- 1Department of Biology, Centre for Animals on the Move, Advanced Facility for Avian Research, University of Western Ontario, London, ON, Canada
- 2Environment and Climate Change Canada, Saskatoon, SK, Canada
Aerial insectivorous songbirds such as swallows and martins have declined substantially in North America in recent decades. Aquatic-emergent insects provide more beneficial omega-3 fatty acids than terrestrial insects, and thus, diet quality is expected to vary among aerial insectivores with differential access to aquatic-emergent insects. We compared the stable hydrogen isotope (δ2H) values of feathers and bulk blood plasma fatty acids of nestling purple martins (Progne subis), tree swallows (Tachycineta bicolor), and barn swallows (Hirundo rustica), at lakeshore and inland sites near Lake Erie, Ontario, Canada. We found that diet quality differed between inland and lakeshore nesting habitats, but differences depended on species. Overall, purple martin and tree swallow nestlings had lower feather δ2H values, indicating a more aquatic-emergent diet, and lakeshore populations of both species had higher omega-3 fatty acid levels in their blood plasma compared to inland populations. Conversely, higher plasma levels of omega-6 fatty acids were found in inland birds. Tree swallows have a low omega-3 conversion efficiency from precursor substrates and so depend on aquatic subsidies to fulfill their nutritional needs. We suggest this may also be the case with purple martins. Barn swallows had the most positive feather δ2H values, regardless of proximity to the lakeshore, indicating a more terrestrial diet. However, barn swallow nestlings had consistently higher plasma omega-3 docosahexaenoic acid (DHA) regardless of nesting location, suggesting that barn swallows can efficiently convert omega-3 precursors into their beneficial elongated fatty acid chains. Our study indicates the benefit of combining plasma fatty acid compositional analyses with bulk feather δ2H values to decipher interspecific differences in adaptations to availability of aquatic-emergent insects.
Introduction
Aerial insectivorous songbirds have declined more than any other avian guild in North America in recent decades (Smith et al., 2015; Sauer et al., 2017; North American Bird Conservation Initiative Canada, 2019; Rosenberg et al., 2019). As these species all forage on insects in the air column, insect population declines have been considered among the likely factors influencing these trends (Hallmann et al., 2017; Møller, 2019; Sánchez-Bayo and Wyckhuys, 2019; Stepanian et al., 2020). However, the role of insect abundance as a limiting factor for aerial insectivore populations remains an open question (Imlay et al., 2017; McClenaghan et al., 2019a; Spiller and Dettmers, 2019). Instead, a growing literature on the effects of diet quality versus quantity on aerial insectivore health suggests a need to identify if diet quality is a contributor to population declines (Twining et al., 2016, 2018b, 2021; Spiller and Dettmers, 2019).
Fatty acids (FA) are nutrients essential to many physiological processes. Omega-3 eicosapentaenoic acid (EPA) and docosahexaenoic acid (DHA) are long-chain polyunsaturated fatty acids (PUFA) known to promote brain development and provide anti-inflammatory properties in humans (Simopoulos, 2011). In contrast to omega-3 FAs, omega-6 arachidonic acid (ARA) competes with EPA in cell membranes and has pro-inflammatory properties in humans, yet ARA is necessary in organ development and gene expression (Simopoulos, 2011). A low omega-6/omega-3 ratio is thought to have positive benefits, while a high ratio may cause susceptibility to inflammatory responses (Roy et al., 2008; Andersson et al., 2015; Isaksson et al., 2017). Insect lipids have high levels of saturated FAs, but also unsaturated FA, the most abundant being oleic acid and linoleic (LA) (Rumpold and Schlüter, 2013; Sosa and Fogliano, 2017). Many terrestrial insects can also be high in ARA (Rumpold and Schlüter, 2013). Depending on the study, LA and alpha-linolenic acid (ALA) can be high in terrestrial and aquatic insects (Rumpold and Schlüter, 2013; Hixson et al., 2015; Twining et al., 2018a, 2019, 2021). More importantly for insectivorous birds, these studies show that aquatic insects are a rich source of omega-3 FAs such as EPA and DHA compared to terrestrial insects (Rumpold and Schlüter, 2013; Hixson et al., 2015; Twining et al., 2018a, 2019, 2021; Shipley et al., 2022). Tree swallows (TRES; Tachycineta bicolor) with higher omega-3 FAs benefit from associated increases in nestling health, growth, and fledging success compared to nestlings provisioned with more terrestrial insects or a lower omega-3 diet (Twining et al., 2016, 2018b). Furthermore, a high EPA and DHA diet fed to nestling eastern phoebe (Sayornis phoebe) increased mass growth 2–3 times more than a high ALA diet (Twining et al., 2019).
Habitat can determine diet composition in birds, as the spatial distribution of prey quality and nutrient content varies across landscapes. Swallows forage close (~1 km) to their nests during the breeding season (Waugh, 1979; McCarty and Winkler, 1999; Evans et al., 2007). Breeding purple martins (PUMA; Progne subis) also forage in proximity (~3 km) to their nest (Lalla, 2022). Thus, birds nesting in one location may differ in diet composition and quality from birds nesting in another nearby location. For example, bank swallows (Riparia riparia) nesting at lakeshore sites in southern Ontario fed on a more aquatic-emergent insect diet represented by low feather stable hydrogen isotope values (δ2H) and had higher plasma EPA than inland birds (Génier et al., 2021). Similarly, spider, reptile, and amphibian diets have been shown to isotopically shift from an aquatic-derived diet to a more terrestrial diet as they became more distant from the riparian zone (Walters et al., 2008; Twining et al., 2021).
Songbirds can compensate for variation in diet quality by internally synthesizing some FAs. This has been demonstrated in nestling tree swallows and blue tits (Cyanistes caeruleus) that are able to convert the 18-carbon omega-3 precursor ALA to its longer EPA and DHA chains (Twining et al., 2018a, 2021). However, this conversion efficiency in nestling tree swallows was not adequate to meet their high omega-3 PUFA demand, whereas blue tits were not limited (Twining et al., 2018a, 2021). A balance likely exists between conversion efficiency, the physiological costs of such conversion and prey quality, where the ability to efficiently convert omega-3 precursors to their long-chain PUFA may alleviate the dependency on aquatic-emergent insects.
It is still unknown how different aerial insectivores occurring in sympatry compare in their diet composition within or between riparian and inland nesting habitats. In general, we expect closely-related species to segregate diets (Orłowski and Karg, 2013; Collins, 2015; Bumelis et al., 2021) depending on relative abundance of prey and density of avian consumers. Furthermore, purple martins, tree swallows, and barn swallows forage in different aerial strata (Dreelin et al., 2018). Swallows feed generally on aerial insects, but there is evidence of dietary segregation occurring among them (Bumelis et al., 2021). Unfortunately, determining origins of prey and their nutritional quality, especially in the context of multispecies communities, is extremely challenging.
In combination with tissue FA profiles that provide insight into diet quality, measurements of naturally occurring ratios of stable hydrogen isotopes (δ2H) in animal tissues provide a strong tool to differentiate between aquatic and terrestrial dietary sources (Voigt et al., 2015; Vander Zanden et al., 2016; Génier et al., 2021). In general, terrestrial plants tend to be enriched in 2H (i.e., have higher δ2H) due to local evaporative conditions compared to aquatic plants that occur in water (Fogel and Cifuentes, 1993; Doucett et al., 2007). Unless a water source is prone to seasonal evaporation, aquatic consumers are expected to have lower δ2H values than terrestrial consumers and the use of this isotope appears more useful for this purpose than stable carbon isotopes (δ13C, Doucett et al., 2007; Voigt et al., 2015; Génier et al., 2021). Combining FA and δ2H measurements in sympatric aerial insectivores, we sought to understand diet composition and the relative use of aquatic-emergent insects by these birds across a broad landscape in southern Ontario.
Following on from our earlier work on bank swallows, we compared plasma FA profiles and feather δ2H among nestling barn swallows (BARS; Hirundo rustica), tree swallows and purple martins nesting inland and in close proximity to Lake Erie, Ontario. All species have broad diets but previous research elsewhere and in our study area confirms that barn swallows have the most terrestrial diet compared to tree swallows and purple martins (McCarty and Winkler, 1999; Helms et al., 2016; McClenaghan et al., 2019b; Bumelis et al., 2021). We used the eastern bluebird (EABL; Sialia sialis) as a “control” species as it feeds largely on terrestrial invertebrates (Weinkam et al., 2017). We expected that all aerial insectivores would consume more aquatic-emergent insects with proximity to major waterbodies, showing low δ2H, high EPA, and low ARA at lakeshore sites compared to more terrestrial locations. In general, we expected segregation within sites with barn swallows the most terrestrial and tree swallows and purple martins the most riparian. However, we had no a priori expectations regarding interactions between species and proximity to lake.
