- CSIRO DATA61, Canberra, ACT, Australia
Citizen observations of the natural world are increasing in detail, growing in volume and increasingly being shared on web-based platforms for the purpose of sharing information and/or the crowd-sourcing of species identification. From a biosecurity perspective, such citizen data streams are important as they are responsible for the majority of post-border reports and most detections of exotic pest species of concern. The sharing of sightings amongst what are effectively communities of practice is a key driver of having the sighting of an exotic pest species recognized and reported. Whilst it is clear that the eyes, ears, cameras, and microphones of citizens are a major component of biosecurity surveillance, it is unclear what level of surveillance this provides in the prospective sense. As an example, what confidence does citizen science provide about “proof of absence” for exotic pests of concern? The taxonomy of surveillance used within the field of biosecurity would classify such citizen activities as contributing to “general surveillance,” for which non-detections are typically not recorded and methods of quantitative analysis are still under development. We argue that while not recorded, there is considerable information about citizens activities that routinely underpins peoples mental inference about the level of surveillance provided by citizen activities. Furthermore, we show that it is possible to make such inference from general surveillance transparent by describing and characterizing the activities that potentially generate sightings in a way that is amenable to quantitative analysis. In the context of evaluating surveillance provided by citizens for incursions of exotic vertebrates, we provide examples of citizen observations providing early warning and hence preventing the establishment of species from a range of animal groups. Historically, analysis of the power of general surveillance has been restricted to being conceptual, based on qualitative arguments. We provide this, but also provide a quantitative model framework and provide examples of how different forms of general surveillance data may be analyzed, particularly in supporting inference of eradication/extinction.
1. Introduction
Invasive exotic vertebrate pests are responsible for considerable environmental, amenity, and agricultural damage. Because of this, preventing the unintentional introduction of additional species, by the early detection of new introductions and hence hopefully cost-effective prevention of establishments is important (Hester and Cacho, 2017). The risk of introduction of exotic pests is typically diffuse, being spread across diverse points of possible entry, multiple modes of entry, and involving multitudes (tens of 1,000s) of possible taxa (see Caley and Cassey, 2022 and references therein). This makes targeted, structured surveillance programs impossible for all but a few high priority pests, typically trade related, and means that authorities charged with biosecurity responsibilities are heavily reliant on what is known as “general surveillance” as a means of detection of the introduction of exotic pests. Most of this general surveillance relates to activities underpinning sightings made by members of the public (“citizens”) undertaking a broad range of activities—often described as “science” but usually not in the true sense of the word (see Welvaert and Caley, 2016). The important role of general surveillance is best recognized in plant biosecurity, with the International Plant Protection Convention ISPM 6 report on “Guidelines for Surveillance” (FAO, 2018) defining general surveillance as “a process whereby information on particular pests which are of concern for an area is gathered from many sources.” The other recognized type of surveillance system is “Specific surveys” (FAO, 2018). General surveillance is further defined as providing a level of confidence that “the pest, if present, would have been detected and notified.” Detections by general surveillance forms a large proportion of all detections of exotic insects (Carnegie and Nahrung, 2019), plants (Epanchin-Niell et al., 2021), and unwanted biosecurity organisms in general (e.g., Beale et al., 2008; Froud et al., 2008; Pawson et al., 2020), but there has been little attention given to its role in detecting exotic vertebrate species.
As the name “general surveillance” suggests, the types of observations and observation processes made are, by their nature, very broad, and span the passive/active and unintentional/intentional dimensions of the reporting process (see Welvaert and Caley, 2016). General surveillance is considered as separate from surveillance arising from “Specific surveys” (see FAO, 2018), that involves structured searching (i.e., intentional and structured) for target pest species. Surveillance implicitly and explicitly undertaken by citizens that is not targeted, and is hence “general” is often described as “passive” surveillance (e.g., see Hester and Cacho, 2017). This is somewhat of a misnomer in that a citizen activity potentially leading to observations can be both quite active and intentional as an activity (e.g., a bird watcher actively seeking to record and share all the bird species detected at a specific location), but not targeted in the sense of seeking to detect (or check for the presence of) a particular exotic bird species of concern. It is clear, however, that the general surveillance “activity” is the key component that needs to be considered. There is a possibility, depending on the activity parameters, that the exotic species of concern would be detected if present.
While it is reasonably straightforward to list the organisms that have been detected by general surveillance, and demonstrate it's relative importance in biosecurity detections (e.g., estimated by Froud et al. 2008 as responsible for around half the detections of exotic organisms), it is more difficult to evaluate the power (surveillance sensitivity) that general surveillance provides. A particular challenge is a pervasive feature of general surveillance in that negative survey results (e.g., surveillance zeros) are rarely recorded, nor is the effort expended. Recording absences is an important aspect of any surveillance program but, by definition, it is not usually done systematically under general surveillance. Typically only positive reporting occurs, that is, people may report what they do see (a “sighting”), but will rarely if ever report on what is not sighted, unless explicitly requested to do so. Furthermore, efforts to get people to voluntarily do this are generally in vain. Within Australia for example, regarding general surveillance for vertebrate species, citizens are simply not going to record “No elephants, meerkats, or macaws were sighted” as the absence of such species is considered unremarkable. In practice there are tens (actually hundreds) of thousands of other theoretical species sighting possibilities should all possible vertebrate species be considered. Hence these data take the form of implicit (unrecorded) “zeros.” As such, it is difficult to apply traditional statistical approaches to estimating the value of these systems. Data analysts confronted with making inference based on general surveillance activities are typically slow to recognize and accept this, and invariably ask that general surveillance programs record whether the species they are interested in were present. They want the citizen activity to conform to their approach to data recording to underpin their methods for analysis. This almost invariably doesn't happen (due to the very nature of general surveillance), and has led to a widely held view that it is not possible to objectively discern the properties of a general surveillance system.
