bioRxiv. doi: 10.1101/2020.11.16.380899
Hu, L., Gan, S., Yuan, X. P., Li, R. B., and Bi, R. (2021). Study on the spatial distribution characteristics of cyanobacteria bloom in Dianchi Lake based on GF-5. Laser Infrared 51, 237–243. doi: 10.3969/j.issn.1001-5078.2021.02.019
Ismael, A. A. (2012). Benthic bloom of cyanobacteria associated with fish mortality in Alexandria waters. Egypt. J. Aquat. Res. 38, 241–247. doi: 10.1016/j.ejar.2013.01.001
Jia, R., Liu, D. P., Lu, J., and Zhang, G. G. (2020). Wetland destruction on migration routes threatens a breeding population of the endangered black-faced spoonbill (Platalea minor). Glob. Ecol. Conserv. 23:e01105. doi: 10.1016/j.gecco.2020.e01105
Karnon, J. (2020). A simple decision analysis of a mandatory lockdown response to the COVID-19 pandemic. Appl. Health Econ. Health Policy 18, 329–331. doi: 10.1007/s40258-020-00581-w
Kunming Information Port. (2016). Gulls love bread but not synthetic feed? Experts of bird association: synthetic feed is the most scientific formula. Available at: https://m.kunming.cn/news/c/2016-01-10/4143567.shtml#/ [Accessed October 25, 2021].
Landsberg, J. H., Hendrickson, J., Tabuchi, M., Kiryu, Y., Williams, B. J., and Tomlinson, M. C. (2020). A large-scale sustained fish kill in the St. Johns River, Florida: a complex consequence of cyanobacteria blooms. Harmful Algae 92:101771. doi: 10.1016/j.hal.2020.101771
Li, X. L., Janssen, A. B. G., de Klein, J. J. M., Kroeze, C., Strokal, M., Ma, L., et al. (2019). Modeling nutrients in Lake Dianchi (China) and its watershed. Agric. Water Manag. 212, 48–59. doi: 10.1016/j.agwat.2018.08.023
Li, S. Z., and Liu, Z. (2012). Surveys on population dynamics and provisioning of wintering black-headed gulls (Larus ridibundus) in Kunming Cuihu Park. Chin. J. Wild. 33, 146–148. doi: 10.3969/j.issn.1000-0127.2012.03.010
Manenti, R., Mori, E., Canio, V. D., Mercurio, S., Picone, M., Caffi, M., et al. (2020). The good, the bad and the ugly of COVID-19 lockdown effects on wildlife conservation: insights from the first European locked down country. Biol. Conserv. 249:108728. doi: 10.1016/j.biocon.2020.108728
Mao, Q., Liao, C. C., Wu, Z. L., Guan, W. B., Yang, W. D., Tang, Y. Q., et al. (2019). Effects of land cover pattern along urban-rural gradient on bird diversity in wetlands. Diversity 11:86. doi: 10.3390/d11060086
Molinos, J. G., Halpern, B. S., Schoeman, D. S., Brown, C. J., Kiessling, W., Moore, P. J., et al. (2016). Climate velocity and the future global redistribution of marine biodiversity. Nat. Clim. Chang. 6, 83–88. doi: 10.1038/nclimate2769
Morrison, J. C., Sechrest, W., Dinerstein, E., Wilcove, D. S., and Lamoreux, J. F. (2007). Persistence of large mammal faunas as indicators of global human impacts. J. Mammal. 88, 1363–1380. doi: 10.1644/06-MAMM-A-124R2.1
Nagendran, M., Higuchi, H., and Sorokin, A.G. (1994). A harnessing technique to deploy transmitters on cranes. Wild Bird Society of Japan, Tokyo, pp. 57–60.