Materials and methods
Field sampling
In 2020, nestlings of barn swallow (n = 101), tree swallow (n = 162), purple martin (n = 66), and eastern bluebird (n = 33) were sampled from colonies in the Lake Erie region (LAT: 42.58° to 43.10°, LON: −80.40° to-81.51°) of southwestern Ontario, Canada (Figure 1). We categorized sites within 5 km of Lake Erie or Lake Huron as lakeshore sites, and all other sites (i.e., 5–70 km from the lakes) as inland. Nestlings, at day 12–18 depending on species, were sampled for blood for FA profiles and tail feathers for δ2H analysis. Blood was taken by brachial vein puncture and separated into cellular and plasma fractions in the field using a portable centrifuge (Scilogex® Model D1008 EZeeMini, Rocky Hill, United States). In the field, plasma was stored in a liquid nitrogen Dewar (−135 to-190°C). Within 7 h, samples were then transferred to an ultracold (−80°C) and regular (−25°C) freezer for plasma and cellular fractions, respectively. Tail feathers were pulled and placed into a labelled paper envelope and kept dry at room temperature. All animal work was approved by the University of Western Ontario Animal Care Committee (AUP 2017–005) and the Canadian Wildlife Service (10613G).
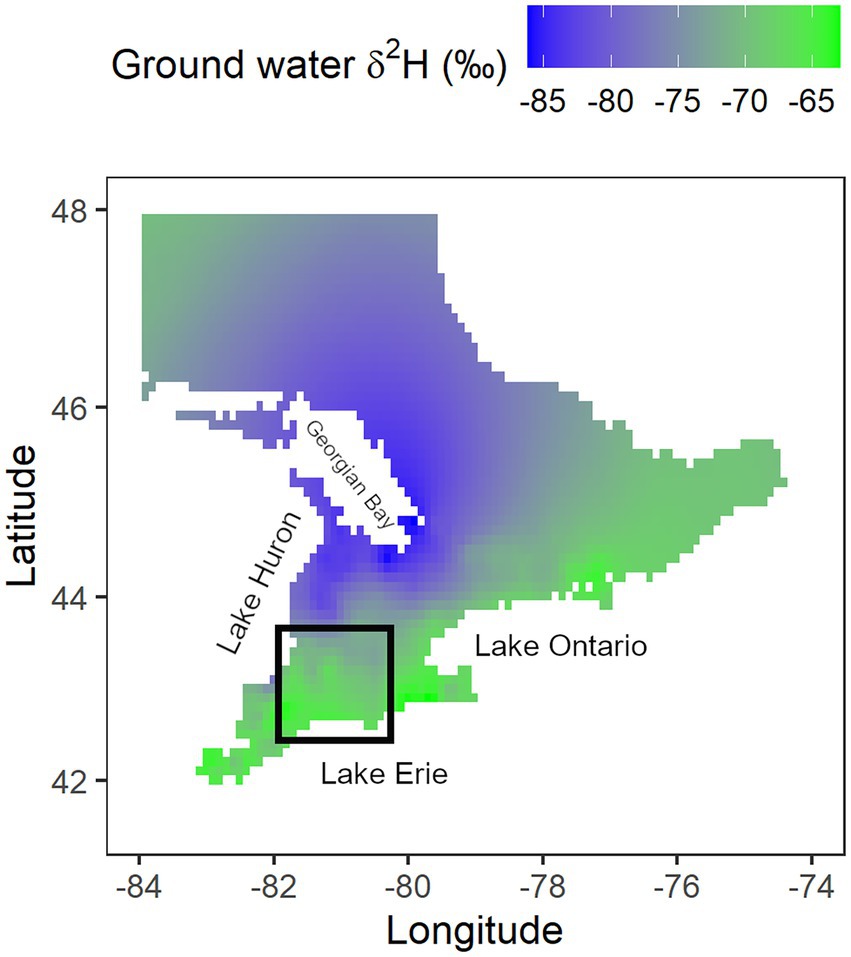
Figure 1. Kriged plot of groundwater δ2H values (‰) from shallow well data (less than 32 m) for southern Ontario. Data were extracted from the waterisotopes.org database (n = 703). Black square represents the study area.
Insects were collected for δ2H from lakeshore and inland sites using a combination of sweep netting, a Malaise trap (Bugdorm, TWN), and a light trap (University of Western Ontario, Department of Physics, London, CAN). In 2020, we installed the Malaise trap twice at inland and lakeshore sites for 5 days each (May 26 and June 2, 11, 16) while alternating between locations throughout the breeding season. In 2021, we installed the Malaise trap among 3 inland and 3 lakeshore sites for 5-day periods (May–July). Sampling was further increased through opportunistic sweep netting over the vegetation surrounding our sites and insect collection from Western’s light trap at the Environmental Sciences Western Field Station. The daily decanting of the Malaise trap targeted smaller flying insects, while sweep netting collected additional insect orders such as orthopterans and odonates and the light trap allowed the collection of nocturnal insects such trichopterans. Insects were transferred at the end of the day and stored in a-80°C freezer. We separated the insects based on orders and further separated aquatic insects of interest (e.g., chironomids from other dipterans).
Stable hydrogen isotope analyses
Tail feathers (2020: n = 266) were soaked in 2:1 chloroform:methanol solution overnight, decanted, rinsed, and dried at ambient temperature in a fume hood. Dragonfly, damselfly (Odonata), and mayfly (Ephemeroptera) wings were separated from their body for soaking, while all other insect groups were soaked with bodies intact (2020: n = 132; 2021: n=510). Grasshopper and cricket (Orthoptera) samples involved the use of legs in addition to wings. In 2021, whole midges (Chironomidae) were dried at 50°C for 24 h and powdered. Feather barbs, insect wing or powdered insect bodies were weighed to 0.33–0.35 mg into silver capsules (Mettler Toledo® XP6 Excellence Plus XP Micro Balance, Greifensee, CHE). Individuals of larger insects such as dragonflies and damselflies were used whereas smaller insects such as flies required multiple individuals for a single composite sample.
Samples were measured by KAH for δ2H at the LSIS-AFAR stable isotope facility at the University of Western Ontario. Compressed silver capsules were loaded onto a UNI-Prep (Eurovector®, Milan, ITA) heated carousel at 60°C coupled with a Eurovector 3,000 elemental analyzer. The samples were combusted pyrolytically at 1350°C on a glassy carbon reactor. The resulting H2 gas was analyzed on a Thermo Delta V Plus (Thermo Scientific®, Bremen, DEU) continuous-flow isotope ratio mass spectrometer via a Conflo device (Thermo Scientific®). Results were expressed in the standard delta notation (δ) in parts per thousand (‰) and normalized using two in-house keratin standards (CBS: −197 ‰; KHS: −54 ‰). We derived our δ2H values from the non-exchangeable H fraction using the comparative equilibrium approach (Wassenaar and Hobson, 2003) and expressed them relative to the Vienna Standard Mean Ocean Water (VSMOW). Within-run standards CBS (n = 5) and KHS (n = 5) measured error to be ±2 ‰.
Fatty acid analyses
Aerial insectivore plasma samples (2020, n = 264) underwent a solvent extraction and derivatization, a protocol modified from Bligh and Dyer (1959) and loaded onto the gas chromatograph (GC)/flame ionization detector (FID) (see Supplementary material for full protocol). Briefly, we mixed the plasma and an internal 17:0 standard in culture tubes with a chloroform:methanol solution containing butylated hydroxytoluene. After centrifuging, the supernatant was mixed with potassium chloride and placed in a 70°C water bath. The organic phase was filtered into vials and dried under N2. Methanolic hydrogen chloride was added and vials were placed in a 90°C oven to derivatize fatty acid methyl esters. Ultrapure water and hexane were added to the vials and the top hexane layer was transferred to GC vials containing dimethoxypropane. Vials were dried under N2 and resuspended in hexane.
We loaded GC vials on a carousel with dichloromethane blank and two standards (Supelco® PUFA and 37 components) and analyzed FA profiles using a GC/FID (Agilent Technologies® 6,890 N G1530N, Santa Clara, United States) equipped with a DB23 column (Agilent Technologies® DB23 122–2,332; see Supplementary material for temperature settings). The retention times of both standards across all runs were averaged for each known FA. We then manually identified each distinct FA peak in a given sample chromatograph by comparing the retention time to our FA library. Relative to the internal 17:0 standard, mass percent was calculated for each FA.