The notion that we cannot make quantitative statements arising from general surveillance (especially when a species is not reported) is at odds with accepted wisdom for the current distribution of much of the world's vertebrate biodiversity. For example, it is almost universally accepted (cryptozoology adherents aside) that there are no wild (free-living) striped skunks (Mephitis mephitis) in Australia, nor kangaroos (Macropus spp.) hopping across the Great Plains of the United States. This acceptance comes despite the fact that in neither country are there any structured surveys for either species providing targeted surveillance. If we accept that this inference from general surveillance holds for tens of thousands of vertebrate species, then we are recognizing that there is an underlying structure to this reasoning (mental reckoning) that arises from general surveillance activities. This becomes more important where inference from general surveillance is needed in less contrived situations. For example, assessing whether a pest eradication program has been successful, or assessing preemptively how sensitive general surveillance may be for a particular incursion threat (e.g., wild pigs Sus scrofa spreading into new areas of the United States).
Making useful inference from a general surveillance activity and the implicit zeros that it contains requires that the underlying observation process be described/captured in such a way that (1) the probability of a sighting given pest presence can be estimated, and (2) a reasoned estimate of the probability of the sighting being reported can be made. Note that “sighting” subsumes all recognized methods of detection (e.g., physical sample, verified photograph, and audio recording). The sighting model of Solow (1993) and the many that followed this seminal paper are essentially models using data from general surveillance activities in its simplest form (marginalized to a non-spatial annual probability of at least one sighting). Caley and Barry (2014) pointed out some of the limitations of this excessively simple assumption with respect to sighting probability and how it relates to population size.
In this paper we address the effectiveness of citizen observations in providing surveillance for exotic vertebrates, with emphasis on Australia. We first describe what is needed in terms of timely detection, locations of surveillance and coverage of taxa of interest. We then document examples of exotic pest incursions that have been detected by citizens as part of general surveillance activities, and make reasoned arguments as to how effective the citizen surveillance system may be. We then present an analytical framework describing how general surveillance can be used to make quantitative statements about whether pest populations are present, and if so at what level. This involves developing a taxonomy for describing general surveillance that focuses on characterizing the surveillance activity in a manner that can be incorporated into quantitative models.
2. Materials and methods
We begin in Section 3.1 by first characterizing citizen surveillance activity in terms of its coverage of the major vertebrate taxonomic groups and the spatial intensity of observations. Section 3.2 then provides illustrative examples of citizen detections and reporting of exotic vertebrate pest incursions. We then examine how the effectiveness of general surveillance activities can be evaluated: first by “reasoned argument” (Section 3.3) and then using more formal quantitative approaches (Section 3.4).
3. Results
3.1. Characterizing citizen surveillance activity
3.1.1. Taxonomic coverage of citizens
The taxonomic coverage of vertebrates (and hence potential exotic vertebrate pests) by citizens appears to be very good. On social media, for example, there are numerous Facebook groups that specialize in the identification of birds, reptiles, and amphibians (see Table 1 for examples). The groups are initiated entirely by and for citizens, and operate as Communities of Practice with various levels of complexity. A universal purpose of the groups is the crowd-sourcing of species identification. Most engagement by citizens with online platforms, such as sharing sightings and seeking identification of sightings, arises from an interest in natural history. Some engagement, however, arises out of necessity. For example, through their desire to have reptiles (snakes in particular) removed from their homes, the public often unintentionally bring taxonomic expertise to their sightings by calling on the services of wildlife rescue agencies (Shine and Koenig, 2001). Likewise the “Snake Identification Australia” Facebook group seeks to assist citizens in providing fast identifications of snakes in situations where the citizen may need to make a decision around personal safety.
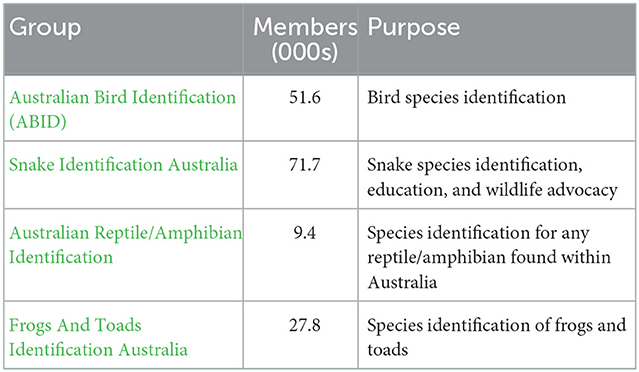
Table 1. Examples of Facebook Groups from Australia dedicated to the identification of vertebrates, including the number of members (as of October 2022), and their stated purpose.
Dedicated smartphone Apps and linked platforms cater to either a different form of identification (e.g., audio), or to citizens with different identification skills and motivations. For example, the FrogId App and platform taps into specialist knowledge from the Australian Museum to identify frogs predominantly from their calls (as submitted using the App). iNaturalist provides a general platform for sharing and crowd-sourcing the identification of all biota, and enables the user to keep and curate records of their sightings, create projects and join other projects of interest. Identification within iNaturalist is strongly predicated on the use of images—for an observation to be considered “research grade” it requires an image from which the community can agree on an identification. Identification within the ebird platform is heavily reliant on the observer, though all sightings are subject to automatic filters based on known distributions, with subsequent moderation of sightings that are flagged as unusual. Flagged sightings are not publicly visible until adequately supported during a review process with a moderator familiar with the location and species involved. Historically there were few if any images associated with eBird sightings but that is rapidly changing with advances in digital camera technology.
3.1.2. Species-dependent reporting probabilities
The propensity of a citizen to generate a general surveillance report of sighting is highly contextual. As a general rule, citizens are more likely to post sightings of species that (1) are unfamiliar to them (seeking identification), (2) they know to be rare, threatened or micro-endemic (and hence they are sharing a special occurrence), or (3) they consider to be physically interesting by being ornate, brightly colored, large, “cute” etc. (in which case they are sharing an experience they feel others may like also). In contrast, bird watchers contributing to platforms such as eBird are often interested in the diversity of their sightings (i.e., list length), hence they intentionally report on all species sighted, regardless of their physical features. For social media platforms (e.g., Twitter and TikTok), uploaded material will be heavily biased toward the “big, bold, bright, and beautiful.”