Oro, D., Genovart, M., Tavecchia, G., Fowler, M., and Abraín, A. M. (2013). Ecological and evolutionary implications of food subsidies from humans. Eco. Lett. 16, 1501–1514. doi: 10.1111/ELE.12187
Rutz, C., Loretto, M. C., Bates, A. E., Davidson, S. C., Duarte, C. M., Jetz, W., et al. (2020). COVID-19 lockdown allows researchers to quantify the effects of human activity on wildlife. Nat. Ecol. Evol. 4, 1156–1159. doi: 10.1038/s41559-020-1237-z
Schaefer, H. M., and Schaefer, V. (2006). The fruits of selectivity: how birds forage on Goupia glabra fruits of different ripeness. J. Ornithol. 147, 638–643. doi: 10.1007/s10336-006-0089-x
Schrimpf, M. B., Brisay, P. G. D., Johnston, A., Smith, A. C., Sánchez-Jasso, J., Robinson, B. G., et al. (2021). Reduced human activity during COVID-19 alters avian land use across North America. Sci. Adv. 7:eabf5073. doi: 10.1126/sciadv.abf5073
Scott, P., Duncan, P., and Green, J. A. (2015). Food preference of the black-headed gull (Chroicocephalus ridibundus) differs along a rural-urban gradient. Bird Study 62, 56–63. doi: 10.1080/00063657.2014.984655
Soh, M. C. K., Pang, R. Y. T., Ng, B. X. K., Lee, B. P. Y.-H., Loo, A. H. B., and Er, K. B. H. (2021). Restricted human activities shift the foraging strategies of feral pigeons (Columba livia) and three other commensal bird species. Biol. Conserv. 253:108927. doi: 10.1016/j.biocon.2020.108927
Thiel, D., Unger, C., Kéry, M., and Jenni, L. (2007). Selection of night roosts in winter by Capercaillie (Tetrao urogallus) in Central Europe. Wildl. Biol. 13, 73–86. doi: 10.2981/0909-6396(2007)13[73,SONRIW]2.0.CO
Ushine, N., Sato, T., Kato, T., and Hayama, S. I. (2017). Analysis of body mass changes in the black-headed gull (Larus ridibundus) during the winter. J. Vet. Med. Sci. 79, 1627–1632. doi: 10.1292/jvms.17-0099
Wang, J. H., Li, C., Xu, Y. P., Li, S. Y., Du, J. S., Han, Y. P., et al. (2021). Identifying major contributors to algal blooms in Lake Dianchi by analyzing river-lake water quality correlations in the watershed. J. Clean. Prod. 315:128144. doi: 10.1016/j.jclepro.2021.128144
Wang, J. H., Wang, Y. N., Dao, G. H., Du, J. S., Han, Y. P., and Hu, H. Y. (2020). Decade-long meteorological and water quality dynamics of northern Lake Dianchi and recommendations on algal bloom mitigation via key influencing factors identification. Ecol. Indic. 115:106425. doi: 10.1016/j.ecolind.2020.106425
Wang, J., Yun, F., Klaus, S., and Sun, Y. H. (2012). Winter foraging strategy of the Chinese grouse (Bonasa sewerzowi): ecological and physiological factors. J. Ornithol. 153, 257–264. doi: 10.1007/s10336-011-0717-y
Xie, G. Q., Li, M., Lu, W. K., Zhou, W. M., Yu, L. X., Li, F. R., et al. (2010). Spectral features, remote sensing identification and breaking-out meteorological conditions of algal bloom in Lake Dianchi. J. Lake Sci. 22, 327–336. doi: 10.18307/2010.0304
Xinhua news agency. (2020). The story of Kunming people and black-headed gulls in winter. Available at: https://baijiahao.baidu.com/s?id=1662584479198613167&wfr=spider&for=pc [Accessed October 25, 2021].
Yang, Y. H., Zhou, F., Guo, H. C., Sheng, H., Liu, H., Dao, X., et al. (2010). Analysis of spatial and temporal water pollution patterns in Lake Dianchi using multivariate statistical methods. Environ. Monit. Assess. 170, 407–416. doi: 10.1007/s10661-009-1242-9
Keywords: behavioral plasticity, black-headed gull, human provisioning, COVID-19 lockdown, satellite tracking
Citation: Xu H, Zhao X, Jia R, Chen L, Yang Z and Zhang G (2022) Behavioral plasticity mediates adaptation to changes in food provisioning following the COVID-19 lockdown in black-headed gulls (Larus ridibundus). Front. Ecol. Evol. 10:1013244. doi: 10.3389/fevo.2022.1013244
Received: 06 August 2022; Accepted: 12 October 2022;
Published: 31 October 2022.
Edited by:
Diana Hamilton, Mount Allison University, CanadaReviewed by:
Gregory J. Robertson, Environment and Climate Change Canada (ECCC), CanadaCopyright © 2022 Xu, Zhao, Jia, Chen, Yang and Zhang. This is an open-access article distributed under the terms of the Creative Commons Attribution License (CC BY). The use, distribution or reproduction in other forums is permitted, provided the original author(s) and the copyright owner(s) are credited and that the original publication in this journal is cited, in accordance with accepted academic practice. No use, distribution or reproduction is permitted which does not comply with these terms.
*Correspondence: Guogang Zhang, em03NjcyQDEyNi5jb20=
Disclaimer: All claims expressed in this article are solely those of the authors and do not necessarily represent those of their affiliated organizations, or those of the publisher, the editors and the reviewers. Any product that may be evaluated in this article or claim that may be made by its manufacturer is not guaranteed or endorsed by the publisher.
Research integrity at Frontiers
Learn more about the work of our research integrity team to safeguard the quality of each article we publish.