Statistical analyses
Our goal was to examine if aerial insectivores differed in use of aquatic-versus terrestrially-derived insects by comparing feather δ2H values and plasma FA percentages. We also assessed the δ2H values and FA percentages of presumed insect prey (see Supplementary material). All figures and statistical analyses were performed with RStudio Version 1.4.1717 and R 4.0.5 statistical software (RStudio Team, 2018; R Core Team, 2019). Significance level was p < 0.05.
Terrestrial foodwebs near large lakes can receive input from meteoric precipitation and from reprecipitated lakewater. If there was significant input of water driving the terrestrial foodweb from Lake Erie, then this would tend to drive that foodweb to lower δ2H values than expected from expected precipitation isotope data alone (i.e., the long-term database of the International Atomic Energy Agency – Global Network of Isotopes in Precipitation IAEA-GNIP and other sources summarized in waterisotopes.org). If this were the case, it would interfere with our ability to test for the relative abundance of aquatic-derived prey in the diet because diets near lakes might simply be naturally labelled as being lower in 2H regardless of actual diet. Unfortunately, there are relatively few GNIP sites in our study area and so the only way to gain an idea of H inputs to the terrestrial foodweb at the spatial resolution needed was to consider a proxy for amount-weighted precipitation. We followed the example of Bowen et al. (2012) by mapping published δ2H values of shallow groundwater (Waterisotopes Database, 2020) in our study area. Bowen et al. (2012) used this approach to investigate the relative contribution of reprecipitated lakewater from Lake Michigan to downwind terrestrial regions. They used shallow groundwater as a proxy for mean annual precipitation and used the deuterium excess values based on both δ2H and δ18O values in water to trace evaporated lakewater. We were simply interested in a proxy for all precipitation driving terrestrial foodwebs and so only focussed on shallow groundwater δ2H values. We extracted groundwater data from a latitude of 41.8° to 45° and longitude of-83° to-75°. We filtered the data to shallow wells of no more than 32 m depth and removed 11 outliers (with δ2H values < −100‰). Shallow wells represented an integration of annual precipitation. The resultant data included 703 groundwater samples dated from 2007 to 2014 and was then auto kriged and mapped onto a raster grid of southern Ontario. We wished to investigate if a gradient in precipitation δ2H from lakeshores to inland sites was present in our study area and if this was of a magnitude great enough to interfere with our hypotheses regarding the use of aquatic-emergent insects by aerial insectivores.
For δ2H values of nestling tail feathers, we performed a linear mixed effect model (LMM) that included location type (inland versus lakeshore) and species (PUMA, TRES, BARS, and EABL) as fixed effects and site (within the landscape) as a random effect. To see if there was an interaction between species and location (lakeshore versus inland), we used an ANOVA to compare two models including and excluding the interaction. While keeping site as the random effect, we manually performed a post-hoc test by running multiple LMM models. We reorganized our data into eight groups that combined species and location (e.g., inland BARS) and ran multiple LMMs by changing the reference group each time to force all possible group comparisons (see Supplementary material for all combinations). We fitted our LMMs with the nlme package (Pinheiro et al., 2019). We used Akaike information criterion corrected for small sample size from the R package MuMIn (Bartoń, 2019) for model selection. The residuals were verified for normality, equal variance, and leverage.
In combination with δ2H, fatty acid profiles confirm diet differences among species across locations and can be used to infer diet quality. We created a matrix for δ2H values of feathers and relative abundance of 8 notable plasma fatty acids (palmitic acid, stearic acid, oleic acid, LA, ALA, ARA, EPA, and DHA) that were standardized (Routti et al., 2012; Gong et al., 2020) to a mean of zero and a standard deviation of one using the vegan R package (Oksanen et al., 2019). Using Euclidean distances, a redundancy analysis compared δ2H values and FA percentages among species and between locations using the vegan package. We finally ran a permutation test on our model that included a species and location interaction.
For insect δ2H, we performed a LMM where the insect group (aquatic, terrestrial and odonates) and year of collection were fixed effects, and site was the random effect. Odonates were grouped separately to reflect their active feeding on both aquatic and terrestrial insect prey as winged (volant) adults and possibly of distant origin. We changed the reference group to include all possible insect comparisons. We then performed an ANOVA to compare two models including and excluding the interaction between insect group and year.
Results
Groundwater stable hydrogen isotopes
To evaluate if there was a gradient in δ2H in our terrestrial foodweb related to proximity to lake vs. aquatic-emergent prey contributions per se, we analyzed groundwater isotopic data in the region of southern Ontario. Over the larger region, shallow groundwater (n = 703) δ2H values ranged from-47 ‰ in the lower latitudes to-98 ‰ in the high latitudes (Figure 1). However, in our study area (n = 43), the groundwater δ2H values (−67.76 ‰ ± 5.12 ‰) remained relatively constant (Figure 1).
Aerial insectivore stable hydrogen isotopes
Nestling feather δ2H values of eastern bluebirds were lower than those of barn swallows (LMM; df = 244, t = 2.92, p < 0.01) and tree swallows (LMM; df = 244, t = 6.94, p < 0.01), but did not differ from those of purple martins (LMM; df = 244, t = 1.17, p = 0.24; Figure 2). Feather δ2H values of all inland nestlings (controlling for species) were not different from those of nestlings at lakeshore sites (LMM; df = 14, t = −0.90, p = 0.38; Figure 2).
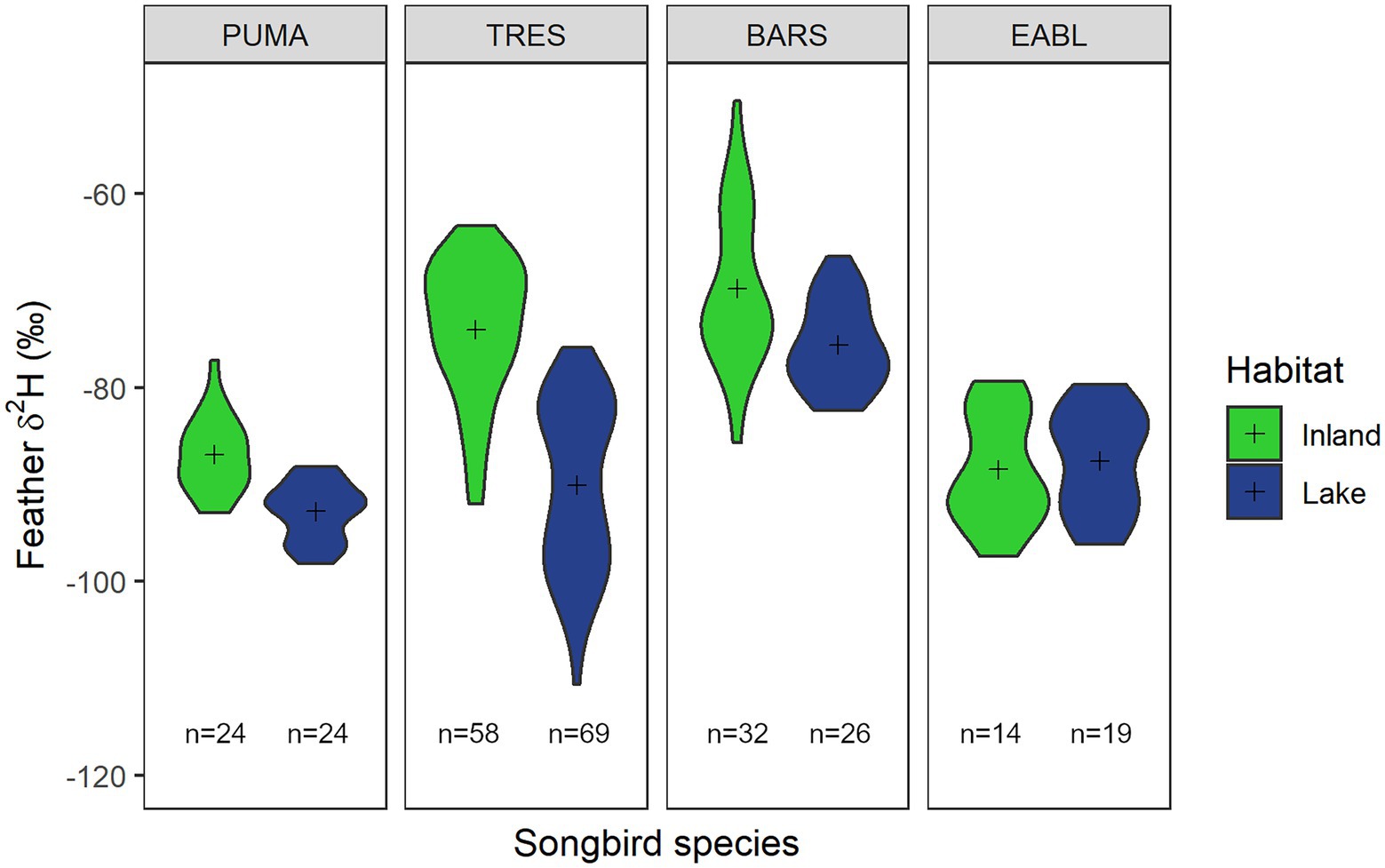
Figure 2. Violin plot showing the distribution of feather δ2H values (‰) from purple martin (PUMA), tree swallow (TRES), barn swallow (BARS), and eastern bluebird (EABL) nestlings from inland (green) or lakeshore (blue) sites. Sample sizes are as shown on the plot and means are represented by (+) symbol.