For the purpose of detecting an exotic vertebrate incursion, only one report of a sighting is required. For an incursion comprising of N individuals of a species, each with probability pi of being detected (i.e., sighted and reported), the probability of at least one detection is:
Under the often-used simplifying assumption that the pi's are all equal, Equation (1) simplifies to a form that we have termed the “1st law of biosecurity risk analysis”:
The formulation in Equation (2), with the combined sighting and detection probability for each individual assumed a constant, overly emphasizes the influence of the population size N on the probability of a report of a positive sighting. In reality, however, pi subsumes both the sighting probability and the probability of reporting given sighting(s), and may be highly variable. A single high pi will ensure a high probability of at least one detection from the population. The sighting probability will clearly vary, depending on the intersection between the general surveillance activity and the distribution of the N individuals. Likewise, the probability of reporting given sighting will vary with individual citizens and the nature of the species in question. Importantly when considering typical general surveillance activities undertaken by citizens, this report could either be from the original observer making a primary sighting, or by any of the individuals that the sighting has been shared with (including reshares). This sharing of sightings underpins the surveillance power of citizen observations, as it increases both the chances of an exotic species being identified, and the chances that at least one of the recipients of the sighting generates a report.
The exact form for the total number of sightings (including shares and reshares) and resulting reports is difficult to write down directly, as it involves the integration over several components some of which are random. To aid the understanding of how these components impact on the citizen surveillance sensitivity, we present a simplified representation. Let S be a random variable denoting the total number of sightings relating to an incursion that includes shares and reshares. Furthermore, if we make the simplifying assumption that the probability of any one of these sightings being reported is a constant (ρ), then:
The key feature to note in Equation (3) is that increasing the probability of having at least one sighting reported can be achieved by either increasing the reporting probability and/or increasing the number of sightings (either by primary observation or by crowd sharing). In reality the reporting probability will differ between sightings depending the individual observer. However, generally speaking, the sharing and crowd-sourcing nature of citizen surveillance activities ensures that although individual reporting probabilities are likely highly variable, and sometimes low, the underpinning mechanics of Equation (3) means that the probability of at least one detection increases inexorably, either due to an increase in the size of the invading population, and/or an increase in citizen sightings, and/or the number of individuals with whom the sighting is shared.
3.1.3. Spatial coverage
It is well-known that the locations where citizens generate their observations is strongly biased (Isaac and Pocock, 2015), based on factors such as where they live (e.g., their homes), where they can access (e.g., along public roads and publicly-accessible areas), and where they like to visit (e.g., nature parks, the coast, and green space) (e.g., Figure 1). Observations are often absent or uncommon from areas away from access trails, and within areas of residential housing (e.g., Figure 1). Sites of known high diversity may also be targeted (particularly within the birding community). There is bias in the temporal pattern of observations also, toward daytime observations and observations on weekends. From an exotic species detection perspective this weekday temporal bias is inconsequential, though the lack of night-time observations is potentially an issue for exotic species that are predominantly nocturnal.
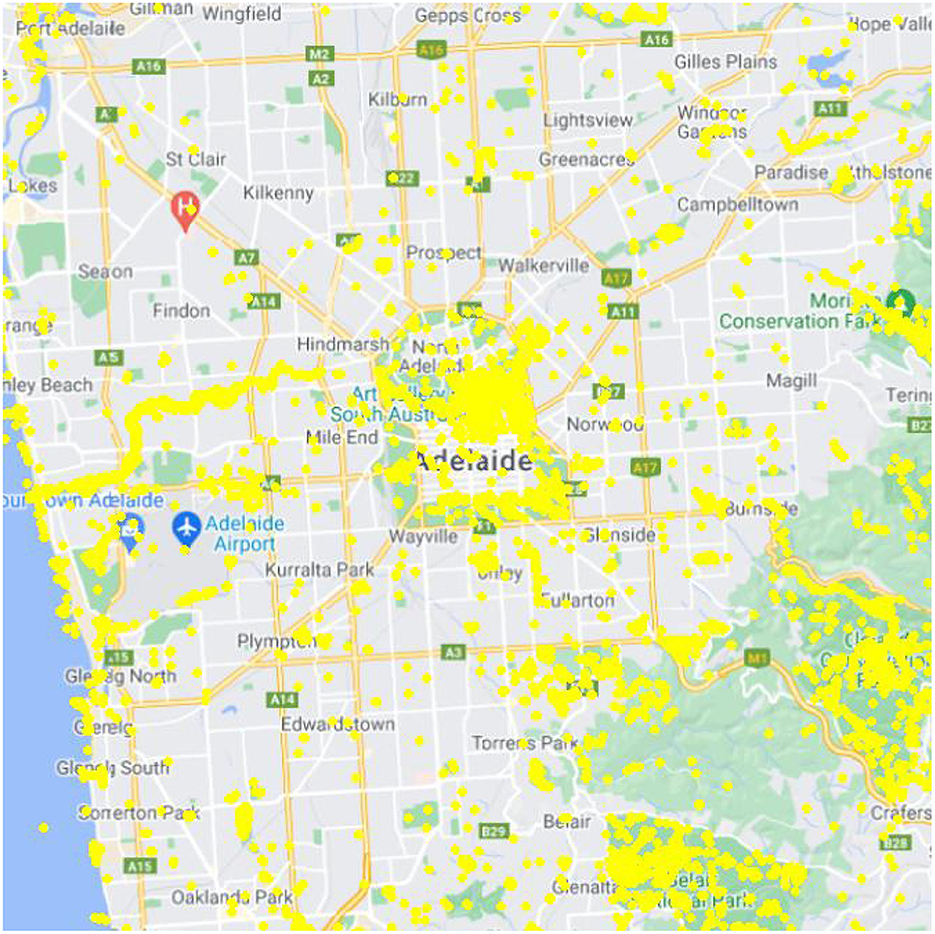
Figure 1. Distribution of citizen observations of birds centered on the city of Adelaide that were uploaded to the Atlas of Living Australia for the period 2016–2021.
As noted previously, a feature of all citizen reported information is that along with no record of what wasn't seen, there will typically be no record of the underlying activity that leads to sightings that underpin reports. This unrecorded information relates to all the locations (private yards, urban streets and parks, and reserves etc.), times and movement modes (e.g., sitting, walking, riding, and driving). As a consequence the spatial extent of the surveillance effort is typically greater than the sightings suggest. Methods to treat this problem in a quantitative manner are explored in Section 3.4.
3.2. Examples of citizen detections of exotic vertebrate pest incursions
What follows are examples of general surveillance activities of citizens that have led to the detection of exotic vertebrate pests.
3.2.1. Self-introduced exotic geese
Citizen surveillance through amateur bird watching detected and led to the eradication of an incursion of the exotic and likely invasive Canada goose (Branta canadensis) into New South Wales, Australia. Through the observations of a number of independent birdwatchers spanning a large stretch of coastline, the incursion of four birds was successfully detected, identified and reported as they moved between wetlands on the South Coast of New South Wales, prior to their successful elimination by authorities (see Boles et al., 2016). It is presumed that the birds had flown from New Zealand where they are an established pest, following deliberate introduction from North America last century. Previously, amateur birdwatchers had detected the entry of a lone individual (Carter, 2006). The subsequent incursion demonstrated this was not a one-off event.
3.2.2. Escaped pet exotic snakes
Escaped pets are the major source of vertebrate introductions, and a recent example of an escaped Boa imperator (native to Central and South America) in Sydney, Australia is a case in point. The escaped animal was sighted by a neighbor, who realizing that it was unusual, posted an image onto an Australian Facebook group (“Snake Identification Australia”) dedicated to snake identification. Through one of the Facebook Groups moderators a reptile relocation expert was mobilized, authorities notified, and the illegally-kept reptile ultimately impounded—all in a matter of hours. This particular Facebook group is one of many that leverages on crowd-sourced species identification, including moderation by members considered to have sufficient identification skills. Interestingly, the group appears to deal effectively with fake images (e.g., photos from overseas purporting to be from within Australia), with members undertaking reverse image searches to identify fake sightings. The moderators of groups are also, however, by their own admission struggling to cope with the increasing workload that providing such an effective (and free) service requires. Group membership as of October 2022 was in the order or 72,000 and increasing rapidly.
3.2.3. Aquatic and semi-aquatic pests
Citizens have been responsible for most of the sightings of red-eared slider turtles (Trachemys scripta elegans), but that hasn't prevented them becoming established in various locations in eastern Australia (Burgin, 2007). Detection is necessary but not always sufficient to prevent exotic vertebrates establishing. Indeed, for reasons of low detection probability and capture rates, eradicating populations of red-eared slider turtles is challenging (García-Díaz et al., 2017). Furthermore, in the case of red-eared sliders, the sheer volume of introductions arising from escapes and releases from captivity could, in combination with the lack of effective response, have made preventing establishment near impossible.
The incursion of the European newt (Lissotriton vulgaris) into Australia was first reported by a member of the public (general surveillance again), though subsequent surveys revealed is was already well established (Tingley et al., 2015). It is a similar story for the establishment of Siamese fighting fish (Betta splendens) in the Adelaide River floodplains of Northern Australia, where the first confirmed sighting was by a member of the public (Hammer et al., 2019), though the population was by this time already well established.
3.3. Evaluating general surveillance activities by reasoned argument
The previous examples have highlighted retrospective examples of general surveillance detecting exotic vertebrate species—often in time to prevent establishment. The obvious question that arises is how effective will citizen surveillance be in the prospective sense? For example, returning to the Canada goose example, the population in New Zealand is currently uncontrolled, so we reasonably expect there will be further incursions of Canada geese into Australia originating from New Zealand. Can we rely on the general surveillance provided by the bird watching community as being adequate for our needs, or do we need a targeted “Canada goose surveillance program”? It appears reasonable that having previously been effective enough to detect an incursion within a sufficient time frame, there is no reason that citizen surveillance couldn't be effective again. It also seems reasonable to extend such apparent surveillance sensitivity to exotic bird species more generally for which conformation and color patterning are distinctive.
Given the high number of exotic vertebrate species that could potentially be introduced if they had an introduction pathway, it makes sense to restrict the evaluation of citizen surveillance to those species with a non-trivial probability of mounting an incursion. Other than birds which are clearly capable of self-introduction in some cases, exotic vertebrates will typically need to be inadvertently transported as stowaways/hitch hikers or be imported deliberately by humans. The deliberate introductions of vertebrates, either by acclimatization societies, or for attempted biological control has now largely ceased, and future exotic vertebrate incursions (excluding birds) will originate mainly from captive individuals (Lockwood et al., 2019). Indeed, research has shown that humans are the biggest direct source of alien invasions (e.g., from escaped pets—kept legally or otherwise) (Vall-llosera and Cassey, 2017; Lockwood et al., 2019). This has implications for the effectiveness of citizens as surveillance agents for invasive pests, in that they are central to the process of both introduction, and hence (potentially) detection, so having citizen surveillance effort biased toward where the most humans are is not a bad thing. In fact, from a risk-based stratification of surveillance effort it would be considered near-optimal.
It is worthwhile considering how timely citizen surveillance needs to be. The need for surveillance to provide “early detection” has essentially become a mantra in the field of biosecurity surveillance, but “early detection” is rarely defined. What is actually needed is “timely” detection. That is, detection needs to occur so that options such as eradication or containment remain logistically and economically feasible. The interplay of range of factors (e.g., immigration rate, detectability, vulnerability to control, benefit-cost, sociopolitical) determine the feasibility of eradication, but the first criterion listed by Bomford and O'Brien (1995) is that the rate of removal (through control actions) exceeds the rate of increase over all densities. In a worst-case scenario (for which we want to be prepared) where we expect an invading population to be growing, the relevant measure is the intrinsic rate of population increase (rm) (Caughley and Birch, 1971). For different species, what will be timely will vary enormously. For large, conspicuous vertebrates with a low rm, the acceptable delay from incursion to detection could be in the order of years to decades (e.g., in the case of elephants), whereas for species that are cryptic and hence hard to detect and remove (e.g., snakes) or have a very high rm, a successful eradication campaign would require the incursion to be detected very early with few individuals involved.
3.4. Evaluating general surveillance activities quantitatively
3.4.1. General
We can conceptualize a model for general surveillance as comprising components of entry, population, activity and detections. An exotic pest, with an approach rate that can lead to the entry of the pest; a resultant pest population arising from dispersal mechanisms and dynamics, and citizen surveillance activity methods that result in detections (or lack thereof). In general Bayesian statistical terms, we can write down an expression for the distribution of the size of population given surveillance detections (noting that detections can also implicitly be a series of “zero” surveillance outcomes) as follows, where the square parentheses ([]) indicates some appropriate probability distribution.