We found an interaction between location and species when comparing models of feather δ2H values (ANOVA; LRt = 70.18, p < 0.01; model-1: AIC = 1,690, df = 10; model-2: AIC = 1,755, df = 7; Figure 2). That is, how different feather δ2H values are between lakeshore and inland birds depended on the species of interest. We further compared the effect of location within and between each species (see Supplementary material for stable isotope data).
Within each species, only tree swallow nestlings differed in feather δ2H values between inland and lakeshore nesting sites (LMM; df = 243, t = −6.54, p < 0.01; Figure 2). As expected, lakeshore purple martin nestlings had the lowest feather δ2H values and differed from all barn swallows and inland tree swallows (p < 0.01 to 0.04). In contrast, inland barn swallow nestlings had the highest feather δ2H values and differed from every group (p < 0.01 to 0.06), except other barn swallows at lakeshore sites. Inland tree swallows had similar feather δ2H values to lakeshore barn swallows (p = 0.29). Inland purple martins were similar to lakeshore barn swallows (p = 0.32) in their feather δ2H values, but surprisingly still differed from lakeshore tree swallows (p < 0.01). We had expected that eastern bluebird nestlings to have more positive feather δ2H values similar to barn swallows, but instead they were most similar to purple martins (p > 0.05) with more negative feather δ2H values.
Fatty acid and stable isotope ordination
We combined isotope and fatty acid data using a redundancy analysis (Oksanen et al., 2019). The redundancy analysis had four significant axes and so we retained the first two axes (Axis1: F = 70.91, p < 0.01; Axis2: F = 57.58, p < 0.01; Figure 3). The first axis divided individual birds based on inland versus lakeshore and species, while the second axis further divided species. The final model explained 38% of the data variation (adj r2 = 0.38). A permutation test on the redundancy analysis found an interaction between location and species (ANOVA; df = 7, F = 23.49, p < 0.01, Figure 3).
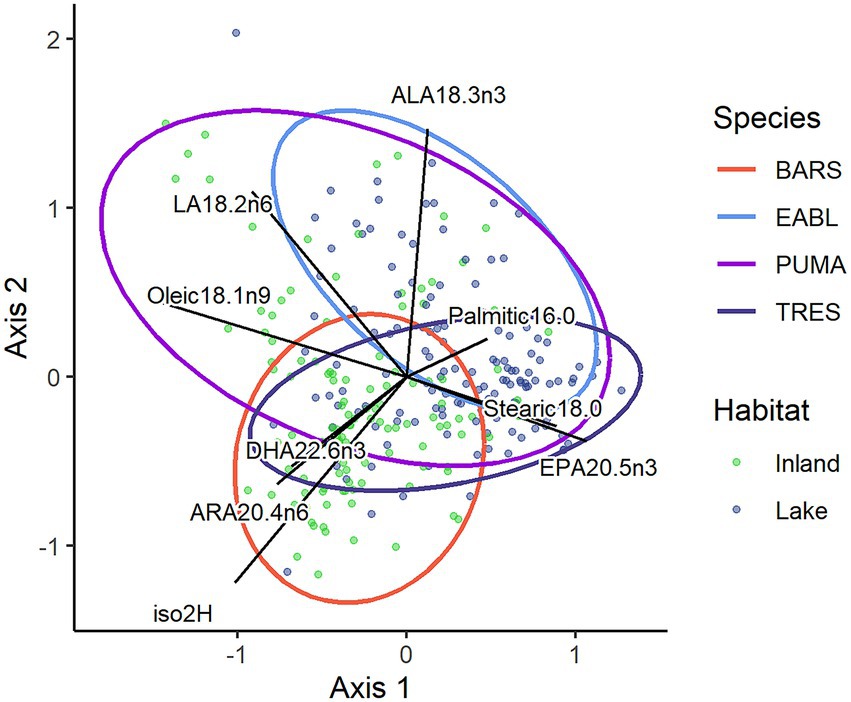
Figure 3. Redundancy analysis of standardized feather δ2H values (‰) and 8 notable plasma fatty acid percentages from purple martin (PUMA), tree swallow (TRES), barn swallow (BARS), and eastern bluebird (EABL) nestlings from inland (green) or lakeshore (blue) sites (n = 261).
Each species of aerial insectivore had some overlap in ordination space (see Supplementary material for fatty acid profiles). As expected, we found a pattern of lower feather δ2H values with higher plasma omega-3 FAs in lakeshore locations and higher feather δ2H values with higher plasma omega-6 FAs in terrestrial locations. Inland birds were associated with more positive feather δ2H values, higher plasma ARA, DHA, oleic acid and LA as seen on the left of the ordination (Figure 3). Lakeshore birds were associated with lower feather δ2H values, higher ALA, palmitic acid, stearic acid, and EPA as shown on the right of the ordination (Figure 3).
Purple martins were the most isotopically variable among the four species and were characterized primarily by more negative feather δ2H values. While this species largely overlapped with eastern bluebirds and tree swallows in the top and middle of the ordination, they were distinct from inland barn swallows found in the lower part of the ordination (Figure 3). Inland purple martins had higher plasma oleic acid (22.75 ± 5.24%) and LA (14.13 ± 3.88%) than other species, while lakeshore purple martins had higher plasma ALA (5.42 ± 2.54%). There was no location difference in eastern bluebirds and they overlapped with lakeshore purple martins. Eastern bluebirds in either location were associated with higher plasma palmitic acid (~18%) and ALA (~ 4%).
Barn swallows and tree swallows overlapped with other species in the center of the ordination, but deviated based on location (Figure 3). However, inland barn swallows and lakeshore tree swallows differed from any other group. Barn swallows were mainly characterized by their more positive feather δ2H values and higher plasma DHA (~7%). Inland barn swallows had also high plasma ARA (12.04 ± 3.66%), though inland purple martins and tree swallows had high ARA as well (~11%). Lakeshore tree swallows on the other hand had higher plasma EPA (6.65 ± 3.18%) than any other species, though following close behind were lakeshore purple martins (4.36 ± 1.66%).
Insect stable hydrogen isotopes
As predicted, values of δ2H of aquatic insects were lower than those of terrestrial insects (LMM; df = 622, t = 5.82, p < 0.01; Figure 4). Odonate δ2H values were between those of aquatic (LMM; df = 622, t = 1.86, p = 0.06; Figure 4) and terrestrial insects (LMM; df = 622, t = −1.44, p = 0.15; Figure 4). However, the difference between these insect groups changed in magnitude depending on the year (ANOVA; AIC1 = 5,946, df1 = 8, AIC2 = 5,958, df2 = 6, LRt = 16.69, p < 0.01; Figure 4).
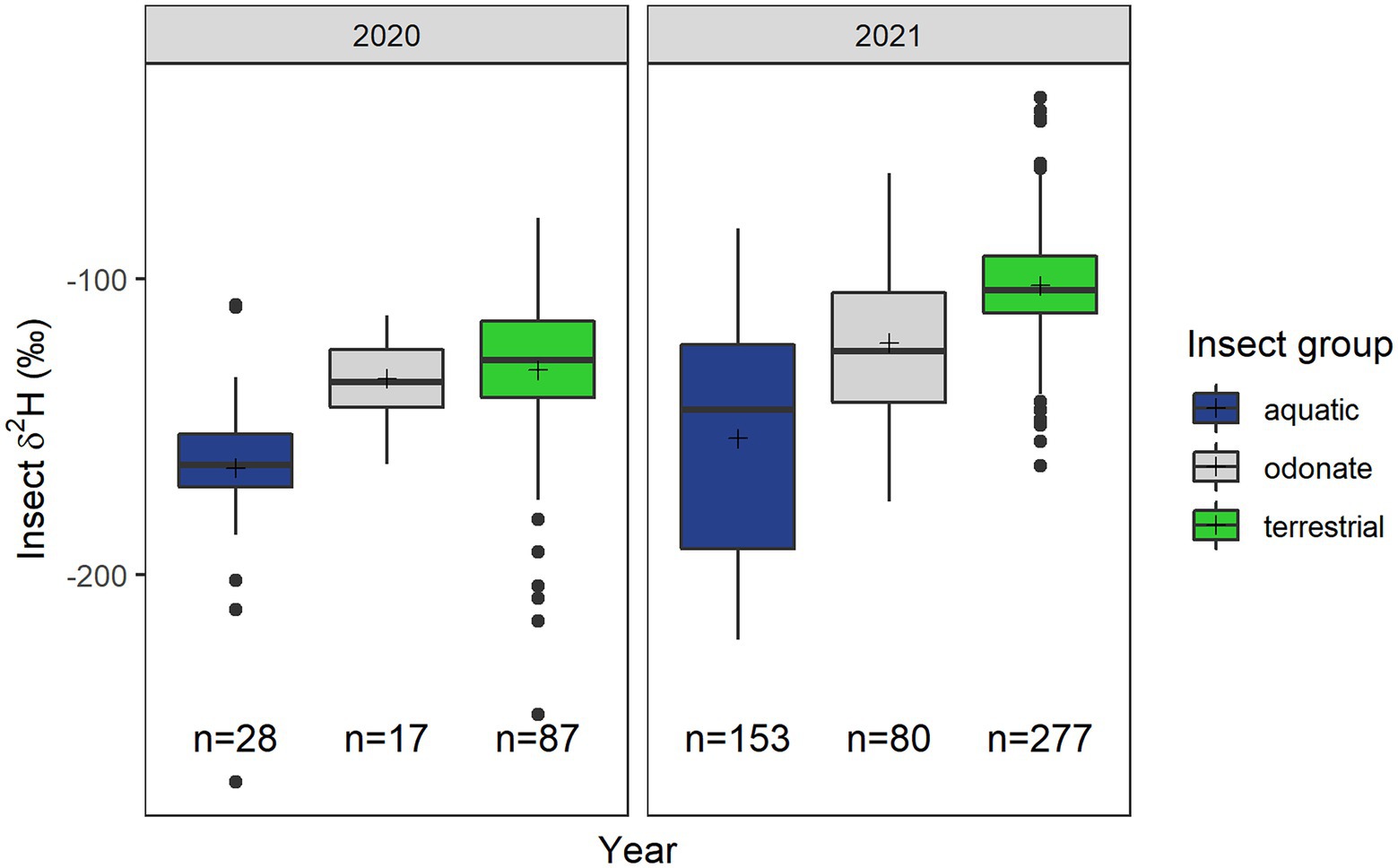
Figure 4. Boxplot showing insect δ2H values (‰) from different insect groups. These groups include smaller aquatic insects, odonates, and terrestrial insects sampled in the study area. Sample sizes are as shown on the plot and means are represented by (+) symbol. Refer to Supplementary material for data of insects included in each group.
Discussion
Shallow groundwater δ2H values remained consistent through our study area, suggesting little evidence for a precipitation δ2H gradient driven by proximity to lake. This provided a firm basis for testing the effects of aquatic versus terrestrial insects in the diets of aerial insectivorous birds using feather δ2H values since we suggest shallow groundwater was a good proxy for long-term precipitation H driving the terrestrial foodweb. We found that aerial insectivore diet composition differed based on nesting location and species. There was an interaction between location and species in both feather δ2H values and in plasma FA. In the following sections, we discuss in detail diet differences among aerial insectivore species and the implications that diet quality and FA conversion can have on aerial insectivore vulnerability to changes in nutritional landscapes.
Groundwater and insect stable isotopes
Using predicted isoscapes developed by Terzer et al. (2013), the amount-weighted mean growing-season (months with average temperature > 0°C) δ2H in precipitation for southern Ontario is expected to range between-48 to-79.9 ‰ following a latitudinal gradient. Shallow groundwater can also be used as a proxy for the mean annual δ2H in precipitation and while not necessarily conforming precisely to the precipitation driving the foodweb leading to birds in our study area, nonetheless it was useful in evaluating high resolution spatial patterning of precipitation δ2H that might have been related to inputs of re-precipitated lakewater (Bowen et al., 2012). For example, using shallow groundwater isotope data, Bowen et al. (2012) showed that up to 18% of the evaporated lakewater was re-precipitated in downwind regions of nearby Lake Michigan. Similarly, we found a northward depletion in groundwater δ2H for our southern Ontario study region (Waterisotopes Database, 2020). However, groundwater δ2H values were remarkably constant, around-68 ± 5 ‰ for our study sites regardless of proximity to Lake Erie (Waterisotopes Database, 2020). We concluded that any difference observed in δ2H of aerial insectivore feathers in our region was thus influenced by the relative contribution of aquatic-emergent insects. In other words, feather δ2H values were associated primarily with diet instead of any underlying baseline precipitation δ2H effects related to proximity to lake.
Feather δ2H values reflect the isotopic value in diet and drinking water during growth (Hobson et al., 1999). For aerial insectivores, feather δ2H will largely reflect the values in their insect prey. As expected, we found δ2H values of aquatic-emergent insects were lower than terrestrial insects. Odonates (i.e., dragonflies and damselflies) were the exception and had δ2H values intermediate between aquatic and terrestrial sources. The wings of odonates represent the isotopic signature of the aquatic larval stage that is dependent on the isotopic composition of the waterbody. Lakes typically have more consistent isotopic compositions than smaller waterbodies such as ponds that may be influenced by evaporation.
Purple martins
Nestling purple martins had lower feather δ2H values than the swallows, suggesting a more aquatic-emergent diet, but there was no difference in feather δ2H values between lakeshore and inland populations. Purple martins forage on a variety of terrestrial and aquatic insects (Helms et al., 2016) and dragonflies are frequently fed to nestlings. Though the wings of odonates reflect aquatic origin, the body could have variable δ2H values due to their broad (terrestrial to aquatic) diets (Chari et al., 2018) and some may move between waterbodies. Thus, the diet of odonates can obscure tracing of the terrestrial versus aquatic signature in martins even though odonates are themselves aquatic-emergent.
Purple martins differed in fatty acid profiles between inland and lakeshore sites. Inland martins had high plasma oleic acid, LA and ARA, while lakeshore martins had high plasma ALA and EPA. In general, aquatic ecosystems are known as a better source of omega-3 EPA and DHA (Rumpold and Schlüter, 2013; Hixson et al., 2015; Twining et al., 2018a, 2019, 2021; Shipley et al., 2022). Inland purple martins likely acquired oleic acid, LA, and ARA from terrestrial insects and odonates that are lower in the omega-3 FAs compared to their lakeshore counterparts. Lakeshore purple martins may benefit from a mix of terrestrial and aquatic insects (including odonates that forage on other aquatic insects) and acquire high amounts of ALA and EPA from aquatic subsidies. Odonates themselves, an undoubtable source of omega-3 FAs from their aquatic life stage, are still dependent on the insect prey available in their habitat (Chari et al., 2018). Thus, we suspect that odonates through trophic enrichment may have enhanced the amount of omega-6 and omega-3 FAs that inland and lakeshore purple martins consumed, respectively. Purple martins select riparian habitats (Lalla, 2022) and forage on diverse insects including aquatic-emergent insects (Helms et al., 2016). Hence, we suspect that martins have a low conversion ability, unable to efficiently synthesize large amounts of omega-3 EPA and DHA similar to the low conversion efficiency of tree swallows (Twining et al., 2018a). Our results suggest that diet quality of purple martins is dependent on nesting habitat. However, the use of odonates that can vary considerably in their use of terrestrial versus aquatic-emergent prey makes the assignment of purple martins to foraging site type more complex.
Tree swallows
Feather δ2H values of nestling tree swallows differed between inland and lakeshore sites according to our expectation of greater use of aquatic-emergent prey near the lakeshore. This result was similar to our previous findings for bank swallow (Génier et al., 2021) in our study area, where lakeshore bank swallows had lower feather δ2H values than inland birds.
Fatty acid profiles of blood plasma further supported the dietary differences we inferred between inland and lakeshore locations. Lakeshore tree swallows had high plasma EPA, while inland tree swallows had high plasma ARA. Aquatic insects are vital sources of omega-3 PUFAs, but chironomids have among the highest amounts of EPA and DHA (Gladyshev et al., 2019; Shipley et al., 2022). Thus, tree swallows foraging on aquatic insects such as chironomids emerging from Lake Erie can benefit from a higher diet quality that is high in omega-3 FAs. Our results align well with the high omega-3 FAs found in tree swallow tissues in Twining et al. (2018a,b) studies in Ithaca, NY, showing the importance of obtaining omega-3 FAs directly from diet. Inland tree swallows, however, could acquire high amounts of ARA from terrestrial insects, but miss an important source of omega-3 FAs.