A key feature of Equation (4) is that the prior belief of entry, as captured by the distribution [entry], can effectively reduce the likelihood of a population being present to zero, regardless of survey activity and detections (even if none have been made). For example, if it is believed that the unseen entry of a particular exotic species is effectively zero (e.g., striped skunks in Australia), then it follows that the belief in the probability of a population being present, regardless of the surveillance effort/activity, is also effectively zero. If however, entry is considered possible, then making inference on the population size on the basis of general surveillance also requires quantitative treatment of all the components in Equation (4). That is, modeling the population size given entry, in conjunction with the detections given population and surveillance activity, and the underlying surveillance activity and prior belief of entry. This represents the “Entry, Establishment, and Spread” (EES) component of an invasive species risk analysis (Andersen et al., 2004).
3.4.2. Quantifying general surveillance example—Fully characterizing activities
Authorities were rightfully concerned when rumors surfaced in the late 1990s of the introduction of the red fox (Vulpes vulpes) to Australia's island state Tasmania, as the small mammal fauna would be highly vulnerable to predation by foxes (Johnson, 2006). The inference of an already widespread and expanding population was based on the extraction of red fox mitochondrial DNA from predator scats assumed to have been collected from Tasmania (Sarre et al., 2013). The reasoned argument from a substantial proportion of citizens, however, was that no such widespread population existed. Following an initial cluster of confirmed specimens of actual foxes (a single individual purportedly shot by a hunter, and three individuals purportedly killed by vehicle collisions), there had been no further specimen reports considered reliable (though see Marks et al., 2014), despite the DNA evidence indicating a persisting and spreading population.
The Tasmanian fox incursion data necessitated applying Equation (4) in an explicitly spatial and temporal manner. The space-time distribution of entry was constructed from news reports of the rumored deliberate introduction, and the corroborated sightings of a fox jumping from a container boat. The general surveillance activity “vehicle collision” was defined by the distribution of roads, and “hunter kill” was defined by the hunting distribution based on the demonstrated high intensity of hunting in Tasmania with detection efficacy informed from the literature of surveying for foxes where they are known to occur. All calculations and associated dynamics were abstracted to a 5 km by 5 km raster with a one year time step. These steps are similar those undertaken when constructing a Bayesian hierarchical model to describe the dynamics of a partially observed population process, however standard likelihood methods become impossible to use as the complexity (of what is still a reasonably simple observation and population process) makes the likelihood intractable. The problem of the intractable likelihood was solved by using Approximate Bayesian Computational methods (Toni et al., 2009).
The resulting inference from what was then considered verifiable data arising from general surveillance (i.e., lack of confirmed road-kills and hunter kills) provided compelling inference that the postulated widespread distribution of red foxes was very unlikely to be true, and in fact the most likely population size was zero (Caley et al., 2015a)—a result that has so far stood the test of time. It should be noted, however, that the uncertain provenance of the carcasses observed and reported by citizens (see Marks et al., 2014) highlights the risk of false-positive citizen observations. A high rate of false positive visual sightings is a given—it is intrinsic to human nature. However, deliberate fabrication of observations is more difficult to detect, and potentially more costly if it triggers an expensive response, as may well have been the case for the postulated fox incursion into Tasmania (Anon, 2017).
Caley et al. (2017) note other forms of general surveillance activity that add further to the inference. For example, searching the prey remains (“orts”) associated with wedge-tailed eagle (Aquila audax) nests in Tasmania (for which the locations are in-the-main known to authorities). Like their northern hemisphere cousins the golden eagle (Aquila chrysaetos), wedge-tailed eagles regularly kill red foxes and red fox remains are consistently found at non-trivial percentages in wedge-tailed eagle diets in a wide range of habitats wherever foxes are present (Glen et al., 2017). Codifying the surveillance “activity” of wedge-tailed eagles would require quantifying the foraging radius of an eagle pair, and calibrating the predation rate from the numerous locations on the Australian mainland where the eagles and foxes coexist. Yet other forms of general surveillance activities also could be added for which calibration would be easier, including extensive camera trapping studies of Tasmanian fauna (e.g., Thalmann et al., 2014) and statewide spotlight count transects undertaken since 1975 (Lazenby et al., 2018). Neither has recorded a fox sighting, though it is safe to assume that if a sighting were made it would be reported. Adding additional forms of observation to the general surveillance inference need not increase the computational burden of such a model if each activity can be essentially marginalized to an activity layer with the same spatio-temporal scale.
3.4.3. Quantifying general surveillance sensitivity example—Use of covariance
A way of quantifying the observation sensitivity (for the possible population sizes that haven't been observed) is via statistical expectation after conditioning on a variable that the population of species in question is known to co-vary with. The nature of the covariance need not be linear. For example, Caley et al. (2017) showed how the numerical response relationship between a predator (in this case the red fox) and it's prey (lagomorphs) could be used to quantitatively infer the population size of the predator from a location where there had been no sightings (see Section 3.4.2). The data in question were airport runway strikes collected by the Australian Transport Safety Bureau (http://data.atsb.gov.au/detaileddata). These are typical industry data arising from general surveillance, whereby there is a clear process of recording all positive “sightings”—that is, all incidents of animals being struck on runways and their identification if possible—but nothing else (excluding near misses). For these types of activities, the assumption that if a species has not been recorded then it has not been detected by the surveillance activity, is by no means an onerous one.
de Groot et al. (2022) explore using knowledge of the set of species that a general surveillance activity is able to detect, in combination with the reporting motivations of individual observers, to infer “constrained” pseudo-absences. They do this by conditioning citizen observations on (1) Observers that had previously reported sightings of the target species, and (2) Locations where these subset of observers had observed at least one other species expected to be observable using the surveillance activity in question. The first conditioning step ensures that observer possesses the necessary identification skills, while the second step confirms that the surveillance activity has occurred at the location in question. de Groot et al. (2022) use this approach of generating pseudo-absences to feed into a species distribution for an invasive species, however it can also be used to quantify the distribution of the surveillance activity in Equation (4).