Barn swallows
More than the other species we examined, barn swallows are typically associated with agro-ecosystems and are the least riparian (McClenaghan et al., 2019b; Bumelis et al., 2021). Our stable isotope and fatty acid results were consistent with this behaviour. Barn swallows had the highest feather δ2H values, representing the most terrestrial diet. Although feather δ2H values of barn swallow nestlings did not differ between inland and lakeshore locations, inland birds had higher plasma ARA, presumably derived from terrestrial insects. Barn swallows forage at lower altitudes than purple martins and tree swallows, and could be targeting different insects than those in higher altitudes (Dreelin et al., 2018). Just as blue tit nestlings in Europe were provisioned with terrestrial insects regardless of aquatic insect availability and distance from lake (Twining et al., 2021), barn swallows in our study area appeared to maintain a terrestrial insect diet regardless of proximity to Lake Erie. Most importantly, barn swallows had high plasma DHA in both inland and lakeshore habitats, more so than the purple martins and tree swallows. We speculate that this high DHA can only be a result of a high omega-3 conversion efficiency, as seen in the blue tits (Twining et al., 2021).
Eastern bluebirds
The diet of eastern bluebirds differed from swallows and martins possibly because they arrive earlier to our study area, breeding in late April through May, and are primarily ground foragers. Based on feather δ2H, we expected nestling bluebirds to have the most terrestrial diet similar to barn swallows. Bluebird diet did not differ between lakeshore and inland habitats and instead had low feather δ2H values similar to purple martins. We speculate that these low values were due to a fossorial diet possibly influenced by early spring snowmelt that was relatively depleted in 2H (Rohwer et al., 2011). Snow was indeed observed at our site during egg laying and chick rearing of bluebirds. In this sense, this species was likely not a good “control” species to compare to later-breeding aerial insectivores.
Plasma of nestling bluebirds was high in palmitic and stearic acid as well as ALA. Many insects have high levels of saturated FA, mostly palmitic, but also stearic acid (Rumpold and Schlüter, 2013; Sosa and Fogliano, 2017). Since both aquatic and terrestrial primary producers can synthesize ALA and LA, insects can also provide sufficient ALA (Hixson et al., 2015; Shipley et al., 2022). Nonetheless, the lack of omega-3 PUFAs suggest a more terrestrial diet. Berries and seeds can also be excellent sources of saturated and unsaturated FAs that could contribute to the fatty acid profiles of bluebirds (Weinkam et al., 2017). Several wild and cultivated northern berries have seed oils high in ALA and LA (Yang et al., 2011).
Implications
In North America, declines in populations of aerial insectivores vary among species (Sauer et al., 2017; North American Bird Conservation Initiative Canada, 2019). We speculate that changes in diet quality may be a contributing factor. In our study area, Bumelis et al. (2021) showed strong evidence of dietary segregation among sympatric swallow species, despite all foraging on flying insects. Dreelin et al. (2018) found that purple martins foraged at the highest altitude, followed by tree swallows and barn swallows, suggesting that these species forage on different insect assemblages found in their respective aerial band. In Japan, the contribution of aquatic subsidies to the annual energy budget of forest birds vastly differed among species (Nakano and Murakami, 2001). As aquatic insects emerge earlier than terrestrial insects, diet is also influenced by the timing of a species breeding season (Nakano and Murakami, 2001; Shipley et al., 2022; Twining et al., 2022). Our previous work has shown that prey quality also varies across the landscape and habitat can be a strong factor in determining diet composition (Génier et al., 2021). Together, time, location and species ecology can determine nutrient availability for provisioning adults and these factors may be related to population dynamics in anthropogenically influenced landscapes.
Our study used nestling feather δ2H measurements and plasma FA profiles jointly to infer diet quality and source to elucidate how certain FAs are being acquired or synthesized. Omega-3 FAs can confer health benefits to nestlings (Twining et al., 2016, 2018b, 2019), but too little omega-3 or too much omega-6 FAs can lead to harmful effects for many bird species (Andersson et al., 2015; Isaksson et al., 2017). Omega-3 and omega-6 PUFAs can be acquired through diet, but also some birds have the ability to synthesize these PUFAs from precursor molecules. A delicate balance between diet quality, conversion efficiency, and hence foraging location, must exist to fulfill the dietary needs of these aerial insectivores.
Barn swallows had a more terrestrial diet regardless of nesting habitat. Lacking in a source of EPA and DHA, we suspect the high plasma DHA observed for this species was a result of conversion of omega-3 precursors. Similar to blue tits (Twining et al., 2021), barn swallows could fulfill their omega-3 FA demand through conversion instead of foraging on aquatic insects. Eastern bluebirds appear to have a mixed diet that had neither high plasma ARA nor EPA or DHA. It is possible that bluebirds do not have a high demand for PUFAs. Bluebirds could also be compensating for the lack in PUFAs in their diet by synthesizing their own supply when needed.
Just as lakeshore bank swallows forage on a more aquatic-emergent insects high in omega-3 FAs (Génier et al., 2021), lakeshore tree swallow and purple martin nestlings were fed more aquatic-emergent insects than their inland counterparts. We suspect, however, that purple martins like tree swallows have a low omega-3 FA conversion efficiency, relying on high-quality aquatic diets to acquire their needed supply of EPA and DHA (Twining et al., 2018a). Purple martins and tree swallows nesting in riparian habitats would benefit from the high EPA and DHA in aquatic-emergent insects, however, in more terrestrial habitats diet quality may decrease. In our study area, Génier et al. (2021) has shown how the distance from Lake Erie decreased the diet quality of bank swallows. Furthermore, Twining et al. (2019) demonstrated that eastern phoebe nestlings fed both aquatic and terrestrial insects depended on the aquatic insects for EPA.
As aquatic-emergent insects decline globally (Gladyshev et al., 2019; Sánchez-Bayo and Wyckhuys, 2019) and locally (Stepanian et al., 2020), a valuable source of omega-3 FAs to terrestrial consumers becomes increasingly limited. Over a 25-year study period, Shipley et al. (2022) also found that insect emergence had advanced and in shorter pulses, outpacing the egg laying phenology of many aerial insectivore species. During energetically demanding life stages such as early development, nestlings can be particularly sensitive to phenological mismatches (Twining et al., 2022). Unable to acquire the necessary omega-3 FAs, aerial insectivores can be vulnerable to such changes in the nutritional landscape and aquatic insect population declines. North America has lost a considerable amount in the aerial insectivore population, even in more recent decades from 1993 to 2015 tree swallows and purple martins have been declining by 0.5 and 0.1% per year, respectively (Sauer et al., 2017). For many aerial insectivores, including tree swallows and purple martins, these declines are more pronounced in the northeast part of their range (Nebel et al., 2010) such as our study area. In addition to previous work on bank swallows (Génier et al., 2021), we identified tree swallows and purple martins to be potentially vulnerable to spatial and temporal loses of aquatic subsidies (Shipley et al., 2022) and highlight the need to support such vitally important aquatic ecosystems.
Clearly, the use of δ2H measurements was crucial to evaluate actual source of insects (aquatic versus terrestrial) to nestlings. This allowed us to evaluate to what degree proximity to Lake Erie had on the nutritional condition of swallows and martins, and permitted much more informed inferences of factors driving fatty acid acquisition and synthesis. Future isotopic studies that investigate other isotopic or elemental tracers of aquatic versus terrestrial foods are thus encouraged to further this area of ecophysiological research. Another particularly useful tool is the use of compound-specific isotope analyses of individual fatty acids (Pilecky et al., 2023). By assessing or tracing 13C of individual fatty acids, we could infer fatty acid conversion efficiencies (Twining et al., 2018a) and dietary sources of each PUFAs with great precision. Ultimately being able to link diet quality to lifetime fitness in these declining aerial insectivores should be a priority of future research. Such research could manipulate breeding location in those species amenable to nest box use (tree swallows and purple martins) and hence proximity to aquatic-derived prey.
Data availability statement
The original contributions presented in the study are included in the article/Supplementary material, further inquiries can be directed to the corresponding author.
Ethics statement
The animal study was reviewed and approved by Animal Care Committee, University of Western Ontario (AUP 2017–005).
Author contributions
All authors conceived the project. CSVG performed the field work, lab work, quantified, and analyzed the data. KAH also analyzed the samples for stable hydrogen isotopes. KAH and CGG supported and funded the research. The manuscript was drafted by CSVG, but was edited and finalized by KAH and CGG. All authors contributed to the article and approved the submitted version.
Funding
This work was supported by an NSERC Discovery Grant (2017-04430) to KAH, by operating grants from Environment and Climate Change Canada to KAH. This work was also supported by an NSERC Discovery Grant (2020-07204) to CGG.
Acknowledgments
We thank the many landowners and Long Point Bird Observatory that kindly allowed us to access bird breeding colonies. We are thankful for the help of our field assistants Chris Posliff and Celina Tang, and colleague Jackson Kusack. We greatly appreciate the assistance of Blanca X. Mora Alvarez with sample preparation for stable isotope analyses. Two reviewers made valuable comments that improved an earlier draft of this manuscript.
Conflict of interest
The authors declare that the research was conducted in the absence of any commercial or financial relationships that could be construed as a potential conflict of interest.
Publisher’s note
All claims expressed in this article are solely those of the authors and do not necessarily represent those of their affiliated organizations, or those of the publisher, the editors and the reviewers. Any product that may be evaluated in this article, or claim that may be made by its manufacturer, is not guaranteed or endorsed by the publisher.
Supplementary material
The Supplementary material for this article can be found online at: https://www.frontiersin.org/articles/10.3389/fevo.2022.1006928/full#supplementary-material
References
Andersson, M. N., Wang, H.-L., Nord, A., Salmon, P., and Isaksson, C. (2015). Composition of physiologically important fatty acids in great tits differs between urban and rural populations on a seasonal basis. Front. Ecol. Evol. 3:93. doi: 10.3389/fevo.2015.00093
Bartoń, K. (2019). MuMIn: multi-model inference. Available at: https://CRAN.R-project.org/package=MuMIn (Accessed May, 2022).
Bligh, E. G., and Dyer, W. J. (1959). A rapid method of total lipid extraction and purification. Can. J. Biochem. Physiol. 37, 911–917. doi: 10.1139/y59-099
Bowen, G. J., Kennedy, C. D., Henne, P. D., and Zhang, T. (2012). Footprint of recycled water subsidies downwind of Lake Michigan. Ecosphere 3:art53. doi: 10.1890/ES12-00062.1
Bumelis, K. H., Cadman, M. D., and Hobson, K. A. (2021). Endogenous biomarkers reveal diet partitioning among three sympatric species of swallows. Ornithology 139:ukab078. doi: 10.1093/ornithology/ukab078
Chari, L. D., Moyo, S., and Richoux, N. B. (2018). Trophic ecology of adult male Odonata. I. Dietary niche metrics by foraging guild, species, body size, and location. Ecol. Entomol. 43, 1–14. doi: 10.1111/een.12458
Collins, C. T. (2015). Food habits and resource partitioning in a guild of Neotropical swifts. Wilson J. Ornithol. 127, 239–248. doi: 10.1676/wils-127-02-239-248.1
Doucett, R. R., Marks, J. C., Blinn, D. W., Caron, M., and Hungate, B. A. (2007). Measuring terrestrial subsidies to aquatic food webs using stable isotopes of hydrogen. Ecology 88, 1587–1592. doi: 10.1890/06-1184
Dreelin, R. A., Shipley, J. R., and Winkler, D. W. (2018). Flight behavior of individual aerial insectivores revealed by novel altitudinal dataloggers. Front. Ecol. Evol. 6:182. doi: 10.3389/fevo.2018.00182
Evans, K. L., Wilson, J. D., and Bradbury, R. B. (2007). Effects of crop type and aerial invertebrate abundance on foraging barn swallows Hirundo rustica. Agric. Ecosyst. Environ. 122, 267–273. doi: 10.1016/j.agee.2007.01.015
Fogel, M. L., and Cifuentes, L. A. (1993). “Isotope fractionation during primary production,” in Organic Geochemistry. eds. M. H. Engel and S. A. Macko (Boston: Springer).
Génier, C. S. V., Guglielmo, C. G., Mitchell, G. W., Falconer, M., and Hobson, K. A. (2021). Nutritional consequences of breeding away from riparian habitats in Bank swallows: new evidence from multiple endogenous markers. Conserv. Phys. 9:coaa140. doi: 10.1093/conphys/coaa140
Gladyshev, M. I., Gladysheva, E. E., and Sushchik, N. N. (2019). Preliminary estimation of the export of omega-3 polyunsaturated fatty acids from aquatic to terrestrial ecosystems in biomes via emergent insects. Ecol. Complex. 38, 140–145. doi: 10.1016/j.ecocom.2019.03.007
Gong, Y., Li, Y., Chen, X., and Yu, W. (2020). Trophic niche and diversity of a pelagic squid (Dosidicus gigas): a comparative study using stable isotope, fatty acid, and feeding apparatuses morphology. Front. Mar. Sci. 7:642. doi: 10.3389/fmars.2020.00642
Hallmann, C. A., Sorg, M., Jongejans, E., Siepel, H., Hofland, N., Schwan, H., et al. (2017). More than 75 percent decline over 27 years in total flying insect biomass in protected areas. PLoS One 12:e0185809. doi: 10.1371/journal.pone.0185809
Helms, J. A., Godfrey, A. P., Ames, T., and Bridge, E. S. (2016). Predator foraging altitudes reveal the structure of aerial insect communities. Sci. Rep. 6:28670. doi: 10.1038/srep28670
Hixson, S. M., Sharma, B., Kainz, M. J., Wacker, A., and Arts, M. T. (2015). Production, distribution, and abundance of long-chain omega-3 polyunsaturated fatty acids: a fundamental dichotomy between freshwater and terrestrial ecosystems. Environ. Rev. 23, 414–424. doi: 10.1139/er-2015-0029
Hobson, K. A., Atwell, L., and Wassenaar, L. I. (1999). Influence of drinking water and diet on the stable-hydrogen isotope ratios of animal tissues. Proc. Natl. Acad. Sci. U. S. A. 96, 8003–8006. doi: 10.1073/pnas.96.14.8003
Imlay, T., Mann, H., and Leonard, M. (2017). No effect of insect abundance on nestling survival or mass for three aerial insectivores. Avian Conserv. Ecol. 12:19. doi: 10.5751/ACE-01092-12021917
Isaksson, C., Andersson, M. N., Nord, A., von Post, M., and Wang, H.-L. (2017). Species-dependent effects of the urban environment on fatty acid composition and oxidative stress in birds. Front. Ecol. Evol. 5:44. doi: 10.3389/fevo.2017.00044
Lalla, K. M. (2022). Purple martin (Progne subis) movement ecology during three stages of the annual cycle. [master’s thesis]. Montréal, QC: McGill University.
McCarty, J. P., and Winkler, D. W. (1999). Foraging ecology and diet selectivity of tree swallows feeding nestlings. Condor 101, 246–254. doi: 10.2307/1369987
McClenaghan, B., Kerr, K. C. R., and Nol, E. (2019a). Does prey availability affect the reproductive performance of barn swallows (Hirundo rustica) breeding in Ontario, Canada? Can. J. Zool. 97, 979–987. doi: 10.1139/cjz-2019-0001
McClenaghan, B., Nol, E., and Kerr, K. C. R. (2019b). DNA metabarcoding reveals the broad and flexible diet of a declining aerial insectivore. Auk 136, 1–11. doi: 10.1093/auk/uky003
Møller, A. P. (2019). Parallel declines in abundance of insects and insectivorous birds in Denmark over 22 years. Ecol. Evol. 9, 6581–6587. doi: 10.1002/ece3.5236
Nakano, S., and Murakami, M. (2001). Reciprocal subsidies: dynamic interdependence between terrestrial and aquatic food webs. Proc. Natl. Acad. Sci. U. S. A. 98, 166–170. doi: 10.1073/pnas.98.1.166
Nebel, S., Mills, A., McCracken, J., and Taylor, P. (2010). Declines of aerial insectivores in North America follow a geographic gradient. Avian. Conserv. Ecol. 5:1. doi: 10.5751/ACE-00391-050201
North American Bird Conservation Initiative Canada (2019). The State of Canada’s Birds, 2019. Environment and Climate Change Canada, Ottawa: North American Bird Conservation Initiative Canada.
Oksanen, J., Blanchet, F. G., Friendly, M., Kindt, R., Legendre, P., McGlinn, D., et al. (2019). Vegan: Community ecology package. Available at: https://CRAN.R-project.org/package=vegan (Accessed May, 2022).
Orłowski, G., and Karg, J. (2013). Diet breadth and overlap in three sympatric aerial insectivorous birds at the same location. Bird Study 60, 475–483. doi: 10.1080/00063657.2013.839622
Pilecky, M., Wassenaar, L. I., Kainz, M. J., Anparasan, L., Ramirez, I., McNeil, J. M., et al. (2023). Isotopic (δ2H, δ13C) tracing of the origin and fate of individual fatty acids fueling migrating animals: A case study of the monarch butterfly (Dannaus plexippus). Front. Ecol. Evol. 8:572140. doi: 10.3389/fevo.2020.572140
Pinheiro, J., Bates, D., DebRoy, S., Sarkar, D., and R Core Team. (2019). Nlme: Linear and nonlinear mixed effects models. Available at: https://CRAN.R-project.org/package=nlme (Accessed May, 2022).