3.4.4. Quantifying general surveillance sensitivity example—Direct empirical
In an ideal situation for the purposes of calibration, the distribution of the population of interest is known, as is the distribution of the general surveillance activity. This enables the sensitivity of the surveillance activity to be calculated directly using empirical methods. This requires independent ground “truth” data of the population of interest to evaluate citizen sightings against. That is, the probability distribution of detections given population size and surveillance activity—that is, the component (detections|population, activity) in Equation (4)—can be calibrated. This approach of direct calibration clearly can't be applied to exotic species that aren't yet present where the surveillance activity is occurring. Again, statistical conditioning can be useful to overcome this problem. It requires features (not necessarily only physical ones) that influence the combined sighting and reporting probability of endemic species that can be used to develop a model-based estimate of sensitivity for exotic species of interest. For example, Caley et al. (2020) used a case-control design in combination with physical covariate features to estimate the reporting probabilities of citizen surveillance. The resulting model was then used to estimate the probability of an incursion being reported by general surveillance for exotic species of interest, conditional on covariates that predict observation and reporting, and the spatial extent of the population incursion.
4. Discussion
We have argued that understanding and using the general surveillance information that is inherent in citizen reporting requires characterizing the activities that will generate reports from positive sightings (if any). Once the activities are adequately described, the surveillance information they provide, including that arising from the implicit zeros/absences, can be calibrated by a range of methods and hence quantitatively evaluated. This is important, as there appears to be both a lack of scientific understanding and accepted methodology for dealing with the implicit absences within data generated by general surveillance activities. This is exemplified by the polemic surrounding the true number of foxes associated with the putative incursions into Tasmania (Section 3.4.2), with a reviewer of Caley et al. (2015b) reasoning that it seems “incredulous that only four dead foxes would contain sufficient information to support the sweeping conclusions presented in this paper [that the size of the fox population was most likely zero or failing that, very small].” This notion that information only comes from detections (positive sightings) is a recurring theme. An implicit contradiction within this line of reasoning is that to have increased confidence that there are no individuals remaining (in this case foxes) you need to have first detected numerous individuals. Furthermore, by extension, if you have not detected any individuals, then you have no information on whether any individuals are present. Such arguments are often presented in conjunction with the maxim that “Absence of evidence is not evidence of absence.” We have argued that there is indeed inference to be had from the “absence of evidence,” providing the citizen activity capable of generating positive sightings can be described in a manner that enables quantitative treatment, and that a non-trivial reporting probability of the sighting can be reasonably assumed.
The case studies we present for the detection of vertebrate pests entering Australia support the assumption that there is a high reporting probability of many exotic vertebrate pest species given a positive sighting. The manner in which sightings by citizens are typically shared within a community of practice increases the probability of a sighting being reported—the probability of the sighting remaining unreported declines exponentially with the increase in the number of people that view the sighting. The internet plays an important role in facilitating the sharing of sightings, and for the bird and mammal animal groups it appears that citizen-based general surveillance provides sensitivity adequate to prevent incursions. This assumes, of course, that an effective incursion response is mounted—timely surveillance can only lead to useful outcomes if acted on. For species from more cryptic groups, such as reptiles, and those inhabiting aquatic habitats (e.g., amphibians and fish) it appears that although citizens are again providing the bulk of the surveillance effort, the detections may not always be timely enough to prevent establishment. For example, community sightings comprise the biggest source of reports of corn snakes (Elaphe guttata) in Australia, but it may be too late as the increasing pattern of detections suggests they have established free-living populations that are increasing (McFadden et al., 2017; Mo and Mo, 2021). Such cryptic species present particular challenges to eradicate, and when individuals are found, the uncertainty as to whether the individual is free-living or simply an escaped specimen introduces uncertainty to the decision-making process. Caley and Cassey (2022) argue that citizen surveillance of aquatic habitats is hampered by the obvious challenges of access and visibility (including the difficulty of obtaining images). Where surveillance is needed in such environments, there is an obvious potential role for alternative detection methods such as eDNA (e.g., Hinlo et al., 2017).
Our findings have application to evaluating the contributions of citizen reporting to the detection of invasive exotic plants and insect pests. Like invasive vertebrates, many invasive plants (particularly fodder species) were deliberately introduced by agencies such as governments (see Cook and Dias, 2006), though it is now largely ornamental plants from within people's gardens that are the source of invasive plants (Groves et al., 2005; Caley et al., 2008). The situation with insects differs in that it is not clear by which pathway exotic insect pests are being introduced (Caley et al., 2015a), and reporting probabilities are strongly influenced by aesthetic features (Caley et al., 2020). For both plants and insects, the use of dedicated platforms for sharing sightings and seeking identification is extensive, however the number of species involved is considerably greater. This may mean an increased number of shares within the community of practice would be required before a sighting would reach a user with the taxonomic skills to identify the species as exotic.
We note that increased surveillance sensitivity arising from citizen activities may require more decision making around the sighting information generated. The adage “Be careful measuring what you cannot manage” has relevance. For some species, the desired outcome may well be no management action (c.f. eradication, containment, managed spread), especially in regard to the treatment of vagrants and self-introductions in the case of birds. Others may require a response to prevent establishment. The decision to eliminate the Canada geese detected in south-eastern Australia, on the assumption they had traveled from New Zealand where they are introduced (as opposed to self-introducing all the way across the Pacific Ocean from North America) points to a gray area in responding to detections of alien species. Can we distinguish a “natural” self-introduction from one that is not part of the “natural” order? For example, the cattle egret (Bubulcus ibis) is now widespread and endemic across much of Australia and New Zealand after self-introducing during the mid-twentieth century as part of a bigger worldwide expansion, no doubt facilitated by the spread of pastoral systems for domestic cattle (Bos spp.). The extensive network of bird watchers across Australia is now regularly reporting on sightings for species classified as vagrants. Which ones should be worried about (if any) and why?
The contributions of citizens to building knowledge of the worlds biota is being increasingly recognized (Chandler et al., 2017). Here we have highlighted the important contribution that citizens play, through their contribution to general surveillance activities, in identifying vertebrate species that are out of place (i.e., exotic and potentially invasive). Furthermore, we have described and illustrated methods for making quantitative statements on the basis of such general surveillance activities.
Data availability statement
The original contributions presented in the study are included in the article/supplementary material, further inquiries can be directed to the corresponding author.
Author contributions
PC and SB contributed to conception and design of the study. PC wrote the first draft of the manuscript. Both authors contributed to manuscript revision, read, and approved the submitted version.
Funding
This work was funded by the Centre for Invasive Species Solutions, Project No. P01-I-003.
Acknowledgments
The authors acknowledge the First Nation's people of the land on which they live and work—the Ngunnawal and Ngambri people of the Canberra region. Petra Kuhnert was involved in useful discussions around conceptualizing models for general surveillance. Sarah Cole assisted with editing. The manuscript was improved by the comments of two reviewers.
Conflict of interest
The authors declare that the research was conducted in the absence of any commercial or financial relationships that could be construed as a potential conflict of interest.
Publisher's note
All claims expressed in this article are solely those of the authors and do not necessarily represent those of their affiliated organizations, or those of the publisher, the editors and the reviewers. Any product that may be evaluated in this article, or claim that may be made by its manufacturer, is not guaranteed or endorsed by the publisher.
References
Andersen, M. C., Adams, H., Hope, B., and Powell, M. (2004). Risk assessment for invasive species. Risk Anal. 24, 787–793. doi: 10.1111/j.0272-4332.2004.00478.x
Anon (2017). An Investigation into Allegations of Misconduct in the Fox Free Taskforce and Fox Eradication Program. Report of the integrity commission no. 4 of 2017. Integrity Commission (of Tasmania), Tasmania.
Beale, R., Fairbrother, J., Inglis, A., and Trebeck, D. (2008). One Biosecurity: A Working Partnership. Canberra: Quarantine and Biosecurity Review Panel, Department of Agriculture.
Boles, W. E., Tsang, L. R., and Sladek, J. (2016). First specimens of free-flying Canada Geese Branta canadensis from Australia. Aust. Field Ornithol. 33, 237–239. doi: 10.20938/afo33148150
Bomford, M., and O'Brien, P. (1995). Eradication or control for Australias vertebrate pests. Wildl. Soc. Bull. 23, 249–255.
Burgin, S. (2007). “Status report on Trachemys scripta elegans: pet terrapin or Australia's pest turtle?” in Pest or Guest: The Zoology of Overabundance, eds D. Lunney, P. Eby, P. Hutchings, and S. Burgin (Sydney, NSW: Royal Zoological Society of New South Wales), 1–7. doi: 10.7882/FS.2007.003
Caley, P., and Barry, S. C. (2014). Quantifying extinction probabilities from sighting records: Inference and uncertainties. PLoS ONE 9, e95857. doi: 10.1371/journal.pone.0095857
Caley, P., and Cassey, P. (2022). Do we need to mine social media data to detect exotic vertebrate pest introductions? Wildl. Res. (in press). doi: 10.1071/WR22116
Caley, P., Groves, R. H., and Barker, R. (2008). Estimating the invasion success of introduced plants. Divers. Distrib. 14, 196–203. doi: 10.1111/j.1472-4642.2007.00440.x
Caley, P., Hosack, G. R., and Barry, S. C. (2017). Making inference from wildlife collision data: inferring predator absence from prey strikes. PeerJ 5, e3014. doi: 10.7717/peerj.3014
Caley, P., Ingram, R., and De Barro, P. (2015a). Entry of exotic insects into Australia: does border interception count match incursion risk? Biol. Invas. 17, 1087–1094. doi: 10.1007/s10530-014-0777-z
Caley, P., Ramsey, D. S. L., and Barry, S. C. (2015b). Inferring the distribution and demography of an invasive species from sighting data: the red fox incursion into Tasmania. PLoS ONE 10, e0116631. doi: 10.1371/journal.pone.0116631
Caley, P., Welvaert, M., and Barry, S. C. (2020). Crowd surveillance: estimating citizen science reporting probabilities for insects of biosecurity concern. J. Pest Sci. 93, 543–550. doi: 10.1007/s10340-019-01115-7
Carnegie, A. J., and Nahrung, H. F. (2019). Post-border forest biosecurity in Australia: response to recent exotic detections, current surveillance and ongoing needs. Forests 10, 336. doi: 10.3390/f10040336
Carter, M. (2006). Canada goose Branta canadensis: first Australian mainland occurrence. Aust. Field Ornithol. 23, 15-20. Available online at https://search.informit.org/doi/10.3316/informit.307065087358167
Caughley, G., and Birch, L. C. (1971). Rate of increase. J. Wildl. Manage. 35, 658–663. doi: 10.2307/3799769
Chandler, M., See, L., Copas, K., Bonde, A. M., Lapez, B. C., Danielsen, F., et al. (2017). Contribution of citizen science towards international biodiversity monitoring. Biol. Conserv. 213, 280–294. doi: 10.1016/j.biocon.2016.09.004
Cook, G. D., and Dias, L. (2006). It was no accident: deliberate plant introductions by Australian government agencies during the 20th century. Aust. J. Bot. 54, 601–625. doi: 10.1071/BT05157
de Groot, M., Ogris, N., van der Meij, M., and Pocock, M. J. O. (2022). Where to search: the use of opportunistic data for the detection of an invasive forest pest. Biol. Invas. 24, 3523–3537. doi: 10.1007/s10530-022-02857-9
Epanchin-Niell, R., Thompson, A. L., and Treakle, T. (2021). Public contributions to early detection of new invasive pests. Conserv. Sci. Pract. 3, e422. doi: 10.1111/csp2.422
FAO (2018). ISPM 6: Surveillance. Technical report, Secretariat of the International Plant Protection Convention (IPPC).
Froud, K. J., Oliver, T. M., Bingham, P. C., Flynn, A. R., and Rowswell, N. J. (2008). Passive Surveillance of New Exotic Pests and Diseases in New Zealand. Surveillance for Biosecurity: Pre-border to Pest Management. Paihia, New Zealand: New Zealand Plant Protection Society.