R Core Team (2019). R: A Language and environment for Statistical Computing. Vienna, AT: R Foundation for Statistical Computing.
RStudio Team (2018). RStudio: integrated development for R. RStudio Inc., Boston, USA. Available at: http://www.rstudio.com/
Rohwer, S., Hobson, K. A., and Yang, S. (2011). Stable isotopes (δD) reveal east—west differences in scheduling of molt and migration in Northern rough-winged swallows (Stelgidopteryx serripennis). Auk 128, 522–530. doi: 10.1525/auk.2011.10273
Rosenberg, K. V., Dokter, A. M., Blancher, P. J., Sauer, J. R., Smith, A. C., Smith, P. A., et al. (2019). Decline of the north American avifauna. Science 366, 120–124. doi: 10.1126/science.aaw1313
Routti, H., Letcher, R. J., Born, E. W., Branigan, M., Dietz, R., Evans, T. J., et al. (2012). Influence of carbon and lipid sources on variation of mercury and other trace elements in polar bears (Ursus maritimus) environ. Toxicol. Chem. 31, 2739–2747. doi: 10.1002/etc.2005
Roy, R., Singh, S., and Pujari, S. (2008). Dietary role of omega-3 polyunsaturated fatty acid (PUFA): a study with growing chicks, Gallus domesticus. Int. J. Poult. Sci. 7, 360–367. doi: 10.3923/ijps.2008.360.367
Rumpold, B. A., and Schlüter, O. K. (2013). Nutritional composition and safety aspects of edible insects. Mol. Nutr. Food Res. 57, 802–823. doi: 10.1002/mnfr.201200735
Sánchez-Bayo, F., and Wyckhuys, K. A. G. (2019). Worldwide decline of the entomofauna: a review of its drivers. Biol. Conserv. 232, 8–27. doi: 10.1016/j.biocon.2019.01.020
Sauer, J. R., Pardieck, K. L., Ziolkowski, D. J., Smith, A. C., Hudson, M.-A. R., Rodriguez, V., et al. (2017). The first 50 years of the North American breeding bird survey. Condor 119, 576–593. doi: 10.1650/CONDOR-17-83.1
Shipley, J. R., Twining, C. W., Mathieu-Resuge, M., Parmar, T. P., Kainz, M., Martin-Creuzburg, D., et al. (2022). Climate change shifts the timing of nutritional flux from aquatic insects. Curr. Biol. 32, 1342.e3–1349.e3. doi: 10.1016/j.cub.2022.01.057
Simopoulos, A. P. (2011). Evolutionary aspects of diet: the omega-6/omega-3 ratio and the brain. Mol. Neurobiol. 44, 203–215. doi: 10.1007/s12035-010-8162-0
Smith, A. C., Hudson, M.-A. R., Downes, C. M., and Francis, C. M. (2015). Change points in the population trends of aerial-insectivorous birds in North America: synchronized in time across species and regions. PLoS One 10:e0130768. doi: 10.1371/journal.pone.0130768
Sosa, D. A. T., and Fogliano, V. (2017). “Potential of insect-derived ingredients for food applications,” in Insect Physiology and Ecology (HR InTech: Rijekay).
Spiller, K. J., and Dettmers, R. (2019). Evidence for multiple drivers of aerial insectivore declines in North America. Condor 121, 1–13. doi: 10.1093/condor/duz010
Stepanian, P. M., Entrekin, S. A., Wainwright, C. E., Mirkovic, D., Tank, J. L., and Kelly, J. F. (2020). Declines in an abundant aquatic insect, the burrowing mayfly, across major North American waterways. Proc. Natl. Acad. Sci. U. S. A. 117, 2987–2992. doi: 10.1073/pnas.1913598117
Terzer, S., Wassenaar, L. I., Araguás-Araguás, L. J., and Aggarwal, P. K. (2013). Global isoscapes for δ18O and δ2H in precipitation: improved prediction using regionalized climatic regression models. Hydrol. Earth Syst. Sci. 17, 4713–4728. doi: 10.5194/hess-17-4713-2013
Twining, C. W., Brenna, J. T., Lawrence, P., Shipley, J. R., Tollefson, T. N., and Winkler, D. W. (2016). Omega-3 long-chain polyunsaturated fatty acids support aerial insectivore performance more than food quantity. Proc. Natl. Acad. Sci. U. S. A. 113, 10920–10925. doi: 10.1073/pnas.1603998113
Twining, C. W., Brenna, J. T., Lawrence, P., Winkler, D. W., Flecker, A. S., and Hairston, N. G. (2019). Aquatic and terrestrial resources are not nutritionally reciprocal for consumers. Funct. Ecol. 33, 2042–2052. doi: 10.1111/1365-2435.13401
Twining, C. W., Lawrence, P., Winkler, D. W., Flecker, A. S., and Brenna, J. T. (2018a). Conversion efficiency of α-linolenic acid to omega-3 highly unsaturated fatty acids in aerial insectivore chicks. J. Exp. Biol. 221:jeb165373. doi: 10.1242/jeb.165373
Twining, C. W., Parmar, T. P., Mathieu-Resuge, M., Kainz, M. J., Shipley, J. R., and Martin-Creuzburg, D. (2021). Use of fatty acids from aquatic prey varies with foraging strategy. Front. Ecol. Evol. 9:735350. doi: 10.3389/fevo.2021.735350
Twining, C. W., Shipley, J. R., and Matthews, B. (2022). Climate change creates nutritional phenological mismatches. Trends Ecol. Evol. 37, 736–739. doi: 10.1016/j.tree.2022.06.009
Twining, C. W., Shipley, J. R., and Winkler, D. W. (2018b). Aquatic insects rich in omega-3 fatty acids drive breeding success in a widespread bird. Ecol. Lett. 21, 1812–1820. doi: 10.1111/ele.13156
Vander Zanden, H. B., Soto, D. X., Bowen, G. J., and Hobson, K. A. (2016). Expanding the isotopic toolbox: applications of hydrogen and oxygen stable isotope ratios to food web studies. Front. Ecol. Evol. 4:20. doi: 10.3389/fevo.2016.00020
Voigt, C. C., Lehmann, D., and Greif, S. (2015). Stable isotope ratios of hydrogen separate mammals of aquatic and terrestrial food webs. Methods Ecol. Evol. 6, 1332–1340. doi: 10.1111/2041-210X.12414
Walters, D. M., Fritz, K. M., and Otter, R. R. (2008). The dark side of subsidies: adult stream insects export organic contaminants to riparian predators. Ecol. Appl. 18, 1835–1841. doi: 10.1890/08-0354.1
Wassenaar, L. I., and Hobson, K. A. (2003). Comparative equilibration and online technique for determination of non-exchangeable hydrogen of keratins for use in animal migration studies. Isot. Environ. Health Stud. 39, 211–217. doi: 10.1080/1025601031000096781
Waterisotopes Database (2020). Query: Country=CA, type=groundwater. Available at: http://waterisotopesDB.org (Accessed December, 2020).
Waugh, D. R. (1979). The diet of sand martins during the breeding season. Bird Study 26, 123–128. doi: 10.1080/00063657909476629
Weinkam, T. J., Janos, G. A., and Brown, D. R. (2017). Habitat use and foraging behavior of eastern bluebirds (Sialia sialis) in relation to winter weather. Northeast. Nat. 24, B1–B18. doi: 10.1656/045.024.s704
Keywords: aerial insectivore, diet quality, stable hydrogen isotopes, fatty acids, nutritional landscapes
Citation: Génier CSV, Guglielmo CG and Hobson KA (2022) Combining bulk stable H isotope (δ2H) measurements with fatty acid profiles to examine differential use of aquatic vs. terrestrial prey by three sympatric species of aerial insectivorous birds. Front. Ecol. Evol. 10:1006928. doi: 10.3389/fevo.2022.1006928
Edited by:
Perry S. Barboza, Texas A&M University, United StatesReviewed by:
Craig Stricker, United States Department of the Interior, United StatesJeremy Ryan Shipley, Max Planck Institute of Ornithology, Germany
Copyright © 2022 Génier, Guglielmo and Hobson. This is an open-access article distributed under the terms of the Creative Commons Attribution License (CC BY). The use, distribution or reproduction in other forums is permitted, provided the original author(s) and the copyright owner(s) are credited and that the original publication in this journal is cited, in accordance with accepted academic practice. No use, distribution or reproduction is permitted which does not comply with these terms.
*Correspondence: Corrine S. V. Génier, cgenier@uwo.ca