García-Díaz, P., Ramsey, D. S. L., Woolnough, A. P., Franch, M., Llorente, G. A., et al. (2017). Challenges in confirming eradication success of invasive red-eared sliders. Biol. Invas. 19, 2739–2750. doi: 10.1007/s10530-017-1480-7
Glen, A. S., Pech, R. P., Davey, C., and Molsher, R. L. (2017). Raptors vs aliens: can indigenous birds of prey help control invasive predators? NZ J. Zool. 44, 99–107. doi: 10.1080/03014223.2016.1261910
Groves, R. H., Boden, R., and Lonsdale, W. M. (2005). Jumping the Garden Fence. Report. World Wildlife Fund, Australia.
Hammer, M. P., Skarlatos Simoes, M. N., Needham, E. W., Wilson, D. N., Barton, M. A., and Lonza, D. (2019). Establishment of Siamese Fighting Fish on the Adelaide River floodplain: the first serious invasive fish in the Northern Territory, Australia. Biol. Invas. 21, 2269–2279. doi: 10.1007/s10530-019-01981-3
Hester, S. M., and Cacho, O. J. (2017). The contribution of passive surveillance to invasive species management. Biol. Invas. 19, 737–748. doi: 10.1007/s10530-016-1362-4
Hinlo, R., Furlan, E., Suitor, L., and Gleeson, D. (2017). Environmental DNA monitoring and management of invasive fish: comparison of eDNA and fyke netting. Manage. Biol. Invas. 8, 89–100. doi: 10.3391/mbi.2017.8.1.09
Isaac, N. J. B., and Pocock, M. J. O. (2015). Bias and information in biological records. Biol. J. Linnean Soc. 115, 522–531. doi: 10.1111/bij.12532
Johnson, C. (2006). Australians Mammal Extinctions: A 50,000-year History. Cambridge University Press, New York.
Lazenby, B. T., Tobler, M. W., Brown, W. E., Hawkins, C. E., Hocking, G. J., Hume, F., et al. (2018). Density trends and demographic signals uncover the long-term impact of transmissible cancer in Tasmanian devils. J. Appl. Ecol. 55, 1368–1379. doi: 10.1111/1365-2664.13088
Lockwood, J. L., Welbourne, D. J., Romagosa, C. M., Cassey, P., Mandrak, N. E., Strecker, A., et al. (2019). When pets become pests: the role of the exotic pet trade in producing invasive vertebrate animals. Front. Ecol. Environ. 17, 323–330. doi: 10.1002/fee.2059
Marks, C. A., Obendorf, D., Pereira, F., Edwards, I., and Hall, G. P. (2014). Opportunistically acquired evidence is unsuitable data to model fox (Vulpes vulpes) distribution in Tasmania. Wildl. Soc. Bull. 38, 757–766. doi: 10.1002/wsb.448
McFadden, M. S., Topham, P., and Harlow, P. S. (2017). A ticking time bomb: is the illegal pet trade a pathway for the establishment of corn snake (Elaphe guttata) populations in Australia? Aust. Zool. 38, 499–504. doi: 10.7882/AZ.2017.006
Mo, M., and Mo, E. (2021). An amelanistic Red Cornsnake (Pantherophis guttatus) as a possible identity for an unusual road-killed snake discovered in Sydney, Australia. Reptiles Amphibians 28, 480–482. doi: 10.17161/randa.v28i3.15874
Pawson, S. M., Sullivan, J. J., and Grant, A. (2020). Expanding general surveillance of invasive species by integrating citizens as both observers and identifiers. J. Pest Sci. 93, 1155–1166. doi: 10.1007/s10340-020-01259-x
Sarre, S. D., MacDonald, A. J., Barclay, C., Saunders, G. R., and Ramsey, D. S. L. (2013). Foxes are now widespread in Tasmania: DNA detection defines the distribution of this rare but invasive carnivore. J. Appl. Ecol. 50, 459–468. doi: 10.1111/1365-2664.12011
Shine, R., and Koenig, J. (2001). Snakes in the garden: an analysis of reptiles “rescued” by community-based wildlife carers. Biol. Conserv. 102, 271–283. doi: 10.1016/S0006-3207(01)00102-1
Solow, A. R. (1993). Inferring extinction from sighting data. Ecology 74, 962–964. doi: 10.2307/1940821
Thalmann, S., Wise, P., and Huxtable, S. (2014). “Sentinel camera traps monitor the emergence of infectious disease in Tasmanian devils (Sarcophilus harrisii),” in Camera Trapping: Wildlife Management and Research (Melbourne: CSIRO Publishing), 27–33.
Tingley, R., Weeks, A. R., Smart, A. S., van Rooyen, A. R., Woolnough, A. P., and McCarthy, M. A. (2015). European newts establish in Australia, marking the arrival of a new amphibian order. Biol. Invas. 17, 31–37. doi: 10.1007/s10530-014-0716-z
Toni, T., Welch, D., Strelkowa, N., Ipsen, A., and Stumpf, M. P. H. (2009). Approximate Bayesian computation scheme for parameter inference and model selection in dynamical systems. J. R. Soc. Interf. 6, 187–202. doi: 10.1098/rsif.2008.0172
Vall-llosera, M., and Cassey, P. (2017). Leaky doors: private captivity as a prominent source of bird introductions in Australia. PLoS ONE 12, e0172851. doi: 10.1371/journal.pone.0172851
Keywords: general surveillance, citizen science, statistics, social media, crowd sourcing
Citation: Caley P and Barry SC (2022) The effectiveness of citizen surveillance for detecting exotic vertebrates. Front. Ecol. Evol. 10:1012198. doi: 10.3389/fevo.2022.1012198
Received: 05 August 2022; Accepted: 08 December 2022;
Published: 22 December 2022.
Edited by:
Oscar Cacho, University of New England, AustraliaReviewed by:
Steve Pawson, University of Canterbury, New ZealandDarius Tubelis, Federal University Rural Semi-Arid, Brazil
Copyright © 2022 Caley and Barry. This is an open-access article distributed under the terms of the Creative Commons Attribution License (CC BY). The use, distribution or reproduction in other forums is permitted, provided the original author(s) and the copyright owner(s) are credited and that the original publication in this journal is cited, in accordance with accepted academic practice. No use, distribution or reproduction is permitted which does not comply with these terms.
*Correspondence: Peter Caley, peter.caley@csiro.au